ABSTRACT
The tapeworm Hymenolepis diminuta fails to establish in mice. Given the potential for helminth-bacteria interaction in the gut and the influence that commensal bacteria exert on host immunity, we tested if worm expulsion was related to alterations in the gut microbiota. Specific pathogen-free (SPF) mice, treated with broad-spectrum antibiotics, or germ-free wild-type mice were infected with H. diminuta, gut bacterial composition assessed by 16S rRNA gene sequencing, and worm counts, blood eosinophilia, goblet cells, splenic IL-4, -5 and -10, and colonic cytokines/chemokines mRNA were assessed. Effects of a PBS-soluble extract of adult H. diminuta on bacterial growth in vitro was tested. H. diminuta-infected mice displayed increased α and β diversity in colonic mucosa-associated and fecal bacterial communities, characterized by increased Lachnospiraceae and clostridium cluster XIVa. In vitro analysis revealed that the worm extract promoted the growth of anaerobic bacteria on M2GSC agar. H. diminuta-infection was accompanied by increased Th2 immune responses, and colon from infected mice had increased levels of IL-10, IL-25, Muc2, trefoil factor 3, and β2-defensin mRNA. SPF-mice treated with antibiotics, or germ-free mice, expelled H. diminuta with kinetics similar to control SPF mice. In both settings, measurements of Th2-immune responses were not significantly different across the groups. Thus, while infection with H. diminuta results in subtle but distinct changes to the colonic microbiota, we have no evidence to support an essential role for gut bacteria in the expulsion of the worm from the mouse host.
Introduction
The mammalian intestine houses an immense and complex bacterial community, is the body’s largest repository of immune cells, and can be infected, acutely or chronically, with helminth parasites. This trans-kingdom – bacteria, helminth, host – interaction has co-evolved for millennia and the relationship can be critical in gut homeostasis and overall well-being of the host.Citation1
It is intuitive to accept that helminths residing in the intestine would affect bacteria in this niche; a postulate reinforced by culture-independent sequencing of the small-subunit ribosomal RNA gene (16S) revealing order/family level shifts in bacterial abundance and diversity in helminth-infected humans, rodents and other mammals.Citation2 Acknowledging parasite-host specificity, infection with helminth parasites often results in contraction of Bacteroidetes and expansion of Firmicutes in the hosts’ colon.Citation3 While reports using 16S rRNA sequence analysis describe alterations in the gut microbiome of helminth-infected individuals, the functional consequences of such shifts on the helminth-bacteria relationship and enteric homeostasis remain incompletely understood. Ova hatching and establishment of the whipworm Trichuris muris in the mouse require the presence of commensal bacteria.Citation4 Similarly, it appears that gut bacteria are essential for the nematode Heligmosomoides polygyrus to thrive.Citation5 The protection against airway inflammation evoked by H. polygyrus was attributed to the short-chain fatty acid (SCFA) acetate, produced by the gut microbiota.Citation6
The cestode, Hymenolepis diminuta, unlike many nematodes and trematodes, does not migrate through host tissues, and lacking hooks or abrasive structures it does no obvious damage to the small intestine. Lacking an alimentary track, H. diminuta worm absorbs nutrients across its tegument. H. diminuta successfully establishes in the permissive rat host but is expelled from mice via signal-transducer and activator of transcription (STAT)-6-mediated immunity.Citation7 In rats, H. diminuta reduces the abundance of aerobic bacteria,Citation8 and metagenomic analyses of rat cecal contents or feces revealed subtle changes in the microbiome with a putative bloom of Clostridiales.Citation9–Citation11 Infection with H. diminuta in wild mouse lemurs is associated with a reduction in specific orders of gut bacteria.Citation12
Data on the effects of infection with H. diminuta on the mouse gut microbiota are lacking. Addressing this knowledge gap will advance understanding of the helminth–host interaction and putative mechanisms by which this helminth, or others, can affect mucosal immunity and susceptibility to disease.Citation13,Citation14 Therefore, the current study addresses two questions. First, does infection with H. diminuta evoke shifts in the mouse microbiome? Second, are commensal bacteria required for expulsion of this helminth from a non-permissive mouse host? Juxtaposing the use of a broad-spectrum antibiotic regimen with the use of germ-free mice, we find that mice infected with H. diminuta display a subtle, but significant, increase in bacterial species richness and diversity, characterized by increased Lachnospiraceae; however, the gut bacteria were not needed for the induction of T helper-2 (Th2) immunity or expulsion of the helminth.
Results
Infection with H. diminuta increases diversity of mouse colonic bacteria
Analysis of the V3-V4 region of 16S rRNA gene amplicon sequencing results revealed no difference in the Shannon α-diversity index (i.e. within an individual sample) between fecal samples and colonic tissue of BALB/c mice (Suppl. Figure 1A). A statistically significant difference in overall taxonomic composition between the two sampling sites from uninfected BALB/c mice was revealed by PERMANOVA analysis (Suppl. Figure 1B); however, significant shifts in specific OTUs were not identified using differential abundance testing (DeSeq2). Thus, we opted to examine mucosa-associated bacteria in H. diminuta-infected mice. The Shannon index revealed a significant increase in α-diversity in samples from mice 8 d post-infection (dpi.) () compared to non-infected mice. As expected, treatment with broad-spectrum antibiotics resulted in substantial loss of bacteria as assessed by aerobic culture on blood agar, total 16S rRNA as determined by qPCR, shifted β-diversity (Suppl. Figure 2), and reduced Shannon index (). Analysis of β-diversity using weighted-UniFrac distance and PERMANOVA analysis () (and also with centered log-ratio transformation (CLR) (Suppl. Figure 3)) confirmed a statistically significant (p < .05) effect of H. diminuta-infection with samples from 5, 8 and 11 dpi clustering together and away from controls.
