ABSTRACT
Targeting the gut-liver axis by modulating the gut-microbiome can be a promising therapeutic approach in nonalcoholic fatty liver disease (NAFLD). The aim of this study was to evaluate the effects of single species and a combination of Lactobacillus and Pediococcus in NAFLD mice model. Six-week male C57BL/6J mice were divided into 9 groups (n = 10/group; normal, Western diet, and 7 Western diet-strains [109 CFU/g, 8 weeks]). The strains used were L. bulgaricus, L. casei, L. helveticus, P. pentosaceus KID7, and three combinations (1: L. casei+L. helveticus, 2: L. casei+L. helveticus+P. pentosaceus KID7, and 3: L. casei+L. helveticus+L. bulgaricus). Liver/Body weight ratio, serum and stool analysis, liver pathology, and metagenomics by 16S rRNA-sequencing were examined. In the liver/body ratio, L. bulgaricus (5.1 ± 0.5), L. helveticus (5.2 ± 0.4), P. pentosaceus KID7 (5.5 ± 0.5), and combination1 and 2 (4.2 ± 0.6 and 4.8 ± 0.7) showed significant reductions compared with Western (6.2 ± 0.6)(p < 0.001). In terms of cholesterol and steatosis/inflammation/NAFLD activity, all groups except for L. casei were associated with an improvement (p < .05). The elevated level of tumor necrosis factor-α/interleukin-1β (pg/ml) in Western (65.8 ± 7.9/163.8 ± 12.2) was found to be significantly reduced in L. bulgaricus (24.2 ± 1.0/58.9 ± 15.3), L. casei (35.6 ± 2.1/62.9 ± 6.0), L. helveticus (43.4 ± 3.2/53.6 ± 7.5), and P. pentosaceus KID7 (22.9 ± 3.4/59.7 ± 12.2)(p < 0.01). Cytokines were improved in the combination groups. In metagenomics, each strains revealed a different composition and elevated Firmicutes/Bacteroidetes ratio in the western (47.1) was decreased in L. bulgaricus (14.5), L. helveticus (3.0), and P. pentosaceus KID7 (13.3). L. bulgaricus, L. casei, L. helveticus, and P. pentosaceus KID7 supplementation can improve NAFLD-progression by modulating gut-microbiome and inflammatory pathway.
Introduction
Nonalcoholic fatty liver disease (NAFLD), the pathogenesis of which is associated with alterations in hepatic metabolism in response to metabolic syndrome, is among the most widespread liver diseases globally.Citation1 NAFLD has become markedly more common in all age groups worldwide because of the increase in the prevalence of obesity.Citation2 However, NAFLD includes a broad range of manifestations from mild to severe form; fatty steatosis occurs when more than 5% of hepatocytes show fatty infiltration and nonalcoholic steatohepatitis (NASH) places patients at risk for progression to cirrhosis and hepatocellular carcinoma (HCC).Citation3 Genetic factors, the gut microbiome, lipid and glucose metabolism, and fat accumulation are implicated in the pathophysiology of NAFLD.Citation4 Patients with stage 3 fibrosis (bridging fibrosis) or cirrhosis are at a substantially higher risk of death from liver disease.Citation5
Current perspectives on the disease and promising treatment modalities have been transformed by recent advances in the basic and applied knowledge of the gut microbiota in humans.Citation6 The human gut microbiota has been established to be a complex ecosystem comprising a diverse range of bacterial species, the total mass of which is approximately 1–2 kg.Citation7,Citation8 Furthermore, bacterial species in the gut have a close relationship with the host and play important roles in bacterial translocation, mucosal immune system, and the production of vitamins.Citation9 Disturbances in the gut microbiota have been causally linked to liver diseases including NAFLD, because the intestines are directly connected to the liver through the portal tract which mediates the transfer of nutrients and microbiota-related components along the gut-liver axis.Citation10-Citation13
Therapies for NAFLD are often ineffective due to poor compliance, lack of efficacy, or change in diet and lifestyle. Theoretically, modulation of the gut microbiota by administering probiotics can be effective, and recent studies have shown some promise and have supported this theory.Citation10,Citation14,Citation15 Lactobacillus bulgaricus, L. casei, L. helveticus, and Pediococcus pentosaceus KID7 are strains known to be beneficial in various diseases, including enteritis, inflammation, and hypertension ().Citation16,Citation17,Citation19,Citation20 In this study, we evaluated the effects of L. bulgaricus, L. casei, L. helveticus, P. pentosaceus KID7, and combinations of each strain in a NAFLD mouse model ().
Table 1. Information of used probiotics strains.
Results
Liver/body weight ratio
In the analysis of the liver/body weight ratio (%), the L. bulgaricus (5.05 ± 0.46), L. helveticus (5.15 ± 0.38), P. pentosaceus KID7 (5.47 ± 0.48), combination 1 (4.24 ± 0.63), and combination 2 (4.8 ± 0.65) groups were associated with significant improvement compared with the Western diet group (6.16 ± 0.56) (p < 0.001) (). The L. casei (5.67 ± 1.07) and combination 3 groups (5.84 ± 0.82) showed no significant difference in the liver/body weight ratio (p > 0.05).
Liver function test and serum cholesterol level
In the liver function test, the Western diet group demonstrated significantly elevated liver enzyme (AST) levels compared with the normal control group (p < 0.05). The mean levels of AST in the L. bulgaricus (106 ± 22 U/L) and L. helveticus (114 ± 19 U/L) groups were lower than those in the Western diet group (181 ± 42 U/L, p < 0.05). All of the strains caused a significant decrease in the serum total cholesterol level compared with the Western diet group (p < 0.05) ().
Table 2. Liver function test and serum total cholesterol level of mice.
Pathologic findings
The 9-week Western diet apparently induced fatty liver, but the probiotic strains significantly inhibited the progression of fatty liver. Differences in steatosis (χ2 = 123.16, p < 0.001) and inflammation (χ2 = 76.56, p < 0.001) scores were significant according to the dietary group (). Moderate to marked macrovesicular steatosis developed in the Western diet group (). Additionally, large fat vacuoles displaced the nuclei to the edges of the cells, representing minimal to mild mixed macro/microvesicular steatosis. The steatosis grade in strain groups was lower than that of the Western diet group (p < 0.05)(c-i). Regarding steatosis scores, all of the Western diet groups had a score of 3, whereas the strain groups generally had scores of 1 and 2 ().
Figure 3. Pathological effects of probiotics on the liver (Hematoxylin and Eosin stain). (a) Steatosis grade. normal control (a), Western diet (b), L. bulgaricus (c), L. casei (d), L. helveticus (e), P. pentosaceus KID7 (f), combination 1 (g), combination 2 (h), and combination 3 (i). (B) Inflammation grade. Mild to moderate inflammation, favoring lymphocytes, is identified in the perivenular area (b, arrow).
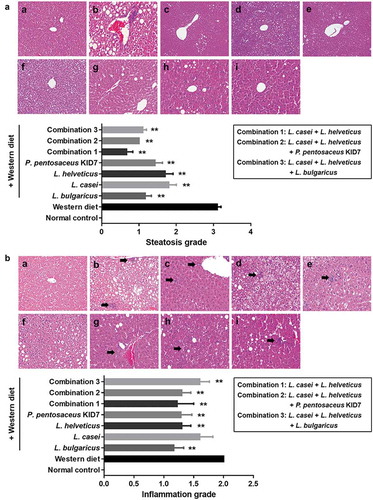
Regarding the hepatitis scores, all of the Western diet group had a score of 2, whereas the strain groups mostly had a score of 1. Only L. casei showed nonsignificance in the comparison of hepatitis scores (). Minimal to mild inflammation was demonstrated in the Western diet and strain groups.
