ABSTRACT
The gut microbiota plays important roles in nutrient absorption, immune system development, and pathogen colonization resistance. Perturbations early in life may be detrimental to host health in the short and the long-term. Antibiotics are among the many factors that influence the development of the microbiota. Because antibiotics are heavily administered during the first critical years of gut microbiota development, it is important to understand the effects of these interventions. Infants, particularly those born prematurely, represent an interesting population because they receive early and often extensive antibiotic therapy in the first months after birth. Gibson et al. recently demonstrated that antibiotic therapy in preterm infants can dramatically affect the gut microbiome. While meropenem, ticarcillin-clavulanate, and cefotaxime treatments were associated with decreased species richness, gentamicin and vancomycin had variable effects on species richness. Interestingly, the direction of species richness response could be predicted based on the abundance of 2 species and 2 genes in the microbiome prior to gentamicin or vancomycin treatment. Nonetheless, all antibiotic treatments enriched the presence of resistance genes and multidrug resistant organisms. Treatment with different antibiotics further resulted in unique population shifts of abundant organisms and selection for different sets of resistance genes. In this addendum, we provide an extended discussion of these recent findings, and outline important future directions for elucidating the interplay between antibiotics and preterm infant gut microbiota development.
Introduction
The infant gut microbiota is shaped by both prenatal and postnatal factors.Citation1,2 Following birth, the infant gut microbiota undergoes a patterned maturation process.Citation3-5 This process begins with a few taxa, but this population inexorably expands in content and concentration until it approaches an adult-like microbial configuration dominated by Bacteroidetes and Firmicutes.Citation3,6-8 Perturbation of the gut microbiota during this key developmental window can have lasting effects on host physiology and disease risk.Citation9-14 One of the most common perturbations during this period, antibiotic therapy,Citation13,15 can substantially alter the gut microbiota and infant physiology.Citation10,16-21 Because preterm infants are at high risk for infection, antibiotics are the most commonly prescribed medications in neonatal intensive care units (NICUs) in the United States ()Citation15,22,23 accounting for 3 of the top 6 medications with the greatest relative increase in use in NICUs in the United States between 2005 and 2010. Citation24 Antibiotic-induced gut microbiota dysbioses in this population have been linked to the pathogenesis of necrotizing enterocolitis,Citation25-27 late onset sepsis,Citation28,29 and other adverse health outcomes.Citation30 While these correlations exist, the underlying etiologies remain unclear, motivating the study of microbiota development in the context of antibiotic therapy in this vulnerable population that accounts for nearly 10% of all births in the United States.Citation31
Figure 1. Antibiotics are the most prescribed medications in the neonatal intensive care unit. Data adapted from Clark et al. Pediatrics 2006. Citation23 Frequency defined as the number of times a unique medication name was reported in the medications table in 220 NICUs in the United States and Puerto Rico between January 1996 and April 2005.
