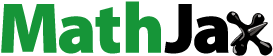
ABSTRACT
Background
Nonalcoholic fatty liver disease (NAFLD) is considered to be associated with diet and gut dysbiosis. Excessive sucralose can induce gut dysbiosis and negatively affect host health. Maternal diet shapes the microbial communities of neonate and this effect continues in later life. We aimed to investigate the effects of maternal sucralose (MS) intake on the susceptibility of offspring to hepatic steatosis in adulthood.
Methods
C57BL/6 pregnant mice were randomized into MS group (MS during gestation and lactation) and maternal control (MC) group (MC diet). After weaning, all offspring were fed a control diet until 8 weeks of age, and then treated with a high-fat diet (HFD) for 4 weeks. The intestinal development, mucosal barrier function, and gut microbiota were assessed in the 3-week-old offspring. Moreover, the severity of hepatic steatosis, serum biochemistry, lipid metabolism, and gut microbiota was then assessed in the 12th week.
Results
MS significantly inhibited intestinal development and disrupted barrier function in 3-week-old offspring. MS also induced intestinal low-grade inflammation, significantly changed the compositions and diversity of gut microbiota including reducing butyrate-producing bacteria and cecal butyrate production with down-regulation of GPR43. Mechanically, blocking GPR43 blunted the anti-inflammatory effect of one of the butyrate-producing bacteria, Clostridium butyricum in vitro. After HFD treatment, MS exacerbated hepatic steatosis, and disturbed fatty acid biosynthesis and metabolism, accompanied by inducing gut dysbiosis compared with MC group.
Conclusions
MS intake inhibits intestinal development, induces gut dysbiosis in offspring through down-regulation of GPR43, and exacerbates HFD-induced hepatic steatosis in adulthood.
Introduction
As one of the most prevalent chronic liver diseases throughout the world, nonalcoholic fatty liver disease (NAFLD) is considered to be connected with inappropriate nutrition. In recent years, increasing pieces of evidence prove that gut microbiota has appeared as a critical environmental aspect in the development of NAFLD.Citation1 Given that the perinatal period is a pivotal developmental stage for the neonatal microbiome,Citation2it is widely believed that the diet of pregnant and lactating mothers can shape the microbial communities of the neonate at birth and this effect continues in later life.Citation3Interestingly, Umesh D. Wankhade et al. reported that maternal high-fat diet (HFD) could induce gut dysbiosis of offspring and enhance their predisposition to hepatic steatohepatitis.Citation4
Sucralose, an organochlorine artificial sweetener, has been widely used around the world. It is increasingly added into commonly consumed foods such as soft drinks, cereals, and sugar-free desserts. However, accumulating evidence shows that sucralose can alter the composition of the gut microbiome, affect host health, and induce hepatic diseases.Citation5−Citation8Bian X et al. showed that sucralose consumption elevated pro-inflammatory gene expression in the liver by disrupting the gut microbiota in mice.Citation9Dhurandhar D et al. revealed that an obvious hepatic damage appeared on daily ingestion of sucralose in rats.Citation10Chih-Wei Liu et al. found that liver protein metabolism disorder appeared in sucralose-treated mice.Citation11 More importantly, maternal exposure to sucralose can downregulate progeny’s hepatic detoxification and alter progeny’s gut microbiome.Citation12Considering the potential influence of maternal sucralose (MS) administration on the liver and the negative effect in offspring on gut microbiota, we assume that MS can cause gut microbiota dysbiosis, thereby increasing hepatic steatosis susceptibility when the offspring reach adulthood.
In our present study, we found that MS inhibited intestinal development, induced imbalance of gut microbiota and low-grade inflammation, and further disrupted gut barrier function in offspring. The butyrate-producing bacteria and butyrate were dramatically decreased by MS. And finally, MS exacerbated HFD-induced hepatic steatosis in adulthood. From in vitro experiments we found that Clostridium butyricum decreased inflammatory cytokines production through activating GPR43. These data suggest that excessive sucralose should be taken with caution especially during pregnancy and lactation. Thus, it provides new insight into a better understanding of the pathogenesis of NAFLD in adulthood.
Results
Subject characteristics of offspring mice
In this study, pups' sex ratio and overall litter sizes were not significantly different between MS and MC groups. No death was found in the two groups. The body weight of two groups was similar at other measured time points before 8 weeks except that the offspring in MS group were heavier than those in MC group at 2–4 weeks of age. During the period with HFD, although no difference was found in the body weight at the very beginning, the offspring in MS group were distinctly heavier than those in MC group after 15 days with HFD. Thus, MS supplementation slightly increased weight gain in offspring at weaning and significantly aggravated weight gain to HFD ().
Figure 1. Maternal sucralose intake inhibited the intestinal development in 3-week-old pups. The experiment protocol was described in (a). Bodyweight of pups was recorded weekly up to 12 weeks (b). Small intestinal and colonic tissues of 3-week-old mice were detected by H&E staining (c). And villi length and crypt depth of at least 100 villi and crypts/mice were detected (d).MC, maternal control diet. MS, maternal sucralose intake. HFD, high-fat diet.MC: n = 19, MS: n = 17. Scale bar: 50 µm. *p < .05, **p < .01, ***p < .001.
