Abstract
Clostridium difficile in one of the most commonly reported nosocomial pathogens worldwide. Beyond antibiotic use, little is known about the host, microbiota, and environmental factors that contribute to susceptibility to and severity of C. difficile infection (CDI). We recently observed that in a mouse model of CDI, excess dietary zinc (Zn) alters the gut microbiota and decreases resistance to CDI. Moreover, we determined that high levels of Zn exacerbate C. difficile-associated disease and calprotectin-mediated Zn limitation is an essential host response to infection. In this addendum, we discuss how these findings add to our understanding of CDI and consider the potential implications of excess metal intake on the microbiota and infection.
Keywords:
- Animal models of GI infection or GI-diseases with microbial components
- Antibiotics in treatment of GI diseases
- calprotectin
- Clostridium
- Defining/profiling gut microbiome
- diet
- Host-pathogen interactions
- microbiome
- Mechanisms of pathogenesis of gut microbes
- Role of commensal flora in GI diseases
- Role of gut microbiome in GI disease
- zinc
Introduction
Clostridium difficile is Gram-positive, spore forming bacterium that infects the colon and causes a spectrum of disorders varying in severity from mild diarrhea to fulminant colitis, and in severe cases can lead to deathCitation1. In the United States, C. difficile is the most commonly reported nosocomial pathogen and C. difficile infection (CDI) is an urgent public health threat worldwideCitation1. Over the past decade, incidence and costs associated with CDI have steadily been on the rise and the risk of recurrence is reported to be as high as 20% following initial infection and 60% following multiple prior recurrent infectionsCitation1,Citation2. The primary risk factor for CDI is antimicrobial use, which paradoxically is also the primary treatment for C. difficile, compounding the risk for dangerous and financially burdening cycles of recurrence in many patients. Antibiotics perturb the resident microbial community that inhabit the gastrointestinal tract, termed the gut microbiota, and reduce colonization resistance to C. difficile. The gut microbiota mediates protection against invading pathogens through several mechanisms, including directly competing with pathogenic bacteria for resources and niches, stimulating the host immune response and production of antimicrobial peptides, and altering the gastrointestinal environmentCitation3. Interestingly, the number of non-antibiotic associated CDI cases is on the rise, suggesting that unexplored factors likely play an important role in mediating susceptibility to C. difficileCitation4.
One largely unexplored factor in both gut microbiota homeostasis and C. difficile pathogenesis is metal availability. Metal availability is one of the most critical factors affecting the outcome of host-microbe interactionsCitation5. Pathogens express dedicated metal sequestration systems to acquire these essential metals during infectionCitation5,Citation6. To combat this, the host exploits the pathogen's need for nutrient metals by producing factors that limit metal availability in a process termed nutritional immunity. One factor likely playing a major role in metal availability during infection is diet. Altered dietary metal levels are associated with increased susceptibility to various infections; however, there is a paucity of data on how altered dietary metal levels affect the gut microbiomeCitation6. Alterations in dietary metal intake likely play a major role in gastrointestinal infections, as the availability of these trace nutrients in food likely impacts community dynamics and interspecies competition, as well as host-microbiota and host-pathogen interactions.