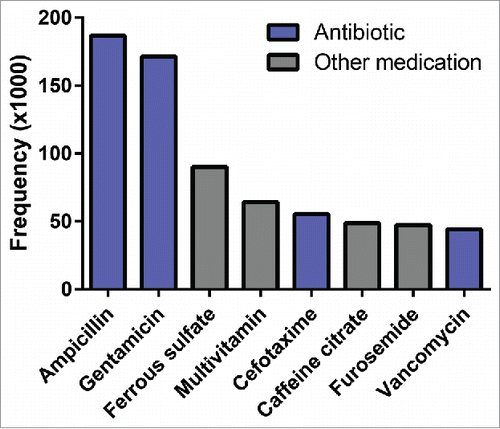
Techniques for studying development of the infant gut microbiota and the developing resistome
Microbial ecology studies, especially those focused on the gut microbiota, have flourished because of widespread adoption of sequencing-based studies, either using the 16s rRNA gene or metagenomic shotgun sequencing, resulting in a deeper appreciation of microbiome diversity.Citation4,20,27,32-34 Until recently, similar efforts to characterize the preterm infant gut reservoir of antibiotic resistance genes (the resistome) have been largely culture or PCR-based. While these methods can provide a high level overview of the resistome, they underestimate diversity, are limited to previously known resistance genes, and are only semi-quantitative. In contrast, deep sequencing of microbiomes paired with functional metagenomics offers an unbiased method to identify functional resistance determinants in stool, and can provide quantitative information on microbiome and resistome architecture. Citation35,36 Gibson et al. recently used this suite of techniques to develop a sequence-unbiased account of the effects of antibiotic therapy on the preterm infant microbiota with respect to phylogeny and antibiotic resistance gene distribution.Citation37
A longitudinal, sequencing based interrogation of the preterm infant gut microbiota
To assess the effects of antibiotics on the dynamics of the preterm infant gut microbiota, Gibson et al. assembled a cohort consisting of 84 preterm infants, of whom 82 received antibiotics within the first 24 to 48 hours of life, as is common practice in the NICU. However, 39% received no further antibiotic therapies after the first week of life, allowing them to serve as controls to study the effects of antibiotic treatment on preterm infants in the first weeks of life. All infants in the cohort were low birthweight and born prematurely, with a median gestational age of 27 weeks and a median birthweight of 865 g. This sample set was complemented by robust metadata including detailed histories of all antibiotic exposures, overall health indications including infections, delivery mode, postmenstrual age, hospital environment, enteral feeding, other medications, and maternal health.
A total of 401 stools from these infants, collected longitudinally during their stay in the NICU and ranging from day 6 to day 158 of life, were interrogated by shotgun metagenomic sequencing. In total, Gibson et al. sequenced and analyzed 551 gigabases (Gb) of preterm infant gut metagenomes (1.37 +/− 1.17 Gb per sample) and a total of 107 Gb of functionally selected preterm infant resistomes (5.1 +/− 3.6 Gb per sample). Unique clade-specific marker genes from approximately 17,000 reference genomes were used to assess the phylogenetic composition allowing both species-level resolution and accurate relative abundance estimations of bacterial species composition of these communities, an advantage over traditional 16S rRNA marker gene sequencing. Citation38,39 This represents the largest metagenomic sequencing-based study of the preterm infant gut microbiota to date, providing the statistical power to address questions of microbiota and resistome development in preterm infant populations subject to heavy antibiotic exposure.
Antibiotics disrupt the preterm gut microbiota richness and composition
Gut microbial species richness, defined as the number of species present in a microbiota, is widely used as a measure of microbiome health. Decreased species richness in infancy has been associated with a number of host pathologies.Citation13,25,40,41 To identify factors that significantly contribute to species richness, Gibson et al. developed a generalized linear mixed model with individual included as a random effect, leveraging available metadata including infant health (e.g. CRIB II (Clinical Risk Index for Babies) score, day of life, gestational age, birthweight, delivery mode, and presence of positive culture), medications (e.g., antibiotics, caffeine, and iron), and maternal health (e.g. preeclampsia and premature membrane rupture). While postmenstrual age and breast milk significantly contribute to increased species richness, the opposite was true with high CRIB II score, meropenem treatment, cefotaxime treatment, and ticarcillin-clavulanate treatment significantly contributing to decreased species richness. Cumulative antibiotic exposure in infants was associated with a significant reduction in species richness and, with the exception of gentamicin, all antibiotics were associated with reduced species richness. This decrease in species richness occurred over intervals during which the gut microbiota of age-matched, antibiotic-naïve preterm infants was increasing in richness, and was accompanied by disruption of microbiota composition (). For example, one individual had a ∼25% decrease in observed species following meropenem treatment, while over the same period a matched control had a 20% increase (). Similar age-matched comparisons can be made for combined vancomycin and meropenem treatment () and for ticarcillin-clavulanate treatment (). Antibiotic-induced perturbations of microbiota phylogenetic composition were observed for these infants as well ().
Figure 2. Antibiotic treatment significantly alters preterm infant gut microbiota development. Preterm infant gut microbiota species richness (top) and composition (bottom) for the 3 individuals with samples analyzed directly before and after depicted antibiotic treatments for (A) meropenem, (B) vancomycin and meropenem, and (C) ticarcillin-clavulanate. Red bars (left) represent species richness for example individual. Black bars (right) represent average species richness for age matched preterm infants with no antibiotic treatment. Error bars depict one standard deviation.
