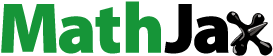
Abstract
Populations in coastal areas are vulnerable to chronic exposure to potentially toxic elements (PTEs) from aquatic ecosystems. In this study, we estimated the health risks from Cd, Cr, Fe, and Pb exposure in river water, sediments, and edible aquatic biota (Anadara granosa and Chanos chanos). Human daily intake was determined by an interview process with 287 respondents that were selected by proportional cluster random sampling. The cumulative probability value for total risk exceeded the acceptable limit value, indicating that adverse health impacts from PTE exposure may occur in the Pangkep coastal population. The sensitivity analysis showed that exposure duration and Cr concentrations are the factors with the greatest influence in a recreational exposure scenario, whereas the frequency of exposure to shellfish and milkfish is the most influential factor for health risks in a residential scenario. Thus, reducing Cr concentrations in river water, restricting swimming duration, and limiting the frequency of shellfish and milkfish consumption are required to mitigate health risks in the future.
Introduction
The presence of potentially toxic elements (PTEs) in aquatic ecosystems is harmful not only to aquatic organisms but also to terrestrial organisms, including humans. This problem remains an issue of major concern worldwide because of the toxicity of such elements and their ability to accumulate in biological tissues. Thus, the presence of PTEs poses a threat to food chains. Several PTEs such as As, Hg, Pb, and Cd can cause adverse health problems at very low concentrations (Rahman et al. Citation2012; Jaishankar et al. Citation2014; Azeh et al. Citation2019; Rovira and Domingo Citation2019). The most recent blood lead reference value (BLRV) proposed by the CDC (Citation2012a) is far lower than the previous blood lead level value (10 µg/dL). Moreover, the new BLRV value for children is 3.5 µg/dL, indicating that special actions must be implemented for children who have BLRV at or above the reference value to prevent further exposure and health impacts (CDC Citation2012b). Pb, Cd, and Cr are considered to be the most toxic substances in the substance priority list produced by ATSDR (Citation2019). Although Fe is considered to be an essential PTE because it plays a role in mitochondrial function and enzyme regulation, exposure to high Fe concentrations can cause protein deterioration and induce DNA alteration, thereby provoking cell death (González et al. Citation2017). Additionally, excess iron leads to the accumulation of reactive oxygen species, affects the transformation of genes that regulate hematopoiesis, and disrupts hematopoiesis in bone marrow (Isidori et al. Citation2018), which also causes hemochromatosis (disruption of five genes responsible for iron absorption, iron transportation, and an iron-sensing protein regulator) (Zhang et al. Citation2019).
Carcinogenic PTEs such as As, Cd, and Cr may disrupt DNA synthesis and repair mechanisms (Clancy et al. Citation2012; Koedrith et al. Citation2013). Additionally, Cd exposure is associated with bone and kidney damage, and Cd exposure can cause liver damage, gastrointestinal disorders, lung injuries, degenerative bone disease, prostatic proliferative lesion, hypertension, and bone fractures and can disrupt Zn and Cu metabolism (Satarug et al. Citation2010, Citation2018; Zeinali et al. Citation2019; Balali-Mood et al. Citation2021). Pb is a neurotoxic PTE that is associated with developmental and neurobehavioral effects, especially in fetuses and children (WHO Citation2007). Several studies have shown that Pb exposure promotes respiratory, urinary, hematological, neurological, neuropathy, and cardiovascular disorders through immune modulation, inflammatory, and oxidative mechanisms (Bernhoft Citation2012; Tsai et al. Citation2017; Balali-Mood et al. Citation2021; Das et al. Citation2021). Chronic exposure to Cr can induce various diseases such as dermal, renal, neurological, and gastrointestinal system disorders as well as cancers (lung, larynx, kidney, testicles, thyroid, and bladder) (Fang et al. Citation2014).
The Pangkajene river is located in the Pangkajene dan kepulauan (Pangkep) regency in South Sulawesi Province, Indonesia. It flows from the east to the west part of the city across the karst hills and ends in the Makassar strait. This river is used as a source of freshwater for many human activities such as irrigation, industrial and aquaculture/fish farming activities, navigation, and domestic activities. Its location near the site of various human activities that can potentially pollute the river ecosystem has caused the water quality of the Pangkajene river to decrease over recent years, which may also influence the nearby coastal areas that receive water containing run-off from the land. PTEs and other pollutants may be carried by river water to coastal areas, where they can disrupt normal aquatic activity, and most PTEs can cause serious threats to communities that use contaminated water for drinking, irrigation, and recreation purposes. Additionally, populations in coastal areas are more vulnerable to PTE exposure through dietary intake, especially from aquatic organisms such as shellfish, milkfish, fish, and shrimp, which are sources of nutritious food. Milkfish (Chanos chanos) and shellfish (Anadara granosa) are often consumed by Pangkep communities and are important sources of protein. Thus, the coastal community experiences greater potential exposure to PTEs through multiple environmental pathways, such as water and sediment while swimming, and/or the consumption of aquatic animals such as shellfish and milkfish taken from river water.
Human health risk assessments (HHRA) have been widely used by several researchers to assess potential adverse health effects that may occur in the future because of chronic exposure to trace elements from the aquatic environment (Yi et al. Citation2017; Ezugwu et al. Citation2019; Gao et al. Citation2019; Mgbenu and Egbueri Citation2019; Ukah et al. Citation2019; Egbueri Citation2020; Egbueri and Mgbenu Citation2020; Onyemesili et al. Citation2020; Astuti et al. Citation2021a; Enyigwe et al. Citation2021; Karmakar et al. Citation2021; Rauf et al. Citation2021b). Those previous studies revealed that many sources of PTE contamination exist in aquatic settings, such as mining; agricultural, domestic, and industrial activities; and geogenic sources. However, very little research has evaluated the health risks to coastal communities related to PTE contamination in the study area investigated here. Previous HHRA studies only assessed exposure to one particular metal (such as As or Cr) (Aripai et al. Citation2012; Bahar and Daud Citation2012). However, PTEs have the potential to cause health problems in the form of combined elements through exposure to multiple mediums and pathways. The complex health risks due to combinations of PTEs in coastal communities have not yet been assessed. Moreover, a prior study only estimated health risks using reference/standard values from the U.S. Environmental Protection Agency (USEPA) and other works of literature. They did not calculate risks on the basis of the real dietary intakes and exposure patterns of the local communities. Failure to address these factors can cause bias in health risk assessments. This is the first study that has calculated risks from a combination of PTEs and used the actual exposure patterns of the Pangkep community.
High uncertainties may be involved in conducting risk estimations. The uncertainty may originate from variation in environmental conditions, insufficient knowledge of the actual value of certain variables, and characteristics of the population (Sanaei et al. Citation2021). Previous studies of human health risks have not dealt with the uncertainty that may be present. Moreover, several studies use the standard value of human exposure patterns from USEPA to calculate risks, which may not be appropriate for the inhabitants of the study area. This can cause policy making designed to reduce health risks from environmental PTE exposure to be incompatible with the existing conditions in the region. Thus, a comprehensive estimation incorporating uncertainties associated with human health risk assessments is needed to more convincingly determine the possibility of further impacts from PTE exposure on human health.
Monte Carlo simulation (MCS) is a mathematical and logical model that has been widely used to assess uncertainty in health risk assessment models by collecting limited data to comprehensively predict various situations (Xiao et al. Citation2018; Sanaei et al. Citation2021). MCS can be applied to calculate uncertainties and conduct sensitivity analyses of random variables in human health risk assessments (Qu et al. Citation2012). This model was used by USEPA as the basis to consider health risk policy. Thus, to adequately consider the uncertainty that may be present, we used MCS to estimate health risks. The aims of this study are Equation(1)(1)
(1) to evaluate potential noncarcinogenic and carcinogenic risks due to PTE exposure through multiple exposure pathways and Equation(2)
(2)
(2) to analyze uncertainties and the sensitivity of risk factors in the health risk assessment. Assessment of PTEs in coastal settings is of great importance. The results of this study may provide valuable information for aquatic ecosystem protection, assurance of food safety, and preservation of human health in the future.
Methods
Study area descriptions
The Pangkajene dan kepulauan (Pangkep) regency is a suburban area located in the north of Makassar city, South Sulawesi, Indonesia within latitudes of 04°40′–08°00′ S and longitudes of 110°–119°48′67″ E (BPS Citation2021). presents a map of sampling sites. The Pangkep regency is approximately 51 km from the provincial capital of South Sulawesi, with a total area of 12,362.73 km2 (11,464.44 km2 of sea and 898.29 km2 of land/terrestrial area). This area lies within the Maros Pangkep karst national park, which is an area of magnificent karst features in Indonesia with spectacular karst tower outcrops. Geologically, the late Eocene- to middle Miocene-age Tonasa limestone formation is the main geologic formation in this area, which comprises solid limestone, coral, wackestones, calcarenite, calcirudite, and bioclastic (Wilson Citation1996; Wilson and Bosence Citation1997; Ahmad and Hamzah Citation2016; Nuhung Citation2016) deposits. The uppermost lithologies in the formation comprise packstones, wackestones, and grainstones. The carbonate outcrops in the Tonasa formation were formed by large benthic foraminifera and sometimes by coralline algae (Wilson Citation1996; Wilson and Bosence Citation1997). The carbonate platform in Sulawesi is part of the Australian margin that experienced rifting beginning in the Jurassic period and is one of the main hydrocarbon reservoirs in Southeast Asia (Wilson Citation1996; Polvé et al. Citation1997; Wilson and Bosence Citation1997). Carbonate platform outcrops in the Pangkajene area are characterized by vertical tower karst features in coastal areas (Wilson and Bosence Citation1997). A report by UNESCO (Citation2019) stated that the Maros–Pangkep geopark is underlain by the Bantimala Melange tectonic complex that comprises low-to-ultrahigh-grade metamorphic rocks, tectonic blocks of oceanic crust, and marine sedimentary rocks. Previous studies showed there are environmental degradations in the Maros Pangkep karst area which may harmful to the ecological system and human health due to cement production and limestone mining activities (WALHI Citation2018; Mallongi et al. Citation2019; Astuti et al. Citation2021a; Rauf et al. Citation2021a, Citation2021c; Mallongi et al. Citation2022; Rauf et al. Citation2022).