Dietary zinc, the gut microbiota, and susceptibility to CDI
In the research article highlighted by this addendum, we sought to define the impact of dietary zinc on the gut microbiota and susceptibility to CDICitation7. To test the effect of dietary zinc on the microbiota, we custom designed three diets with differing levels of zinc. The first diet, intended to model zinc limitation, had no zinc added back to the base diet (Low Zn diet; 0 ppm Zn per gram of diet). The second diet had the minimum recommended value of dietary zinc for a mouse (Control Zn diet; 29 ppm Zn per gram of diet) and the third diet was designed to model excess Zn intake and contained approximately 12-fold the amount of Zn in standard mouse chow (Excess Zn diet; 1,000 ppm Zn per gram of diet). To explore the effect of these various levels of Zn on the structure and stability of the microbial community, we longitudinally sampled feces from mice prior to and throughout a five-week diet manipulation (). Analysis of the microbial communities revealed that the gut microbiota was dramatically altered in conditions of excess dietary Zn. Surprisingly, Zn starvation did not significantly change the structure of the microbiota in our mice relative to the control diet. This observation may be a reflection of our inability to completely remove trace levels of Zn from the diet, water source, and cage bedding, thus allowing the microbiota access to necessary levels of Zn to maintain community homeostasis. Excess Zn-mediated perturbations in the microbiota community occurred as early as one-week following introduction of the excess Zn diet and remained relatively stable over the five-week study. These alterations were highlighted by a significant decrease in alpha-diversity and significant blooms in operational taxonomic units (OTUs) affiliated with the Enterococcus and Clostridium genera and a loss in an OTU affiliated with the Turicibacter genera.
The mechanisms by which excess Zn alters the structure of the gut microbiota remains to be determined, but we postulate that it is a combination of several factors. One mechanism is likely metal toxicity. Although essential for life, metals can be toxic to bacteria at high concentrations through redox activity, inter-metal competition, and mis-metallation of metalloproteinsCitation8. Zn is a redox-inactive metal, but can be highly toxic to bacteria through its ability to mis-metallate proteins and liberate redox-active metals, such as copper and ironCitation9. In the context of a complex microbial community like the gut microbiota, high gastrointestinal Zn levels likely kill off numerous bacterial species and create a competitive advantage for species that are resistant to metal toxicity. Interestingly, some of the most well studied metal-resistant organisms in the gastrointestinal tract are Enterococci. The Enterococcus genus are highly resistant to many metals and are also highly resistant to Zn toxicity, which likely contributes to the blooms we observe in our miceCitation10,Citation11. Our data support the hypothesis that Zn liberates previously filled niches and creates an advantage for commensal Enterococci to thrive in the gut.
Following the observation that excess Zn dramatically impacts the structure of the gut microbiota, we reasoned that susceptibility to C. difficile may also be altered. To test this, following diet manipulation, we treated mice with a low-level dose (0.01 mg/ml) of the broad-spectrum antibiotic, cefoperazone, and infected with C. difficile spores. Under these conditions, mice fed the control and low Zn diet are resistant to C. difficile and no colony forming units were detectable from stool for the duration of the four-day infection. Conversely, mice fed the excess Zn diet were highly colonized one day post-infection and showed the development of severe CDI. These data demonstrate that Zn-mediated perturbations to the microbiota reduce the threshold of antibiotic needed to confer susceptibility to CDI. It is important to note that the significant shift in the microbiota caused by excess Zn was not sufficient to render mice susceptible to C. difficile without the introduction of an additional antibiotic perturbation. We suspect that this observation is likely a reflection of the robust colonization resistance conferred by the murine microbiota coupled with the intrinsic ability of the healthy microbiota to withstand major perturbation. To begin to understand how this low-level perturbation and excess Zn was impacting the ecology of the microbiota, we sequenced the microbiota following this treatment with 0.01 mg/ml cefoperazone. Mice fed the low Zn and control diets showed an alteration in the community in response to antibiotics, but quickly recovered and began to return to baseline after two-days. Following cefoperazone treatment, mice fed the excess Zn diet showed a dramatic loss in alpha-diversity, no recovery to baseline following two-days, and a community composition dominated by a single OTU affiliated with the Enterococcus genus. These observations demonstrate that excess Zn-mediated microbiota alterations not only impact structure, but also impact community stability, decreasing resistance to perturbation and in turn decreasing colonization resistance to C. difficile. Collectively, these data reveal that dietary Zn plays an important role in modulating the gut microbiota and these ecological changes impact susceptibility to CDI.
