ABSTRACT
Antimicrobials have become a mainstay of healthcare in the past century due to their activity against pathogens. More recently, it has become clear that they can also affect health via their impact on the microbiota and inflammation. This may explain some of their clinical benefits despite global increases in antimicrobial resistance (AMR) and reduced antimicrobial effectiveness. We showed in a randomized controlled trial of stopping versus continuing cotrimoxazole prophylaxis among HIV-positive Zimbabwean children taking antiretroviral therapy (ART), that continuation of cotrimoxazole persistently suppressed gut-resident viridans group streptococcal species (VGS) that were associated with intestinal inflammation. In this addendum, we provide a broader overview of how antibiotics can shape the microbiota and use high read-depth whole metagenome sequencing data from our published study to investigate whether (i) the impact of cotrimoxazole on gut VGS and (ii) VGS associated inflammation, is attributable to strain-level variability. We focus on S. salivarius, the VGS species that was most prevalent in the cohort and for which there was sufficient genome coverage to differentiate strains. We demonstrate that suppression of S. salivarius by cotrimoxazole is not strain specific, nor did stool concentration of the pro-inflammatory mediator myeloperoxidase vary by S. salivarius strain. We also show that gut-resident S. salivarius strains present in this study population are distinct from common oral strains. This is the first analysis of how cotrimoxazole prophylaxis used according to international treatment guidelines for children living with HIV influences the gut microbiome at the strain-level. We also provide a detailed review of the literature on the mechanisms by which suppression of VGS may act synergistically with cotrimoxazole’s anti-inflammatory effects to reduce gut inflammation. A greater understanding of the sub-clinical effects of antibiotics offers new insights into their responsible clinical use.
Introduction
More than 2000 years ago, Egyptian and Greek civilizations utilized a variety of substances in wound treatment, a handful of which are now known to have antimicrobial properties.Citation1,Citation2 The concept of an antibiotic, however, was not introduced until 1890, when antibiose (an antonym to symbiosis) was used to describe antagonistic interactions between microorganisms.Citation3 Microbes naturally produce antimicrobial agentsCitation4 to interfere with the growth of other microbes in inter and intraspecies competition. Mycophenolic acid was the first such naturally produced antibiotic to be discovered and isolated in 1893, although it was not synthesized until 1969 as Salvarsan.Citation3 The discovery of penicillin in 1928, its eventual synthesis in 1957 as penicillin V, and its subsequent success as a clinical therapy,Citation3 led to a surge in antibiotic discovery and synthesis in the 1950–1980s.Citation4
With the increase in diversity and accessibility of antibiotics, their use in people and animals (including misuse and overuse) has increased. The clearest consequence of increasing antibiotic use has been an alarming rise in antimicrobial resistance (AMR) in recent years, which has made the treatment of many common infections more challenging.Citation5 It has thus become equally important to understand the longer-term and sub-clinical effects of antibiotics aside from their role in killing or inhibiting pathogens.
Antibiotics also have the potential to shape the microbiome – the community of microorganisms (bacteria, archaea, fungi, viruses, and protists) that coexist in an intimate relationship with their host. Growing research interest in the composition and function of the microbiome has demonstrated its wide-reaching effects on physiologic development, function, and protection against infections. However, the concentrations at which antibiotics are administered elicit antimicrobial effects that extend beyond targeted pathogen elimination, to impact commensal microbiota members. This can have either detrimental or beneficial effects, depending on the underlying composition and function of an endogenous microbiome.
Antimicrobial effects of antibiotic use on the microbiome
Next-generation sequencing has demonstrated that antibiotics induce a reduction in gut bacterial biomass, bacterial taxonomic diversity, and functional diversity.Citation6 A single antibiotic dose can lead to the replacement of bacteria that are susceptible to its mechanisms of action (antibiotic-sensitive) with the subset of microbes that are not susceptible (antibiotic-tolerant).Citation7 In some individuals, the microbiota may not return to its initial composition, depending on the class of antibiotic used, the underlying health status of the patient and the use of other medications.Citation8,Citation9
A recent review reported the impact of antibiotics on the gut microbiota and revealed that bacteria belonging to a restricted set of clades (phyla or genera) are most strongly affected by antibiotic use. The breadth of bacterial clades affected was largest for fluoroquinolones (32 phyla), but was as few as a single phylum for several antibiotics.Citation6 The number of affected bacterial clades varied both between and within antibiotic classes.Citation6 Suppression of antibiotic-susceptible microbes can disrupt colonization resistance and open an ecological niche for opportunistic or pathogenic bacteria, increasing the host’s susceptibility to post-antibiotic infection.Citation6,Citation7 Antibiotic use can also produce an increase in antibiotic-tolerant commensal bacteria.Citation10,Citation11 The impact of antibiotics on the microbiota, therefore, depends on the antibiotic used and the bacterial clades that respond. Sub-species level adaptation of the microbiota to the use of different antibiotic classes is even less well characterized and the clinical relevance of such changes is only beginning to be understood. Such effects may be particularly pronounced and persistent in young children in whom colonization by commensal microbes is ongoing and stable communities have yet to be fully established.