Concerning the NAFLD activity score (NAS), the Western diet-induced increase (5.0 ± 1.6) was significantly decreased in the L. bulgaricus (2.4 ± 0.8), L. helveticus (3.4 ± 1.3), P. pentosaceus KID7 (3.4 ± 1.3), combination 1 (2.2 ± 1.8), combination 2 (2.8 ± 0.6), and combination 3 (3.3 ± 1.3) groups (p < 0.05) (). However, the L. casei group (4.7 ± 1.8) did not show improvement in the NAS (p = 0.733). The NAS was significantly reduced in the Western diet with strain fed groups.
Figure 4. Gut-liver axis analysis. (a) NAFLD Activity Score (NAS). (b) Immunohistochemical analyses for CD68 in representative cases. normal control (a), Western diet (b), L. bulgaricus (c), L. casei (d), L. helveticus (e), P. pentosaceus KID7 (f), combination 1 (g), combination 2 (h), and combination 3 (i). x400. (C) Stool endotoxin level. (D) Trans-epithelial electrical resistance (TEER) measurement.
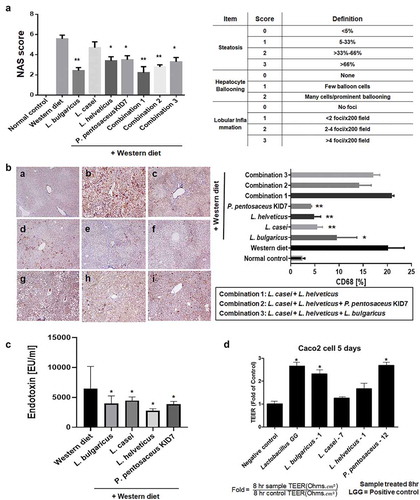
Immunohistochemical analyses for CD68, marker for macrophages, in representative cases. Mean value of positive-stained area measured in 10 random areas of liver, respectively: a. normal control (2.4 ± 1.6%), b. Western diet (20.1 ± 6.1%), c. L. bulgaricus (9.6 ± 6.6%), d. L. casei (5.5 ± 3.2%), e. L. helveticus (4.9 ± 2.8%), f. P. pentosaceus KID7 (4.2 ± 1.9%), g. combination 1 (20.9 ± 4.1%), h. combination 2 (14.1 ± 5.6%), i. combination 3 (17.1 ± 5.3%)(). The L. bulgaricus, L. casei, L. helveticus, and P. pentosaceus KID7 showed a significant reduction of the stained area compared to the area of Western diet group (p < 0.05).
Stool endotoxin levels
Elevated levels of endotoxin in the Western group (6,475 ± 5,231 EU/ml) were significantly reduced in the L. bulgaricus (3,979 ± 1,779 EU/ml), L. casei (4,468 ± 864 EU/ml), L. helveticus (2,773 ± 472 EU/ml), and P. pentosaceus KID7 (3,850 ± 687 EU/ml) groups (p < 0.05) ().
Trans-epithelial electrical resistance measurement
Caco-2 cells were cultured for complete confluence and co-incubated with bacterial suspension in MEM media for 8 h. While the L. casei and L. helveticus had no effect on trans-epithelial electrical resistance (TEER) of Caco-2 cells, treatment of Caco-2 cells with the L. bulgaricus and P. pentosaceus KID7 increased TEER values by 2.3- and 2.7-fold, respectively, when compared with those of no-treatment controls (). These results suggest that the L. bulgaricus and P. Pentosaceus KID7 increased gut-barrier function between Caco-2 cells and might regulate endotoxin infiltration in the gut.
Stool analysis for metagenomics
In the phylum analysis of stool samples, the compositions of Proteobacteria, Verrucomicrobia, Deferribacteres, Actinobacteria, Bacteroidetes, Firmicutes, and others (under 1% on average) were different according to the diet groups (normal control [3%, 2%, 0%, 2%, 52%, 41%, and 1%], Western diet [1%, 7%, 5%, 4%, 2%, 72%, and 0%], L. bulgaricus [7%, 19%, 0%, 0%, 5%, 68%, and 1%], L. casei [8%, 9%, 2%, 0%, 41%, 38%, and 0%], L. helveticus [7%, 33%, 2%, 0%, 14%, 43%, and 1%] and P. pentosaceus KID7 [8%, 6%, 2%, 3%, 6%, 75%, and 1%]) (). We evaluated the significant difference between groups by utilizing distance measures of beta diversity. In the analytics for beta diversity for the relationship between microbiome taxonomic profiling, each group showed a different location (). Alpha diversity using OTUs was 446 ± 69, 156 ± 18, 166 ± 10, 374 ± 90, 212 ± 43, and 161 ± 17 in normal control, Western diet, L. bulgaricus, L. casei, L. helveticus, and P. pentosaceus KID7 group, respectively. In the comparison of 10 prevalent species that commonly exist in groups, the composition was different among groups and the most common strains were L. murinus, Helicobacter japonicas, Akkermansia muciniphila, B. vulgatus A. muciniphila and L. murinus in normal control, Western diet, L. bulgaricus, L. casei, L. helveticus, and P. pentosaceus KID7 group, respectively (). We selected 16 species with a high percentage in each group (not all species). Percentages of the upper 16 strains were 46% in normal group and 76-84% in Western and Western+strain groups ().
Table 3. Comparison of species among groups.
Figure 5. Results of animal stool analysis. (a) Phylum analysis. (b) Analytics for beta diversity for the relationship between microbiome taxonomic profiling (principle coordinates analysis, Jensen-Shannon, species, include unclassified OTUs). (c) Comparison of the 10 prevalent species. (d) Firmicutes/Bacteroidetes ratio. (e) Heatmap for comparison of species diversity in mice feces.
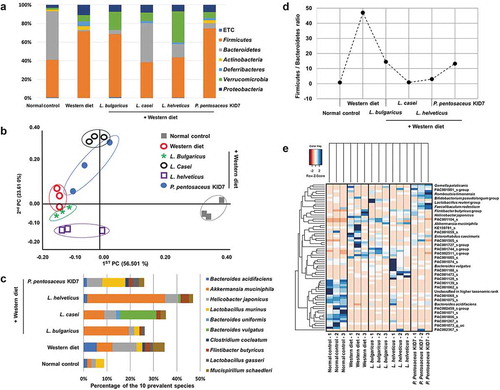
Firmicutes and Bacteroidetes are two major phyla of the domain bacteria and are dominant in human gut microbiota.Citation21 The Firmicutes-to-Bacteroidetes ratio (F/B ratio, the Bacteroides and Firmicutes relative abundance changed) has been extensively studied in human and mouse gut microbiota.Citation22 Multiple studies have shown that the F/B ratio is correlated with obesity and other diseases: the Western diet increases the number of the Firmicutes phylum and decreases the number of the Bacteroidetes phylum. The elevated F/B ratio in the Western diet group (47.1) was decreased in the L. bulgaricus (14.5), L. casei (0.9), L. helveticus (3.0), and P. pentosaceus KID7 (13.3) groups ().
