Abstract
The evolution of reservoir landslides upstream of river buildings is a key issue because landslides continue to cause damage to the ecological environment, especially the safety of dams and the operation of reservoirs. Based on geographic information system (GIS), four high-resolution remote sensing images captured at a hydropower reservoir in southwest China during four different periods are used to interpret landslides. Landslide maps coupled with lithological setting, water level change history and some other factors are used to illustrate the spatial-temporal evolution of landslides in the upstream reservoir of river buildings. The results from this analysis indicate that the landslides are more likely to occur in Quaternary deposits, fractured rock masses and water softened rock masses, whose engineering properties are quite sensitive to water. Three main types of landslides are observed in the reservoir, include gully landslide, bank collapse and deep-seated landslide, among which bank collapse is the dominate type. Fluctuation of water level, together with the human activities and lithological setting, affect the deformation and failure process of the landslides. The deep-seated landslides in the reservoir were observed to experience a relative long period of large deformation until the occurrence of a disaster.
1. Introduction
River buildings such as dams, ship locks and ports have been built extensively around the world, causing changes in upstream water levels. Therefore, the stability of the upstream slope is disturbed to varying degrees by the fluctuation of the water level, resulting in various types of landslides, such as, bank collapse and deep-seated landslide (Paronuzzi et al. Citation2013; Benza et al. Citation2016; Zhang et al. Citation2018). Landslides, especially the deep-seated landslides, in the upstream river generally experience a long period of large deformation process until a disruptive collapse, which can cause severe disasters, for example, disruptions of the functioning of downstream river buildings and the downstream cities over a relatively short time (Petley et al. Citation2005; Chi et al. Citation2008; Sun et al. Citation2015; Zhou et al. Citation2016). Therefore, studying the temporal and spatial evolution of landslides under the influence of water level fluctuations can provide references and suggestions for disaster prevention and reduction.
Methods such as field observation and real-time monitoring are widely used to study the deformation characteristics and triggering mechanisms of landslides (Jian et al. Citation2009; Macfarlane Citation2009; Ma et al. Citation2016; Palis et al. Citation2017; Li et al. Citation2017; Chen et al. Citation2018). In addition, indoor model tests and theoretical studies are used to clarify the effects of various detrimental factors such as the fluctuation of water levels, composition of the rock and soil, pore-water pressures and rainfall history, on the dynamic evolution of landslides (Jia et al. Citation2009). Combined with finite element method, finite volume method and digital elevation model, numerical methods can study more in details the sliding mechanism and deformation failure mode of landslides (Li et al. Citation2004; Yan et al. 2010; Song et al. Citation2015; Huang and Gu Citation2017; Huang et al. Citation2018). By adjusting parameters, various reservoir operating conditions can be simulated, and the relationships between various factors can be clearly summarized, providing important indicators for the operation of hydropower stations (Hong et al. Citation2017). With the emergence of remote sensing technology, the overall perspective of landslides in reservoir areas can be interpreted (Henriques et al. Citation2015; Hong et al. Citation2016; Uhlemann et al. Citation2016; Hong, Liu et al. Citation2018). Moreover, analytical hierarchy process, frequency ratio and logistic regression models are often used for landslide susceptibility analysis, providing better technical support for reservoir operation (Bui et al. Citation2016; Ilia and Tsangaratos Citation2016; Moosavi and Niazi Citation2016; Youssef et al. Citation2016; Hong, Pradhan et al. Citation2018). It cannot be denied that previous research has made a great contribution to knowledge about landslides. However, due to a wide range of reservoir areas and the inaccessibility of some areas, global monitoring of reservoirs can hardly be achieved, resulting in an insufficient understanding of reservoir areas. Indoor model tests and numerical methods can be used to study the effects of detailed parameters and dynamic response processes, but most of them have been focused only on individual landslides. In addition, many scholars have also attempted to make predictions about landslides in reservoir areas through spatial analysis. However, the accuracy of this method for landslide prediction is difficult to judge.
In this study, taking the Jinping I reservoir area as an example, four high-resolution remote sensing images (obtained on the 21 March 2012, 17 September 2013, 21 October 2014 and 23 October 2016, respectively) were interpreted using the geographic information system (GIS). Therefore, a rough distribution of large-scale landslides over four periods was obtained. A large number of field surveys have been carried out to correct these landslide distribution maps. Combined with the water level fluctuation, rainfall data, bank slope lithology and faults and human activities, the failure mode of the slope landslide and the landslide-prone area are discussed. In addition, the temporal and spatial evolution of landslides during water level fluctuations is analyzed in detail.