Excess zinc intake and C. difficile-associated disease
A single strain of C. difficile can cause a wide-spectrum of gastrointestinal disorders, ranging from asymptomatic carriage and mild-diarrhea to fulminant colitis, toxic megacolon, and/or deathCitation12. We reasoned that environmental factors, such as dietary Zn levels, could play a role in governing this broad window of disease severity following CDI. To test the impact of Zn levels on severity of CDI, mice fed the altered Zn diets were treated with a high dose of cefoperazone (0.5 mg/ml) to induce susceptibility in all miceCitation13. Following induction of susceptibility with broad spectrum antibiotics, mice were infected with C. difficile spores from two strains, 630 and R20291. The 630 strain of C. difficile is a ribotype 012 strain that causes mild disease in mice, while the strain R20291 is a ribotype 027 epidemic strain that causes more severe disease in miceCitation14,Citation15. Surprisingly, following infection with C. difficile strain 630, mice fed an excess Zn diet showed severe C. difficile-associated disease that was highlighted by robust epithelial damage, edema, inflammation, and the development of pseudomembranes. This was in stark contrast to mice fed the control diet and infected with C. difficile 630, which had low levels of disease. These results were recapitulated with the divergent epidemic strain R20291, indicating that this was not a strain specific phenomenon. Interestingly, we did not observe a significant difference in C. difficile colony forming units in mice fed the excess Zn diet compared to the control diet. This suggested that the mechanisms by which excess Zn alters disease severity is independent of C. difficile burden.
To identify potential mechanisms by which high levels of Zn in the gastrointestinal tract could exacerbate CDI, we first looked at the primary virulence factors for C. difficile, TcdA and TcdB. These two large and potent toxins are glucosyltransferases that inactivate Rho-GTPases in host cells and cause massive damage to the epithelium, compromise barrier function, and lead to robust inflammation at the site of infectionCitation16. In mice fed the excess Zn diet, we observed significant toxin-mediated cytotoxicity in stool for both strains of C. difficile. This increase in toxin titer in stool likely contributes to this dramatic increase in disease following infection; however, the mechanism by which Zn impacts toxin production and/or toxin activity in C. difficile remains to be elucidated. Interestingly, structural studies have revealed that several C. diffiicle virulence factors require Zn to functionCitation17,Citation18. Most notably, recent work has shown that TcdA and TcdB require Zn ions for their activityCitation17. It is tempting to speculate that availability of nutrient Zn in the gastrointestinal tract may impact the function and activity of C. difficile toxins during infection; however, more studies are needed to test these hypotheses. Notably, future research exploring the impact of altered Zn levels on the activity of TcdA and TcdB will begin to clarify the role of environmental Zn on the pathogenesis of CDI. Moreover, studies exploring the influence of Zn on transcriptional regulation of these toxins will shed light on the effect of altered Zn concentrations in the gut.
Based on the marked impact of excess Zn on the structure of the gut microbiota and the expected loss in barrier function following severe epithelial damage, we hypothesized that microbiota translocation may play a role in disease exacerbation in mice fed a diet high in Zn. To explore this, we harvested livers from mice infected with C. difficile strain R20291 and quantified bacterial burdens. The concept that increased epithelial damage and loss of barrier function is associated with microbiota translocation across the epithelium is not uniqueCitation19. Thus, it was not surprising that mice fed an excess Zn diet harbored higher burdens of commensal organisms in their livers compared to control mice. However, when we sequenced cultivars from livers of mice fed the control diet and high Zn diet with 16S rRNA gene sequencing, we observed noteworthy differences. Isolates from mice fed a control diet classified as Enterobacteriacaea, while isolates from mice fed an excess Zn diet classified as Enterobacteriacaea, Enterococcus, and Clostridium species. Enrichment of members of the Enterococcus genus have been reported in the gut microbiota of patients with CDI and co-infection with Enterococcus is a frequent cause of CDI-related sepsisCitation20. Together, these observations suggest that Zn-mediated alterations in the microbiota may enrich for members of the microbiota that opportunistically invade and may cause more complicated disease.