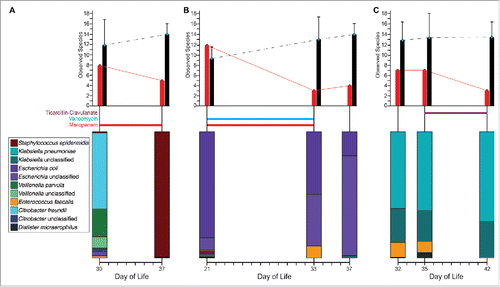
Antibiotic therapy enriches for antibiotic resistance genes and multidrug resistant bacteria
For further insight into the effects of antibiotics on the resistome of the preterm infant gut microbiota, Gibson et al. constructed functional metagenomic libraries from 21 representative fecal samples. Functional metagenomics is a valuable technique for identifying functional resistance determinants in microbiomes. Because functional metagenomics circumvents the bias introduced by culture and the need for a priori knowledge of resistance function, the method provides a high throughput, sequence-unbiased characterization of reservoirs of antibiotic resistance genes in a given environment and can identify novel resistance genes.Citation35,42 These libraries, representing a total of 107 Gb of bacterial DNA, were screened against 16 antibiotics, including those routinely used in NICUs. Of the 794 functionally identified antibiotic resistance genes, 42% were encoded by E. coli, Enterobacter cloacae, and K. pneumoniae, taxa which include many pathogenic strains implicated in nosocomial infections in preterm infants. Citation34,43 Interestingly, the sequences of the majority of resistance determinants uncovered by the functional metagenomics screens were already present in protein databases. However, they were not found in antibiotic resistance specific databases, indicating that they had not previously been ascribed a resistance function and highlighting a key advantage of using functional metagenomics.
One third of the antibiotic resistance genes identified by functional selections conferred resistance to multiple antibiotics, often across classes, or were co-selected with another antibiotic resistance gene. For example, the loci selected by piperacillin also conferred resistance to other β-lactam antibiotics. In fact, many β-lactamases identified in this study conferred resistance to multiple classes of β-lactam antibiotics. In addition to these genes, which may be extended spectrum β-lactamases (ESBLs), Gibson et. al. identified multiple Ambler class A β-lactamases, and β-lactamases that conferred resistance to both penicillins and 2nd and 3rd generation cephalosporins. ESBLs are of particular concern due to their increasing abundance in community acquired infections and in clinical settings such as the NICU, often in Enterobacteriaceae family members, including those that Gibson et. al. identified as dominant members of the preterm infant gut microbiota. Citation44 In addition to cross resistance among β-lactam antibiotics, Gibson et. al. also observed contigs that conferred resistance across classes of antibiotic. For example, many tetracycline resistance contigs also conferred resistance to both chloramphenicol and cefoxitin. Our findings suggests that many of these genes may be present in the context of a multidrug drug resistance cassette and are threats for dissemination via horizontal gene transfer. Multidrug-resistance is one of 2 key features associated with spread of antimicrobial resistance in the preterm infant gut microbiota. The other feature is mobility, or the likelihood of horizontal gene transfer. In order to investigate the potential mobility of resistance genes in the preterm infant gut microbiome Gibson et al. assembled metagenomic contigs from shotgun reads, and found widespread plasmid and chromosomally encoded multidrug resistance clusters. Alarmingly, the authors identified plasmid-associated antibiotic resistance genes encoding resistance to more than 6 antibiotic classes in 96% of preterm infant gut samples. Furthermore, the preterm infant resistome was found to be highly resilient. Over the course of time represented by the longitudinally sampled resistome, only about one fifth of the resistance gene classes showed significant change, implying that early life antibiotic therapy may select for a lasting expansion of the gut resistome.