2. Study area
The Jinping I Hydropower Station is located on the Yalong River, situated at the junction of Yanyuan County and Muli County, Liangshan Yi Autonomous Prefecture, Sichuan Province, as shown in (Lan et al. Citation2004). It is a controlled reservoir cascade hydropower station with the richest downstream hydropower resources in the Yalong River. The dam site of the Jinping I Hydropower Station controls a basin area of 102,600 km2, accounting for 75.3% of the entire Yalong River basin area. The multi-year average flow in the reservoir area is reported to be 1200 m3/s. The reservoir normal water level and dead water level are 1880 and 1800 m, respectively.
Figure 1. Location of the Jinping I Hydropower Station: (a) location of study area in the southwest China and (b) location of the Jinping I Hydropower Reservoir study area. Source: Author
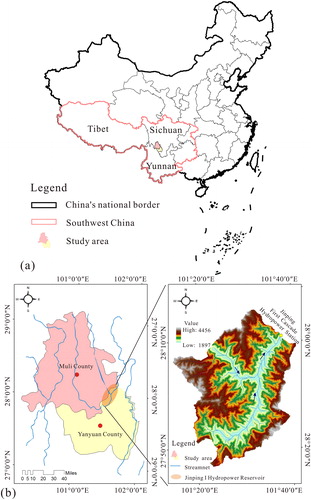
The reservoir of the Jinping I Hydropower Station is divided into 14 sections according to engineering geological conditions controlled by many factors such as lithology, bank structure type and valley topography. As shown in , four main reservoir sections and four branch sections have been selected as the study area. The main reservoir section is divided into four areas namely, the dam site, Aizi Gully, export of Xiaojin River and Biji. The branch reservoir section is divided into four areas as well namely, the export of Xiaojin River, Huang Jia Wu Ji, Nadi Gully, Yanjiao and Luofu.
2.1. Tectonic and lithology setting
The Jinping I Hydropower Station is located in a mountainous area where the slopes are quite steep and geological disasters occur frequently (Liu et al. Citation2010). Valleys in the reservoir area are deep and narrow, mostly in an asymmetrical ‘V’ shape (). The width of the river ranges from 50 to 200 m, and the riverbed longitudinal gradient is generally greater than 3‰. The primary faults in the reservoir area include the Woluo River fault (, No. 9), the Jinping Mountain fault (, No. 7), the Xiaojin River fault (, No. 8) and the Qianbo fault (, No. 15). The Jinping Mountain fault and the Xiaojin River fault are closer to the reservoir area. These faults generally run from the north of the Jinping (N20°–40°E) to the south of the Liewa Mountains (near east-west direction), showing an arc-shaped rupture zone with a south-east bulge. These faults dip from the northwest to the north, with the inclination angles of approximately 70°–85°. They are exposed in the form of arc-shaped nappe, and the inclination angle has decreased to 45°–70°. As shown in , metamorphic rocks are the main reservoir bank rock, which are distributed over the entire main reservoir and the branch of the reservoir area. Furthermore, the lithology of the reservoir area is mainly comprised of metamorphic sandstone, limestone and phyllite, which are prone to landslides. Marble is distributed only in the dam area and in the reservoir tail region, forming narrow deep valleys. Due to river sedimentation and aeolian deposits, Quaternary deposits are widely distributed in the banks of the Jinping I Hydropower reservoir, which have not been fully explored and mapped in the geological maps, because of their fragmented distribution.
2.2. Hydrology conditions
The upstream water level process has been divided from stage I to stage V, with four reservoir impoundment stages and one reservoir drawdown stage as shown in . The wet-dry season in the dam and reservoir area is distinct. The dry season starts from November to April with more sunshine, humidity and diurnal temperature differences. While the rainy season spans over the remaining period (May–October) of each year with concentrated rainfall, humid temperatures, less sunshine, humidity and small temperature difference. Rainfall in wet season accounts for approximately 90–95% of the annual rainfall. Due to the climate of the western Sichuan Plateau, the number of days with daily precipitation exceeding 0.1 mm from in wet season exceeds half of the total number of days per month. According to the data of the Muli Hydrological Station available from the China Meteorological Data Network (http://data.cma.cn/data/cdcindex/cid/6d1b5efbdcbf9a58.html), the rainfall conditions in the area where the Jinping I Reservoir is located are shown in . The accumulative rainfall values between stage I and stage II, stage II and stage III, stage III and stage IV, and stage IV and stage V are 323, 474.4, 474 and 341.5 mm.
3. Methods
In this study, five different datasets in the Jinping I Hydropower Reservoir were used: (i) Tectonic and lithology setting (obtained by a professional geological survey company). (ii) Upstream water level process (provided by Jinping I Hydropower Station Design Unit). (iii) Climate and rainfall pattern (available from the China Meteorological Data Network (http://data.cma.cn/)). (iv) On-site deep-seated landslides displacement monitoring data (provided by Jinping I construction unit). (v) Four high-resolution remote sensing data (2 × 2 m, Gaofen-1 satellite and Gaofen-2 satellite, provided by China Center for Resources Satellite Data and Application).