Interestingly, A. muciniphila which is known to be beneficial microbiome, was increased in the L. bulgaricus [19.4%], L. casei [8.8%], and L. helveticus [33.4%] groups compared with Western group ( and ).
Primary metabolite profiling of the cecal samples
We evaluated stool metabolites of P. pentosaceus KID7 group because the P. pentosaceus KID7 group revealed a moderate effect among the four strains. In the mass-spectrometric analysis of primary metabolites, differential metabolic modulation was observed according to the types of diet and supplementation (normal control group vs. P. pentosaceus KID7 treatment group vs. western diet group). Hierarchical clustering analysis captured two distinctive clusters that presented comparable abundances in metabolite composition between normal diet group and KID7-treated group (). Glucose, mannose, sorbitol, threonic acid, oleamide, and uracil showed the significantly higher levels in both the normal control and P. pentosaceus KID7-treated groups than in the western diet group. By contrary, the levels of certain amino acids (glutamate and proline), dihydroxyacetone, urea, and stearic acid were significantly higher in the Western diet group. Additionally, unsaturated fatty acid (oleic acid) and cholesterol were at marginally higher abundances in the Western diet group.
Figure 6. Unique primary metabolic profiles of the cecal samples according to different strains. (a) The heatmap generated by hierarchical clustering analysis of 140 metabolites from the cecal samples. (b) Box-and-whisker plots of the metabolites that represent characteristic abundances in P. pentosaceus KID7 treatment group compatible with the normal control group.
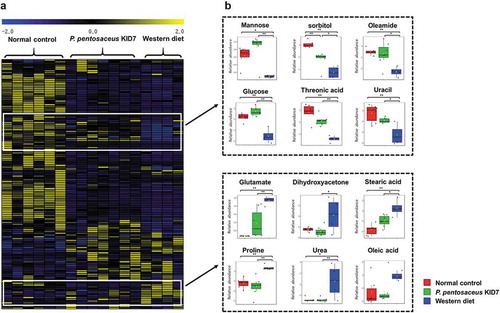
Inflammatory cytokines in liver tissue
Enzyme-linked immunosorbent assays revealed elevated levels of TNF-α, IL-1β, and IL-6 in the Western diet group (65.8 ± 7.9, 163.8 ± 12.2, and 633.6 ± 25.8 pg/ml, respectively), and these levels were significantly reduced in the L. bulgaricus (24.2 ± 1.01, 58.9 ± 15.3, and 388.3 ± 42.0 pg/ml, respectively), L. casei (35.6 ± 2.1, 62.9 ± 6.0, and 323.2 ± 30.6 pg/ml, respectively), L. helveticus (43.4 ± 3.2, 53.6 ± 7.5, and 337.4 ± 12.8 pg/ml, respectively), and P. pentosaceus KID7 (22.9 ± 3.4, 59.7 ± 12.2, and 412.0 ± 20.6 pg/ml, respectively) groups (p < .01)().
Figure 7. Probiotics block proinflammatory cytokines. (a) Enzyme-linked immunosorbent assay. (b and c) Real-time reverse transcription-polymerase chain reaction.
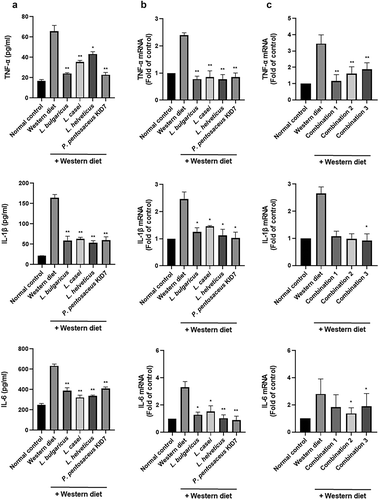
Real-time reverse transcription-polymerase chain reaction showed the same results (,). Probiotics-fed groups were protected against the Western diet. Strain groups and combination groups showed improvement in the levels of inflammatory TNF-α, IL-1β, and IL-6. In the comparison between the Western diet group and combination groups, all combination groups showed improvement in the level of TNF-α.
Discussion
Dysbiosis of intestinal microorganisms causes metabolic imbalance and can lead to various diseases such as irritable bowel disease, diabetes, or coronary heart disease.Citation23-Citation25 New therapeutic approaches for the modulation of dysbiosis, including the administration of probiotics, prebiotics, synbiotics, pharmabiotics, or fecal microbiota transplantation, have been proposed and have shown their potential as a treatment in recent studies.Citation10,Citation26 Probiotics are living organisms that can be orally administered and promote the health and growth of humans or animals while providing benefits above the nutritional values.Citation16
In our study, improvements were found in the liver/body weight ratio in the L. bulgaricus, L. helveticus, and P. pentosaceus KID7 groups compared with that in the Western diet group. A previous study reported that L. kefiri improved high-fat-diet-induced obesity without changing the liver weight.Citation27 Another report using L. rhamnosus GG showed the same result in the liver/body weight ratio.Citation28 Altogether, the data showed that strains such as L. bulgaricus, L. helveticus, and P. pentosaceus KID7 are effective for liver/body weight ratio reduction in mouse models of NAFLD. L. casei also improved liver/body weight ratio and NAS score. However, the difference was not statistically significant. Thus, L. casei is effective in improving steatosis not liver inflammation.
In a recent study, some Lactobacillus species were found to promote good health while at the same time exhibiting detrimental effects, indicating that the human microbiome is a complex ecosystem with diversity.Citation29,Citation30 In our results, the combination 3 group did not show better results than the single-strain groups, although that group contained L. helveticus and L. bulgaricus. Additionally, L. casei exhibited the greatest restoration of the F:B ratio, but had the least effect on the liver/body weight ratio. This discrepancy might be due to the complex ecosystem between the host and bacteria, with each strain having different functions and different growth positions according to the situation. However, there is little known to date, and further research on the efficacy and mechanism of species on NAFLD is needed.
Most probiotic studies have used a single species, and few studies have evaluated the efficacy of a mixture of species. Particularly, it is not well known whether the mixing of species is less effective due to mutual inhibition by different species or is more potent due to additive or even synergistic effects. A recent meta-analysis suggested that the administration of mixtures containing B. breve, B. longum, Streptococcus salivarius subsp. thermophilus, L. acidophilus, L. casei group, and L. delbrueckii were associated with reductions in the body weight, body mass index, and liver enzymes.Citation31 A previous meta-analysis suggested that different Lactobacillus species are associated with different effects on weight change that are largely host-specific.Citation32 In our results, the liver/body weight ratio was improved in all of the combination groups. However, statistical significance was only observed in the L. casei + L. helveticus and L. casei + L. helveticus + P. pentosaceus KID7 groups. Although the L. casei + L. helveticus + L. bulgaricus combination group showed no difference, all combination groups revealed synergistic effects on improving steatosis and inflammation.