The predictable response of the preterm infant gut microbiota to antibiotic therapy
To quantify changes to the gut microbiota before and after antibiotic treatment Gibson et al. utilized metagenomic shotgun sequencing to interrogate the phylogenetic architecture of the bacterial community. While meropenem, cefotaxime, and ticarcillin-clavulanate each significantly and immediately reduced species richness, gentamicin and vancomycin (2 of the most commonly used antibiotics in preterm infant populations, and which are often co-administered) resulted in variable species richness responses. Using random forests classification, the direction of species richness response to vancomycin and gentamicin treatment can be predicted based on the relative abundance of only 2 species (Staphylococcus aureus and E. coli) and 2 antibiotic resistance genes (cpxR and cpxA) with an error rate of only 15%. We hypothesize that this finding might be due to the innate vancomycin-resistance of Gram-negative bacteria such as E. coli and the innate vancomycin-susceptibility of S. aureus, coupled with the ability of cpxR and cpxA to confer gentamicin resistance to E. coli. Citation45 These analyses reveal significant antibiotic-specific microbial and compositional responses of the preterm infant gut microbiota. For a commonly prescribed antibiotic regimen, co-therapy with vancomycin and gentamicin, Gibson et al. identified important species and antibiotic resistance gene biomarkers that can predict with high accuracy the short-term species richness response and therefore potential for disruption of the developing preterm infant gut microbiota.
Implications for future research
It is evident that use of specific antibiotics in the NICU, such as next generation β-lactam antibiotics, acutely (but perhaps not durably) perturb the gut microbiota while others, such as gentamicin, are less disruptive. Going forward, it is important to understand the enduring effects of antibiotics on the preterm infant gut microbiota and resistome. Recent studies have observed that multidrug resistant clones present in preterm infants in the NICU are absent at 2 y of age, suggesting that the preterm infant gut may recover following discharge to home. Citation46 On the other hand, resistance genes persist for months or years after antibiotic therapy in adults. Citation47-49 Sequence-based characterizations of gut microbiota composition and resistome of preterm infants after they enter the community are needed to fully understand the longitudinal effects of antibiotics on microbiota composition, multidrug resistant organism persistence, and resistance gene carriage. Longitudinal studies also provide the opportunity to correlate dysbioses that originate in the NICU with health outcomes that are manifest later in life. Additionally, it is important to consider the effect of prenatal and intrapartum antibiotics, and prenatal exposure to microbes, on the infant gut microbiota. This is of particular note in preterm infants, 1 in 4 of whom are born to mothers with an intrauterine infection, Citation50 and in light of the increasing recognition that the uterus is a nonsterile environment. It is necessary to validate the results of this study and others outside of the preterm human infant context. Murine models of antibiotic use have been invaluable in informing microbiome research. Humanized gnotobiotic models in particular have benefited the field greatly. Citation10,16,51 Development of a humanized infant mouse model for preterm antibiotic therapy would allow for testing of clinical antibiotic protocols in a controlled environment in order to discern their relative impacts on the developing gut microbiota. Such analyses would build on the foundation laid by Gibson et al. to provide an evidence-base supporting a personalized medicine approach to antibiotic treatment, wherein antibiotic use in preterm infant populations will be tailored to limit collateral microbiota disruptions and selection for resistance.
Abbreviations
CRIB | = | Clinical Risk Index for Babies |
ESBL | = | Extended Spectrum β-Lactamase |
NICU | = | Neonatal intensive care unit |
Disclosure of potential conflicts of interest
No potential conflicts of interest were disclosed.
Funding
The work discussed in this addendum was supported in part by awards to G.D. from the Children's Discovery Institute (MD-II-2011-117 and 127), the March of Dimes Foundation (6-FY12-394), and the National Institute of General Medical Sciences (R01-GM099538) and awards to P.I.T. by the National Institute of Diabetes and Digestive and Kidney Diseases (P30DK052574) and the National Institute of Allergy and Infectious Diseases (UH3AI083265). A.J.G. is supported by the National Institute of General Medical Sciences Cell and Molecular Biology Training Grant (T32 GM007067). T.S.C. is supported by the National Institute of Child Health and Development (T32 HD049305, Kelle H. Moley, Principal Investigator).