Figure 1. Sequence analysis of the V3-V4 region of the bacterial 16S rRNA gene reveals subtle shifts in the composition of colonic mucosa-associated bacteria following infection with H. diminuta. Male BALB/c mice were infected with 5 H. diminuta and assessed 5, 8 and 11 days post-infection (dpi). (A) Shannon diversity index (α-diversity) (horizontal line, median; black diamond, mean; box plots, 25–75% quartiles; vertical line, minimum and maximum value; * and **, p < .05 and p < .01 compared to control (uninfected). (B) Weighted-Unifrac distance metric for β-diversity, followed by a PERMANOVA test for significance, demonstrates that infection with H. diminuta changes the taxonomic composition of the colonic microbiota. (C) Relative family abundance based on amplicon sequence variants assessment. (D) Differential abundance analysis using DESeq2 comparing control and H. diminuta-infected mice (n = 4–5; * indicated Clostridium cluster XIVa across the time-line). (E) Bacterial colony-forming units (CFU) after 24 h of 37°C anaerobic culture of feces from control and 8-d-infected mice on M2GSC medium agar (n = 7–8 mice from two experiments; *, p < .05; o, order; f, family; g, genus)).
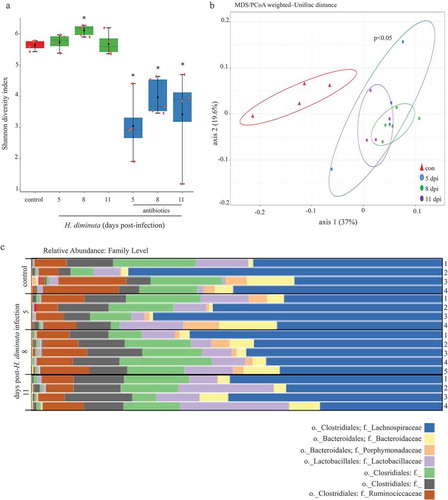
Sequence variant analyses indicated family-level changes in mucosa-associated bacteria in mice infected with H. diminuta for 8 d. Lactobacillaceae and members of the Clostridiales order () increased in abundance following infection with H. diminuta. DESeq2 analysis revealed that the relative sequence abundance of bacteria affiliated with Clostridium cluster XIVa, Lachnospiraceae, and Ruminococcaceae were increased by ~150-, ~130- and 65-fold, respectively (with adjusted p values = .0002, 0.0004, 0.0003, respectively), at 8 dpi (). Increased Lachnospiraceae following infection with H. diminuta was supported by CFU growth on selective M2GSC medium under anaerobic conditions (). H. diminuta-evoked changes in the gut microbiota were not observed in H. diminuta+antibiotic-treated mice, likely being masked by the major impact of the antibiotics (Suppl. Figure 2C).
Despite the subtle difference between mucosal and fecal samples, the latter have the advantage of being able to show changes in a single animal over time. Adopting this approach, we confirmed the increase in gut microbiota α and β diversity in H. diminuta-infected mice compared to time-matched, non-infected controls by comparing feces from the same mouse over time (Suppl. Figure 4).
Hymenolepis diminuta extract (HdE) promotes growth of anaerobic bacteria
Exposure to HdE in vitro did not affect the growth of fecal bacteria grown under aerobic conditions on LB or blood agar (). In contrast, HdE caused a significant bloom of anaerobic bacteria cultured on LB or M2GSC agar (but not blood agar), resulting in a ~3-fold increase in CFU upon treatment with 500 μg/mL of HdE ().
Figure 2. Extract of H. diminuta promotes the growth of murine fecal bacteria. Feces (100 mg) collected from male BALB/c mice (10 weeks old) was suspended in sterile PBS and cultured with a PBS-soluble whole worm extract of H. diminuta (HdE) for 6 h at 37°C, prior to a 24 h (37°C) culture under (A) aerobic or (B) anaerobic conditions on blood, Luteni broth (LB) or M2GSC (specific for anaerobes only) agars. Colony-forming units (CFU) were counted (data are mean± SEM; n = 4–8 mice from 1 to 2 experiments; *, p < .05 compared to 0 HdE).
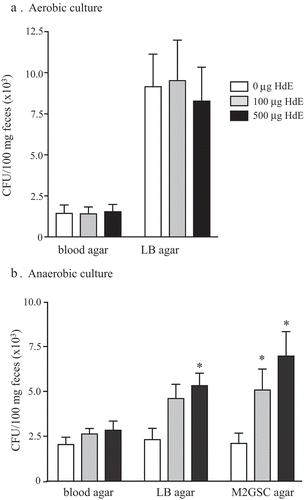
H. diminuta-evoked changes in gut immunity
Comparison of segments of mid-colon from non-infected control BALB/c mice with those from mice infected 8 d previously with H. diminuta revealed significant changes in the expression of mRNA for adaptive and innate immune factors. While Il4 and Il22 expression levels were unchanged, transcription of Il10 and Il25 were increased (). Expression of mRNAs of the goblet cell factors, mucin 2 (Muc2) and trefoil factor 3 (TFF3) were increased, as was that of β-defensin. In contrast, transcription of the antimicrobial C-type lectins, regenerating islet-derived (Reg)IIIβ, and RegIIIγ were unaffected by infection with H. diminuta ().
Figure 3. Infection with H. diminuta increases colonic expression of immune genes. Male BALB/c mice were infected with 5 H. diminuta and 8 days post-infection (dpi) segments of mid-colon were excised, processed and qPCR performed for interleukin (IL)-4, 10, 22 and 25, mucin-2 (Muc2), trefoil factor 3 (TFF3) and the antimicrobial peptides, β2-defensin (β2Def), regenerating islet-derived protein 3 (RegIII) β and γ (data are mean ± SEM; n = 6 mice from 1 experiment; *, p < .05 compared to uninfected control mice).
