Abstract
This paper aimed to screen the potential species suitable for ecological restoration and slope stability from local natural growing plants in China Loess Plateau under a semiarid climate. As part of the field investigations of local natural growing plants, potential species, which are suitable candidates for ecological restoration and slope stability, were nominated in the hilly-gullied region in the Yan'an area. The results showed that Artemisia spp. is the best candidate to form a stable root-soil composite system to support the loose loess and reinforce the loose soil, particularly suitable as pioneer plant in the initial stage of loess slope ecosystem reconstruction. Field root pull-out test and direct shear test for soil without roots and root-soil composite systems were conducted to analyse the reinforcement effect of Artemisia spp. The results from quantitative analysis of the slope protection effect showed that the slope safety factor could be obviously improved by the growth of Artemisia spp. As the survey, test, stability analysis and case study shown, Artemisia spp. can effectively prevent the occurrence of loess flow slides and shallow landslides, which has extensive application prospect.
Introduction
In recent years, the occurrence of slope failure and soil erosion induced by local environment, such as seasonal heavy rainfall, has been increasing all around world especially in arid and semiarid region worldwide (Eab et al. Citation2014). Due to the fragile ecological environment and the influence of human engineering activities, the loess slope geological disasters such as soil erosion and landslides have been reported frequently in China Loess Plateau (), which covers 6 × 105 km2 over the northern Shaanxi province and extensive areas of the Shanxi, Gansu, Ningxia and Henan provinces (Wang et al. Citation2015; Hu et al. Citation2018).
Loess has distinctive physical and mechanical properties (Hessel and Asch Citation2003; Jefferson et al. Citation2003; Acharya et al. Citation2011; Shroder et al. Citation2011; Wang et al. Citation2013), such as high porosity, sets of vertical joints and metastable structure failure (loess water collapsibility), so that the Loess Plateau is one of the regions prone to soil erosion and geological hazards in China (Sun Citation1989; Dijkstra et al. Citation1994; Derbyshire et al. Citation2000; Derbyshire et al. Citation2001; Li et al. Citation2013a; Li et al. Citation2013b, Hu et al. Citation2018), where about 20% of the Chinese population lives.
On the other side, in Yan’an area (), the man-made engineering activities to expand the urban areas due to the growth of economy and population may exacerbate the environmental destruction and geological disaster (Liu and Li Citation2014). The Yan'an area has experienced several geological disasters in the past. For example, in July 2013, a heavy rainfall in Yan'an area induced more than 8000 landslides (about 0.22 landslides/km2), most of which within the loess flow slides and killed 45 residents (Wang et al. Citation2015). These loess slope geological disasters not only pose a serious threat to the life of local residents but also worsen the problems of soil erosion and natural environment deterioration, which would be feeding back into the still fragile loess slope and induce new geological disasters.
. Location of study area. a) Location of Loess Plateau and Yan’an, b) Location and topography of Yan’an area (including Yan’an city and other counties), c) Yan’an new area (Satellite image from Google Earth), d) Huangjiaguagou high fill slope (Satellite image from Google Earth). Source: Juang et al. (Citation2019)
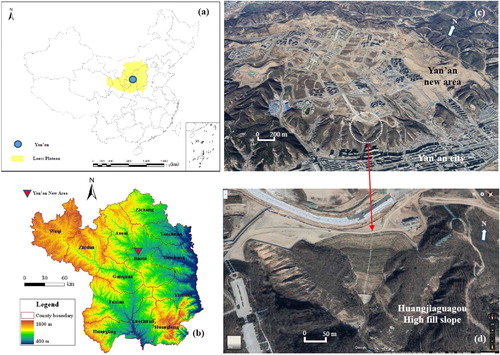
Therefore, the enhancement of geological disaster treatment and environmental protection is one of the most important tasks in China Loess Plateau, especially in Yan'an area. Traditional geological hazard treatment methods, such as anti-slide pile, anchor, retaining wall, satisfy the safety requirements and have been widely used in loess slope engineering.
The instability of loess slope can be mitigated by the traditional method of treating slope, but the negative impact that they will have on the appearance of its surrounding environment is of a concern. A soil-bioengineering approach is an alternative which is the use of live materials such as plants, vegetation and grasses in protection of the slope against failure and soil erosion (Eab et al. Citation2014, Citation2015). With the theory of soil-bioengineering, one solution may be the combination of engineering technical projects and ecological restoration measures, which must be also locally applicable (Marco Citation2007). These researches show that the contents of soil-bioengineering are not related to the mere use of vegetation for stabilizing purposes, but they focus on broader environmental concerns (Bischetti et al. Citation2014). There is an emphasis on the necessity to reconcile both natural hazard control and ecological restoration (Rey et al. Citation2019).
With the development of soil-bioengineering, the concept of slope ecological protection has emerged and the relevant practices of soil-bioengineering are arising in recent years (O’Loughlin Citation1974; Gray and Leiser Citation1982; Gray and Sotir Citation1996; Greenwood et al. Citation2006; Barker Citation1995; Niu et al. Citation2016; Sidle and Bogaard Citation2016). The significance of plants in water and soil conservation has been generally an accepted and established approach (Ren Citation2004; Zhang et al. Citation2007; Evette et al. Citation2009; Burylo et al. Citation2011; Eab et al. Citation2014, Citation2015), but vegetation growing on a slope has traditionally been considered to have a minor effect on slope stability. However, in some cases, this assumption may not be correct, and a growing body of research suggests that the loess slope protection by vegetation is an effective method for restoration ecology and geological disaster prevention (Waldron Citation1977; Cislaghi et al. Citation2019; Nguyen et al. Citation2019).
There are three main slope protection effects of vegetation: hydrologic effect of stems and leaves, soil reinforcement of roots and ecological restoration. Stems and leaves can effectively reduce surface erosion of loess slope in rainfall, mainly with rainfall interception effect and rainfall reallocation effect by plant stems and leaves, the effect of reduction in raindrop splash erosion by foliage, and plant residues and surface runoff retardation effect by plant aboveground residues. The developed root system can not only effectively enhance the survivability of vegetation, but also form a stable root-soil composite system to protect slope with reinforcement effect by fibril roots, anchorage effect by taproots and traction effect by horizontal roots.
