ABSTRACT
In this work, we introduce the results of a multi-temporal analysis of UAV (unmanned aerial vehicle) images of the Uggiano castle, a highly degraded medieval archaeological site located about 5 km to the north-west of the Ferrandina town (Basilicata, southern Italy). The geomorphological analysis carried out by multi-temporal images has been integrated with a geological field survey in order to retrace the hillslope evolution of the site as well as the adjacent slopes. This kind of analysis allows to investigate the main stratigraphical and topographic factors responsible for the slope processes, acting along the outer edges of the archaeological site, which is today reduced to a few ruins located at the top of a circular ridge with steep slopes shaped on sandy-conglomerate Pleistocene regressive deposits. The instability phenomena are mainly due to localized erosional processes and falls in conglomerates, which affected the edges of the top surface, where the castle was built. High-resolution images also highlight several collapses of artificial cavities (cisterns) in the central sector of the archaeological site. In addition, a comparison between oblique and vertical UAV images, historical maps, aerial-photo images and the relationships with rainfalls permitted to acquire key data about evolution of slope and erosional processes. Such integrated approach allowed us to individuate in safety conditions the more exposed sectors of archaeological site, where the slope failure processes and collapse cavities occurred, as well as their time activation.
1. Introduction
Satellite and aerial remote-sensing has proved to be a powerful and widely used tool in many geoscience applications (Scaioni Citation2013, Toumi et al. Citation2013, Zhang et al. Citation2005, among others), although high costs and low-spatial resolution can represent a significant limitation for the investigation of small areas. Over the last few years, one of the most promising and innovative methodologies for landscape and cultural heritage investigation is the UAV (unmanned aerial vehicle)-based low-aerial photogrammetry (Fernández-Hernandez et al. Citation2015). The use of UAV images as a tool to support studies in the field of natural and human hazard and cultural heritage has significantly increased, which has contributed to their rapid development due to:
flight performances: UAVs can operate in a wide range of operating heights (from 100 m to over 30000 m) and have a high degree of autonomy (1–48 hours); the use of small UAV's or drones allows the quick collection of aerial images at low and medium altitudes from both vertical and oblique angles;
adaptability to different types of missions;
inexpensiveness: the possibility to plan unmanned aerial vehicles of variable sizes and with a relatively low weight allows performing flight operations at low costs with respect to those required by conventional aerial vehicles.
The first works dealing with UAV-derived images suffered some limitations such as the difficulty in performing ortho-rectification and mosaic of images and an extreme sensibility to wind-induced perturbations (Hendrickx et al. Citation2011). At the same time, however, they already demonstrated a high potential for several types of applications (see for example Hendrickx et al. Citation2011). Lately, advances in UAV technologies and the rapid development of photogrammetry software packages used for generating high-resolution ortophotos and 3D models of complex landscapes have fostered the first applications of UAV images for monitoring and mapping of archaeological excavations (Eisenbeiss & Sauerbier Citation2011; Emaus & Goossens Citation2015; Fernández-Hernandez et al. Citation2015), landslide evolution (Niethammer et al. Citation2012; Turner et al. Citation2015), landscape analysis (Hugenholtz et al. Citation2013; Mirijovskỳ & Langhammer Citation2015) and soil erosion (D'Oleire-Oltmanns et al. Citation2012; Eltner et al. Citation2015). Today, the main advantages of this approach for geomorphological studies are the opportunity to obtain low-cost and accurate multi-temporal digital elevation models (DEMs), which can be very useful to unravel geomorphological evolution and to contribute to provide data for extracting rates of landscape evolution over small areas. In this work, we present the results of a multi-temporal analysis of UAV images of the Uggiano castle (Basilicata, southern Italy), a degraded medieval archaeological site located about 5 km to the NW of the Ferrandina town (), affected by erosional and landslide phenomena. By using an integrated approach based on geomorphological photo-aerial interpretation and field surveys, we performed a detailed geoarchaelogical study of the Uggiano site.
More in detail, the major objectives achieved through this kind of analysis have been:
to detect the usefulness of UAV-derived images in the geomorphological analysis of an archaeological site highly prone to slope failures;
to investigate the historical and recent evolution of slope failure processes affecting the archaeological site through the multi-temporal comparison of historical maps, ortophotos and UAV images;
to define the geomorphological evolution of the hillslope and mass movement processes affecting the archaeological site;
to infer the main predisposing and triggering factors responsible for the widespread instability phenomena.
