ABSTRACT
Probiotic bacteria are increasingly gaining importance in human nutrition owing to their multifaceted health beneficial effects. Studies have also shown that probiotic supplementation is useful in mitigating age-associated oxi-inflammatory stress, immunosenescence, and gut dysbiosis thereby promoting health and longevity. However, our current understanding of the process of aging suggests a strong interrelationship between the accumulation of senescent cells and the development of aging phenotype, including the predisposition to age-related disorders. The present review studies the documented pro-longevity effects of probiotics and highlights how these beneficial attributes of probiotics could be related to the mitigation of cellular senescence. We present a perspective that to fully understand and comprehend the anti-aging characteristics of probiotic bacteria; it is imperative that probiotics or their synbiotic amalgamation with plant polyphenols, be studied under the purview of cellular senescence, that may ultimately help devise probiotic-based anti-senescence strategies.
Introduction
Human aging is a stochastic, deleterious and progressive phenomenon that renders elderly prone to increasing morbidity and mortality. However, aging itself is not a disease and several attempts have been made to understand its origin, causes and effects considering both genetic and epigenetic factors.Citation1 Amongst these, the free radical theory of aging has gained considerable attention as it emphasizes that the underlying cause of organismal aging is related to the cellular accumulation of harmful and partially reduced metabolites of molecular oxygen due to dysfunctions in various metabolic processes.Citation2,Citation3 Since then, different extensions of the original theory have been proposed including the recent concept of ‘oxi-inflamm-aging’ that highlights the role of immune cells as chief sources of chronic oxidative and inflammatory stress which ultimately contribute to the accelerated rate of aging of organisms.Citation4 Another landmark observation by HayflickCitation5 propagated the notion that aging could be associated with the inherent tendency of somatic cells to resist proliferation or undergo ‘senescence’ after a finite number of divisions in vitro. While this phenomenon was subsequently attributed to gradual telomere attrition (replicative senescence); it is now understood that senescence can be accelerated and induced in cells through different extrinsic as well as intrinsic insults such as oxidative and genotoxic stress,Citation6,Citation7 oncogenic activation,Citation8 or irradiation.Citation9 In particular, the accumulation of reactive oxygen species (ROS) has been implicated as an important mediator of different aspects of cellular senescence due to their ability to not only disrupt cellular oxidative and inflammatory homeostasis but to also accelerate telomere attrition.Citation10-Citation15 However, Hayflick’s observations were initially dismissed as mere in vitro artifact and the connection between limited cell proliferative capacity and aging largely remained controversial.Citation16,Citation17 This scenario now appears to be changing as convincing data are emerging which not only confirm the in vivo presence of senescent cells, but that their gradual accumulation directly contributes to organ dysfunction and the characteristic-aged phenotype.Citation18-Citation20 Another recent report substantiates this fact wherein an in vivo bystander effect of senescent cells has been identified that acts as a potential trigger of the initiation of senescent-cell accumulation.Citation21 It is also pertinent to note here that senescent cells are not only characterized by the loss of proliferative capacity but also show several unique-associated features such as high metabolic activity,Citation22 activation of nutrient-sensing pathways such as mammalian target of rapamycin (mTOR),Citation23 and the development of senescence-associated secretory phenotype (SASP), which is a complex milieu of pro-inflammatory cytokines and growth factors that can contribute to inflammatory damage in nearby cells.Citation24,Citation25 Therefore, it is not surprising to contemplate that elimination of senescent cells or enabling their delayed development could be a potent way of targeting several age-associated disorders, including the hallmark diseases of twenty-first century, i.e. cancer and diabetes. In this context, a breakthrough study has shown that life-long removal of cells expressing a biomarker of cellular senescence-p16Ink4a, delayed the onset of age-related pathologies in several tissues such as adipose, skeletal muscle, and eye while late-life clearance of p16Ink4a cells also attenuated the progression of already established age-related disorders.Citation26 Similarly, in another recent work, it was observed that targeting senescent cells could alleviate the symptoms of obesity-induced type II diabetes thereby providing a novel perspective on the therapeutic applications of senescent cells.Citation27 Overall, it seems plausible that even during aging, healthier cellular functions can be maintained by inhibiting the development or selective removal of senescent cells, which may result in a lower rate of incidences of tumorigenesis and inflammatory disorders.
The emerging deleterious impact of accumulating senescent cells on aging and associated disorders has accelerated the need for identification of modulators of cellular senescence that may improve or maintain cellular functions during aging. In this context, nutritional interventions could play an important role, as several bioactive phytomolecules have been identified which target different aspects of cellular senescence and SASP.Citation28-Citation30 Lactic acid bacteria mediated modulation of human health and longevity was first highlighted by MetchinkoffCitation31 in 1907. The term ‘probiotic’ however was coined by Lilly and StillwellCitation32 in 1965 and presently probiotics are defined as “live microorganisms which when administered in adequate amounts confer a health benefit on the host”.Citation33 A plethora of research has established that gut microbiome plays a critical role in influencing several facets of human health including metabolic regulation and energetic homeostasis,Citation34,Citation35 immune stimulation,Citation36,Citation37 gut barrier integrityCitation38,Citation39 as well as neurological behavior.Citation40,Citation41 Dietary supplementation of probiotic microorganisms is known to influence the composition, diversity, and functions of the gut microbiome and probiotics have been documented for several health beneficial effects to the host through diverse mechanisms.Citation42-Citation44 In the context of aging, evidence is emerging that probiotic bacteria are useful modulators of age-related pathologies and morbidity. This has been especially affirmed in cases such as gastrointestinal healthCitation45-Citation47 and immunosenescenceCitation48-Citation50 wherein probiotic supplementation has shown potential as natural alleviators of these deleterious aspects of aging. However, the role of probiotics as modulators of cellular senescence per se is only beginning to be understood. The present review describes the known anti-aging effects of probiotics and discusses evidences and mechanisms suggesting how probiotics could be useful in mitigating the progression and severity of cellular senescence in an attempt to advocate the development of probiotic-based anti-senescence therapies.
Cellular senescence relates causes and effects of aging
Some of the most dangerous and hallmark disorders known to humanity in the 21st century are deeply related to aging. However, why and how we age have remained the most enduring of questions-both philosophically and scientifically. In this regard, recent advances in geroscience have led to a functional and provable hypothesis suggesting that microscopic damage in the wake of accumulating senescent cells in tissues and organs gradually manifests as an observable aged phenotype and various associated morbidities.Citation51 However, cellular senescence is not exclusively a deleterious process, but is thought to have evolved as an important barrier to tumorigenesisCitation52 and has also been shown as an important regulator of wound healing in tissues.Citation53 The SASP in senescent cells of young organisms provides inflammatory signals that help attract immune cells enabling the removal of senescent cells, while growth signals in SASP stimulate proliferation in nearby cells to allow subsequent replacement of senescent cells.Citation54 However, as we age, this delicate balance is seemingly perturbed and senescent cells begin to accumulate in tissues resulting in chronic inflammatory stress thorough persistent SASP which now induces a pro-inflammatory and pro-tumorigenic micro-environment characteristically indicative of antagonistic pleiotropyCitation55 (). The apparent age-related increase in senescent-cell burden reflects either an enhanced rate of senescent-cell production due to accumulating oxidative damage in cellular macromolecules including in the replicatively sensitive telomeric regions of the DNA,Citation14,Citation15 and/or decreased senescent-cell clearance by the immune system. In the latter aspect, senescent cells are considered to be highly immunogenic targets due to the upregulation of certain ligands that are recognized by immune cells such as natural killer (NK) resulting in their specific clearance.Citation56,Citation57 However, the immune system itself is known to undergo multi-faceted age-associated changes (immunosenescence) including impaired immune surveillanceCitation58 and reduced cytotoxic abilityCitation59 which may directly hinder the efficiency of immune cell-mediated clearance of senescent cells and thus enabling their gradual accumulation. This notion has been recently substantiated when it was observed that mice deficient in perforin-mediated cell cytotoxicity exhibited higher senescent-cell tissue burden, chronic inflammation and also suffered from multiple age-related disorders suggesting that impaired immune surveillance can accelerate the accumulation of senescent cells and aging phenotype.Citation60
Figure 1. Schematic diagram depicting the role of senescent cells in driving the process of aging. Oxi-inflammatory stress contributes to the development of senescent cells which gradually accumulate and promote inflamm-aging, resulting in tissue and organ dysfunctions and pro-tumorigenic environment characteristic of aging-phenotype.