Many researchers focus on the relationships between slope stability and slope plants worldwide (Stokes, Norris, et al. Citation2008; Stokes, Sotir, et al. Citation2010), including the study in Loess Plateau (Li and Li Citation2011; Ji et al. Citation2012; Ma et al. Citation2012; Zhang et al. Citation2014; Fu et al. Citation2016; Liu et al. Citation2017). The stability of loess slope protection by vegetation can be affected by the complex interactions of factors beyond geological engineering conditions, such as local soil characteristics (Charlafti Citation2014), plant traits, human activity and climate (Stokes et al. Citation2009). Because the distribution of the plant species is local, there are complex interactions of factors, as there are in any part of the technology promotion and application, where obstacles tend to be complicated and localized. In the international literature, data on the effect of slope plant can be found and generalized for similar environments, and many researchers agree that those values need to be localized for any specific project. Study on loess slope stability by vegetation requires the screening for right plants adapted to local environmental strains.
In this paper, the engineering geological characteristic and the vegetation community structure were studied through field investigation in Yan'an area. The vegetation community structure was analysed by data statistics to screen out the potential species for ecological restoration and slope protection. The results present the findings of an exploratory analysis into the use of native Artemisia spp. for ecological restoration and slope stability. Field root pull-out test and direct shear test for soil without roots and root-soil composite systems were conducted to analyse the reinforcement effect of Artemisia spp. The results from quantitative analysis of the slope protection effect showed that the slope safety factor could be obviously improved by the growth of Artemisia spp. As the survey, test, stability analysis and case study shown, Artemisia spp. can effectively prevent the occurrence of loess flow slides and shallow landslides, which has extensive application prospect.
Materials and methods
Study area
Situated in Northern Shaanxi Province on the south-central part of the Loess Plateau (35°21′–37°31′ N and 107°41′–110°31′ E) (Lü et al. Citation2012), the Yan'an area overlooks the middle reaches of the Yellow River, the cradle of Chinese Civilization, and covers an area of about 3.7 × 104 km2. This survey, of more than 300 natural loess slopes and very few artificial slopes, began in 2014 and continues to present day, has been expanded from Yan’an city (Baota county) to other 11 counties (.
Yan'an has a semiarid continental climate that borders on a steppe climate, with cold, dry and moderately long winters, and hot, somewhat humid summers. Spring and autumn are short transition seasons in between. The monthly 24-hour average temperature ranges from –5.5 °C (22.1 °F) in January to 23.1 °C (73.6 °F) in July, and the annual mean is 9.90 °C (49.8 °F). The mean annual precipitation is 511 mm (20.1 in) of precipitation, and more than 70% of the annual precipitation may fall in a few heavy rainstorms between June and September (). The greatest single storm event within a 24-h period was 139.9 mm in 1981, and the maximum monthly rainfall was 577 mm in July 2013. Moreover, the rainfall events with high intensities and shorter durations play dominant roles in causing soil and water loss in the study area and then induce various geological hazards. However, with strong evaporation and arid climate, this region has a weak ecological environment in general, and it is difficult to breed and propagate plants.
In Yan’an area, elevations generally increase from south-east to north-west with an altitude between 500 and 1600 m.a.s.l. There is a huge difference between daytime and night-time temperatures because of the climate and geographical condition. The geomorphic landscape is usually characterized by gullies, where the gravity erosion such as slide and collapse is very active.
In general, the stratum in Yan’an is deposited in sequences of sandstone (J), mudstone (N) and thick-bedded eolian Pleistocene loess (Q). The Pleistocene loess (Q) is, in order from old to young formations, Late Pleistocene Wucheng Loess (Q1) in some regions, Middle Pleistocene Lishi Loess (Q2) and Early Pleistocene Malan Loess (Q3) with a thickness of about 10–30 m (). In particular, the Malan loess (Q3) has a well-distributed particle size, is porous (commonly with porosities of more than 50%) and possesses unstratified structures, obvious perpendicular fissures, large bearing capacity, collapsibility and structural behaviour. These characteristics make the Malan loess (Q3) sensitive to water infiltration. Water increases the bulk weight of the Malan loess and reduces its shear strength. As a result, shallow loess flow slides and landslides frequently occur along the loess slopes (Wang et al. Citation2015).
Vegetation survey
Considered as the most basic measure of plant–environment relationships, vegetation community structure is a collection or association of plant species within a designated geographical unit, which forms a relatively uniform patch, distinguishable from neighbouring patches of different vegetation types. The components of each plant community are influenced by soil type, topography, climate and human disturbance.
In order to characterize the topography and physiognomy of the Loess Plateau, field investigations were carried out in loess hilly and gully region, mainly in spring, summer and early autumn. In order to get more statistic of local natural plants and analyse vegetation community structure, the investigators mainly drive along the country roads and then take a walking deep into loess hilly and gully where it is usually hard for car to get in. Representative loess slopes were chosen for the survey, with thick loess cover and well-grown natural plants. At each investigation site, we considered a quadrat of 1 × 1 m for the investigation and took photographs. With ruler and shovel, we could cut an outline of the investigation quadrat (1 × 1 m) on the slope. It was ensured that the distance between the investigation point and the upper or lower edges of slope is not less than 1.5 m. The information that was collected throughout the survey included the following: (1) The latitude, longitude, slope gradient, hydrogeological condition, etc.; (2) the statistics of plants, such as dominant families, genera and vegetation density as well as growth characteristics; and (3) photographs and videos. The data were analysed using statistical methods. Typical investigation point and simple field book are exemplified in .
Table 1. An example of the field book completed during the survey.
Soil section survey
In the process of vegetation survey, the status of the soil section was examined using some typical samples of natural loess slope. The dry excavation method was carefully conducted along the plant’s growth position in both directions of slope’s elevation and section. In order to measure, record and assess the root distribution and architecture characteristics with original natural state, the excavation should be no less than the root depth.
After the soil section survey, the soils without roots and root-soil composite system samples were excavated out carefully from the soil section. Then, plastic bags were used to envelop all blocks of soil samples immediately, and they were individually put into the testing cases and were taken back to the laboratory for direct shear tests and moisture content tests. Typical section excavation and sampling process on some of the slopes mostly covered with Artemisia spp. are shown in .
