ABSTRACT
Desmosomes are junctional protein complexes that confer strong adhesive capacity to adjacent host cells. In a recent study, we showed that enteropathogenic Escherichia coli (EPEC) disrupts desmosomes, weakens cell-cell adhesion and perturbs barrier function of intestinal epithelial (C2BBe) cells. Desmosomal damage was dependent on the EPEC effector protein EspH and its inhibitory effect on Rho GTPases. EspH-mediated Rho inactivation resulted in retraction of keratin intermediate filaments and degradation of desmosomal cadherins. Immunofluorescence studies of EPEC-infected C2BBe cells revealed keratin retraction towards the nucleus coincident with significant cytoplasmic redistribution of the desmosomal cadherin desmoglein-2 (DSG2). In this addendum, we expand on how EPEC-induced keratin retraction leads to loss of DSG2 anchoring at the junctions, and show that maturity of the epithelial cell monolayer impacts the fate of desmosomes during infection.
Desmosomes are protein complexes comprised of desmosomal cadherins, armadillo proteins and plakins, which are stabilized at paracellular junctions by the tethering of intermediate filaments (IF).Citation1 Desmosomes connect the walls of adjacent host cells at multiple discrete regions forming “spot-welds” that provide tensile strength to the cell layer. Much of our current understanding of the formation and maintenance of desmosomes is based on studies on the epidermis and on cardiomyocytes. However, desmosomal plaque composition, as well as spatial organization of cells held together by desmosomes, differ in various tissue types ().Citation2–Citation6 In contrast to the stratified epithelial cells of the skin and the branched myocytes of the heart, a single layer of simple columnar epithelial cells blankets the intestinal mucosa. Moreover, while all desmosomal cadherin isoforms are expressed in the skin albeit in different combinations and abundances through the epidermal layers, only desmoglein-2 (DSG2) and desmocolin-2 (DSC2) are found in normal intestinal epithelium and in cardiac muscles.Citation1,Citation5
Figure 1. Schematic comparison of desmosomal plaque composition and spatial organization of cells connected by desmosomes in the intestinal epithelium, skin/epidermis and cardiac muscle. Desmosomes are shown in red.
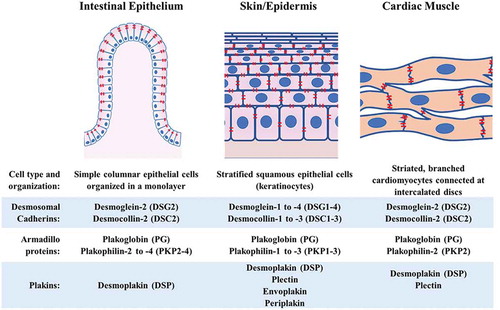
Reflecting this varied composition and tissue topology, desmosomal perturbations have distinct impacts in different tissues. Loss of desmosomal contacts in the skin manifests as blisters due to acantholysis or separation of keratinocytes, as observed in patients suffering from the auto-immune conditions pemphigus vulgaris and pemphigus foliaceus, or from Staphylococcus aureus-induced bullous impetigo.Citation7 Desmosomes are critical in heart morphogenesis and function. Plakophilin-2- or plakoglobin-null mouse embryos die during mid-gestation due to rupturing of cardiac ventricles.Citation8–Citation10 In humans, mutations in desmosome-related genes are strongly linked to arrhythmogenic cardiomyopathy, which is characterized by fibrofatty replacement of the heart muscle, right ventricular arrhythmias and sudden death.Citation4,Citation11–Citation14 In the intestine, desmosomal disruptions could weaken the monolayer and lead to breaches in the epithelial barrier and consequent transfer of microbial components into the underlying tissue. As a virulence strategy, some enteric pathogens, including Entamoeba histolytica, dismantle desmosomes to invade the host.Citation15
