Abstract
The brief review of a number of recent publications regarding pre-earthquake anomalies of ionosphere electron density is presented. It is shown that the effects of earthquakes with their epicentres located at different latitudes are observable in the low-latitude ionosphere. The paper analyses the retrospective plasma frequency data obtained by a topside ionosonde onboard the satellite Alouette 1 at the peak height of the ionospheric F region prior to several strong earthquakes. These are the earthquakes of magnitudes M ≥6 that have occurred in the American longitudinal zone with their epicentres located within the ±20° range of magnetic inclination. Bottomside ionograms of nearby ionospheric stations Huancayo, La Paz, Talara and Bogota are also used. Only the data for quiet geomagnetic conditions are considered. The main peculiarities of the F peak electron density modification at low latitudes are summarized. Their possible explanation within the framework of already existing approaches is proposed.
1. Introduction
The ionospheric disturbances that occurred around the time of the Alaskan earthquake of 28 March 1964 were reported as early as in 1965 (Davies and Baker 1965). Though the problem of lithosphere/ionosphere coupling has been of interest to researchers for many years, both its phenomenology and interaction mechanism still continue to attract attention.
Ionospheric effects following earthquakes have been more or less carefully observed and investigated as travelling ionospheric disturbances (TIDs) (Jung et al. 2006). From the practical point of view, the problem is to find out whether an ionosphere modification during the preparation stage of disastrous earthquakes really exists. A great number of publications (Liu et al. 2001, Molchanov et al. 2002, Singh et al. 2004, Hobara and Parrot 2005, Zakharenkova et al. 2006, Pulinets et al. 2007, Oyama et al. 2008, Zhao et al. 2008, Akhoondzadeh et al. 2010, Lin 2010, Singh 2010) are devoted to this problem. At the same time, there are still well-grounded doubts about the existence of seismo-ionospheric relationships, because even the morphological features of ionosphere modification before earthquakes are not studied enough (Kouris et al. 2001, Rishbeth 2006). Our knowledge in this area is mainly based on the retrospective case and random statistical analysis of ionospheric data before earthquakes, irrespective of specific regional features of tectonic structures and the ionosphere. In our opinion, this approach leads to mutual disagreements and makes it impossible to find an appropriate explanation of observations. However, it is reasonable to suppose that the ionospheric response to the impact from below (including a pending earthquake as one of the reasons) depends on the location of the observational point on the Earth's surface. This assumption is made because there is an analogy with ionospheric disturbances, which happen due to solar and geomagnetic activities.
The progress in earthquake forecasting using radio physical methods, from our point of view, can be achieved by creating separate regional ionosphere modification models for different geographic positions of seismic zones on the globe.
This means that it is necessary to estimate the following quantities e.g. temporal and spatial changes of different ionospheric parameters; height boundaries of the observed disturbance; area covered by the disturbance (how far from the epicentre it could be observed); time lag between the disturbance appearance and earthquake shock; preferable time of the disturbance existence; longitudinal variability; possible relation to solar and geomagnetic activity.
Herein, it is proposed to distinguish between the following cases of earthquake epicentre position:
near the magnetic equator with magnetic inclination I ≤±5°) (equatorial earthquakes); between the equatorial anomaly northern and southern crests (±5° <I <±15–20°) (low-latitude earthquakes); at the equatorial anomaly northern or southern crests (I = ±15–20°); and even further, at higher latitudes (I ≥±30–50°).
Keeping in mind that physical processes at the low-latitude ionosphere differ remarkably from those at middle and high latitudes, some examples of low-latitude ionosphere responses related to preparation processes of strong earthquakes, with their epicentres located at different latitudes with emphasis on equatorial and low-latitude earthquakes, have been analysed. The analysis is limited by the effects observed in the F region peak plasma frequency (foF2, NmF2), and by the total electron content (TEC) during the quiet geomagnetic conditions.
2. Recent results on F region modification prior to strong earthquakes
A review of recent results regarding the manifestation of earthquake preparation processes prior to earthquakes is presented herein. The epicentres may be located anywhere, even at middle latitudes outside the northern and southern crests of the equatorial anomaly, because in the general case, the ionosphere modifications caused by the latter could be observed at the low latitudes as well.
Molchanov et al. (2002) tried to find a correlation between changes in ion density with seismic activity, using the data of ion density distribution obtained onboard the IK-24 satellite in 1989–1990 over the height range of 500–2500 km. Based on the changes in the ion density, they observed some indication of this correlation, but only for earthquakes located in the near equatorial area.
Hobara and Parrot (2005) examined the worldwide vertical sounding data for the distances between ionosondes and the epicentre ranging from 290 to 11 402 km. Their goal was to retrieve any significant changes in the plasma frequency at the peak of the ionospheric F layer foF2 records around the time of the strong Hachinohe earthquake (ϕ = 40.8° N, λ = 143.2° E, I = +54°, M = 8.3) on 16 May 1968. Here and further, ϕ and λ denote geographic latitude and longitude of the earthquake epicentre position, respectively; I – magnetic inclination of this point; M–earthquake magnitude. The earthquake epicentre was in the middle-latitude region. Four days before the event, a substantial decrease in the electron density in the afternoon was indicated by ionosondes at distances as long as 1500 km from the epicentre. Measurements were made during the quiet geomagnetic activity.
Singh et al. (2004), Singh and Singh (2007) examined the effects on the ionosphere of six major earthquakes that occurred in different parts of India (ϕ = 18.1–30.8° N, λ = 70.0–86.6° E, I = +25–45°, M = 6.3–7.6) at night (18:00–06:00 LT) analysing foF2 records from Ahmedabad (ϕ = 23.0° N, λ = 72.4° E, I = +32°). The distances between the station and epicentres were over the range of 250–2500 km. The results show that the foF2 level during pre- and post-midnight hours decreased compared with its monthly median both on the 0–4 and 1–15 days before the occurrence of the earthquakes. In the pre-midnight sector, the reduction is between 24 and 30%, and in the post-midnight sector, it is between 18 and 26%. The effects of magnetic storms (Kp and Dst variations) on the data are identified clearly, but they do not vitiate the effects of earthquakes.