Field root pull-out test
Root-soil mechanical interaction is a key to loess slope stability, so we conducted field root pull-out test to analyse the reinforcement effect of dominant species during the process of the vegetation survey. To implement the experiment, a equipment for field root pull-out test was designed. Its tripod is portable and adjustable to irregular slope, and can support a force of up to 1.8 kN. The guy wire of the winch passes through a centring guide which is mounted at the top of the tripod. A force transducer with a max support of 1.5 kN and a displacement transducer with ranging accuracy of millimeter are installed in this equipment. The history data can be stored automatically in the transducer, or can also be send to a laptop computer that instantly stores the data and generates a displacement versus force graph. Afterwards, the peak in the generated graph indicates the maximum force required for a plant to be uprooted. The equipment for field root pull-out test is shown in .
These tests were performed under similar weather and soil conditions. No rain showers had occurred during the previous days so that the soil was mostly dry and dense (most soil moisture content is about 15%∼18% according to moisture content tests). Each selected plant was identified with a specific number, and the ground surface around the plants was cleared to facilitate the setting of the equipment. In the next step, the lengths of the plants were measured with a yardstick and the diameter of each stem was taken using a Vernier calliper. The pull-out process is performed at a constant rate of uplift (about 100 mm/min). The test was continued until the entire root system was pulled out of the soil. Additionally, the root characteristics of the plants were measured on site after the pull-out test. In total, 103 pull-out tests, in similar conditions, performed for 11 species, including five species of Artemisia spp. (Artemisia ordosica (Artemisia ordosica Krasch.), Artemisia hedinii (Artemisia hedinii Ostenf. et Pauls.) and Artemisia sacrorum (Artemisia sacrorum Ledeb.), Artemisia annua (Artemisia annua Linn.), Artemisia dracunculus (Artemisia dracunculus Linn.)) and other six species of Herb, which were the most common species on natural loess slopes in this survey. The data generated during the pull-out test would be analysed statistically in order to get a better understanding of the maximum uprooting resistance of different species.
Soil direct shear test
It is well known that root-soil interactions can effectively improve slope stability. Most studies have shown that the physical and mechanical parameters of soil without roots are obviously lower than the parameters of root-soil composite systems (Waldron Citation1977; Wu et al. Citation1979; Waldron and Dakessian Citation1981; Ziemer Citation1981; Ekanayake and Phillips Citation1999; Nilaweera and Nutalaya Citation1999; Operstein and Frydman Citation2000).
As the Artemisia spp. was one of the most common species during the survey, the six blocks of soil without roots and the six blocks of root-soil composite system samples, from the slopes mostly covered with Artemisia spp. (Artemisia ordosica (Artemisia ordosica Krasch.)) in the same area, were sent back to the laboratory for indoor soil test, mainly including moisture content test and direct shear test. First, the moisture content of soil was measured. Then, the soil samples for large direct shear test were obtained with the rigid steel-cutting ring (sample diameter 300 mm, height 150 mm) from the blocks of soil, and four samples were taken from each block of soil. At last, direct shear tests were conducted on each sample under four vertical pressures (50, 100, 200 and 300 kPa). The process was performed at a constant shear rate (0.8 mm/min). The test was continued until the sample was completely destroyed.
Case study
In addition to general constructions such as building and roads, there is a large-scale project on going in the Loess Plateau, which is part of the campaign to remove the upper level of the mountains to fill in the valleys to create flat land for more constructions (Li et al. Citation2014). This is one of the largest projects in the Yan’an new area (), which started in April 2012 and is expected to double the city’s current area by adding 78.5 km2 of flat ground (Bai et al. Citation2014). The location and topography of Yan’an and Yan’an new area, as well as typical high fill slope formed by removing the tops of mountains to fill the valley, are shown in . This will enhance the probability of the hazards associates with the changes in the rock and soil structure. As shown in , Huangjiaguagou high fill slope is located on the edge of Yan’an new area, which was once a several hundred meters deep natural gully. We continued to investigate the local natural growing plants in Huangjiaguagou high fill slope since 2014. With this case study, the relative dominance of local pioneer plant was investigated. Moreover, the change of diversity of species in an ecological community, as well as the slope protects effect of local pioneer plant, can be further analysed.
Results
Vegetation community structure
The statistics of plant species is one of the most important indexes of vegetation community structure. In this survey, although a great diversity of plants was observed, only 33 main plant species on natural loess slope in Yan’an area were analysed, including 15 species of Herb, nine species of Shrub, eight species of Arbor and 1 species of Moss. The statistic on the number of occurrences of main vegetation species in surveys is listed in .
The above statistics indicate that the vegetation species diversity in Yan’an is below the medium level, which accords with the slope vegetation composition characteristics of loess plateau semiarid areas. The main vegetation species statistics is also indicative of the dominance of Herb, and therein Artemisia spp., mainly including Artemisia ordosica (Artemisia ordosica Krasch.), Artemisia hedinii (Artemisia hedinii Ostenf. et Pauls.) and Artemisia sacrorum (Artemisia sacrorum Ledeb.). These are the main varieties of loess slope naturally growing vegetation and repeated most often in the data statistic, which are the potential species for ecological restoration and slope protection in Yan’an area.
The state of plant community is directly affected by the local slope habitat with many influencing factors, such as slope height, slope degree, slope aspect, soil thickness and soil matrix. illustrates the number of species, and illustrates the number of plant in every slope. Based on the data analysis shown in , the main influencing factors in this study appear to be the slope and soil moisture. Generally, the number of species as well as plants declines obviously with less soil moist and deeper slope, such as the statistical mean value shown in . However, with regard to the statistical max value shown in , the number of species in ‘wet’ condition is less than that in ‘medium’ condition of soil moisture. The reason, soil moisture is the main factor of plant growth, but some local drought-tolerant species may not stand the long-term high soil moisture environment.
Figure 7. Statistics of species, plant number, soil moisture and slope. a) statistics of species, soil moisture and slope and b) statistics of plant number, soil moisture and slope.