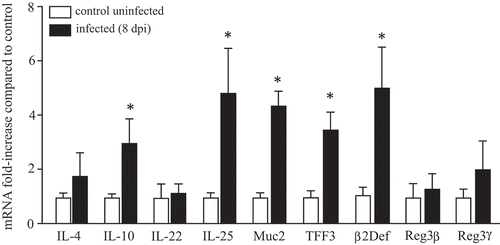
Gut bacteria are not required for expulsion of H. diminuta
BALB/c and C57BL/6 mice mobilize Th2 cytokines and Th2-driven immune effector mechanisms following infection with H. diminuta, and expel a five-worm burden within 9–12 dpi.Citation15 The kinetics of these events was not appreciably different in BALB/c and C57BL/6 mice, with only a marginally quicker response from the male C57BL/6 mice (Suppl. Table 1). Co-treatment of BALB/c mice with broad-spectrum antibiotics did not affect the kinetics of expulsion of H. diminuta, blood eosinophilia, jejunal goblet cell hyperplasia (–) or IL-4, IL-5 or IL-10 from stimulated splenocytes at 5 or 8 dpi (–). Interestingly, splenocytes from infected mice that also received antibiotics had reduced IL-5 output in response to conA stimulation at 11 dpi (, ). Given the lack of effect of antibiotics on worm expulsion, we did not pursue the mechanism(s) or putative significance of the reduced IL-5 at 11 dpi, although it hints at helminth-bacteria cross-talk in the control of systemic immune responses.
Figure 4. Broad-spectrum antibiotic treatment does not affect the expulsion of H. diminuta. Male BALB/c mice were given antibiotic-supplemented drinking water 5 days prior to infection with 5 H. diminuta and maintained on antibiotics until necropsy when (A) worms were counted in small intestinal washings, (B) blood eosinophils counted, (C) jejunal periodic-acid Schiff positive goblet cells counted, and (D-E) concanavalin A (2 μg/mL for 48 h) stimulated cytokine production from spleen cells (5 × 106) measured by ELISA (data are mean ± SEM; n = 4–8 mice from two experiments; dpi, days post-infection; abx, antibiotics; * and **, p < .05 and p < .01 compared to control (con) uninfected mice, respectively; #, p < .05 compared to no abx group).
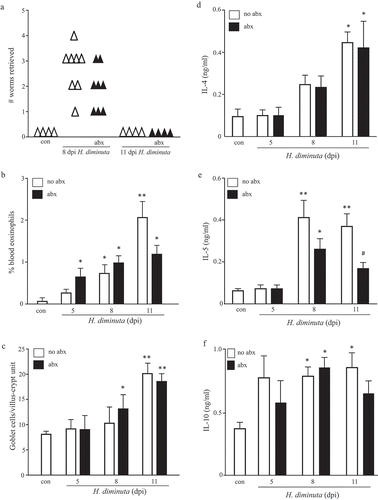
In order to assess whether intestinal microbes are required for successful expulsion of the parasite, GF mice were infected with H. diminuta. To ensure that bacteria were not transferred to the GF mice, cysticercoids were first treated with antibiotics, which had no effect on the ability of the helminth to excyst in vitro (Suppl. Figure 5A), or their ability to infect mice, as demonstrated by successful infection in Il4rα−/- mice that do not expel the worm (Suppl. Figure 5B). 16S rRNA analysis of freshly isolated cysticercoids, antibiotic-treated cysticercoids, and cecal slurry from germ-free mice administered antibiotic-treated cysticercoids confirmed a lack of bacterial contamination of the cysticercoids (Suppl. Figure 5C). Comparison of GF and SPF BALB/c mice revealed similar baseline Th2 parameters (Suppl. Table 2). Similar to SPF and antibiotic-treated SPF mice, H. diminuta failed to establish in GF BALB/c (inset ) or GF C57BL/6 mice () with the majority of GF mice expelling the parasite by 8–11 dpi, although a single mouse bore a stunted worm in the small intestine at 14 dpi. H. diminuta-infected GF C57BL/6 mice displayed blood eosinophilia and jejunal goblet cell hyperplasia (, ), and increased IL-4, IL-5 and IL-10 production by mitogen-stimulated splenocytes (–).
Figure 5. Germ-free mice successfully expel H. diminuta. Male C57BL/6 germ-free mice were infected with 6–8 H. diminuta and on necropsy at 5, 8, 11 and 14 days post-infection (dpi) (A) worms were counted in small intestinal washings (inset: infectivity of germ-free BALB/c mice in the same experimental paradigm), (B) blood eosinophils counted, (C) jejunal periodic-acid Schiff positive goblet cells counted, and (D-E) concanavalin A (2 μg/mL for 48 h) stimulated cytokine production from spleen cells (5 × 106) measured by ELISA (data are mean ± SEM; n = 3 (day 5 and 14) or 8, 1–2 experiments; *, p < .05 compared to control uninfected mice).
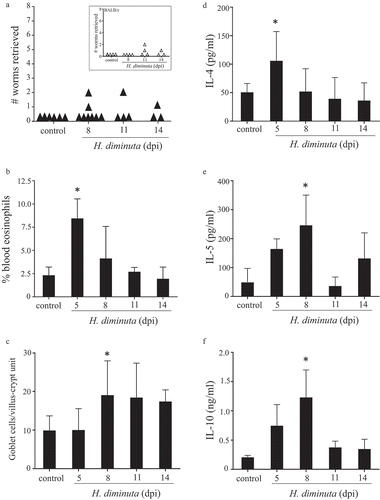
Discussion
Enteric helminths and bacteria that can cause mild to severe disease have been the focus of extensive research to define host immune responses to combat these pathogens and identify mechanisms of immunopathology.Citation16 Thus far, research has typically focused on helminths or bacteria, but seldom both simultaneously.Citation2 This is a simplified approach to a complex ecosystem. It is germane to ask if infection with a helminth affects the composition of the gut bacteria and vice-versa, and the consequences, if any, of helminth-bacteria interaction for host immunity and susceptibility to disease.