P. pentosaceus KID7 is an engineered strain that exhibits a beneficial effect through cholesterol-lowering effects and inhibition of intestinal bacterial growth.Citation33 In a previous report, low-density lipoprotein receptor, cholesterol-7α-hydroxylase, and apolipoprotein E mRNA expression were significantly increased in the P. pentosaceus KID7 treatment group.Citation33 In this study, cholesterol levels were significantly improved in the P. pentosaceus KID7 and the three combination groups. Additionally, the cecal cholesterol levels were marginally downregulated by P. pentosaceus KID7-treatment (p = 0.09). Considering that cholesterol is another risk factor for NAFLD and that elevated serum lipid levels are involved in the accumulation of fat droplets observed in the liver, P. pentosaceus KID7 can be considered as a therapeutic candidate for cholesterol-lowering agents in NAFLD pending further clinical trials.Citation33
L. bulgaricus is the main microbe used to produce yogurt and VSL#3, and it has been demonstrated to significantly prevent liver injury and alleviates histopathological changes by downregulation of NF-κB p65 expression.Citation34 By contrast, probiotic capsules containing L. acidophilus, L. bulgaricus, B. lactis, and S. thermophile showed no effect in improving the progression of liver cirrhosis. In our present study, L. bulgaricus was associated with the improvement of NAFLD progression and might be regarded as a candidate for NAFLD treatment.
In the present study, the Western diet was associated with elevated levels of serum chemistry markers (AST, ALT, and serum cholesterol) compared with the normal diet, a find in that is consistent with the findings of Amin et al.Citation35 Elevated AST and ALT levels are widely established as markers of hepatic injury, with possible involvement in the progression of NAFLD. In pathologic analysis, probiotics ameliorated Western diet–induced macro and microvesicular steatosis and lobular inflammation. A recent meta-analysis showed that probiotics exerted beneficial effects on cholesterol levels and liver enzymes in patients with NAFLD.Citation36 Other studies have additionally found that oral antibiotic and probiotic treatment slowed the progression of NAFLD to NASH in obese animals and improved portal circulation hemodynamics in patients with cirrhosis and portal hypertension.Citation37,Citation38 Taken together, these results indicate that probiotics provide an effective means to reduce liver inflammation and steatosis in NAFLD. However, the use of probiotics in NAFLD and NASH patients has not been directly supported or contraindicated by randomized clinical trials,Citation39 indicating that further well-designed long-term clinical trials are needed to assess the effects of probiotics on NAFLD and steatohepatitis.
A Western diet can also contribute to alterations in the composition of the intestinal microbiome, which can increase bacterial lipopolysaccharide levels and gut permeability. Some intestinal microbiota can lead to endotoxemia, which is related to Kupffer cell activation and the inflammatory cytokine pathway in the liver.Citation40 In our present study, elevated stool-endotoxin levels in the Western group were reduced in the strain groups and each strain showed improvement in gut leakage analysis using Caco2 cells. In an immunohistochemical staining for macrophages, the CD68 stained area was significantly decreased in the strain groups. Elevated levels of TNF-α, IL-1β, and IL-6 in the Western group were significantly reduced by probiotics. In a previous clinical study, probiotic treatment with Lactobacillus, Lactococcus, Propionic bacterium, Bifidobacterium, and Acetic bacterium was shown to be associated with reduced levels of inflammatory cytokines such as IL-1β, TNF-α, IL-6 and IL-8 in patients with NAFLD.Citation41 The above results suggest that probiotics are effective in improving NAFLD outcomes through controlling the inflammatory pathway in the liver.
It is conceivable that the gut-microbiota-liver communication plays an important role in the pathophysiology of NAFLD. Probiotics can modulate the components of the gut-liver axis, especially gut microbiota. In a rodent model, the Enterococcus fecal population size, which is larger in patients with NASH, was found to be reduced following the administration of L. casei Shirota.Citation42 In this study, probiotic treatment altered Western diet-induced dysbiosis and improved elevated the F/B ratio in the Western group. Interestingly, A. muciniphila and F. rodentium which are well-functioning microbiota in humans, were increased in the L. bulgaricus, L. helveticus, and P. pentosaceus KID7 groups. In the metabolomics, differential metabolic modulation was observed according to the types of diet and supplementation, indicating probiotic strains act by changing endotoxins and metabolites on the host. Therefore, probiotics improve the gut environment by modulating dysbiosis and, consequently, demonstrate potential effectiveness in the treatment of NAFLD.
Currently, common treatments for NAFLD include lifestyle changes with healthy diets and exercise. Although therapeutic options for patients are now emerging with NAFLD. Vitamin E and obeticholic acid have shown protective effects in randomized controlled trials, these options have not been approved by the US FDA. Our results suggest that treatment with probiotics can be used to improve the prognosis of NAFLD and inhibit its progression.
In conclusion, the intestinal axis appears to play an important role in the pathogenesis of NAFLD. Therefore, it would be logical to identify a method that alters of this axis, especially the intestinal microbiota. Probiotic treatment with L. bulgaricus, L. helveticus, and P. pentosaceus KID7 improved steatohepatitis through the modulation of gut microbiota composition and the inflammatory pathway in the gut liver axis of NAFLD (). The use of mixed strains requires further study. Overall, this study highlights the therapeutic potential of probiotics as a novel approach for the treatment of NAFLD.
Materials and methods
Strain
L. bulgaricus, L. casei, and L. helveticus are lactic acid bacteria that have been isolated from various including sour milk, cheese, and healthy Korean adult (30 years old) newborn baby feces, respectively. The fecal samples from which the strains were isolated were obtained by voluntary donation of the members of the coauthors’ institutes and the consent form was also obtained for the use of the samples. P. pentosaceus KID7 (KCTC 18308P) is a lactic acid bacterium that has been isolated from finger millet (Eleusine coracana) gruel. Lactobacillus spp. and P. pentosaceus KID7 were inoculated into a flask containing de Man, Rogosa and Sharpe medium (BD/Difco). The strains were incubated under anaerobic conditions at 37°C for 24 h. Stocks of each strain were prepared by mixing the culture broth with an equivalent 20% skim milk solution and then storing at −80°C until use.
Preparation
The seed culture was grown in a flask containing MRS broth for lactobacillus spp. At 37°C for 24 h. Each broth was inoculated in an optimized medium in a fermenter (Bio Control & Science, MARADO-05D-PS). The fermentation was carried out with a constant pH of 5.5 ~ 6.0 by automatically adding NaOH solution (25% w/v) under the conditions of 120 rpm agitation at 37°C for 18 ~ 20 h. At the end of the fermentation, the cells were harvested by centrifugation at 6000 rpm for 10 min (Hanil, Supra R12). The lyophilization of 40X concentrated cells was accomplished in accordance with the manual (Cooling & Heating System, Lab-Mast 10). After lyophilization, the colony-forming units (CFU) per gram of each probiotics powder was measured by a serial dilution method. Probiotics were suspended in 0.1 M PBS and adjusted to a density of 109 CFU/mL prior to use. The baseline characteristics of the probiotics strain are reported in .
Animals
Six-week-old specific-pathogen-free male C57BL/6J mice were purchased from Dooyeol Biotech (Seoul, Korea). All mice were individually housed in steel microisolator cages at 22°C ± 2°C with a 12/12-h light/dark cycle. Mice had free access to water and food throughout the experiment and were monitored daily. The experiment included an adaptation period for all groups, during which mice were fed normal diet for a week, and groups fed a Western diet had a 3-day intake adaptation period. The animals received humane care and all procedures were performed in accordance with National Institutes of Health Guidelines for the Care and Use of Laboratory Animals. All procedures were approved by the Institutional Animal Care and Use Committee of the College of Medicine, Hallym University (2018–04).