Figure 1. Map of sampling site (S for water and sediment of river; C for milkfish sampling; and A for shellfish sampling). Source: Author.
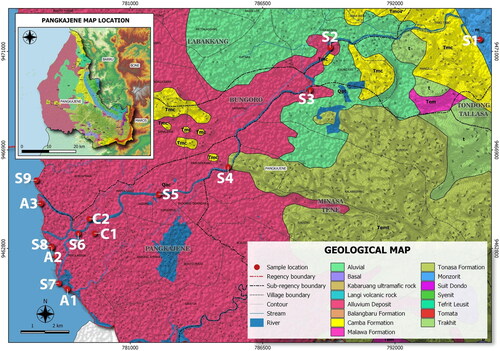
Hydrologically, this karst area has a high-capacity aquifer (100–500 L/s) (Nuhung Citation2016) that is dominated by subsurface drainage. Rainwater that falls on the limestone surface can pass through Dolina and Ponor and/or immediately infiltrate into rock cavities (Nuhung Citation2016). The aquifers and subsurface drainages in this area act as the catchment area for many rivers, including the Pangkajene, Pute, and Bantimurung rivers. The mainland is inhabited by a population of 345,775 (in 2020) dominated by people of Bugis–Makassar ethnicity who work in the livestock, agriculture, fishery, industry, mining, and tourism sectors (BPS Citation2021). The climate is characterized as a tropical monsoon climate (Astuti et al. Citation2021b): in 2020, the highest air temperatures occurred in October at 28.3 °C and the lowest air temperature occurred in March at 26.9 °C (BPS Citation2021). The amount of precipitation and rainy days in the Pangkep regency range from 8 to 966 mm/months and 3 to 27 days, respectively, in 2020 (BPS Citation2021). The soil in the Pangkep regency is highly fertile and is used to produce various crops and agricultural commodities such as rice, chili, shallots, garlic, spinach, watermelon, pomelo, mango, coconut, and medicinal plants (BPS Citation2021). In the fishery sector, milkfish is one of the main commodities in the Pangkep regency. Marble and limestone are the main mining resources in this area, although several minerals and other minable materials exist in the Pangkep regency, such as kaolin, basalt, coal, propylite, marble, clay/loam, limestone, feldspar, alluvial gold, gems, gravel, and radioactive minerals (Depdikbud Citation1976; DPU Citation2017; Astuti et al. Citation2021b, Citation2021c; Mallongi et al. Citation2022).
Sampling methods
This study was conducted in the Pangkajene dan Kepulauan (Pangkep) regency in South Sulawesi Province, Indonesia. The sampling was done in November 2020 and June 2021. A total of 18 samples of river water and 18 samples of river sediment, three samples of shellfish (Anadara granosa), and four samples of milkfish (Chanos chanos) were collected. The shellfish were taken from three sampling sites on the coast and they represent the biota most frequently consumed by residents. Additionally, we collected edible organisms (shellfish and milkfish) from coastal areas. Shellfish and milkfish make up the main foodstuffs in the Pangkep regency because they are cheap and abundantly available in that area. More broadly, milkfish is a major commodity in the Pangkep regency. Milkfish samples were taken from the two largest aquaculture fields in a downstream area of the Pangkajene river. River water and river sediment were collected twice, once in November 2020 (wet season) and once in June 2021 (dry season). Shellfish and milkfish were collected only once, during the month of harvest in June 2021.
The water samples were placed in high-density polyethylene (HDPE) bottles (1L). To sterilize equipment and prevent contamination, pre-cleaned bottle was prepared. The riverine water was collected using a water sampler from 10 to 30 cm below the water surface, whereas sediments were taken by Ekman grab from the sediment surface (10–30 cm depth). Approximately 500 g of sediment, 350 g of milkfish, and 500 g of shellfish samples were stored in clean polyethylene plastic, after which samples were stored in a cooler box with ice and directly transported to a laboratory.
Sediment analysis
All sediment samples were separated from rocks, grass, and other litter, and then oven-dried at 40 °C. All dried samples were crushed into powder and sieved through a 0.5-mm-mesh sieve. The samples were then ground by a blender into a fine powder and sieved using a 0.5-mm-mesh sieve. Afterward, 0.5 g of sediment was added to a solution of 5 mL 65% HNO3 and 1 mL 60% HClO4. This method complies with the established acid digestion method outlined by APHA (Citation1999). Adding the HNO3 and HClO4 solution is necessary to digest silicate minerals or difficult-to-oxidize organic matter (OM) (APHA Citation1999). This method is also used as the established heavy-metal analysis protocol in Indonesia (Departemen Pertanian RI Citation2009). The digested samples were heated on a hot plate at 200 °C until they formed a clear ±3 mL solution. One milliliter of the clear solution was added to 25 mL distilled water, filtered, and homogenized.
Water sample analysis
A 5 mL water sample was added to 5 mL HNO3 and 1 mL HClO4. The acid digestion method was performed according to the APHA (Citation1999) protocol and Indonesia’s standard for heavy metal analysis by Departemen Pertanian RI (Citation2009). The solution was then heated on a hot plate at 200 °C until 1–3 mL of clear solution was formed. Then, 25 mL of distilled water was added to 1 mL of the clear solution.
Anadara granosa and Chanos chanos analysis
The flesh of Anadara granosa and Chanos chanos was ground using a blender, and 5 g of the ground flesh was added to a solution of 10 mL of 65% HNO3 and 2 mL 60% HClO4 and left overnight. Acid digestion was conducted according to the APHA (Citation1999) protocol and Indonesia’s standard for heavy metal analysis by Departemen Pertanian RI (Citation2009). The digested solutions were stirred and heated at 200 °C until a clear solution formed. After digestion, 1 mL of the clear solution was added to 25 mL of distilled water, filtered, and homogenized. Cd, Cr, Fe, and Pb concentrations were conducted using an AAS Thermo scientific iCE 3000 series instrument with wavelength absorption at 248.3 (Fe), 283.3 (Pb), 228.8 (Cd), and 357.9 (Cr).
Quality assurance
For accurate analysis, blanks and sample duplicates were included in the PTE analytical process. To sterilize equipment and prevent contamination, the glassware was properly precleaned by soaking in 6% HCl for at least 24 h and then rinsing three times with double-distilled water and oven-drying at a temperature of 105 °C. Analytical blanks were analyzed similarly as for sample analysis but without samples. The percent recovery achieved for each PTE was >90% with linear calibration curves (R2 of 0.999).
Estimation of human health risk
Two exposure scenarios were considered in this study: Equation(1)(1)
(1) a recreational scenario, which results from incidental ingestion and dermal contact when swimming in the Pangkajene river, and Equation(2)
(2)
(2) a residential scenario that arises from the consumption of shellfish and milkfish (Supporting Information ). When people are in recreational activities such as swimming or fishing, there is a potential for pollutant exposure through dermal contact with water and sediment (USEPA Citation2021a). They are also potentially exposed by PTE through incidental ingestion of water or sediment (USEPA Citation2015, Citation2021a; Canpolat et al. Citation2020). Due to the Pangkep population does not use the river water as the main drinking water resource, thus, PTEs exposures from river water and sediment were only included in the recreational exposure scenarios. Whereas, the health risks from shellfish and milkfish consumption were included in the residential exposure scenario.
The hazard quotient (HQ) and carcinogenic risk (CR) values were used in the present study to estimate the noncarcinogenic (Cd, Pb, Cr, and Fe) and carcinogenic (Pb, Cd, and Cr) risks, respectively, due to PTE exposure. Noncarcinogenic and carcinogenic health risks in the recreational exposure scenario were calculated using EquationEqs. (1)–(14) (USEPA Citation1989, Citation2011, Citation2015, Citation2021b, Citation2021c; ATSDR Citation2005; Shakeri et al. Citation2020):
Noncarcinogenic risks in the recreational exposure scenario:
(1)
(1)
(2)
(2)
(3)
(3)
(4)
(4)
(5)
(5)
(6)
(6)
(7)
(7)
Reference dose (RfD) and cancer slope factor (CSF) for dermal exposure were presented in Eqs. (5) and Equation(12)(12)
(12) (RAIS Citation2020). If Hazard quotient (HQ), Hazard Index(HI), and Total Hazard Index (THI) value >1 indicates that the adverse health consequences from PTEs exposure are likely to occur (USEPA Citation1989, Citation2011, Citation2015, Citation2021b, Citation2021c; ATSDR Citation2005; Shakeri et al. Citation2020). If the total carcinogenic risk (TCR), (Carcinogenic Risk of multiple PTEs (CRm), and Carcinogenic risk (CR) value exceed 1 × 10−04 indicates that the cancer is likely to occur due to the chronic exposure or the risk was unacceptable (USEPA Citation1991, Citation2005).