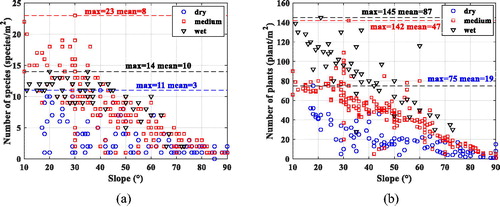
Loess in Yan’an is a porous (commonly with porosities of more than 50%) formation and possesses unstratified structures, with obvious perpendicular fissures, collapsibility and structural behaviour. These characteristics significantly reduce the soil’s capacity to store water and nutrients at ordinary times, which dries out most plants. On the other hand, the loess’s sensibility to water infiltration significantly increases the bulk weight of loess and reduces its shear strength during the heavy rainfall, so shallow loess flow slides and landslides frequently occur along the loess slopes; then, soil erosion, as well as land degradation, is aggravated. Therefore, the soil’s capacity to store water and nutrients is one of the most important influencing factors. The ‘soil moist’ of the root zone layer, recorded in vegetation investigation, presents a good reflection of above influencing factors on the soil’s capacity to store water and nutrients. Similarly, the ‘slope’ is another most important influencing factor, which is often viewed as direct parameter impacting the slope stability, plants attachment, soil erosion as well as above factors.
Growth characteristics
As mentioned before, the vegetation species diversity in Yan’an is below the medium level, which accords with the slope vegetation composition characteristics of loess plateau semiarid areas. The drought and freezing-tolerant plant types are the main varieties of loess slope vegetation. However, there are significant differences in growth status between plant species. For example, Herb accounts more than 70% of the total number of plants were seen in all slopes under this investigation (), and a considerable part of Herb is Artemisia spp. (. Moreover, as shown in , this trend of high proportion of Herb and its Artemisia spp. is even more marked with less soil moist and more slope.
Artemisia spp. is the main species of loess slope naturally growing vegetation in the data statistic, which is the potential species for ecological restoration and slope protection in Yan’an area. Study on its slope protection effect, as well as growth characteristics, plays an important role in loess slope stability and ecological restoration.
Field root pull-out test
The collected data from the pull-out test plus the most correlating variable of the plants’ morphological properties and the maximum uprooting resistance are shown in , together with the linear curve fitting and coefficient of determination (R2).
As show, among these correlating variable of the plants’ morphological properties, maximum taproot diameter (R2 = 0.61) is most relevant to maximum uprooting resistance force, so the relationship between maximum taproot diameter and maximum uprooting resistance force was chosen to analyse the differences between species. In general, the root density is one of the most major factors to influence the ultimate uprooting resistance, but maximum taproot diameter also could be the factor to reflect indirectly the influence degree of root density, easier for statistics and analysis. In some studies, maximum uprooting resistance force is the same parameter as shear strength to compare the qualification of the species to be used in loess slope protection (Florineth Citation2012). The greater the maximum uprooting resistance force (or shear strength) of a single plant, the stronger its ability to reinforce soil shear strength (Gray and Sotir Citation1996; Zhu et al. Citation2008; Hu et al. Citation2009; Yang et al. Citation2009). Comparing with , Artemisia spp. show a better performance than other Herb (R2 = 0.04) in uprooting resistance, which is important in engineering applications, so that Artemisia spp. has great potential in ecological restoration and slope protection in Yan’an area.
Soil direct shear test
Indoor soil test, mainly including moisture content test and direct shear test, was conducted for both Artemisia ordosica (Artemisia ordosica Krasch.) root-soil composite systems and soil without roots. The results showed line relationships between shear strength and vertical pressure. The results were derived from 48 samples, the number of tested replication was 6, and root area ratio (RAR) after test averaged 0.3%. According to the moisture content tests of 12 samples, average soil moisture content is 16.85% and 16.75% for Artemisia spp. root-soil composite systems and soil without roots, respectively. The reinforcement effect of topsoil with Artemisia ordosica (Artemisia ordosica Krasch.) roots through the direct shear tests is shown in . In this figure, values of shear strengths are shown as a function of the vertical stress. Both cohesion force and internal friction angle of the root-soil composite system for Artemisia ordosica (Artemisia ordosica Krasch.) are notably greater than those of the soil without roots, with an incremental cohesion force value of around 34.45% (from 8.95° to 10.26°) and internal friction angle value of around 14.64% (from 4.18 to 5.26) greater than soil without roots, respectively.
Discussion
Relative dominance of Artemisia spp. on loess slope
The harsh conditions, such as geology, soil and climate, produce an unfavourable environment for plants grown in Yan’an area, where common slope-protecting plants in other parts of China can be difficult to grow on loess slope. Besides the plant adaptation to the severe environment in Yan’an area, species colonization is a great potential risk with the introduction of alien species.
Therefore, it is necessary to screen out local native plants to choose potential species, which are suitable for ecological restoration and slope protection in Yan’an area. Among these plant species on natural loess slope in this vegetation survey, Artemisia spp. found to be the main species of loess slope naturally growing vegetation. As above statistical analysis showed, Artemisia spp. quantity decreases with the increase of gradient or the decrease of soil moisture as all other species, but the relative dominance of Artemisia spp. increases on loess slope, accounting for about over half of all the plants with the slope more than 40° (), which shows that Artemisia spp. has a better adaptability to surrounding and its advantage will be more obvious with habitat destruction.
Taking Huangjiaguagou high fill slope as an example, the relative dominance of Artemisia spp. was further analysed. As shown in , Huangjiaguagou high fill slope is located on the edge of Yan’an new area, which was once a several hundred meters deep natural gully (. In July 2015, shortly after this high fill slope was completed, we carried out a field survey on it and analysed its environment changes, aiming to identify the potential species for ecological restoration and slope protection. Taken in July 2015, photographs of give a visual representation of the situation. Soil erosion and desiccation in this slope were very serious. Most of the artificial cultivated plants with good care, such as cypress (Platycladus orientalis), have shrivelled and died. On the other hand, wild Artemisia spp., almost the only species, has completely covered the entire slope () like many other investigation slopes.
Figure 14. Huangjiaguagou high fill slope. a) An overview of the slope (July 2015), b) the growth state of plants (July 2015) and c) the growth state of plants (July 2016).
