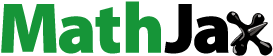
ABSTRACT
Excessive hydrogen sulfide (H2S) production from gut microbial metabolism may have clinically important relevance in the pathogenesis of gut disorders, including ulcerative colitis. However, little is known regarding factors that alter its production. Using a newly-designed in vitro gas-profiling technology, the study aimed to verify real-time H2S measurement reproducibility and thereafter, assess its production following exposure to dietary factors and 5-aminosalicylate acid (5-ASA). Measurements of H2S, carbon dioxide, hydrogen and methane measurements were compared between gas-profiling systems. Homogenized slurries were prepared from freshly-passed healthy human feces. Fifty ml slurries were aliquoted into separate fermentation chambers and substrates added including 1 g highly fermentable fructo-oligosaccharides (FOS) or resistant starch Hi-Maize (RS), or minimally fermentable psyllium or sterculia, 1 g cysteine, 0.9 g sodium sulfate or 1.2 mL of 1 M 5-ASA alone or in combinations. H2S release was sampled every 5 mins over 4-h and expressed relative to unspiked controls. RS suppressed H2S production by a mean 89.0 (SEM 4.8)% and FOS by 82.2 (6.2)% compared to <35 (17)% by psyllium and sterculia (p<0.001, two-way ANOVA). Cysteine stimulated H2S production by 1557 (532)%. The addition of FOS to slurries containing cysteine significantly suppressed H2S by 90 (2)% over the addition of 5-ASA (0.3 (2)%, p<0.001). Sulfate and 5-ASA had minimal overall effects. In conclusion, the H2S-profiling technology is a reproducible tool. Production of H2S is greatly enhanced by sulfur-amino acids but not inorganic sulfate, and is effectively suppressed by readily fermentable fibers. These findings inform potential designs of dietary therapies to reduce H2S production in vivo.
Introduction
Alterations in colonic microbial metabolism of gaseous end-products have been implicated in the development of ulcerative colitis (UC). Evidence for such observations include elevated luminal hydrogen sulfide (H2S) concentrations driven either by increased metabolism of sulfur-containing substrates or impaired detoxification mechanisms.Citation1 Prolonged exposure of colonocytes to excessive luminal H2S and nitric oxide, another toxic gas, produce biochemical lesions in the mucosal lining described in UC.Citation2 Indeed, one of the possible mechanisms of 5-aminosalicylic acid (5-ASA), a first-line drug therapy, in inducing remission in UC, is the inhibition of H2S production.Citation3.
It is well known that microbial fermentation of undigested carbohydrates yield hydrogen (H2), methane (CH4) and carbon dioxide (CO2).Citation4 On the contrary, H2S production reflects either fermentation of sulfur-amino acids or the reduction of inorganic sulfate.Citation5 The relative contributions of each microbial pathway towards luminal H2S production is not well documented. Additionally, the contribution of other dietary factors to H2S production require further investigation. Limited data suggest that readily fermentable fibers, such as resistant starch and fructo-oligosaccharides (FOS) may influence H2S production by shifting fermentation away from sulfur-amino acids,Citation6,Citation7 Since H2S may be pathogenic in UC and its microbial pathways are potentially modulable by diet, this gas may be a valuable biomarker to assess the success of dietary interventions in correcting functional dysbiosis in UC. Evidence that such a dietary strategy may be efficacious is limited to a single report in which patients with active UC had marked clinical and biochemical improvement after dietary restriction of sulfur-amino acids and inorganic sulfate.Citation8 Whilst this dietary approach was targeted at modulating the involvement of H2S in pathogenic mechanisms of UC, a strategy was needed for assessing whether target end-points were achieved.
A key limitation in the design of new dietary therapies that target luminal H2S is the ability to measure its production and, perhaps more importantly, changes associated with such interventions. Existing measurement techniques in vivo and in vitro are either limited by volatility of H2S and, therefore, poorly reliable faecal assays.Citation9 or direct and unpleasant rectal sampling procedures.Citation10 In order to address these research gaps, we have developed a novel in vitro gas-profiling technology that enables accurate measurement of multiple gases in real time. The gas profiler, shown in , is composed of three different compartments – a fermentation chamber, an array of gas sensors capable of simultaneous measurements of CO2, CH4, H2 and H2S, and a pressure sensor that assesses total gas production. The gas profiler has advantages over previous in vitro fermentation techniques where simultaneous measurements of individual gas production has been difficult, and dynamics and kinetics of gas production cannot be obtained.Citation11 The other significant benefit of the system is the ability to monitor oxygen levels in the gas chamber's headspace using an oxygen sensor to achieve an nearly oxygen-deplete anaerobic environment (>98% oxygen-free gas). As such, the new technology allows for qualitative prediction of the kinetics of microbial activity in a rapid, convenient and well-controlled environment.