2. Methods
The recent geomorphological evolution of the site and the adjacent slopes has been retraced through two different surveys (field and UAV images acquisition) of the Uggiano archaeological site, made respectively in September 2012 and April 2013.
The instrumentation consists of an octocopter microdrone platform () equipped with a high-resolution reflex photocamera (Nikon D90 with CMOS image sensor DX format 12.3 megapixel). The reflex camera is located on a movable mechanical arm, which can be rotated during the flight in order to acquire zenithal or perspective pictures. UAV is controlled by a radio with a telemetry system, which transfers the flight information (battery level, geolocation, flight time, velocity and distance from the start point) to the base station in real time. An user-friendly navigation software allows us to define the automatic plan flight (point of acquisition, altitude and timing of flight) through the simple delimitation of the area to be surveyed.
Figure 2. Site investigation with octocopter drone and perspective scheme of images detected at different levels with octocopter drone.
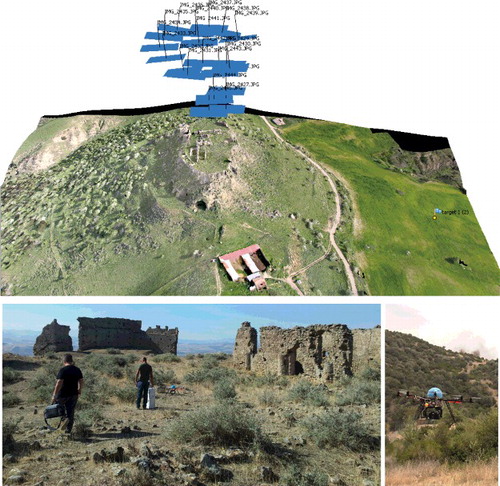
The UAV-derived images have been elaborated through a commercial software package able to process the overlapping photographs with the Structure from Motion (SfM) techniques (Micheletti et al. Citation2015; Turner et al. Citation2015). SfM photogrammetry employs overlapping images acquired from multiple viewpoints of an area of interest () and it is able to reconstruct the 3D full geometry of the scene through the identification of common feature points across the image set (Micheletti et al. Citation2015). Software workflow starts with the detection of tens of thousands of features in each image, which are then matched between the images. Using the common points of the different images, the software extrapolates the spatial relationships between the original image locations in an arbitrary 3D coordinate system. The result is a dense point cloud, which can be used to extract high-resolution DEMs of the scene through the introduction of ground control point (GPCs) with known geographical coordinates.
This kind of approach based on the ortho-rectification of remote-sensing images has been also carried out using several sets of historical aerial photographs, thus permitting a multi-temporal geomorphological analysis of the study area. Specifically, chronological constraints about the slope failure events have been acquired through the comparison of 3D scene of images acquired in 1964, 1966, 1972 and 1982 (see for more details about the flight times and scales).
Table 1. Synthesis of the multi-temporal images used in this study.
The comparison between UAV images and aerial-photos ( and ), historical documents and maps (the data-set is related to the cartographic resources of State archive in Potenza, such as the Military Technical Institute (ITM), the Military Geographic Institute (IGM), the Platee of the Magnifica Università di Ferrandina (1737) of the Intendenza Basilicata) allowed to acquire key data to infer the initiation and evolution of slope processes and land denudation.
The photo-aerial interpretation of the reconstructed 3D scene has been integrated with a detailed geoarchaeological study of the Uggiano site based on geological and geomorphological field surveys. More specifically, a stratigraphic study and facies analysis were performed in the Uggiano archaeological site and the surrounding slope areas in order to define its main stratigraphic features. Such approach allowed us to define the major geological and litho-stratigraphic factors responsible for the instability phenomena of the study area.
3. The Uggiano archaeological site: stratigraphical and historical setting
3.1. Geological framework and stratigraphic features
The archaeological site is located on the top of a circular ridge with steep slopes shaped on sandy-conglomerate regressive deposits (lower-middle Pleistocene) and is today reduced to a few ruins (). From a geological point of view, the study area is located in the foredeep of the southern Apennines chain, a northeast-verging fold-and-thrust belt, built on the western border of the African–Apulian plate from late Oligocene – early Miocene times (Schiattarella et al. Citation2006). The belt is mainly composed of shallow-water and deep-sea sedimentary covers, deriving from Mesozoic-Cenozoic circum-Tethyan domains, covered by Neogene-Pleistocene foredeep and satellite-basin deposits (Pescatore et al. Citation1999). In the early Pleistocene, the foredeep was affected by a subsidence, which resulted in the deposition of marine clays (Argille Subappennnine Fm., see Tropeano et al. Citation2002). Since the middle Pleistocene, a moderate uplift occurred with rates of about 0.3–0.5 mm/yr−1 (Lazzari & Pieri Citation2002; Gioia et al. Citation2014) and the emerged foredeep diachronically was interested by deposition of coarse-grained coastal and continental deposits (Tropeano et al. Citation2002). A coeval and more severe tectonic stage related to a NE–SW trending extensional faulting occurred in the inner and axial sectors of the chain, which promoted a marked uplift and the generation of endorheic conditions in many intermontane basins (Schiattarella et al. Citation2013; Giano et al. Citation2014).