Singh et al. (2009) monitored ionospheric total electron content (TEC) at Agra (ϕ = 27.2° N, λ = 78.0° E, I = +42°), India. They analysed 15 months of data for the period between 1 September 2006 and 30 November 2007. As magnetic conditions remained quiet throughout the period of data, except for some sporadic cases, the majority of the TEC anomalies were linked to earthquakes. It was observed that in 23 out of 43 cases of moderate and high magnitude earthquakes (M ≥5.0), both depletions and enhancements occurred, and in 17 cases either enhancement or depletion occurred. No data were available for the three remaining cases. On average, the anomalies occurred 0–9 days before the earthquakes.
Liu et al. (2001) detected the anomalous events before the Chi-Chi earthquake (ϕ = 31.0° N, λ = 103.4° E, I = +42°, M = 7.6) on 20 September 1999. foF2 observed by Chung-Li ionosonde (ϕ = 25.0° N, λ = 121.2° E, I = +35°), and the corresponding TEC area were analysed. It was found that the variations in foF2 and the overhead TEC recorded at Chung-Li have similar trends and decrease significantly at 1, 3, and 4 days prior to the earthquake. A comparison between the disturbed and reference (previous 15-day median) days confirms that the TEC decreases significantly around the epicentre in the afternoons of these days. The results of this study show that the equatorial anomaly crest moves equatorwards.
The island of Taiwan (I = +32°) is located in the region, where a large number of earthquakes occur frequently over a small territory. Therefore it is important to mention the publication of Liu et al. (2006), in which the relationship between foF2 for 184 earthquakes with M ≥5.0 in the Taiwan area was investigated statistically. The abnormal decrease by more than 25% during the afternoon period, 12:00–18:00 LT, significantly occurred within 5 days before the earthquakes. The odds of pre-earthquake anomalies were observed to increase with the magnitude, and decrease with the distance between an epicentre and an ionospheric station.
Recent studies have shown the anomalous changes in the land, atmosphere and ionosphere parameters associated with the disastrous Wenchuan earthquake (ϕ = 31.0° N, λ = 103.4° E, I = +42°, M = 8.0) of 12 May 2008 (Singh et al. 2010).
Zhao et al. (2008) studied the foF2 records prior to this earthquake. An unusually large foF2 enhancement during the afternoon/sunset sector was registered by ionosondes over Wuhan (ϕ = 30.5° N, λ = 114.4° E, I = +42°) and Xiamen (ϕ = 24.4° N, λ = 123.9° E, I = +35°), which are close to the earthquake epicentre. The average increase with respect to the median value for these two stations was about double that of a geomagnetic quiet day, which occurred three days prior to the earthquake. The increase was significantly less as compared to Yamagawa (ϕ = 31.2° N, λ = 130.6° E, I = +45°) and Okinawa (ϕ = 26.7° N, λ = 128.2° E, I = +38°). Combining the Wenchuan data from the network of GPS receivers, the variations in the TEC reveal the region, where enhancement persisted to be within longitudes 90–130° E.
Liu et al. (2009) found that the GPS TEC above the same earthquake epicentre at its forthcoming stage anomalously decreases in the afternoon period of days 6–4, and the late evening period of day 3 before the earthquake. However, there is an enhanced TEC in the afternoon of day 3 before the earthquake. The spatial dimensions of the disturbed area were about 1650 and 2850 km from the epicentre in the latitudinal and longitudinal directions, respectively. The satellite results show that when the GPS TEC reduces anomalously, the ionospheric F2 peak electron density significantly decreases by approximately 40%, and the height descends by about 50–80 km.
Lin (2010) examined the same Wenchuan earthquake using the principal component analysis and image processing of the global ionospheric map for the region. The data was processed for 4, 8 and 9 May 2008. The principal component analysis supports the findings of Liu et al. (2009) that the TEC anomalies for the Wenchuan earthquake existed on 8 May 2008. In addition, the analysis was also able to discriminate the TEC enhancement for the afternoon–sunset period, similar to the result of Zhao et al. (2008).
Jhuang et al. (2010) also used the TEC of the global ionosphere maps to study the seismo-ionospheric anomalies during the Wenchuan earthquake. It was found that the remarkable reductions appear locally around the epicentre and their conjugate points during the daytime of 29 April and 6–10 May 2008. A global study and a strict criterion are applied to detect anomalies. The results show that the anomalies on 29 April and 6 and 7 May 2008, which are respectively days 13, 6 and 5 before the earthquake, are possibly related to the earthquake.
Xia et al. (2011) investigated the TEC anomalies that occurred in the Qinghai-Tibet region before three large earthquakes: aforesaid Wenchuan on 12 May 2008 (ϕ = 31.0° N, λ = 103.4° E, I = +42°, M = 8.0); Yutian on 20 March 2008 (ϕ = 35.4° N, λ = 81.4° E, I = +55°, M = 7.2); and Kunlun on 14 November 2001 (ϕ = 36.2° N, λ = 90.9° E, I = +55°, M = 8.1). They identified two TEC enhancements in the afternoon local time 9 days and 2–3 days before each earthquake, between which the TEC decrement occurred 3–6 days prior to the earthquakes. These anomalies occurred in the area of about 30° in latitude and the maximum localized equatorward from the epicentres. These TEC anomalies can be found in all three earthquakes regardless of the geomagnetic conditions.
Akhoondzadeh et al. (2010) using the plasma analyser and the Langmuir probe experiments onboard the DEMETER satellite and GPS measurements, have statistically analysed the variations of the electron and ion densities to search for disturbances in the vicinity of four large earthquakes prior to events. The Samoa Islands earthquake took place on 29 September 2009 with M = 8.1; Sichuan (Wenchuan), the China earthquake took place on 12 May 2008 with M = 8.0; the earthquake in L'Aquila, Italy happened on 6 April 2009 with M = 6.3; and the Hormozgan, Iran earthquake was on 28 February 2006 with M = 6.0. The statistical analysis shows that the anomalous deviations prior to earthquakes have different signs in different cases, and their amplitudes depend on the magnitude of the earthquake. It has also been found that the electron density measured by the Langmuir probe experiment at night detects anomalous variations significantly before the earthquakes. The positive and negative anomalies in both the DEMETER and TEC data obtained 1 to 5 days before all the studied earthquakes during the quiet geomagnetic conditions indicate that the anomalous behaviours are of seismo-ionospheric origin.
Liu et al. (2010) reported the anomalous decreases in the TEC appearing 5 days prior to an outstanding earthquake (ϕ = 3.30° N, λ = 95.5° E, I = –17°, M = 9.3), the largest one in the last five decades, which occurred in Sumatra–Andaman, Indonesia on 26 December 2004. Sequences of global ionosphere maps of the TEC derived from the worldwide ground-based GPS receivers were used. It was found that the temporal precursor of the GPS TEC around the epicentre was significantly reduced during the afternoon period 5 days before the earthquake.