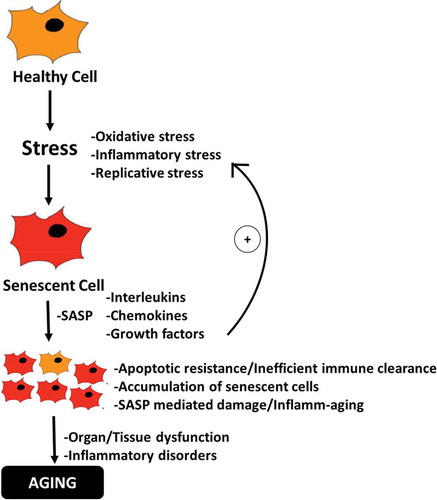
Apart from cell cycle inhibition, perturbations in oxidative and inflammatory pathways are amongst the most discernible features of cellular senescence that are common to different cell types. Therefore, not surprisingly, it has been shown that the application of non-lethal stressors in vitro can initiate a cell senescence program with characteristics similar to replicative senescence.Citation13,Citation61 In fact, chronic oxidative and inflammatory stress and decrease in antioxidant potency can augment the development of ROS-mediated molecular and eventual DNA damageCitation4 which is often followed by the induction of DNA damage response (DDR)Citation62 and downstream activation of cell cycle inhibitory pathways (p53/p21WAF1/p16INK4a)Citation13,Citation63,Citation64 ultimately resulting in senescence. Although the inhibition of cell cycle is desirable to prevent the division of unhealthy cells; however, senescent cells are known to resist apoptosis by dysregulating pro-apoptotic and anti-apoptotic pathways (Bax/Bcl-2)Citation65 thereby resulting in their enhanced survival and gradual accumulation in tissues and organs (). In contrast to replicative senescence, which is an inherent cellular feature, oxidative stress-induced induction of senescence is more dynamic and presents an added modifiable opportunity that can be targeted to prevent or deaccelerate senescence. Considering this, it can be envisaged that various natural antioxidants, or even putative probiotics with strong antioxidant capacity, could be important regulators of oxidative stress-induced senescence and aging. Indeed, it has been shown that different phytomolecules can influence diverse aspects of senescence including suppression of both replicative or stress-induced senescence, development of SASP or even induction of apoptosis in senescent cells (senolytics).Citation28 Further, given the key role of immunosenescence in dysregulating immune cells mediated surveillance and clearance of senescent cells; strategies aimed at augmenting specific immune cells functions during aging may also contribute to the delayed accumulation of senescent cells as well as SASP.
Probiotic bacteria and healthy aging
Studies pertaining to age-related changes in the gut microbiome have shown that gut dysbiosis is related to the development of age-associated pathologies that may reduce longevity.Citation66 Gut dysbiosis can disrupt the host nutrient signaling pathways and metabolismCitation67 which are presumed to have negative effects on host health ultimately contributing to the pathology of various age-associated disorders.Citation68 Studies have also shown that interventions of dietary probiotics are useful in improving elderly health by preventing diarrheal diseases,Citation69 improvement in inflammatory disorders,Citation70 prevention of recurring infections,Citation71 and colon cancer.Citation72 Here, we present evidence indicating that probiotic bacteria can also intervene and modulate different aspects of cellular senescence and SASP that may contribute to observed improvements in organ functions as well as enhancement in longevity.
Probiotics enhance lifespan in model organisms
Probiotic supplementation has shown promising results in improving the longevity of experimental animals. In this regard, Matsumoto and KuriharaCitation73 first hypothesized that probiotic bacteria may extend lifespan by inducing production of small metabolites called polyamines, that act via suppression of inflamm-aging. Subsequently, using the probiotic strain Bifidobacterium animalis subsp. lactis LKM512, Matsumoto et al.Citation74 observed a higher survival rate and reduced incidence of skin ulcers and tumors in probiotic fed mice as compared to control. Authors also noted that LKM512 administration downregulated the expression of aging-associated and inflammation-associated genes in 21 months old animals which were comparable to 10 months old untreated (younger) mice. Another report by Zhao et al.Citation75 observed that feeding probiotic Lactobacillus salivarius strain FDB89 to the nematode Caenorhabditis elegans (C. elegans) extended lifespan by 11.9% as compared to control nematodes in a dietary restriction-dependent manner. Similarly, Grompone et al.Citation76 observed that Lactobacillus rhamnosus CNCM I-3690 enhanced lifespan in C. elegans by about 20% and transcriptomic analysis of C. elegans fed with this strain showed that apparent increase in the nematode lifespan was correlated with differential expression of the DAF-16/insulin-like pathway. Using the probiotic Bacillus licheniformis, Park et al.Citation77 observed enhanced longevity in C. elegans via modulation of expression of genes associated with host serotonin signaling. Nakagawa et al.Citation78 observed that feeding of Lactobacillus gasseri SBT2055 (LG2055) to C. elegans enhanced the survival rate of nematodes by strengthening the resistance to oxidative stress and by stimulating the innate immune response signaling including p38MAPK signaling pathway. Authors also observed that amounts of mitochondria were significantly increased by LG2055 feeding in comparison to the control. Similarly, Park et al.Citation79 observed that Lactobacillus fermentum strain JDFM216 improved resistance of C. elegans to foodborne pathogens and enhanced nematode lifespan mediated by a nuclear hormone receptor family and PMK-1 signaling. In a recent report by Westfall et al.,Citation80 it was observed that a novel probiotic and synbiotic formulation combinatorially extended longevity in male Drosophila melanogaster through the mechanisms of gut-brain-axis communication. Both the probiotic and synbiotic formulations rescued markers of metabolic stress by managing insulin resistance and energy regulatory pathways and further ameliorated aggravation in inflammation, oxidative stress and the loss of mitochondrial complex integrity.Citation80 In another recent work, it was reported that feeding of Lactobacillus fermentum MBC2 to C. elegans enhanced the lifespan and attenuated age-related markers such as pumping rate, lipofuscin accumulation, and body bending in nematodes.Citation81
Probiotics as suppressors of age-associated oxi-inflammatory stress
Dysregulation in the processes pertaining to maintenance of redox and inflammatory homeostasis is central to the etiology of senescence as well as to the pathogenesis of various age-related predispositions to inflammatory disorders. It is thus not surprising that the preservation of oxi-inflammatory cellular environment has shown potential as modulators of age and associated disorders. In this context, Lin et al.Citation82 observed that oral administration of L. plantarum AR113 and AR501 improved the antioxidant status of d-galactose-induced oxidative stress in aging mice and alleviated liver damage and abnormal activities of superoxide dismutase, glutathione peroxidase, and catalase to normal levels, while also enhancing gene expression of nuclear factor erythroid-2-related factor 2 and antioxidant genes such as glutathione reductase, glutathione S-transferase and NAD(P)H quinone oxidoreductase 1. Zhao et al.Citation83 further observed that administration of L. plantarum strains protected against d-galactose induced oxidative damage and gut dysbiosis in aging mice and inferred that the protective effects of L. plantarum strain on the host microbiota could be one of the mechanisms of their resistance to age-related oxidative stress. Similarly, a recent study by Hor et al.Citation84 demonstrated that administration of probiotic Lactobacilli strains improved gut dysbiosis, modulated the metabolism of amino acids such as urocanic acid, citrulline, cystamine, and 5-oxoproline as well as antioxidant capacity in d-galactose senescence-induced aging rats thereby highlighting the potential of probiotics as anti-aging therapeutics through healthy gut modulation. In a similar report by Lew et al.,Citation85 the administration of different Lactobacillus strains to a high-fat diet and d-galactose-induced model of aging rats led to strain-specific effects on gut microbiota diversity and composition, short-chain fatty acids production as well as higher fecal contents of compounds related to amino acid or carbohydrate metabolism. Jeong et al.Citation86 observed anti-inflammaging effects of probiotic Lactobacillus brevis OW38 in aged mice. Authors reported that oral administration of Lactobacillus brevis OW38 significantly reduced the LPS level in colon fluid and blood, while also inhibiting the expression of inflammatory markers, such as myeloperoxidase, tumor necrosis factor (TNF), interleukin (IL)-1β, and activation of NF-κB. An interesting report by Lee et al.Citation87 observed gender-specific beneficial effects of an anti-inflammatory probiotic strain Lactobacillus reuteri BM36301 on aging C57BL/6 mice. Authors observed that males treated with the probiotic bacteria experienced less weight gain and higher testosterone level while females treated with the same bacteria maintained lower serum TNF-α as well as healthy skin with active folliculogenesis and hair growth. Pan et al.Citation88 observed that calorie restriction caused changes in the gut microbiome of mice wherein a selective increase in Lactobacilli species was observed that correlated with decreased markers of systemic inflammation and circulating microbial antigens. One of the isolates, Lactobacillus murinus CR147, downregulated interleukin-8 production in TNF-α-stimulated Caco-2 cells and significantly increased the lifespan and the brood size of the C. elegans indicating that proliferation of Lactobacillus murinus in calorie-restricted mice causatively contributed to the attenuation of aging-associated inflammation. Previously, we have also observed that supplementation of probiotic Lactobacillus rhamnosus to aged mice suppressed an age-associated increase in systemic inflamm-aging markers as evident by a decrease in circulatory TNF-α and MCP-1 proteins along with enhanced antioxidant capacity in liver and RBCs as compared to control.Citation70 Together, these observations provide evidence that probiotic bacteria can mitigate age-associated cellular oxidative and inflammatory stress that can ultimately help alleviate cell senescence and rate of occurrence of inflammatory disorders in the elderly.