Carcinogenic risks in the recreational exposure scenario:
(8)
(8)
(9)
(9)
(10)
(10)
(11)
(11)
(12)
(12)
(13)
(13)
(14)
(14)
In dermal exposure, body surface area was calculated using follows equation EquationEq. (15)(15)
(15) (Reading and Freeman Citation2005):
(15)
(15)
where SA is the body surface area (m2), BW is the body weight (Kg), H represents body height (m). Then, SA is multiplied by 10,000 to convert in cm2.
In residential exposure scenario, noncarcinogenic and carcinogenic health risk were estimated using EquationEqs. (16)–(21) (USEPA Citation1989, Citation2015, Citation2021b, Citation2021d):
Noncarcinogenic risks in the residential exposure scenario:
(16)
(16)
(17)
(17)
(18)
(18)
Carcinogenic risks in the residential exposure scenario:
(19)
(19)
(20)
(20)
(21)
(21)
All abbreviations, units, and values related to the risk assessment (THQ, HI, CR, CRm, TCR) equations were expressed in .
Table 1. Symbol, definitions, units, and values related to health risk assessment.
Human data collection
Human data were obtained by interviewing 287 individuals, including adults and children, to obtain daily exposure pattern data used in the risk assessment metrics (ingestion rate (IR), body weight (BW), exposure frequency (EF), exposure duration (ED), surface area (SA), averaging time (AT), exposure time (ET), and exposure event (EV)). Data for this study were collected using proportional cluster random sampling from two village populations (Mappasaile and Tekolabbua villages).
Uncertainty and sensitivity analysis
The uncertainty and sensitivity analysis was conducted using Crystal Ball 11.1.2 (Oracle Inc Software, USA) in a Microsoft Excel (2019 version) environment. After estimating the HQ and CR, the MCS technique was run with 10,000 iterations to calculate THI and TCR, whereas considering the distribution of independent health risk variables in the study area (IR, BW, EF, ED, AT, ET, and EV) that were directly obtained from the interview process. A final human health risk result for each metal was produced by comparing the lower bound uncertainty (the 5th percentile) and the upper bound uncertainty (the 95th percentile) with the permissible value (<1 for HQ and <1 × 10−06 for CR). In this study, the estimated values for HQ, CR, THI, and TCR are presented for the 5% and 95% percentiles. A sensitivity analysis was used to determine the most influential factor in the health risk estimations (Qu et al. Citation2018; Tajdar-Oranj et al. Citation2018). A high correlation coefficient indicates a greater influence on the final health risk calculation. Using USEPA human health risk assessment methods with MCS provides a probability distribution of HQ and CR values to estimate the uncertainty related to human health risks due to PTE exposure from various pathways (Qu et al. Citation2018).
Statistical analysis
IBM SPSS version 24 (SPSS Inc, USA) software was performed for statistical analysis. This study presented a parametric test result (at a significant level >.05). The statistical analysis was used to assess descriptive analysis (mean, standard deviation, minimum and maximum value). Then, the uncertainty and sensitivity tests were analyzed by Crystal Ball software (version 11.1.2).
Results and discussion
PTEs concentrations in water, sediment, and aquatic biotas
and provide the concentration of selected PTEs (Cd, Cr, Fe, and Pb) in water, sediments, shellfish, and milkfish. The highest mean concentration in river water was found for Cr (1.64 mg/L), followed by Fe (1.31 mg/L), Pb (0.79 mg/L), and Cd (0.42 mg/L). According to , the mean concentration of PTEs in river water exceeded the water quality standards set by the WHO, USEPA, and the Indonesian standard, indicating that the water was highly polluted by PTEs and unacceptable as drinking water. Compared with world average concentrations, the PTE concentrations in the Pangkajene river were very high. Compared with prior studies by Aripai et al. (Citation2012) and Salamahu (Citation2012), the Pb and Cd concentrations in river water were higher than in previous studies, indicating that actions taken to control PTEs have not been fully successful in recent years. Cr and Pb concentrations in the Pangkajene river were lower than a river in India studied by Pandion et al. (Citation2022); however, the Cd concentration in the Pangkajene river was higher than in the Indian river. High concentrations of PTEs may be influenced by a surplus amount of rainwater to the river system during wet season (Pandion et al. Citation2022). Aside from the factor of excess rainwater, anthropogenic factors such as untreated sewage disposal and direct industrial discharge to the river, as well as natural activities like underwater currents and sediment movement, may elevate PTE concentrations in the water column (Najmeddin et al. Citation2018; Pandion et al. Citation2022). Many anthropogenic activities take place in the area surrounding the Pangkajene river, such as limestone and marble mining; cement production; and domestic, agricultural, and fishery activities (Mallongi et al. Citation2022). Additionally, wastewater treatment remains rarely practiced in the Pangkep regency. These conditions may influence PTE accumulation in the Pangkajene river.
Table 2. Comparison of measured selected PTEs in River Water (RW) and River sediment (RS) with environmental quality criteria and world average concentrations.
Table 3. Comparison of mean selected PTEs in shellfish (Anadara granosa), milkfish (Chanos chanos) with food quality criteria.
The mean concentration of Cd, Cr, Fe, and Pb in sediments of the Pangkajene river followed the order: Fe > Cr > Pb > Cd. The Cd concentration was lower than the detection limit in the sediment column, indicating that Cd mainly occurs in the aqueous phase in the river ecosystem and is readily available to aquatic organisms. This finding is inconsistent with a study by Maddusa and Asrifuddin (Citation2008), which reported higher Cd concentrations in sediment than in the water. This condition may be influenced by the chemical properties of Cd, which is known as a labile PTE that migrates rapidly within the environment (Jiang et al. Citation2013). Several studies have demonstrated that Cd is easily transported into water is highly bioavailable to aquatic organisms (Jiang et al. Citation2013; Liu et al. Citation2019). Cd, Cr, and Pb concentrations in sediment were lower than the probable effect level (PEL) value (). When PTE concentrations in sediments are lower than the PEL, adverse effects on benthic organisms will be infrequent (Kuang et al. Citation2021).
Fe in sediment was higher than the PEL value, indicating that harmful effects may be expected to occur frequently in sediment-dwelling benthic organisms. High concentrations of PTEs in the sediment may result from the deposition of suspended materials containing PTEs and downstream water movement (Yi et al. Citation2011). The concentration of Pb in this study was higher than that in prior studies (Salamahu Citation2012; Fitriani et al. Citation2013, Citation2014; Ali Citation2017), and the Fe concentration in this study was also higher than that reported in an earlier study by Amina (Citation2012). A study by Paena et al. (Citation2015) reported that sediment in the Pangkajene river contained a high concentration of OM and phosphate, which might influence the accumulation of PTEs in the river ecosystem. Compared with results reported by Yenny et al. (Citation2020), Pb and Cr concentrations in the Pangkajene river sediment were higher than those in the Citarum river. As the Citarum river is among the most polluted rivers in Indonesia, these findings demonstrate an alarming state of contamination in the Pangkajene river.
lists the mean PTE concentrations in shellfish (Anadara granosa) and milkfish (Chanos chanos) from the Pangkajene river. Based on the mean concentrations, the order of PTEs in shellfish samples was Fe > Cr > Cd > Pb, and the order in milkfish samples was Fe > Cr > Pb > Cd. The Cd concentration in shellfish in this study was higher than that reported in prior studies by Maddusa and Asrifuddin (Citation2008) and Haspullah et al. (Citation2018). Pb and Cr concentrations in shellfish in the present study are also higher than those in shellfish studied by Haspullah et al. (Citation2018). This result reveals that Cd, Cr, and Pb concentrations in Anadara granosa in the years of this study are higher than in the prior studies, indicating that PTE pollution in the Pangkajene river is becoming increasingly severe in recent years.
In comparison with other studies, Fe and Pb concentrations in shellfish from the Pangkajene river were higher than in shellfish from the Java sea and Madura straits (Yona et al. Citation2020, Citation2021). Cd and Cr concentrations in shellfish studied here were higher than those in a study by Soegianto et al. (Citation2020), whereas Cd and Pb concentrations in milkfish were lower than those in previous studies (Syarif Citation2015; Zulfiah et al. Citation2017; Haeriah Citation2019). A study by Awaluddin et al. (Citation2020) showed that pond water and sediments in the Pangkep regency had high Pb concentrations of 0.0182 mg/L and 25.210 mg/kg, respectively. A high concentration of Pb in pond water and sediment may influence the PTE concentration in milkfish (Awaluddin et al. Citation2020).
The concentrations of PTEs in shellfish and milkfish shown in indicate that all PTE concentrations in shellfish and milkfish were lower than the food quality criteria values set by EFSA, except for Fe. Aquatic organisms such as fish regulate their intake of trace elements from water via intake of suspended foods and ion transfer through lipophilic membranes (Yi et al. Citation2011), whereas bottom-feeder organisms like Anadara granosa take up nutrients and contaminants from sediments (Mrozińska and Bąkowska Citation2020; Soegianto et al. Citation2020). Hence, the high concentration of PTEs in sediments and water contribute to bioaccumulation in aquatic organisms.