In addition, the methodology used in this research is presented in . These four images captured on 21 March 2012, 17 September 2013, 21 October 2014 and 23 October 2016, illustrate the overall behavior of the landslides at four important times, namely, before the impoundment, after the second stage impoundment, after the fourth stage impoundment and after the water level drop, respectively. Prior to the interpretation, geometric correction, radiation correction and color correction have been made to reduce (i) the sensor errors, (ii) the impact of satellite attitudes changes, (iii) the influence of the atmosphere on radiation and (iv) the effect of the terrain on radiation. Furthermore, through on-site investigation, the area, especially the type and location of the 31 deep-seated landslides (8 of them are located in the main section) and several bank collapses in the Jinping I Hydropower Reservoir were measured. Typical landslide patterns can be identified from the high-resolution remote sensing image data. Although all the landslides cannot be measured through on-site investigation due to the large reservoir area, the on-site investigation plays an important role in correcting the interpretation of landslides, thus increasing the interpretation accuracy. After the interpretation, overall evolution of the reservoir landslides is concluded by comparing landslide distribution maps of the four important times. Moreover, deformation monitoring of a deep-seated landslides is carried out by means of on-site displacement tester. Eventually, combined with the overall evolution of reservoir landslides, the evolution trend of various types of landslides and the response of deep landslides to water level changes, the spatial and temporal evolution of landslides in reservoir areas under water level fluctuations is obtained. This can be used as a reference to the operation of other water buildings.
4. Landslides interpretation
4.1. Landslides mapping
After geometric correction, radiation correction and color correction, landslides in the reservoir area of the Jinping I Hydropower Station were interpreted visually. Four interpreted landslide maps were overlaid on four high-resolution remote sensing images, as shown in . Landslide distribution varied among the eight reservoir sections and were mainly concentrated in Sections 1 and 2 before reservoir impoundment (as shown in . After reservoir water impoundment and multiple cycles of water level fluctuation, landslides were mainly distributed in Sections 2 and 5. These areas where the landslides were intensely distributed are observed to be of the same lithology, as shown in . Thus, the formation of reservoir landslides was closely related to the lithology of these areas where they were located. It is not observed that there exists a clear connection between distribution of landslides and tectonic faults in the studied area.
Figure 9. Interpreted maps of reservoir landslides at the Jinping I Hydropower Station: (a) interpreted map before impoundment; (b) interpreted map after stage II of reservoir impoundment; (c) interpreted map after stage IV of reservoir impoundment and (d) interpreted map after stage V of reservoir drawdown (China Center for Resources Satellite Data and Application, http://www.cresda.com/).
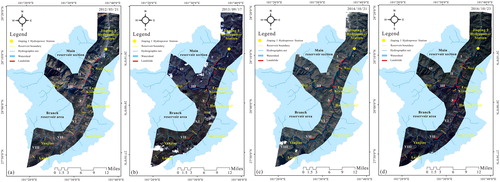
Figure 10. Spatial distribution of landslides in the Jinping I Hydropower Reservoir: (a) landslide projected area distribution in the Jinping I Hydropower Reservoir and (b) proportion of landslide types in the Jinping I Hydropower Reservoir. Source: Author
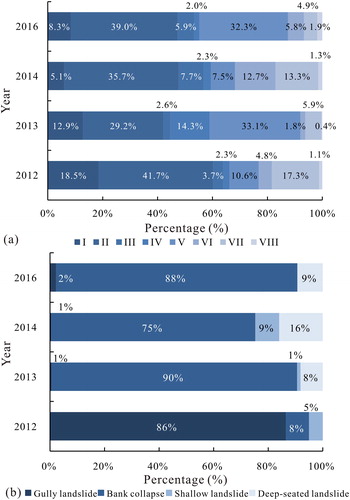
In addition to the distinctions in the types of landslides in different reservoir sections, the landslides also underwent significant changes at different times. The reservoir landslides all showed an increase trend in both landslide quantities and projected areas. To be more specific, the projected area of landslides had been interpreted by using the image taken on 21 March 2012 (), consisting of a great proportion of gully landslides, along with several bank collapses. After experiencing two stages of reservoir impoundments (17 September 2013), most of the landslides interpreted before stage I were submerged. Thus, new landslides took place and landslide density in several parts of the region became higher, as shown in . After stage III and stage IV (21 October 2014, ), the landslide projected area increased significantly, especially in reservoir Sections 2 and 5. The same phenomenon of increasing landslides area was also observed after reservoir drawdown (23 October 2016, . As shown in , the main type of landslides in the Jinping I Hydropower Reservoir changed from gully landslide to bank collapse after reservoir impoundment.