Probiotic-mediated suppression of immunosenescence: potential against cell senescence?
Immunosenescence is an umbrella term that describes the characteristic remodeling of different aspects of the immune system which contribute to age-related disorders.Citation4,Citation89,Citation90 Emerging evidence is now suggesting that impaired surveillance and antigenic uptake by immune cells during aging could directly contribute to the inefficient clearance of senescent cells and associated increase in senescent-cell burden in tissues.Citation58,Citation60 Studies in both experimental animals and human subjects have shown that probiotic bacteria can alter some of the deleterious aspects of immunosenescence. For instance, in a study by Gill et al.,Citation48 it was observed that consumption of Lactobacillus rhamnosus HN001 or Bifidobacterium lactis HN019 by healthy elderly subjects for three weeks enhanced the numbers and activity of NK cells. Similarly, consumption of Lactobacillus gasseri TMC0356 enhanced splenic NK cell activity and expression of IL-2 and IFN-α/β receptor 1 in lung cells of senescence-accelerated mice.Citation91 We have also observed that consumption of probiotic Lactobacillus fermentum in aged swiss albino mice attenuated markers of inflamm-aging, improved neutrophil functions and enhanced resistance to infectious E. coli as compared to control.Citation50 Spaiser et al. reported that consumption of a probiotic mixture enhanced circulatory IL-10 levels and reduced inflammatory cytokine profile in older adults.Citation92 Miyazawa et al.Citation93 observed that consumption of heat-killed Lactobacillus gasseri increased the number of CD8+ T cells and reduce CD28 expression loss in CD8+ T cells of the elderly subjects. Vidal et al.Citation94 reported that supplementation of Lactobacillus paracasei NCC2461 in aged mice improved specific adaptive immune response as evident with higher IgG2a levels after antigenic challenge. Consumption of probiotic Bacillus subtilis CU1 enhanced fecal and salivary secretory IgA concentrations in elderly subjects that decreased the frequency of respiratory infections as compared to placebo.Citation95 A recent report also suggests that supplementation of fermented milk containing probiotic Lactobacillus casei DN-114001 to aged mice improved macrophage functions, NK cell activity and proliferative index as compared to control.Citation96 However, despite these encouraging observations, studies exploring the role of probiotic treatment in the augmentation of immune surveillance and the subsequent clearance of senescent cells are lacking. It is also pertinent to note that although immunosenescence is a recognized age-associated immunological disorder; however, it should not be confused with cell senescence, as immunosenescence encompasses a variety of changes that are unrelated to cell senescence.Citation97 Whether or not probiotics can influence the senescence of immune cells per se is also not investigated and therefore, probiotics mediated modulation of the immune system vis-à-vis immune surveillance and immune cell senescence represents an exciting area for exploring probiotic-based therapeutics during aging.
Probiotics as modulators of cellular senescence
Evidences presented in this manuscript thus far suggest that probiotics can attenuate age-associated inflamm-aging, immunosenescence and oxidative stress that may promote longevity. Although these disorders are intimately linked with the macro-phenotype of senescent cells; however, studies directly relating the observed anti-aging or healthy aging effects of probiotics with the progression and development of cellular senescence per se are very rare. Jeong et al.Citation98 initially showed that administration of Lactobacillus pentosus var. plantarum C29 to aged Fisher rats prevented senescence of colon tissue as evident by suppression of senescence markers viz. p16 and p53 along with the inhibition of activation of NF-κB and mTOR pathway providing evidence of anti-senescence and SASP inhibitory effects of probiotics. Similarly, the application of probiotic mixture IRT5 ameliorated age-dependent onset of colitis in Fisher rats and suppressed an age-associated increase in expression of senescence markers p16 and p53 in the colon of aged rats while also enhancing age-suppressed expression of SIRT1.Citation99 In another study, oral administration of Lactobacillus brevis OW38 suppressed the expression of senescence markers p16, p53, and SAMHD1 in the colon and the hippocampus of aged mice, inhibited inflamm-aging and restored spontaneous alternation as well as the expression of brain-derived neurotrophic factor and doublecortin in aged mice.Citation86 In a recent work, we sought to directly address whether probiotic bacteria can prevent the progression of cellular senescence using a stress-induced premature model of murine preadipocytes. It was observed that supplementation of secretory metabolites of probiotic Lactobacillus fermentum sufficiently rescued cells from stress-induced senescence as evident by a decrease in p16Ink4a/p53/p21WAF1 expression, markers of SASP, NF-κB activation and ROS-induced DNA damage.Citation100 These effects of probiotic bacteria were correlated with the inhibition of PI3K/Akt/mTOR pathway, which is rapidly emerging as a central regulator of senescenceCitation101 and longevity,Citation102 thereby indicating that probiotic bacteria may suppress senescence by inhibiting aberrant mTOR activation and maintaining oxidative homeostasis. Together, these observations suggest that probiotic bacteria can influence the progression and severity of cell senescence which may have a major impact on tissue homeostasis and also provide mechanisms governing the known health-promoting effects of probiotics during aging (). However, more detailed studies, especially targeting the identification of probiotic bacteria that may mitigate the development of SASP, selectively induce apoptosis in senescent cells or inhibit mTOR signaling during cellular senescence and aging are required to fully ascertain and establish the anti-senescence attributes of probiotics.
Figure 2. Probiotics can influence the process of senescence. The secretory metabolites of probiotic bacteria such as polyamines, SCFAs or probiotic-fermented polyphenols could affect different aspects of cell senescence including cell cycle regulators, oxi-inflammatory stress, mTOR pathway and immune activation that may delay the initiation of senescence program leading to the augmentation of healthy aging.
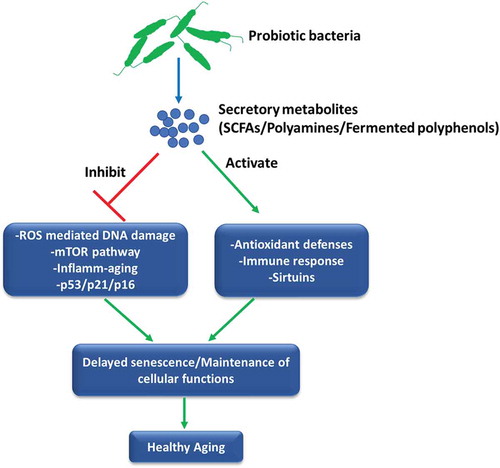
Secretory metabolites of probiotics that influence aging
Probiotics mediate their health beneficial effects by different mechanisms including altering the microbiota composition, maintaining epithelial barrier function and modulating mucosal and systemic immune responses. Probiotic bacteria also secrete various small bioactive metabolites in the gut which interact with epithelial cells and are absorbed into circulation by passive and active mechanisms thereby allowing their cellular modulatory effects at sites distant to the gut.Citation103,Citation104 During the last few years, several studies have reported the effects of probiotic secretory factors on immunomodulation,Citation105 cell cycle regulation,Citation106 resisting infections,Citation107 and antioxidant activity.Citation108 In fact, identification and characterization of these metabolites have been implicated for the development of a new generation of functional foods, without involving live probiotic bacteria, which may have clinical relevance.Citation109 Probiotic secretory factors are diverse in nature and often include short-chain fatty acids (SCFAs), peptides, lactic acid, vitamins, polyamines, and polyphosphates.Citation110 A cause-and-effect relationship regarding aging and health has been documented with these molecules, especially with SCFAs and polyamines. It has been shown that levels of SCFAs decline with ageCitation111 which may contribute to perturbations in the gut mucus barrier,Citation112 neural microglia maturation, and function,Citation113 as well as host cells insulin sensitivity and energy expenditure,Citation114 that can be implicated in the pathogenesis of aging and associated inflammatory disorders. SCFAs are produced by bacterial fermentation in the gut and supplementation of these molecules has shown evidence of improvement in inflammation, neural health, and aging.Citation115,Citation116 Similarly, polyamines are small molecules that are abundantly produced by commensal bacteria and play a critical role in various cell processes.Citation117 The levels of polyamines also gradually decline in various organs with ageCitation118 and supplementation of polyamines producing probiotic bacteriaCitation74 or direct exogenous supply of polyamin-esCitation119 has shown life-span enhancing effects.Citation120,Citation121 However, despite their apparent health-promoting effects during aging, to the best of our knowledge, no studies thus far have been reported that assess the anti-senescence, anti-SASP or senolytic attributes of these probiotic metabolites and therefore represent a niche area in the domain of probiotics and aging research.