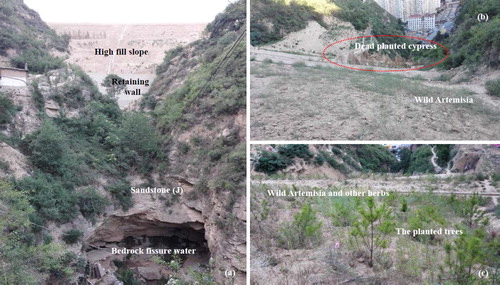
Various reasons contribute to the relative dominance of Artemisia spp., with its strong resistance to cold, drought, barren and salinity believed to be the primary ones. Secondly, the root distribution characteristic is another limiting factor for plant growth in Yan’an area. Although Artemisia spp. is one of the hardy herbaceous plants that belongs to the daisy family Asteraceae, the roots of Artemisia spp. have the characteristic of woody plants, including long, thick, sturdy and woody taproot as well as developed lateral root, which can adapt to the local harsh environment of soils and slope. Artemisia spp. usually has a root length of over 0.5 m, more than its height of the plant (), and considerable part of Artemisia spp. species can be over 2 m in this survey (), which can form a more stable root-soil composite system, resulting in stronger uprooting resistance () to allow themselves to take root in the loose loess. Moreover, Artemisia spp. can fully use water and nutrients in barren loess with its developed root system.
Loess slope ecological protection of Artemisia spp
Yan'an area is the worst vulnerable eco-environment, soil erosion and loess geological disaster area in China, where loess slope geological disasters seriously aggravate the problems of soil erosion and natural environment deterioration, which, in turn, would be feeding back into the fragile loess slope and induce new geological disasters. The best way to avoid this vicious circle is loess slope stabilization by vegetation to fundamentally improve slope stability and environment. Artemisia spp. is the main species on natural loess slope due to its viability, which is the suitable species for ecological restoration and slope protection. The hydrologic effect of stems and leaves, soil reinforcement of roots and ecological restoration of Artemisia spp. is significant. Artemisia spp. can effectively reduce surface erosion of loess slope in rainfall. The developed root system can not only effectively enhance the survivability of Artemisia spp., but also form a stable root-soil composite system to greatly improve the mechanical parameters of soil, such as cohesion force and internal friction angle, as above large direct shear test shown ().
Compared to other species, another important advantage of Artemisia spp. is an obvious effect of ecological restoration. The vegetation surveys for many years in a row have shown that the community structure of the ecosystem can be restored by Artemisia spp. It is all known that the diversity of species in an ecological community is the core of ecosystem stability, sustainability and rehabilitation. Although Artemisia spp. is an absolute majority in most steep loess slopes, the relative dominance of Artemisia spp. will keep decreasing with time. Through two-year observations (2015–2016) and comparison in community structure of Huangjiaguagou high fill slope, it can be seen that plant species remarkably increased from a single Artemisia spp. (comparing .
The developed root system of Artemisia spp. not only reduces soil erosion and desiccation, and improves the stability of the slope, but also improves soil’s ability of fertilizer and water conservation and then encourages suitable habitats for microorganisms. With the improvement of soil condition, various kinds of other plants, which might not once survive in this barren soil, are able to grow well. This illustrates the ecological restoration and reconstruction of loess slope are gradually improved because of the ecological plasticity of Artemisia spp. Therefore, Artemisia spp. is particularly suitable as pioneer plant for loess slope ecosystem reconstruction without special maintenance, and then, the diversity of species in an ecological community can be increased effectively by natural growth or human interaction in this improving process of soil.
Loess slope stability protection of Artemisia spp
It is well known that root-soil interactions can effectively improve slope stability. As shown in , both cohesion force (c) and internal friction angle (ϕ) of the Artemisia spp. root-soil composite system are notably greater than those of the soil without roots, with an incremental cohesion force value of around 34.45% and internal friction angle value of around 14.64% greater than soil without roots, respectively. On the other hand, of the plants analysed in our field root pull-out test, Artemisia spp. has obviously more stable performance than other plants in uprooting resistance (), which is an indicator for the stability of the soil-root composite systems and expresses the stabilizing effects of plants on the soil.
The quantitative analysis of the slope protection effect of Artemisia spp. was provided by the calculation of the safety factor (Fs) of different slopes by a classical shear reduction method with the software FLAC in 2D. The model is shown in . The safety factor (Fs) was determined based on strength reduction technique which consists to decrease the shear strength of the soil matrix until failure occurs. A detailed description of this kind of procedure can be found in previous works (Kokutse et al. Citation2016; Nguyen et al. Citation2018). In this slope model of 10-m height, the effects of the roots are modelled by increasing the cohesion force and internal friction angle value of slope topsoil as shown in . We examined the influence of different angles α of a slope ranging from 30° to 60° (α = 30°, 45° and 60°). As simulation results shown in , an improvement of the slope’s stability, with the slope protection effect of Artemisia spp., is easily perceptible. It is all known that mild or moderate slope (smaller inclination) is more stable than a sharp (peaked) slope. More importantly, the more slope angle, the better are the slope protection effect of Artemisia spp. Simulation results show an improvement of the safety factor from the slope angle from 30°, 45° to 60°, and the safety factor increased respectively by 2.63%, 9.52% and 26.97% with the slope protection effect of Artemisia spp.
Figure 15. Numerical modelling. a) contour of max shear strain increment and b) safety factor as function of slope angle.
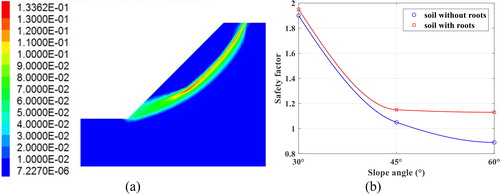
Of course, the selected plants for slope protection should not only have better mechanical properties ( and ) but also be suitable for the characteristics of local geological disaster, such as rain-triggered shallow slider. Yan'an is a geological disaster-prone and vulnerable eco-environmental area. Besides soil erosion, loess slope failure, including landslide and loess flow-slide, is the most severe problem of geological disaster in Yan’an. Loess flow slide is the most common hazard following rainfall, characterized by a small thickness, narrow-long shape, rapid surface runoff and high water content (meeting or exceeding the liquid limit), and the occurrence of loess flow slide is closely related to rainfall intensity, infiltration depth and weak strength of the saturated loess (Wang et al. Citation2015). Based on previous researches (Dai et al. Citation1999; Billard et al. Citation2000; Tu et al. Citation2009; Zhang and Liu Citation2010), the loess flow slides are usually induced by rainfall and occur in a surface layer of completely saturated loess with a depth of <2 m, corresponding to a surface layer of completely saturated loess. The consequence of an infiltration depth of <2 m was essentially consistent with our investigation in Yan’an area ().