4.2. Evolution of total landslides
Landslides in the reservoir area were mainly near the reservoir water level. This was essentially consistent to the typical deformation and failure mode of landslides caused by the operation of reservoir water levels. Different from the growth trend of landslides in many other reservoir areas, the total amount of reservoir landslides of the Jinping I hydropower station decreased initially and increased thereafter (Chen Citation2005). As shown in , the total projected area of reservoir landslides was estimated to be 4.1 × 105 m2 before reservoir impoundment. After stages I and II, the projected area of reservoir landslides dropped to 2.7 × 105 m2, but the landslide quantity increased from 59 to 159 which was exactly the opposite of the tendency of the projected landslide change observed in other places. With the continuous rise of the reservoir water level, both total projected landslide area and landslide quantity showed increases but with different rates. The projected landslide area dramatically increased to 6.2 × 105 m2, while the landslide quantity slowly increased to 194. It meant that the reservoir landslide was considerably developing at this stage, and the rising water level is main triggering factor leading to the occurrence of reservoir landslides. In addition to reservoir impoundment, fluctuation of water level played another important role in triggering the instability of slopes at both sides of the reservoir and in increasing the likelihood of landslides of the reservoir area, especially for the case of rapid drawdown of water level. During this stage, the projected area of the landslides increased to 7.4 × 105 m2, but the landslide quantities decreased to 182 because of the collapses of the several deep-seated landslides.
4.3. Evolution of gully landslides
Gully landslides, which are directly linked to the reservoir, have also been interpreted. As shown in , the gully landslides declined sharply during both stages of reservoir impoundment and reservoir drawdown. Large quantity of gully landslides before reservoir impoundment was related partly to construction disturbance and partly to the Wenchuan earthquake that occurred on 12 May 2008. Quaternary deposits were well developed on the slope surface and the mountain gully in Jinping I reservoir, which were likely to be transported downstream or into the downstream river under heavy rainfalls, resulting in debris avalanches and gully landslides. Having been accumulated for 4 years after that strong earthquake and been disturbed by engineering construction constantly, the number of gully landslides increased to some extent. After reservoir impoundment, almost all of the gully landslides were submerged by the increased reservoir level, resulting in the sudden decrease of gully landslides. In addition, due to the natural growth of vegetation on both banks of the reservoir, the rock and soil of the bank slope were more stable, thus the gully landslides are less likely to occur. The generation rate of gully landslides fell below the rate of submergence and showed therefore a sharp decline.
4.4. Evolution of bank collapse
Large quantities of Bank collapses, as the main type of landslide, are widely distributed within the Jinping I Hydropower Reservoir. Through interpretation, both the total projected area and the quantity of bank collapse are found to increase sharply after the first two stages of reservoir impoundment (. Because the original bank collapses were submerged after reservoir impoundment, all bank collapses interpreted from the latest remote sensing image (17 September 2013) were new generated landslides. After stages III and IV, 145 bank collapses were interpreted, including 142 new generated landslides. The dramatic increase in bank collapses illustrated that the reservoir impoundment had a negative effect on slope stability. As the water level of the reservoir continues to rise, the previously collapsed banks remain to be submerged, while a large portion of banks are further emerged. After the reservoir drawdown, the collapses of bank slope with deep-seated slip surface became the most obvious phenomenon, and bank collapses with huge volume of failure mass have been interpreted.
4.5. Evolution of deep-seated landslides
In addition to bank collapse, deep-seated landslides can never be neglected due to their great threat to the operation of hydropower stations. Through remote sensing interpretation, two states (development and collapse) of deep-seated landslides in the reservoir area of Jinping I Hydropower Station have been distinguished. As shown in , 13 deep-seated landslides occurred after reservoir impoundment, which continued to slide/deform with continuously rising water level. After stage IV, a total amount of 31 deep-seated landslides were observed with the projected area increased to 3.7 × 105 m2. This illustrated that there was a direct relationship between the formation and development of deep-seated landslides and the rise of water levels. Meanwhile, a deep-seated landslide usually requires a long period of deformation process, and a disaster can only occur after a sufficient number of cycles of water level fluctuations.
5. Landslide dynamics and evolution
5.1. Overall landslide problems
The degree of development of landslides is affected by many factors, including the topography, geomorphology, stratigraphy lithology and water level fluctuations in the reservoir area (Gupta and Joshi Citation1990; Wu et al. Citation2014). Besides, human activities in the reservoir area, including traffic construction and manual disturbance, have additional impacts on the occurrence of landslides (Bozzano et al. Citation2011; Jaboyedoff et al. Citation2016). As shown in , the construction of Road #5 has destroyed the integrity of the mountainside, accelerating the sliding speed of the deep-seated landslide. The deformation rates of TP-2 and TP-5 measuring points on Road #5 are significantly larger than those measured at other points, especially during stages IV and V (as shown in ). At present, the Jinping I Hydropower Station has been in normal operation for 6 years. A large number of landslides have occurred with failure mass slipped into the reservoir area. Newly generated landslides will definitely occur, which will not be too large in terms of the volume of failure mass. Judging from the landslide interpretation, field investigation and comparison with the landslide hazards in other hydropower areas, the degree of landslide hazard in the Jinping I hydropower area is relatively high, although it has not yet reached a very serious level. The effective measures for disaster prevention and mitigation such as slope cutting, load reduction, anchoring, road rerouting and relocation of residents, should be taken and still deserve the attention of the relevant departments. If some projects are constructed on the upstream slope of river buildings, building in a low elevation area should be avoided whenever possible.