References
- Tamburini S, Shen N, Wu HC, Clemente JC. The microbiome in early life: implications for health outcomes. Nat Med 2016; 22:713-22; PMID:27387886; http://dx.doi.org/10.1038/nm.4142
- Aagaard K, Ma J, Antony KM, Ganu R, Petrosino J, Versalovic J. The placenta harbors a unique microbiome. Sci Transl Med 2014; 6:237ra65; PMID:24848255; http://dx.doi.org/10.1126/scitranslmed.3008599
- Yatsunenko T, Rey FE, Manary MJ, Trehan I, Dominguez-Bello MG, Contreras M, Magris M, Hidalgo G, Baldassano RN, Anokhin AP, et al. Human gut microbiome viewed across age and geography. Nature 2012; 486:222-7; PMID:22699611; http://dx.doi.org/10.1038/nature11053
- La Rosa PS, Warner BB, Zhou Y, Weinstock GM, Sodergren E, Hall-Moore CM, Stevens HJ, Bennett WE, Jr., Shaikh N, Linneman LA, et al. Patterned progression of bacterial populations in the premature infant gut. Pro Natl Acad Sci U S A 2014; 111:12522-7; PMID:25114261; http://dx.doi.org/10.1073/pnas.1409497111
- Backhed F, Roswall J, Peng Y, Feng Q, Jia H, Kovatcheva-Datchary P, Li Y, Xia Y, Xie H, Zhong H, et al. Dynamics and Stabilization of the Human Gut Microbiome during the First Year of Life. Cell Host Microbe 2015; 17:690-703; PMID:25974306; http://dx.doi.org/10.1016/j.chom.2015.04.004
- Subramanian S, Huq S, Yatsunenko T, Haque R, Mahfuz M, Alam MA, Benezra A, DeStefano J, Meier MF, Muegge BD, et al. Persistent gut microbiota immaturity in malnourished Bangladeshi children. Nature 2014; 510:417-21; PMID:24896187
- Favier CF, Vaughan EE, De Vos WM, Akkermans AD. Molecular monitoring of succession of bacterial communities in human neonates. Appl Environ Microbiol 2002; 68:219-26; PMID:11772630; http://dx.doi.org/10.1128/AEM.68.1.219-226.2002
- Koenig JE, Spor A, Scalfone N, Fricker AD, Stombaugh J, Knight R, Angenent LT, Ley RE. Succession of microbial consortia in the developing infant gut microbiome. Pro Natl Acad Sci U S A 2011; 108 Suppl 1:4578-85; PMID:20668239; http://dx.doi.org/10.1073/pnas.1000081107
- Mshvildadze M, Neu J, Mai V. Intestinal microbiota development in the premature neonate: establishment of a lasting commensal relationship? Nutr Rev 2008; 66:658-63; PMID:19019028; http://dx.doi.org/10.1111/j.1753-4887.2008.00119.x
- Cox LM, Yamanishi S, Sohn J, Alekseyenko AV, Leung JM, Cho I, Kim SG, Li H, Gao Z, Mahana D, et al. Altering the intestinal microbiota during a critical developmental window has lasting metabolic consequences. Cell 2014; 158:705-21; PMID:25126780; http://dx.doi.org/10.1016/j.cell.2014.05.052
- Clarke G, O'Mahony SM, Dinan TG, Cryan JF. Priming for health: gut microbiota acquired in early life regulates physiology, brain and behaviour. Acta Paediatr 2014; 103:812-9; PMID:24798884; http://dx.doi.org/10.1111/apa.12674
- Vatanen T, Kostic AD, d'Hennezel E, Siljander H, Franzosa EA, Yassour M, Kolde R, Vlamakis H, Arthur TD, Hamalainen AM, et al. Variation in Microbiome LPS Immunogenicity Contributes to Autoimmunity in Humans. Cell 2016; 165:842-53; PMID:27133167; http://dx.doi.org/10.1016/j.cell.2016.04.007
- Vangay P, Ward T, Gerber JS, Knights D. Antibiotics, pediatric dysbiosis, and disease. Cell Host Microbe 2015; 17:553-64; PMID:25974298; http://dx.doi.org/10.1016/j.chom.2015.04.006