We hypothesize that, with the invention of this novel gas-profiling technology, gas profiles generated are accurate, reproducible and comparable to existing data from the literature for carbohydrate fermentation; and that the technology can be applied to assess changes in the metabolism of colonic microbiota in faecal samples when their microenvironment is modulated by relevant dietary substrates and drugs. Specifically, sulfur-containing amino acids such as cysteine, and inorganic sulfate promote colonic H2S production, while its production will be suppressed by fermentation of readily fermentable fibers, FOS and resistant starch, over poorly fermentable fibers, psyllium and sterculia, and exposure to 5-ASA. Thus, the aims of the present study are first to validate measurements of the in vitro gas-profiling system for overall and individual gas constituents (H2, CH4, CO2 and H2S) in faecal samples; secondly, to determine its utility to provide kinetic data on gas production; thirdly, to examine its experimental utility in identifying factors in the diet that might modulate H2S production; and fourthly, to use 5-ASA as a model drug for its ability to modulate ex-vivo production of H2S.
Results
Reproducibility of gas measurements
shows the inter-variability of three gas-profiling modules using the unspiked faecal sample from one donor. Variations in individual gas measurements between modules were minimal over the 2.5 h period. The coefficient variations for individual sensors at 2.5 h were 2% for H2, 5.7% for CO2 and 1.6% for H2S. shows the reproducibility of gas measurements across three replicate experiments with resistant starch. Measurements between experiments were consistent for total gas, H2, CO2 production and H2S suppression over 4 h (coefficients of variation: total gas 2.5%; CO2 1.9%; H2 4.6%; H2S suppression 5.2%).
Figure 2. Repeatability of gas measurements between individual gas-profiling modules using an unspiked faecal samples of an individual donor. Data for CH4 was not displayed as the individual was not a methane producer.
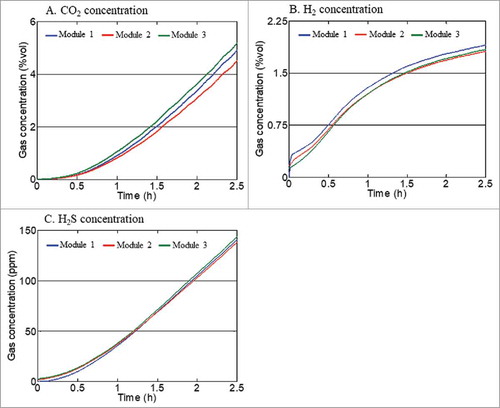
Figure 3. Reproducibility of gas measurements between three replicate fermentation experiments of pooled slurries from three faecal donors using resistant starch as a substrate: (A) Total gas production; (B) CO2 production; (C) H2S suppression or (D) H2 production relative to an unspiked faecal slurries.
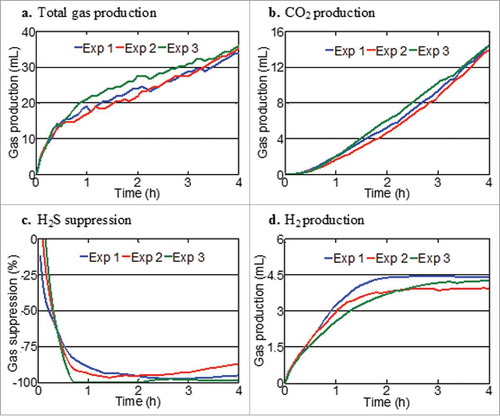
Subjects
Seven healthy controls (3 males, 4 females) were enrolled in the study. The mean age of these volunteers was 34 [range: 23 – 56] years old. Median 4-h H2S production from unspiked faecal slurries of all seven volunteers was documented to be 64.6 [11.3-202.9] µL.
Fermentation profiles of the fibers
Four replicate experiments were completed for each fiber, each replicate representing pooled faecal slurries from three healthy volunteers. shows the extent and rate of gas production with different fibers over 4 h of incubation. The volume of each gas produced at the end of the 4-h period in response to varying fibers is also shown in . Mean total gas production was the greatest for FOS, moderate for resistant starch and minimal for both psyllium and sterculia. The production of CO2 and H2 had a rapid onset and was highest in magnitude for FOS followed by resistant starch, but occurred passively and minimally for psyllium or sterculia (). The production of CO2 for all indigestible carbohydrates was about four times higher than that of H2 during the 4-h fermentation period (). Compared to H2, much smaller amounts of CH4 were produced by all volunteers.
Figure 4. Kinetics of gas production over 4 h for the different fiber substrates (A-H). Values are mean (n = 4) ± SEM. Legend: STR, sterculia; PSY, psyllium; FOS, fructo-oligosaccharides; RS, resistant starch. (A-F) The interaction of substrate x time was statistically significant for gas production and rates of CO2, H2 and CH4 (**p<0.001 for the respective gases; Two-way ANOVA), (G) percentage H2S suppression (p<0.01; Two-way ANOVA) and (H) rate of suppression (p<0.01).
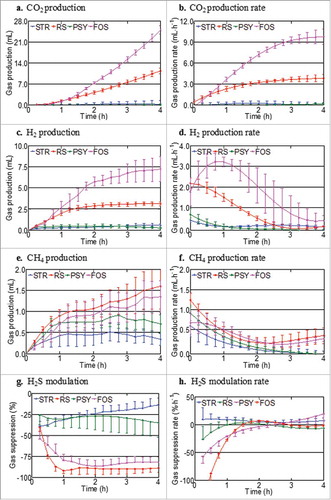
Table 1. The magnitude of gas production at the end of the 4-h experimental period and timing of first significant rise for each fiber substrate.