Figure 12. Influence of human activities on reservoir-induced landslides in the Jinping I Hydropower Station: (a) the position of large deformation bodies and the distribution of monitoring points on deformable bodies; (b) cracks on the Road 5# and (c) edge crack of deep-seated landslides. Source: Author
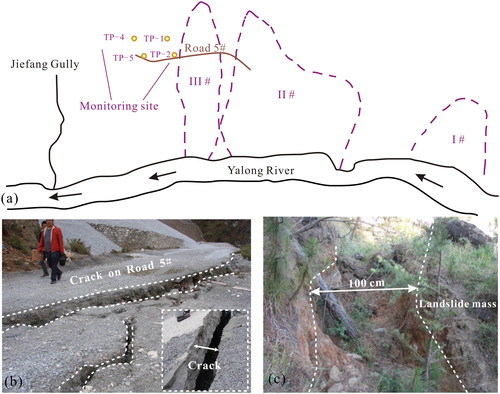
5.2. Landslide prone areas
Landslides are closely related to the topography and lithology and mainly occur within the ancient landslide bodies and slope coverings (Ghirotti Citation2006; Qi et al. Citation2006). The landslide-prone distribution map of the Jinping I Hydropower Reservoir is shown in . Through interpretation and site investigation, it is found that landslides are more likely to occur in Quaternary deposits because of their loose structure and extremely variable mechanical properties in response to water infiltration. Besides, landslides are mainly distributed in metamorphic sandstones and phyllite rocks, which are usually fractured and also sensitive to water. Due to the changes in the internal hydraulic conditions, the strength of the soils will be reduced, which makes it easier for landslides to occur in the Quaternary deposits under fluctuations in the water level. Furthermore, some bank collapses occurred in the rock slope with severe weathering and unloading, as well as the development of joint fissures due to a series of mechanical responses, and the changes of such rock mass occur under a fluctuating reservoir water level. According to previous studies and this research, the water will alter the behavior of the bank slope through at least two ways: (i) softening and decomposing the slope materials (including rock and soil) and (ii) causing an increase in pore water pressure. The drainage of water through the bank slope shows hysteresis, which is related to the poor permeability of rock mass such as metamorphic sandstones. During reservoir drawdown, the water in both bank slopes cannot be discharged in time, causing the hysteresis of the water level dissipation and increase in the hydraulic gradients inside slopes. This hydraulic response within the potential sliding mass will accelerate the deformation of landslides. In practice, quaternary deposits and some rocks that are fractured and sensitive to water should be under key surveillance to ensure the safety of river buildings.
5.3. Deformation failure mode
From the visual interpretation of the Jinping I reservoir, the main deformation and failure modes in the upstream area of river buildings are found to be bank collapses and large-scale deep-seated landslides, while the traction gradual destruction is the most common form of deep-seated landslides. During reservoir impoundment and fluctuation of water level, for some of the deep-seated landslides, the entire failure masses have basically slipped into the reservoir area, for the others, the trailing edges and two wing cracks were clearly observed. The traction type progressive destruction essentially undergoes several main processes, including water erosion, foot softening, high altitude fracture development, fracture development and landslide collapse. This observation is similar to the disaster pattern of most traction landslides and can be referred to other projects (Xu et al. Citation2009).
The deformation and failure mode of reservoir-induced landslides in the upstream area of river buildings is shown in . With the increase in water level, the rock and soil below the water level have been subjected to wetting for a long term, leading to an increase in pore water pressure and a decrease in shear strength. Furthermore, the flow erosion has constantly caused several local failures below the water level, triggering small bank collapses in the relatively upper part of the slopes (. As a result, free surfaces appear at the foot of the bank slope. In addition, human activities, such as the construction of roads, have caused the incompleteness of the mountain at high altitudes coupled with a lack of support from the mountain, leading to the appearance of tension cracks at the back of bank slopes (. Rainfall runoff, infiltration and accumulation occur alternatively or simultaneously under the condition of heavy rainfall. Rainfall runoff will wash away the deposits near the surface of the bank slopes. Moreover, rainfall infiltration can change the physical properties of slopes soils, particularly reducing the shear strength of rock and soil mass significantly. Most seriously, rainfall accumulation will cause the further expansion of cracks, leading to the slow sliding of deep-seated landslides (. However, a large portion of deep-seated landslides rarely collapse when the water level rises, because rising hydro-static water force acting at the lower part of the slope surface provides some resistance for the bank slopes. When the water level is drawn down rapidly, the decline in the water level inside the bank slopes lags that in the external water level. The slow dissipation of the excessive pore water pressure and infiltration-induced pore water pressure will simultaneously change the stress distribution of the slope and generate traction force, which act on the potential sliding body (. Thus, the stability of the bank slopes reduces substantially, and the catastrophic collapses are very likely to occur during this stage.