Because the water content of loess is the main controlling factor of the shear strength, the loess flow slide usually occurred under a certain thickness of saturated, consistent with the infiltration depth of loess slope under heavy rainfall. It is well known that plants are beneficial for preventing soil erosion with hydrological and mechanical effects, but vegetation growing on a slope has traditionally been considered to have a temporary and limited effect on a slope stability, because the roots of some grasses (e.g. Setaria viridis (Setaria viridis (Linn.) Beauv.)) and bushes (e.g. Sabina vuglaris (Sabina vulgaris Ant.)) generally only extend <50 cm in depth, much less than infiltration depth of loess in rainfall. However, our field investigation showed that the root length of most Artemisia spp. is usually more than its plant height (), and many perennial Artemisia spp. species, having been growing for a year or two, can reinforce deeper loess mass and effectively prevent the occurrence of loess flow slides as well as shallow landslides with over 2-m root system as an anchor for the overlying materials. Although many trees also have longer and stronger roots than many other plants in Yan’an area, their great weight makes themselves difficult to stick on the steep and loose slope, as shows that trees can only grow on the platform on top of the slope, consistent with the statistics of species (). Moreover, the environment requirements of trees, such as water, nutrients and soil condition, are much more than Artemisia spp. Therefore, Artemisia spp. is particularly suitable as pioneer plant for loess slope ecosystem reconstruction and slope stability protection.
In conclusion, the conceptual vegetation strategies of loess slope protection in Yan’an are suggested in . As this figure shown, all kinds of plants can protect slope with soil reinforcement of roots. For example, herbaceous plants have only shorter and superficial roots which can hardly reinforce deeper soil, but the reinforcement effect by their fibril roots can maximize ground cover and associated interception to reduce the effects of rainsplash and runoff in rainfall. So it is an appropriate strategy that we should use all kinds of plants as many as possible for ecological restoration and slope protection, especially selected Artemisia spp. that can effectively prevent the occurrence of loess flow slides and shallow landslides (with slip planes less than 2 m deep). Despite the importance of species diversity, it should be noted that many species, such as shrubs and trees, are restricted to the lower and wetter parts of slope, as these species are hardly adapted to high steep slopes () the barren areas where protection against soil erosion, shallow landslides, loess flow slides and debris flows is most required (Wang and Lee Citation1998; Zhou and Zhang Citation2003; Guo et al. Citation2004; Li et al. Citation2007; Hu et al. Citation2013).
Because Artemisia spp. has a better adaptability to surrounding and its advantage will be more obvious with habitat destruction, Artemisia spp. accounts for about over half of all the plants if the slope is more than 40° (). Therefore, Artemisia spp. is particularly suitable as pioneer plant for loess slope ecosystem reconstruction without special maintenance. However, the species diversity in an ecological community is beneficial to the ecosystem stability, sustainability, and rehabilitation (). Besides Artemisia spp. without special maintenance, some selected plants, such as shrubs and herbs, should be sown and watered at the beginning of growth until their relatively longer roots ensure that they are able to extract water from depth, sustaining their growth. And if necessary, the engineering measures, such as geocell and drainage network, are suggested to ensure plants survival in high steep slopes.
Conclusions
This paper has presented a long-term investigation of the local natural growing plants in the hilly-gullied region in the Yan'an area since 2014 and aims to screen the potential species suitable for ecological restoration and slope stability in China Loess Plateau under a semiarid climate. The conclusion can be highlighted as follows:
With investigation statistics, it is found that the drought and freezing tolerant plant types are the main varieties of loess slope vegetation. Among these, Artemisia spp. is the main species of loess slope naturally growing vegetation in Yan'an area and has a better adaptability to surrounding;
Through the long-term observation of the evolution process of slope, the ecological restoration and reconstruction of loess slope are gradually improved with the ecological plasticity of Artemisia spp. This illustrates that Artemisia spp. is particularly suitable as pioneer plant in the initial stage of loess slope ecosystem reconstruction;
The reinforcement effect of Artemisia spp. root-soil composite system was considered through the field root pull-out test and soil direct shear test. The results from quantitative analysis of the slope protection effect showed that the slope safety factor (Fs) could be obviously improved by the growth of Artemisia spp.
Artemisia spp. may reinforce deeper loess (up to about 2 m) and effectively prevent the occurrence of loess flow slides and shallow landslides, which has extensive application prospect.
In future studies, more detailed analysis on the complex correlation among the factors will be addressed by continuous measurements of the relationship among community structure, soil erosion and long evolution process of slope.
Author’s contributions
R. X. and X. C. L. involved in study design and conduct; R. X. involved in data collection, management and analysis, and R. X., W. Y., and M. R. involved in interpretation; R. X. and M. R. prepared manuscript, reviewed or approved; and R. X. and C. J. revised the manuscript.
Acknowledgements
Finally, the authors would like to thank Professor Vamegh Rasouli, University of North Dakota, for valuable comments on an earlier draft of this paper
Disclosure statement
No potential conflict of interest was reported by the authors.
Additional information
Funding
References
- Acharya G, Cochrane T, Davies T, Bowman E. 2011. Quantifying and modeling post failure sediment yields from laboratory-scale soil erosion and shallow landslide experiments with silty loess. Geomorphology. 129(1–2):49–58.
- Bai XM, Shi PJ, Liu YS. 2014. Realizing China's urban dream. Nature. 509(7499):158–160.
- Barker DH. 1995. Vegetation and slopes: stabilisation, protection and ecology. Proceedings of the international conference held at the university museum, Oxford; September 29–30, 1994.
- Billard A, Muxart T, Andrieu A, Derbyshire E. 2000. Loess and water In: Derbyshire E, Meng XM, Dijkstra TA, editors. Landslide in the thick loess terrain of North-West China. Chichester: John Wiley & Sons Ltd; p. 91–130.
- Bischetti GB, Di Fi Dio M, Florineth F. 2014. On the origin of soil bioengineering. Landsc Res. 39(5):583–595.
- Burylo M, Hudek C, Rey F. 2011. Soil reinforcement by the roots of six dominant species on eroded mountainous manly slopes (Southern Alps, France). Catena. 84(1–2):70–78.
- Charlafti M. 2014. Slope stability and vegetation. J Archit Eng Tech. 3(4):1–8.