Probiotics and polyphenols: prospects of novel anti-senescence combination(s)?
Nutritional interactions in vivo, and particularly in the gut, are complex and multifaceted. Probiotics and plant-polyphenols are perhaps the most vital natural sources of bioactive components present in our daily diet. As such, strategies aimed at developing potent nutritional interventions for the modulation of human health would invariably require gauging both probiotics and plant polyphenols as potential candidates. However, the mutual compatibility of probiotic bacteria and polyphenols has not always been considered favorable. While a general assumption is that polyphenols are anti-bacterial in nature; evidence is accumulating that pathogenic bacteria are actually more sensitive to polyphenols as compared to probiotics, and that polyphenols may act as prebiotic compounds.Citation122-Citation124 Also, it has been shown that probiotic bacteria can metabolize polyphenols into novel bioactive compounds that may significantly enhance the biological effects of parent polyphenols.Citation125,Citation126 Further, there is evidence to consider that probiotic-fermented traditional polyphenol-rich beverages are more potent in antioxidant and anti-inflammatory activities enabling their health-promoting effects on various disorders such as obesityCitation127 and liver damage.Citation128 Considering this, recently it has been advocated that amalgamation of plant polyphenols with probiotic bacteria could act as a second generation of synbiotics which would be more beneficial to human health, particularly in the mitigation of aging and associated disorders.Citation129,Citation130 The known-limited bioavailability of several bioactive polyphenols (e.g. curcumin or catechins), that hampers their biological relevance, could actually be useful in this strategy as it would allow greater interactions between probiotic bacteria and polyphenols in the gut. Since several polyphenols have already been identified as anti-senescence and anti-SASP agents, at least in vitro,Citation29 it is plausible that carefully identified combinations of polyphenols with probiotic bacteriaCitation130 could offer potent and extensive effects on regulating several facets of cell senescence, aging, and associated pathologies.
Conclusions
Advances in our understanding of the role of cellular senescence has provided a causative and modifiable factor that can simultaneously affect several aspects of aging, including the known predisposition to various diseases. There is enough evidence to consider that probiotic bacteria can suppress several deleterious aspects of aging. The anti-inflammaging, antioxidant and anti-immunosenescence effects of probiotics suggest their potential as modulators of SASP and cell senescence, especially oxidative stress-induced senescence. Till now, a vast majority of studies focussing on anti-aging and longevity modulatory potential of probiotics have only targeted oxi-inflammatory and nutrient modulatory capacities of probiotic bacteria. However, given our expanding understanding of the molecular etiology of the aging process; it is imperative to identify and assess probiotic bacteria or their secretory metabolites with potential anti-senescence, anti-SASP, senolytic or specific immune-enhancing attributes that may be correlated with their observed health beneficial effects during aging. Together, it represents an exciting area of research as the diversity and abundance of probiotic bacteria, coupled with their known functional efficacy in modulating the regulatory systems of human body, highlights enormous opportunities in developing probiotic-based therapies for the modulation of cellular senescence and aging.
Disclosure of potential conflicts of interest
No potential conflict of interest was reported by the authors.
Additional information
Funding
References
- Jin K. Modern biological theories of aging. Aging Dis. 2010;1:72–74.
- Harman D. Aging: a theory based on free radical and radiation chemistry. J Gerontol. 1956;11(3):298–300. doi:10.1093/geronj/11.3.298. PMID 13332224.
- Harman D. The biologic clock: the mitochondria? J Am Geriatr Soc. 1972;20(4):145–147. doi:10.1111/j.1532-5415.1972.tb00787.x.
- De la Fuente M, Miquel J. An update of the oxidation-inflammation theory of aging: the involvement of the immune system in oxi-inflamm-aging. Curr Pharm Des. 2009;15(26):3003–3026. doi:10.2174/138161209789058110.
- Hayflick L, Moorhead PS. The serial cultivation of human diploid cell strains. Exp Cell Res. 1961;25:585–621. doi:10.1016/0014-4827(61)90192-6.
- Kiyoshima T, Enoki N, Kobayashi I, Sakai T, Nagata K, Wada H, Fujiwara H, Ookuma Y, Sakai H. Oxidative stress caused by a low concentration of hydrogen peroxide induces senescence-like changes in mouse gingival fibroblasts. Int J Mol Med. 2012;30(5):1007–1012. doi:10.3892/ijmm.2012.1102.
- Velichko AK, Petrova NV, Razin SV, Kantidze OL. Mechanism of heat stress-induced cellular senescence elucidates the exclusive vulnerability of early S-phase cells to mild genotoxic stress. Nucleic Acids Res. 2015;43(13):6309–6320. doi:10.1093/nar/gkv573.
- Serrano M, Lin AW, McCurrach ME, Beach D, Lowe SW. Oncogenic RAS provokes premature cell senescence associated with accumulation of p53 and p16INK4a. Cell. 1997;88(5):593–602. doi:10.1016/S0092-8674(00)81902-9.
- Panganiban RA, Mungunsukh O, Day RM. X-irradiation induces ER stress, apoptosis, and senescence in pulmonary artery endothelial cells. Int J Radiat Biol. 2013;89(8):656–667. doi:10.3109/09553002.2012.711502.
- Colavitti R, Finkel T. Reactive oxygen species as mediators of cellular senescence. IUBMB Life. 2005;57(4–5):277–281. doi:10.1080/15216540500091890.
- Sasaki M, Kajiya H, Ozeki S, Okabe K, Ikebe T. Reactive oxygen species promotes cellular senescence in normal human epidermal keratinocytes through epigenetic regulation of p16(INK4a). Biochem Biophys Res Commun. 2014;452(3):622–628. doi:10.1016/j.bbrc.2014.08.123.
- Dimozi A, Mavrogonatou E, Sklirou A, Kletsas D. Oxidative stress inhibits the proliferation, induces premature senescence and promotes a catabolic phenotype in human nucleus pulposus intervertebral disc cells. Eur Cell Mater. 2015;30:89–102. doi:10.22203/eCM.v030a07.
- Kumar R, Sharma A, Kumari A, Gulati A, Padwad Y, Sharma R. Epigallocatechin gallate suppresses premature senescence of preadipocytes by inhibition of PI3K/Akt/mTOR pathway and induces senescent cell death by regulation of Bax/Bcl-2 pathway. Biogerontology. 2019;20(2):171–189. doi:10.1007/s10522-018-9785-1.
- von Zglinicki T. Oxidative stress shortens telomeres. Trends Biochem Sci. 2002;27(7):339–344. doi:10.1016/S0968-0004(02)02110-2.
- Ludlow AT, Spangenburg EE, Chin ER, Cheng WH, Roth SM. Telomeres shorten in response to oxidative stress in mouse skeletal muscle fibers. J Gerontol A Biol Sci Med Sci. 2014;69(7):821–830. doi:10.1093/gerona/glt211.
- de Magalhães JP. From cells to ageing: a review of models and mechanisms of cellular senescence and their impact on human ageing. Exp Cell Res. 2004;300(1):1–10. doi:10.1016/j.yexcr.2004.07.006.
- Hayflick L. How and why we age. Exp Gerontol. 1998;33(7–8):639–653. doi:10.1016/S0531-5565(98)00023-0.
- Melk A, Schmidt BM, Takeuchi O, Sawitzki B, Rayner DC, Halloran PF. Expression of p16INK4a and other cell cycle regulator and senescence associated genes in aging human kidney. Kidney Int. 2004;65(2):510–520. doi:10.1111/j.1523-1755.2004.00438.x.