Advances in sequencing and bioinformatics now provide strain-level resolution of bacteria from whole metagenome shotgun sequencing data.Citation12 Strains are sub-species level genetic variants of a microorganism which reflect adaptation to specific environmental selection pressures. It has become clear that sub-species variation in microbial genomes determines whether a commensal species is beneficialCitation13 or harmful.Citation14,Citation15 Strain-level metagenomic analysis allows for more granular investigation of the alterations in microbiota composition and function caused by antibiotic use.
Non-antimicrobial effects of antibiotic use in vulnerable populations
Despite the threat of AMR and the impact on the microbiota, antibiotics continue to save lives in vulnerable populations.Citation16,Citation17 This is particularly relevant to low- and middle-income countries (LMIC) where exposure to pathogens,Citation18 the prevalence of pathogen carriage,Citation19 and the occurrence of severe bacterial infections are all high.Citation20 Specifically, for immunocompromised individuals (e.g. people living with HIV or malnutrition) with limited access to rapid bedside diagnostic testing, the widespread use of prophylactic antibiotics, has become essential to treat and prevent infections. A natural question that follows is how antibiotic treatment continues to reduce morbidity and mortality in the face of high rates of AMR, and selection for resistant bacteria over time.Citation21,Citation22
Over six decades ago, researchers first reported that sulfonamide antibiotics had beneficial effects that were not explained by their antibiotic properties.Citation23 Other antibiotics are now known to exhibit non-antibacterial effects. Macrolides, for example, modulate pro-inflammatory cytokine secretion, promote phagocytosis of apoptotic cells, reduce neutrophil chemotaxis, enhance granule exocytosis by neutrophils, augment bactericidal activity by modulating oxidative bursts, and accelerate neutrophil apoptosis.Citation24 The tetracyline, minocycline can exert anti-inflammatory and anti-apoptotic effects, inhibit proteolysis, and suppress angiogenesis and tumor metastasis.Citation25 Cotrimoxazole has also exhibited immunosuppressive properties derived from the same antifolate activity that confers its antimicrobial effects.Citation26
The gut microbiome and antibiotic synergy
Tension between the benefits and potential harms of widespread antimicrobial use is well illustrated by cotrimoxazole – a broad-spectrum antibiotic that is now recommended long term for people living with HIV in areas where malaria or severe bacterial infections are highly prevalent.Citation27 This recommendation arises from multiple studies that have demonstrated reductions in morbidity and mortality among HIV-positive adults and children taking cotrimoxazole prophylaxis, regardless of HIV disease stage, degree of immunosuppression or use of antiretroviral therapy (ART).Citation27 However, there have been understandable concerns about the benefits to morbidity and mortality versus the risks of AMR posed by increasing the coverage and duration of cotrimoxazole use in LMIC. Interestingly, cotrimoxazole retains its clinical benefits despite a high preexisting prevalence of AMR among common pathogens,Citation28 for reasons that are unclear. We recently reported findings from a subgroup of HIV-positive children (median [IQR] age, 8.9 [5.7,11.1] years) in Zimbabwe who were on ART and randomized within the Anti-Retroviral Research for Watoto trial (ARROW; Trial registration: ISRCTN24791884) to continue or stop taking prophylactic cotrimoxazole after an average of two years of daily use.Citation29 We found that continuing daily cotrimoxazole reduced levels of biomarkers associated with systemic (plasma C-reactive protein, CRP, and interleukin-6, IL-6) and intestinal (stool myeloperoxidase) inflammation compared to stopping cotrimoxazole. This finding was not simply due to typical antimicrobial effects on pathogenic organisms, since the relative abundance of gastrointestinal Enterobacteriaceae detected in stool and the reported frequency of infection symptoms were unaffected.Citation29 Instead, we found that cotrimoxazole reduced inflammation through three pathways: (1) suppressing viridans group streptococci (VGS), whose relative abundance was associated with increased intestinal neutrophil activity; (2) directly reducing activation of innate immune cells, which produce pro-inflammatory cytokines; and (3) suppressing production of the neutrophil chemoattractant IL-8 by gut epithelial cells ().Citation29 Importantly, the continued use of daily cotrimoxazole at prophylactic doses for an additional two years of follow-up did not affect measures of microbiota alpha or beta diversity,Citation29 suggesting that the persistent alterations in gut microbiome composition and function caused by long-term use in this target population were narrow. We proposed that synergy between the antibiotic effects on gastrointestinal VGS and direct inhibition of pro-inflammatory mediator production may contribute to the sustained morbidity and mortality benefits of cotrimoxazole among people living with HIV.Citation17 These observations were made despite high levels of resistance to cotrimoxazole in the ARROW trial target population.Citation21,Citation22
Figure 1. Cotrimoxazole has multiple anti-inflammatory effects in people living with HIV. Graphical summary of the anti-inflammatory effects of cotrimoxazole identified using samples from the ARROW trial and complimentary in vitro models.Citation29 Effects of cotrimoxazole include (a) reduced circulating inflammatory mediators (CRP and IL-6), (b) suppression of viridans group streptococci (VGS), a type of bacteria that lives in the gut and promotes intestinal inflammation, which can be measured by stool levels of myeloperoxidase (MPO; a neutrophil-derived reactive oxygen species involved in antimicrobial immune responses). Stool MPO is an indicator of neutrophil accumulation and activity in the gut mucosa, (c) inhibition of pro-inflammatory mediator production (TNFα and IL-6) by immune cells activated by being experimentally exposed to bacteria, and 4) suppression of neutrophil chemoattractant protein production (IL-8) by gut epithelial cells. IL-8 is one of the signals that attracts neutrophils to the gut mucosa and perpetuates intestinal inflammation. IL-8 production is also stimulated by mevalonate pathway metabolites.