Treatment protocol
The male C57BL/6J mice had free access to water and food throughout the experiment and were monitored daily (n = 10 mice per each group). Mice were divided into 9 groups: normal (n = 10), Western diet (n = 10), and 7 for Western diet with probiotic groups (n = 10 per group). The Western diet was purchased from Dooyeol Biotech and the proportions of the Western diet (TD88137, Seoul, Korea) were 42% fat, 42.7% of carbohydrate, and 15% protein. Probiotics were suspended in distilled water at a concentration of 109 CFU/g for 8 weeks. The strains used were L. bulgaricus, L. casei, L. helveticus, P. pentosaceus KID7, and 3 combinations (1: L. casei + L. helveticus, 2: L. casei + L. helveticus + P. pentosaceus KID7, and 3: L. casei + L. helveticus + L. bulgaricus) ().
The animals were sacrificed via inhalation anesthesia overdose (isoflurane, Aerane; Baxter, Deerfield, IL, USA) at the end of the treatment period. They were weighed, and the blood, liver, stool, and small intestine were collected. Whole blood (800 μL) samples were centrifuged (19,000 × g for 5 min) to collect serum. The liver and stool were rapidly excised and stored at 80°C.
Serum analysis
Serum aspartate aminotransferase (AST), alanine aminotransferase (ALT), and total cholesterol were analyzed using a biochemical blood analyzer (KoneLab 20, Thermo Fisher Scientific, Waltham, Finland).
Pathology
Specimens were fixed with 10% formalin and routinely embedded in paraffin, and tissue sections were processed with hematoxylin and eosin, Masson’s trichrome, and reticulin fiber staining. Fatty liver was classified according to NASH clinical research network scoring system for NAFLD from grades 0 to 3 (0: <5%, 1: 5–33%, 2: 34–66%, and 3: >66% of steatosis). Inflammation was classified from grades 0 to 3 (0: none, 1: 1–2 foci per ×20 field, 2: 2–4 foci per ×20 field, and 3: >4 foci per ×20 field). All biopsy specimens were analyzed by a hepato-pathologist (S. H. H.).
The NAS, an objective index for classifying the grade of fatty liver, is suggested by Kleiner which is a sum of the scores of diabetes, bovine inflammation, and balloon dilatation.Citation43 According to the guidelines based on NAS, it can help to recognize A histological scoring the system addressing the full spectrum of NAFLD.Citation44 For statistical analyses, the patients were grouped into the three different NAS groups (group 1 = NAS 0–2: probable no NASH; group 2 = NAS 3–4: borderline; group 3 = NAS 5–8: probable NASH). NAS components: Grade = Total score: Steatosis + Hepatocyte Ballooning + Lobular Inflammation. Range, 0–8. The NAS of >5 correlated with a diagnosis of NASH. The chart is the criteria for the NAS score ().
In addition, CD 68 stain was performed by the method of immunohistochemistry also in the serial sections. Sections of formalin-fixed, paraffin wax-embedded tissue were stained in the Bond-Max automatic immunostaining device (Leica Biosystem, Newcastle, UK) using a bond polymer intensity detection kit (Leica Biosystem) for formalin-fixed, paraffin-embedded tissue sections. Using antibodies was a cluster of differentiation 68 (CD 68; Leica Biosystem, Newcastle, UK, RTU). These sections were counterstained with Harris hematoxylin. From CD68 immunohistochemical stain, the findings were checked each on an average field of ⅹ200 and ⅹ400 as the area of stained cells using an imaging tool. All of these analyses were performed by one pathologist (S. H. H.) who was blinded to the experimental conditions.
Analysis of endotoxin levels in fecal supernatant
The same fecal samples were used for endotoxin measurement and 16S rRNA gene sequencing. Mouse fecal samples were collected from 16-week-old controls and treated mice at 24 h after the last oral gavage. Fecal supernatant was obtained according to a previously described protocol with some modifications.Citation45 Briefly, the fecal samples were suspended in sterile PBS to a concentration of 1 g per 10 mL (human) or 50 mg per 500 μL (mouse) and vortexed mildly to avoid disruption of bacterial cells. After centrifugation for 15 min at 3000 rpm, the supernatant was collected, sterilized by filtration through a 0.45-μm filter followed by refiltration through a 0.22-μm filter, inactivated for 15 min at 90°C, and stored at ‒80°C.
The fecal endotoxin levels were determined using a limulus amebocyte lysate assay (No. 50-647U; Lonza Inc) according to the manufacturer’s instructions.Citation45 The plasma was diluted 10-fold, and the fecal supernatant was diluted 10,000-fold in pyrogen-free water and inactivated for 15 min at 90°C. Endotoxin measurements were performed in pyrogen-free glass tubes, eppendorf tubes, and plates.
Trans-epithelial electrical resistance assay
Caco-2 cells were seeded onto Transwell-Clear inserts (12-well clusters, 6.5-mm inserts with polyester membrane, pore diameter 0.4 μm, Corning NY) at a density of 105 cells/insert. Each insert was placed on top of a well in a 24-well plate with 1 ml in the bottom and 200 μl media in the top as described previously.Citation46 Caco-2 cells were grown for 5 days until confluence in Minimum Essential Medium Eagle with 20% fetal bovine serum without antibiotic-antimycotic (Gibco, Carlsbad, CA, USA) at 37°C in a humidified 5% atmosphere.
TEER measurements were performed using a Millicell Electrical resistance system (Millipore, Billerica, MA, USA). When monolayer of cells reached the confluence, Caco-2 cells were co-incubated with 200 μl of OD600 0.3 bacterial suspension (7 ⅹ 107 CFU/ml) in MEM media for 8 hrs and the TEER was measured respectively. TEER values were compared with those of the control and expressed as the ratio of the TEER value of sample after 8 hr co-incubation to the TEER value of control after 8 hr co-incubation.
Stool analysis for the metagenomics
Metagenomic DNA was extracted with a QIAamp stool kit (cat. no. 51504) and amplification of the V3 – V4 region of the bacterial 16S rRNA gene was conducted using barcoded universal primers (). Due to the lack of collected stool and time-consuming, we used only three samples for microbiome analysis. Each stool sample was collected from pooled stool pellets of 2–3 mice. PCR was performed with the following conditions: an initial denaturation at 95°C for 5 min, 20 cycles of 95°C for 30 s, 55°C for 30 s, and 72°C for 30 s, followed by a final extension at 72°C for 10 min. Purification of the amplicons was conducted with an Agencourt AMPure XP system (Beckman, USA) and quantification of the purified amplicons was conducted using PicoGreen and quantitative PCR. After pooling of the barcoded amplicons, sequencing was carried out using a MiSeq sequencer on the Illumina platform (ChunLab Inc., Republic of Korea) according to the manufacturer’s specification.
Table 4. Universal primers for V3 – V4 region of the bacterial 16S rRNA gene.