- Hsiao EY, McBride SW, Hsien S, Sharon G, Hyde ER, McCue T, Codelli JA, Chow J, Reisman SE, Petrosino JF, et al. Microbiota modulate behavioral and physiological abnormalities associated with neurodevelopmental disorders. Cell 2013; 155:1451-63; PMID:24315484; http://dx.doi.org/10.1016/j.cell.2013.11.024
- Chai G, Governale L, McMahon AW, Trinidad JP, Staffa J, Murphy D. Trends of outpatient prescription drug utilization in US children, 2002-2010. Pediatrics 2012; 130:23-31; PMID:22711728; http://dx.doi.org/10.1542/peds.2011-2879
- Nobel YR, Cox LM, Kirigin FF, Bokulich NA, Yamanishi S, Teitler I, Chung J, Sohn J, Barber CM, Goldfarb DS, et al. Metabolic and metagenomic outcomes from early-life pulsed antibiotic treatment. Nat Commun 2015; 6:7486; PMID:26123276; http://dx.doi.org/10.1038/ncomms8486
- Gibson MK, Crofts TS, Dantas G. Antibiotics and the developing infant gut microbiota and resistome. Curr Opin Microbiol 2015; 27:51-6; PMID:26241507; http://dx.doi.org/10.1016/j.mib.2015.07.007
- Cho I, Yamanishi S, Cox L, Methe BA, Zavadil J, Li K, Gao Z, Mahana D, Raju K, Teitler I, et al. Antibiotics in early life alter the murine colonic microbiome and adiposity. Nature 2012; 488:621-6; PMID:22914093; http://dx.doi.org/10.1038/nature11400
- Trasande L, Blustein J, Liu M, Corwin E, Cox LM, Blaser MJ. Infant antibiotic exposures and early-life body mass. Int J Obes (2005) 2013; 37:16-23; PMID:22907693
- Arrieta MC, Stiemsma LT, Dimitriu PA, Thorson L, Russell S, Yurist-Doutsch S, Kuzeljevic B, Gold MJ, Britton HM, Lefebvre DL, et al. Early infancy microbial and metabolic alterations affect risk of childhood asthma. Sci Transl Med 2015; 7:307ra152; PMID:26424567; http://dx.doi.org/10.1126/scitranslmed.aab2271
- Jernberg C, Lofmark S, Edlund C, Jansson JK. Long-term ecological impacts of antibiotic administration on the human intestinal microbiota. ISME J 2007; 1:56-66; PMID:18043614; http://dx.doi.org/10.1038/ismej.2007.3
- Zhang T, Smith MA, Camp PG, Shajari S, MacLeod SM, Carleton BC. Prescription drug dispensing profiles for one million children: a population-based analysis. Eur J Clin Pharmacol 2013; 69:581-8; PMID:22791273; http://dx.doi.org/10.1007/s00228-012-1343-1
- Clark RH, Bloom BT, Spitzer AR, Gerstmann DR. Reported medication use in the neonatal intensive care unit: data from a large national data set. Pediatrics 2006; 117:1979-87; PMID:16740839; http://dx.doi.org/10.1542/peds.2005-1707
- Hsieh EM, Hornik CP, Clark RH, Laughon MM, Benjamin DK, Jr., Smith PB. Medication use in the neonatal intensive care unit. Am J Perinatol 2014; 31:811-21; PMID:24347262; http://dx.doi.org/10.1055/s-0033-1361933
- Warner BB, Deych E, Zhou Y, Hall-Moore C, Weinstock GM, Sodergren E, Shaikh N, Hoffmann JA, Linneman LA, Hamvas A, et al. Gut bacteria dysbiosis and necrotising enterocolitis in very low birthweight infants: a prospective case-control study. Lancet 2016; PMID:26969089
- Cotten CM, Taylor S, Stoll B, Goldberg RN, Hansen NI, Sanchez PJ, Ambalavanan N, Benjamin DK, Jr. Prolonged duration of initial empirical antibiotic treatment is associated with increased rates of necrotizing enterocolitis and death for extremely low birth weight infants. Pediatrics 2009; 123:58-66; PMID:19117861; http://dx.doi.org/10.1542/peds.2007-3423