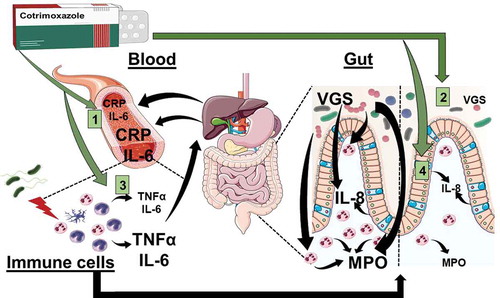
The role of bacterial species in intestinal immune regulation
We also identified a potential gut VGS-dependent mechanism for this beneficial antimicrobial-anti-inflammatory synergy (). Continued use of cotrimoxazole decreased the abundance of bacterial genes that encode mevalonate metabolism.Citation29 These genes predominantly mapped to VGS and were positively associated with fecal myeloperoxidase concentrations (a marker of neutrophil activation and accumulation in the gut).Citation29
The mevalonate pathway is one of two metabolic pathways utilized by bacteria to produce isoprenoids.Citation30 Isoprenoids are precursors to eukaryote cholesterol and prokaryote cell wall peptidoglycan production.Citation30 Mevalonate pathway activity has been shown to promote leukocyte recruitment and pro-inflammatory cytokine responses in vitro. Statins inhibit 3-hydroxy-3-methylglutaryl coenzyme A reductase (HMG-CoA), the rate-limiting enzyme in mevalonate production. Treating neutrophils with pravastatinCitation31 or cerivastatinCitation32 to block HMG-CoA reductase activity significantly decreased neutrophil chemotaxis and endothelial transmigration in vitro. The addition of exogenous mevalonate restored neutrophil chemotaxis.
The effect of mevalonate on chemotaxis and neutrophil migration may involve inhibition of protein prenylation by isoprenoids. Protein prenylation by isoprenoids is a key step in cell membrane receptor binding, activation of cell membrane trafficking, cytoskeletal organization, growth, apoptosis, and differentiation. Isoprenoids are responsible for the prenylation of several small GTPase molecules such as Rho.Citation33 In neutrophils, Rho activity is required for neutrophil migration.Citation34 Pravastatin, simvastatin, and atorvastatin were effective at reducing neutrophil transendothelial migration through a reduction in Rho activity.Citation35 Neutrophil migration was restored by exogenous isoprenoids.Citation35 Inhibition of isoprenoid synthesis also reduced neutrophil priming by IL-8 through reduction of protein prenylation;Citation36 while inhibition of mevalonate pathway isoprenoid synthesis reduced IL-6 and IL-8 production by monocytes.Citation37 VGS species also stimulate IL-8 secretion by monocyte-derived dendritic cells.Citation38 Our original publication demonstrated that the production of IL-8 by gut epithelial cells activated with the pro-inflammatory cytokine IL-1β in vitro was also reduced by pre-treatment with cotrimoxazole.Citation29 Streptococcus sp. are susceptible to cotrimoxazole,Citation28 and the mevalonate pathway they encode is the less common of the two pathways for isoprenoid synthesis found in bacterial genomes.Citation30 Together, our results support the notion that suppression of VGS, specifically, by cotrimoxazole in the gut of HIV-positive children, has a synergistic effect on suppressing molecular triggers for neutrophil chemotaxis to the gut.
Neutrophils kill pathogens via oxidative bursts involving myeloperoxidase.Citation39 Fungal and bacterial pathogens, including group A and B streptococci, are effectively killed by myeloperoxidase in vitro.Citation40 The bactericidal effect of myeloperoxidase on VGS, by contrast, is very limited at low myeloperoxidase concentrations.Citation40 Hydrogen peroxide (H2O2) is a key substrate in the bactericidal activity of myeloperoxidase.Citation40 Streptococcus sp. produce H2O2 as a metabolic by-product, and the bactericidal activity of myeloperoxidase is enhanced in the presence of VGS-produced H2O2 in vitro.Citation40 Thus, VGS appear to benefit from a competitive advantage over other pathogens and phenotypically related group A and B streptococci that are more effectively killed by myeloperoxidase in their presence. However, myeloperoxidase activity may also contribute to host tissue damage.Citation41 The gut is a key site of inflammation in HIV-positive people (HIV enteropathy).Citation42 Suppression of VGS may thus limit the detrimental effects of inflammation on the host gut, by reducing myeloperoxidase activity. This could have additive benefits during HIV infection.