We recently reported that enteropathogenic Escherichia coli (EPEC) perturbs desmosomes.Citation16 EPEC effector protein EspH, via its Rho GTPase inhibitory function, induces keratin IF retraction and desmosomal disruption. Beyond EPEC pathogenesis, our studies provide insights on how desmosomes are maintained, particularly in the intestines, and expand our knowledge of the role of Rho GTPases in desmosomal assembly and stability. Impaired Rho GTPase signaling has also been implicated in desmosomal disorders of the heart and the skin.Citation17–Citation19 In the murine heart, inhibition of Rho-kinase, a downstream signaling effector of Rho GTPases, during early cardiac development resulted in junctional abnormalities including less dense desmosomes, widened intercellular gaps at the intercalated discs and translocation of plakoglobin to the nucleus.Citation19 In keratinocytes, Rho GTPase inhibition plays a critical role in the pathophysiology of pemphigus vulgaris and pemphigus foliaceus. Similar to our findings, locking RhoA in the active state via the use of cytotoxic necrotizing factor abolished keratin retraction and the DSG3 loss associated with pemphigus.Citation18
While the exact mechanism by which Rho inactivation leads to desmosomal perturbations remains to be defined, our studies suggest an intersecting role for Rho-mediated modulation of actin in the maintenance of keratin IFs and in the transport of component proteins to the desmosomal plaques. In the prevailing model for IF assembly, the transport of cytokeratin precursors to the periphery, bundling, and maturation towards the cell center is dependent on actin retrograde flow.Citation20,Citation21 Actin cytoskeleton dynamics are controlled by a balance of actin polymerization and depolymerization. Rho activation is known to promote actin polymerization. Consistent with a role for Rho-mediated actin polymerization in the maintenance of keratin IFs in intestinal epithelial cells, we observed that the actin-stabilizing compound jasplakinolide rescues infected cells from EPEC-induced keratin retraction.Citation16
But how does keratin retraction promote desmosomal plaque protein loss? Is the half-desmosome pulled into the cytoplasm while still tethered to the keratin IF, or does keratin dissociate from the desmosome prior to internalization and degradation of the desmosomal plaque components? In our recent study, EPEC-induced keratin retraction occurred simultaneously with cytoplasmic redistribution of DSG2, though some DSG2 remained at the cell junctions of C2BBe cells.Citation16 In those experiments, cells were grown until 3-days post-confluence, and were infected at a multiplicity of infection (MOI) of 100.
We herein speculate that maturity of the desmosomal plaques, specifically desmosomal density at the lateral junctions, and whether the plaques have achieved hyper-adhesive states, dictates the fate of desmosomes during keratin retraction. In vitro, junctional staining of desmosomal cadherins becomes denser as the confluent cell monolayer is cultured longer.Citation22 Desmosomal cadherin dimerization is calcium-dependent in young monolayers (i.e., cell culture that has just reached confluence), but transitions to calcium-independence, and a hyper-adhesive phenotype, in older monolayers.Citation23,Citation24 We hypothesized that keratin retraction in young monolayers would pull the weakly-tethered immature desmosomes into the cytoplasm as half-desmosomes and would track closely with IFs. In contrast, IF retraction in older cells would likely untether keratin from the hyper-adhesive desmosomal plaques; the loss of scaffolding may subsequently result in desmosomal instability at the lateral junctions and internalization of the plaque proteins.