The archaeological site was built-up on coarse-grained deposits (sand and gravel of transitional/marine environment), which are related to the lower-middle Pleistocene depositional regression of the uplifting foredeep. They disconformally overlie grey-blue silty-clay hemipelagites of the Argille Subappennine Fm. (Pieri et al. Citation1998; Lazzari e Pieri Citation2002; Lazzari Citation2008). Physical stratigraphy and facies analysis have been carried out along 15 natural and artificial stratigraphic sections located along the archaeological site and its surrounding. The reconstructed stratigraphic succession () is made up of about 20 meters of siliciclastic sand and gravel of marine-to-transitional environment, which unconformably overlie the grey-blue silty clay.
Figure 4. Stratigraphic section of the Pleistocene regressive marine and transitional sedimentary sequence, outcropping in the Uggiano site.
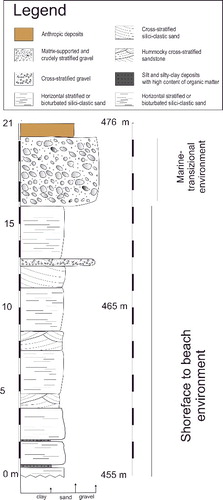
The basal part of the succession is constituted by horizontally stratified siliciclastic sand, organized into 40-to-100 cm-thick strata. These deposits, characterized by a high bioturbation, crop out along the toe of the Uggiano site steep slopes, at an altitude of about 455 m a.s.l. Locally, dark-grey silt and silty-clay deposits with a high content of organic matter are present. Upward, the sand deposits are interbedded with 10-to-40 cm thick arenites showing a hummocky cross-stratification.
The upper part of the succession consists of an alternation of horizontally stratified and cross-stratified sand with gravel deposits, passing upward to well cemented and stratified-to-massive clast-supported conglomerates. The top of the conglomerate represents the surface where the archaeological site was built ()
Figure 5. Outcropping of cemented sandy-pebbly shoreface deposits (a) on which the foundations of wall are based (b). The picture (b) shows clearly the erosional processes acting at the base of relict castle walls.
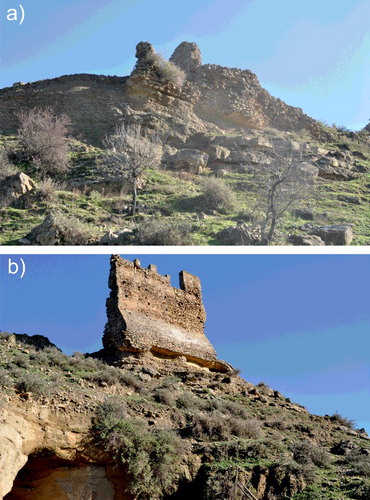
From a lithotechnical point of view, the conglomerates are characterized by high values of friction angle (35°–40°) and by a higher erosion resistance than the stratigraphically lowermost sandy-gravelly and silty-sandy deposits. Moreover, the conglomerates are locally interested by fractures mainly located along the scarp that limits the archaeological site.
3.2. Historical framework and fortified settlement
From a historical point of view, the first settlement is attributed to the Byzantine epoch, while the site reached its maximum splendour in the thirteenth century with the Angevins (Palestina Citation1994).
The toponym ‘Uggiano’ is the latest evolution of the original name ‘Oblanum and/or Obbiano’, falling in Gastaldato ‘Latinianum’ (VII and VIII sections) and used to indicate a high flat shelf. It occupies a clear guard position, strategic and fortified toward the Cavone river valley and the settlement of Craco. The castle had suffered a slow decline since 1400, which led to its complete abandonment in the late fifteenth century.