Point estimate of human health risk
reports the exposure pattern of Pangkajene communities, with noncarcinogenic indicator values shown in . All HQ values were below 1 in both exposure scenarios, except for the HQingestion of Cr in the residential exposure scenario. This indicates that chronic exposure to Cr through milkfish and shellfish consumption can potentially induce adverse effects and noncarcinogenic risks to humans in the future (HQ > 1). This result is inconsistent with other studies (Bandara and Pathiratne Citation2019; Mwakalapa et al. Citation2019; Pandion et al. Citation2022) that found HQ values lower than 1. Based on the HI value in the recreational scenario, PTE (Cd, Cr, Fe, and Pb) exposure from both ingestion and dermal routes was safe from noncarcinogenic health effects. This result is consistent with the findings of several other studies (Alidadi et al. Citation2019; Canpolat et al. Citation2020; Shakeri et al. Citation2020). Based on the study by Paustenbach et al. (Citation2003), dermal exposure poses an insignificant health risk due to the minor amount of Cr that can penetrate deep skin layers. Another study stated that speciation of PTEs plays a role in health risk estimations because it influences the bioavailability, water-soluble properties, and the mobile fraction of PTEs in the human body (Sah et al. Citation2017). For example, Cr(VI) is more toxic than Cr(III), because it can be absorbed into deep skin layers (Paustenbach et al. Citation2003; Alidadi et al. Citation2019). Thus, dermal contact may make a small contribution to total health risks. Additionally, a study by Tong et al. (Citation2021) demonstrated that ingestion is the primary exposure route of PTEs in an aquatic system because the values of HQingestion of PTEs were higher than those of HQdermal for both children and adults.
Table 4. Human exposure characteristics in the coastal community of Pangkep regency, Indonesia.
Table 5. Noncarcinogenic risk of PTEs exposure in the Pangkajene’s coastal community (N = 287).
Generally, humans that are exposed to more than one PTE are vulnerable to adverse health impacts (Pandion et al. Citation2022). Therefore, a cumulative risk assessment (HI and THI) must be conducted to determine the cumulative effects of PTEs. The order of HIingestion values decreased as shellfish > milkfish > river water > river sediment, whereas HIdermal values due to river water exposure were higher than those of HIdermal values from river sediment exposure. In the recreational exposure scenario, the THI value was greater than 1, suggesting that adverse health effects may potentially occur in people who swim in the Pangkajene river due to incidental ingestion of and dermal contact with river water and sediments containing PTEs. The HQ, HI, and THI values in children are higher than in adults (), which indicates that children are more at risk than adults. This result is similar to that of another study by Han et al. (Citation2021). This result is also supported by several reports mentioning that children are more susceptible to exposure to water containing PTEs than adults (Gao et al. Citation2019; Xiao et al. Citation2019; Canpolat et al. Citation2020; Tong et al. Citation2021). This difference may be caused by their physiological immaturity, as well as their unprotected behavior (Creel Citation2002; Bose-O'Reilly et al. Citation2010; Al osman et al. Citation2019; Karatela et al. Citation2020). Furthermore, the exposure of children to pollutants is greater in terms of proportional body weight, and enduring long-term adverse effects may result from early exposure (Creel Citation2002; Sly and Carpenter Citation2012). Different metabolic rates in children also increase their susceptibility to illness; moreover, exposure to some chemicals at a young age when organs are still growing and developing appears to be more harmful than at older ages (Etzel Citation2020).
The carcinogenic risk indicators are presented in . In the recreational exposure scenario, the concentration of Cd in river water significantly contributed to carcinogenic risk (CR > 1 × 10−04). Like the noncarcinogenic risk, the contribution of river sediment exposure to carcinogenic risk is small. This result is consistent with the findings of several other studies (Wojciechowska et al. Citation2019; Yang et al. Citation2019; Ustaoğlu et al. Citation2020). Based on the CRm value, exposure to river water through incidental ingestion and dermal contact is not safe (CRm > 1 × 10−04). This finding is contrary to that of a previous study by Canpolat et al. (Citation2020), which stated that there is no carcinogenic risk of PTE exposure through recreation activities. Conversely, exposure to river sediment is still deemed safe (CRm < 1 × 10−06). The CR, CRm, and TCR values tend to be higher in adults than in children. As demonstrated in a prior study (Tong et al. Citation2021), this trend illustrates that adults are more susceptible to carcinogenic effects of PTE exposure from water systems.
Table 6. Carcinogenic risk of PTEs exposure in the Pangkajene’s coastal community (N = 287).
In the residential exposure scenario, the order of CRshellfish decreased as Cd > Cr > Pb, whereas the order of CRmilkfish decreased as Cr > Pb > Cd. Based on the CRm value, the consumption of shellfish and milkfish is not safe because it is potentially associated with carcinogenic effects in humans (CRm > 1 × 10−04). Thus, action to reduce PTE concentrations in the river ecosystem is required immediately. The TCR values in the recreational and residential scenarios indicate that adults have a higher carcinogenic risk than children. These findings further support the idea that cancer vulnerability increases in midlife and at older ages (White et al. Citation2014). Cancer development is a complex process; nevertheless, the life course approach can be used to investigate the influence of prenatal and early life exposure on cancer in adults (White et al. Citation2014; Bhatt Citation2019). Age can reflect the ED and accumulation of CR factors; additionally, a multifactor process of CR may influence changes in normal cell processes that lead to cancer, including DNA damage accumulation and mutation over time.
Probabilistic risk assessment
Using a probabilistic risk approach based on MCS, the mean, 5th, and 95th percentile values of the THI and TCR distributions for different population groups are shown in . In the recreational exposure scenario, the mean THI values estimated by the probabilistic approach for adults and children were 1.81 and 1.75, respectively (), whereas in the residential scenario, the mean THI values calculated using MCS were 3.05 (adult) and 3.97 (children). Based on those values, both children and adults were at risk of noncarcinogenic health problems related to PTE exposure (THI > 1).
The THI values for adults and children in either exposure scenario were greater than 1 at the 95th percentile, indicating that 95% of the different age group populations experienced noncarcinogenic risk from multiple exposure pathways and that noncarcinogenic risk may not have occurred in 5% of the population (Sanaei et al. Citation2021). For noncarcinogenic risk, the sensitivity test results () revealed that the exposure frequency to milkfish (EF-MF) is the main factor influencing noncarcinogenic risk for adults in the residential scenario, with a contribution of 31.00%. Exposure frequency to shellfish (EF-SF) was the main factor in total noncarcinogenic risk in children, with a contribution of 30.90%. In the recreational exposure scenario, ED as the main factor related to noncarcinogenic risk for the adult population, with a contribution of 35.10%, and the Cr concentration in river water (Cr-RW) contributed most to noncarcinogenic risk in child populations, with a contribution amounting to 22.4%.
Figure 2. Cumulative probability of THI (A) and TCR (B) through combined pathways. THI: total hazard index; TCR: total cancer risk.
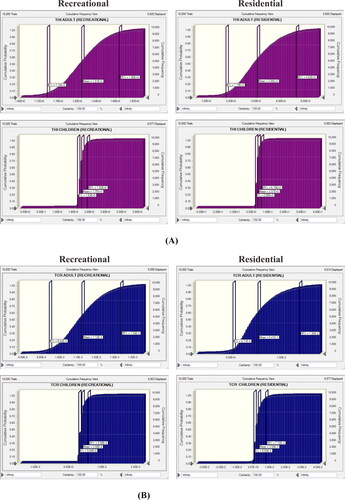
presents the cumulative probability value for estimated TCR in recreational and residential scenarios. In the recreational exposure scenario, the mean values for adults and children using a probability risk approach are 1.12 × 10−03 and 3.98 × 10−04, respectively. In the residential exposure scenario, the mean values for adults and children are 5.41 × 10−03 and 3.08 × 10−03, respectively. Those values showed that both in the recreational and residential scenarios TCR values were higher than 1 × 10−04, indicating that a potential CR exists from multiple PTE exposure pathways in the Pangkep coastal communities. depicts the TCR value sensitivity analysis results. Exposure frequency to shellfish is the most influential factor for both adult and child populations in the residential scenario, with contributions of 67.7% and 45.6%, respectively. In the recreational scenario, ED is the most important factor for adult and child populations, with contributions of 74.2% and 33.5%, respectively. This result is in line with a study by Gao et al. (Citation2019).
Figure 3. Sensitivity results for THI value in residential (A), and in recreational receptors (B); the result of Monte Carlo Simulation, Total Hazard Index (THI) defined as a forecast value, and the assumptions include PTEs concentration in river water (RW), in river sediment (RS), milkfish (MF) and shellfish (SF), Ingestion rate (IR), exposure frequency (EF), Body weight (BW), Exposure duration (ED).
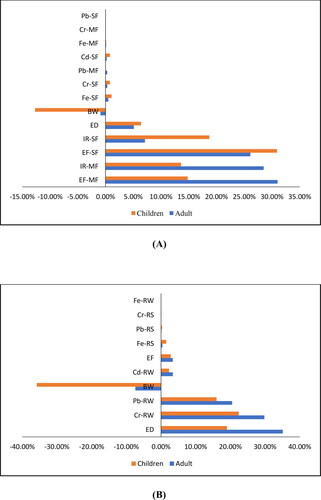
Figure 4. Sensitivity results for TCR value in residential (A), and in recreational receptors (B); the result of Monte Carlo Simulation, Total Cancer Risk (TCR) is defined as a forecast value, and the assumptions include PTEs concentration in river water (RW), river sediment (RS), milkfish (MF), and shellfish (SF), Ingestion rate (IR), exposure frequency (EF), Body weight (BW), Exposure duration (ED).