We tested this hypothesis by assessing the impact of EPEC-induced cytokeratin 8/18 retraction on DSG2 localization in young (1-day post-confluence) vs. old (7-days post-confluence) C2BBe monolayers. Uninfected young and old cell monolayers display junctional DSG2 staining and a rich and extensive network of keratin distributed throughout the cells. Both cell monolayers were infected with EPEC at MOI = 100 for 3 hours. In infected young cell monolayers, DSG2 localized at the ends of retracting keratin IFs (). In contrast, mature monolayers infected with EPEC displayed dense DSG2 staining mostly along lateral junctions, unanchored from the retracting keratin filaments. (). Moreover, DSG2 puncta redistributed in the cytoplasm of infected old monolayers did not colocalize with the retracted keratin. Our results indicate that the maturity of the epithelial monolayer impacts how easily EPEC dismantles desmosomes. It should be noted, however, that there was a near-complete loss of DSG2 in most cells by 3–4 hours post-EPEC infection, irrespective of monolayer maturity.Citation16 To gain insight into the underlying mechanistic processes, we specifically focused on the few cells in which DSG2 was still present [, and in the immunofluorescence images in our previous study.Citation16] Collectively, our studies underscore the importance of keratin IF tethering in desmosome plaque stability in the intestinal epithelium, and the potency of RhoGTPase-inactivating compounds such as EPEC EspH in inducing desmosomal damage.
Figure 2. Epithelial monolayer maturity impacts desmoglein-2 (DSG2) redistribution during EPEC infection. Young (A) and old (B) C2BBe monolayers cultured up to 1-day or 7-days post-confluence, respectively, were infected with enteropathogenic Escherichia coli O127:H6 strain E2348/69 at a MOI = 100 for 3 hours. Cartoons depict expected distribution of DSG2 (magenta) and keratin intermediate filaments (green) in adjacent epithelial cells. Lower panels in (A) and (B) show immunofluorescence staining of DSG2 (magenta) and cytokeratin 8/18 (green) in uninfected or EPEC-infected young and old C2BBe monolayers, respectively. DAPI (blue) was used to stain DNA. A broken white line outlines cell junctions.
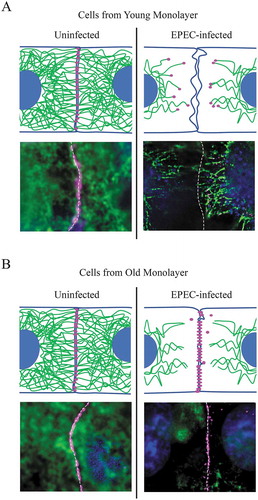
In vivo, younger intestinal epithelial cells are found near progenitor cells at the base of the crypt. As these cells mature and differentiate, they migrate toward the villus tip in small intestines or the flat surface epithelium of the colon. We, thus, speculate that the newly emerging cells from crypts are held together by less mature desmosomal plaques; older cells moving up the crypt-villus axis will likely have mature and hyperadhesive desmosomes. By extending our in vitro model in , it can be inferred that younger enterocytes near crypts are inherently susceptible to desmosomal damage. Not surprisingly, intrinsic enteric defense mechanisms afford increased protection of the more vulnerable cells in the crypt. For instance, Paneth cells, residing within small intestinal crypts, secrete high concentrations of the antimicrobial peptides α-defensins in response to bacterial stimuli.Citation25 In the colon, a dense inner mucus layer, closely attached to the entire colonic epithelium, provides a physical barrier impervious to bacteria.Citation26,Citation27 The inner mucus layer, which progressively transitions into a looser outer mucus layer, is formed by the oligomerization of mucin secreted by goblet cells located throughout the intestinal epithelium.Citation28 Specialized sentinel goblet cells guard the colonic crypt entrance, sample the luminal environment for the presence of microbial components and initiate a signaling cascade to induce mucin secretion from adjacent non-sentinel goblet cells in the upper crypt. Increased mucin secretion, in turn, facilitates the clearance of bacteria surrounding colonic crypts.Citation29 Pathogens must overcome these intrinsic enteric defenses to gain access to the younger enterocytes within the crypts.
While a systematic analysis for tissue tropism of EPEC has not been done, several studies have reported that EPEC can colonize the small and large intestine.Citation30–Citation33 Early electron micrograph images of pig ileum showed attachment of EPEC to both crypt and the lateral regions of villi.Citation32 Weakened intercellular junctions via EspH-mediated desmosomal damage thus likely contributes to enterocyte sloughing and villus shortening during EPEC infection.