Intestinal VGS strain diversity in the ARROW cohort
VGS are a heterogenous group of 30 streptococci speciesCitation43 that have been classified as part of the core oral microbiome, but have also frequently been isolated from the small intestine.Citation44 We previously reported that compared to the children randomized to stop cotrimoxazole treatment in the ARROW trial, children who continued cotrimoxazole had a lower relative abundance of the VGS species S. salivarius, S. parasanguinis, S. mutans, and S. vestibularis, all of which were positively associated with fecal myeloperoxidase concentration post-randomization. The most commonly identified species was S. salivarius.
A recent publication proposed that oropharyngeal bacteria, such as VGS, may be unrecognized drivers of intestinal inflammation, with important implications for treatment.Citation45 However, distinguishing between VGS originating from the oral cavity versus other body sites requires strain-level rather than species-level analysis. If the long-term clinical benefits of continuing cotrimoxazole that we previously reported are partly driven by suppression of VGS, it is important to determine whether cotrimoxazole’s antimicrobial effects and VGS driven gut inflammation are associated with sub-species characteristics of VGS, since strain-level variation can determine pathogenic properties.Citation13-Citation15
We initially analyzed microbial taxon abundance profiles generated using MetaPhlAn2 to demonstrate suppression of gastrointestinal VGS species among HIV-positive children randomized to continue taking cotrimoxazole relative to those who stopped. We further applied Pangenome-based Phylogenomic Analysis (PanPhlAn)Citation12 to confirm the species identity of the streptococci that were suppressed.Citation29 With PanPhlAn, the pangenome for a species is first obtained by compiling available, sequenced strain genomes into a single set of all genes that the species is known or predicted to carry. In addition to providing greater specificity for species identification, sub-species variants can be identified by PanPhlAn when bacterial genes with a similar depth of coverage are identified that map to a distinctive subset of the genes in that particular species’ pangenome. Identified gene sets can then be compared to determine differences in the functional capacity of unique strains across specimens.
In our original publication, 140 stool samples were collected at 84 (n = 36 continue and n = 36 stop) and 96 weeks (n = 33 continue and n = 35 stop) after randomization to stop or continue cotrimoxazole. Of these, PanPhlAn identified stool samples from six children who continued and 14 children who stopped cotrimoxazole that were positive for VGS (S. salivarius, S. parasanguinis, S. vestibularis, and/or S. mutans) at both follow-up time points,Citation28 confirming VGS as the Streptococcal species suppressed by the continuation of cotrimoxazole. Here, we build on these observations by further exploring strain-level patterns within the VGS identified as being suppressed by long-term cotrimoxazole prophylaxis. S. salivarius was the most prevalent VGS species identified and was the only VGS species with sufficient genome coverage to undertake strain-level analyses.Citation29 We used PanPhlAn to further characterize S. salivarius sub-species variants in order to determine whether: 1) S. salivarius strains in HIV-positive children on ART who continued cotrimoxazole use are different from those who stopped; 2) stool myeloperoxidase concentration differs by S. salivarius strain in ARROW samples; 3) S. salivarius strains in ARROW children are functionally distinct from S. salivarius reference strain genomes, most of which are derived from the oral cavity.
We used non-metric multi-dimensional scaling (NMDS) of the Jaccard distance between strain gene presence/absence profiles as an ordination technique. NMDS illustrates that the genetic composition of S. salivarius strains identified in ARROW samples did not differ between continue and stop groups (p = .310 by permutation test) (). In light of our original findings that VGS abundance was reduced in children who continued cotrimoxazole use, the strain-level similarity of S. salivarius between treatment groups indicates that sub-species variation in S. salivarius between children does not explain the ability of cotrimoxazole to suppress this VGS species, nor did continue cotrimoxazole favor expansion of specific strains.
Figure 2. Comparison of gene profiles from S. salivarius strains in stool samples from ART-treated HIV-positive children randomized to continue versus stop cotrimoxazole prophylaxis. (a) Nonmetric multidimensional scaling ordination plot of S. salivarius gene presence/absence profiles in ARROW strains identified in ART-treated HIV-positive Zimbabwean children randomized to stop cotrimoxazole (orange) or continue cotrimoxazole (green), and the PanPhlAn reference strain genomes (black). The blue circle represents the minimal area occupied by ARROW strains. (b) Nonmetric multidimensional scaling ordination plot of S. salivarius gene presence/absence in ARROW strains colored from lowest stool myeloperoxidase concertation (light blue) to highest stool myeloperoxidase concentration (dark blue). (c) Boxplots showing the distance of ARROW S. salivarius strains (blue) and PanPhlAn reference strains (white) from their respective group centroids.