The spatial precursors prominently, persistently, and simultaneously appeared around the epicentre and its conjugate areas.
Zakharenkova et al. (2006) analysed the TEC map for the same Sumatra–Andaman earthquake. They reported the modification of the equatorial anomaly that had occurred a few days prior to this earthquake. The daytime amplification of the Appleton anomaly was registered a few days prior to the main event. The spatial size increased, and the maximum enhancement took place 2 days before the earthquake. The ratio of crests and trough electron concentration was more pronounced than for the other days of the period of tests.
In addition, according to the ionosphere TEC data, it was found that on the days prior to the earthquake, the meridian section of the TEC spatial structure took the shape of a double-crest curve with a trough near the epicentre. The effect was most pronounced in the evening and night hours (local time) on 25 December 2005 though usually at this time the restored normal latitudinal distribution with a maximum near the magnetic equator is observed.
Above, we have summarized conclusions of recent publications regarding pre-earthquake anomalies caused by pending strong earthquakes. Earthquakes with epicentres located at middle latitudes (outside the equatorial anomaly region) were studied by many authors. They report anomalies connected with mid-latitude earthquakes that were often observed just over the epicentre, or at some distance around the epicentre position. Their observation is that the effect decreases as the distance between an epicentre and an observation station increases. Low-latitude ionosphere parameters did not change anomalously before these earthquakes e.g. before the Hachinohe earthquake. At the same time, there is evidence that some of these mid-latitude earthquakes, e.g. Wenchuan, somehow manifest themselves in the low-latitude ionosphere electron density measurements as well.
Singh et al. (2004), Singh and Singh (2007), Zakharenkova et al. (2006), Liu et al. (2010) reported that low-latitude earthquakes cause ionosphere modification presumably inside the equatorial anomaly region.
The features of anomalies reported by different authors have something in common, but at the same time reveal different behaviour even before the same events. Sometimes it is impossible to reconstruct the whole picture of the earthquake-prone disturbance on the basis of the presented data because of their diversity.
However, there is a high probability that the low-latitude seismo-ionospheric effects prior to earthquakes really exist, and they are actually caused by the processes predetermining the origin of the earthquakes with their epicentres located either at low or even at middle latitudes. Additional efforts to clearly define and interpret these phenomena are still needed.
3. Main features of F region modification before strong earthquakes with epicentres at equatorial and low latitudes
Strong (M ≥6.0) earthquakes with their epicentres located at low latitudes are of great interest from the point of view of the ionosphere F region response.
First of all, the specific structure of the Earth's crust in the low-latitude region, predominantly the meridional alongation of tectonic fractures, may result in patches with the enhanced possibility of earthquakes of great magnitudes. Moreover, within a few degrees of the magnetic equator the ionosphere has features that are not found elsewhere.
The latitudinal variations are rather peculiar showing an equatorial foF2 trough or Appleton anomaly existing normally (in quiet conditions) from mid-morning to late evening. The reason for the Appleton anomaly is the so-called fountain-effect. This effect is in the plasma upward vertical drift over the magnetic equator caused by specific equatorial ionosphere geometry, i.e. crossed electric and magnetic fields in the horizontal plane. With the exception of the region near the magnetic equator, the plasma motion produced by electric fields tends to be horizontal. This property causes the main distinction of the low-latitude ionosphere and its response to events at higher and, possibly, lower levels.
In general, all of the earthquake precursory phenomena are related to deformations near the fault prior to the earthquake. Therefore usually all precursory anomalies tend to occur close to the eventual epicentre of the earthquake (Cicerone et al. 2009). This statement also applies to ionospheric effects in the majority of mid-latitude earthquakes, as those considered in §2.
Keeping in mind the close connection between the F region peak density over the magnetic equator and in-between northern and southern crests of the Appleton anomaly, we assume that earthquake precursory anomalies over the F region heights could be observed not only over the epicentre, but may also depend on the electrodynamic processes of the low-latitude ionosphere.
Therefore, the ionosphere F region responses are assumed to be different for equatorial (the magnetic inclination of the epicentre position I = ±5°); low-latitude (5° <I <±15–20°) and crest (I = ±20–35°) earthquakes, depending on the mutual position of the magnetic equator, the observation place and the epicentre of the pending earthquake.
3.1. F region response to equatorial earthquakes
Depueva and Rotanova (2001) discussed the Appleton anomaly modification prior to earthquake that occurred on 15 August 1963 at 17:25 UT (ϕ = 13.8° S, λ = 69.3° W, I = +1.1°, M = 7.75). The data obtained by Alouette 1 topside ionosonde foF2 (Alouette 1 1963) from 31 July to 14 August 1963 within the longitudinal sector λ = (55–95°) W (with a step of 5°) and interval of inclinations I = ±40° (with a step of 1–2°) for the time period between 16:30 and 18:30 LT were analysed. The presented foF2 spatial distribution (map) prior to this earthquake resembles a trough around the epicentre position (Depueva and Rotanova 2001). On average, the decreased foF2 value was as low as 5.5 MHz, falling during some passes down to 4.5 MHz against the ambient background level of 8–10 MHz.
The second equatorial earthquake occurred on 20 March 1979, at 07:53 UT (ϕ = 7.6° N, λ = 126.6° E, I = –0.86°, M = 5.3). The satellite ISS-b data (incomplete, available only for the southern hemisphere) obtained from 4 March to 19 March 1979 for the time interval 18:00–21:00 LT were used by Depueva and Rotanova (2000) for constructing a NmF2 spatial distribution (map) prior to the aforesaid earthquake, and this distribution looked like a smaller sized trough in comparison with the previous case.
Depueva and Rotanova (2000, 2001) have presented some reasons in favour of the supposition that the observed foF2 decrease was connected to forthcoming earthquakes. Unfortunately, to the best of our knowledge, the ionosphere modification prior to more earthquakes with epicentres located so close to the magnetic equator has not been highlighted in the up-to-date published works.
The peculiarities of the peak electron density prior to the two abovementioned equatorial earthquakes may be presented according to Depueva and Rotanova (2000, 2001) as follows.