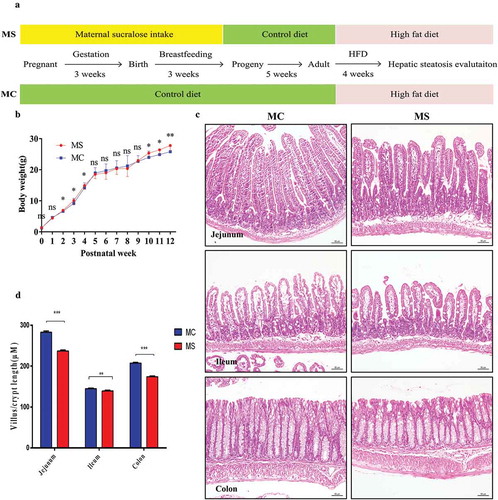
MS inhibited intestinal development and disrupted gut barrier in 3-week-old offspring
The development of the gastrointestinal tract is mainly influenced by maternal nutrition until the third week after birth in mice.Citation13,Citation14 So we investigated the effect of sucralose on the intestinal development and integrity of gut barrier in 3-week-old offspring. We measured the villi length and crypt depth of 3-week-old offspring. The length and depth of 3-week-old pups in MS group were significantly shorter than those in MC group (). Gut barrier, in physical, consists of mucous layer, intestinal epithelial cells, as well as the tight junctions located at the top of the intestinal membrane.Citation15 Epithelial cells renew by cell proliferation, differentiation, and migration. The number of Ki67 positive proliferating cells per crypt was counted, and decreased Ki67 positive cells were observed in MS group compared with MC group (). Similarly, the number of Periodic Acid Schiff (PAS) positive cells (indicating goblet cells) also decreased (). MUC2, one of the most important products of goblet cells, is closely related to the formation of mucous layer. According to the number of MUC2 positive cells and the mRNA expression, MUC2 production was downregulated in MS group compared with MC group ().
Figure 2. Maternal sucralose intake disturbed intestinal proliferation and differentiation and induced colonic low-grade inflammation of 3-week-old pups. Ki67 staining in the small intestine (a). Periodic acid Schiff staining for Goblet cells and MUC2 staining in the colon was shown. The number of positive staining cells in each crypt was also measured (b–c). Total RNA was extracted from the colonic tissues for Real-time-PCR analysis. The relative expression of MUC2 was presented (d). The relative expression of inflammatory cytokines including TNF-α, IL-1β, and IFN-γ was shown (e). MC, maternal control diet.MS, maternal sucralose intake. MC: n = 7, MS: n = 5. Scale bar: 50 µm. **p < .01, **p < .01, ***p < .001.
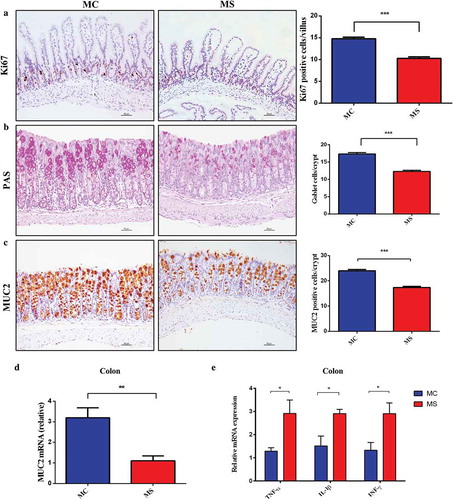
The tight junction proteins including ZO-1, Claudin-1, Claudin-3, and Occludin are considered as the most important factors in determining the integrity of gut barrier.Citation16According to polymerase chain reaction (PCR) data, the expression of Claudin-1, Claudin-3, and ZO-1 significantly decreased in MS group, while that of Occludin was similar in the two groups (). Besides, immunofluorescence also suggested that the expression of ZO-1 protein decreased in MS group (). Immunoglobulin A (IgA), functioning as inhibiting pathogens from adhering to the mucous, plays a critical role in the gut immunological barrier function.Citation15As immunofluorescence of IgA showed, MS dramatically decreased the level of IgA expression in the small intestine (). These data indicate that MS can inhibit intestinal development and disrupt the integrity of gut barrier in early life of offspring.
Figure 3. Maternal sucralose intake destroyed tight junction in the colon and decreased IgA in the small intestine of 3-week-old pups. The relative expression of ZO-1, Claudin-1, Claudin-3, and Occludin was shown (a). Detection of ZO-1membrane location by immunostaining (red, 200×), DAPI (blue) counterstains nuclei (b). IgA in the small intestine was presented by immunostaining analysis (green, 200×), and DAPI (blue). IgA positive cells were pointed by arrows (c). IgA positive cells in 100 villi were counted (d). MC, maternal control diet. MS, maternal sucralose intake.MC: n = 7, MS: n = 5. *p < .05, **p < .01.
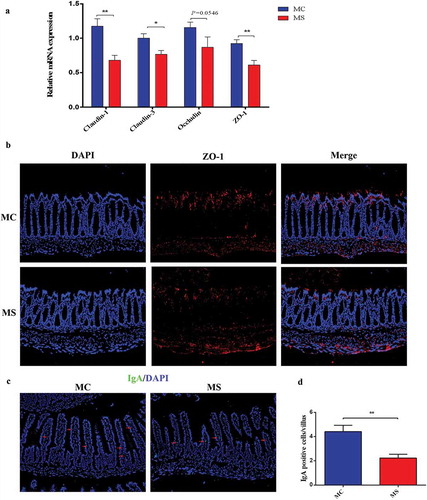
MS-induced intestinal low-grade inflammation in 3-week-old offspring
In this study, we have not found apparent microscopic inflammation in either small intestine or colon by H&E staining in two groups (). We further assessed the levels of the proinflammatory cytokine including IL-1β, IFN-γ, and TNF-α in colon tissues. Interestingly, the relative mRNA expression levels of these proinflammatory cytokines were significantly higher in MS group than those in MC group (). These results implied that MS supplementation could induce intestinal low-grade inflammation and impair gut barrier function in offspring.
MS altered the composition of gut microbiota in 3-week-old offspring mice
The gut microbiota of 3-week-old offspring was characterized and quantitatively analyzed via 16 S rRNA sequencing. We assessed operational taxonomic units(OTUs) of MS group and MC group to identify the shared and unique species. Venn diagram showed that there were 356 OTUs in MC group and 281OTUs in MS group, with 268 OTUs shared (). Then, we analyzed the microbiota community structure in two groups. There were four dominant bacteria at phylum level, which were Firmicutes, Bacteroidetes, Proteobacteria, and Verrucomicrobia. The relative abundance of Verrucomicrobia and Proteobacteria elevated in the MS group with the reduction of Bacteroidetes. At genus level, there was a significant difference between MS and MC groups, indicating that MS disrupts the balance of gut microbiota. The main genera included Akkermansia, Alistipes, Barnesiella, Blautia, Corynebacterium, Paraprevotella, Robinsoniella, Saccharibacteria_genera_incertaesedis, and Streptococcus. Compared with MC group, MS dramatically increased the abundance of Akkermansia, Blautia, Corynebacterium, and Robinsoniella, meanwhile, Alistipes, Barnesiella, Paraprevotella, Saccharibacteria_genera_incertae_sedis, and Streptococcus were depleted ().