Nutritional immunity during CDI
During infection, the host produces numerous factors that limit metals at the site of infection in a process termed nutritional immunityCitation21. One such protein factor, calprotectin, controls infection by binding and limiting the nutrient metals zinc (Zn) and manganese (Mn)Citation21,Citation22. Calprotectin is antimicrobial against numerous pathogens, including Staphylococcus aureus, Acinetobacter baumannii, and Helicobacter pyloriCitation21,Citation23-Citation25. Furthermore, calprotectin is a marker for inflammation in the gastrointestinal tract and recent studies, including our own, have demonstrated that calprotectin levels are positively correlated with disease severity in patients with CDICitation7,Citation26,Citation27. During Salmonella Typhimurium infection, calprotectin levels can surpass concentrations of 1.0 mg/ml in the inflamed intestine Citation28. Surprisingly, the contribution of calprotectin during CDI has not been explored prior to our work. To begin to define the impact of calprotectin on C. difficile, we first treated C. difficile with recombinant calprotectin in vitro. Similar to other pathogens, we observed that calprotectin inhibits C. difficile growth at concentrations expected in the inflamed gut (1.0 mg/ml) and this antimicrobial activity is dependent on the ability of this protein to limit Zn and manganese. Using matrix-assisted laser desorption ionization imaging mass spectrometry (MALDI-IMS), we demonstrated that calprotectin is highly abundant in the ceca of mice infected with C. difficile, supporting previous findings in human stool samples and suggesting a role for this protein in the immune response to CDI. To test the importance of calprotectin during CDI, we next infected s100a9-deficient mice with C. difficile strain R20291. Calprotectin is a heterodimer of two S100 proteins, S100A8 and S100A9, thus animals defective in production of S100A9 do not produce functional calprotectin. Following infection, we observed that s100a9-deficient animals became moribund or succumbed to infection by three days post-infection, demonstrating the essentiality of calprotectin during CDI. Beyond the metal binding capacity, calprotectin also has numerous immunomodulatory roles in the hostCitation21. We sought to determine if the ability of calprotectin to limit Zn levels in the gastrointestinal tract was contributing to its essentiality during CDI. To mimic calprotectin metal sequestration during infection, we treated s100a9-deficient mice with the low Zn diet and compared that to s100a9-deficient mice fed a control diet. Mice on a low Zn diet had significantly lower histopathology following infection, suggesting that the ability of calprotectin to limit Zn during infection plays an important role in limiting C. difficile-associated disease.
Interestingly, although s100a9-deficient mice had significantly enhanced disease and decreased survival, we did not detect any difference in C. difficile burden between s100a9-deficient mice and wildtype C57BL/6 mice. Moreover, calprotectin levels are positively correlated with disease severity in patients with CDICitation26,Citation27. These observations combined with our data demonstrating the essentiality of calprotectin during CDI leads to an interesting paradox. Calprotectin is a necessary factor in the host-response to C. difficile, but it is not sufficient to neutralize C. difficile pathology in severe CDI. This suggests that C. difficile must have mechanisms to combat calprotectin during infection and overcome nutritional immunity. This phenomenon is seen in other pathogens, such as Staphylococcus aureus, which express high affinity metal import systems that can overcome host-mediated metal limitation by outcompeting host factors for metalsCitation29. To date, little is known about metal acquisition in C. difficile and the battle for metals during CDI. We believe that the identification of these metal uptake systems could increase our understanding of C. difficile disease and provide novel therapeutic targets for treating CDI. Ongoing work in our laboratory is seeking to define and characterize the factors utilized by C. difficile to compete with the host and microbiota for metals during infection.