Figure 15. Typical deformation failure mode of the reservoir-induced landslides in the Jinping I hydropower reservoir. Source: Author
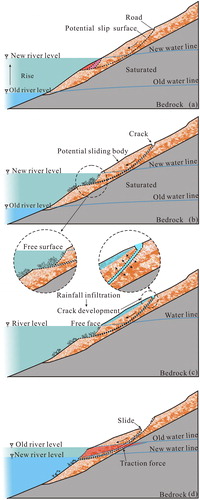
In this study, four remote sensing data representing four different times were used to interpret the temporal and spatial evolution of the landslide of the bank slope, from which the effect of the rainfall between two successive periods recorded to be around 400 mm is also deduced. However, the detailed impact of rainfall on bank slopes still deserves further studies.
5.4. Spatial-temporal distribution
Landslides in the upstream area of river buildings have been mainly affected by the manmade disturbance, the shock of earthquake and the fluctuation of water levels. Set the Jinping I Hydropower Reservoir as an example, a large number of gully landslides occurred before the reservoir impoundment, in the widely distributed Quaternary deposits, which were subjected to the manmade disturbance, occasional heavy rainfalls and seismic loadings of earthquake. With the rise of water level, lots of gully landslides have been submerged, resulting in a decline in landslides located above the water. Furthermore, natural regeneration and ecological recovery in this reservoir area may greatly reduce the frequency of secondary disasters, especially for the gully landslides. Therefore, it is necessary to maintain the ecological environment of the upstream area of river buildings.
During the rise of water level, the landslide on the upstream area of river buildings is mainly affected by fluctuations in water level. Taking Jinping level as an example, during stage I, a large number of earthquake-induced gully landslides were submerged. Along with the rising of water level, the pore-water pressure on both sides of the bank slopes showed a hysteretic increase, causing a low rate of landslides’ generation. The rate of newly generated landslides is not as fast as that of the submergence of the reservoir water level, resulting in a decrease in landslides. In addition, flow erosion and slope inundation due to rising water levels have led to a large number of small bank collapses and formation of free surfaces. In the meantime, the integrity of the mountain has been damaged due to human activities, the several cracks were found to form at high altitudes due to a lack of support at low place. However, the possibilities of landslides vary in different parts of the reservoir area, depending on the material structure. Landslides are more likely to occur in loose deposits or metamorphic sandstones where the water softening is more serious. Therefore, when constructing projects (such as roads, railways or houses) on the upper areas of river buildings, they should be as arranged at higher elevation as possible or built as underground buildings. In addition, it should be avoided, whenever possible, on rock masses with unfavorable engineering properties.
As the water level of the reservoir continues to rise, the pore water pressures increase inside the slopes, while the shear strength of the rocks and soils decreases, causing cracks and formation of deep-seated landslides. Furthermore, with the participation of rainfall, the cracks gradually expand, making deep-seated landslides easier to slide. Where the external pressures are the same as or greater than the internal pressures, the water pressure will make a beneficial contribution to the stability of the slope, rarely leading to the collapse of deep-seated landslides. However, such a balance will be broken by the fluctuations in the water level, especially under reservoir drawdown. During the sudden drop in water level, the degradation of rock and soil occurs. The traction force caused by the lagging water discharge inside the slope is the main reason for the landslide of the typical mode of traction in the reservoir area. Thus, rapid drawdown of water level will more easily to trigger landslides in the reservoir Therefore, in regulating the upstream water level, the upstream water level should be changed as slowly as possible, especially when the water level drops.
6. Conclusions
Taking Jinping I reservoir as an example, this study uses GIS to interpret and classify the temporal and spatial evolution of landslides in reservoir areas under water level changes, and to explore the effects of human activities, lithology settings, and water level change history on landslide evolution. Most of the conclusions obtained can also be applied to other engineering projects. The results are summarized as follows:
The landslide type in the upstream area of river buildings are mainly bank collapse, and most of them are close to the water surface in the reservoir.
Fluctuation of water level, occasional heavy rainfall and human activities (such as the construction of roads), jointly lead to the deep-seated landslides.
Flow erosion, foot softening, high-altitude crack generation, crack development and landslide collapse are the main processes for large-scale landslides.