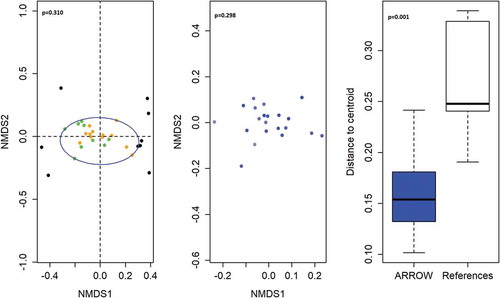
To investigate whether gut neutrophil activity depends on S. salivarius strains, we compared gene presence/absence profiles of S. salivarius strains by stool myeloperoxidase concentration with permutation testing. Strain genetic composition was not associated with stool myeloperoxidase concentration (p = .298 by permutation test) (). These analyses provide evidence that the increase in stool myeloperoxidase with increasing S. salivarius abundance observed in our original studyCitation28 was not driven by sub-species genetic variants. Thus, our original findings demonstrated that continued cotrimoxazole use suppressed S. salivarius and that S. salivarius abundance was associated with greater gut neutrophil activity. Here we further demonstrate that suppression of S. salivarius by continued cotrimoxazole confers these anti-inflammatory benefits regardless of strain-level genetic variation between individual patients. It is unclear whether this broad suppression of S. salivarius by long-term cotrimoxazole use and the associated reduction in intestinal inflammation is specific to children living with HIV in Zimbabwe or a more universal feature of cotrimoxazole treatment.
Next, we investigated whether the S. salivarius strains identified in stool samples from ARROW were distinct from the S. salivarius reference strains utilized by PanPhlAn, which were predominantly isolated from the oral cavities of subjects from high-income countries. We found that the genetic composition of ARROW S. salivarius strains was more similar to each other than to the genetic composition of S. salivarius reference strain genomes used by PanPhlAn (p = .001 by permutation test) (). In high-income settings, VGS are considered to predominantly reside in the oral cavity and to form part of the core oral microbiome; our data do not support that this is the case for S. salivarius strains identified in the stool of ART-treated children living with HIV in Zimbabwe.
Finally, to identify gene families that uniquely identify ARROW strains, we used Fisher’s Exact test to explore whether individual gene families were more likely to be present or absent in ARROW S. salivarius strains, pooled across treatment groups, compared to PanPhlAn S. salivarius reference strains. If the probability of observing a greater (or lower) proportion of strains with a specific gene in the ARROW group, compared to the reference group, was <0.05 after false discovery rate correction, the gene family was reported as enriched (or depleted) in the ARROW group. Forty-eight gene families were more likely to be present in ARROW S. salivarius strains and two were less likely to be present compared to the PanPhlAn reference strain genomes. Gene families disproportionately present in the ARROW S. salivarius strain genomes predominantly encoded cell membrane components, membrane biosynthesis, transmembrane transporters, transmembrane channels, membrane-bound surface-exposed environmental sensors, and enzymes involved in gene translation (). ARROW strains are therefore more likely than the reference strains to encode mechanisms to sense and respond to changing conditions in their environment,Citation46 to survive in harsh environmental conditions,Citation47 for nutrient acquisitionCitation48 and bacterial communication.Citation49
Table 1. Gene families that were more likely (enriched) or less likely (depleted) to be present in ARROW sub-study S. salivarius strains based on PanPhlan strain profiling.
We did not have stool samples from HIV-uninfected, ART-naïve or cotrimoxazole-naïve children to determine whether S. salivarius strains differ by HIV or treatment status. However, it is possible that these are useful genetic attributes for bacterial strains residing in an intestinal milieu characterized by enteropathy, inflammation, and increased susceptibility to enteric infection. This finding may also reflect a lack of representative reference genomes for this species of VGS from children in LMICs.Citation50 We also did not have oral samples from the same children to more directly explore the similarities between oral and intestinal strains. However, we have shown that strain-level analysis is crucial to the determination of niche-specific microbe adaptations of potential clinical relevance. Since our stool samples were collected at 84- and 96-weeks post-randomization to stop versus continue cotrimoxazole, we do not know the impact of stopping or continuing cotrimoxazole on S. salivarius strain diversity at earlier time-points. We did not have sufficient coverage of other VGS genomes to determine whether our findings also apply to those species.
Future directions
This is the first analysis of how cotrimoxazole prophylaxis used according to international treatment guidelines for children living with HIV influences the gut microbiome at the strain-level. We characterized S. salivarius strain gene compositions in stool samples from the ARROW trial and found that, in HIV-positive Zimbabwean children on ART, S. salivarius strains were distinct from the predominantly oral reference strains, and may be adapted to the gut milieu induced by chronic HIV-infection. There was no evidence that suppression of this VGS species in the gut by the continuation of cotrimoxazole prophylaxis, or its association with stool levels of myeloperoxidase, is dependent on sub-species characteristics. This suggests that broad suppression of VGS by cotrimoxazole is sufficient to confer these synergistic antimicrobial-anti-inflammatory benefits.
Our current understanding of how the microbiota contributes to human health is focused on composition and function at the level of species or, more commonly, higher-order taxa. New analytical tools allow us to characterize the microbiome at the strain-level, which provides new insights into the impact of antibiotics. This may be of particular importance for chronic infections, like HIV, where long-term cotrimoxazole prophylaxis is recommended, but the basis for the sustained clinical benefit of long-term therapy is not well understood. Furthermore, AMR to cotrimoxazole is already high in LMIC and several studies demonstrate that the impact of treatment on the microbiome may be limited, as few differences in the alpha- or beta-diversity of microbial species or metabolic functions have been observed.Citation29,Citation51 As the proportion of HIV-infected children taking cotrimoxazole expands in-line with international guidelines,Citation28 investigating changes in microbiome strain membership and functions may become increasingly important for promoting the health of these children.Citation13,Citation14 Our work and that of others demonstrates that continued cotrimoxazole use reduces systemic inflammation associated with poor clinical outcomes for children and adults living with HIV in sub-Saharan Africa.Citation17,Citation52 Our prior data suggest that this is partly due to a highly specific signature of sustained suppression of gut-resident VGS.Citation28 These additional analyses suggest that this beneficial effect is independent of sub-species genetic variants. Current and future treatment of infectious diseases will benefit from a better understanding of immunopathology; the role of the microbiota in inflammation; the sub-clinical, non-antibiotic effects of different antimicrobials; and the effect of antimicrobials on strain-specific host–microbiota interactions. It is important to better understand the risks and benefits of widespread antibiotic use such as cotrimoxazole, to inform policy around their use, to balance population health and antimicrobial stewardship.