Microbiome profiling was conducted with the 16S-based Microbial Taxonomic Profiling platform of EzBioCloud Apps (ChunLab Inc., Republic of Korea). After taxonomic profiling of each sample, a comparative analyzer of EzBioCloud Apps was used for comparative analysis of the samples. Taxonomic assignment of the reads was conducted with ChunLab’s 16S rRNA database (DB ver. PKSSU4.0).Citation47 OTU picking was conducted with UCLUST and CDHIT with 97% of similarity cutoff.Citation48 Then, Good’s coverage, rarefaction, and alpha-diversity indices including ACE, Chao1, Jackknife, Shannon, Simpson, and NPShannon were calculated. Beta-diversity including PCoA and UPGMA clustering was shown in the comparative MTP analyzer. All 16S rRNA sequences were deposited in the ChunLab’s EzBioCloud Microbiome database and sequencing reads of the 16S rRNA gene from this study were deposited in the NCBI Short Read Archive under the bioproject number PRJNA532302.
Metabolite extraction and derivatization for GC-TOF MS analysis
Fecal samples were thawed at 4°C and mixed with 1.2 mL of cold extraction solvent (acetonitirile:methanol:water, 3:3:2, v/v/v). The mixtures were vortexed for 1 min, sonicated for 5 min under ice, and centrifuged at 13,200 rpm for 5 min at 4°C. The supernatants (500 µl) were aliquoted and transferred to new 1.5 mL tubes. The aliquots were concentrated to complete dryness in a speed vacuum concentrator (SCANVAC, Korea).Citation49 The dried cecal extracts were derivatized with 5 µl of 40mg/ml methoxyamine hydrochloride (Sigma-Aldrich, St. Louis, MO, USA) in pyridine (Thermo, USA) and then incubated for 90 min (800 rpm at 30°C). After the first derivatization step, 2 μL of fatty acid methyl esters (FAME) as a retention time index and 45 μL of N-methyl-N-trimethylsilyltrifluoroacetamide (MSTFA +1% TMCS; Thermo, USA) were added into the derivative and reacted for 60 min at 800rpm at 37°C. The FAME mixture included C8, C9, C10, C12, C14, C16, C18, C20, C22, C24, C26, C28, and C30.Citation50
GC-TOF MS analysis
GC-TOF MS analysis was conducted using an Agilent 7890B gas chromatograph (Agilent Technologies) and Leco Pegasus HT time of fight mass spectrometer (LECO, St. Joseph, MI, USA). Gas oven temperature was set to 50°C for 1 min, incremented at 20°C/min to 330°C, and hold for 5 min. Transfer line and ion source temperatures were set to 280°C and 250°C, respectively. Mass spectra were acquired ranging from 85 to 500 m/z at a scan rate of 17 spectra/sec.Citation51 Data pre-processing was done upon data acquisition using ChromaTOF software (version 4.5), which included apex mass values, entire spectrum, signal-to-noise ratio, peak purity, and retention time. Post-process was performed using the Binbase algorithm consisting of chromatogram validation, primary RI detection, and validation of unique mass. The quantitative value was computed using the peak height of the single unique mass. Missing values that did not pass the primary criteria were imputed by post-matching and replacement using raw data as previously described.Citation52 To check analytical precision, a mixture of 30 pure reference compounds were analyzed every 6 samples.
Liver tissue analysis for pro-inflammatory cytokines
Isolation of total RNA from tissue was performed using a TRIzol reagent kit (Invitrogen, Gaithersburg, MD, USA) according to the manufacturer’s instructions. Aliquots of total RNA (2 μg) were converted to cDNA using cDNA reverse transcription kit (Applied Biosystems, Foster City, CA). The cDNA was amplified for quantitative PCR using Luna® Universal Probe qPCR Master Mix (New England Biolabs Beverly, MA, USA) and each target-specific probe-primer (Applied Biosystems, Foster City, CA).
Tissue homogenates were incubated with PRO-PREP™ Protein Extraction Solution (iNtRON Biotechnology, Korea) at 4°C for 30 min, and then centrifuged at 10,000 g for 10 min. The resulting supernatants were harvested and analyzed for the levels of pro-inflammatory cytokines including tumor necrosis factor (TNF)-α, interleukin (IL)-1β, and IL-6, which were analyzed by enzyme-linked immunosorbent assay (R & D Systems, Minneapolis, MN), according to the manufacturer’s instructions.
Data analysis
Continuous variables were expressed as the means and standard deviations. One-way ANOVA, the Kruskal-Wallis test, and independent sample T-test were performed for the body weight, liver function test, and histology analysis. For further statistical analysis, data was normalized based on MSTUSCitation53 implemented in NOREVA (http://idrb.zju.edu.cn/noreva). Hierarchical clustering analysis (HCA) were and analysis of variance (ANOVA) with post hoc test were performed using Multiple Experiment Viewer (MeV).Citation52 A p value < .05 was considered to indicate statistical significance. All statistical analyses were performed using SPSS software (ver. 19, SPSS Inc., Chicago, IL, USA).
Disclosure of Potential Conflicts of Interest
No potential conflicts of interest were disclosed.
Author contributions
NYL, SJY, DHH, SHH, and KTS designed the study and interpreted the work. NYL, SHH, and KTS wrote the manuscript. HG, GSY, MJS, YLH, MJK, BYK, JSY, DYL, TSP, SHP, BKK, HCJ, and ISC performed experiments. JTH and DJK provided tissue specimens. All authors contributed to manuscript revision, and read and approved the submitted version.
Disclosure of potential conflicts of interest
Authors MJK and BYK were employed by company ChunLab, Inc. Authors BKJ, HCJ, and ISC were employed by company Chong Kun Dang Bio. All other authors attest that there are no commercial associations that might be a conflict of interest in relation to the submitted manuscript.
Ethical approval statement
The animals received humane care and all procedures were performed in accordance with National Institutes of Health Guidelines for the Care and Use of Laboratory Animals. All procedures were approved by the Institutional Animal Care and Use Committee of the College of Medicine, Hallym University (2018-04).
Correction Statement
This article has been republished with minor changes. These changes do not impact the academic content of the article.
Additional information
Funding
References
- Kneeman JM, Misdraji J, Corey KE. Secondary causes of nonalcoholic fatty liver disease. Therap Adv Gastroenterol. 2012;5:199–207. doi:10.1177/1756283X11430859.
- Paolella G, Mandato C, Pierri L, Poeta M, Di Stasi M, Vajro P. Gut-liver axis and probiotics: their role in non-alcoholic fatty liver disease. World J Gastroenterol. 2014;20:15518–15531. doi:10.3748/wjg.v20.i42.15518.
- Mavrogiannaki AN, Migdalis IN. Nonalcoholic fatty liver disease, diabetes mellitus and cardiovascular disease: newer data. Int J Endocrinol. 2013;2013:450639. doi:10.1155/2013/450639.
- Rinella ME. Nonalcoholic fatty liver disease: a systematic review. JAMA. 2015;313:2263–2273. doi:10.1001/jama.2015.5370.
- Caussy C, Soni M, Cui J, Bettencourt R, Schork N, Chen CH, Ikhwan MA, Bassirian S, Cepin S, Gonzalez MP, et al. Nonalcoholic fatty liver disease with cirrhosis increases familial risk for advanced fibrosis. J Clin Invest. 2017;127:2697–2704. doi:10.1172/JCI93465.
- Jiang W, Wu N, Wang X, Chi Y, Zhang Y, Qiu X, Hu Y, Li J, Liu Y. Dysbiosis gut microbiota associated with inflammation and impaired mucosal immune function in intestine of humans with non-alcoholic fatty liver disease. Sci Rep. 2015;5:8096. doi:10.1038/srep08096.