- Liu Y, Sanoff HK, Cho H, Burd CE, Torrice C, Ibrahim JG, Thomas NE, Sharpless NE. Expression of p16(INK4a) in peripheral blood T-cells is a biomarker of human aging. Aging Cell. 2009;8(4):439–448. doi:10.1111/j.1474-9726.2009.00489.x.
- Baker DJ, Childs BG, Durik M, Wijers ME, Sieben CJ, Zhong J, Saltness RA, Jeganathan KB, Verzosa GC, Pezeshki A, et al. Naturally occurring p16(Ink4a)-positive cells shorten healthy lifespan. Nature. 2016;530:184–189. doi:10.1038/nature16932.
- da Silva PFL, Ogrodnik M, Kucheryavenko O, Glibert J, Miwa S, Cameron K, Ishaq A, Saretzki G, Nagaraja-Grellscheid S, Nelson G, et al. The bystander effect contributes to the accumulation of senescent cells in vivo. Aging Cell. 2019;18(1):e12848. doi:10.1111/acel.12848.
- James EL, Lane JA, Michalek RD, Karoly ED, Parkinson EK. Replicatively senescent human fibroblasts reveal a distinct intracellular metabolic profile with alterations in NAD+ and nicotinamide metabolism. Sci Rep. 2016;6:38489. doi:10.1038/srep38489.
- Houssaini A, Breau M, Kebe K, Abid S, Marcos E, Lipskaia L, Rideau D, Parpaleix A, Huang J, Amsellem V, et al. mTOR pathway activation drives lung cell senescence and emphysema. JCI Insight. 2018;3(3):pii: 93203. doi:10.1172/jci.insight.93203.
- Coppé JP, Patil CK, Rodier F, Sun Y, Muñoz DP, Goldstein J, Nelson PS, Desprez PY, Campisi J. Senescence-associated secretory phenotypes reveal cell-nonautonomous functions of oncogenic RAS and the p53 tumor suppressor. PLoS Biol. 2008;6(12):2853–2868. doi:10.1371/journal.pbio.0060301.
- Watanabe S, Kawamoto S, Ohtani N, Hara E. Impact of senescence-associated secretory phenotype and its potential as a therapeutic target for senescence associated diseases. Cancer Sci. 2017;108:563–569. doi:10.1111/cas.13184.
- Baker DJ, Wijshake T, Tchkonia T, LeBrasseur NK, Childs BG, van de Sluis B, Kirkland JL, van Deursen JM. Clearance of p16Ink4a-positive senescent cells delays ageing-associated disorders. Nature. 2011;479(7372):232–236. doi:10.1038/nature10600.
- Palmer AK, Xu M, Zhu Y, Pirtskhalava T, Weivoda MM, Hachfeld CM, Prata LG, van Dijk TH, Verkade E, Casaclang-Verzosa G, et al. Targeting senescent cells alleviates obesity-induced metabolic dysfunction. Aging Cell. 2019;18(3):e12950. doi:10.1111/acel.12950.
- Sharma R, Padwad Y. In search of nutritional anti-aging targets: TOR inhibitors, SASP modulators, and BCL-2 family suppressors. Nutrition. 2019;65:33–38. doi:10.1016/j.nut.2019.01.020.
- Mária J, Ingrid Ž. Effects of bioactive compounds on senescence and components of senescence associated secretory phenotypes in vitro. Food Funct. 2017;8(7):2394–2418. doi:10.1039/c7fo00161d.
- Wang Y, Chang J, Liu X, Zhang X, Zhang S, Zhang X, Zhou D, Zheng G. Discovery of piperlongumine as a potential novel lead for the development of senolytic agents. Aging (Albany NY). 2016;8(11):2915–2926. doi:10.18632/aging.101100.
- Metchinkoff E. The prolongation of life. Eds Mitchell PC. New York (USA): Putmans Sons; 1908. 151 pp.
- Lilly DM, Stillwell RH. Probiotics: growth-promoting factors produced by microorganisms. Science. 1965;147(3659):747–748. doi:10.1126/science.147.3659.747.
- Food and Agriculture Organization of the United Nations, World Health Organization. Report on Joint FAO/WHO expert consultation on evaluation of health and nutritional properties of probiotics in food including powder milk with live lactic acid bacteria. 2001. https://www.who.int/foodsafety/fs_management/en/probiotic_guidelines.pdf
- Wa Y, Yin B, He Y, Xi W, Huang Y, Wang C, Guo F, Gu R. Effects of single probiotic- and combined probiotic-fermented milk on lipid metabolism in hyperlipidemic rats. Front Microbiol. 2019;10:1312. doi:10.3389/fmicb.2019.01312.
- Jang HR, Park HJ, Kang D, Chung H, Nam MH, Lee Y, Park JH, Lee HY. A protective mechanism of probiotic Lactobacillus against hepatic steatosis via reducing host intestinal fatty acid absorption. Exp Mol Med. 2019;51(8):95. doi:10.1038/s12276-019-0293-4.
- Perdigón G, Maldonado Galdeano C, Valdez JC, Medici M. Interaction of lactic acid bacteria with the gut immune system. Eur J Clin Nutr. 2002;56(suppl 4):S21–S26. doi:10.1038/sj.ejcn.1601658.
- Sichetti M, De Marco S, Pagiotti R, Traina G, Pietrella D. Anti-inflammatory effect of multistrain probiotic formulation (L. rhamnosus, B. lactis, and B. longum). Nutrition. 2018;53:95–102. doi:10.1016/j.nut.2018.02.005.
- Wang J, Ji H, Wang S, Liu H, Zhang W, Zhang D, Wang Y. Probiotic Lactobacillus plantarum promotes intestinal barrier function by strengthening the epithelium and modulating gut microbiota. Front Microbiol. 2018;9:1953. doi:10.3389/fmicb.2018.01953.
- Yi H, Wang L, Xiong Y, Wang Z, Qiu Y, Wen X, Jiang Z, Yang X, Ma X. Lactobacillus reuteri LR. Improved expression of genes of tight junction proteins via the MLCK pathway in IPEC-1 cells during infection with enterotoxigenic Escherichia coli K88. Mediators Inflamm. 2018;2018:6434910. doi:10.1155/2018/6434910.
- Messaoudi M, Lalonde R, Violle N, Javelot H, Desor D, Nejdi A, Bisson JF, Rougeot C, Pichelin M, Cazaubiel M, et al. Assessment of psychotropic-like properties of a probiotic formulation (Lactobacillus helveticus R0052 and Bifidobacterium longum R0175) in rats and human subjects. Br J Nutr. 2011;105(5):755–764. doi:10.1017/S0007114510004319.
- Marotta A, Sarno E, Del Casale A, Pane M, Mogna L, Amoruso A, Felis GE, Fiorio M. Effects of probiotics on cognitive reactivity, mood, and sleep quality. Front Psychiatry. 2019;10:164. doi:10.3389/fpsyt.2019.00164.
- Toscano M, De Grandi R, Stronati L, De Vecchi E, Drago L. Effect of Lactobacillus rhamnosus HN001 and Bifidobacterium longum BB536 on the healthy gut microbiota composition at phyla and species level: A preliminary study. World J Gastroenterol. 2017;23(15):2696–2704. doi:10.3748/wjg.v23.i15.2696.
- Kechagia M, Basoulis D, Konstantopoulou S, Dimitriadi D, Gyftopoulou K, Skarmoutsou N, Fakiri EM. Health benefits of probiotics: a review. ISRN Nutr. 2013;2013:481651. doi:10.5402/2013/481651.
- Azad MAK, Sarker M, Li T, Yin J. Probiotic species in the modulation of gut microbiota: an overview. Biomed Res Int. 2018;2018:9478630. doi:10.1155/2018/9478630.
- Metugriachuk Y, Marotta F, Pavasuthipaisit K, Kuroi O, Tsuchiya J, Lorenzetti A, Fesce E, Minelli E. The aging gut motility decay: may symbiotics be acting as “implantable” biologic pace-makers? Rejuvenation Res. 2006;9(2):342–345. doi:10.1089/rej.2006.9.342.
- Ahmed M, Prasad J, Gill H, Stevenson L, Gopal P. Impact of consumption of different levels of Bifidobacterium lactis HN019 on the intestinal microflora of elderly human subjects. J Nutr Health Aging. 2007;11:26–31.