Concluding Remarks and Implications
It has long been appreciated that metal availability is an important factor governing the outcome of host-pathogen interactions during infection. Recently, there has been a growing appreciation for the role of diet and dietary metal levels in impacting host-pathogen interactions and susceptibility to infectionsCitation6. For example, increased dietary manganese has been shown to dramatically increase S. aureus infection of the heart and increase mortality in miceCitation30. Moreover, in hamsters, iron limitation increases pathogenesis of H. pylori infection and accelerates gastric carcinogenesisCitation31. The role of diet in CDI has also continued to gain momentum, as dietary trehalose was recently linked to virulence in C. difficile and has been postulated to have played a role in the emergence of epidemic lineages of C. difficileCitation32. In the study highlighted by this addendum, excess Zn was shown to impact the gut microbiota, susceptibility to CDI, and C. difficile-associated disease. At present, many of the mechanisms defining the impact of Zn on the microbiota and C. difficile remain to be elucidated. However, these findings have clear implications on our understanding of C. difficile pathogenesis and can provide insights into prevention and treatment of CDI in high-risk patients.
In this study, we modeled excess Zn intake by designing a diet that contains approximately twelve-fold the Zn level of normal mouse chow. Moreover, mice were fed an altered Zn diet for a five-week time course. The relevance of this model to dietary Zn intake and Zn supplementation in humans remains to be directly tested. Controlled dietary manipulation studies in humans are needed to validate these results and define the impact of excess Zn on the gut microbiota. Interestingly, patients with inflammatory bowel disease (IBD) have decreased Zn absorption, and thus increased gastrointestinal Zn, and are at high risk of developing CDICitation33,Citation34. Furthermore, patients with IBD are frequently given high-dose Zn supplementation to combat Zn-deficiencyCitation35. We hypothesize that altered levels of gastrointestinal Zn, caused by conditions of decreased Zn absorption, could have a similar impact on the gut microbiota and susceptibility to CDI as excess Zn supplementation in our model. Our group is interested in further exploring these hypotheses in future studies in patients with IBD.
One potentially impactful effect of excess dietary Zn on CDI is the selection for Enterococci in the microbiota. Vancomycin-resistant Enterococcus (VRE) are emerging nosocomial pathogens that colonize the GI tract and can cause numerous infections including bacteremia, endocarditis, and urinary tract infectionsCitation36. Colonization with VRE is associated with more severe C. difficile-associated disease and in patients with acute leukemia, CDI increases the risk of bacteremia due to VRECitation37. Moreover, these pathogens have been shown to transfer conjugative transposons that confer antibiotic resistance, making their co-occurrence particularly worrisomeCitation38. We hypothesize that excess Zn could create a competitive advantage for Enterococci in the gut and the combination of excess Zn and antibiotics may increase rates of VRE and C. difficile co-infection. Co-infection with these two pathogens could increase pathology and promote the transfer of antibiotic resistance. Further studies are needed to define the impact of Zn on co-infection and the role of commensal or pathogenic Enterococci in CDI.
In agriculture, metal supplemented diets are frequently utilized to administer drugs, provide nutrients and prevent deficiencies, and increase size and output of animalsCitation39. In support of our study in mice, recent studies have also suggested that high dietary Zn has an unforeseen impact on the structure of the gut microbiota of livestockCitation40. Furthermore, it has been shown that Zn and copper supplementation in livestock feed increases the proportion of antibiotic resistant bacteria, such as E. coli and Enterococci, in the gutCitation41. This is particularly alarming, as C. difficile has emerged over the past decade as a major cause of infection and mortality in livestock, such as pigsCitation42. Although Zn supplementation is essential to avoid Zn deficiency in livestock, it is possible that excess Zn supplementation in livestock feed could contribute to increasing rates of C. difficile colonization. Although there is no direct evidence for the role of Zn supplementation and CDI carriage in food animals, further studies are warranted to explore this potential.