Disclosure of Potential Conflicts of Interest
The authors report no conflict of interest.
Additional information
Funding
References
- Forrest RD. Early history of wound treatment. J R Soc Med. 1982;75:198–205.
- Wainwright M. Moulds in ancient and more recent medicine. Top Catal. 1989;3:21–23.
- Nicolaou KC, Rigol S. A brief history of antibiotics and select advances in their synthesis. J Antibiot (Tokyo). 2018;71:153–184.
- Spížek J, Sigler K, Řezanka T, Demain A. Biogenesis of antibiotics—viewing its history and glimpses of the future. Folia Microbiol (Praha). 2016;61(4):347–358. doi:10.1007/s12223-016-0462-y.
- Cosgrove SE. The relationship between antimicrobial resistance and patient outcomes: mortality, length of hospital stay, and health care costs. Clin Infect Dis. 2006;42(Suppl 2):S82–9. doi:10.1086/499406.
- Ferrer M, Méndez-García C, Rojo D, Barbas C, Moya A. Antibiotic use and microbiome function. Biochem Pharmacol. 2017;134:114–126. doi:10.1016/j.bcp.2016.09.007.
- Bucci V, Bradde S, Biroli G, Xavier JB. Social interaction, noise and antibiotic-mediated switches in the intestinal microbiota. PLoS Comput Biol. 2012;8(4):e1002497. doi:10.1371/journal.pcbi.1002497.
- Gerber GK, Onderdonk AB, Bry L. Inferring dynamic signatures of microbes in complex host ecosystems. PLoS Comput Biol. 2012;8(8):e1002624. doi:10.1371/journal.pcbi.1002624.
- Shaw LP, Bassam H, Barnes CP, Walker AS, Klein N, Balloux F. Modelling microbiome recovery after antibiotics using a stability landscape framework. ISME J. 2019;13(7):1845–1856. doi:10.1038/s41396-019-0392-1.
- Newton DF, Macfarlane S, Macfarlane GT. Effects of antibiotics on bacterial species composition and metabolic activities in chemostats containing defined populations of human gut microorganisms. Antimicrob Agents Chemother. 2013;57(5):2016–2025. doi:10.1128/AAC.00079-13.
- Ponziani FR, Scaldaferri F, Petito V, Paroni Sterbini F, Pecere S, Lopetuso LR, Palladini A, Gerardi V, Masucci L, Pompili M, et al. The role of antibiotics in gut microbiota modulation: the eubiotic effects of rifaximin. Dig Dis. 2016;34(3):269–278. doi:10.1159/000443361.
- Scholz M, Ward DV, Pasolli E, Tolio T, Zolfo M, Asnicar F, Truong DT, Tett A, Morrow AL, Segata N, et al. Strain-level microbial epidemiology and population genomics from shotgun metagenomics. Nat Methods. 2016;13(5):435–438. doi:10.1038/nmeth.3802.
- Cani PD, Van Hul M. Novel opportunities for next-generation probiotics targeting metabolic syndrome. Curr Opin Biotechnol. 2015;32:21–27. doi:10.1016/j.copbio.2014.10.006.
- Wick EC, Sears CL. Bacteroides spp. and diarrhea. Curr Opin Infect Dis. 2010;23(5):470–474. doi:10.1097/QCO.0b013e32833da1eb.
- Sitkiewicz I. How to become a killer, or is it all accidental? Virulence strategies in oral streptococci. Mol Oral Microbiol. 2018;33(1):1–12. doi:10.1111/omi.2018.33.issue-1.
- Keenan JD, Arzika AM, Maliki R, Boubacar N, Adamou SE, Ali MM, Cook C, Lebas E, Lin Y, Ray KJ, et al. Longer-term assessment of azithromycin for reducing childhood mortality in Africa. N Engl J Med. 2019;380(23):2207–2214. doi:10.1056/NEJMoa1817213.
- Bwakura-Dangarembizi M, Kendall L, Bakeera-Kitaka S, Nahirya-Ntege P, Keishanyu R, Nathoo K, Spyer MJ, Kekitiinwa A, Lutaakome J, Mhute T, et al. A randomized trial of prolonged co-trimoxazole in HIV-infected children in Africa. N Engl J Med. 2014;370(1):41–53. doi:10.1056/NEJMoa1214901.