1. | The funnel-shaped decrease in the electron density in the vicinity of the epicentre (and in the vicinity of the magnetic equator at the same time) by 15–30% of the background level was observed, foF2 being slightly changed at both Appleton anomaly crests. Five days before the main shock, the ionosphere modification, i.e. reduction of the peak electron density, can be observed at the peak height of the F region. | ||||
2. | The size of the modified area in the N–S direction was (±25–30°) in both cases and corresponded to the distance between the northern and southern crests of the usual undisturbed Appleton anomaly, the E–W dimension of the modified area being 30° (≈3000 km) and 5° (≈500 km), respectively. Oraevsky et al. (1994), Ruzhin and Depueva (1996) estimated the horizontal dimension of the area disturbed by the forthcoming earthquake at the ionospheric heights. This area is approximately equal to the preparation zone dimension on the ground level. The latter according to the threshold deformation theory was estimated by Dobrovolsky et al. (1979). It depends on the earthquake magnitude M, and for the considered cases the preparation zone radii were equal to 2315 km and 200 km, respectively. These numbers roughly correspond to the observed latitudinal dimensions of the modified area. Thus, the N–S dimension of the modified area depends mainly on the Appleton anomaly formation mechanism, while the E–W dimension is rather associated with the earthquake magnitude. | ||||
3. | Maps were constructed for the afternoon and evening hours (from 16:30 till 21:00 LT), while for the available morning orbits (from 04:30 until 09:00) such a type of disturbance was not observed. It appears that morning hours were ‘a forbidden’ time for pre-earthquake anomaly observation. |
3.2. F region response to low-latitude earthquakes
A few more earthquakes with their epicentres situated relatively far from the magnetic equator as compared to the previous case, are analysed herein.
The first of them occurred on 13 April 1963, at 02:21 UT (ϕ = 6.3° S, λ = 76.7° W, I = +11°, M = 6.88). The Alouette 1 topside foF2 map based on data obtained from 2 April to 13 April 1963 for 21:01–22:26 LT (Alouette 1, 1963) is presented in . The calculations based on the IRI model (Bilitza 2001) were also made. For the actually observed helio- and geophysical conditions, these foF2 values were the following: foF2 = 7.3–8.3 MHz for trough, 8.2–8.8 MHz for crest regions, and the average measured values were 4.5 and 8.5 MHz, respectively. Thus, the degree of the equatorial anomaly development (foF2 crest /foF2 trough) was in reality around 2.0 in comparison with the expected model value of not more than 1.2.
Figure 1. F region peak plasma frequency foF2 spatial distribution a few days prior to low-latitude earthquake M = 6.88 occurred on 13 April 1963 at 02:21 UT with the epicentre position ϕ = 6.3° S, λ = 76.7° W (I = +11°) shown by asterisk. Available in colour online.
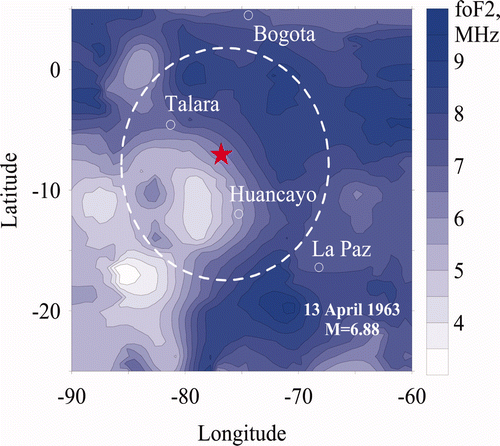
The second earthquake occurred on 29 December 1962 at 10:41 UT (ϕ = 20.2° S, λ = 70.3° W, I = –11°, M = 6.75). The Alouette 1 topside foF2 map for this event, based on data obtained from 21 December to 28 December 1962 for 22:21–23:47 LT is presented in . With the IRI model calculations, we obtain foF2 = 6.2–7.5 MHz for trough, 6.2–7.8 MHz for crest, and the average observed values are 3.5 and 6.5 MHz, respectively. Again, (foF2 crest /foF2 trough) was close to 1.8 instead of the expected 1.2.
Figure 2. F region peak plasma frequency foF2 spatial distribution a few days prior to low-latitude earthquake M = 6.75 occurred on 29 December 1962 at 10:41 UT with the epicentre position ϕ = 20.2° S, λ = 70.3° W (I = –11°) shown by an asterisk. Available in colour online.
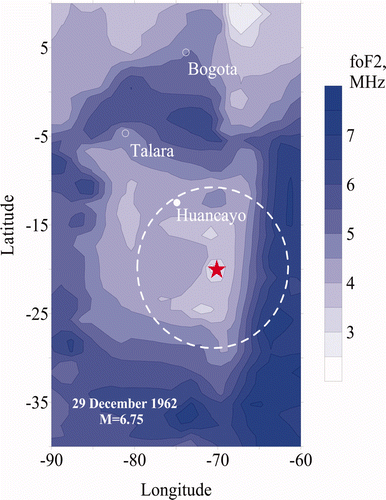
Dst and AE indices show the relatively quiet geomagnetic conditions a few days prior to both earthquakes under consideration. An exception is very slight magnetic disturbance (Dst≤–30 nT) during 26 December 1962. These data are shown in and (low panels).
Figure 3. Temporal foF2 and foF2 27med variations for 13 April 1963 earthquake at ionospheric stations Huancayo, La Paz, Talara and Bogota (top panels). Dst and AE indices (low panel). Available in colour online.
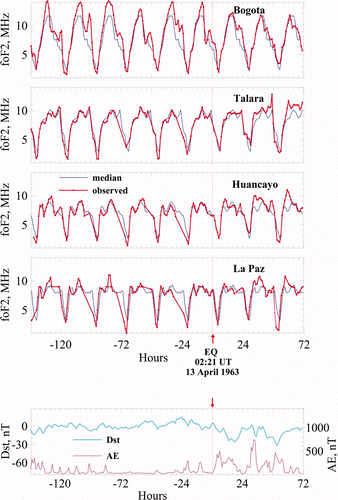
Figure 4. Temporal foF2 and foF2 27med variations for 29 December 1962 earthquake at ionospheric stations Huancayo, Talara and Bogota (top panels). Dst and AE indices (low panel). Available in colour online.