Disclosure statement
No relevant conflicts of interest exist for studies reported in this manuscript.
Additional information
Funding
References
- Nekrasova O, Green KJ. Desmosome assembly and dynamics. Trends Cell Biol. 2013;23:537–546.
- Berika M, Garrod D. Desmosomal adhesion in vivo. Cell Commun Adhes. 2014;21:65–75.
- Angst BD, Nilles LA, Green KJ. Desmoplakin II expression is not restricted to stratified epithelia. J Cell Sci. 1990;97:247–257.
- Uzumcu A, Norgett EE, Dindar A, Uyguner O, Nisli K, Kayserili H, Sahin SE, Dupont E, Severs NJ, Leigh IM, et al. Loss of desmoplakin isoform I causes early onset cardiomyopathy and heart failure in a naxos-like syndrome. J Med Genet. 2006;43:e05.
- Holthofer B, Windoffer R, Troyanovsky S, Leube RE. Structure and function of desmosomes. Int Rev Cytol. 2007;264:65–163.
- Li J, Radice GL. A new perspective on intercalated disc organization: implications for heart disease. Dermatol Res Pract. 2010;2010:207835.
- Stanley JR, Amagai M. Pemphigus, bullous impetigo, and the staphylococcal scalded-skin syndrome. N Engl J Med. 2006;355:1800–1810.
- Ruiz P, Brinkmann V, Ledermann B, Behrend M, Grund C, Thalhammer C, Vogel F, Birchmeier C, Gunthert U, Franke WW, et al. Targeted mutation of plakoglobin in mice reveals essential functions of desmosomes in the embryonic heart. J Cell Biol. 1996;135:215–225.
- Grossmann KS, Grund C, Huelsken J, Behrend M, Erdmann B, Franke WW, Birchmeier W. Requirement of plakophilin 2 for heart morphogenesis and cardiac junction formation. J Cell Biol. 2004;167:149–160.
- Bierkamp C, McLaughlin KJ, Schwarz H, Huber O, Kemler R. Embryonic heart and skin defects in mice lacking plakoglobin. Dev Biol. 1996;180:780–785.
- McKenna WJ, Thiene G, Nava A, Fontaliran F, Blomstrom-Lundqvist C, Fontaine G, Camerini F. Diagnosis of arrhythmogenic right ventricular dysplasia/cardiomyopathy. task force of the working group myocardial and pericardial disease of the european society of cardiology and of the scientific council on cardiomyopathies of the international society and federation of cardiology. Br Heart J. 1994;71:215–218.
- Basso C, Bauce B, Corrado D, Thiene G. Pathophysiology of arrhythmogenic cardiomyopathy. Nat Reviews Cardiol. 2011;9:223–233.
- Awad MM, Dalal D, Cho E, Amat-Alarcon N, James C, Tichnell C, Tucker A, Russell SD, Bluemke DA, Dietz HC, et al DSG2 mutations contribute to arrhythmogenic right ventricular dysplasia/cardiomyopathy. Am J Hum Genet. 2006;79:136–142.
- Heuser A, Plovie ER, Ellinor PT, Grossmann KS, Shin JT, Wichter T, Basson CT, Lerman BB, Sasse-Klaassen S, Thierfelder L, et al Mutant desmocollin-2 causes arrhythmogenic right ventricular cardiomyopathy. Am J Hum Genet. 2006;79:1081–1088.
- Ralston KS, Petri WA. Tissue destruction and invasion by Entamoeba histolytica. Trends Parasitol. 2011;27:254–263.
- Roxas JL, Monasky RC, Roxas BAP, Agellon AB, Mansoor A, Kaper JB, Vedantam G, Viswanathan VK. Enteropathogenic Escherichia coli EspH-Mediated Rho GTPase inhibition results in desmosomal perturbations. Cell Mol Gastroenterol Hepatol. 2018;6:163–180.