Modulation of faecal H2S production
Four-hour continuous gas profiling for H2S following the addition of various fiber types is also shown in . The magnitude of H2S suppression throughout the 4-h experimental period was much greater with resistant starch and FOS (p<0.001; two-way ANOVA) than the effects seen for sterculia or psyllium. The kinetics featured in shows two mechanisms of suppression – passive and active. Sterculia and psyllium featured low suppression rates whereas FOS and resistance starch are featured with high suppression rates in the initial 2 h period (p<0.001; two-way ANOVA).
In addition, a total of four replicates from four different subjects were also completed for each of the following substrates. Mean shows the degree and rate of H2S production in response to the addition of cysteine and sulfate relative to an unspiked sample. Stimulation of H2S occurred in a linear fashion for cysteine and, at the end of the 4-h period, mean H2S production had increased by 1557 (532)%. In contrast, the degree of stimulation by sulfate was small (10 (40)%) at the end of the 4-h period.
Figure 5. The degree of H2S modulation over 4 h in response to the addition of various stimulatory substrates (A & B) and combinations of fructo-oligosaccharides (FOS) or 5-aminosalicylate (5-ASA) with cysteine relative to cysteine alone (C & D). Values are mean (n = 4) ± SEM. (A & B). Legend: CYS, cysteine; SSL, sodium sulfate; The interaction of substrate x time was statistically significant between cysteine and sulfate (p<0.001; Two-way ANOVA).(C & D) The reduction in H2S over time was significantly different for cysteine and FOS in comparison to either cysteine alone or in combination with 5-ASA (p<0.001; Two-way ANOVA).
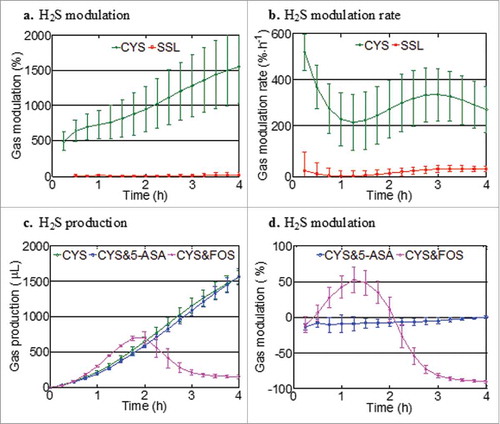
The addition of 5-ASA to faecal slurries produced an initial suppressive effect in H2S production that tapered off as the experiment progressed. At the end of 4 h, the degree of H2S suppression was minimal (-7(30) %).
Effects of fermentable fiber and 5-ASA on cysteine-stimulated H2S production
shows the mean 4-h H2S profile following stimulation with cysteine and concurrent additions of 5-ASA and FOS. In the presence of cysteine, the addition of FOS significantly reduced H2S production particularly after 2.5 h but no changes were found with 5-ASA at any time point throughout the experiment. Percentage H2S suppression at the end of the 4-h period was 90 (2) % for both cysteine and FOS vs 0.3 (2) % for 5-ASA and cysteine.
The 4-h suppression of H2S with the addition of cysteine and FOS was associated with a much greater mean 4-h production of CO2 (68 (16) ml) and hydrogen (19 (4) ml) than the addition of cysteine alone (CO2: 1 (0.8) ml; H2: 0.2 (0.2) mL) or with 5-ASA (CO2: -1.4 (1.0) ml, p = 0.03; H2: 0.5 (0.1) ml, p = 0.02; one-way ANOVA). On the other hand, as shown in , methane production was relatively minimal throughout the 4-h period, in comparison to H2S.
Discussion
The profile of gases produced in the colon depend not only on the functional capabilities of the unique microbiota of the individual, but also by the nature of the substrates to which the bacteria are exposed. Specific gases such as H2S can influence the physiology and pathology of the colon.Citation12 For example, at lower luminal concentrations, H2S has been seen to promote resolution of inflammation in rat studiesCitation13 but at higher concentrations, activates pro-inflammatory pathways leading to the development of colitis.Citation14 Thus, the quantification of its production in vivo and in response to changing substrate availability provide potentially important clinical information. However, difficulties in accessing luminal gaseous content and production rate in vivo have limited this line of enquiry. The novel gas-profiling technology utilized in this study was developed to enable simultaneous, real-time measurement of a spectrum of gases produced by the faecal bacteria to improve current understanding of the interactions of substrates with end-products of likely pathogenic significance. Indeed, our pilot experimentation has demonstrated the potential of the system to be a novel tool in studying H2S production and its modulation in the colon, a previously almost inaccessible target.
The H2S-sensing technique in the current study was able to overcome existing challenges in sampling and measuring H2S. First, despite the volatile nature of H2S, its release was measured in a strictly anaerobic environment with minimal variability between the three gas-profiling modules or across experiments. The sensitivity and specificity of measurements by the gas-sensing technology has already been demonstrated in an earlier study.Citation15 Furthermore, faecal H2S measurements in unspiked samples of volunteers are comparable to other reported measurements in faecal slurries in healthy controls.Citation16 Secondly, the system was able to measure free H2S released into the gaseous headspace, a crucial ability as adsorption by sampling materials can result in reduced H2S recovery.Citation17 Exposure to air can also reduce H2S levels appreciably.Citation16 and this necessitates the rapid determination of H2S under anaerobic conditions when using gas chromatography or high-performance liquid chromatography. Thirdly, we have demonstrated the experimental utility of the gas-profiling technology by describing gas production kinetics when faecal microbiota were exposed to different common fiber supplements as a model with physiological relevance.