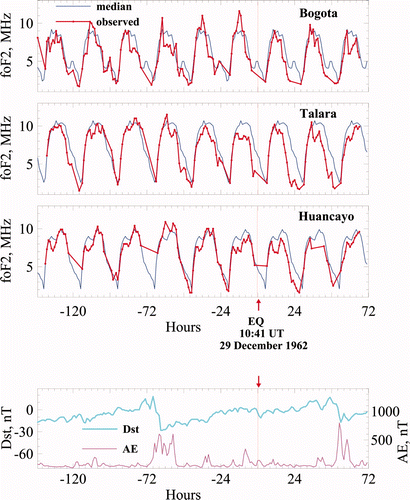
The foF2 depletions inside the modified areas in comparison with the surrounding values, as shown in and , and the IRI model calculations are straightforward for both cases. In the first event, the modified area was located just over the magnetic equator, and the epicentre was on the outskirts of it. In the second case, it covers the entire region including the epicentre and the magnetic equator. The E–W dimensions are approximately 1300 km and 1700 km, and they roughly correspond to the earthquake preparation radii. These radii can be calculated according to Dobrovolsky et al. (1979), and they equal 947 km and 858 km, respectively. The equatorial anomaly crests are approximately at the same position as in the usual quiet conditions.
The temporal foF2 records made in Huancayo, the station inside the preparation zones of both earthquakes under consideration, 2–5 days prior to the earthquakes reveal the negative deviation (k = 10–30%) of the observed foF2 values in comparison with the 27-day running median. The temporal foF2 records made in Bogota, the station outside the preparation zones of both considered earthquakes, also 2–5 days prior to the earthquakes, reveal the positive deviation (k = 15–20%) of the observed foF2 values in comparison with the 27-day running median. Ionosondes Talara and La Paz, prior to the former i.e. 13 April 1963, earthquake, reveal the afternoon/late evening negative Q-disturbances. We have no La Paz foF2 data for the 29 December 1962 earthquake. The foF2 data measured in Talara show the same behaviour as the foF2 data measured in Huancayo. It is necessary to point out the existence of the visible () negative foF2 deviation (k = 10–30%) in comparison with the 27-day running median (except 3 days before the earthquake, when slight magnetic disturbance was observed) existing not only during afternoon/evening hours, but also earlier, at noontime. This is a specific feature of the 29 December 1962 earthquake, and it will be discussed below.
Herein, the foF2 depletions seen in , and the negative foF2 deviations observed by the ground-based ionosondes inside the modified areas are assumed to be connected with the pending earthquakes.
This hypothesis is based on the following observations. First, in all the considered cases, the modified areas with the foF2 values reduced compared to the ambient values in the neighbouring vicinity have a limited and much smaller dimension in the E–W direction than a regular equatorial anomaly. The zonal length of the latter is about 9000 km, while the region with the reduced electron concentration does not exceed 2000–3000 km. Second, the degree of the equatorial anomaly development (foF2 crest /foF2 trough) inside the modified area exceeds the expected results of calculations. Finally, negative Q-disturbances exist inside the modified area, but there are no such disturbances outside the modified area.
At the same time it is necessary to stress that there were some low-latitude earthquakes e.g. of 10 November 1962 (ϕ = 1.3° S, λ = 80.4° W, I = +15°, M = 6.2, preparation zone radius of 472 km), which did not show any difference in comparison with regular behaviour, independently of the local time of observations.
Recently, some papers on seismo-ionospheric effects for earthquakes with the epicentres located at approximately the same low latitudes (I = +8 and +15°) based on bottomside ionosondes and the GPS TEC measurements have been published (Oyama et al. 2008, Zakharenkova et al. 2008).
Oyama et al. (2008) examined the ionospheric data from a chain of four ionospheric stations located in the longitude zone of 120–130° E (Manila (I = +20°), Taipei (I = +32°), Okinawa and Yamagawa (I = +42°)) during three earthquakes: 22 November 1981 (ϕ = 18.8° N, λ = 120.8° E, I = +22°, M = 6.7); 11 January 1982 (ϕ = 13.8° N, λ = 124.4° E, I = +8°, M = 7.4); 24 January 1981 (ϕ = 14.1° N, λ = 124.3° E, I = +8°, M = 6.6). The data foF2, and the virtual height, h′F, are used along with the electron density Ne observed onboard the HINOTORI satellite. The common features for all the three cases are the following. First, a region with high plasma density stays late in the afternoon; and second, an equatorial ionospheric anomaly cleft moves toward the lower latitude. The results of Oyama et al. (2008) appear to be similar to ours, taking into account the following. The epicentres were located near the magnetic equator, however, observations were made away from the magnetic equator, near the northern crest regions (Manila etc.), so they were able to register how the enhancement degree of the equatorial anomaly develops. The results of Zakharenkova et al. (2008) also agree very well with ours. They used the global TEC maps for the analysis of the ionospheric effects of the Peru earthquake, which occurred on 26 September 2005 (ϕ = 5.7° S, λ = 76.4° W, I = +15°, M = 7.5). The geomagnetic situation was fairly quiet on all days prior to the earthquake. The analysis in Zakharenkova et al. (2008) has shown that the modification of the equatorial anomaly had occurred a few days prior to the earthquake. During the evening and night hours LT, a specific transformation of the TEC distribution took place. This modification had the shape of a double-crest structure with a trough near the epicentre, though usually at this time the restored normal latitudinal distribution with a maximum near the magnetic equator is observed. Additional measurements by the CHAMP satellite have also confirmed the presence of such a double-crested structure.
The coincidence of our results with the published is promising, but to construct an adequate model more data points are needed.
3.3. F region response to crest earthquakes
For earthquakes with epicentres located near the crests of the Appleton anomaly, the F2 peak electron density reveals a different behaviour: a few days prior to the events either depletion, or enhancement of the foF2 and the TEC were reported (e.g. Devi et al. 2001, Singh et al. 2009). In contrast to the equatorial and low-latitude earthquakes, the crest earthquakes were discussed in many publications. It looks like that the case of the crest earthquakes is more complex, and may include action of not a single, but several physical processes. We leave it beyond the scope of this article.
4. Discussion
An attempt was made to find an explanation for the aforesaid results in the framework of already existing physical theories. It is known that equatorial and low-latitude ionosphere peculiarities are affected by the specific processes in plasma exposed to the crossed electric E and magnetic B fields. Our main hypothesis is that a few days preceding the main shock, an electric field of lithospheric origin appears on the Earth's surface over the entire area inside the earthquake preparation zone. The size of this zone can be evaluated as in Dobrovolsky et al. (1979). Moreover, the usual regular electric field of the equatorial electrojet could be modulated by an anomalous additional horizontal electric field of seismic origin, which penetrates into the ionosphere dynamo region height from the Earth's surface. This way the Appleton anomaly profile could be changed to some extent, as was observed at the onset of several earthquakes. This idea was proposed by Depueva and Ruzhin (1995).