- Greenwood C, Morrow AL, Lagomarcino AJ, Altaye M, Taft DH, Yu Z, Newburg DS, Ward DV, Schibler KR. Early empiric antibiotic use in preterm infants is associated with lower bacterial diversity and higher relative abundance of Enterobacter. J Pediatr 2014; 165:23-9; PMID:24529620; http://dx.doi.org/10.1016/j.jpeds.2014.01.010
- Sherman MP. New concepts of microbial translocation in the neonatal intestine: mechanisms and prevention. Clin Perinatol 2010; 37:565-79; PMID:20813271; http://dx.doi.org/10.1016/j.clp.2010.05.006
- Carl MA, Ndao IM, Springman AC, Manning SD, Johnson JR, Johnston BD, Burnham CA, Weinstock ES, Weinstock GM, Wylie TN, et al. Sepsis from the gut: the enteric habitat of bacteria that cause late-onset neonatal bloodstream infections. Clin Infect Dis 2014; 58:1211-8; PMID:24647013; http://dx.doi.org/10.1093/cid/ciu084
- Groer MW, Gregory KE, Louis-Jacques A, Thibeau S, Walker WA. The very low birth weight infant microbiome and childhood health. Birth Defects Res C, Embryo Today 2015; 105:252-64; PMID:26663857; http://dx.doi.org/10.1002/bdrc.21115
- Hamilton BE, Martin JA, Osterman MJK. Births: Preliminary data for 2015. National vital statistics reports; vol 65 no 3. Hyattsville, MD: National Center for Health Statistics. 2016.
- Arboleya S, Sanchez B, Milani C, Duranti S, Solis G, Fernandez N, de los Reyes-Gavilan CG, Ventura M, Margolles A, Gueimonde M. Intestinal microbiota development in preterm neonates and effect of perinatal antibiotics. J Pediatr 2015; 166:538-44; PMID:25444008; http://dx.doi.org/10.1016/j.jpeds.2014.09.041
- Abubucker S, Segata N, Goll J, Schubert AM, Izard J, Cantarel BL, Rodriguez-Mueller B, Zucker J, Thiagarajan M, Henrissat B, et al. Metabolic reconstruction for metagenomic data and its application to the human microbiome. PLoS Comput Biol 2012; 8:e1002358; PMID:22719234
- Brooks B, Firek BA, Miller CS, Sharon I, Thomas BC, Baker R, Morowitz MJ, Banfield JF. Microbes in the neonatal intensive care unit resemble those found in the gut of premature infants. Microbiome 2014; 2:1; PMID:24468033; http://dx.doi.org/10.1186/2049-2618-2-1
- Pehrsson EC, Forsberg KJ, Gibson MK, Ahmadi S, Dantas G. Novel resistance functions uncovered using functional metagenomic investigations of resistance reservoirs. Front Microbiol 2013; 4:145; PMID:23760651; http://dx.doi.org/10.3389/fmicb.2013.00145
- Moore AM, Patel S, Forsberg KJ, Wang B, Bentley G, Razia Y, Qin X, Tarr PI, Dantas G. Pediatric fecal microbiota harbor diverse and novel antibiotic resistance genes. PloS one 2013; 8:e78822; PMID:24236055; http://dx.doi.org/10.1371/journal.pone.0078822
- Gibson MK, Wang B, Ahmadi S, Burnham C-AD, Tarr PI, Warner BB, Dantas G. Developmental dynamics of the preterm infant gut microbiota and antibiotic resistome. Nature Microbiology 2016; 1:16024; http://dx.doi.org/10.1038/nmicrobiol.2016.24
- Segata N, Waldron L, Ballarini A, Narasimhan V, Jousson O, Huttenhower C. Metagenomic microbial community profiling using unique clade-specific marker genes. Nat Methods 2012; 9:811-4; PMID:22688413; http://dx.doi.org/10.1038/nmeth.2066