The two major pathways of H2S production by the colonic microbiota comprise fermentation of amino acid or dissimilatory sulfate reduction utilizing hydrogen as an electron donor.Citation18 The current study found that the sulfur-amino acid, cysteine, was a more powerful and reliable stimulator of H2S production than inorganic sulfur. This information could be used to speculate that, quantitatively, sulfate reduction in the large bowel by sulfate-reducing bacteria may not be the major pathway of generating free luminal H2S, although further experiments may be needed to examine factors that increase its activity. It is possible that a larger quantity of sulfate is needed to stimulate a larger response of H2S in our study. Lewis et al.Citation19 observed an almost 2-fold increase in faecal sulfide concentrations following a 14-day dietary supplementation with 20 mmol/day of sodium sulfate in a small cohort of healthy volunteers.Citation20 but their findings likely reflected an increase in both free and bound H2S levels. In contrast, our findings are in agreement with those of two other studies. Levine et al.Citation16 observed a 300 -fold stimulation of free faecal H2S production by cysteine relative to that of an unspiked faecal slurry and this was much greater than the two fold stimulation with sodium sulfate at the end of the 24-h experiment.Citation16 Similarly, Florin et al.Citation21 found sulfate to be a poor stimulant of H2S production in vitro compared to cysteine, or another sulfur-containing substrate, mucin. One of the reasons for the ineffective production of H2S by sulfate could be related to the greater presence of protein-fermenting bacterial species within the colonic microbiota, whereas the process of sulfate-reduction is highly specialized and limited to the less abundant sulfate-reducing bacteria. Hence, it may be prudent for dietary strategies to reduce excessive H2S production in the large bowel to target a reduction in the fermentation of sulfur-amino acids rather than sulfate-reduction.
The administration of 5-ASA has previously been postulated to inhibit the genesis of luminal H2S. Initial observations were based on the lower content of total faecal sulfide (both bound and unbound) in patients with active UC and who were taking 5-ASA than those not on 5-ASA therapy. The inhibitory effects of 5-ASA appear to be mediated by interfering with both the rate of dissimilatory sulfate reduction and, to a lesser extent, the fermentation of sulfur-amino acids. This has been demonstrated in two studies in vitro with a reduction of 30–60% in H2S production being observed after 18-h incubation periods with 20 mmol/L 5-ASA.Citation3,Citation22 one with faecal cultures of amino-acid fermenting bacteria.Citation22 and the other measuring dissimilatory sulfate-reduction rates. Somewhat in contrast, we did not see such potent inhibitory effects at the end of our 4-h experiments whether with the incubations of faecal slurries was with 5-ASA alone or in combination with cysteine. Reasons for this discrepancy could potentially lie in the differences in experimental time courses or the methodology employed for H2S measurement. A longer incubation period could have provided more time for adsorption of H2S by 5-ASA compared to our experiments. Alternatively, 5-ASA may exert its effects mostly on bound H2S as seen in the earlier studies,Citation3,Citation22 which may be less relevant to its pathogenicity. This suggests that, in patients with UC, the efficacy of 5-ASA therapy is less likely to be via reduction in metabolically active H2S in the large bowel.
Of great interest, and potential therapeutic importance, are the findings that resistant starch and, to a lesser extent, FOS, modulate H2S production in vitro. Only a few studies have observed such effects of these fermentable fibers on reducing bacterial H2S production. In in vitro fermentation experiments using colonic content of pigs as the inoculum.Citation23 both inulin and FOS added to cecal content (as the ‘control’ substrate) reduced H2S levels by 15% and 5% respectively at the end of 24 h. Lewis and co-workers.Citation19 also observed a reduction in faecal sulfide concentrations following a 12-day supplementation with oligofructose, a type of FOS, in healthy controls. No changes in concentrations of faecal sulfate, populations of sulfate-reducing bacteria or sulfate-reducing rates were observed in their study. It is possible that the mechanism of action for oligofructose was via reducing fermentation of sulfur-amino acids. This was demonstrated in a study in ruminants where increasing overall carbohydrate fermentation via a decrease in colonic pH effectively decreased ruminant production of H2S.Citation24 This is highly likely as resistant starch and FOS have previously been shown to reduce biomarkers of colonic protein fermentation, including faecal ammonia, phenols and branched-chain fatty acids in animal studies,Citation25,Citation26 and several human interventional studies,Citation27-Citation29 An increase in nitrogen disposal.Citation26 or utilization of nitrogen to support increased bacteria mass following increased carbohydrate fermentation shifts the colonic microbiota away from utilizing protein as substrates for fermentation,Citation27,Citation29 The reduction in protein fermentation by supplying readily fermentable carbohydrate to increase carbohydrate fermentation and thus, divert ‘attention’ of bacteria to protein as a fermentative substrate most likely explains the current findings that, following the addition of FOS to cysteine, the production of H2S is actively and markedly reduced (by 90%) in comparison to cysteine alone. The shift to carbohydrate fermentation was confirmed by a concurrent 12- to 16-fold increase in both CO2 and H2 production during the experiment following the addition of FOS to cysteine compared to cysteine alone. The corollary of this finding is that effective suppression of H2S production by faecal microbiota requires active carbohydrate fermentation with concurrent reduction in protein fermentative activity.