A successful numerical model, similar to the aforesaid approach, was made recently for middle and low latitudes by Namgaladze et al. (2009). This computational model shows that the NmF2 and TEC disturbances observed before earthquakes could be due to the vertical drift of the F region ionospheric plasma under the influence of the zonal electric field of seismic origin. In the case of the TEC enhancements in the middle latitudes and when deepening the equatorial anomaly trough, this field is directed towards the east. This field induces an electromagnetic drift of the plasma across the geomagnetic field with the velocity directed straight upwards over the magnetic equator, and upwards and polewards in the middle latitudes. The upward plasma drift in the middle latitudes provokes the increase of electron density in the F region of the ionosphere due to the decrease of the dominating ions O+ loss rate in the ion–molecular reactions. In the low latitudes, it provokes the decrease of the NmF2 over the magnetic equator due to the fountain effect.
Namgaladze et al. (2009), in their modelling, used zonal electric fields of 3–10 mV m−1, which are too high in comparison with the available observations for this value. Ampferer et al. (2010) estimated that the 10 kV m−1 vertical electric field above the ground would be enough to produce a horizontal electric field of a few mV m−1 in the ionosphere dynamo region. Typically, the vertical component of the electric field observed near the ground is as much as 100 V m−1. Vershinin et al. (1999) report electric fields up to 1 kV m−1 observed before strong earthquakes. The model of Ampferer et al. (2010) is a linear one, and the horizontal component of the electric field in the ionosphere before an earthquake could be estimated as 10 times less, i.e. a few hundred μV m−1. The magnitude of this electric field of lithospheric origin penetrating into the ionosphere is too weak to have a noticeable effect upon the middle latitude ionosphere. However, the situation is quite different for the low-latitude ionosphere. A few hundred μV m−1 value is comparable with the usually existing horizontal electric field of an equatorial electrojet, which approximately equals 1 mV m−1, and the latter could be modulated by an additional electric field of lithospheric origin. As a consequence, the Appleton anomaly profile could be modified to some extent.
However, two questions still remain. First, it is not clear why the F2 peak electron density modification prior to earthquakes is observed mainly in the afternoon/evening hours, though typically an equatorial anomaly exists in the morning hours as well.
Let us suppose that an additional horizontal E–W electric field of seismic origin appears at the dynamo region height. The effect of a relatively small additional electric field of lithospheric origin could hardly be observed against a background of typically high upward vertical drift, or downward drift. Then, the most noticeable influence of such an additional electric field could be observed, when the ordinary (regular) vertical plasma drift over the magnetic equator is not too large, and descends to zero and even slightly below zero.
The velocity of the vertical drift (Vd) depends on the local time, season, and solar activity (Fejer 1981). For instance, during the phase of low solar activity, the lowest Vd values exist from 14:00 until 21:00 LT in summer; from 15:00 until 19:00 LT at equinox, and from 12:00 until 22:00 LT in winter.
It is worth mentioning that the observational results in question were obtained during different seasons of the low solar activity years (1962, 1963, 2005). Therefore in summer and during the equinox, we really come across a ‘forbidden’ time in the early morning hours, and the effect is visible in the afternoon/evening hours, as was stated in §3.1 and §3.2 for the earthquakes on 15 August and 13 April 1963. For the winter earthquake on 29 December 1962 the effect is even more evident around noon in comparison with the evening time (see ). Therefore the ‘forbidden’ time arises from different diurnal behaviour of the usual vertical electrodynamic drift.
During high solar activity the diurnal Vd behaviour is substantially different, and shows the pre-sunset enhancement. Therefore, we assume that during the phase of high solar activity, the ‘forbidden’ time for earthquake precursor appearance has to be quite different compared to that observed during the low solar activity years.
The second question is why the F2 peak electron density modification was not observed at all prior to some cases of low-latitude earthquakes (see §3).
schematically shows the mutual positions of the earthquake epicentre, the preparation area inside the preparation zone, and the magnetic equator. As was assumed above, the degree of the trough deepening and the E–W dimension of the modified area prior to the earthquake depend on the additional vertical upward drift.
Figure 5. Scheme of the mutual positions of the earthquake epicentre, preparation area and magnetic equator. The estimated modified area limits in the E–W direction are marked by straight horizontal lines. (a) The epicentre position is just under the equatorial electrojet (15 August 1963); (b) the epicentre position is not under the equatorial electrojet, the earthquake preparation area crosses the magnetic equator (13 April 1963 and 29 December 1962); (c) the epicentre position is not under the equatorial electrojet, the earthquake preparation area does not cross the magnetic equator (10 November 1962). Available in colour online.
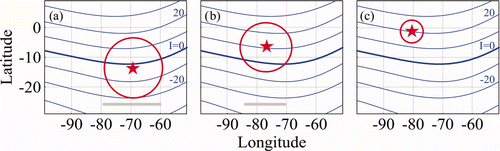
In the case presented in , the phenomenon may always take place when the epicentre position is very close to the magnetic equator (earthquakes of 15 August 1963 and 29 March 1979). The estimated modified area limits in the E–W direction correspond to the diameter of the preparation zone, and are marked by the straight horizontal lines.
If the epicentre position is not directly under the equatorial electrojet (magnetic equator), two different cases arise: either earthquake preparation area intersects the magnetic equator (as in the earthquakes of 13 April 1963 and of 29 December 1962), or does not (the earthquake of 10 November 1962).
illustrates the situation, when the modulation of the equatorial electrojet by an additional electric field of seismic origin is still possible. This case is similar to the one in , but the E–W dimension of the modified area is smaller than the preparation zone diameter. It is reasonable to assume that the E–W dimension of the modified area depends on the preparation zone radius (or earthquake magnitude), and on the distance between the epicentre and the magnetic equator.
In , the situation corresponds to the abovementioned earthquake of 10 November 1962, when the preparation zone radius is less than the distance from the epicentre to the magnetic equator. The modulation is evidently impossible, and no seismic disturbance was really observed.