Figure 4. Maternal sucralose intake altered composition of gut microbiota in 3-week-old pups. Stool samples of 3-week-old offspring were collected for 16 S rRNA gene sequencing. Venn diagram was shown (a). Relative abundance of bacteria at the genus level between two groups (b). Alpha diversity including Shannon and Simpson diversity index (c). PCoA with Adonis test indicating Beta diversity was shown (d). MC, maternal control diet. MS, maternal sucralose intake. PCoA, principal coordinate analysis.MC: n = 8, MS: n = 5. *p < .05, **p < .01.
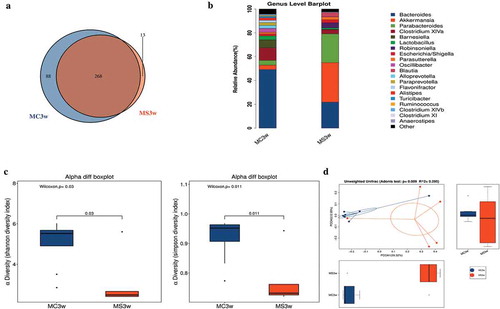
We used the Shannon and Simpson diversity index to evaluate microbial alpha diversity of 3-week-old pups. MS significantly decreased alpha diversity (). Next, we used principal coordinate analysis (PCoA) with Adonis test on unweighted UniFrac distances to calculate the differences among the samples of two groups. The results revealed that MS had a significant influence on the microbial composition in PCOA of 3-week-old pups ().
Finally, we studied the differentially abundant bacteria at the phylum, class, order, family, and genus level between the two groups by LDA Effect Size analysis (). And Heatmap in each mouse was also presented (). Specifically, the relative abundance of anti-inflammation associated microbiota AlistipesCitation17 were lower in MS group. Parabacteroides,Citation18 which can modulate host metabolism and alleviate obesity and metabolic dysfunctions were dramatically reduced in MS group. In contrast, BlautiaCitation19which are highly related to obesity and metabolic disorders significantly increased in MS group. Moreover, the relative abundance of Akkermansia of Verrucomicrobia, a mucin-degrading bacterium,Citation20 increased in MS group, possibly due to the disrupted mucosal barrier function and increased inflammation in the 3-week-old progeny. Consistent with the increased intestinal low-grade inflammation in MS group, Escherichia/Shigella and Anaerostipes which were shown to be more abundant in inflammatory bowel disease and metabolic disorder also increased in MS group.Citation21,Citation22 Streptococcus and Ruminococcus, which were reduced in MS group, were shown to be negatively associated with inflammation in previous studies.Citation23,Citation24 Intriguingly, butyrate-producing Saccharibacteria_genera_incertae_sedis, Prevotellaceae, and Clostridium XlVa dramatically decreased in the MS group of 3-week-old offspring. Overall, these results suggest that during pregnancy and lactation MS can perturb the gut microbiome in the offspring.
Figure 5. Maternal sucralose intake altered the key bacteria and SCFAs production in 3-week-old pups. Differentially abundant bacteria at the phylum, class, order, family, and genus level between two groups by LDA EffectSize analysis was shown (a) and Heatmap in each mouse (b). The levels of SCFAs including butyrate, acetate, and propionate in cecal contents were determined by HPLC analysis method (c). MC, maternal control diet. MS, maternal sucralose intake. SCFAs, short-chain fatty acids. MC: n = 8, MS: n = 5. *p < .05.
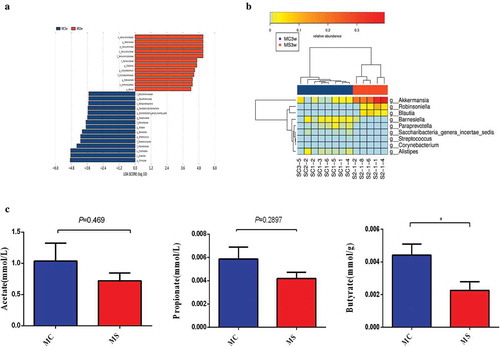
MS reduced short-chain fatty acids (SCFAs) in cecal contents of 3-week-old offspring mice
Bacterial fermentation of carbohydrates can produce intestinal SCFAs such as acetate, propionate, and butyrate. It has been reported that the gut barrier function was positively related to the concentrations of intestinal SCFAs.Citation25,Citation26 In the present study, we found a significantly lower level of butyrate production in MS group than that in MC group, and the trend of acetate and propionate production in MS group also went downward ().
These findings along with the microbiota change observed above indicate that decreased SCFAs-producing bacteria and drawdown of butyrate production may play a part in the inhibition of intestinal development and the destruction of the intestinal barrier driven by MS intake.
MS increased the expression of GPR43 in 3-week-old offspring mice
It has been reported that butyrate activates the G-protein-coupled receptors (GPCRs) including GPR41, GPR43, and GPR109A, which are expressed on intestinal epithelial cells and adipocytes. Activation of these GPCRs appears to alleviate the progression of NAFLD via multiple mechanisms.Citation27
We evaluated the expression of GPR41, GPR43, and GPR109A in the colon in 3-week-old offspring by immunohistochemistry. We found that GPR41, GPR43, and GPR109A were all expressed in colon tissues both in MS and MC groups. However, the expression of GPR43 in the colon in MS group significantly decreased than that in MC group, accompanied by the reduction of the butyrate-producing bacteria and butyrate (). The Real-time-PCR data also indicated that the relative expression of GPR43 decreased in the colon tissues of 3-week-old offspring than that in MC group ().