Sequencing the V3-V4 region of the bacterial 16S rRNA gene revealed increased complexity of the gut microbiota of H. diminuta-infected male BALB/c mice. These statistically significant changes were characterized by increased Clostridiales (i.e. Firmicutes), specifically Ruminococcaceae and Lachnospiraceae (e.g. Clostridium XIVa). It is difficult to compare between the mouse and rat host with respect to changes in gut bacteria following infection with H. diminuta as the models, kinetics of infection and samples examined (gut, feces, cecal contents) all differ. The data available for the rat suggest that infection with H. diminuta leads to a reduced α-diversity and that there may be an outgrowth of Ruminococcaceae.Citation9–Citation11 Mice infected with the cestode Echinococcus granulosus for 4 months had a largely normal gut microbiota with enrichment of Eisenbergiella and Parabacteroides genera.Citation17 Extending these studies, a burgeoning literature demonstrates shifts in the composition of bacterial communities in the gut of mice, humans and other mammals infected with helminths, primarily nematodes,Citation18–Citation21 but also flukes, such as Schistosoma mansoni, Clonorchis sinensis, and Opisthorchis felineus that do not inhabit the gut.Citation22–Citation24
Two scenarios can explain the H. diminuta-evoked changes in the colonic microbiota. First, it could be a direct effect of products released from the worm. Second, it could be a consequence of helminth-evoked changes in host immunity (the possibility of H. diminuta cysticercoids having bacteria are disputed by the absence of 16S rRNA gene detection in the cysticercoids or cecal contents of infected GF mice). Remarkably, we found that a crude extract of H. diminuta directly stimulated the growth of feces-derived anaerobes cultured on LB- or M2GSC-agar. This is a notable finding and contrasts with the antibiotic effect of H. polygyrus- and Ascaris suum-derived molecules.Citation25,Citation26 Corroborating the direct effect of HdE on bacterial growth, more CFUs grew on M2GSC-agar when feces from H. diminuta-infected mice were cultured anaerobically. Speculating on the nature of the HdE-promoted bacteria, M2GSC agar facilitates the growth of acetate-producing actinobacteria and butyrate-producing Clostridiales. To determine unequivocally how helminth-derived molecules affect bacterial growth will require concerted efforts with multiple media/agars.
The fact that non-gut helminths elicited changes in the colonic microbiota of their hostsCitation24 points to an indirect effect via the host. In accordance with this, the local changes in the transcription of immune genes after infection with H. diminuta (i.e. colon cytokines, muc2, TFF3, β2-defensin), could potentially affect the composition and function of the gut microbiota. Additional studies with knockout mice are needed to determine a functional link between these H. diminuta-evoked immune changes in the colon and the changes in the composition of gut bacteria. These data underscore how a small-intestine dwelling helminth can affect the colon and align with reports of mice infected with H. diminuta being protected from colitis.Citation13
As data continue to accumulate on helminth-evoked changes in the composition of their hosts’ enteric microbiota, the functional significance of these changes needs to be determined. The apparent increase in Lachnospiraceae in H. diminuta-infected mice is noteworthy, because members of this family produce short-chain fatty acids from dietary fiber that affect many aspects of host physiology, including immunity.Citation27 Similarly, increases in the probiotic bacteria Lactobacillus spp. and Bifidobacter spp. were reported in C. sinesis- and Enterobius vermicularis-infected mice, respectively.Citation23,Citation28 Thus, one can hypothesize that helminth-evoked changes in the composition of the gut bacteria affect the helminth–host relationship or concomitant immunity. Neither scenario is unprecedented. For example, hatching of T. muris eggs required a gut microbiota,Citation4 suppression of airways inflammation or improved insulin sensitivity in mice infected with nematodes involved the gut microbiotaCitation6,Citation29 and, contrarily, H. polygyrus-evoked exaggeration of Citrobacter rodentium-induced colitis occurred, at least in part, via the microbiota.Citation30
We assessed a role for the gut bacteria in the expulsion of H. diminuta from mice. Treating mice with broad-spectrum antibiotics did not affect the kinetics of expulsion of H. diminuta, mobilization of local effector cells (e.g. goblet cells) or the development of systemic Th2 responses. In accordance with these findings, antibiotic treatment of S. mansoni-infected C57BL/6 mice did not affect worm burden, eggs per female, or cytokine levels in the spleen; however, serum IgE and mitogen evoked synthesis of IFNγ and IL-10 by mesenteric lymph node cells were reduced.Citation31 Notably the antibiotic+S. mansoni-treated mice had fewer granulomas in the liver and reduced intestinal pathology, suggesting that part of S. mansoni-evoked disease was indirect via bacteria.
The use of antibiotics has two caveats: antibiotics can have non-antibiotic effects (e.g. induction of neutrophil apoptosis or increased peristalsisCitation32,Citation33) and some bacteria remain after treatment. Consequently, we utilized germ-free mice, noting that the immune system in these mice differs from SPF mice, and they may have a propensity to enhanced Th2 immunity.Citation34,Citation35 Increased Th2 immunity in GF mice would predict expedited loss of H. diminuta, although if a microbiota is critical to overall immune development, expulsion could be delayed. While there was variability between the individual GF mice, there was no obvious defect (or enhancement) in their local or systemic immune responses following infection with H. diminuta (compare and ). This translated into effective expulsion of the worm, with the majority of the mice expelling their entire worm burden by 8 dpi, marginally quicker than SPF mice. Thus, we have no data to support enteric bacteria significantly affecting the mobilization of Th2 responses following infection or affecting the expulsion of the parasite.