Consider a disseminated situation, when the epicentre is near the anomaly crest region, or even further, outside the equatorial anomaly action. Only the earthquakes of M ≥7.8 (preparation zone radius approximately 2000 km and greater) are able to cause an additional vertical drift due to the proposed mechanism, as seen from . Possibly, the Wenchuan and Sumatra–Andaman earthquakes could be examples supporting this idea. This problem has to be studied yet. Anyway, the further search for the other mechanism(s) is needed to explain the numerous results obtained for earthquakes, the epicentres of which are located at crest regions and behind them. This applies to such mechanisms as e.g. acoustic-gravity waves from a seismic source that could influence the equatorial anomaly electrodynamics (Molchanov et al. 2002).
5. Conclusion
Herein, a few examples of relatively small, not more than 15–30%, foF2 depletions a few hundred to a few thousand kilometres in size, occurring several days prior to strong equatorial and low-latitude earthquakes, are presented. These disturbances are observable by the topside (spatial structures or maps) and the ground-based (temporal variations) ionosondes, and by the GPS TEC measurements. It turns out that for the low solar activity, the depletions could be preferably observed during the afternoon/evening local time in summer and equinox, while in winter they could last a few hours longer. The existence or absence of the pre-earthquake modification, as well as the size and location of the depleted foF2 area, depends on such factors as the earthquake magnitude (preparation zone radius), and the mutual position of the epicentre and the magnetic equator. That is why the point of the foF2 minimum does not always coincide with the epicentre. This is quite different from the mid-latitude behaviour.
The appearance and behaviour of depletions can be interpreted in the framework of already existing ideas of low-latitude ionosphere physics. It appears that the additional zonal electric field of lithospheric origin at the dynamo-region height could influence the usual equatorial anomaly and to some extent change its shape.
Unfortunately, these results could not be used presently in practice for forecasting purposes. The observed earthquake-prone disturbances are too small. Therefore they could not be easily distinguished from the other types of ionosphere variability e.g. atmospheric fronts, typhoons, hurricanes, TIDs, possibly caused by the impact from below. Of course, the task is much more difficult during the helio- and geomagnetic disturbances, which remain the main sources of the ionosphere F region variability (Forbes et al. 2000). Nevertheless, the problem of manifestation of the earthquake preparation processes at low-latitude ionosphere still remains of great interest from the physics point of view.
References
- Akhoondzadeh , M. , Parrot , M. and Saradjian , M. R. 2010 . Electron and ion density variations before strong earthquakes (M>6.0) using DEMETER and GPS data . Natural Hazards and Earth System Sciences , 10 : 7 – 18 .
- Alouette , 1 . 1963 . “ Ionospheric data ” . Ottawa , Canada : Alosyn .
- Ampferer , M. , Denisenko , V. V. , Hausleitner , W. , Krauss , S. , Stangl , G. , Boudjada , M. Y. and Biernat , H. K. 2010 . Decrease of the electric field penetration into the ionosphere due to low conductivity at the near ground atmospheric layer . Annales Geophysicae , 28 : 779 – 787 .
- Bilitza , D. 2001 . International Reference Ionosphere 2000 . Radio Science , 36 : 261 – 275 .
- Cicerone , R. D. , Ebel , J. E. and Britton , J. 2009 . A systematic compilation of earthquake precursors . Tectonophysics , 476 : 371 – 396 .
- Davies , K. and Baker , D. M. 1965 . Ionospheric effects observed around the time of the Alaskan earthquake of March 28, 1964 . Journal of Geophysical Research , 70 : 2251 – 2253 .
- Depueva , A.Kh. and Ruzhin , Yu.Ya. 1995 . Seismoionospheric fountain-effect as analogue of active space experiment . Advances in Space Research , 15 : 151 – 154 .
- Depueva , A.Kh. and Rotanova , N. M. 2000 . Modification of the low-latitude and equatorial ionosphere before earthquakes . Geomagnetism and Aeronomy , 40 : 728 – 732 .
- Depueva , A. and Rotanova , N. 2001 . Low-latitude ionospheric disturbances associated with earthquakes . Annali di Geofisica , 44 : 221 – 228 .
- Depueva , A.Kh. , Mikhailov , A. V. and Depuev , V.Kh. 2005 . Quiet time F2-layer disturbances at geomagnetic equator . International Journal of Geomagnetism and Aeronomy , 5 GI3001, DOI: 10.1029/2004GI000071
- Depueva , A.Kh. , Mikhailov , A. V. , Devi , M. and Barbara , A. K. 2007 . Spatial and time variations in critical frequencies of the ionospheric F region above the zone of equatorial earthquake preparation . Geomagnetism and Aeronomy , 47 : 129 – 133 .
- Devi , M. , Barman , M. K. , Barbara , A. K. and Depueva , A. 2001 . Ionospheric electron content near anomaly crest as a precursor of earthquake . Indian Journal of Radio and Space Physics , 30 : 209 – 213 .
- Dobrovolsky , I. P. , Zubkov , S. I. and Miachkin , V. I. 1979 . Estimation of the size of earthquake preparation zones . Pure and Applied Geophysics , 117 : 1025 – 1044 .
- Fejer , B. G. 1981 . The equatorial ionospheric electric fields. A review . Joumal of Atmospheric and Terrestrial Physics , 43 : 377 – 386 .
- Forbes , J. M. , Palo , S. E. and Zhang , X. 2000 . Variability of the ionosphere . Journal of Atmospheric and Solar-Terrestrial Physics , 62 : 685 – 693 .
- Hobara , Y. and Parrot , M. 2005 . Ionospheric perturbations linked to a very powerful seismic event . Journal of Atmospheric and Solar-Terrestrial Physics , 67 : 677 – 685 .
- Jhuang , H.-K. , Ho , Y.-Y. , Kakinami , Y. , Liu , J.-Y. , Oyama , K.-I. , Parrot , M. , Hattori , K. , Nishihashi , M. and Zhang , D. 2010 . Seismo-ionospheric anomalies of the GPS-TEC appear before the 12 May 2008 magnitude 8.0 Wenchuan Earthquake . International Journal of Remote Sensing , 31 : 3579 – 3587 .
- Jung , T. K. , Liu , J. Y. , Tsai , H. F. , Huang , B. S. and Lin , C. H. 2006 . Ionospheric disturbances triggered by the Mw 7.6 earthquake off the coast of El Salvador on 13 January, 2001 . Terrestrial, Atmospheric and Oceanic Sciences , 17 : 345 – 351 .
- Kouris , S. S. , Spalla , P. and Zolesi , B. 2001 . Could ionospheric variations be precursors of a seismic event? A short discussion . Annali di Geofisica , 44 : 395 – 402 .