- Truong DT, Franzosa EA, Tickle TL, Scholz M, Weingart G, Pasolli E, Tett A, Huttenhower C, Segata N. MetaPhlAn2 for enhanced metagenomic taxonomic profiling. Nat Methods 2015; 12:902-3; PMID:26418763; http://dx.doi.org/10.1038/nmeth.3589
- Fujimura KE, Slusher NA, Cabana MD, Lynch SV. Role of the gut microbiota in defining human health. Expert Rev Anti Infect Ther 2010; 8:435-54; PMID:20377338; http://dx.doi.org/10.1586/eri.10.14
- Abrahamsson TR, Jakobsson HE, Andersson AF, Bjorksten B, Engstrand L, Jenmalm MC. Low gut microbiota diversity in early infancy precedes asthma at school age. Clin Exp Allergy 2014; 44:842-50; PMID:24330256; http://dx.doi.org/10.1111/cea.12253
- Riesenfeld CS, Goodman RM, Handelsman J. Uncultured soil bacteria are a reservoir of new antibiotic resistance genes. Environ Microbiol 2004; 6:981-9; PMID:15305923; http://dx.doi.org/10.1111/j.1462-2920.2004.00664.x
- Rosenthal VD, Bijie H, Maki DG, Mehta Y, Apisarnthanarak A, Medeiros EA, Leblebicioglu H, Fisher D, Alvarez-Moreno C, Khader IA, et al. International Nosocomial Infection Control Consortium (INICC) report, data summary of 36 countries, for 2004-2009. Am J Infect Control 2012; 40:396-407; PMID:21908073; http://dx.doi.org/10.1016/j.ajic.2011.05.020
- Harris PN, Tambyah PA, Paterson DL. β-lactam and β-lactamase inhibitor combinations in the treatment of extended-spectrum β-lactamase producing Enterobacteriaceae: time for a reappraisal in the era of few antibiotic options? Lancet Infect Dis 2015; 15:475-85; PMID:25716293; http://dx.doi.org/10.1016/S1473-3099(14)70950-8
- Mahoney TF, Silhavy TJ. The Cpx stress response confers resistance to some, but not all, bactericidal antibiotics. J Bacteriol 2013; 195:1869-74; PMID:23335416; http://dx.doi.org/10.1128/JB.02197-12
- Moles L, Gomez M, Jimenez E, Fernandez L, Bustos G, Chaves F, Canton R, Rodriguez JM, Del Campo R. Preterm infant gut colonization in the neonatal ICU and complete restoration 2 years later. Clin Microbiol Infect 2015; 21:936.e1-10; PMID:26086569; http://dx.doi.org/10.1016/j.cmi.2015.06.003
- Jakobsson HE, Jernberg C, Andersson AF, Sjolund-Karlsson M, Jansson JK, Engstrand L. Short-term antibiotic treatment has differing long-term impacts on the human throat and gut microbiome. PloS one 2010; 5:e9836; PMID:20352091; http://dx.doi.org/10.1371/journal.pone.0009836
- Lofmark S, Jernberg C, Jansson JK, Edlund C. Clindamycin-induced enrichment and long-term persistence of resistant Bacteroides spp. and resistance genes. J Antimicrob Chemother 2006; 58:1160-7; PMID:17046967; http://dx.doi.org/10.1093/jac/dkl420
- Jernberg C, Lofmark S, Edlund C, Jansson JK. Long-term impacts of antibiotic exposure on the human intestinal microbiota. Microbiology 2010; 156:3216-23; PMID:20705661; http://dx.doi.org/10.1099/mic.0.040618-0
- Romero R, Dey SK, Fisher SJ. Preterm labor: one syndrome, many causes. Science 2014; 345:760-5; PMID:25124429; http://dx.doi.org/10.1126/science.1251816
- Turnbaugh PJ, Ridaura VK, Faith JJ, Rey FE, Knight R, Gordon JI. The effect of diet on the human gut microbiome: a metagenomic analysis in humanized gnotobiotic mice. Science Transl Med 2009; 1:6ra14; PMID:20368178; http://dx.doi.org/10.1126/scitranslmed.3000322