A small effect on H2S suppression was also seen with slowly or poorly fermentable fibers despite minimal participation in bacterial fermentation. As poorly fermentable but highly soluble and viscous carbohydrates, both sterculia and psyllium can exert other gel-forming effects.Citation30 It is quite possible that these carbohydrates ‘mop up’ H2S produced spontaneously by faecal microbiota as they attract water to form a viscous gel within the faecal slurries. This would explain the passive H2S suppression by these fibers. No other data are available to corroborate these observations.
There are several limitations of this study. First, the use of faecal samples provides a representation of fermentative events in the distal colon and will not necessarily reflect events in the proximal colon.Citation31 Secondly, interpretation of the in vitro findings must be interpreted in light of the fate of dietary carbohydrates as they traverse the colon. Orally-ingested FOS and resistant starch, when delivered as a single supplement, are completely fermented in the proximal colon.Citation30 Translating these findings into the in vivo situation will require a strategy that will carry the fermentation of these fibers to the distal colon. Indeed, addition of wheat bran to the same resistant starch used in the present study carries the fermentation distally in rats, pigs and humans,Citation32-Citation34 Thirdly, as the in vitro fermentation method is a closed system, it is unable to simulate the absorptive and detoxification systems occurring in vivo. It is for this reason that the fermentation period was limited to 4 h as pH dropped below 5 following this timeframe. As most metabolic activities of the colonic bacteria change (mostly inhibited) when the pH is 5 or lower.Citation35 the drop in pH below 5 would have significantly affected the metabolic ability of the faecal bacteria in our study.
In conclusion, this simple, proof-of-concept study has demonstrated the development of a novel faecal gas-profiling technology that provides reliable and simultaneous measurements of the highly volatile H2S gas with results comparable to the published literature where available. More importantly, this pilot study has also demonstrated the utility of this gas-profiling system as an assessment tool for predicting factors that modulate H2S production in vivo. Readily fermentable fibers, resistant starch and fructans, were shown to effectively suppress H2S, even in the presence of excessive H2S stimulation with cysteine, compared to poorly fermentable fibers. In addition, not all sulfur-containing substrates stimulate H2S to a similar extent, with fermentation of a sulfur-containing amino acid generating H2S more vigorously than dissimilatory sulfate reduction. On the other hand, 5-ASA exerted minimal inhibitory effects, disproving our initial hypothesis. In this way, the gas profiling system provides a means of assessing individual characteristics and might be very important for studies in which therapeutic strategies to modulate gas production (such as H2S in patients with UC) are being evaluated. It would also be worthwhile utilizing clinical samples from patients with active or quiescent UC to demonstrate the repeatability of these findings. These findings also have significant implications for the design of dietary strategies for the reduction of luminal H2S concentrations as a therapy for ulcerative colitis and even malodorous rectal gas. They suggest the importance of incorporating dietary strategies that increase fermentable fiber intake whilst concurrently reducing consumption foods rich in sulfur-amino acid, but not the restriction of inorganic sulfate or the use of 5ASA. Future studies should investigate faecal H2S responses in patients with UC following dietary manipulation with varying substrates to support the design of therapies that reduce the involvement of H2S in the pathogenesis of UC.
Materials and methods
Assessment of repeatability of gas measurements
Gas-profiling modules were pre-calibrated using an external setup that includes an oxygen-free pressured chamber and a set of factory-calibrated gas-tanks with known concentration of the gases of interest. Each individual gas sensor was calibrated with a fixed gas concentration value and tested against typical concentrations of all common gases found in the colon to ensure specificity of measurements.
The inter-variability of the three gas-profiling modules was also assessed by measuring baseline gas production with unspiked fresh faecal inoculum from one healthy donor, incubated under anaerobic conditions at 37°C for 2.5 h. Intra-variability in measurements between three replicate experiments were also investigated by spiking pooled faecal slurries from three healthy donors with resistant starch under the same anaerobic conditions. Based on validation tests, the upper and lower limits of detection for each gas were determined and expressed as the volume of gas measured relative to the total headspace of the bottle or ‘% vol’.
Subjects
Faecal samples were collected from healthy volunteers with no known gastrointestinal diseases. Volunteers were excluded if they had taken any antibiotics, laxatives or fiber supplements in the preceding one month or probiotics in the three months prior to collection. Volunteers were instructed not to change their diets during the collection period. All subjects provided written informed consent prior to collection of faecal samples. Ethical approval was obtained from Monash University Human Research and Ethics Committee (CF15/2454) for this study.