- Malaguarnera G1, Leggio F, Vacante M, Motta M, Giordano M, Bondi A, Basile F, Mastrojeni S, Mistretta A, Malaguarnera M, et al. Probiotics in the gastrointestinal diseases of the elderly. J Nutr Health Aging. 2012;16(4):402–410. doi:10.1007/s12603-011-0357-1.
- Gill HS, Rutherfurd KJ, Cross ML. Dietary probiotic supplementation enhances natural killer cell activity in the elderly: an investigation of age-related immunological changes. J Clin Immunol. 2001;21(4):264–271. doi:10.1023/A:1010979225018.
- Kaburagi T, Yamano T, Fukushima Y, Yoshino H, Mito N, Sato K. Effect of Lactobacillus johnsonii La1 on immune function and serum albumin in aged and malnourished aged mice. Nutrition. 2007;23(4):342–350. doi:10.1016/j.nut.2007.02.001.
- Sharma R, Kapila R, Kapasiya M, Saliganti V, Dass G, Kapila S. Dietary supplementation of milk fermented with probiotic Lactobacillus fermentum enhances systemic immune response and antioxidant capacity in aging mice. Nutr Res. 2014;34(11):968–981. doi:10.1016/j.nutres.2014.09.006.
- Bhatia-Dey N, Kanherkar RR, Stair SE, Makarevm EO, Csokam AB. Cellular senescence as the causal nexus of aging. Front Genet. 2016;7:13. doi:10.3389/fgene.2016.00013.
- Prieur A, Peeper DS. Cellular senescence in vivo: a barrier to tumorigenesis. Curr Opin Cell Biol. 2008;20(2):150–155. doi:10.1016/j.ceb.2008.01.007.
- Demaria M, Ohtani N, Youssef SA, Rodier F, Toussaint W, Mitchell JR, Laberge RM, Vijg J, Van Steeg H, Dollé ME, et al. An essential role for senescent cells in optimal wound healing through secretion of PDGF-AA. Dev Cell. 2014;31(6):722–733. doi:10.1016/j.devcel.2014.11.012.
- Borodkina A, Deryabin PI, Giukova AA, Nikolsky NN. “Social life” of senescent cells: what is SASP and why study it? Acta Naturae. 2018;10:4–14. doi:10.32607/20758251-2018-10-1-4-14.
- Campisi J. Aging, cellular senescence, and cancer. Annu Rev Physiol. 2013;75:685–705. doi:10.1146/annurev-physiol-030212-183653.
- Sagiv A, Burton DGA, Moshayev Z, Vadai E, Wensveen F, Ben-Dor S, Golani O, Polic B, Krizhanovsky V. NKG2D ligands mediate immunosurveillance of senescent cells. Aging (Albany NY). 2016;8:328–344. doi:10.18632/aging.v8i2.
- Antonangeli F, Soriani A, Ricci B, Ponzetta A, Benigni G, Morrone S, Bernardini G, Santoni A. Natural killer cell recognition of in vivo drug-induced senescent multiple myeloma cells. Oncoimmunology. 2016;5(10):e1218105. doi:10.1080/2162402X.2016.1218105.
- Dussault I, Miller SC. Decline in natural killer cell-mediated immunosurveillance in aging mice–a consequence of reduced cell production and tumor binding capacity. Mech Ageing Dev. 1994;75(2):115–129. doi:10.1016/0047-6374(94)90080-9.
- Butcher SK, Chahal H, Nayak L, Sinclair A, Henriquez NV, Sapey E, O’Mahony D, Lord JM. Senescence in innate immune responses: reduced neutrophil phagocytic capacity and CD16 expression in elderly humans. J Leukoc Biol. 2001;70:881–886.
- Ovadya Y, Landsberger T, Leins H, Vadai E, Gal H, Biran A, Yosef R, Sagiv A, Agrawal A, Shapira A, et al. Impaired immune surveillance accelerates accumulation of senescent cells and aging. Nat Commun. 2018;9(1):5435. doi:10.1038/s41467-018-07825-3.
- Toussaint O, Dumont P, Remacle J, Dierick JF, Pascal T, Frippiat C, Magalhaes JP, Zdanov S, Chainiaux F. Stress-induced premature senescence or stress-induced senescence-like phenotype: one in vivo reality, two possible definitions? Sci World J. 2002;2:230–247. doi:10.1100/tsw.2002.100.
- Benkafadar N, François F, Affortit C, Casas F, Ceccato JC, Menardo J, Venail F, Malfroy-Camine B, Puel JL, Wang J. ROS-induced activation of DNA damage responses drives senescence-like state in postmitotic cochlear cells: implication for hearing preservation. Mol Neurobiol. 2019;56(8):5950–5969. doi:10.1007/s12035-019-1493-6.
- Gambino V, De Michele G, Venezia O, Migliaccio P, Dall’Olio V, Bernard L, Minardi SP, Della Fazia MA, Bartoli D, Servillo G, et al. Oxidative stress activates a specific p53 transcriptional response that regulates cellular senescence and aging. Aging Cell. 2013;12(3):435–445. doi:10.1111/acel.12060.
- Mas-Bargues C, Viña-Almunia J, Inglés M, Sanz-Ros J, Gambini J, Ibáñez-Cabellos JS, García-Giménez JL, Viña J, Borrás C. Role of p16INK4a and BMI-1 in oxidative stress-induced premature senescence in human dental pulp stem cells. Redox Biol. 2017;12:690–698. doi:10.1016/j.redox.2017.04.002.
- Wang E. Senescent human fibroblasts resist programmed cell death, and failure to suppress bcl2 is involved. Cancer Res. 1995;55:2284–2292.
- Lu M, Wang Z. Linking gut microbiota to aging process: a new target for anti-aging. Food Sci Human Well. 2018;7(2):111–119. doi:10.1016/j.fshw.2018.04.001.
- Yan J, Herzog JW, Tsang K, Brennan CA, Bower MA, Garrett WS, Sartor BR, Aliprantis AO, Charles JF. Gut microbiota induce IGF-1 and promote bone formation and growth. Proc Natl Acad Sci USA. 2016;113(47):E7554–E7563. doi:10.1073/pnas.1607235113.
- Kim S, Jazwinski SM. The gut microbiota and healthy aging: A mini-review. Gerontology. 2018;64(6):513–520. doi:10.1159/000490615.
- Beausoleil M, Fortier N, Guénette S, L’ecuyer A, Savoie M, Franco M, Lachaine J, Weiss K. Effect of a fermented milk combining Lactobacillus acidophilus Cl1285 and Lactobacillus casei in the prevention of antibiotic-associated diarrhea: a randomized, double-blind, placebo-controlled trial. Can J Gastroenterol. 2007;21(11):732–736. doi:10.1155/2007/720205.
- Sharma R, Kapila R, Dass G, Kapila S. Improvement in Th1/Th2 immune homeostasis, antioxidative status and resistance to pathogenic E. coli on consumption of probiotic Lactobacillus rhamnosus fermented milk in aging mice. Age (Dordr). 2014;36(4):9686. doi:10.1007/s11357-014-9686-4.
- Guillemard E, Tondu F, Lacoin F, Schrezenmeir J. Consumption of a fermented dairy product containing the probiotic Lactobacillus casei DN-114001 reduces the duration of respiratory infections in the elderly in a randomised controlled trial. Br J Nutr. 2010;103(1):58–68. doi:10.1017/S0007114509991395.
- Comalada M, Bailón E, de Haro O, Lara-Villoslada F, Xaus J, Zarzuelo A, Gálvez J. The effects of short-chain fatty acids on colon epithelial proliferation and survival depend on the cellular phenotype. J Cancer Res Clin Oncol. 2006;132(8):487–497. doi:10.1007/s00432-006-0092-x.
- Matsumoto M, Kurihara S. Probiotics-induced increase of large intestinal luminal polyamine concentration may promote longevity. Med Hypotheses. 2011;77(4):469–472. doi:10.1016/j.mehy.2011.06.011.
- Matsumoto M, Kurihara S, Kibe R, Ashida H, Benno Y. Longevity in mice is promoted by probiotic-induced suppression of colonic senescence dependent on upregulation of gut bacterial polyamine production. PLoS One. 2011;6(8):e23652. doi:10.1371/journal.pone.0023652.
- Zhao Y, Zhao L, Zheng X, Fu T, Guo H, Ren F. Lactobacillus salivarius strain FDB89 induced longevity in Caenorhabditis elegans by dietary restriction. J Microbiol. 2013;51(2):183–188. doi:10.1007/s12275-013-2076-2.