Data in this area are scant. In accordance with the findings with H. diminuta, the immunological response of GF mice to infection with H. polygyrus was similar to that in SPF mice: worm burdens were not different at 2-weeks post-infection but worms were slightly smaller, and egg output from the infected GF mice was slightly reduced.Citation26 However, 4–6 weeks post-infection, GF mice harbored fewer H. polygyrus (previously designated Nematospiroides dubius) than conventional mice and mono-colonization with Lactobacillus favored survival and development of the helminth.Citation36 Thus, there is host-parasite specificity in the role of gut bacteria in modifying the outcome of the host-parasite interaction, which may be influenced by the amount of tissue damage caused by the helminth that in turn allows bacteria greater access to immune cells in the mucosa.
In summary, having found distinct changes in the composition of the colonic bacteria following infection of mice with the tapeworm H. diminuta (likely a combination of direct effects of molecules released from the worm and indirectly via host immunity), mechanistic studies revealed that gut bacteria were not required for the successful eradication of the helminth. However, this does not dismiss the possibility that the perturbation of the gut microbiota following infection with H. diminuta has other physiological/immunological consequences for the host.Citation37 With the increasing characterization of tri-kingdom interactions, meeting the challenge of defining the functional ramifications for each player – helminth, bacteria, and host – will significantly advance understanding of host–parasite interactions.
Material and methods
Mice and H. diminuta infection
Male BALB/c or C57BL/6 (7–9 weeks old, Charles River Laboratories, Quebec, Canada) and BALB/c Il4rα−/- (colony at Univ. Calgary) mice were housed in HEPA filtered micro-isolator cages with free access to food and water in a temperature-controlled facility with a 12 h:12 h light:dark cycle. Germ-free BALB/c and C57BL/6 mice were bred and maintained in flexible film isolators at the IMC, University of Calgary, Canada. Germ-free status was routinely monitored by culture-dependent and culture-independent methods and all mouse colonies were independently confirmed to be pathogen-free. All experimental procedures were approved from the University of Calgary, Canada, Animal Care Committee and followed the Canadian Council on Animal Care guidelines for the use of animals in biomedical research, under protocol AC17-0115.
Mice under mild manual restraint were infected with five H. diminuta cysticercoids via oral gavage in 200 μL of 0.9% NaCl.Citation38 On necropsy, the small intestine was removed, flushed with PBS, and the intestine opened longitudinally and rinsed in PBS, and the number of worms counted under a dissection microscope. GF mice received 6–8 cysticercoids that had been treated with antibiotics for 2 h (kanamycin 400 mg/L, gentamicin 35 mg/L, colistin 42 mg/L, and metronidazole 215 mg/L). Viability of antibiotic-treated cysticercoids was tested in vitro by induced excystment (exposure to pepsin (1%), trypsin/sodium tauroglycalate (1%) in Hanks solution) and the ability to infect Il4rα-/- mice that do not expel the worm.
Antibiotic (abx) treatment
Mice were administered abx 5 d prior to infection in drinking water, which was maintained throughout the experiment: kanamycin (40 mg/L), gentamicin (3.5 mg/L), colistin (4.2 mg/L), and metronidazole (21.5 mg/L). Abx-water was renewed every 48 h. In addition, mice received an intraperitoneal injection of vancomycin (4.5 mg/kg) 2 d prior to and 3 d after infection with H. diminuta.Citation39
Blood eosinophilia and enteric goblet cells
Blood smears, collected on coded slides, were stained with Wright-Giemsa and, in a blinded fashion, 200 immune cells were counted and percentage eosinophilia determined. A ~ 1 cm segment of mid-ileum was immersion-fixed in 10% formalin, and following dehydration in graded ethanol and clearing in xylene, samples were embedded in paraffin, and 5 μm sections collected onto coded microscope slides. Sections were stained using periodic-acid Schiff’s (PAS) reagent and goblet cells counted in 15 villus-crypt units (VCU)/section.Citation15
Cytokines
Spleen and mesenteric lymph nodes (MLN) were excised aseptically, dispersed into a single cell suspension and red blood cells were lysed in ACK (ammonium-chloride-potassium) lysing buffer. Subsequently, cells (5 × 106/ml in RPMI 1640 medium with 10% FBS and antibiotics (Gibco, USA)) were treated with concanavalin A (2 μg/mL) for 48 h at 37°C.Citation38 Interleukins (IL)-4, −5 and −10 were measured in duplicate samples of the cell-free culture medium by ELISA using paired antibodies following manufacture's instructions (R&D Systems Inc.).
qPCR of gut immune factors
Colonic RNA was isolated using the Aurum Total RNA Mini Kit (Bio-Rad Laboratories, Hercules, Ca) as per the manufacturer’s protocol, quantified with the Nanodrop 1000 Spectrophotometer (Thermo Fisher Scientific, Wilmington, DE), and 0.5 μg of RNA was converted to cDNA using an iScript kit (Bio-Rad Laboratories Canada, Mississauga, ON, Canada). Quantitative real-time polymerase chain reaction (qPCR) of murine colonic tissue was performed as previously described inCitation40 using primer sequences shown in Suppl. Table 3.