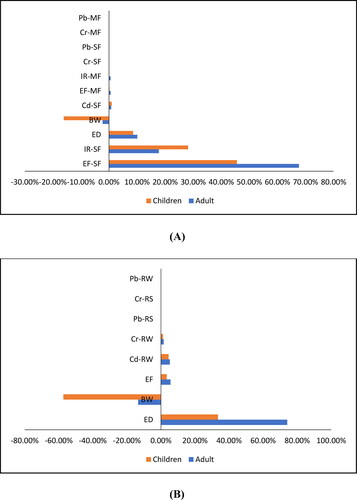
Risk management
The 95th percentile value represents the highest exposure that is reasonably predicted to occur at the site and is known as the reasonable maximum exposure (RME) (USEPA Citation2001). An RME value that exceeds the target level risk or the allowable risk means that implementation of risk reduction measures is needed. Based on the equation by Ditjen (Citation2012), a recommended value for restricting shellfish consumption (EF-SF: 3 days/year for adults and EF-SF: 7 days/year for children) should be put in place to avoid carcinogenic risk. Aside from limiting shellfish consumption, several studies recommend implementing a well-balanced diet to avoid chronic diseases such as cardiometabolic disease and death (Zhai et al. Citation2015; Micha et al. Citation2017). A recent review by Zhai et al. (Citation2015) stated that the consumption of vegetables, fruits, and other edible plants containing vitamins and essential metals can reduce the toxicity of metals in the human body. Vitamins C and E are antioxidants that have the benefit of scavenging free radicals and decreasing lipid peroxidation. For the recreational scenario, limiting ED may protect the population from carcinogenic risk with recommended ED values of 13 years (adults) and 9 years (children).
To protect populations from noncarcinogenic risks, reducing milkfish consumption frequency (EF-MF) to 170 and 140 days/year for adults and children, respectively, must be implemented. Additionally, reducing the Cr concentration in river water needs to be implemented immediately. A study by Yoshinaga et al. (Citation2018) recommends using a magnesium (Mg)- and iron (Fe)-based hydrotalcite-like compound as a low-cost and highly effective depurative method to reduce Cr pollution in the river. Additionally, strong regulatory actions, PTE concentration monitoring in river ecosystems, as well as the use of wastewater treatment plants, should be performed by the local authority.
Conclusions
The mean value of PTE concentrations in river water was found to be above the water quality standard set by the WHO, indicating that the river water is highly polluted and unacceptable for daily consumption purposes. Except for Fe, the mean PTE concentrations in sediments were lower than the PEL values, indicating that negative effects on sediment-dwelling benthic organisms are not expected to occur frequently. The mean PTE values in the aquatic organisms (Anadara granosa and Chanos chanos) were below the limit for food quality standards, except for Fe concentrations in shellfish. Based on THI and TCR values, the coastal area communities of the Pangkep regency are at high risk of noncarcinogenic and carcinogenic effects from multiple PTE exposure pathways. This conclusion is also supported by the cumulative risk value determined using the Monte Carlo simulation, which demonstrates mean values of THI and TCR value above the allowable values (THI > 1 and TCR > 1 × 10−04). The sensitivity test results revealed that ED and the concentration of Cr in river water (Cr-RW) are the most influential factors in noncarcinogenic risk in the recreational scenario, whereas the consumption frequency of milkfish and shellfish were the greatest noncarcinogenic and carcinogenic risk factors in the residential scenario. Thus, we recommend reducing Cr concentrations in the river ecosystem, restricting swimming durations, and limiting the consumption frequency of shellfish and milkfish to overcome future health risks.
Author’s contributions
All authors have materially participated and have approved the final article to publish. Ratna Dwi Puji Astuti: conceptualization, data curation, writing original draft and review, edit, data analysis; Anwar Mallongi: conceptualization, review manuscript, supervised the project; Kyungho Choi: critical feedback and helped shape the research, review manuscript; Ridwan Amiruddin: review manuscript, referencing; Muh Hatta: Review Manuscript, referencing; Kraichat Tantrakarnapa: contribute to final version of the manuscript, referencing; Annisa Utami Rauf: data collecting, draft editing.
Competing interest
The authors declared that there is no conflict of interest
Declaration of submission
This work has not been published previously and/or not under consideration for publication elsewhere. We declare that all authors were agree will not published the manuscript elsewhere if it accepted in the same form, in English or in any other languages, including electronically without the written consent of the copyright-holder.
Human ethical statement
This study has passed the ethical test with protocol number 28920093024 issued by the health research ethics commission, Hasanuddin University
Supplemental Material
Download MS Word (53.5 KB)Acknowledgements
We would like to thank the Pangkajene dan kepulauan (Pangkep) government for the warm welcome during this study occurs. The appreciation also goes to Dr. Sarinah Basri K, S.K.M, M.Kes, and Zulfikar S.Si., M.M. for their contribution during the sampling process.
Disclosure statement
No potential conflict of interest was reported by the authors.
Data availability
The datasets obtained and/or analyzed during the current study are not publicly available but are available from the corresponding author on reasonable request.
Additional information
Funding
References
- Ahmad A, Hamzah AS. 2016. Database karst sulawesi selatan; Makassar: Badan Lingkungan Hidup Daerah Provinsi Sulawesi Selatan.
- Al Osman M, Yang F, Massey IY. 2019. Exposure routes and health effects of heavy metals on children. Biometals. 32(4):563–573.
- Ali NA. 2017. Analisis Kandungan Logam Berat Timbal (Pb) Pada Kerang Di Perairan Biringkassi Kabupaten Pangkep, Sulawesi Selatan. UIN Alaudin Makassar. [Accessed August 30 2021]. http://repositori.uin-alauddin.ac.id/4177/1/NURAFDALIAALI_opt.pdf.
- Alidadi H, Tavakoly Sany SB, Zarif Garaati Oftadeh B, Mohamad T, Shamszade H, Fakhari M. 2019. Health risk assessments of arsenic and toxic heavy metal exposure in drinking water in northeast Iran. Environ Health Prev Med. 24(1):1–17.
- Ameh SO, Kgabi N, Taole SH, Ameh S, N, Anna K, S, Halahala T. 2016. Heavy metal seasonal distribution in shore sediment samples along the coastline of Erongo region, Western Namibia. Eur J Sci Res. 139(1):49–63.
- Amina. 2012. Studi kandungan logam berat besi (Fe) dalam sedimen dan kerang Anodonta Woodiana di Sungai Pangkajene Kabupaten Pangkep. Hasanuddin University.
- APHA. 1999. Standard methods for the examination of water and wastewater. Washington DC. USA
- Aripai M, Daud A, La AR. 2012. Analisis Risiko Paparan Kadmium (Cd) Pada Air Dan Kerang Putih (Anadonta Woodiana) Di Sungai Pangkajene Tahun 2012. Repos Unhas.
- Astatkie H, Ambelu A, Mengistie E. 2021. Contamination of stream sediment with heavy metals in the Awetu watershed of southwestern Ethiopia. Front Earth Sci. 9(July):1–13.
- Astuti RDP, Mallongi A, Amiruddin R, Hatta M, Rauf AU. 2021a. Risk identification of heavy metals in well water surrounds watershed area of Pangkajene, Indonesia. Gac Sanit. 35:S33–S37.
- Astuti RDP, Mallongi A, Rauf AU. 2021b. Natural enrichment of chromium and nickel in the soil surrounds the karst watershed. Glob J Environ Sci Manag. 7(3):1–18.
- Astuti RDP, Mallongi A, Rauf AU. 2021c. Risk identification of Hg and Pb in soil: a case study from Pangkep regency, Indonesia. Soil Sci Annu. 72(1):1–15.
- ATSDR. 2005. Appendix G: calculating exposure doses. Public heal assess guid man; [accessed 2021 Sept 30]. https://www.atsdr.cdc.gov/hac/phamanual/appg.html.
- ATSDR. 2019. ATSDR’s substance priority list; [accessed 2020 Dec 25]. https://www.atsdr.cdc.gov/spl/index.html.
- Awaluddin A, Kabangnga A, Noor RJ. 2020. Kajian Cemaran Timbal (Pb) pada Tambak Tradisional Ikan Bandeng (Chanos chanos). J Berk Kesehatan. 6(2):62.
- Ayers RS, Westcot DW. 1985. Water quality for agriculture. water qual agric; [accessed 2021 Oct 21]. https://www.fao.org/3/t0234e/T0234E06.htm#tab21.
- Azeh EG, Udoka FP, Nweke NF, N. Unachukwu M. 2019. Mechanism and health effects of heavy metal toxicity in humans. In: Poisoning mod world - New tricks an old dog? IntechOpen. https://www.intechopen.com/books/poisoning-in-the-modern-world-new-tricks-for-an-old-dog-/mechanism-and-health-effects-of-heavy-metal-toxicity-in-humans.
- Bahar SN, Daud AI. 2012. Risiko Paparan Arsen Pada Masyarakat Sekitar Sungai Pangkajene Kecamatan Bungoro Kabupaten Pangkep. Universitas Hasanuddin. http://pasca.unhas.ac.id/jurnal/files/2eb383b28e5a7ce1a1372b81eead68d9.pdf.
- Balali-Mood M, Naseri K, Tahergorabi Z, Khazdair MR, Sadeghi M. 2021. Toxic mechanisms of five heavy metals: mercury, lead, chromium, cadmium, and arsenic. Front Pharmacol. 12(April):1–19.
- Bandara P, Pathiratne A. 2019. Heavy metal levels in Chanos chanos (Milk fish) from Negombo estuary, Sri Lanka and human health risk assessment associated with their consumption. In: 4th International Research Symposium on Pure and Applied Sciences. Sri Lanka: University of Kelaniya. p. 2019. http://repository.kln.ac.lk/handle/123456789/20530.
- Barone G, Dambrosio A, Storelli A, Garofalo R, Busco V, Storelli M. 2018. Estimated dietary intake of trace metals from swordfish consumption: a human health problem. Toxics. 6(2):22–11.