The Uggiano castle is located at the top of a hill at 479 m a.s.l., at about 5 km to the north-west of the Ferrandina built-up area, between the La Vella river and the Fosso del Varvaro river (). Because of its geographical position and the altitude, the hill overlooks a vast territory, which gave it a strategic importance.
Based on the description contained in the inventory of Pirro del Balzo's assets, count of Andria (1489), the engineer Giuseppe Apollaro suggested a graphic elaboration of the “castle” (), which occupies a wide area (about 6.700 m2) inside a polygon describing the outside walls. Inside the castle wall, six main structural elements () are characterized by different damage levels. In particular:
The main entrance, reachable only through a very steep, narrow road which winds through the collapsed remains. It consists of an arch flanked and protected by two towers of which only one still presents its features. The tower on the left of the entrance gate has completely collapsed.
The walls and the towers. The Castle was surrounded by 7-to-12 m-high walls, whose thickness varied from 1.50 to 3.00 m. Each vertex of the polygon ends in a variable-sized square tower, towards the inside. The southern buttress of the walls is currently made of two structurally independent dividing walls. The collapsed fragments, some of large dimensions, are on the hillside partially covered with soil and vegetation. The north-western wall, the highest, shows a vertical slit, with a simple horizontal shifting of the stone lines. The south tower and the wall walk, clearly visible in aerial images until 1972, collapse, definitively between 1964 and 1973.
The courtyards, separated from each other by thick walls so as to allow the defence also from the interior of the Castle in case the enemy had managed to cross the outer wall. The bigger courtyards were provided with reservoirs (cisterns), which collected rainwater.
The chapel, probably dedicated to St. Lorenzo, located on the west side of the residential apartments, shows a rectangular plan and an apse (partially collapsed), whose foundations are still visible.
The residence of the Lord of the Castle, located in the interior and protected by the surrounding buildings. The entrance to the apartment, consisting of a large doorway topped by an Angevin pointed arch, led directly into a large access corridor between the north and south wings of the Lord of the Castle's apartment.
The barracks, NE and WSW, housed the quarters of the garrison.
The outside of the Castle. Along the hillsides, below the walls, stood many small houses made of poor materials, of which a few traces remain visible. Today, to witness their presence, there are only a few walls, stripped by erosion and the collapses of the overlying walls of the Castle.
Figure 6. Graphic reconstruction of the Uggiano Castle. Legend: tower of the five cantons (1); the tower of Gennaro (2); entrance (3); prison (4); courtyard or square (5); courtyard (6); chapel (7), or a Church just begun and below it a tank under construction; building/stable (8); kitchen (9); courtyard (10), where there is a big cystern with two openings, partially collapsed (see photos); garden (11), around which stand the defensive walls, all around the castle, with other towers adorned with battlements and other buildings.
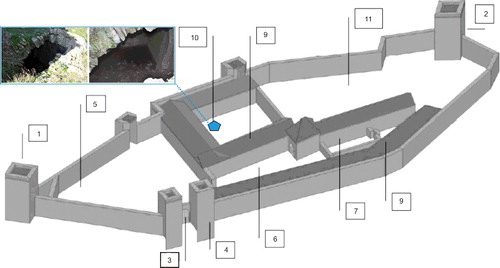
3.3. Structural damage evolution by historical aerial-photos and UAV images
Today, the archaeological site is strongly degraded and most of the elements of the ancient settlement are completely destroyed. Little remains of the fortified site are the fourteenth century pointed arch, the remains of the chapel and some portions of the walls and towers.
The use of azimuthal UAV images and, especially, of those oblique allowed to define, in safety conditions:
areas with homogeneous structural degradation
to facilitate the cataloguing of the wall structures, that still exist;
the main structural damage and discontinuity;
the morphoevolutive processes ongoing and interacting with the walls.
These preliminary information are crucial to take appropriate diagnostic investigations, the project of restoration and consolidation of masonry structures and to stabilize slopes subjected to widespread erosion.
The multi-temporal UAV perspective images permitted to better analyse the state of conservation of walls offering different points of view respect to azimuthal aerial-photos and ground-based observations.
The comparison between the historical oblique photos of 1964, 1966 and 2013 made it possible to define, in greater detail, the distribution of structural damage and the interaction with local morphoevolutive processes. highlights that in 1964 (a) there was a patrol communication trench outside the south tower, interested below by evident voids erosional. This communication trench disappears in the 1966 images (b), as it collapsed in the previous period, even for the erosion and water leachant action of the intense rainfalls, recorded at Ferrandina meteo station, occurred in May (101.2 mm) and November (109 mm) of 1964, December (101.2 mm) 1965, November (197.2 mm) 1968, December/January (442) 1971/1972, June (145.2) and December (111.8) 1972 and, finally, February (128.8) 1973.