- Cislaghi A, Giupponi L, Tamburini A, Giorgi A, Bischetti GB. 2019. The effects of mountain grazing abandonment on plant community, forage value and soil properties: observations and field measurements in an alpine area. CATENA. 181:104086.
- Dai FC, Lee CF, Wang SJ. 1999. Analysis of rainstorm-induced slide-debris flows on natural terrain of Lantau Island, Hong Kong. Eng Geol. 51:279–290.
- Derbyshire E. 2001. Geological hazards in loess terrain, with particular reference to the loess regions of China. Earth Sci Rev. 54(1–3):231–260.
- Derbyshire E, Meng XM, Dijkstra TA. 2000. Landslides in the thick loess terrain of northwest China. Chichester: Wiley, p. 1–256.
- Dijkstra TA, Rogers CDF, Smalley IJ, Derbyshire E, Li YJ, Meng XM. 1994. The loess of north-central China: geological properties and their relation to slope stability. Eng Geol. 36(3–4):153–171.
- Eab KH, Takahashi A, Likitlersuang S. 2014. Centrifuge modelling of root-reinforced soil slope subjected to rainfall infiltration. Géotechn Lett. 4(3):211–216.
- Eab KH, Likitlersuang S, Takahashi A. 2015. Laboratory and modelling investigation of root-reinforced system for slope stabilization. Soils Found. 55(5):1270–1281.
- Ekanayake JC, Phillips CJ. 1999. A method for stability analysis of vegetated hillslopes: an energy approach. Can Geotech J. 36(6):1172–1184.
- Evette A, Labonne S, Rey F, Liebault F, Jancke O, Girel J. 2009. History of bioengineering technique for erosion control in rivers in Western Europe. Environ Manage. 43(6):972–984. doi:10.1007/s00267-009-9275-y.
- Florineth F. 2012. Pflanzen statt Beton. Handbuch zur Ingenieurbiologie und Vegetationstechnik. Berlin – Hannover: Patzer Verlag.
- Fu J, Hu X, Brierley G, Qiao N, Yu Q, Lu H, Li G, Zhu H. 2016. The influence of plant root system architectural properties upon the stability of loess hillslopes, Northeast Qinghai, China. J Mt Sci. 13(5):785–801. doi:10.1007/s11629-014-3275-y.
- Gray DH, Leiser AT. 1982. Biotechnical slope protection and erosion control. New York: Van Nostrand Reinhold Company Inc..
- Gray DH, Sotir RB. 1996. Biotechnical and soil bioengineering slope stabilization: a practical guide for erosion control. Chichester: Wiley and Sons.
- Greenwood JR, Norris JE, Wint J. 2006. Site investigation for the effects of vegetation on ground stability. Geotech Geol Eng. 24(3):467–481.
- Guo HR, Lu XL, Liao ZW. 2004. Ecological restoration method for rock slopes in Hong Kong. Pratacultural Sci. 21(9):83–86. (In Chinese)
- Hessel R, Asch T. 2003. Modelling gully erosion for a small catchment on the Chinese Loess Plateau. Catena. 54(1–2):131–146.
- Hu S, Jiao J, García-Fayos P, Kou M, Chen Y, Wang W. 2018. Telling a different story: plant recolonization after landslides under a semi-arid climate. Plant Soil. 426(1–2):163.
- Hu XS, Brierley G, Zhu HL, Li GR, Fu JT, Mao XQ, Yu QQ, Qiao N. 2013. An exploratory analysis of vegetation strategies to reduce shallow landslide activity on loess hillslopes, northeast Qinghai-Tibet plateau, China. J Mt Sci. 10(4):668–686.
- Hu XS, Li GR, Zhu HL. 2009. Research on interaction between vegetation root and soil for protection and its mechanical effect in cold and arid environments. Chin J Rock Mech Eng. 28(3):613–620. (In Chinese)
- Jefferson IF, Evstatiev D, Karastanev D, Mavlyanova NG, Smalley IJ. 2003. Engineering geology of loess and loess-like deposits: a commentary on the Russian literature. Eng Geol. 68(3–4):333–351.
- Ji J, Kokutse N, Genet M, Fourcaud T, Zhang Z. 2012. Effect of spatial variation of tree root characteristics on slope stability. A case study on Black Locust (Robinia pseudoacacia) and Arborvitae (Platycladus orientalis) stands on the Loess Plateau, China. Catena. 92:139–154. doi:10.1016/j.catena.2011.12.008.
- Juang CH, Dijkstra T, Wasowski J, Meng X. 2019. Loess geohazards research in china: advances and challenges for mega engineering projects. Engn Geol. 251:1–10.
- Kokutse NK, Temgoua AGT, Kavazović Z. 2016. Slope stability and vegetation: conceptual and numerical investigation of mechanical effects. Ecol Eng. 86:146–153.
- Li GR, Mao XQ, Ni SC. 2007. Brief analysis of the effect of slope protection by shrub-grass vegetation. Pratacultual Sci. 24(6):86–89. (In Chinese)
- Li PY, Qian H, Wu JH. 2014. Environment: accelerate research on land creation. Nature. 510(7503):29–31.
- Li TL, Wang P, Xi Y. 2013a. The mechanisms for initiation and motion of Chinese loess landslides. In: Wang F, editor. Progress of geo-disaster mitigation technology in Asia, environmental science and Engineering. Berlin, Heidelberg: Springer; p. 105–122.
- Li TL, Zhao JF, Li P, Wang FW. 2013b. Failure and motion mechanisms of a rapid loess flowslide triggered by irrigation in the Guanzhong irrigation area Shaanxi, China. In: Wang F, Miyajima M, Li T, Shan W, Fathani TF, editors. Progress of geo-disaster mitigation technology in Asia. Berlin Heidelberg: Springer.
- Li P, Li Z. 2011. Soil reinforcement by a root system and its effects on sediment yield in response to concentrated flow in the loess plateau. Agric Sci. 02:86–93. doi:10.4236/as.2011.22013.
- Liu Y, Hu X, Yu D, Li S, Yang Y. 2017. Research on the microstructure features and friction characteristics of shrub roots and soil interface in loess area of Xining Basin. Chin J Rock Mech Eng. 37:198–205.
- Liu YS, Li YH. 2014. Environment: China's land creation project stands firm. Nature. 511(7510):410–410.