- Bernhoft RA. 2012. Mercury toxicity and treatment: a review of the literature. J Environ Public Health. 2012:460508.
- Bhatt VR. 2019. Cancer in older adults: understanding cause and effects of chemotherapy-related toxicities. Future Oncol. 15(22):2557–2560.
- Bose-O'Reilly S, McCarty KM, Steckling N, Lettmeier B. 2010. Mercury exposure and children's health. Curr Probl Pediatr Adolesc Health Care. 40(8):186–215.
- BPS. 2021. Kabupaten Pangkajene dan Kepulauan dalam angka 2021. Kabupaten Pangkajene dan Kepulauan. Indonesia
- BSNI. 2009. Batas maksimum cemaran logam berat dalam pangan. Badan Standardisasi Nasional Indonesia. Indonesia
- Canpolat Ö, Varol M, Okan ÖÖ, Eriş KK, Çağlar M. 2020. A comparison of trace element concentrations in surface and deep water of the Keban Dam Lake (Turkey) and associated health risk assessment. Environ Res. 190:110012.
- CCME. 2001. Canadian Sediment Quality Guidelines for the Protection of Aquatic Life: summary tables. Can Counc Minist Environ; p. 5.
- CDC. 2012a. Low level lead exposure harms children: a renewed call for primary prevention.
- CDC. 2012b. Response to advisory committee on childhood lead poisoning prevention recommendations in low level lead exposure harms children: a renewed call of primary prevention. Morb Mortal Wkly. 61(20):383.
- China Food and Drug Administration. 2017. GB2762-2017: National Food Standard Maximum Level of Contaminants in Foods.
- Clancy HA, Sun H, Passantino L, Kluz T, Muñoz A, Zavadil J, Costa M. 2012. Gene expression changes in human lung cells exposed to arsenic, chromium, nickel or vanadium indicate the first steps in cancer. Metallomics. 4(8):784–793.
- Commission Regulation (EU). 2006. Commission Regulation (EC) No 118/2006 of 19 December 2006 setting maximum levels for certain contaminants in foodstuffs. Off J Eur Union. (364):5–24. https://eur-lex.europa.eu/LexUriServ/LexUriServ.do?uri=OJ:L:2006:364:0005:0024:EN:PDF.
- Creel L. 2002. Children’s environmental health: risks and remedies. Washington D.C. [accessed 2021 September 30]. http://www.prb.org/pdf/ChildrensEnvironHlth_Eng.pdf
- Das SC, Varadharajan K, Shanmugakonar M, A-NHA. 2021. Chronic cadmium exposure alters cardiac matrix metalloproteinases in the heart of Sprague-Dawley rat. Front Pharmacol. 12(August):1–11.
- Departemen Pertanian RI. 2009. Analisis Kimia Tanah, Tanaman, Air dan Pupuk.
- Depdikbud RI. 1976. Geografi Budaya Daerah Sulawesi Selatan.
- Ditjen PPPL. 2012. Pedoman Analisis Risiko Kesehatan Lingkungan (ARKL).
- DPU. 2017. Gambaran umum dan kondisi wilayah kabupaten Pangkajene dan Kepulauan. Kabupaten Pangkajene dan Kepulauan: Dinas PU Kabupaten Pangkep.
- EFSA. 2009. Scientific opinion of the panel on contamintant in the food chain: cadmium in food. EFSA J. 980:1–139.
- EFSA. 2010. Scientific opinion on lead in food. EFSA J. 8(4):1570.
- EFSA. 2013. Scientific opinion on the safety assessment of the active substances iron, iron oxides, sodium chloride and calcium hydroxide for use in food contact materials. EFSA J. 11(10):3387.
- Egbueri JC. 2020. Heavy metals pollution source identification and probabilistic health risk assessment of shallow groundwater in Onitsha, Nigeria. Anal Lett. 53(10):1620–1638.
- Egbueri JC, Mgbenu CN. 2020. Chemometric analysis for pollution source identification and human health risk assessment of water resources in Ojoto Province, southeast Nigeria. Appl Water Sci. 10(4):1–18.
- Enyigwe MT, Onwuka OS, Egbueri JC. 2021. Geochemical distribution, statistical and health risk assessment of toxic elements in groundwater from a typical mining district in Nigeria. Environ Forensics. 22:1–13.
- Etzel RA. 2020. The special vulnerability of children. Int J Hyg Environ Health. 227:113516.
- Ezugwu CK, Onwuka OS, Egbueri JC, Unigwe CO, Ayejoto DA. 2019. Multi-criteria approach to water quality and health risk assessments in a rural agricultural province, southeast Nigeria. HydroResearch. 2:40–48.
- Fang Z, Zhao M, Zhen H, Chen L, Shi P, Huang Z. 2014. Genotoxicity of tri- and hexavalent chromium compounds in vivo and their modes of action on DNA damage in vitro. PLoS One. 9(8):e103194.
- FAO/WHO. 2019. Codex alimentarius: general standard for contaminants and toxins in food and feed.
- Fitriani A, Sulfikar, Dini I. 2014. Analisis Kandungan Logam Timbal (Pb) pada Sedimen dan Udang Windu (Penaeus monodon) di Pantai Biringkassi Kecamatan Bungoro Kabupaten Pangkep. Sainsmat. III(2):191–202.
- Fitriani AN, Daud A. Ruslan 2013. Studi Konsentrasi Logam Berat Timbal (Pb) Dalam Sedimen dan Udang Di Perairan Sungai Pangkajene Kabupaten Pangkep. Repos Unhas. 1–8.
- Gao B, Gao L, Gao J, Xu D, Wang Q, Sun K. 2019. Simultaneous evaluations of occurrence and probabilistic human health risk associated with trace elements in typical drinking water sources from major river basins in China. Sci Total Environ. 666:139–146.
- González LT, Longoria Rodríguez FE, Sánchez-Domínguez M, Cavazos A, Leyva-Porras C, Silva-Vidaurri LG, Askar KA, Kharissov BI, Villarreal Chiu JF, Alfaro Barbosa JM. 2017. Determination of trace metals in TSP and PM2.5 materials collected in the Metropolitan Area of Monterrey, Mexico: a characterization study by XPS, ICP-AES and SEM-EDS. Atmos Res. 196:8–22.
- Haeriah H. 2019. Analisis Kandungan Logam Berat Kadmium (Cd) Pada Ikan Bandeng (Chanos chanos) di Pertambakan Kecamatan Pangkajene. Teknosains Media Inf Sains Dan Teknol. 12(2):176–188.
- Han JL, Pan XD, Chen Q, Huang BF. 2021. Health risk assessment of heavy metals in marine fish to the population in Zhejiang, China. Sci Rep. 11(1):1–9.
- Haspullah R, Ambeng, Hasyim Z, Soekendarsi E. 2018. Analisis Kandungan Logam Berat Timbal (Pb), Kromium (Cr), dan Kadmium (Cd) pada Kerang Darah Anadara granosa L. di Wilayah Pesisir Kabupaten Pangkep. Universitas Hasanuddin.
- Isidori A, Borin L, Elli E, Latagliata R, Martino B, Palumbo G, Pilo F, Loscocco F, Visani G, Cianciulli P. 2018. Iron toxicity – Its effect on the bone marrow. Blood Reviews. 32(6):473–479.
- Jaishankar M, Tseten T, Anbalagan N, Mathew BB, Beeregowda KN. 2014. Toxicity, mechanism and health effects of some heavy metals. Interdiscip Toxicol. 7(2):60–72.
- Jiang M, Zeng G, Zhang C, Ma X, Chen M, Zhang J, Lu L, Yu Q, Hu L, Liu L. 2013. Assessment of heavy metal contamination in the surrounding soils and surface sediments in Xiawangang River, Qingshuitang District. PLoS One. 8(8):e71176.
- Karatela S, Coomarasamy C, Paterson J, Ward NI. 2020. Exposure to toenail heavy metals and child behavior problems in nine-year-old children: a cross-sectional study. Int J Environ Res Public Health. 17(11):1–12.
- Karmakar B, Singh MK, Choudhary BK, Singh SK, Egbueri JC, Gautam SK, Rawat KS. 2021. Investigation of the hydrogeochemistry, groundwater quality, and associated health risks in industrialized regions of Tripura, northeast India. Environ Forensics. 22:1–22
- Kļaviņs M, Briede A, Rodinov V, Kokorite I, Parele E, Kļaviņa I. 2000. Heavy metals in rivers of Latvia. Sci Total Environ. 262(1–2):175–183.
- Koedrith P, Kim HL, Weon J, Seo YR. 2013. Toxicogenomic approaches for understanding molecular mechanisms of heavy metal mutagenicity and carcinogenicity. Int J Hyg Environ Health. 216(5):587–598.
- Kuang Z, Gu Y, Rao Y, Huang H. 2021. Biological risk assessment of heavy metals in sediments and health risk assessment in marine organisms from Daya Bay, China. J Mar Sci Eng. 9(1):1–17.
- Liu M, Chen J, Sun X, Hu Z, Fan D. 2019. Accumulation and transformation of heavy metals in surface sediments from the Yangtze River estuary to the East China Sea shelf. Environ Pollut. 245:111–121.
- MacDonald DD, Ingersoll CG, Berger TA. 2000. Development and evaluation of consensus-based sediment quality guidelines for freshwater ecosystems. Arch Environ Contam Toxicol. 39(1):20–31.