Figure 7. Temporal evolution of damages suffered by fortified archaeological site from 1964 (a) and 1966 (b) to 2013 (c). c1) inlet points of piping erosion phenomena affecting the study area.
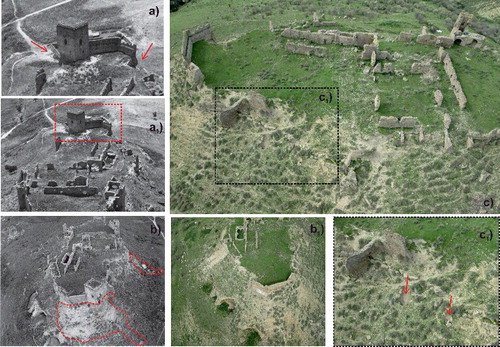
Between 1966 and 1973, the south tower collapsed and parts of the surrounding walls for the erosion and excavation to the foot. From the 1970s to 2013 several collapses occurred, confined to the remaining wall parts of the outer perimeter, favoured by phenomena of piping erosion (C1) and water leachant action ().
4. Geomorphological features
The Uggiano hill is located at the top of a circular relief showing an elongated, almost sub-elliptical, shape with the major axis aligned along the north-south direction. The lowermost sectors of the landscape is carved by channels incising into the Early-Pleistocene blue silty clay of the Argille Subappennine Fm, which are locally affected by badlands, shallow landslides and gullies ().
The archaeological site is located on the flat top of a landscape showing a complex slope profiles. Landscape close to the outer walls of the Castle is characterized by a high slope (i.e. >50°), as typically observed in location of medieval fortresses. Slopes of the study area become less pronounced, particularly on the west-northwest side where the remains of the urban settlement are present. In fact, altitude passes rapidly from 479 m to 440 m, where a terraced area of probable anthropic origin can be observed along the hillside, very noticeable on the east side ().
Figure 8. Geomorphological hazard map of the study area on a photo mosaic of images detected by the drone on 12 April 2013.
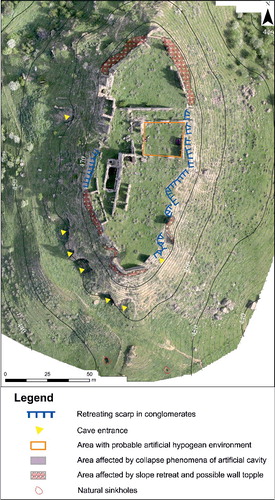
Field geomorphological survey and photo-aerial interpretation allowed to detect the main geomorphological features linked to the instability phenomena (falls) affecting the edge of the sub-horizontal surface, where the castle was built. In fact, slopes of the study area are not featured by landforms related to linear erosion such as gullies and rills, whereas the most relevant mass movement processes are represented by falls and topples, related to slope retreating and erosion acting on the base of the foundations of the perimetral wall structures (). The absence of geomorphological evidences of slides along the slopes of the archaeological site seems to confirm that the main geomorphological processes responsible for the instability phenomena are the preferential erosion of finer deposits along the stratigraphic boundary with the conglomerates. High-resolution images also highlight several collapses of artificial cavities (cisterns) in the central sector of the archaeological site (), but it is possible that other cavities could be hidden under heaps of rubble of collapsed walls inside the castle perimeter. To define the spatial distribution of these cavities other specific investigations should be carried out, useful for drawing the geometry of the voids and estimation of their susceptibility to collapse (Parise Citation2015).
The comparison between the present situation and the oldest repertory aerial-photos (1964, 1966, 1972, 1982, 2008; see ) also allowed to verify that over the last 50 years, several collapses of structures have occurred (–), due to erosional processes probably accelerated by severe rainfall events.
Figure 9. Old repertory images of the three towers collapsed presumably in March 1973 in a photo of the 1960s. Arrows indicate their position in the reconstruction of .