Clostridium butyricum decreased inflammatory cytokines production through GPR43 activation in vitro
Compared with the control, the levels of GPR43 mRNA were up-regulated 2.2-fold and 2.8-fold in HCT116 cells stimulated by sodium butyrate and Clostridium butyricum supernatant, respectively. The levels of GPR109A mRNA were upregulated by 2.9-fold and 2.1-fold. However, no significant change was observed in GPR41 mRNA expression. Meanwhile, Clostridium butyricum supernatant and sodium butyrate also caused 26.3% and 36.1% downregulation of the expression of TNF-α, respectively. Furthermore, we also studied whether anti-inflammatory function of Clostridium butyricum relayed on the GPR43 through siRNA-mediated GPR43 gene silencing. We found that the silencing of GPR43 blocked the Clostridium butyricum-mediated downregulation of TNF-α expression. The similar results were also presented in HCT8 cells (). These results indicated that the Clostridium butyricum has an anti-inflammatory effect through GPR43 activation.
MS exacerbated HFD-induced hepatic steatosis in adult mice
Accumulating studies have demonstrated that gut microbial imbalance and chronic inflammation in early life are potentially associated with adverse outcomes in adulthood.Citation3,Citation28,Citation29Here we further investigated whether MS intake in early life could increase the risk of hepatic steatosis in adulthood.
In general, the liver color of MS group appeared light yellow while that of MC group was dark red (). The weight of the liver and abdominal adipose tissues in MS increased more remarkably than that of the MC group (). Alanine aminotransferase and aspartate aminotransferase increased dramatically in MS group compared with MC group. Furthermore, MS group had significantly higher serum levels of total cholesterol (TC) and triglyceride (TG) compared with MC group ().
Figure 6. Maternal sucralose intake exacerbated high-fat diet-induced hepatic steatosis in 12-week-old offspring mice. The liver weight and abdominal adipose mass were measured at 12 weeks (a-b). Serum biochemical index including ALT, AST, TC, and TG was detected (c). Hepatic histology by H&E staining was shown, and liver inflammatory scoring was evaluated (d).Relative expression of IL-6 and TNF-$${\rm{\alpha }}$$
in the liver (e). Relative expression of lipid metabolism genes including Cyp7a1, shp, Fasn, and Acaca in liver was detected (f). MC, maternal control diet. MS, maternal sucralose intake. ALT, alanine aminotransferase. AST, aspartate aminotransferase. TC, total cholesterol. TG, triglyceride.MC: n = 10, MS: n = 14. Scale bar: 50 µm. *p < .05, **p < .01.
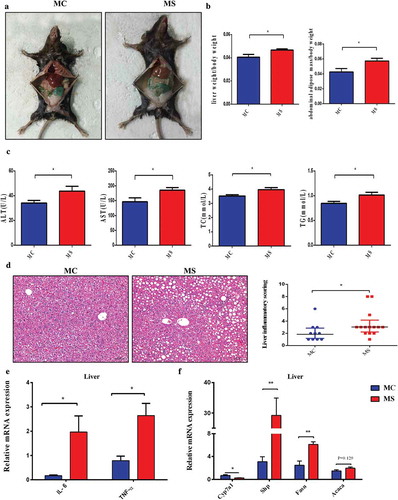
We assessed the hepatic histology in 3-week-old offspring by H&E staining. There was no significant macroscopic inflammation in the two groups (Figure S1A). However, the relative expression of TNF-α, IL-1β, and IL-6 significantly increased in MS group compared with MC group (Figure S1B). H&E staining confirmed prominent fatty liver in both two groups of 12-weeks old. More importantly, the steatosis in MS group was more serious than that in MC, with plenty infiltration of inflammatory cells and emergence of partial piecel necrosis. Consistent with this, NAFLD Activity Score (NAS) was also significantly higher in MS group than that in MC (). Additionally, the inflammatory cytokines in liver such as IL-6 and TNF-α also elevated in MS group compared with MC group ().
MS disturbed hepatic lipid metabolism in adult mice
The levels of several genes associated with lipid metabolism in liver were detected. The fatty acid synthesis genes such as Fasn and Acaca elevated in MS group compared with MC. Cyp7a1, the rate-limiting enzyme for bile acid synthesis was suppressed in MS group, and shp, also a key gene regulating bile acid synthesis in the liver, was highly upregulated (). These genes expression alteration suggested that MS disturbed the biosynthesis and metabolism of fatty acid and bile acids.Citation30
HFD exacerbated dysbiosis in adult mice
Our studies then focused on the gut microbiota change after 4 weeks HFD in MS and MC groups. A total of 336 OTUs in MS group and 285 OTUs in MC group were shown by Venn diagram, with 269 OTUs shared (). Compared with 4 weeks ago, the relative abundance of Firmicutes and Proteobacteria elevated while Bacteroidetes reduced in both two groups. At phylum level, even after 9 weeks HFD, the abundance of Proteobacteria decreased more significantly in MS group than in MC group ().
Figure 7. Maternal sucralose intake exacerbated gut dysbiosis in 12-week-old offspring mice. Venn diagram (a). Relative abundance of bacterial genera in two groups (b). Alpha diversity including Shannon and Simpson diversity index (c). PCoA with Adonis test indicating Beta diversity was shown (d). PCoA at 3 and 12 weeks offspring based on unweighted UniFrac distances (e). MC, maternal control diet. MS, maternal sucralose intake. PCoA, principal coordinate analysis. MC: n = 5, MS: n = 9. *p < .05, **p < .01.