Bacterial composition
One gram of proximal colon (or 100 mg feces) was homogenized using 0.2 g of 2.8 mm ceramic beads (Mo Bio Laboratories, #13114-50) in a Bullet Blender (Next Advance (http://www.nextadvance.com)). Samples were homogenized for 3 min (speed setting 10), followed by a 45 sec stop period and then a second centrifugation. Next, 0.2 g of 0.1 mm glass beads was added to the sample and blended for 3 min (speed setting 10). DNA isolation was performed using a previously described protocol.Citation41
Bacterial community profiling was performed through 16S rRNA sequencing. First, forward and reverse barcoded primers of the V3-V4 region (341F-785R) of the bacterial 16S rRNA gene were selected for amplification (Platinum Taq Polymerase, Invitrogen Life Tech)Citation42 and purification prior to undergoing Illumina MiSeq analysis. Adaptor sequences were removed using “cutadapt” (cutadapt.readthedocs.io/en/stable/guide.html). Resulting fastq files were truncated to 250nt in length and reads with a quality score less than 2 were eliminated using the R phyloseq package DADA2 (joey711.github.io/phyloseq/). Taxonomy was assigned to an amplicon sequence variant (ASV) table using the SILVA SSU Ref v.128 classifier. Prior to analyzing the generated microbiome count data, prevalence filtering was performed. In addition, and due to the compositional nature of the data, in another analysis, the centered log-ratio transformation was performed as a follow-up measure to ensure correct assumptions on the original data.Citation43 Following a previously published workflow (f1000research.com/articles/5-1492/v1) diversity indices (Shannon for α-diversity), and distance metrics were generated (weighted unifrac distance and PERMANOVA for β-diversity). Differential abundance analysis was performed using the R program, “DESeq2” (bioconductor.org/packages/release/bioc/html/DESeq2.html) to test for differentially expressed variants between experimental groups.
PCR for the V3-V4 region of the 16S rRNA gene was applied to DNA (as above) extracted from H. diminuta cysticercoids freshly retrieved from beetles, abx-treated cysticercoids and extracts of cecal slurry from germ-free mice that had been infected with abx-treated cysticercoids. Extracts of feces from SPF-mice served as a positive control. Polyacrylamide gel electrophoresis was performed and the PCR product visualized using ethidium bromide.
Preparation of H. diminuta extract (HdE)
H. diminuta antigen was isolated as previously.Citation44 Fifteen live adult H. diminuta worms (removed from small intestines of rats) were rinsed in sterile PBS, treated with gentamicin (1 h, 37°C), rinsed twice in sterile PBS and then homogenized in PBS. Homogenates were centrifuged and the PBS-soluble supernatant was collected. Bradford assay was used to determine protein concentrations.
In vitro culture of bacteria
Fecal samples (100 mg) were collected from naïve mice and placed in 1 mL of sterile PBS. Samples were vortexed for 2 min and centrifuged for 5 min at 8000 x g. Under both aerobic and anaerobic conditions, supernatant (200 μL in replicates of three per sample) was transferred to the first row of a 96 well plate. The three replicates were treated with H. diminuta antigen (100 or 500 μg/mL) and incubated for 6 h at 37°C, plated onto Luteni broth (LB) media, blood and M2GSC media (anaerobic only) agars for 24 h at 37°C in aerobic or anaerobic conditions, after which colony-forming units (CFU) were counted.
Data presentation and analysis
Results are expressed as the mean ± SE (unless stated otherwise) and n is the number of mice. Data are analyzed using Graph Pad Prism 5.0 in which statistical comparisons for parametric data were performed via one-way ANOVA with Tukey’s posttest and the Kruskal–Wallis test with Dunn’s posttest was used for non-parametric data. P< .05 was set as the level of acceptable statistical difference.
Disclosures
The authors declare no conflict of interest.
Supplemental Material
Download Zip (300.9 KB)Acknowledgments
This research was supported by a Natural Sciences and Engineering Research Council of Canada (NSERC) Discovery Grant to DMM. AS was supported by graduate studentships from Beverly Phillips Rising Scholars Award, Snyder Institute, University, NSERC CREATE in Host-Parasite Interactions (HPI), and the Canadian Association of Gastroenterology (CAG). TSJ was supported in part by NSERC CREATE HPI. DMM is recipient of a Canada Research Chair (Tier 1) in Intestinal Immunophysiology in Health and Disease. Support from the International Microbiome Center (IMC) at the University of Calgary (Dr. McCoy) as well as the Canadian Foundation for Innovation to Dr. Strous is gratefully acknowledged (the IMC is supported by the Cumming School of Medicine, University of Calgary, Western Economic Diversification (WED) and Alberta Economic Development and Trade (AEDT), Canada). We thank Dr. M. Workentine (Faculty Veterinary Medicine, Univ. Calgary) for assistance with bioinformatic analyses and Dr. T. Arai for initial help with eosinophil counting.
Supplementary material
Supplemental data for this article can be accessed on the publisher’s website.
Additional information
Funding
References
- Ramanan D, Bowcutt R, Lee SC, Tang MS, Kurtz ZD, Ding Y, Honda K, Gause WC, Blaser MJ, Bonneau RA, et al. Helminth infection promotes colonization resistance via type 2 immunity. Science. 2016;352:608–612. doi:10.1126/science.aaf3229.
- Brosschot TP, Reynolds LA. The impact of a helminth-modified microbiome on host immunity. Mucosal Immunol. 2018;11:1039–1046. doi:10.1038/s41385-018-0008-5.
- McKay DM, Shute A, Lopes F. Helminths and intestinal barrier function. Tissue Barriers. 2017;5:e1283385. doi:10.1080/21688370.2017.1283385.
- Hayes KS, Bancroft AJ, Goldrick M, Portsmouth C, Roberts IS, Grencis RK. Exploitation of the intestinal microflora by the parasitic nematode Trichuris muris. Science. 2010;328:1391–1394. doi:10.1126/science.1187703.
- Reynolds LA, Smith KA, Filbey KJ, Harcus Y, Hewitson JP, Redpath SA, Valdez Y, Yebra MJ, Finlay BB, Maizels RM, et al. Commensal-pathogen interactions in the intestinal tract: lactobacilli promote infection with, and are promoted by, helminth parasites. Gut Microbes. 2014;5:522–532. doi:10.4161/gmic.32155.
- Zaiss MM, Rapin A, Lebon L, Dubey LK, Mosconi I, Sarter K, Piersigilli A, Menin L, Walker A, Rougemont J, et al. The intestinal microbiota contributes to the ability of helminths to modulate allergic inflammation. Immunity. 2015;43:998–1010. doi:10.1016/j.immuni.2015.09.012.