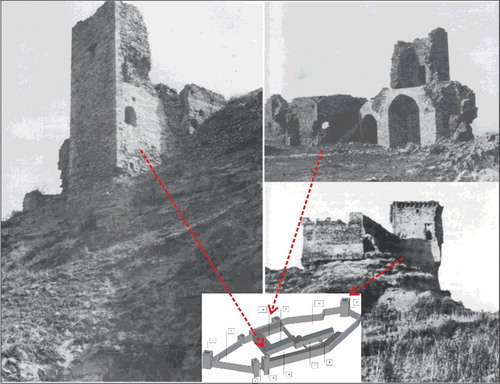
To test the latter possibility, we have considered the rainfall data, recorded at the meteorological station of Ferrandina (National Hydrographic Service; LON 16°26’33’’ LAT 40°29’25’’), homogenized and calculated on a period of 59 years (1951–2010). It shows a maximum yearly rainfall of 1,078 mm (1972), significantly higher than the average annual rainfall of 513 mm; the historical sources give the date of March 1973 (Palestina Citation1994) as a reference to the collapse of two towers (, and ); in this month, between the days 24 and 31, an extreme rainfall event occurred, where 124 mm of rain fell on a total of 138.80 mm ().
Figure 10. The picture shows the ‘Gennaro's Tower’ (n° 2 in ) existing until 1972, compared with the relict structures of the tower and toppled walls after the collapse, related to slope retreating and erosion, acting at the base of the foundations.
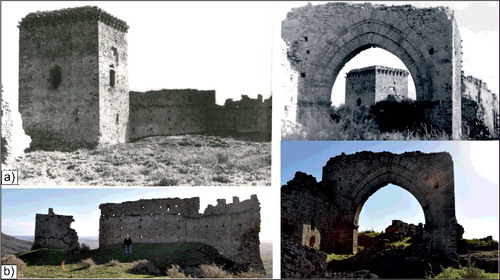
Figure 11. Comparison between 1972 and 1982 images in which it is possible to observe the presence of the towers in 1972 (circles) and their disappearance in 1982 with the presence of heaps of rubble of collapsed walls (arrows and dashed circle).
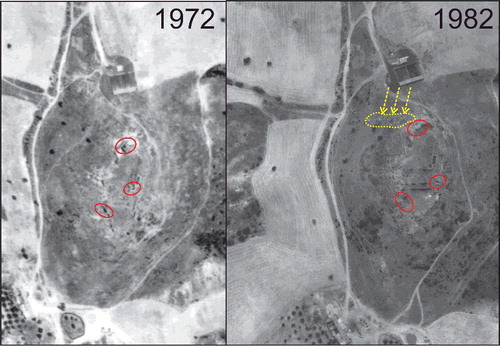
Figure 12. Histograms that show the trend of the total rainfall and average monthly rainfall measured by the Ferrandina meteorological station, with evidence of the extreme rainfall peak, which has accelerated the erosion processes responsible of the collapse of the towers.
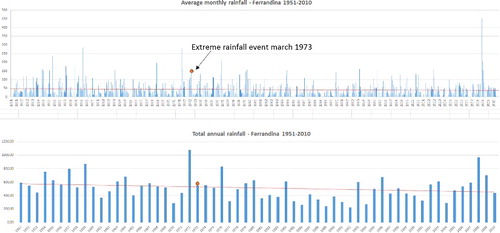
Therefore, by crossing information derived from observations of aerial photographs of 1964, 1966 and 1972 (before the collapse of the towers) and 1982 (towers already collapsed) with the rainfall data described above, we can infer that extreme rainfall events (Lazzari et al. Citation2013; Piccarreta et al. Citation2013), occurred during the 1972–1973 biennium, accelerated the erosive and water leaching actions, determining the conditions to the collapse of the fortified structures, weakened to the foot of their foundations; thus confirming the relationship of cause-effect between climate and the main collapses of 1973.
Multi-temporal analysis carried out by UAV images compared with other recent remotely sensed images also suggested a substantial stability of the landslide phenomena affecting the outer edge (perimeter) of the archaeological site in the last 10 years. This observation is also confirmed by the comparison of the DEM deriving by the two UAV surveys.
5. Concluding remarks
This work demonstrated the usefulness of multi-temporal analysis of historical aerial-photo and UAV images as a tool to support the geomorphological analysis and the identification of activation time and predisposing and triggering factors of slope processes affecting the edges of a highly degraded archaeological site. Mass movement processes are mainly represented by falls and topples, related to slope retreating and erosion acting on the base of the foundations of the outer wall structures.
The oblique images have allowed to observe in detail and in safe conditions, the presence of piping phenomena (both inlet and outlets points) in interbedded sandy-silty deposits, which favour the formation of voids and cavities beneath the foundations as well as the acceleration of erosion. These processes promoted the retreat of upper escarpment in conglomerates and the consequent collapse of relict wall structures. The comparison of historical oblique images allowed us to verify the existence and action of these phenomena since at least 1964.