Substrates
Fiber
One gram of purified fiber substrates varying in degrees of fermentation were chosen. These comprised of FOS (Orafti P-95, Beneo, Belgium) as a rapidly fermentable, short-chain carbohydrate; resistant starch (Hi-Maize®, Ingredion ANZ Pty Ltd, NSW Australia) as moderately fermentable; psyllium (Metamucil, Procter & Gamble Australia Pty Ltd, NSW Australia) as a slowly fermentable fiber; and sterculia (Karaya gum, Sigma-Aldrich Co. LLC) being minimally fermented.Citation30.
Sulfur-containing substrates
Two forms of sulfur-containing substrates were utilized to reflect differences in bacterial metabolism of sulfur-containing amino acid and inorganic sulfate. These comprised of 1.0 g cysteine (Sigma-Aldrich Co. LLC, NSW, Australia), a sulfur-amino acid, and 0.9 g sodium sulfate (Sigma-Aldrich Co. LLC, NSW, Australia), an inorganic sulfate (equivalent to 6.5 mmol). The dose of each substrate was calculated from the amount likely to be delivered to the colon from dietary intake in ileostomy studies,Citation36,Citation37.
5-ASA
A dose of 20 mmol/L of 5-ASA (MW 389.4, Sigma-Aldrich Co. LLC, NSW, Australia) was utilized to reflect the concentration of 5-ASA delivered to the large bowel during maintenance therapy.Citation38.
In vitro fermentation procedure
The in vitro fermentation protocol was adapted from that of Edwards et al.Citation35 Fresh feces were collected into a sealed container, immediately gassed with oxygen-free nitrogen (N2), and kept at 37 °C in a waterbath. Samples from individual donors were used within 1.5 h of passage to ensure that colonic microbiota maintained viability. The inoculum was then prepared by mixing fresh feces with the sodium phosphate buffer to make up a 16% slurry (wt/vol). In the first set of experiments (assessing fermentation profiles of indigestible carbohydrates), the inoculum was initially made of faecal samples from three healthy donors and several replicates with the same fibre substrate was performed. Whilst this is standard procedure for in vitro fermentation experiments.Citation 39 subsequent experiments (second, third and fourth sets) were repeated with faecal slurries from individual donors to capture potential individual variations in faecal bacterial metabolism and if necessary, exclude for outliers.
The mixture was homogenized in a household blender for 2 min under a stream of oxygen-free N2 and filtered through four layers of muslin cloth to remove particulate materials. Aliquots (50 mL) of slurry were transferred to 200 mL bottles containing 10 mL of sodium phosphate buffer alone as a blank control or with test substrate. The headspace of the bottles were gassed with oxygen-free N2 before incubation and then sealed. Each bottle was incubated with a shaking water bath at 37°C and at 50 shakes/minutes.
Gaseous response to dietary substrates or 5-ASA
Gaseous release from unspiked faecal slurries were measured via automated headspace sampling every 5 min over a 4-h period. In vitro experiments were conducted in four separate sets according to the postulated effects on H2S production. The first experimental set comprised spiking with various fiber substrates, the second with sulfur-containing substrates and third set with 5-ASA. Finally, samples were spiked with cysteine to stimulate excessive H2S production as well as with a H2S inhibiting substrate, either FOS or 5-ASA.
Total gas production was measured via a pressure sensor (ANKOMRF Gas Production System, ANKOM technology, Macedon NY) at 5-min intervals over 4 h. The data generated were then converted into volume in mL using the ‘ideal-gas’ and Avogadro's equations. The individual gases (CO2, H2S, H2 and CH4) were also sampled at a similar frequency and duration. Simultaneous measurements of CO2, H2 and CH4 response were used to assess the extent of bacterial fermentation.
Gas production of different gas species was calculated by multiplying the gas concentration at each timepoint with the headspace volume and expressed as volume (mL/g of fiber) after adjustment for any gas production originating from the unspiked faecal inoculum. Cumulative gas production at the end of the 4-h experimental period was used to assess the extent of fermentation. The rate of gas production was calculated using the derivative of the cumulative gas production volume as follows:
Percentage of H2S suppression by each fiber was calculated using the equation below
Statistical analysis
Statistical analyses were performed using SPSS v.22 and MATLAB. Data were presented as mean (SEM) of cumulative gas volume per gram of fiber at different time points. Differences in the kinetics of gas production between substrates over the 4-h period were analyzed using two-way ANOVA. Differences in the percentage of change (whether stimulation or suppression) in gas production at the end of 4-h relative to an unspiked sample were analyzed using one-way ANOVA and, where significant differences identified between substrates, Tukey's multiple comparison analysis was performed.
Declaration of conflict of interest
All authors declare no competing interests in relation to the study.
Financial disclosures
No external funding was received for this study.
Acknowledgements
We would like to sincerely thank the Monash University, Department of Gastroenterology laboratory staff – Kelly Liels, Alex Bogatyrev and Elizabeth Ly for their assistance with the in vitro experiments. We also thank Greg Yelland for statistical support.
References
- Roediger WEW, Moore J, Babidge W. Colonic sulfide in pathogenesis and treatment of ulcerative colitis. Dig Dis Sciences. 1997;42:1571–9. doi:10.1023/A:1018851723920.