- McKay DM. The immune response to and immunomodulation by Hymenolepis diminuta. Parasitology. 2010;137:385–394. doi:10.1017/S0031182009990886.
- Dwinell MB, Bass P, Schaefer DM, Oaks JA. Tapeworm infection decreases intestinal transit and enteric aerobic bacterial populations. Am J Physiol Gastrointest Liver Physiol. 1997;273:G480–G485. doi:10.1152/ajpgi.1997.273.2.G480.
- McKenney EA, Williamson L, Yoder AD, Rawls JF, Bilbo SD, Parker W. Alteration of the rat cecal microbiome during colonization with the helminth Hymenolepis diminuta. Gut Microbes. 2015;6:182–193. doi:10.1080/19490976.2015.1047128.
- Wegener Parfrey L, Jirku M, Sima R, Jalovecka M, Sak B, Grigore K, Jirků Pomajbíková K. A benign helminth alters the host immune system and the gut microbiota in a rat model system. PLoS One. 2017;12:e0182205. doi:10.1371/journal.pone.0182205.
- Jirku Pomajbikova K, Jirku M, Leva J, Sobotkova K, Morien E, Parfrey LW. The benign helminth Hymenolepis diminuta ameliorates chemically induced colitis in a rat model system. Parasitology. 2018;145:1–12.
- Aivelo T, Norberg A. Parasite-microbiota interactions potentially affect intestinal communities in wild mammals. J Animal Ecol. 2018;87:438–447. doi:10.1111/1365-2656.12708.
- Hunter MM, Wang A, Hirota CL, McKay DM. Neutralizing anti-IL-10 antibody blocks the protective effect of tapeworm infection in a murine model of chemically induced colitis. J Immunol. 2005;174:7368–7375. doi:10.4049/jimmunol.174.11.7368.
- Ledesma-Soto Y, Callejas BE, Terrazas CA, Reyes JL, Espinoza-Jiménez A, González MI, León-Cabrera S, Morales R, Olguin JE, Saavedra R, et al. Extraintestinal helminth infection limits pathology and proinflammatory cytokine expression during DSS-induced ulcerative colitis: a role for alternatively activated macrophages and prostaglandins. BioMed Res Internat. 2015;2015:563425. doi:10.1155/2015/563425.
- McKay DM, Halton DW, McCaigue MD, Johnston CF, Fairweather I, Shaw C. Hymenolepis diminuta: intestinal goblet cell response to infection in male C57 mice. Exp Parasitol. 1990;71:9–20. doi:10.1016/0014-4894(90)90003-U.
- Grencis RK. Immunity to helminths: resistance, regulation, and susceptibility to gastrointestinal nematodes. Ann Rev Immunol. 2015;33:201–225. doi:10.1146/annurev-immunol-032713-120218.
- Bao J, Zheng H, Wang Y, Zheng X, He L, Qi W, Wang T, Guo B, Guo G, Zhang Z, et al. Echinococcus granulosus infection results in an increase in Eisenbergiella and Parabacteroides genera in the gut of mice. Front Microbiol. 2018;9:2890. doi:10.3389/fmicb.2018.02890.
- Jenkins TP,Formenti F, Castro C, Piubelli C, Perandin F, Buonfrate D, Otranto D, Griffin JL, Krause L, Bisoffi Z, et al. A comprehensive analysis of the faecal microbiome and metabolome of Strongyloides stercoralis infected volunteers from a non-endemic area. Scient Rep. 2018;8:15651. doi:10.1038/s41598-018-33937-3.
- Wu S, Li RW, Li W, Beshah E, Dawson HD, Urban JF Jr. Worm burden-dependent disruption of the porcine colon microbiota by Trichuris suis infection. PLoS One. 2012;7:e35470. doi:10.1371/journal.pone.0035470.
- Walk ST, Blum AM, Ewing SA, Weinstock JV, Young VB. Alteration of the murine gut microbiota during infection with the parasitic helminth Heligmosomoides polygyrus. Inflam Bowel Dis. 2010;16:1841–1849. doi:10.1002/ibd.21299.
- Rosa BA,Supali T, Gankpala L, Djuardi Y, Sartono E, Zhou Y, Fischer K, Martin J, Tyagi R, Bolay FK, et al. Differential human gut microbiome assemblages during soil-transmitted helminth infections in Indonesia and Liberia. Microbiome. 2018;6:33. doi:10.1186/s40168-018-0416-5.
- Jenkins TP,Peachey LE, Ajami NJ, MacDonald AS, Hsieh MH, Brindley PJ, Cantacessi C, Rinaldi G. Schistosoma mansoni infection is associated with quantitative and qualitative modifications of the mammalian intestinal microbiota. Scient Rep. 2018;8:12072. doi:10.1038/s41598-018-30412-x.
- Kim JY,Kim Em, Yi MH, Lee J, Lee S, Hwang Y, Yong D, Sohn WM, Yong TS. Chinese liver fluke Clonorchis sinensis infection changes the gut microbiome and increases probiotic Lactobacillus in mice. Parasitol Res. 2019;118:693–699. doi:10.1007/s00436-018-6179-x.
- Saltykova IV, Petrov VA, Logacheva MD, Ivanova PG, Merzlikin NV, Sazonov AE, Ogorodova LM, Brindley PJ. Biliary microbiota, gallstone disease and infection with Opisthorchis felineus. PLoS Negl Trop Dis. 2016;10:e0004809. doi:10.1371/journal.pntd.0004809.
- Midha A, Janek K, Niewienda A, Henklein P, Guenther S, Serra DO, Schlosser J, Hengge R, Hartmann S. The intestinal roundworm Ascaris suum releases antimicrobial factors which interfere with bacterial growth and biofilm formation. Front Cell Infect Microbiol. 2018;8:271. doi:10.3389/fcimb.2018.00271.