- Grompone G, Martorell P, Llopis S, González N, Genovés S, Mulet AP, Fernández-Calero T, Tiscornia I, Bollati-Fogolín M, Chambaud I, et al. Anti-inflammatory Lactobacillus rhamnosus CNCM I-3690 strain protects against oxidative stress and increases lifespan in Caenorhabditis elegans. PLoS One. 2012;7(12):e52493. doi:10.1371/journal.pone.0052493.
- Park MR, Oh S, Son SJ, Park DJ, Oh S, Kim SH, Jeong DY, Oh NS, Lee Y, Song M, et al. Bacillus licheniformis isolated from traditional Korean food resources enhances the longevity of Caenorhabditis elegans through serotonin signaling. J Agric Food Chem. 2015;63(47):10227–10233. doi:10.1021/acs.jafc.5b03730.
- Nakagawa H, Shiozaki T, Kobatake E, Hosoya T, Moriya T, Sakai F, Taru H, Miyazaki T. Effects and mechanisms of prolongevity induced by Lactobacillus gasseri SBT2055 in Caenorhabditis elegans. Aging Cell. 2016;15(2):227–236. doi:10.1111/acel.12431.
- Park MR, Ryu S, Maburutse BE, Oh NS, Kim SH, Oh S, Jeong SY, Jeong DY, Oh S, Kim Y. Probiotic Lactobacillus fermentum strain JDFM216 stimulates the longevity and immune response of Caenorhabditis elegans through a nuclear hormone receptor. Sci Rep. 2018;8(1):7441. doi:10.1038/s41598-018-25333-8.
- Westfall S, Lomis N, Prakash S. Longevity extension in Drosophila through gut-brain communication. Sci Rep. 2018;8(1):8362. doi:10.1038/s41598-018-25382-z.
- Schifano E, Zinno P, Guantario B, Roselli M, Marcoccia S, Devirgiliis C, Uccelletti D. The foodborne strain Lactobacillus fermentum MBC2 triggers pept-1-dependent pro-longevity effects in Caenorhabditis elegans. Microorganisms. 2019;7(2):pii: E45. doi:10.3390/microorganisms7020045.
- Lin X, Xia Y, Wang G, Xiong Z, Zhang H, Lai F, Ai L. Lactobacillus plantarum AR501 alleviates the oxidative Stress of d-galactose-induced aging mice liver by upregulation of Nrf2-mediated antioxidant enzyme expression. J Food Sci. 2018;83(7):1990–1998. doi:10.1111/1750-3841.14200.
- Zhao J, Tian F, Yan S, Zhai Q, Zhang H, Chen W. Lactobacillus plantarum CCFM10 alleviating oxidative stress and restoring the gut microbiota in d-galactose-induced aging mice. Food Funct. 2018;9(2):917–924. doi:10.1039/c7fo01574g.
- Hor YY, Lew LC, Jaafar MH, Lau AS, Ong JS, Kato T, Nakanishi Y, Azzam G, Azlan A, Ohno H, et al. Lactobacillus sp. improved microbiota and metabolite profiles of aging rats. Pharmacol Res. 2019;146:104312. doi:10.1016/j.phrs.2019.104312.
- Lew LC, Hor YY, Jaafar MH, Lau AS, Khoo BY, Sasidharan S, Choi SB, Ong KL, Kato T, Nakanishi Y, et al. Effects of potential probiotic strains on the fecal microbiota and metabolites of d-galactose-induced aging rats fed with high-fat diet. Probiotics Antimicrob Proteins. 2019. doi:10.1007/s12602-019-09545-6.
- Jeong JJ, Kim KA, Hwang YJ, Han MJ, Kim DH. Anti-inflammaging effects of Lactobacillus brevis OW38 in aged mice. Benef Microbes. 2016;7(5):707–718. doi:10.3920/BM2016.0016.
- Lee J, Yang W, Hostetler A, Schultz N, Suckow MA, Stewart KL, Kim DD, Kim HS. Characterization of the anti-inflammatory Lactobacillus reuteri BM36301 and its probiotic benefits on aged mice. BMC Microbiol. 2016;16:69. doi:10.1186/s12866-016-0686-7.
- Pan F, Zhang L, Li M, Hu Y, Zeng B, Yuan H, Zhao L, Zhang C. Predominant gut Lactobacillus murinus strain mediates anti-inflammaging effects in calorie-restricted mice. Microbiome. 2018;6(1):54. doi:10.1186/s40168-018-0440-5.
- Fülöp T, Dupuis G, Witkowski JM, Larbi A. The role of immunosenescence in the development of age-related diseases. Rev Invest Clin. 2016;68:84–91.
- Fuentes E, Fuentes M, Alarcón M, Palomo I. Immune system dysfunction in the elderly. An Acad Bras Cienc. 2017;89(1):285–299. doi:10.1590/0001-3765201720160487.
- Kawase M, He F, Miyazawa K, Kubota A, Yoda K, Hiramatsu M. Orally administered heat-killed Lactobacillus gasseri TMC0356 can upregulate cell-mediated immunity in senescence-accelerated mice. FEMS Microbiol Lett. 2012;326(2):125–130. doi:10.1111/j.1574-6968.2011.02440.x.
- Spaiser SJ, Culpepper T, Nieves C Jr, Ukhanova M, Mai V, Percival SS, Christman MC, Langkamp-Henken B. Lactobacillus gasseri KS-13, Bifidobacterium bifidum G9-1, and Bifidobacterium longum MM-2 ingestion induces a less inflammatory cytokine profile and a potentially beneficial shift in gut microbiota in older adults: a randomized, double-blind, placebo-controlled, crossover study. J Am Coll Nutr. 2015;34(6):459–469. doi:10.1080/07315724.2014.983249.
- Miyazawa K, Kawase M, Kubota A, Yoda K, Harata G, Hosoda M, He F. Heat-killed Lactobacillus gasseri can enhance immunity in the elderly in a double-blind, placebo-controlled clinical study. Benef Microbes. 2015;6(4):441–449. doi:10.3920/BM2014.0108.
- Vidal K, Benyacoub J, Moser M, Sanchez-Garcia J, Serrant P, Segura-Roggero I, Reuteler G, Blum S. Effect of Lactobacillus paracasei NCC2461 on antigen-specific T-cell mediated immune responses in aged mice. Rejuvenation Res. 2008;11(5):957–964. doi:10.1089/rej.2008.0780.
- Lefevre M, Racedo SM, Ripert G, Housez B, Cazaubiel M, Maudet C, Jüsten P, Marteau P, Urdaci MC. Probiotic strain Bacillus subtilis CU1 stimulates immune system of elderly during common infectious disease period: a randomized, double-blind placebo-controlled study. Immun Ageing. 2015;12:24. doi:10.1186/s12979-015-0051-y.
- Hunsche C, Cruces J, de la Fuente M. Improvement of redox state and functions of immune cells as well as of behavioral response in aged mice after two-week supplementation of fermented milk with probiotics. Curr Microbiol. 2019;76(11):1278–1289. doi:10.1007/s00284-019-01759-9.
- Burton DGA, Stolzing A. Cellular senescence: immunosurveillance and future immunotherapy. Ageing Res Rev. 2018;43:17–25. doi:10.1016/j.arr.2018.02.001.
- Jeong JJ, Kim KA, Jang SE, Woo JY, Han MJ, Kim DH. Orally administrated Lactobacillus pentosus var. plantarum C29 ameliorates age-dependent colitis by inhibiting the nuclear factor kappa B signaling pathway via the regulation of lipopolysaccharide production by gut microbiota. PLoS One. 2015;10(2):e0116533. doi:10.1371/journal.pone.0116533.
- Jeong JJ, Woo JY, Ahn YT, Shim JH, Huh CS, Im SH, Han MJ, Kim DH. The probiotic mixture IRT5 ameliorates age-dependent colitis in rats. Int Immunopharmacol. 2015;26(2):416–422. doi:10.1016/j.intimp.2015.04.021.
- Kumar R, Sharma A, Gupta M, Padwad Y, Sharma R. Cell-free culture supernatant of probiotic Lactobacillus fermentum protects against H2O2-induced premature senescence by suppressing ROS-Akt-mTOR axis in murine preadipocytes. Probiotics Antimicrob Proteins. 2019. doi:10.1007/s12602-019-09576-z.