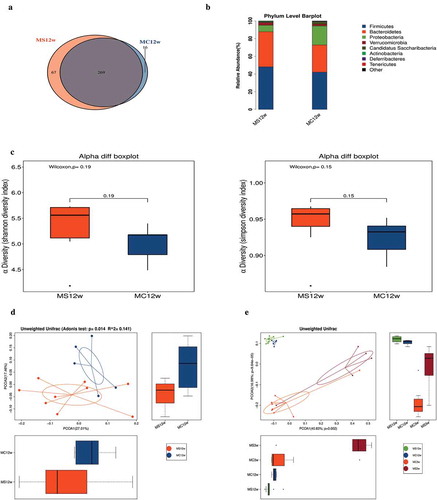
Figure 8. Clostridium butyricum decreased inflammatory cytokines production through activating GPR43. Immunohistochemical staining of GPR43 in the colonic tissues of 3-week-old offspring (a). The relative expression of GPR41, GPR43, and GPR109A in colonic tissues of 3-week-old offspring was presented by real-time PCR (b). The relative mRNA expression of GPR41, GPR43, and GPR109A when stimulating with Clostridium butyricum and sodium butyrate in HCT116 cells were presented (c). The relative mRNA expression of GPR41, GPR43, and GPR109A when stimulating with Clostridium butyricum and sodium butyrate in HCT8 cells were presented (d). The relative mRNA expression of TNF-α when stimulating with Clostridium butyricum and sodium butyrate in HCT116 and HCT8 cells was shown (e). The expression of GPR43 in Control SiRNA and GPR43 siRNA groups of HCT116 and HCT8 cells (f). Expression of TNF-α in GPR43-knockdown HCT116 and HCT8 cells relative to the control (D). MC, maternal control diet. MS, maternal sucralose intake. CB, Clostridium butyricum. NaBu, sodium butyrate. MC: n = 7, MS: n = 5. Scale bar: 50 µm. *P < .05, **P < .01, ***P < .001.
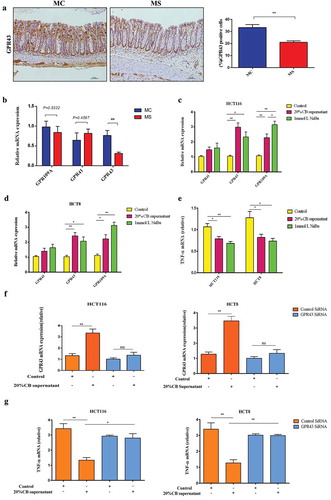
There was no significant difference found between two groups by Shannon and Simpson diversity index which represent alpha diversity after 4 weeks HFD (). Referring to Beta diversity, Adonis test showed significant difference between the two groups (). Notably, PCoA at 3- and 12-week-old offspring based on unweighted UniFrac distances revealed that the HFD indeed partly diluted the difference of gut microbiome driven by MS (). Together, our results revealed that HFD seriously exacerbated the dysbiosis of offspring gut microbiota. However, even after HFD, the gut microbiota of MS group was still partly different from MC group after 4 weeks HFD.
Discussion
NAFLD has become the most common liver disease worldwide but the pathogenesis has not been fully illuminated. A growing body of evidence supports the concept that maternal diet is associated with NAFLD through altering gut microbiota.Citation31,Citation32It is reported that sucralose can alter the composition of the gut microbiome and affect host health.Citation8,Citation9For example, maternal exposure to sucralose and acesulfame-K during pregnancy and lactation impacts hepatic metabolism and gut microbiome of offspring pre- and post-weaning.Citation12However, the long-term results of offspring in adulthood with MS consumption remain unknown. The main finding of our study was that MS supplement during pregnancy and lactation can inhibit intestinal development, disrupt gut barrier integrity, alter intestinal microbial composition, and increase inflammatory cytokines formation in offspring mice and finally aggravate HFD-induced hepatic steatosis in adulthood. In addition, MS intake reduces the butyrate-producing bacteria and butyrate production of the offspring, impairs gut barrier and then leads to low-grade inflammation through GRP43. This is the first experimental evidence in support of the hypothesis that MS in early life may increase the susceptibility to hepatic steatosis of offspring in adulthood. More importantly, the potential mechanism may lie in that MS induces gut dysbiosis including decreasing butyrate-producing bacteria and butyrate production and alters the intestinal barrier function which supports the concept of “gut-liver axis.”
It is widely believed that maternal diet is associated with progeny’s body weight gain and obesity.Citation33,Citation34 However, the effects of sucralose on the weight gain were not fully consistent in previous studies. Abou-Donia reported that administration of sucralose approximately 1.1 to 11 mg/kg BW/day from the 4th week after birth for 12 weeks significantly increased body weight in rats.Citation35 While Takashi Uebanso showed that sucralose supplement approximately 14.2 mg/kg BW/day for 8 weeks did not change the body weight of mice.Citation7Chih-Wei Liu et al. also revealed that no significant body weight change was observed after sucralose intake with 0.1 mg/ml from 8 weeks old for 6 months. One of the main reasons of the differences may lie in the time and amount of sucralose intake. In our study, pups in MS group showed more body weight gain at weaning and after HFD. Interestingly, similar results were found in previous studies when maternal HFD was fed in the perinatal period.Citation4,Citation36Three-week-old is the key point for gut barrier formation and intestinal microbiota colonization, and microbes in early-life play a key role in body weight gain.Citation13,Citation37 We may presume the effect of MS on offspring body weight through its impact on gut barrier and gut microbiota.
Undoubtedly, maternal nutrients are of the essence to the progeny’s intestinal development.Citation38−Citation40Our previous report has already shown that probiotics supplementation in early life can promote intestinal proliferation and differentiation.Citation41In contrast, the effect of early sucralose consumption on the offspring intestinal development has not been investigated before. In our study, MS negatively impacts the intestinal development of offspring in early life. It is in accord with the hypothesis that nutrients are thought to regulate initial colonization of perinatal microbiota, and gut dysbiosis can change biological process in the intestinal tract and throughout the body.Citation29
The gut microbiota is a dynamic community, and maintaining a proper balance of it is vital for the host health.Citation42 Transmission of the microbiome from mother to offspring indeed exists and maternal gut microbiota is proved to be more persistent in the infant's gut and ecologically better adapted than those acquired from other sources.Citation43 Several studies have demonstrated that sucralose could alter the microbiota.Citation5–Citation7,Citation9,Citation44In our present study, maternal sucralose significantly altered composition and diversity of gut microbiota in 3-week-old offspring mice. It is consistent with the notion that the gut microbiota colonization initiates already prenatally by placenta and amniotic fluid, and continues by breast milk after birth.Citation45 In the report of Takashi Uebanso, sucralose intake from weaning reduced the relative amount of Clostridium cluster XIVa in feces of the mice. Our results showed that more abundant genera were involved in offspring mice with MS supplement, which supported the notion that the age of initiating intervention is a critical factor which influences the gut microbiota.Citation46 It is noteworthy that the gut microbiota in MS group was still partly different from MC group after 4 weeks HFD, which indicates that the impact of dysbiosis induced by MS is far-reaching.