Visual comparisons between ortho-rectified historical aerial-photo and UAV-based images allowed us to reconstruct the occurrence time of significant structure collapses, probably due to erosional processes related to severe rainfall events in the 1972–1973 period. Geomorphological analysis and photo-aerial interpretation indicate the absence of fall phenomena over the last 10 years.
The approach here adopted based on the integration of field survey and mapping of geomorphological processes, multi-temporal photo-aerial interpretation and analysis of archive and historical data can represent a basic and effective tool to constrain the geomorphological evolution of the archaeological site and surrounding slopes and to outline the possible safeguard planning. Collected data could be useful to plan possible mitigation actions and priorities of intervention for the historical heritage conservation.
In fact, it is possible to define, in a qualitative way, the sectors of the Uggiano site where it is more urgent to plan actions aimed at the risk mitigation to protect relict historic structures. In particular, our data suggest the following four warning areas characterized by increasing risk:
probable hypogeal environment (areas subjected to instrumental verify);
areas affected by possible collapses of vault cave (areas subjected to field survey and instrumental verify);
areas affected by collapse cavity phenomena;
areas affected by slope retreat and falls of the perimetral surrounding walls; in these sectors, clearly it is necessary to take urgent measures to mitigate the effects of erosion and realize the consolidations of relict walls in order to prevent their irreversible loss.
It is concluded that the targeted use of small remote–controlled aerial platforms can significantly add to existing practices of both site-recognition and heritage management in the heavily threatened historical sites, and that it can be feasibly and efficiently integrated within standard methodologies applied in the field of geoarchaeology and applied geomorphology.
Acknowledgments
The authors sincerely thank the two anonymous referees and the guest editor Daniele Giordan for helpful suggestions and constructive reviews.
Disclosure statement
No potential conflict of interest was reported by the authors.
References
- D'Oleire-Oltmanns S, Marzolff I, Peter KD, Ries JB. 2012. Unmanned aerial vehicle (UAV) for monitoring soil erosion in Morocco. Remote Sens-Basel. 4:3390–3416.
- Eisenbeiss H, Sauerbier M. 2011. Investigation of UAV systems and flight modes for photogrammetric applications. Photogramm Rec. 26:400–421.
- Eltner A, Baumgart P, Maas HG, Faust D. 2015. Multi-temporal UAV data for automatic measurement of rill and interrill erosion on loess soil. Earth Surf Proc Land. 40:741–755.
- Emaus R, Goossens R. 2015. Low cost 3D-modelling of a complex archaeological site using aerial photography in the hinterland of Petra, Jordan. Int Arch Photogramm. Remote Sens Spatial Inf Sci. XL-5/W4:77–84.
- Fernández-Hernandez J, González-Aguilera D, Rodríguez-Gonzálvez P, Mancera-Taboada J. 2015. Image-based modelling from unmanned aerial vehicle (UAV) photogrammetry: an effective, low-cost tool for archaeological applications. Archaeometry. 57:128–145.
- Giano SI, Gioia D, Schiattarella M. 2014. Morphotectonic evolution of connected intermontane basins from the southern Apennines, Italy: the legacy of the pre-existing structurally controlled landscape. Rendiconti Lincei. 25:241–252.
- Gioia D, Gallicchio S, Moretti M, Schiattarella M. 2014. Landscape response to tectonic and climatic forcing in the foredeep of the southern Apennines, Italy: insights from quaternary stratigraphy, quantitative geomorphic analysis, and denudation rate proxies. Earth Surf Proc Land. 39:814–835.
- Hendrickx M, Gheyle W, Bonne J, Bourgeois J, de Wulf A, Goossens R. 2011. The use of stereoscopic images taken from a microdrone for the documentation of heritage – an example from the Tuekta burial mounds in the Russian Altay. J Archaeol Sci. 38:2968–2978.
- Hugenholtz CH, Whitehead K, Brown OW, Barchyn TE, Moorman BJ, LeClair A, Riddell K, Hamilton T. 2013. Geomorphological mapping with a small unmanned aircraft system (sUAS): feature detection and accuracy assessment of a photogrammetrically-derived digital terrain model. Geomorphology. 194:16–24.