- Demidenko ZN, Zubova SG, Bukreeva EI, Pospelov VA, Pospelova TV, Blagosklonny MV. Rapamycin decelerates cellular senescence. Cell Cycle. 2009;8(12):1888–1895. doi:10.4161/cc.8.12.8606.
- Bjedov I, Toivonen JM, Kerr F, Slack C, Jacobson J, Foley A, Partridge L. Mechanisms of life span extension by rapamycin in the fruit fly Drosophila melanogaster. Cell Metab. 2010;11(1):35–46. doi:10.1016/j.cmet.2009.11.010.
- Sharon G, Garg N, Debelius J, Knight R, Dorrestein Pieter C, Mazmanian Sarkis K. Specialized metabolites from the microbiome in health and disease. Cell Metab. 2014;20(5):719–730. doi:10.1016/j.cmet.2014.10.016.
- Wikoff WR, Anfora AT, Liu J, Schultz PG, Lesley SA, Peters EC, Siuzdak G. Metabolomics analysis reveals large effects of gut microflora on mammalian blood metabolites. Proc Natl Acad Sci USA. 2009;106(10):3698–3703. doi:10.1073/pnas.0812874106.
- Mazmanian SK, Round JL, Kasper DL. A microbial symbiosis factor prevents intestinal inflammatory disease. Nature. 2008;453:620–625. doi:10.1038/nature07008.
- Matsuki T, Pédron T, Regnault B, Mulet C, Hara T, Sansonetti PJ. Epithelial cell proliferation arrest induced by lactate and acetate from Lactobacillus casei and Bifidobacterium breve. PLoS One. 2013;8:e63053. doi:10.1371/journal.pone.0063053.
- Corr SC, Li Y, Riedel CU, O’Toole PW, Hill C, Gahan CG. Bacteriocin production as a mechanism for the anti-infective activity of Lactobacillus salivarius UCC118. P Natl Acad Sci USA. 2007;104:7617–7621. doi:10.1073/pnas.0700440104.
- Zhang L, Liu C, Li D, Zhao Y, Zhang X, Zeng X, Yang Z, Li S. Antioxidant activity of an exopolysaccharide isolated from Lactobacillus plantarum C88. Int J Biol Macromol. 2013;54:270–275. doi:10.1016/j.ijbiomac.2012.12.037.
- Tsilingiri K, Rescigno M. Postbiotics: what else? Benef Microbes. 2013;4(1):101–107. doi:10.3920/BM2012.0046.
- Wu R, Jeffrey M, Johnson-Henry K, Green-Johnson J, Sherman P. Impact of prebiotics, probiotics and gut derived metabolites on host immunity. LymphoSign J. 2016;4(1):1–24. doi:10.14785/lymphosign-2016-0012.
- Salazar N, Arboleya S, Fernández-Navarro T, de Los Reyes-gavilán CG, Gonzalez S, Gueimonde M. Age-associated changes in gut microbiota and dietary components related with the immune system in adulthood and old age: a cross-sectional study. Nutrients. 2019;11(8):pii: E1765. doi:10.3390/nu11081765.
- Lee H, Lee Y, Kim J, An J, Lee S, Kong H, Song Y, Lee CK, Kim K. Modulation of the gut microbiota by metformin improves metabolic profiles in aged obese mice. Gut Microbes. 2018;9(2):155–165. doi:10.1080/19490976.2017.1405209.
- Erny D, Hrabě de Angelis AL, Jaitin D, Wieghofer P, Staszewski O, David E, Keren-Shaul H, Mahlakoiv T, Jakobshagen K, Buch T, et al. Host microbiota constantly control maturation and function of microglia in the CNS. Nat Neurosci. 2015;18(7):965–977. doi:10.1038/nn.4030.
- Gao Z, Yin J, Zhang J, Ward RE, Martin RJ, Lefevre M, Cefalu WT, Ye J. Butyrate improves insulin sensitivity and increases energy expenditure in mice. Diabetes. 2009;58(7):1509–1517. doi:10.2337/db08-1637.
- Matt SM, Allen JM, Lawson MA, Mailing LJ, Woods JA, Johnson RW. Butyrate and dietary soluble fiber improve neuroinflammation associated with aging in mice. Front Immunol. 2018;9:1832. doi:10.3389/fimmu.2018.01832.
- Smith BJ, Miller RA, Ericsson AC, Harrison DC, Strong R, Schmidt TM. Changes in the gut microbiome and fermentation products concurrent with enhanced longevity in acarbose-treated mice. BMC Microbiol. 2019;19(1):130. doi:10.1186/s12866-019-1494-7.
- Minois N, Carmona-Gutierrez D, Madeo F. Polyamines in aging and disease. Aging (Albany NY). 2011;3(8):716–732. doi:10.18632/aging.v3i8.
- Nishimura K, Shiina R, Kashiwagi K, Igarashi K. Decrease in polyamines with aging and their ingestion from food and drink. J Biochem. 2006;139(1):81–90. doi:10.1093/jb/mvj003.
- Eisenberg T, Knauer H, Schauer A, Büttner S, Ruckenstuhl C, Carmona-Gutierrez D, Ring J, Schroeder S, Magnes C, Antonacci L, et al. Induction of autophagy by spermidine promotes longevity. Nat Cell Biol. 2009;11(11):1305–1314. doi:10.1038/ncb1975.
- Eisenberg T, Abdellatif M, Schroeder S, Primessnig U, Stekovic S, Pendl T, Harger A, Schipke J, Zimmermann A, Schmidt A, et al. Cardioprotection and lifespan extension by the natural polyamine spermidine. Nat Med. 2016;22(12):1428–1438. doi:10.1038/nm.4222.
- Madeo F, Carmona-Gutierrez D, Kepp O, Kroemer G. Spermidine delays aging in humans. Aging (Albany NY). 2018;10(8):2209–2211. doi:10.18632/aging.101517.
- Lee HC, Jenner AM, Low CS, Lee YK. Effect of tea phenolics and their aromatic fecal bacterial metabolites on intestinal microbiota. Res Microbiol. 2006;157(9):876–884. doi:10.1016/j.resmic.2006.07.004.
- Su P, Henriksson A, Nilsson C, Mitchell H. Synergistic effect of green tea extract and probiotics on the pathogenic bacteria, Staphylococcus aureus and Streptococcus pyogenes. World J Microbiol Biotechnol. 2008;24:1837–1842. doi:10.1007/s11274-008-9682-x.
- Sourabh A, Kanwar SS, Sud RG, Ghabru A, Sharma OP. Influence of phenolic compounds of Kangra tea [Camellia sinensis (L) O Kuntze] on bacterial pathogens and indigenous bacterial probiotics of Western Himalayas. Braz J Microbiol. 2014;44(3):709–715. doi:10.1590/S1517-83822013000300007.
- Paraiso IL, Plagmann LS, Yang L, Zielke R, Gombart AF, Maier CS, Sikora AE, Blakemore PR, Stevens JF. Reductive metabolism of xanthohumol and 8-prenylnaringenin by the intestinal bacterium Eubacterium ramulus. Mol Nutr Food Res. 2019;63(2):e1800923. doi:10.1002/mnfr.201800923.
- Ishiwata N, Melby MK, Mizuno S, Watanabe S. New equol supplement for relieving menopausal symptoms: randomized, placebo-controlled trial of Japanese women. Menopause. 2009;16:141–148. doi:10.1097/gme.0b013e31818379fa.
- Wang LC, Pan TM, Tsai TY. Lactic acid bacteria-fermented product of green tea and Houttuynia cordata leaves exerts anti-adipogenic and anti-obesity effects. J Food Drug Anal. 2018;26(3):973–984. doi:10.1016/j.jfda.2017.11.009.
- Park JH, Kim Y, Kim SH. Green tea extract (Camellia sinensis) fermented by Lactobacillus fermentum attenuates alcohol-induced liver damage. Biosci Biotechnol Biochem. 2012;76(12):2294–2300. doi:10.1271/bbb.120598.
- Banerjee A, Dhar P. Amalgamation of polyphenols and probiotics induce health promotion. Crit Rev Food Sci Nutr. 2018:1–24. doi:10.1080/10408398.2018.1478795.
- Sharma R, Kumari M, Kumari A, Sharma A, Gulati A, Gupta M, Padwad Y. Diet supplemented with phytochemical epigallocatechin gallate and probiotic Lactobacillus fermentum confers second generation synbiotic effects by modulating cellular immune responses and antioxidant capacity in aging mice. Eur J Nutr. 2019;58(7):2943–2957. doi:10.1007/s00394-018-01890-6.