- Penakalapati G, Swarthout J, Delahoy MJ, McAliley L, Wodnik B, Levy K, Freeman MC. Exposure to animal feces and human health: a systematic review and proposed research priorities. Environ Sci Technol. 2017;51(20):11537–11552. doi:10.1021/acs.est.7b02811.
- Platts-Mills JA, Liu J, Rogawski ET, Kabir F, Lertsethtakarn P, Siguas M, Khan SS, Praharaj I, Murei A, Nshama R. Use of quantitative molecular diagnostic methods to assess the aetiology, burden, and clinical characteristics of diarrhoea in children in low-resource settings: a reanalysis of the MAL-ED cohort study. Lancet Global Health. 2018;6(12):e1309–18. doi:10.1016/S2214-109X(18)30349-8.
- Liu J, Platts-Mills JA, Juma J, Kabir F, Nkeze J, Okoi C, Operario DJ, Uddin J, Ahmed S, Alonso PL, et al. Use of quantitative molecular diagnostic methods to identify causes of diarrhoea in children: a reanalysis of the GEMS case-control study. Lancet. 2016;388(10051):1291–1301. doi:10.1016/S0140-6736(16)31529-X.
- Cotton MF, Wasserman E, Smit J, Whitelaw A, Zar HJ. High incidence of antimicrobial resistant organisms including extended spectrum beta-lactamase producing Enterobacteriaceae and methicillin-resistant Staphylococcus aureus in nasopharyngeal and blood isolates of HIV-infected children from Cape Town, South. BMC Infect Dis. 2008;8:40. doi:10.1186/1471-2334-8-40.
- Musiime V, Cook A, Bakeera-Kitaka S, Vhembo T, Lutakome J, Keishanyu R, Prendergast AJ, Lubwama S, Robertson V, Hughes P, et al. Bacteremia, causative agents and antimicrobial susceptibility among HIV-1-infected children on antiretroviral therapy in Uganda and Zimbabwe. Pediatr Infect Dis J. 2013;32(8):856–862. doi:10.1097/INF.0b013e31828c3991.
- Tunnicliff R. The action of prontosil-soluble and sulfanilamide on the phagocytic activity of leukocytes and on the dissociation of Streptococci. J Infect Dis. 2019;64(1):59–65. doi:10.1093/infdis/64.1.59.
- Altenburg J, De Graaff CS, Van Der Werf TS, Boersma WG. Immunomodulatory effects of macrolide antibiotics - Part 1: biological mechanisms. Respiration. 2010;81(1):67–74. doi:10.1159/000320319.
- Garrido-Mesa N, Zarzuelo A, Gálvez J. What is behind the non-antibiotic properties of minocycline? Pharmacol Res. 2013;67(1):18–30. doi:10.1016/j.phrs.2012.10.006.
- Ghilchik MW, Morris AS, Reeves DS. Immunosuppressive powers of the antibacterial agent trimethoprim. Nature. 1970;227:393–394. doi:10.1038/227393a0.
- WHO. Guidelines on post-exposure prophylaxis for HIV and the use of co-trimoxazole prophylaxis for HIV-related infections among adults, adolescents and children: recommendations for a public health approach - December 2014 supplement to the 2013 consolidated A. Geneva (Switzerland): WHO Press; 2014.
- Church JA, Fitzgerald F, Walker AS, Gibb DM, Prendergast AJ. The expanding role of co-trimoxazole in developing countries. Lancet Infect Dis. 2015;15:327–339. doi:10.1016/S1473-3099(14)71011-4.
- Bourke CD, Gough EK, Pimundu G, Shonhai A, Berejena C, Terry L, Baumard L, Choudhry N, Karmali Y, Bwakura-Dangarembizi M, et al. Cotrimoxazole reduces systemic inflammation in HIV infection by altering the gut microbiome and immune activation. Sci Transl Med. 2019;11(486):1–14. doi:10.1126/scitranslmed.aav0537.
- Heuston S, Begley M, Gahan CGM, Hill C. Isoprenoid biosynthesis in bacterial pathogens. Microbiology (UK). 2012;158:1389–1401.
- Dunzendorfer S, Rothbucher D, Schratzberger P, Reinisch N, Kähler CM, Wiedermann CJ. Mevalonate-dependent inhibition of transendothelial migration and chemotaxis of human peripheral blood neutrophils by pravastatin. Circ Res. 1997;81:963–969. doi:10.1161/01.RES.81.6.963.
- Kaneider NC, Reinisch CM, Dunzendorfer S, Meierhofer C, Djanani A, Wiedermann CJ. Induction of apoptosis and inhibition of migration of inflammatory and vascular wall cells by cerivastatin. Atherosclerosis. 2001;158(1):23–33. doi:10.1016/S0021-9150(00)00764-4.
- Zhang FL. Protein prenylation: molecular mechanisms and functional consequences. Annu Rev Biochem. 1996;65(1):241–269. doi:10.1146/annurev.bi.65.070196.001325.
- Niggli V. Rho-kinase in human neutrophils: A role in signalling for myosin light chain phosphorylation and cell migration. FEBS Lett. 1999;445(1):69–72. doi:10.1016/S0014-5793(99)00098-8.