In the present study, MS can promote the production of pro-inflammatory mediators and trigger low-grade intestinal inflammation in offspring at 3 weeks of age, which supports the widely accepted notion that inflammation is one of the most common effects associated with gut microbiome dysbiosis.Citation47,Citation48 On one hand, dysbiosis can influence the maturation of inducible regulatory T cells (iTregs) induction, which may be responsible for the decreased secretion of anti-inflammatory cytokines.Citation49,Citation50 On the other hand, among the more abundant genera in MS group, several genera were found to be associated with inflammation. Moreover, decreased abundance of SCFA-producing bacteria was also associated with the reduction of iTregs and activation of proinflammatory cells.Citation51–Citation53
As one of the most important aspects of gut barrier function, further damage to the integrity of the gut barrier also goes along with dysbiosis and intestinal low-grade inflammation.Citation15 In this study, we observed a reduction of MUC2 positive cells and goblet cells and in the colon, together with reduced tight junction proteins. These findings suggest that MS significantly disrupts the gut barrier integrity of offspring at 3 weeks. Meanwhile, the increased abundance of Akkermansia may be partly responsible for the decreased secretion of mucin in goblet cells.Citation54
Additionally, our results revealed that MS reduced the butyrate-producing bacteria in 3-week-old offspring, which was consistent with their cecal butyrate decline. Butyrate-producing bacteria and butyrate have positive effects on the host’s health in several aspects. Butyrate has been reported to inhibit histone deacetylase activity, regulate macrophage function, as well as remodel regulatory T cell expansion in mice.Citation55,Citation56 Butyrate also fine-tunes the immune system and metabolic homeostasis in early life, potentially setting the stage for long-term metabolic and immune health.Citation57–Citation59 In addition, increasing evidence has shown that butyrate also plays a critical role in the development of NAFLD.Citation27 It has been reported that butyrate enhances the intestinal barrier function by facilitating tight junction assembly via activation of AMP-activated protein kinase (AMPK) and attenuates HFD-induced steatohepatitis.Citation60,Citation61Moreover, Hitoshi Endo et al. showed that treatment with a butyrate-producing probiotic, MIYAIRI 588, significantly reduced hepatic lipid deposition and improved the triglyceride content, insulin resistance, serum endotoxin levels, and hepatic inflammatory indexes.Citation62 In our present study, we also found that the expression of pro-inflammatory factors such as TNF-αsignificantly increased in liver of 3-week offspring in MS group. Besides, they found that MIYAIRI 588 also improved the expression of the intestinal tight junction proteins such as ZO-1 and Occludin. And we may consider the down-regulated expression of tight junction proteins in our present study was partly due to the reduced butyrate-producing bacteria and butyrate. It has been reported that butyrate activates the GPCRs including GPR41, GPR43, and GPR109A, which are expressed on intestinal epithelial cells and adipocytes. Activation of these GPCRs appears to alleviate the progression of NAFLD via multiple mechanisms.Citation27In our present study, we found a significantly decreased expression of GPR43 in colon in MS group. Moreover, in in vitro study, we found that one of the butyrate-producing bacteria inhibited pro-inflammatory factors via activating GPR43. Thus, we consider that sucralose intake could reduce the butyrate-producing bacteria and butyrate production of the offspring, impair gut barrier and then lead to low-grade inflammation through GRP43.
According to the conception of “gut-liver axis,” low-grade inflammation and impaired intestinal barrier play a critical role in promoting the progression and possibly the initiation of NAFLD.Citation63,Citation64 The increased pro-inflammation mediators and detrimental metabolites in the gut such as endotoxin can translocate into portal system through the impaired gut barrier, leading to multiple biologic damage to the liver. In the study of Stephanie Olivier-Van Stichelen et al,Citation12they investigated how the progeny’s hepatic metabolic changed till 19 days after birth with maternal non-nutritive sweeteners intake. The offspring were breastfed during the experiment without other kinds of diet. They found no macroscopic damage to the liver, but both carbohydrate and lipid metabolism were found to be altered in the non-nutritive sweetener pups. In our present study, we also found MS did not induce obvious macroscopic hepatic inflammation in offspring at weaning, although the pro-inflammatory cytokines significantly increased. And we found that MS finally exacerbated HFD-induced hepatic steatosis in adult mice. These data strongly support the conception of “gut-liver axis.”
MS intake during pregnancy and lactation disturbs the microbiome of mothers. Transmission of the microbiome from mother to offspring indeed exists, thus gut dysbiosis can be found in the neonatal stage of the offspring, accompanied by the reduction of butyrate-producing bacteria and butyrate production. Butyrate has an effect of suppressing inflammation and improving gut barrier function through activating GRP43. With the reduction of butyrate, pro-inflammatory factors in the gut lumen are highly increased, and the tight junction is dramatically impaired. According to the concept of “gut-liver axis,” the endotoxin and the other detrimental microbial metabolites may rush into portal vein through the impaired gut barrier and upregulate pro-inflammatory cytokines production in the liver. The constant low-grade inflammation further promotes hepatic steatosis in adulthood in MS group.
The potential mechanisms of how MS intake alters gut microbiota of offspring and exacerbates hepatic steatosis in adulthood are presented in .
Figure 9. The potential mechanism of how maternal sucralose intake alters gut microbiota of offspring and exacerbates hepatic steatosis in adulthood. Maternal sucralose intake during pregnancy and lactation disturbs the microbiome of mothers. Transmission of the microbiome from mother to offspring indeed exists, thus gut dysbiosis can be found in the neonatal stage of the offspring, accompanied by the reduction of butyrate-producing bacteria and butyrate production. Butyrate has an effect of suppressing inflammation and improving gut barrier function through activating GRP43. With the reduction of butyrate, pro-inflammatory factors in the gut lumen are highly increased, and the tight junction is dramatically impaired. According to the concept of “gut-liver axis,” the endotoxin and the other detrimental microbial metabolites may rush into portal vein through the impaired gut barrier and upregulate pro-inflammatory cytokines production in the liver. The constant low-grade inflammation further promotes hepatic steatosis in adulthood in MS group.