- Rausch S, Midha A, Kuhring M, Affinass N, Radonic A, Kuhl AA, Bleich A, Renard BY, Hartmann S. Parasitic nematodes exert antimicrobial activity and benefit from microbiota-driven support for host immune regulation. Front Immunol. 2018;9:2282. doi:10.3389/fimmu.2018.02282.
- Chang PV, Hao L, Offermanns S, Medzhitov R. The microbial metabolite butyrate regulates intestinal macrophage function via histone deacetylase inhibition. Proc Nat Acad Sci (USA). 2014;111:2247–2252. doi:10.1073/pnas.1322269111.
- Yang CA, Liang C, Lin CL, Hsiao CT, Peng CT, Lin HC. Impact of Enterobius vermicularis infection and mebendazole treatment on intestinal microbiota and host immune response. PLoS Negl Trop Dis. 2017;11:e0005963. doi:10.1371/journal.pntd.0005963.
- Pace F, Carvalho BM, Zanotto TM, Santos A, Guadagnini D, Silva KLC, Mendes MCS, Rocha GZ, Alegretti SM, Santos GA, et al. Helminth infection in mice improves insulin sensitivity via modulation of gut microbiota and fatty acid metabolism. Pharmacol Res. 2018;132:33–46. doi:10.1016/j.phrs.2018.04.008.
- Su C, Su L, Li Y, Long SR, Chang J, Zhang W, Walker WA, Xavier RJ, Cherayil BJ, Shi HN. Helminth-induced alterations of the gut microbiota exacerbate bacterial colitis. Mucosal Immunol. 2018;11:144–157. doi:10.1038/mi.2017.20.
- Holzscheiter M, Layland LE, Loffredo-Verde E, Mair K, Vogelmann R, Langer R, Wagner H, Prazeres da Costa C. Lack of host gut microbiota alters immune responses and intestinal granuloma formation during schistosomiasis. Clin Exp Immunol. 2014;175:246–257. doi:10.1111/cei.12230.
- Moges R, De Lamache DD, Sajedy S, Renaux BS, Hollenberg MD, Muench G, Abbott EM, Buret AG. Anti-inflammatory benefits of antibiotics: tylvalosin induces apoptosis of porcine neutrophils and macrophages, promotes efferocytosis, and inhibits pro-inflammatory CXCL-8, IL1α, and LTB4 production, while inducing the release of pro-resolving lipoxin A4 and resolvin D1. Front Vet Sci. 2018;5:57. doi:10.3389/fvets.2018.00040.
- Broad J, Sanger GJ. The antibiotic azithromycin is a motilin receptor agonist in human stomach: comparison with erythromycin. Brit J Pharmacol. 2013;168:1859–1867. doi:10.1111/bph.12077.
- Macpherson AJ, McCoy KD. Standardised animal models of host microbial mutualism. Mucosal Immunol. 2015;8:476–486. doi:10.1038/mi.2014.113.
- Kennedy EA, King KY, Baldridge MT. Mouse microbiota models: comparing germ-free mice and antibiotics treatment as tools for modifying gut bacteria. Front Physiol. 2018;9:1534. doi:10.3389/fphys.2018.01534.
- Chang J, Wescott RB. Infectivity, fecundity, and survival of Nematospiroides dubius in gnotobiotic mice. Exp Parasitol. 1972;32:327–334. doi:10.1016/0014-4894(72)90060-4.
- Giacomin PR, Siracusa MC, Walsh KP, Grencis RK, Kubo M, Comeau MR, Artis D. Thymic stromal lymphopoietin-dependent basophils promote Th2 cytokine responses following intestinal helminth infection. J Immunol. 2012;189:4371–4378. doi:10.4049/jimmunol.1200691.
- Reyes JL, Fernando MR, Lopes F, Leung G, Mancini NL, Matisz CE, Wang A, McKay DM. IL-22 restrains tapeworm-mediated protection against experimental colitis via regulation of IL-25 expression. PLoS Pathog. 2016;12:e1005481. doi:10.1371/journal.ppat.1005481.
- Lopes F, Wang A, Smyth D, Reyes J-L, Doering A, Schenck LP, Beck P, Waterhouse C, McKay DM. The Src kinase Fyn is protective in acute chemical-induced colitis and promotes recovery from disease. J Leukocyte Biol. 2015;97:1089–1099. doi:10.1189/jlb.3A0814-405RR.
- Leung G, Petri B, Reyes JL, Wang A, Iannuzzi J, McKay DM. Cryopreserved IL-4-treated macrophages attenuate murine colitis in an integrin beta7-dependent manner. Mol Med. 2015;21:924–926. doi:10.2119/molmed.2015.00193.
- Heirali A, McKeon S, Purighalla S, Storey DG, Rossi L, Costilhes G, Drews SJ, Rabin HR, Surette MG, Parkins MD, et al. Assessment of the microbial constituents of the home environment of individuals with cystic fibrosis (CF) and their association with lower airways infections. PLoS One. 2016;11:e0148534. doi:10.1371/journal.pone.0148534.
- Klindworth A, Pruesse E, Schweer T, Peplies J, Quast C, Horn M, Glöckner FO. Evaluation of general 16S ribosomal RNA gene PCR primers for classical and next-generation sequencing-based diversity studies. Nucleic Acids Res. 2013;41:e1. doi:10.1093/nar/gks808.
- Aitchison J. The statistical analysis of compositional data. London (UK): Published by Chapman and Hall; 1986.
- Wang A, McKay DM. Immune modulation by a high molecular weight fraction from the rat tapeworm Hymenolepis diminuta. Parasitology. 2005;130:575–585. doi:10.1017/S0031182004006985.