Together, this study helps further our understanding of the environmental and host factors associated with CDI and begins to shed light on the previously unappreciated battle for metals during CDI. Furthermore, these findings and the points highlighted in this addendum provide the basis for shaping future prevention and treatment strategies for CDI. Alteration in diet and limitation of excess Zn intake may prove efficacious for the prevention of CDI in high-risk patients and livestock, and may help limit morbidity during CDI.
Figure 1. Schematic of dietary zinc manipulation and Clostridium difficile infection. C57BL/6 mice are given altered diets for 5-weeks and treated with cefoperazone in their drinking water for 5 days. Following 2-days of recovery, mice are infected with C. difficile via oral gavage. Fresh fecal samples are collected for microbiota analysis during diet manipulation and antibiotic treatment, and fecal samples are utilized to monitor bacterial burden and toxin titers following infection.
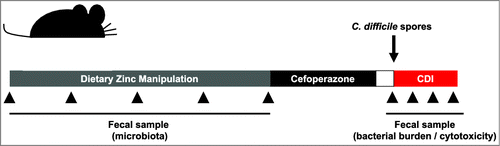
References
- Lessa FC, Mu Y, Bamberg WM, Beldavs ZG, Dumyati GK, Dunn JR, Farley MM, Holzbauer SM, Meek JI, Phipps EC, et al. Burden of Clostridium difficile infection in the United States. N Engl J Med. 2015;372:825–834.
- Aslam S, Hamill RJ, Musher DM. Treatment of Clostridium difficile-associated disease: old therapies and new strategies. Lancet Infect Dis. 2005;5:549–557.
- Buffie CG, Pamer EG. Microbiota-mediated colonization resistance against intestinal pathogens. Nat Rev Immunol. 2013;13:790–801.
- Rupnik M, Wilcox MH, Gerding DN. Clostridium difficile infection: new developments in epidemiology and pathogenesis. Nat Rev Microbiol. 2009;7:526–536.
- Hood MI, Skaar EP. Nutritional immunity: transition metals at the pathogen-host interface. Nat Rev Microbiol. 2012;10:525–537.
- Palmer LD, Skaar EP. Transition Metals and Virulence in Bacteria. Annu Rev Genet. 2016;50:67–91.
- Zackular JP, Moore JL, Jordan AT, Juttukonda LJ, Noto MJ, Nicholson MR, Crews JD, Semler MW, Zhang Y, Ware LB, et al. Dietary zinc alters the microbiota and decreases resistance to Clostridium difficile infection. Nat Med. 2016;22:1330–1334.
- Barwinska-Sendra A, Waldron KJ. The Role of Intermetal Competition and Mis-Metalation in Metal Toxicity. Adv Microb Physiol. 2017;70:315–379.
- Chandrangsu P, Helmann JD. Intracellular Zn(II) Intoxication Leads to Dysregulation of the PerR Regulon Resulting in Heme Toxicity in Bacillus subtilis. PLoS Genet. 2016;12, e1006515.
- Abrantes MC, Lopes Mde F, Kok J. Impact of manganese, copper and zinc ions on the transcriptome of the nosocomial pathogen Enterococcus faecalis V583. PLoS One. 2011;6, e26519.
- Ramsey M, Hartke A, Huycke M. The Physiology and Metabolism of Enterococci. In Gilmore MS, Clewell DB, Ike Y, Shankar N, eds. Enterococci: From Commensals to Leading Causes of Drug Resistant Infection. Boston; 2014.
- Kelly CP, LaMont JT. Clostridium difficile infection. Annu Rev Med. 1998;49:375–390.
- Theriot CM, Koumpouras CC, Carlson PE, Bergin II, Aronoff DM, Young VB. Cefoperazone-treated mice as an experimental platform to assess differential virulence of Clostridium difficile strains. Gut Microbes. 2011;2:326–334.