- Maher BM, Dhonnchu TN, Burke JP, Soo A, Wood AE, Watson RWG. Statins alter neutrophil migration by modulating cellular Rho activity–a potential mechanism for statins-mediated pleotropic effects? J Leukoc Biol. 2008;85(1):186–193. doi:10.1189/jlb.0608382.
- Elmore MA, daniels RH, Hill ME, Finnen MJ. Inhibition of neutrophil priming by inhibitors of farnesyl transferase. Biochem Soc Trans. 1997;25:253S. doi:10.1042/bst025253s.
- Terkeltaub R, Solan J, Barry M, Santoro D, Bokoch GM. Role of the mevalonate pathway of isoprenoid synthesis in IL-8 generation by activated monocytic cells. J Leukoc Biol. 1994;55(6):749–755. doi:10.1002/jlb.55.6.749.
- Van Den Bogert B, Meijerink M, Zoetendal EG, Wells JM, Kleerebezem M. Immunomodulatory properties of streptococcus and veillonella isolates from the human small intestine microbiota. PLoS One. 2014;9(12):1–20. doi:10.1371/journal.pone.0114277.
- Bokoch GM, Prossnitz V. Isoprenoid metabolism is required for stimulation of the respiratory burst oxidase of HL-60 cells. J Clin Invest. 1992;89(2):402–408. doi:10.1172/JCI115599.
- Allen RC, Stephens JT. Myeloperoxidase selectively binds and selectively kills microbes. Infect Immun. 2011;79(1):474–485. doi:10.1128/IAI.00910-09.
- Davies M. Myeloperoxidase?derived oxidation: mechanisms review the society for free radical research Japan journal of clinical biochemistry and nutrition of biological damage and its prevention. J Clin Biochem Nutr. 2011 10.3164/jcb. 1880 50860912-0009 R oto, Japan Kyjcbn11-006FR11-006FR JJCBN;48(1):8–19. doi:10.3164/jcbn.11-006FR.
- Brenchley JM, Douek DC. HIV infection and the gastrointestinal immune system. Mucosal Immunol. 2008;1(1):23–30. doi:10.1038/mi.2007.1.
- Doern CD, Burnham CAD. It’s not easy being green: the viridans group streptococci, with a focus on pediatric clinical manifestations. J Clin Microbiol. 2010;48(11):3829–3835. doi:10.1128/JCM.01563-10.
- Van Den Bogert B, Boekhorst J, Herrmann R, Smid EJ, Zoetendal EG, Kleerebezem M. Comparative genomics analysis of Streptococcus isolates from the human small intestine reveals their adaptation to a highly dynamic ecosystem. PLoS One. 2013;8(12):e83418. doi:10.1371/journal.pone.0083418.
- Vonaesch P, Morien E, Andrianonimiadana L, Sanke H, Mbecko JR, Huus KE, Naharimanananirina T, Gondje BP, Nigatoloum SN, Vondo SS, et al. Stunted childhood growth is associated with decompartmentalization of the gastrointestinal tract and overgrowth of oropharyngeal taxa. Proc Natl Acad Sci U S A. 2018;115(36):E8489–98. doi:10.1073/pnas.1806573115.
- Tefsen B, Ram AFJ, Van Die I, Routier FH. Galactofuranose in eukaryotes: aspects of biosynthesis and functional impact. Glycobiology. 2012;22(4):456–469. doi:10.1093/glycob/cwr144.
- Biswas I, Drake L, Erkina D, Biswas S. Involvement of sensor kinases in the stress tolerance response of Streptococcus mutans. J Bacteriol. 2008;190(1):68–77. doi:10.1128/JB.00990-07.
- Feltcher ME, Braunstein M. Emerging themes in SecA2-mediated protein export. Nat Rev Microbiol. 2012;10(11):779–789. doi:10.1038/nrmicro2874.
- Prindle A, Liu J, Asally M, Ly S, Garcia-Ojalvo J, Süel GM. Ion channels enable electrical communication in bacterial communities. Nature. 2015;527(7576):59–63. doi:10.1038/nature15709.
- Pasolli E, Asnicar F, Manara S, Zolfo M, Karcher N, Armanini F, Beghini F, Manghi P, Tett A, Ghensi P, et al. Extensive unexplored human microbiome diversity revealed by over 150,000 genomes from metagenomes spanning age, geography, and lifestyle. Cell. 2019;176(3):649–662. doi:10.1016/j.cell.2019.01.001.
- Oldenburger IB, Wolters VM, Kardol-Hoefnagel T, Houwen RHJ, Otten HG. Serum intestinal fatty acid–binding protein in the noninvasive diagnosis of celiac disease. Apmis. 2018;126(3):186–190. doi:10.1111/apm.2018.126.issue-3.
- Kyosiimire-Lugemwa J, Anywaine Z, Abaasa A, Levin J, Gombe B, Musinguzi K, Kaleebu P, Grosskurth H, Munderi P, Pala P, et al. Effect of Stopping Cotrimoxazole preventive therapy on microbial translocation and inflammatory markers among human immunodeficiency virus-infected ugandan adults on antiretroviral therapy: the COSTOP trial immunology substudy. J Infect Dis. 2019. doi:10.1093/infdis/jiz494.