- Maddusa SS, Asrifuddin A. 2008. Studi Kandungan Kadmium (Cd) pada Biota, Sedimen dan Air Kabupaten, pada Sungai Pangkajene Kecamatan Bungoro Pangkep. Paradigma; p. 70–78. http://www.ejournalhealth.com/index.php/paradigma/article/viewFile/216/210.
- Mallongi A, Astuti RDP, Amiruddin R, Hatta M, Rauf AU. 2022. Identification source and human health risk assessment of potentially toxic metal in soil samples around karst watershed of Pangkajene, Indonesia. Environ Nanotechnol Monit Manag. 17:100634.
- Mallongi A, Stang Manyullei S, Natsir MF, Astuti RDP, Rauf AU. 2019. Risks assessment of silica contamination on the communities living surround the cement industry, Pangkep Indonesia. Indian J Public Heal Res Dev. 10(10):1619.
- MFDS. 2017. MRL pesticides and other contaminants in Food [Internet]. South Korea. https://apps.fas.usda.gov/newgainapi/api/report/downloadreportbyfilename?filename=FoodandAgriculturalImportRegulationsandStandardsReport_Seoul_Korea-Republicof_3-22-2019.pdf.
- Mgbenu CN, Egbueri JC. 2019. The hydrogeochemical signatures, quality indices and health risk assessment of water resources in Umunya district, southeast Nigeria. Appl Water Sci. 9(1):1–19.
- Micha R, Peñalvo JL, Cudhea F, Imamura F, Rehm CD, Mozaffarian D. 2017. Association between dietary factors and mortality from heart disease, stroke, and type 2 diabetes in the United States. JAMA. 317(9):912–924.
- Ministry of Health Republic Indonesia. 2010. Peraturan Menteri Kesehatan Republik Indonesia No. 492/Menkes/PER/IV/2010 tentang peryaratan kualitas air minum. Indonesia.
- Mrozińska N, Bąkowska M. 2020. Effects of heavy metals in lake water and sediments on bottom invertebrates inhabiting the brackish coastal lake Łebsko on the southern Baltic coast. Int J Environ Res Public Health. 17(18):1–19.
- Mwakalapa EB, Simukoko CK, Mmochi AJ, Mdegela RH, Berg V, Bjorge Müller MH, Lyche JL, Polder A. 2019. Heavy metals in farmed and wild milkfish (Chanos chanos) and wild mullet (Mugil cephalus) along the coasts of Tanzania and associated health risk for humans and fish. Chemosphere. 224:176–186.
- Najmeddin A, Keshavarzi B, Moore F, Lahijanzadeh A. 2018. Source apportionment and health risk assessment of potentially toxic elements in road dust from urban industrial areas of Ahvaz megacity, Iran. Environ Geochem Health. 40(4):1187–1208.
- Nuhung S. 2016. Karst Maros Pangkep Menuju Geopark Dunia (Tinjauan Dari Aspek Geologi Lingkungan). J Plano Madani. 5(1):1–7.
- Onyemesili OO, Egbueri JC, Ezugwu CK. 2020. Assessing the pollution status, ecological and health risks of surface waters in Nnewi urban, Nigeria: implications of poor waste disposal. Environ Forensics. 21:1–14.
- Paena M, Asaad AIJ, Suhaimi RA. 2015. Analisa Karakteristik Sedimen Sungai Pangkajene Kepulauan dan Tanah Tambak di Sekitarnya (Studi Kasus Kematian Ikan Massal di Sungai Pangkajene Kabupaten Pangkep Provinsi Sulawesi Selatan). In: Sugama K, Kristanto AH, Radiarta IN, Lusiastuti AM, Kusdiarti, Priono B, Insan I, Dewi RRSP S, Gardenia L, editors. Pros Forum Inov Teknol Akuakultur [Internet]. Jakarta: Pusat Penelitian dan Pengembangan Perikanan Budidaya; p. 769–779. https://bppbapmaros.kkp.go.id/wp-content/uploads/2016/07/FITA_025.pdf
- Pandion K, Khalith SBM, Ravindran B, Chandrasekaran M, Rajagopal R, Alfarhan A, Chang SW, Ayyamperumal R, Mukherjee A, Arunachalam KD. 2022. Potential health risk caused by heavy metal associated with seafood consumption around coastal area. Environ Pollut. 294(December):118553.
- Paustenbach DJ, Finley BL, Mowat FS, Park M, Kerger BD. 2003. Human health risk and exposure assessment of chromium (VI) in tap water. J Toxicol Environ Health A. 66(14):1295–1339.
- Polvé M, Maury RC, Bellon H, Rangin C, Priadi B, Yuwono S, Joron JL, Soeria Atmadja R. 1997. Magmatic evolution of Sulawesi (Indonesia): constraints on the Cenozoic geodynamic history of the Sundaland active margin. Tectonophysics. 272(1):69–92.
- Qu C, Sun K, Wang S, Huang L, Bi J. 2012. Monte Carlo simulation-based health risk assessment of heavy metal soil pollution: a case study in the Qixia Mining Area, China. Hum Ecol Risk Assess. 18(4):733–750.
- Qu L, Huang H, Xia F, Liu Y, Dahlgren RA, Zhang M, Mei K. 2018. Risk analysis of heavy metal concentration in surface waters across the rural-urban interface of the Wen-Rui Tang River, China. Environ Pollut. 237:639–649.
- Rahman MS, Molla AH, Saha N, Rahman A. 2012. Study on heavy metals levels and its risk assessment in some edible fishes from Bangshi River, Savar, Dhaka, Bangladesh. Food Chem. 134(4):1847–1854.
- RAIS. 2020. Frequently Asked Questions. Risk Assess Inf Syst. [accessed 2021 Sept 30]. https://rais.ornl.gov/home/faq.html.
- Rauf AU, Mallongi A, Daud A, Amiruddin R, Stang S, Wahyu A, Astuti RDP 2022. Spatial distribution and ecological risk of potentially toxic elements in maros regency, Indonesia. Carpathian J Earth Environ Sci. 17(1):93–100.
- Rauf AU, Mallongi A, Daud A, Hatta M, Al-Madhoun W, Amiruddin R, Rahman SA, Wahyu A, Astuti RDP. 2021a. Community health risk assessment of total suspended particulates near a cement plant in Maros Regency, Indonesia. J Heal Pollut. 11(30):1–13.
- Rauf AU, Mallongi A, Daud A, Hatta M, Astuti RDP. 2021b. Ecological risk assessment of hexavalent chromium and silicon dioxide in well water in Maros Regency, Indonesia. Gac Sanit. 35:S4–S8.
- Rauf AU, Mallongi A, Lee K, Daud A, Hatta M, Al Madhoun W, Astuti RDP. 2021c. Potentially toxic element levels in atmospheric particulates and health risk estimation around industrial areas of Maros, Indonesia. Toxics. 9(12):328.
- Reading BD, Freeman B. 2005. Simple formula for the surface area of the body and a simple model for anthropometry. Clin Anat. 18(2):126–130.
- Rovira J, Domingo JL. 2019. Human health risks due to exposure to inorganic and organic chemicals from textiles: a review. Environ Res. 168:62–69.
- Sah D, Verma PK, Kumari KM, Lakhani A. 2017. Chemical partitioning of fine particle-bound As, Cd, Cr, Ni, Co, Pb and assessment of associated cancer risk due to inhalation, ingestion and dermal exposure. Inhal Toxicol. 29(11):483–493.
- Salamahu A. 2012. Kandungan logam berat timbal (Pb) pada air dan sediment sungai pangkajene di sekitar pabrik semen tonasa Sulawesi Selatan [Internet]. Hasanuddin University. http://digilib.unhas.ac.id/uploaded_files/temporary/DigitalCollection/NGNmYmIzNzhjMGM4ZjgxZmNiMmIwOGY5ODU4ZTg1NGZhMGUwZjRmNw==.pdf.
- Sanaei F, Amin MM, Alavijeh ZP, Esfahani RA, Sadeghi M, Bandarrig NS, Fatehizadeh A, Taheri E, Rezakazemi M. 2021. Health risk assessment of potentially toxic elements intake via food crops consumption: Monte Carlo simulation-based probabilistic and heavy metal pollution index. Environ Sci Pollut Res Int. 28(2):1479–1490.
- Satarug S, Garrett SH, Sens MA, Sens DA. 2010. Cadmium, environmental exposure, and health outcomes. Environ Health Perspect. 118(2):182–190.
- Satarug S, Nishijo M, Ujjin P, Moore MR. 2018. Chronic exposure to low-level cadmium induced zinc-copper dysregulation. J Trace Elem Med Biol. 46:32–38.
- Shakeri A, Sharifi Fard M, Mehrabi B, R, Mehr M. 2020. Occurrence, origin and health risk of arsenic and potentially toxic elements (PTEs) in sediments and fish tissues from the geothermal area of the Khiav River, Ardebil Province (NW Iran). J Geochemical Explor. 208(2020):106347.
- Sly JL, Carpenter DO. 2012. Special vulnerability of children to environmental exposures. Rev Environ Health. 27(4):151–157.
- Soegianto A, Putranto TWC, Lutfi W, Almirani FN, Hidayat AR, Muhammad A, Firdaus RA, Rahmadhani YS, Fadila DAN, Hidayati D. 2020. Concentrations of metals in tissues of cockle Anadara granosa (Linnaeus, 1758) from east Java coast, Indonesia, and potential risks to human health. Int J Food Sci. 2020:1–9.
- Syarif EJ. 2015. Visualisasi deposit logam berat timbel (Pb) pada organ hati ikan bandeng (Chanos chanos) dengan pewarnaan rhodizonate melalui metode histoteknik. Hasanuddin University. Makassar, Indonesia.