- Wust J, Sullivan NM, Hardegger U, Wilkins TD. Investigation of an Outbreak of Antibiotic-Associated Colitis by Various Typing Methods. Journal of Clinical Microbiology. 1982;16:1096–1101.
- Stabler RA, He M, Dawson L, Martin M, Valiente E, Corton C, Lawley TD, Sebaihia M, Quail MA, Rose G, Gerding DN, et al. Comparative genome and phenotypic analysis of Clostridium difficile 027 strains provides insight into the evolution of a hypervirulent bacterium. Genome Biol. 2009;10:R102.
- Voth DE, Ballard JD. Clostridium difficile toxins: mechanism of action and role in disease. Clin Microbiol Rev. 2005;18:247–263.
- Chumbler NM, Rutherford SA, Zhang Z, Farrow MA, Lisher JP, Farquhar E, Giedroc DP, Spiller BW, Melnyk RA, Lacy DB. Crystal structure of Clostridium difficile toxin A. Nature Microbiology. 2016;1:15002.
- Rubino JT, Martinelli M, Cantini F, Castagnetti A, Leuzzi R, Banci L, Scarselli M. Structural characterization of zinc-bound Zmp1, a zinc-dependent metalloprotease secreted by Clostridium difficile. J Biol Inorg Chem. 2016;21:185–196.
- Hasegawa M, Kamada N, Jiao Y, Liu MZ, Núñez G, Inohara N. Protective role of commensals against Clostridium difficile infection via an IL-1beta-mediated positive-feedback loop. J Immunol .2012;189:3085–3091.
- Poduval, R.D., Kamath, R.P., Corpuz, M., Norkus, E.P. & Pitchumoni, C.S. Clostridium difficile and vancomycin-resistant Enterococcus: the new nosocomial alliance. Am J Gastroenterol. 2000;95:3513–3515.
- Zackular, J.P., Chazin, W.J. & Skaar, E.P. Nutritional Immunity: S100 Proteins at the Host-Pathogen Interface. J Biol Chem. 2015;290:18991–18998.
- Damo SM, Kehl-Fie TE, Sugitani N, Holt ME, Rathi S, Murphy WJ, Zhang Y, Betz C, Hench L, Fritz G, et al. Molecular basis for manganese sequestration by calprotectin and roles in the innate immune response to invading bacterial pathogens. Proc Natl Acad Sci U S A. 2013;110:3841–3846.
- Hood MI, Mortensen BL, Moore JL, Zhang Y, Kehl-Fie TE, Sugitani N, Chazin WJ, Caprioli RM, Skaar EP. Identification of an Acinetobacter baumannii zinc acquisition system that facilitates resistance to calprotectin-mediated zinc sequestration. PLoS Pathog. 2012;8, e1003068.
- Kehl-Fie TE, Chitayat S, Hood MI, Damo S, Restrepo N, Garcia C, Munro KA, Chazin WJ, Skaar EP. Nutrient metal sequestration by calprotectin inhibits bacterial superoxide defense, enhancing neutrophil killing of Staphylococcus aureus. Cell Host Microbe. 2011;10:158–164.
- Gaddy JA, Radin JN, Loh JT, Piazuelo MB, Kehl-Fie TE, Delgado AG, Ilca FT, Peek RM, Cover TL, Chazin WJ, et al. The host protein calprotectin modulates the Helicobacter pylori cag type IV secretion system via zinc sequestration. PLoS Pathog. 2014;10, e1004450.
- Hanania A, Jiang, Zhi-Dong, Smiley, Casey, Lasco, Todd, Garey, Kevin W, DuPont, Herbert L. Fecal Calprotectin in the Diagnosis of Clostridium difficile Infection. Infectious Diseases in Clinical Practice. 2016;24:31–34.
- Rao K, Santhosh K, Mogle JA, Higgins PD, Young VB. Elevated fecal calprotectin associates with adverse outcomes from Clostridium difficile infection in older adults. Infect Dis (Lond). 2016 Sep; 48(9):663–669. doi: 10.1080/23744235.2016.1186832.