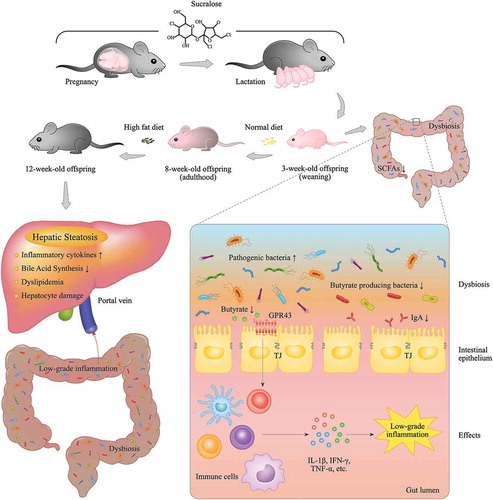
In summary, this study first demonstrates that MS intake can change the composition and diversity of gut microbiome, reduce butyrate-producing bacteria and butyrate, interfere intestinal development, induce low-grade inflammation as well as disrupt gut barrier function in 3-week-old offspring. Additionally, the microbial analysis in adulthood reveals that the impact of dysbiosis induced by MS is long-lasting, which may contribute to the exacerbation of the susceptibility of hepatic steatosis in adulthood. And finally, MS exacerbated HFD-induced hepatic steatosis in adulthood. These data strongly support the conception of “gut-liver axis,” and that MS intake may be a potential threat for NAFLD in adulthood. As sucralose is widely used around the world, our findings may remind the pregnant women that more caution should be given to excessive sucralose consumption.
Methods and materials
Animals and diet
Eight-weeks old female C57BL/6 mice were purchased from the Institute of Laboratory Animal Science, Chinese Academy of Medical Sciences & Peking Union Medical College. After 2 weeks of acclimation in the mouse facility, they were exposed to a 12-h light/dark cycle in a temperature and humidity-controlled specificpathogen-free environment. After mating with C57BL/6 males, all the pregnant female mice were randomly divided into two groups: On the basis of standard rodent chow (3.85 kcal/g; 10 E% fat, 20 E% protein, 70 E% carbohydrate; H10010, Research Diets, Peking, China), maternal control group mice were given distilled water; MS group mice were given sucralose solution of 0.1 mg/ml, approximately 5–15 mg/kg BW/day, and equal to upper limit of FDA acceptable daily intake.Citation7,Citation12 Throughout the period of gestation (3 weeks) and lactation (3 weeks), MS group mice consumed sucralose solution, whereas MC group mice consumed the same amount of distilled water as control on the basis of standard diet consistently.
Subsequently, pups were delivered through the vagina and the body weight was recorded weekly until 8 weeks. After weaning at 3 weeks of age, pups were separated from the dams, received standard rodent chow and regular drinking water ad libitum till 8 weeks. Finally, all the pups were treated with a HFD (HFD,5.24 kcal/g; 20 E% carbohydrate, 20 E% protein, 60 E% fat; H10060, Research Diets, Peking, China) for 4 weeks (). The research program was evaluated and approved by the Animal Ethical and Welfare Committee of Tianjin Medical University.
Blood measurements and tissue collection
Blood was drawn, centrifuged (3000 rpm, 8 min, 4°C) and serum was collected. Serum liver enzymes including ALT, AST, and lipid including TG and TC were determined by enzymatic colorimetric method.
Mice were sacrificed via CO2 inhalation at 3 and 12 weeks of age. Liver, small intestine, colonic tissues, cecal contents, and abdominal adipose tissue were dissected immediately. The weight of liver and abdominal adipose was measured. Liver tissues and intestinal specimens were quickly stored in a refrigerator at −80°C for further extraction of RNA and protein. A part of liver and intestinal tissues were washed with ice-cold phosphate-buffered saline (PBS) solution and formalin-fixed for further pathological evaluation. Before ending the experiment, we collected the fresh stool samples and then froze in the liquid of nitrogen for gut microbiota analysis.
Histology, immunohistochemistry, and immunofluorescence
To evaluate the histology of liver, the paraffin sections of 4-µm thick were stained by hematoxylin-eosin (HE) in the 3-week-old and 12-week-old offspring. The general histological features, extent of steatosis, inflammation, and fibrosis were evaluated. NAS was used for scoring the severity of hepatic steatosis as previously detailed.Citation65,Citation66
The paraffin sections of small intestine and colon were also stained with H&E. The histological inflammation of colon was evaluated by the degree of crypt damage. The intestinal development was evaluated by measuring villus/crypt length. We randomly chose 300 well-orientated villi/crypts and observed under light microscopy at 200× magnification for each mouse. The expression of Ki67 in small intestine and mucin 2 (MUC2), G-protein coupled receptor 43 (GPR43) in colon were evaluated by immunohistochemistry. Deparaffinized sections were incubated with primary antibodies rabbit monoclonal anti-Ki67 (ab16667, Abcam, Cambridge, MA, USA), rabbit anti-MUC2 (Santa Cruz Biotechnology, Inc), and GPR43 (ab118449, Abcam, Cambridge, MA, USA) overnight at 4°C. After cleaning with PBS, the secondary antibody (Santa Cruz Biotechnology, Inc.) labeled with horseradish peroxidase (HRP) was cultured for half an hour. Finally, 3,3ʹ-diaminobenzidine was used for re-staining. The same pathologists observed them blindly with optical microscopy (Lycra, Germany).