- Roediger W, Babidge W. Nitric oxide effect on coloncyte metabolism: Co-action of sulfides and peroxide. Mol Cell biochem. 2000;206:159–67. doi:10.1023/A:1007034417320. PMID:10839206
- Pitcher MCL, Beatty ER, Cummings JH. The contribution of sulphate reducing bacteria and 5-aminosalicylic acid to faecal sulphide in patients with ulcerative colitis. Gut. 2000;46:64–72. doi:10.1136/gut.46.1.64. PMID:10601057
- Cummings JH, Hill MJ, Bone ES, Branch WJ, Jenkins DJ. The effect of meat protein and dietary fiber on colonic function and metabolism. II. Bacterial metabolites in feces and urine. Am J Clin Nutr. 1979;32:2094–101. doi:10.1093/ajcn/32.10.2094. PMID:484528
- Blachier F, Davila AM, Mimoun S, Benetti PH, Atanasiu C, Andriamihaja M, Benamouzig R, Bouillaud F, Tome D. Luminal sulfide and large intestine mucosa: friend or foe? Amino Acids. 2010;39:335–47. doi:10.1007/s00726-009-0445-2. PMID:20020161
- Le Leu RK, Young GP, Hu Y, Winter J, Conlon MA. Dietary red meat aggravates dextran sulfate sodium-induced colitis in mice whereas resistant starch attenuates inflammation. Dig Dis Sci. 2013;58:3475–82. doi:10.1007/s10620-013-2844-1. PMID:23990000
- Yao CK, Muir JG, Gibson PR. Review article: insights into colonic protein fermentation, its modulation and potential health implications. Aliment Pharmacol & Ther. 2016;43:181–96. doi:10.1111/apt.13456.
- Roediger W. Decreased sulphur amino acid intake in ulcerative colitis. Lancet. 1998;351:1555. doi:10.1016/S0140-6736(05)61120-8. PMID:10326542
- Tangerman A. Measurement and biological significance of the volatile sulfur compounds hydrogen sulfide, methanethiol and dimethyl sulfide in various biological matrices. Journal of chromatography B, Analytical technologies in the biomedical and life sciences. 2009;877:3366–77. doi:10.1016/j.jchromb.2009.05.026. PMID:19505855
- Herulf M, Ljung T, Hellström P, Weitzberg E, Lundberg J. Increased luminal nitric oxide in inflammatory bowel disease as shown with a novel minimally invasive method. Scand J Gastroenterol. 1998;33:164–9. doi:10.1080/00365529850166897. PMID:9517527
- Rotbart A, Yao C, Ha N, Chrisp MD, Muir JG, Gibson PR, Kalantar-zadeh K, Ou JZ. Designing an in-vitro gas profiling system for human faecal samples. Sensors and Actuators B: Chemical. 2017;238:754–64. doi:10.1016/j.snb.2016.07.120.
- Pimentel M, Mathur R, Chang C. Gas and the microbiome. Curr Gastroenterol Rep. 2013;15:1–6. doi:10.1007/s11894-013-0356-y.
- Wallace JL, Ferraz JG, Muscara MN. Hydrogen sulfide: an endogenous mediator of resolution of inflammation and injury. Antioxidants & Redox Signaling. 2012;17:58–67. doi:10.1089/ars.2011.4351.
- Beaumont M, Andriamihaja M, Lan A, Khodorova N, Audebert M, Blouin JM, Grauso M, Lancha L, Benetti PH, Benamouzig R, et al. Detrimental effects for colonocytes of an increased exposure to luminal hydrogen sulfide: The adaptive response. Free Radical Biology & Medicine. 2016;93:155–64. doi:10.1016/j.freeradbiomed.2016.01.028.
- Rotbart A, Yao CK, Ha N, Muir J, Gibson P, Kalantar-zadeh K, Ou JZ. Designing an in-vitro gas profiling system for human faecal samples. Sensors and Actuators B. 2017;238:754–64.
- Levine J, Ellis CJ, Furne JK, Springfield J, Levitt MD. Fecal hydrogen sulfide production in ulcerative colitis. Am J Gastroenterol. 1998;93:83–7. doi:10.1111/j.1572-0241.1998.083_c.x. PMID:9448181
- Strocchi A, Furne JK, Levitt MD. A modification of the methylene blue method to measure bacterial sulfide production in feces. J Microbiol Meth. 1992;15:75–82. doi:10.1016/0167-7012(92)90071-B.
- Carbonero F, Benefiel AC, Alizadeh-Ghamsari AH, Gaskins HR. Microbial pathways in colonic sulfur metabolism and links with health and disease. Front physiol. 2012;3:448. doi:10.3389/fphys.2012.00448. PMID:23226130
- Lewis S, Brazier J, Beard D, Nazem N, Proctor D. Effects of metronidazole and oligofructose on faecal concentrations of sulphate-reducing bacteria and their activity in human volunteers. Scand J Gastroenterol. 2005;40:1296–303. doi:10.1080/00365520510023585. PMID:16334439
- Lewis S, Cochrane S. Alteration of sulfate and hydrogen metabolism in the human colon by changing intestinal transit rate. Am J Gastroenterol. 2007;102:624–33. doi:10.1111/j.1572-0241.2006.01020.x. PMID:17156141
- Florin THJ. Hydrogen sulphide and total acid-volatile sulphide in faeces, determined with a direct spectrophotometric method. Clinica Chimica Acta. 1991;196:127–34. doi:10.1016/0009-8981(91)90065-K.