- Liu JZ, Jellbauer S, Poe AJ, Ton V, Pesciaroli M, Kehl-Fie TE, Restrepo NA, Hosking MP, Edwards RA, Battistoni A, et al. Zinc sequestration by the neutrophil protein calprotectin enhances Salmonella growth in the inflamed gut. Cell Host Microbe. 2012;11:227–239.
- Kehl-Fie TE, Zhang Y, Moore JL, Farrand AJ, Hood MI, Rathi S, Chazin WJ, Caprioli RM, Skaar EP. MntABC and MntH contribute to systemic Staphylococcus aureus infection by competing with calprotectin for nutrient manganese. Infect Immun. 2013;81:3395–3405.
- Juttukonda LJ, Berends ETM, Zackular JP, Moore JL, Stier MT, Zhang Y, Schmitz JE, Beavers WN, Wijers CD, Gilston BA, et al. Dietary Manganese Promotes Staphylococcal Infection of the Heart. Cell Host Microbe. 2017;22(4):531–542.
- Noto JM, Gaddy JA, Lee JY, Piazuelo MB, Friedman DB, Colvin DC, Romero-Gallo J, Suarez G, Loh J, Slaughter JC, et al. Iron deficiency accelerates Helicobacter pylori-induced carcinogenesis in rodents and humans. J Clin Invest. 2013;123:479–492.
- Collins J, Robinson C, Danhof H, Knetsch CW, van Leeuwen HC, Lawley TD, Auchtung JM, Britton RA. Dietary trehalose enhances virulence of epidemic Clostridium difficile. Nature. 2018;553:291–294.
- Ainley C, Cason J, Slavin BM, Wolstencroft RA, Thompson RP. The influence of zinc status and malnutrition on immunological function in Crohn's disease. Gastroenterology. 1991;100:1616–1625.
- Fiocchi C. Inflammatory bowel disease: etiology and pathogenesis. Gastroenterology. 1998;115:182–205.
- Lochs H, Steinhardt HJ, Klaus-Wentz B, Zeitz M, Vogelsang H, Sommer H, Fleig WE, Bauer P, Schirrmeister J, Malchow H. Comparison of enteral nutrition and drug treatment in active Crohn's disease. Results of the European Cooperative Crohn's Disease Study. IV. Gastroenterology. 1991;101:881–888.
- Cetinkaya Y, Falk P, Mayhall CG. Vancomycin-resistant Enterococci. Clin Microbiol Rev. 2000;13:686–707.
- Roghmann MC, McCarter RJ, Brewrink J, Cross AS, Morris JG. Clostridium difficile infection is a risk factor for bacteremia due to vancomycin-resistant Enterococci (VRE) in VRE-colonized patients with acute leukemia. Clin Infect Dis. 1997;25:1056–1059.
- Wasels F, Monot M, Spigaglia P, Barbanti F, Ma L, Bouchier C, Dupuy B, Mastrantonio P. Inter- and intraspecies transfer of a Clostridium difficile conjugative transposon conferring resistance to MLSB. Microb Drug Resist. 2014;20:555–560.
- Nielsen FH. History of zinc in agriculture. Adv Nutr. 2012;3:783–789.
- Starke IC, Pieper R, Neumann K, Zentek J, Vahjen W. The impact of high dietary zinc oxide on the development of the intestinal microbiota in weaned piglets. FEMS Microbiol Ecol. 2014;87:416–427.
- Yazdankhah S, Rudi K, Bernhoft A. Zinc and copper in animal feed – development of resistance and co-resistance to antimicrobial agents in bacteria of animal origin. Microb Ecol Health Dis. 2014;25. doi:10.3402/mehd.v25.25862.
- Songer JG, Anderson MA. Clostridium difficile: an important pathogen of food animals. Anaerobe. 2006;12:1–4.