The accumulation of reservoir impoundment will lead to the occurrence of bank collapse and the continuous development of deep landslides. The sudden reservoir water level drawdown may trigger the collapse of deep-seated landslides.
Landslides are more likely to occur in Quaternary deposits, fractured rock masses and water-softened rock mass, which are all sensitive to the water. Bank collapses are often observed in the Quaternary deposits and fractured rock masses (such as metamorphic rocks), but deep-seated landslides usually appear in water-softened rock mass (such as phyllite) and Quaternary deposits.
Disclosure statement
No potential conflict of interest was reported by the authors.
Additional information
Funding
References
- Benza M, Weeks JR, Stow DA, López-Carr D, Clarke KC. 2016. A pattern-based definition of urban context using remote sensing and GIS. Remote Sens Environ. 183:250–264.
- Bozzano F, Cipriani I, Mazzanti P, Prestininzi A. 2011. Displacement patterns of a landslide affected by human activities: insights from ground-based InSAR monitoring. Nat Hazards. 59(3):1377–1396.
- Bui DT, Tuan TA, Klempe H, Pradhan B, Revhaug I. 2016. Spatial prediction models for shallow landslide hazards: a comparative assessment of the efficacy of support vector machines, artificial neural networks, kernel logistic regression, and logistic model tree. Landslides 13(2):361–378.
- Chen J. 2005. On the distribution and mechanism of landslides in the Three Gorges reservoir area. J Eng Geol. 13(3):305–309. Chinese.
- Chen ML, Lv PF, Zhang SL, Chen XZ, Zhou JW. 2018. Time evolution and spatial accumulation of progressive failure for Xinhua slope in the Dagangshan Reservoir, Southwest China. Landslides 15(3):565–580.
- Chi C, Liu Z, Zhang J. 2008. Interpretation of landslide from SPOT-5 imageries in the Three Gorges reservoir area. International Workshop on Earth Observation and Remote Sensing Applications, IEEE. p. 1–5.
- Ghirotti M. 2006. Edoardo Semenza: the importance of geological and geomorphological factors in the identification of the ancient Vaiont landslide. In: Evans SG, Mugnozza GS, Strom A, Hermanns RL, editor. Landslides from Massive Rock Slope Failure. Dordrecht: Springer; p. 395–406.
- Gupta RP, Joshi BC. 1990. Landslide hazard zoning using the GIS approach—a case study from the Ramganga catchment, Himalayas. Eng Geol. 28(1–2):119–131.
- Henriques C, Zêzere JL, Marques F. 2015. The role of the lithological setting on the landslide pattern and distribution. Eng Geol. 189:17–31.
- Hong H, Ilia I, Tsangaratos P, Chen W, Xu C. 2017. A hybrid fuzzy weight of evidence method in landslide susceptibility analysis on the Wuyuan area, China. Geomorphology. 290:1–16.
- Hong H, Liu J, Bui DT, Pradhan B, Acharya TD, Pham BT, Zhu AX, Chen W, Ahmad BA. 2018. Landslide susceptibility mapping using J48 decision tree with AdaBoost, bagging and rotation Forest ensembles in the Guangchang area (China). Catena. 163:399–413.
- Hong H, Pradhan B, Sameen MI, Kalantar B, Zhu A, Chen W. 2018. Improving the accuracy of landslide susceptibility model using a novel region-partitioning approach. Landslides. 15(4):753–753.
- Hong H, Pourghasemi HR, Pourtaghi ZS. 2016. Landslide susceptibility assessment in Lianhua County (China): a comparison between a random forest data mining technique and bivariate and multivariate statistical models. Geomorphology. 259:105–118.
- Huang D, Gu DM. 2017. Influence of filling-drawdown cycles of the Three Gorges reservoir on deformation and failure behaviors of anaclinal rock slopes in the Wu Gorge. Geomorphology. 295:489–506.
- Huang D, Gu DM, Song YX, Cen DF, Zeng B. 2018. Towards a complete understanding of the triggering mechanism of a large reactivated landslide in the Three Gorges Reservoir. Eng Geol. 238:36–51.
- Ilia I, Tsangaratos P. 2016. Applying weight of evidence method and sensitivity analysis to produce a landslide susceptibility map. Landslides. 13(2):379–397.
- Jaboyedoff M, Michoud M, Derron MH, Voumard J, Leibundgut G, Sudmeier-Rieux K, Nadim F, Leroi E. 2016. Human-induced landslides: toward the analysis of anthropogenic changes of the slope environment. In: Avresa S, Cascini L, Picarelli L, Scavia C, editor. Landslides and engineering slopes—experiences, theory and practices. London: CRC Press; p. 217–232.
- Jia GW, Zhan TL, Chen YM, Fredlund DG. 2009. Performance of a large-scale slope model subjected to rising and lowering water levels. Eng Geol. 106(1–2):92–103.