- Tajdar-Oranj B, Shariatifar N, Alimohammadi M, Peivasteh-Roudsari L, Khaniki GJ, Fakhri Y, Mousavi Khaneghah A. 2018. The concentration of heavy metals in noodle samples from Iran’s market: probabilistic health risk assessment. Environ Sci Pollut Res. 25(31):30928–30937.
- Tong S, Li H, Tudi M, Yuan X, Yang L. 2021. Comparison of characteristics, water quality and health risk assessment of trace elements in surface water and groundwater in China. Ecotoxicol Environ Saf. 219:112283.
- Tsai M-T, Huang S-Y, Cheng S-Y. 2017. Lead poisoning can be easily misdiagnosed as acute porphyria and nonspecific abdominal pain. Case Rep Emerg Med. 2017:9050713–9050714.
- Ukah BU, Egbueri JC, Unigwe CO, Ubido OE. 2019. Extent of heavy metals pollution and health risk assessment of groundwater in a densely populated industrial area, Lagos, Nigeria. Int J Energ Water Res. 3(4):291–303.
- UNESCO. 2019. Geographical and geological summary of Maros-Pangkep Geopark, Indonesia; p. 954.
- USEPA. 1989. Risk assessment guidance for superfund. Volume I Human Health Evaluation Manual (Part A); 289. https://rais.ornl.gov/documents/HHEMA.pdf.
- USEPA. 1991. Risk Assessment Guidance for Superfund. Volume I Human Health Evaluation Manual Part (B, Development of Risk-basedPreliminary Remediation Goals). US Environ Prot Agency. I(December); p.66. https://nepis.epa.gov/Exe/ZyPDF.cgi/100020LF.PDF?Dockey=100020LF.PDF.
- USEPA. 2001. Risk assessment guidance for superfund (rags) volume iii - part a: process for conducting probabilistic risk assessment. Off Emerg Remedial Response US Environ Prot Agency [Internet]. III(December); p. 1–385. http://www.epa.gov/sites/production/files/2015-09/documents/rags3adt_complete.pdf.
- USEPA. 2002. Supplemental guidance for developing soil screening levels for superfund sites. United States Environ Prot Agency.(December); p. 1–187. https://nepis.epa.gov/Exe/ZyPDF.cgi/91003IJK.PDF?Dockey=91003IJK.PDF.
- USEPA. 2004. Risk assessment guidance for superfund volume I: human health evaluation manual. (Part e) Supplemental Guidance for Dermal Risk Assessment); p. 1–156.
- USEPA. 2005. Guidelines for carcinogen risk assessment. Washington, DC. http://www.epa.gov/sites/production/files/2013-09/documents/cancer_guidelines_final_3-25-05.pdf.
- USEPA. 2006. National Recommended Water Quality Criteria - Aquatic Life Criteria Table. [accessed 2021 Oct 21]. https://www.epa.gov/wqc/national-recommended-water-quality-criteria-aquatic-life-criteria-table.
- USEPA. 2011. Exposure Factors Handbook: 2011 Edition; p. 1–1436.
- USEPA. 2015. Mid-Atlantic Risk Assessment: equations (June 2015). Mid-Atlantic Risk Assess. [accessed 2021 Oct 3]. https://archive.epa.gov/region9/superfund/web/html/equations.html.
- USEPA. 2021a. Exposure assessment tools by routes - Dermal. [accessed 2022 Jan 16]. https://www.epa.gov/expobox/exposure-assessment-tools-routes-dermal.
- USEPA. 2021b. Regional screening levels (RSLs) - User’s guide. [accessed 2021 Sept 30]. https://www.epa.gov/risk/regional-screening-levels-rsls-users-guide#target.
- USEPA. 2021c. Regional Screening Level (RSL) Summary Table (TR = 1E-06, HQ = 1). [accessed 2021 Sept 30]. https://semspub.epa.gov/work/HQ/400750.pdf.
- USEPA 2021d. Regional screening levels (RSLs) - equations.
- Ustaoğlu F, Tepe Y, Taş B. 2020. Assessment of stream quality and health risk in a subtropical Turkey river system: a combined approach using statistical analysis and water quality index. Ecol Indic. 113(May):105815.
- Viers J, Dupré B, Gaillardet J. 2009. Chemical composition of suspended sediments in World Rivers: new insights from a new database. Sci Total Environ. 407(2):853–868.
- WALHI. 2018. Sulawesi Selatan 2018: Degradasi lingkungan dan pengaplingan ruang hidup rakyat terus meningkat. Indonesia.
- White MC, Holman DM, Boehm JE, Peipins LA, Grossman M, J, Henley S. 2014. Age and cancer risk: a potentially modifiable relationship. Am J Prev Med. 46(Suppl. 3):1):1–16.
- WHO. 2007. Health risks of heavy metals from long-range transboundary air pollution. Copenhagen: Joint WHO/Convention Task Force on the Health Aspects of Air Pollution. https://www.euro.who.int/__data/assets/pdf_file/0007/78649/E91044.pdf.
- WHO. 2017. Guideline for drinking water quality: fourth edition incorporating the first addendum; p. 1–631.
- Wilson MEJ. 1996. Evolution and hydrocarbon potential of the tertiary tonasa limestone formation, Sulawesi, Indonesia. 25th Annu Conv Proc; p. 227–240. http://searg.rhul.ac.uk/pubs/wilson_1996HydrocarbonpotentialTertiaryTonasaLimestone.pdf.
- Wilson MEJ, Bosence DWJ. 1997. Platform-top and ramp deposits of the Tonasa Carbonate Platform, Sulawesi, Indonesia. Geol Soc Spec Publ. 126(1):247–279.
- Wojciechowska E, Nawrot N, Walkusz-Miotk J, Matej-Łukowicz K, Pazdro K. 2019. Heavy metals in sediments of urban streams: contamination and health risk assessment of influencing factors. Sustain. 11(3):5–10.
- Xiao CC, Chen MJ, Mei FB, Fang X, Huang TR, Li JL, Deng W, Li YD. 2018. Influencing factors and health risk assessment of microcystins in the Yongjiang river (China) by Monte Carlo simulation. PeerJ. 2018(11):1–21.
- Xiao J, Wang L, Deng L, Jin Z. 2019. Characteristics, sources, water quality and health risk assessment of trace elements in river water and well water in the Chinese Loess Plateau. Sci Total Environ. 650:2004–2012.
- Yang D, Liu J, Wang Q, Hong H, Zhao W, Chen S, Yan C, Lu H. 2019. Geochemical and probabilistic human health risk of chromium in mangrove sediments: a case study in Fujian, China. Chemosphere. 233:503–511.
- Yenny MOP, Hartono A, Anwar S, Kang Y. 2020. Assessment of heavy metals pollution in sediment of Citarum River, Indonesia. J Pengelolaan Sumberd Alam dan. Lingkung (J Nat Resour Environ Manag. 10(4):584–593.
- Yi Y, Tang C, Yi T, Yang Z, Zhang S. 2017. Health risk assessment of heavy metals in fish and accumulation patterns in food web in the upper Yangtze River, China. Ecotoxicol Environ Saf. 145:295–302.
- Yi Y, Yang Z, Zhang S. 2011. Ecological risk assessment of heavy metals in sediment and human health risk assessment of heavy metals in fishes in the middle and lower reaches of the Yangtze River basin. Environ Pollut. 159(10):2575–2585.
- Yona D, Sari SHJ, Iranawati F, Fathur Rayyan M, Rini NM. 2020. Heavy metals accumulation and risk assessment of Anadara granosa from eastern water of Java Sea, Indonesia. IOP Conf Ser Earth Environ Sci. 416(012007):1–12.
- Yona D, Sartimbul A, Rahman MA, Sari SHJ, Mondal P, Hamid A, Humairoh T. 2021. Bioaccumulation and health risk assessments of heavy metals in mussels collected from madura strait, Indonesia. JIPK. 13(1):20–28.
- Yoshinaga M, Ninomiya H, Al Hossain MMA, Sudo M, Akhand AA, Ahsan N, Alim MA, Khalequzzaman M, Iida M, Yajima I, et al. 2018. A comprehensive study including monitoring, assessment of health effects and development of a remediation method for chromium pollution. Chemosphere. 201:667–675.
- Zakir HM, Sharmin S, Akter A, Rahman MS. 2020. Assessment of health risk of heavy metals and water quality indices for irrigation and drinking suitability of waters: a case study of Jamalpur Sadar area, Bangladesh. Environ Adv. 2:100005.
- Zeinali T, Salmani F, Naseri K. 2019. Dietary intake of cadmium, chromium, copper, nickel, and lead through the consumption of meat, liver, and kidney and assessment of human health risk in Birjand, Southeast of Iran. Biol Trace Elem Res. 191(2):338–347.
- Zhai Q, Narbad A, Chen W. 2015. Dietary strategies for the treatment of cadmium and lead toxicity. Nutrients. 7(1):552–571.
- Zhang H, Zhabyeyev P, Wang S, Oudit GY. 2019. Role of iron metabolism in heart failure: from iron deficiency to iron overload. Biochim Biophys Acta Mol Basis Dis. 1865(7):1925–1937.
- Zulfiah A, Seniwati S, Sukmawati S. 2017. Analisis kadar timbal (Pb), seng (Zn), dan tembaga (Cu) pada ikan bandeng (Chanos chanos Forsk.) yang berasal dari Labakkang Kab. Pangkep secara spektrofotometri serapan atom (SSA). Jurn as-Syifaa. 9(1):85–91.