- Edmond LM, Hopkins MJ, Magee EA, Cummings JH. The effect of 5-aminosalicylic acid-containing drugs on sulfide production by sulfate-reducing and amino acid-fermenting bacteria. Inflamm Bowel Dis. 2003;9:10–7. doi:10.1097/00054725-200301000-00002. PMID:12656132
- Deng YF, Di Liao X, Wang Y, Liang JB, Tufarelli V. Prebiotics Mitigate In Vitro Sulfur-Containing Odour Generation in Caecal Content of Pigs. Ital J Anim Sci. 2015;14:3762. doi:10.4081/ijas.2015.3762.
- Morine SJ, Drewnoski ME, Johnson AK, Hansen SL. Determining the influence of dietary roughage concentration and source on ruminal parameters related to sulfur toxicity. J Anim Sci. 2014;92:4068–76. doi:10.2527/jas.2013-6925. PMID:24663190
- Toden S, Bird AR, Topping DL, Conlon MA. Resistant starch attenuates colonic DNA damage induced by higher dietary protein in rats. Nutr Cancer. 2005;51:45–51. doi:10.1207/s15327914nc5101_7. PMID:15749629
- Younes H, Garleb K, Behr S, Remesy C, Demigne C. Fermentable fibers or oligosaccharides reduce urinary nitrogen excretion by increasing urea disposal in the rat cecum. J Nutr. 1995;125:1010–6. PMID:7722679
- Birkett A, Muir J, Phillips J, Jones G, O'Dea K. Resistant starch lowers fecal concentrations of ammonia and phenols in humans. Am J Clin Nutr. 1996;63:766–72. doi:10.1093/ajcn/63.5.766. PMID:8615362
- Geboes KP, De Hertogh G, De Preter V, Luypaerts A, Bammens B, Evenepoel P, Ghoos Y, Geboes K, Rutgeerts P, Verbeke K. The influence of inulin on the absorption of nitrogen and the production of metabolites of protein fermentation in the colon. Br J Nutr. 2006;96:1078–86. doi:10.1017/BJN20061936. PMID:17181883
- Ross AB, Pere-Trepat E, Montoliu I, Martin FP, Collino S, Moco S, Godin JP, Cleroux M, Guy PA, Breton I, et al. A whole-grain-rich diet reduces urinary excretion of markers of protein catabolism and gut microbiota metabolism in healthy men after one week. J Nutr. 2013;143:766–73. doi:10.3945/jn.112.172197. PMID:23616503
- Eswaran S, Muir J, Chey WD. Fiber and functional gastrointestinal disorders. Am J Gastroenterol. 2013;108:718–27. doi:10.1038/ajg.2013.63. PMID:23545709
- Topping DL, Clifton PM. Short-chain fatty acids and human colonic function: roles of resistant starch and nonstarch polysaccharides. Physiol Rev. 2001;81:1031–64. doi:10.1152/physrev.2001.81.3.1031. PMID:11427691
- Govers MJAP, Gannon NJ, Dunshea FR, Gibson PR, Muir JG. Wheat bran affects the site of fermentation of resistant starch and luminal indexes related to colon cancer risk: a study in pigs. Gut. 1999;45:840–7. doi:10.1136/gut.45.6.840. PMID:10562582
- McIntyre A, Young GP, Taranto T, Gibson PR, Ward PB. Different fibers have different regional effects on luminal contents of rat colon. Gastroenterol. 1991;101:1274–81. doi:10.1016/0016-5085(91)90077-X.
- Muir JG, Yeow EG, Keogh J, Pizzey C, Bird AR, Sharpe K, O'Dea K, Macrae FA. Combining wheat bran with resistant starch has more beneficial effects on fecal indexes than does wheat bran alone. Am J Clin Nutr. 2004;79:1020–8. doi:10.1093/ajcn/79.6.1020. PMID:15159232
- Edwards CA, Gibson G, Champ M, Jensen BB, Mathers JC, Nagengast F, Rumney C, Quehl A. In vitro method for quantification of the fermentation of starch by Human faecal bacteria. J Sci Food Agri. 1996;71:209–17. doi:10.1002/(SICI)1097-0010(199606)71:2%3c209::AID-JSFA571%3e3.0.CO;2-4.
- Florin T, Neale G, Gibson GR, Christl SU, Cummings JH. Metabolism of dietary sulphate: absorption and excretion in humans. Gut. 1991;32:766–73. doi:10.1136/gut.32.7.766. PMID:1855683
- Florin TH, Neale G, Cummings JH. The effect of dietary nitrate on nitrate and nitrite excretion in man. Br J Nutr. 1990;64:387–97. doi:10.1079/BJN19900040. PMID:2223742
- Mardini H, Lindsay D, Deighton C, Record C. Effect of polymer coating on faecal recovery of ingested 5-amino salicylic acid in patients with ulcerative colitis. Gut. 1987; 28:1084–9. doi:10.1136/gut.28.9.1084. PMID:3678967
- McBurney M, Thompson L. Effect of human faecal donor on in vitro fermentation variables. Scand J Gastroenterol. 1989;24:359–67. doi:10.3109/00365528909093060. PMID:2544024