- Lin , J-W. 2010 . Two-dimensional ionospheric total electron content map (TEC) seismo-ionospheric anomalies through image processing using principal component analysis . Advances in Space Research , 45 : 1301 – 1310 .
- Liu , J. Y. , Chen , Y. I. , Chuo , Y. J. and Tsai , H. F. 2001 . Variations of ionospheric total electron content during the Chi-Chi earthquake . Geophysical Research Letters , 28 : 1383 – 1386 .
- Liu , J. Y. , Chen , Y. I. , Chuo , Y. J. and Chen , C. S. 2006 . A statistical investigation of preearthquake ionospheric anomaly . Journal of Geophysical Research , 111 A05304, DOI: 10.1029/2005JA011333
- Liu , J. Y. , Chen , Y. I. , Chen , C. H. , Liu , C. Y. , Chen , C. Y. , Nishihashi , M. , Li , J. Z. , Xia , Y. Q. , Oyama , K. I. , Hattori , K. and Lin , C. H. 2009 . Seismoionospheric GPS total electron content anomalies observed before the 12 May 2008 Mw = 7.9 Wenchuan earthquake . Journal of Geophysical Research , 114 A04320, DOI: 10.1029/2008JA013698
- Liu , J. Y. , Chen , Y. I. , Chen , C. H. and Hattori , K. 2010 . Temporal and spatial precursors in the ionospheric global positioning system (GPS) total electron content observed before the 26 December 2004 M9.3 Sumatra–Andaman Earthquake . Journal of Geophysical Research , 115 A09312, DOI: 10.1029/2010JA015313
- Molchanov , O. A. , Hayakawa , M. , Afonin , V. V. , Akentieva , O. A. and Mareev , E. A. 2002 . “ Possible influence of seismicity by gravity waves on ionospheric equatorial anomaly from data of IK-24 satellite 1. Search for idea of seismo-ionosphere coupling ” . In Seismo-electromagnetics: Lithosphere-atmosphere-ionosphere coupling , Edited by: Hayakawa , M. and Molchanov , O. A. 275 – 285 . Tokyo : Terra Scientific .
- Namgaladze , A. A. , Klimenko , M. V. , Klimenko , V. V. and Zakharenkova , I. E. 2009 . Physical mechanism and mathematical modeling of earthquake ionospheric precursors registered in Total Electron Content . Geomagnetism and Aeronomy , 49 : 252 – 262 .
- Oraevsky , V. N. , Ruzhin , Yu.Ya and Depueva , A.Kh. 1994 . Seismoionospheric precursors and atmospheric electricity . Turkish Journal of Physics , 18 : 1229 – 1234 .
- Oyama , K.-I. , Kakinami , Y. , Liu , J.-Y. , Kamogawa , M. and Kodama , T. 2008 . Reduction of electron temperature in low latitude ionosphere at 600 km before and after large earthquakes . Journal of Geophysical Research , 113 A11317, DOI: 10.1029/2008JA013367
- Pulinets , S. A. , Biagi , P. , Tramutoli , V. , Legen'ka , A. D. and Depuev , V. 2007 . Irpinia earthquake 23 November 1980 – lesson from nature reviled by joint data analysis . Annals of Geophysics , 50 : 61 – 70 .
- Rishbeth , H. 2006 . F-region links with the lower atmosphere? . Journal of Atmospheric and Solar-Terrestrial Physics , 68 : 469 – 478 .
- Ruzhin , Yu.Ya. and Depueva , A.Kh. 1996 . Seismoprecursors in space as plasma and wave anomalies . Journal of Atmospheric Electricity , 16 : 271 – 288 .
- Singh , B. and Singh , O. P. 2007 . Simultaneous ionospheric E- and F-layer perturbations caused by some major earthquakes in India . Annals of Geophysics , 50 : 111 – 122 .
- Singh , B. , Kushwah , V. , Singh , O. P. , Lakshmi , D. R. and Reddy , B. M. 2004 . Ionospheric perturbations caused by some major earthquakes in India . Physics and Chemistry of the Earth , 29 : 537 – 550 .
- Singh , O. P. , Chauhan , V. , Singh , V. and Singh , B. 2009 . Anomalous variation in total electron content (TEC) associated with earthquakes in India during September 2006–November 2007 . Physics and Chemistry of the Earth , 34 : 479 – 484 .
- Singh , R. P. 2010 . Satellite observations of the Wenchuan Earthquake, 12 May 2008 . International Journal of Remote Sensing , 31 : 3335 – 3339 .
- Singh , R. P. , Mehdi , W. , Gautam , R. , Kumar , J. S. , Zlotnicki , J. and Kafatos , M. 2010 . Precursory signals using satellite and ground data associated with the Wenchuan Earthquake of 12 May 2008 . International Journal of Remote Sensing , 31 : 3341 – 3354 .
- Vershinin , E. F. , Buzevich , A. V. , Yumoto , K. , Saita , K. and Tanaka , Y. 1999 . “ Correlation of seismic activity with electromagnetic emissions and variations in Kamchatka region ” . In Atmospheric and Ionospheric Electromagnetic Phenomena Associated with Earthquakes , Edited by: Hayakawa , M. 513 – 517 . Tokyo : Terra Scientific .
- Xia , C. , Wang , Q. , Yu , T. , Xu , G. and Yang , S. 2011 . Variations of ionospheric total electron content before three strong earthquakes in the Qinghai-Tibet region . Advances in Space Research , 47 : 506 – 514 .
- Zakharenkova , I. E. , Krankowski , A. and Shagimuratov , I. I. 2006 . Modification of the low-latitude ionosphere before the 26 December 2004 Indonesian earthquake . Natural Hazards and Earth System Science , 6 : 817 – 823 .
- Zakharenkova , I. E. , Shagimuratov , I. I. , Tepenitzina , N.Yu. and Krankowski , A. 2008 . Anomalous modification of the ionospheric total electron content prior to the 26 September 2005 Peru earthquake . Journal of Atmospheric and Solar-Terrestrial Physics , 70 : 1919 – 1928 .
- Zhao , B. , Wang , M. , Yu , T. , Wan , W. , Lei , J. and Lio , L. 2008 . Is an unusual large enhancement of ionospheric electron density linked with the 2008 great Wenchuan earthquake? . Journal of Geophysical Research , 113 A11304, DOI: 10.1029/2008JA013613