- Lazzari M. 2008. Il comportamento tettonico e sedimentario del bacino d'avanfossa Bradanica durante il Pleistocene inferiore. [Tectonic and sedimentary behaviour of the bradano foredeep during the Early Pleistocene]. Mem Descr Carta Geol d'It, LXXVII:61–76.
- Lazzari M, Piccarreta M, Capolongo D. 2013. Landslide triggering and local rainfall thresholds in Bradanic foredeep, Basilicata region (southern Italy). In: Margottini C, Canuti P, Sassa K, editors. Landslide science and practice. p. 671–678.
- Lazzari M, Pieri P. 2002. Modello stratigrafico-deposizionale della successione regressiva infrapleistocenica della Fossa bradanica nell'area compresa tra Lavello, Genzano e Spinazzola [Stratigraphical and depositional model of the lower Pleistocene regressive succession of the Bradano foredeep in the area between Lavello, Genzano and Spinazzola]. Memorie Società Geologica Italiana. 57:231–237.
- Micheletti N, Chandler JH, Lane SN. 2015. Structure from motion (SFM) photogrammetry. London: LE Clarke, Nield JM editors.
- Mirijovskỳ J, Langhammer J. 2015. Multitemporal monitoring of the morphodynamics of a mid-mountain stream using UAS photogrammetry. Remote Sens-Basel. 7:8586–8609.
- Niethammer U, James MR, Rothmund S, Travelletti J, Joswig M. 2012. UAV-based remote sensing of the Super-Sauze landslide: evaluation and results. Eng Geol. 128:2–11.
- Palestina C. 1994. Ferrandina. La terra di Oblano. Dagli insediamenti enotri alla città di Ferrante Venosa [Ferrandina. Oblano's land. From the Enotri settlements to the town of Ferrante Venosa]. Potenza (Italy): Appia 2 Editrice.
- Parise M. 2015. A procedure for evaluating the susceptibility to natural and anthropogenic sinkholes. Georisk. 9:272–285
- Pescatore T, Renda P, Schiattarella M, Tramutoli M. 1999. Stratigraphic and structural relationships between Meso-Cenozoic Lagonegro basin and coeval carbonate platforms in southern Apennines, Italy. Tectonophysics. 315:269–286.
- Piccarreta M, Pasini A, Capolongo D, Lazzari M. 2013. Changes in daily precipitation extremes in the Mediterranean from 1951 to 2010: the Basilicata region, southern Italy. Int J Climatol. 33:3229–3248.
- Pieri P, Tropeano M, Sabato L, Lazzari M, Moretti M. 1998. Quadro stratigrafico dei depositi regressivi della Fossa bradanica (Pleistocene) nell'area compresa fra Venosa e il Mar Ionio [Stratigraphic outline of the regressive deposits of the Bradano foredeep in the area between Venosa and Ionian coastal belt]. Giornale di Geologia. 60:318–320.
- Scaioni M. 2013. Remote sensing for landslide investigations: from research into practice. Remote Sens-Basel. 5:5488–5492.
- Schiattarella M, Giano SI, Gioia D, Martino C, Nico G. 2013. Age and statistical properties of the Summit Palaeosurface of southern Italy. Geografia Fisica E Dinamica Quaternaria. 36:289–302.
- Schiattarella M, Leo PD, Beneduce P, Giano SI, Martino C. 2006. Tectonically driven exhumation of a young orogen: an example from the southern Apennines, Italy. Geol S Am S ( Special Paper of the Geological Society of America). 398:371–385.
- Toumi S, Meddi M, Mahe G, Brou YT. 2013. Remote sensing and GIS applied to the mapping of soil loss by erosion in the Wadi Mina catchment. Hydrolog Sci J. 58:1542–1558.
- Tropeano M, Sabato L, Pieri P. 2002. Filling and cannibalization of a foredeep: the Bradanic Trough, southern Italy. In: Jones SJ, Frostick LE, editors. Sediment flux to basins: causes, controls and consequences. London: Geological Society, Special Publications. p. 55–79. doi:10.1144/GSL.SP.2002.191.01.05
- Turner D, Lucieer A, de Jong SM. 2015. Time series analysis of landslide dynamics using an unmanned aerial vehicle (UAV). Remote Sens-Basel. 7:1736–1757.
- Zhang ZQ, Gong HL, Zhao WJ, Zhang YQ. 2005. Application of remote sensing to study of landslide. In: Proceedings of 2005 IEEE International Geoscience and Remote Sensing Symposium, 2005 Jul 25–29; IGARSS '05 p. 1546–1549.