- Cui X, Ye L, Li J, Jin L, Wang W, Li S, Bao M, Wu S, Li L, Geng B, et al. Metagenomic and metabolomic analyses unveil dysbiosis of gut microbiota in chronic heart failure patients. Sci Rep. 2018;8:635. doi:10.1038/s41598-017-18756-2.
- Kundu P, Blacher E, Elinav E, Pettersson S. Our gut microbiome: the evolving inner self. Cell. 2017;171:1481–1493. doi:10.1016/j.cell.2017.11.024.
- Lindheim L, Bashir M, Munzker J, Trummer C, Zachhuber V, Leber B, Horvath A, Pieber TR, Gorkiewicz G, Stadlbauer V, et al. Alterations in gut microbiome composition and barrier function are associated with reproductive and metabolic defects in women with polycystic ovary syndrome (PCOS): a pilot study. PLoS One. 2017;12:e0168390. doi:10.1371/journal.pone.0168390.
- Suk KT, Kim DJ. Gut microbiota: novel therapeutic target for nonalcoholic fatty liver disease. Expert Rev Gastroenterol Hepatol. 2019;13:193–204. doi:10.1080/17474124.2019.1569513.
- Hong M, Han DH, Hong J, Kim DJ, Suk KT. Are probiotics effective in targeting alcoholic liver diseases? Probiotics Antimicrob Proteins. 2018. doi:10.1007/s12602-018-9419-6.
- Yuan J, Chen C, Cui J, Lu J, Yan C, Wei X, Zhao X, Li N, Li S, Xue G, et al. Fatty liver disease caused by high-alcohol-producing Klebsiella pneumoniae. Cell Metab. 2019;30:675–88 e7. doi:10.1016/j.cmet.2019.08.018.
- Chen J, Thomsen M, Vitetta L. Interaction of gut microbiota with dysregulation of bile acids in the pathogenesis of nonalcoholic fatty liver disease and potential therapeutic implications of probiotics. J Cell Biochem. 2019;120:2713–2720. doi:10.1002/jcb.27635.
- Ma YY, Li L, Yu CH, Shen Z, Chen LH, Li YM. Effects of probiotics on nonalcoholic fatty liver disease: a meta-analysis. World J Gastroenterol. 2013;19:6911–6918. doi:10.3748/wjg.v19.i40.6911.
- Cho MS, Kim SY, Suk KT, Kim BY. Modulation of gut microbiome in nonalcoholic fatty liver disease: pro-, pre-, syn-, and antibiotics. J Microbiol. 2018;56:855–867. doi:10.1007/s12275-018-8346-2.
- Ghouri YA, Richards DM, Rahimi EF, Krill JT, Jelinek KA, DuPont AW. Systematic review of randomized controlled trials of probiotics, prebiotics, and synbiotics in inflammatory bowel disease. Clin Exp Gastroenterol. 2014;7:473–487. doi:10.2147/CEG.S27530.
- Cats A, Kuipers EJ, Bosschaert MA, Pot RG, Vandenbroucke-Grauls CM, Kusters JG. Effect of frequent consumption of a Lactobacillus casei-containing milk drink in Helicobacter pylori-colonized subjects. Aliment Pharmacol Ther. 2003;17:429–435. doi:10.1046/j.1365-2036.2003.01452.x.
- Messaoudi M, Lalonde R, Violle N, Javelot H, Desor D, Nejdi A, Bisson J-F, Rougeot C, Pichelin M, Cazaubiel M, et al. Assessment of psychotropic-like properties of a probiotic formulation (Lactobacillus helveticus R0052 and Bifidobacterium longum R0175) in rats and human subjects. Br J Nutr. 2011;105:755–764. doi:10.1017/S0007114510004319.
- Pritchard GG, Coolbear T. The physiology and biochemistry of the proteolytic system in lactic acid bacteria. FEMS Microbiol Rev. 1993;12:179–206. doi:10.1111/j.1574-6976.1993.tb00018.x.
- Jauhiainen T, Ronnback M, Vapaatalo H, Wuolle K, Kautiainen H, Groop PH, Korpela R. Long-term intervention with Lactobacillus helveticus fermented milk reduces augmentation index in hypertensive subjects. Eur J Clin Nutr. 2010;64:424–431. doi:10.1038/ejcn.2010.3.
- Bahl MI, Bergstrom A, Licht TR. Freezing fecal samples prior to DNA extraction affects the Firmicutes to Bacteroidetes ratio determined by downstream quantitative PCR analysis. FEMS Microbiol Lett. 2012;329:193–197. doi:10.1111/j.1574-6968.2012.02523.x.
- Koliada A, Syzenko G, Moseiko V, Budovska L, Puchkov K, Perederiy V, Gavalko Y, Dorofeyev A, Romanenko M, Tkach S, et al. Association between body mass index and Firmicutes/Bacteroidetes ratio in an adult Ukrainian population. BMC Microbiol. 2017;17:120. doi:10.1186/s12866-017-1027-1.
- Wang Z, Klipfell E, Bennett BJ, Koeth R, Levison BS, Dugar B, Feldstein AE, Britt EB, Fu X, Chung Y-M, et al. Gut flora metabolism of phosphatidylcholine promotes cardiovascular disease. Nature. 2011;472:57–63. doi:10.1038/nature09922.
- Wu H, Esteve E, Tremaroli V, Khan MT, Caesar R, Manneras-Holm L, Ståhlman M, Olsson LM, Serino M, Planas-Fèlix M, et al. Metformin alters the gut microbiome of individuals with treatment-naive type 2 diabetes, contributing to the therapeutic effects of the drug. Nat Med. 2017;23:850–858. doi:10.1038/nm.4345.
- Fourie NH, Wang D, Abey SK, Sherwin LB, Joseph PV, Rahim-Williams B, Ferguson EG, Henderson WA. The microbiome of the oral mucosa in irritable bowel syndrome. Gut Microbes. 2016;7:286–301. doi:10.1080/19490976.2016.1162363.
- Sung H, Kim SW, Hong M, Suk KT. Microbiota-based treatments in alcoholic liver disease. World J Gastroenterol. 2016;22:6673–6682. doi:10.3748/wjg.v22.i29.6673.
- Kim DH, Jeong D, Kang IB, Kim H, Song KY, Seo KH. Dual function of Lactobacillus kefiri DH5 in preventing high-fat-diet-induced obesity: direct reduction of cholesterol and upregulation of PPAR-alpha in adipose tissue. Mol Nutr Food Res. 2017:61. doi:10.1002/mnfr.201700252.
- Ji Y, Park S, Park H, Hwang E, Shin H, Pot B, Holzapfel WH. Modulation of active gut microbiota by lactobacillus rhamnosus GG in a diet induced obesity murine model. Front Microbiol. 2018;9:710. doi:10.3389/fmicb.2018.00710.
- Humphries C. Microbiome: detecting diversity. Nature. 2017;550:S12–S4. doi:10.1038/550S12a.
- Foster KR, Schluter J, Coyte KZ, Rakoff-Nahoum S. The evolution of the host microbiome as an ecosystem on a leash. Nature. 2017;548:43–51. doi:10.1038/nature23292.