- Lü YH, Sun RH, Fu BJ, Wang YF. 2012. Carbon retention by check dams: regional scale estimation. Ecol Eng. 44(1):139–146.
- Ma L, Wu P, Wang Y. 2012. Spatial distribution of roots in a dense jujube plantation in the semiarid hilly region of the Chinese Loess Plateau. Plant Soil. 354(1–2):57–68.
- Marco J. Van De Wiel 2007. A new model to analyse the impact of woody riparian vegetation on the geotechnical stability of riverbanks. Earth Surf Process Landforms. 32(14):2185–2198.
- Nguyen TS, Likitlersuang S, Jotisankasa A. 2018. Stability analysis of vegetated residual soil slope in Thailand under rainfall conditions. Environ Geotech (online). 1.
- Nguyen TS, Likitlersuang S, Jotisankasa A. 2019. Influence of the spatial variability of the root cohesion on a slope-scale stability model: a case study of residual soil slope in Thailand. Bull Eng Geol Environ. 78(5):3337–3351.
- Nilaweera NS, Nutalaya P. 1999. Role of tree roots in slope stabilisation. Bull Eng Geol Environ. 57(4):337–342.
- Niu Q, Zhao K, Wang YH, Wu Y. 2016. Examining the influence of vegetation on slope hydrology in Hong Kong using the capacitive resistivity technique. J Appl Geophys. 129:148–157.
- O’Loughlin CL. 1974. The effect of timber removal on the stability of forest soils. J Hydrol NZ. 13:121–134.
- Operstein V, Frydman S. 2000. The influence of vegetation on soil strength. Ground Improv. 4(2):81–89.
- Ren YS. 2004. Geological hazard and prevention measures in Xining City. Qinghai Soil Econ Strategy. 3:31–33 (In Chinese).
- Rey F, Bifulco C, Bischetti GB, Bourrier F, De Cesare G, Florineth F, Graf F, Marden M, Mickovski SB, Phillips C, et al. 2019. Soil and water bioengineering: practice and research needs for reconciling natural hazard control and ecological restoration. Sci Total Environ. 648:1210–1218. doi:10.1016/j.scitotenv.2018.08.217.
- Shroder JF, Schettler MJ, Weihs BJ. 2011. Loess failure in northeast Afghanistan. Phys Chem Earth. 36(16):1287–1293.
- Sidle RC, Bogaard TA. 2016. Dynamic earth system and ecological controls of rainfall-initiated landslides. Earth-Sci Rev. 159:275–291. doi:10.1016/j.earscirev.2016.05.013.
- Stokes A, Atger C, Bengough AG, Fourcaud T, Sidle RC. 2009. Desirable plantroot traits for protecting unstable slopes against landslides. Plant Soil. 324(1–2):1–30.
- Stokes A, Norris JE, Beek L, Bogaard T, Cammeraat E, Mickovski SB, Jenner A, Iorio A, Fourcaud T. 2008. How vegetation reinforces soil on slopes. In: Norris JE, Stokes A, Mickovski SB, Cammeraat E, van Beek R, Nicoli BC, Achim A, editors. Slope stability and erosion control ecotechnological solutions. Dordrecht: Springer; p. 65–118.
- Stokes A, Sotir R, Chen W, Ghestem M. 2010. Soil bio- and eco-engineering in China: past experience and future priorities. Ecol Eng. 36(3):247–257. doi:10.1016/j.ecoleng.2009.07.008.
- Sun GZ. 1989. Engineering geological and mechanical characteristics of loess in northwestern China and related problems in geological engineering. Lanzhou: Lanzhou University Press.
- Tu XB, Kwong AKL, Dai FC, Tham LG, Min H. 2009. Field monitoring of rainfall infiltration in a loess slope and analysis of failure mechanism of rainfall-induced landslides. Eng Geol. 105(1–2):134–150.
- Waldron LJ. 1977. The shear resistance of root-permeated homogeneous and stratified soil. Soil Sci Soc Am J. 41(5):843–849.
- Waldron LJ, Dakessian S. 1981. Soil reinforcement by roots: calculation of increased soil shear resistance from root properties. Soil Sci. 132(6):427–435.
- Wang GL, Li TL, Xing XL, Zou Y. 2015. Research on loess flow-slides induced by rainfall in July 2013 in Yan’an, NW China. Environ Earth Sci. 73(12):7933–7944.
- Wang KJ, Lee CF. 1998. Brief mechanical analysis of bioengineering techniques for slope protection. Chin J Rock Mech Eng. 17(6):687–691. (In Chinese)
- Wang JJ, Liang Y, Zhang HP, Wu Y, Lin X. 2013. A loess landslide induced by excavation and rainfall. Landslides. 11(1):141–152.
- Wu TH, McKinnell Iii WP, Swanston DN. 1979. Strength of tree roots and landslides on Prince of Wales Island, Alaska. Can Geotech J. 16(1):19–33.
- Yang P, Xiang ZH, Hu XS. 2009. Limit load of soil-root composites. CMC. 10(2):117–137.
- Zhang C-B, Chen L-H, Jiang J. 2014. Vertical root distribution and root cohesion of typical tree species on the Loess Plateau, China. J Arid Land. 6(5):601–611. doi:10.1007/s40333-014-0004-x.
- Zhang GQ, Chai NY, Zhao HF. 2007. Actual conditions of soil and water erosion, ecology and control measure in the drainage area of Huangshui River. J Northwest Forest Univ. 22(4):70–72. (In Chinese)
- Zhang M, Liu J. 2010. Controlling factors of loess landslides in western China. Environ Earth Sci. 59(8):1671–1680.
- Zhou DP, Zhang JY. 2003. Bio-geotechnical technology of vegetation. Beijing: China Communications Press (In Chinese).
- Zhu HL, Hu XS, Mao XQ. 2008. Study on mechanical characteristics of shrub roots for slope protection in loess area of Tibetan plateau. Chin J Rock Mech Eng. 27(Supp. 2):3445–3452 (In Chinese).
- Ziemer R. 1981. Roots and the stability of forested slopes. In: Timothy R, Davies H, Andrew JP, editors. Erosion and Sediment Transport in Pacific Rim Steeplands, Proceedings of the Christchurch Symposium. Christchurch, NZ: International Association of Hydrological Sciences Publication