- Jian W, Wang Z, Yin K. 2009. Mechanism of the Anlesi landslide in the Three Gorges reservoir, China. Eng Geol. 108(1–2):86–95.
- Lan H, Wu F, Yan F, Qi S. 2004. Mechanism of deep cracks in the left bank slope of Jinping first stage hydropower station. Eng Geol. 73(1):129–144.
- Li PY, Ba RJ, Ni HY, Wang DH. 2017. Simulation analysis of the influence of the reservoir water level fluctuation rate on the stability of Shuangjiaping accumulation landslide in Ya’an. J Geomech. 23(2):288–295. Chinese.
- Li Z, Li X, Wang Y, Ma A, Wang J. 2004. Land-use change analysis in Yulin prefecture, northwestern China using remote sensing and GIS. Int J Remote Sens. 25(24):5691–5703.
- Liu ZB, Xu WY, Jin HY, Liu DW. 2010. Study on warning criterion for rock slope on left bank of Jinping No. 1 Hydropower Station. J Hydraul Eng. 39(1):101–107.
- Ma J, Tang H, Hu X, Bobet A, Zhang M, Zhu T, Song Y, Ez Eldin MA. 2016. Identification of causal factors for the Majiagou landslide using modern data mining methods. Landslides. 14(1):1–12.
- Macfarlane DF. 2009. Observations and predictions of the behaviour of large, slow-moving landslides in Schist, Clyde dam reservoir, New Zealand. Eng Geol. 109(1–2):5–15.
- Moosavi V, Niazi Y. 2016. Development of hybrid wavelet packet-statistical models (wp-sm) for landslide susceptibility mapping. Landslides. 13(1):97–114.
- Palis E, Lebourg T, Tric E, Malet JP, Vidal M. 2017. Long-term monitoring of a large deep-seated landslide (La Clapiere, South-East French Alps): initial study. Landslides. 14(1):155–116.
- Paronuzzi P, Rigo E, Bolla A. 2013. Influence of filling–drawdown cycles of the Vajont reservoir on mt. toc slope stability. Geomorphology. 191(5):75–93.
- Petley DN, Mantovani F, Bulmer MH, Zannoni A. 2005. The use of surface monitoring data for the interpretation of landslide movement patterns. Geomorphology. 66(1–4):133–147.
- Qi S, Yan F, Wang S, Xu R. 2006. Characteristics, mechanism and development tendency of deformation of Maoping landslide after commission of Geheyan reservoir on the Qingjiang river, Hubei province, China. Eng Geol. 86(1):37–51.
- Song K, Yan E, Zhang G, Lu S, Yi Q. 2015. Effect of hydraulic properties of soil and fluctuation velocity of reservoir water on landslide stability. Environ Earth Sci. 74(6):5319–5329.
- Sun G, Zheng H, Huang Y. 2015. Stability analysis of statically indeterminate blocks in key block theory and application to rock slope in Jinping-I Hydropower Station. Eng Geol. 186:57–67.
- Uhlemann S, Smith A, Chambers J, Dixon N, Dijkstra T, Haslam E, Meldrum P, Merritt A, Gunn D, Mackay J. 2016. Assessment of ground-based monitoring techniques applied to landslide investigations. Geomorphology. 253:438–451.
- Wu X, Ren F, Niu R. 2014. Landslide susceptibility assessment using object mapping units, decision tree, and support vector machine models in the Three Gorges of China. Environ Earth Sci. 71(11):4725–4738.
- Xu Q, Tang M, Huang R. 2009. An evaluation study of bank collapse prediction in the Three Gorges Reservoir area. In: Wang F, Li T (Eds). The landslide disaster mitigation in Three Gorges reservoir, China. Berlin Heidelberg: Springer; p. 147–172.
- Yan ZL, Wang JJ, Chai HJ. 2010. Influence of water level fluctuation on phreatic line in silty soil model slope. Eng Geol. 113(1–4):90–98.
- Youssef AM, Pourghasemi HR, Pourtaghi ZS, Al-Katheeri MM. 2016. Landslide susceptibility mapping using random forest, boosted regression tree, classification and regression tree, and general linear models and comparison of their performance at Wadi Tayyah basin, Asir region, Saudi Arabia. Landslides. 13(5):839–856.
- Zhang SL, Zhu ZH, Qi SC, Hu YX, Du Q, Zhou JW. 2018. Deformation process and mechanism analyses for a planar sliding in the Mayanpo massive bedding rock slope at the Xiangjiaba Hydropower Station. Landslides. 15(10):2061–2073.
- Zhou JW, Xu FG, Yang XG, Yang YC, Lu PY. 2016. Comprehensive analyses of the initiation and landslide-generated wave processes of the 24 June 2015 Hongyanzi landslide at the Three Gorges Reservoir, China. Landslides. 13(3):589–601.