- Gliem M, Heupel WM, Spindler V, Harms GS, Waschke J. Actin reorganization contributes to loss of cell adhesion in pemphigus vulgaris. Am J Physiol Cell Physiol. 2010;299:C606–13.
- Waschke J, Spindler V, Bruggeman P, Zillikens D, Schmidt G, Drenckhahn D. Inhibition of Rho A activity causes pemphigus skin blistering. J Cell Biol. 2006;175:721–727.
- Ellawindy A, Satoh K, Sunamura S, Kikuchi N, Suzuki K, Minami T, Ikeda S, Tanaka S, Shimizu T, Enkhjargal B, et al Rho-kinase inhibition during early cardiac development causes arrhythmogenic right ventricular cardiomyopathy in mice. Arterioscler Thromb Vasc Biol. 2015;35:2172–2184.
- Kolsch A, Windoffer R, Leube RE. Actin-dependent dynamics of keratin filament precursors. Cell Motil Cytoskeleton. 2009;66:976–985.
- Windoffer R, Beil M, Magin TM, Leube RE. Cytoskeleton in motion: the dynamics of keratin intermediate filaments in epithelia. J Cell Biol. 2011;194:669–678.
- Schlegel N, Meir M, Heupel W-M, Holthöfer B, Leube RE, Waschke J. Desmoglein 2-mediated adhesion is required for intestinal epithelial barrier integrity. Am J Physiology-Gastrointestinal Liver Physiol. 2010;298:G774–G83.
- Harrison OJ, Brasch J, Lasso G, Katsamba PS, Ahlsen G, Honig B, Shapiro L. Structural basis of adhesive binding by desmocollins and desmogleins. Proc Natl Acad Sci U S A. 2016;113:7160–7165.
- Garrod D, Kimura Tomomi E. Hyper-adhesion: a new concept in cell–cell adhesion. Biochem Soc Trans. 2008;36:195–201.
- Ayabe T, Satchell DP, Wilson CL, Parks WC, Selsted ME, Ouellette AJ. Secretion of microbicidal alpha-defensins by intestinal paneth cells in response to bacteria. Nat Immunol. 2000;1:113–118.
- Hansson GC, Johansson ME. The inner of the two Muc2 mucin-dependent mucus layers in colon is devoid of bacteria. Gut Microbes. 2010;1:51–54.
- Johansson ME, Phillipson M, Petersson J, Velcich A, Holm L, Hansson GC. The inner of the two Muc2 mucin-dependent mucus layers in colon is devoid of bacteria. Proc Natl Acad Sci U S A. 2008;105:15064–15069.
- Kim JJ, Khan WI. Goblet cells and mucins: role in innate defense in enteric infections. Pathogens. 2013;2:55–70.
- Birchenough GM, Nystrom EE, Johansson ME, Hansson GC. A sentinel goblet cell guards the colonic crypt by triggering Nlrp6-dependent Muc2 secretion. Sci. 2016;352:1535–1542.
- Peeters JE, Charlier GJ, Halen PH. Pathogenicity of attaching effacing enteropathogenic Escherichia coli isolated from diarrheic suckling and weanling rabbits for newborn rabbits. Infect Immun. 1984;46:690–696.
- Phillips AD, Frankel G. Intimin-mediated tissue specificity in enteropathogenic Escherichia coli interaction with human intestinal organ cultures. J Infect Dis. 2000;181:1496–1500.
- Moon HW, Whipp SC, Argenzio RA, Levine MM, Giannella RA. Attaching and effacing activities of rabbit and human enteropathogenic Escherichia coli in pig and rabbit intestines. Infect Immun. 1983;41:1340–1351.
- Girard F, Dziva F, Stevens MP, Frankel G. Interactions of typical and atypical enteropathogenic Escherichia coli strains with the calf intestinal mucosa ex vivo. Appl Environ Microbiol. 2009;75:5991–5995.