- Koutnikova H, Genser B, Monteiro-Sepulveda M, Faurie JM, Rizkalla S, Schrezenmeir J, Clément K. Impact of bacterial probiotics on obesity, diabetes and non-alcoholic fatty liver disease related variables: a systematic review and meta-analysis of randomised controlled trials. BMJ Open. 2019;9:e017995. doi:10.1136/bmjopen-2017-017995.
- Million M, Angelakis E, Paul M, Armougom F, Leibovici L, Raoult D. Comparative meta-analysis of the effect of Lactobacillus species on weight gain in humans and animals. Microb Pathog. 2012;53:100–108. doi:10.1016/j.micpath.2012.05.007.
- Damodharan K, Lee YS, Palaniyandi SA, Yang SH, Suh JW. Preliminary probiotic and technological characterization of Pediococcus pentosaceus strain KID7 and in vivo assessment of its cholesterol-lowering activity. Front Microbiol. 2015;6:768. doi:10.3389/fmicb.2015.00768.
- Chen Y, Li R, Chang Q, Dong Z, Yang H, Xu C. Lactobacillus bulgaricus or lactobacillus rhamnosus suppresses NF-kappaB signaling pathway and protects against AFB(1)-induced hepatitis: a novel potential preventive strategy for aflatoxicosis? Toxins (Basel). 2019;11:17. doi:10.3390/toxins11010017.
- Amin KA, Kamel HH, Eltawab MAA. Protective effect of Garcinia against renal oxidative stress and biomarkers induced by high fat and sucrose diet. Lipids Health Dis. 2011;10:6. doi:10.1186/1476-511X-10-6.
- Shavakhi A, Minakari M, Firouzian H, Assali R, Hekmatdoost A, Ferns G. Effect of a probiotic and metformin on liver aminotransferases in non-alcoholic steatohepatitis: a double blind randomized clinical trial. Int J Prev Med. 2013;4:531–537.
- De Santis A, Famularo G, De Simone C. Probiotics for the hemodynamic alterations of patients with liver cirrhosis. Am J Gastroenterol. 2000;95:323–324. doi:10.1111/j.1572-0241.2000.01726.x.
- Li Z, Yang S, Lin H, Huang J, Watkins PA, Moser AB. Probiotics and antibodies to TNF inhibit inflammatory activity and improve nonalcoholic fatty liver disease. Hepatology. 2003;37:343–350. doi:10.1053/jhep.2003.50048.
- Lirussi F, Mastropasqua E, Orando S, Orlando R. Probiotics for non-alcoholic fatty liver disease and/or steatohepatitis. Cochrane Database Syst Rev. 2007. doi:10.1002/14651858.CD005165.pub2.
- Nagata K, Suzuki H, Sakaguchi S. Common pathogenic mechanism in development progression of liver injury caused by non-alcoholic or alcoholic steatohepatitis. J Toxicol Sci. 2007;32:453–468. doi:10.2131/jts.32.453.
- Mykhal’chyshyn HP, Bodnar PM, Kobyliak NM. Effect of probiotics on proinflammatory cytokines level in patients with type 2 diabetes and nonalcoholic fatty liver disease. Lik's Sprava. 01 Mar 2013;2:56–62.
- Okubo H, Sakoda H, Kushiyama A, Fujishiro M, Nakatsu Y, Fukushima T, Matsunaga Y, Kamata H, Asahara T, Yoshida Y, et al. Lactobacillus casei strain Shirota protects against nonalcoholic steatohepatitis development in a rodent model. Am J Physiol Gastrointest Liver Physiol. 2013;305:G911–8. doi:10.1152/ajpgi.00225.2013.
- Kleiner DE, Brunt EM, Van Natta M, Behling C, Contos MJ, Cummings OW, Ferrell LD, Liu Y-C, Torbenson MS, Unalp-Arida A, et al. Design and validation of a histological scoring system for nonalcoholic fatty liver disease. Hepatology. 2005;41:1313–1321. doi:10.1002/hep.20701.
- Brunt EM, Janney CG, Di Bisceglie AM, Neuschwander-Tetri BA, Bacon BR. Nonalcoholic steatohepatitis: a proposal for grading and staging the histological lesions. Am J Gastroenterol. 1999;94:2467–2474. doi:10.1111/j.1572-0241.1999.01377.x.
- Yoshida N, Emoto T, Yamashita T, Watanabe H, Hayashi T, Tabata T, Hoshi N, Hatano N, Ozawa G, Sasaki N, et al. Bacteroides vulgatus and bacteroides dorei reduce gut microbial lipopolysaccharide production and inhibit atherosclerosis. Circulation. 2018;138:2486–2498. doi:10.1161/CIRCULATIONAHA.118.033714.
- Anderson RC, Cookson AL, McNabb WC, Park Z, McCann MJ, Kelly WJ, Roy NC. Lactobacillus plantarum MB452 enhances the function of the intestinal barrier by increasing the expression levels of genes involved in tight junction formation. BMC Microbiol. 2010;10:316. doi:10.1186/1471-2180-10-316.
- Yoon SH, Ha SM, Kwon S, Lim J, Kim Y, Seo H, Chun J. Introducing EzBioCloud: a taxonomically united database of 16S rRNA gene sequences and whole-genome assemblies. Int J Syst Evol Microbiol. 2017;67:1613–1617. doi:10.1099/ijsem.0.001755.
- Edgar RC. Search and clustering orders of magnitude faster than BLAST. Bioinformatics. 2010;26:2460–2461. doi:10.1093/bioinformatics/btq461.
- Park SJ, Kim JK, Kim -H-H, Yoon B-A, Ji DY, Lee C-W, Kim HJ, Kim KH, Shin HY, Park SJ, et al. Integrative metabolomics reveals unique metabolic traits in Guillain-Barré Syndrome and its variants. Sci Rep. 2019;9:1077. doi:10.1038/s41598-018-37572-w.
- Ji DY, Park SH, Park SJ, Kim KH, Ku CR, Shin DY, Yoon JS, Lee DY, Lee EJ. Comparative assessment of Graves’ disease and main extrathyroidal manifestation, Graves’ ophthalmopathy, by non-targeted metabolite profiling of blood and orbital tissue. Sci Rep. 2018;8:9262. doi:10.1038/s41598-018-27600-0.
- Polyzos AA, Lee DY, Datta R, Hauser M, Budworth H, Holt A, Mihalik S, Goldschmidt P, Frankel K, Trego K, et al. Metabolic reprogramming in astrocytes distinguishes region-specific neuronal susceptibility in Huntington Mice. Cell Metab. 2019;29:1258–1273.e11. doi:10.1016/j.cmet.2019.03.004.
- Lee EM, Park SJ, Lee J-E, Lee BM, Shin BK, Kang DJ, Choi H-K, Kim Y-S, Lee DY. Highly geographical specificity of metabolomic traits among Korean domestic soybeans (Glycine max). Food Res Int. 2019;120:12–18. doi:10.1016/j.foodres.2019.02.021.
- Li B, Tang J, Yang Q, Li S, Cui X, Li Y, Chen Y, Xue W, Li X, Zhu F, et al. NOREVA: normalization and evaluation of MS-based metabolomics data. Nucleic Acids Res. 2017;45:W162–W70. doi:10.1093/nar/gkx449.