The expression of zona occludens-1 (ZO-1) in colon and IgA in small intestine was assessed by immunofluorescence. Deparaffinized sections were first incubated at 4°C overnight with rabbit anti-ZO-1 antibody (Invitrogen Corporation, Carlsbad, CA) or anti-IgA antibody (Invitrogen Corporation, Carlsbad, CA), and then secondary incubation was processed for 1 h at room temperature with FITC-labeled anti-rabbit antibody (Cell Signaling Technology). Finally, the nuclei were stained with DAPI (4,6-diamino-2-phenylindole, blue) and observed with fluorescence microscope (Leica, Germany).
PAS staining
Deparaffinized sections of colon were immersed for 10 min with 1% Periodic acid solution (Sigma-Aldrich), then stained with Schiff reagent (Sigma-Aldrich) for 40 min again. The PAS-stained sections were counterstained for another 2–5 min using Hematoxylin. During each step, washing buffer was an extensive PBS solution.
Real-time PCR analysis
Total RNA of the liver, intestine, and cells were extracted with the RNeasy mini kit (Qiagen, Carlsbad, CA, USA). cDNA synthesis was performed using the TIANScript RT kit (TIANGEN, Inc. Beijing, China). And real-time-PCR analyses were performed as described previously.Citation67 We chose glyceraldehyde-3-phosphate dehydrogenase (GAPDH) as the endogenous control, and the mRNA levels were calculated using2−∆∆Ct method. The primer sequences of target genes are listed in .
Table 1. Primer sequences used in real-time PCR.
SCFAs analysis
The concentrations of SCFAs (including acetate, propionate, and butyrate) in cecal contents were determined by high-performance liquid chromatography (HPLC) analysis method. Sample preparation and HPLC analysis were carried out according to the previous studies.Citation66,Citation68,Citation69 For data interpretation, Agilent Chem Station software was used.
Gut microbiota analysis
Stool samples of 3-week-old pups and 12-week-old mice in two groups were sent to the Real bio Genomics Institute (Shanghai, China) for 16 S rRNA gene sequencing. Each stool sample was extracted according to the manufacturer’s instructions (QIAamp DNA Stool Mini Kit, Qiagen, Hilden, Germany). General primers (341 F: ACTCCTACGGGAGGCAGCAG, and 806 R: GGACTACHVGGGTWTCTAAT) were used to amplify 16 S rDNA, targeting 16 S genes V3–4 region. KAPA HiFi Hot start PCR kit was used for high fidelity PCR. Illumina TruSeq DNA PCR-Free Library Preparation Kit (Illumina, USA) was utilized to generate QIIME software package (Quantitative Insights Into) as well as sequencing libraries. Sequences were expressed as OTUs. Afterward, QIIME platform was used for further classification analysis on the basis of OTUs sequences. Diversity was also analyzed.
Cells and bacterial preparation
Clostridium butyricum was used as the representative of butyrate-producing bacteria because it was one of the most commonly used butyrate-producing bacteria in clinical studies and basic science.Citation70–Citation74 Clostridium butyricum (ATCC 19398), which was kindly provided by China General Microbiological Culture Collection Center was incubated in brain heart infusion medium at 37°C in an anaerobic environment for 24 h. And then the bacteria were harvested with centrifugation (3000 g × 5 min) and resuspended in sterile PBS at the density of 1 × 1010 CFU/ml. Then, we collected the supernatant, filtered it through aperture filters of 0.22-μm, and then diluted it with complete culture medium.
We obtained human colorectal cancer cell lines (HCT8 and HCT116) from American Type Culture Collection (ATCC, Manassas, VA, USA). HCT116 cells were grown in Dulbecco’s Modified Eagle Medium supplemented with 10% fetal bovine serum (FBS); while HCT8 cells were grown in Roswell Park Memorial Institute 1640 medium with 10% FBS. Both the cells were maintained in a humidified atmosphere with 95% air and 5% carbon dioxide at 37°C.
Treatment of HCT116 and HCT8 cells with Clostridium butyricum
HCT116 and HCT8 cells were stimulated with 1 mmol/L sodium butyrate or Clostridium butyricum supernatant at a concentration of 20% for 24 h for further analysis. The treating concentration and time were selected based on our previous research.Citation74 The treatment lasted for 24 h and then the cells were collected for real-time PCR analysis. The relative expression of GPR43 and TNF-α was detected.
Gene silencing of GPR43 using siRNA
We applied small interfering RNA technology at the aim of knocking down GPR43 in the HCT8 and HCT116 cells as described in previous experiment. The targeted sequences of the selected region to be targeted by siRNA for GPR43 were the sense strand, 5'-GGCCUGGGUUAUGUCCUUUTT-3', the antisense strand, 5'-AAAGGACAUAACCCAGGCCTT-3' (GenePharma, Shanghai, China) and negative control siRNA (nontargeting siRNA)were sense strand, 5'-UUCUCCGAACGUGUCACGUTT-3', antisense strand, 5'-ACGUGACACGUUCGGAGAATT-3'. HCT116 and HCT8 Cells were seeded into six-well plates and transfected with GPR43 siRNA of 2 μg or control siRNA with Lipofectamine 2000 (Invitrogen) in Opti-MEM. The process followed the manufacturer's instructions, respectively. After 6 h, culture medium was replaced and 20% Clostridium butyricum supernatant or 1 mmol/L sodium butyrate was added. The treatment lasted for 24 h and then the cells were collected for real-time PCR analysis. The relative expression of GPR43 and TNF-α was detected.
Data analysis
Data Statistical analysis and graph design were implemented using SPSS 21.0 (SPSS, Chicago, IL, USA) and Prism (GraphPad Software, La Jolla, CA, USA). Data were expressed as the mean ± SEM. Rank sum test or non-paired t-test was used for the comparison of the two groups. One-way ANOVA was applied for comparison of multiple groups. A p value less than 0.05 was defined as statistically significant.
Author Contributions
XD, ZXG, DFC, LL, XLS, TYL, GJ, YL, YL, and AA performed experiments and analyzed data. XD, ZXG, and DFC drafted the manuscript and created figures; XD and HLC conceived the project; CY, BMW, and HLC directed the research and made the critical revision. All authors identified the manuscript and finally approved the article.
Disclosure of potential conflicts of interest
No potential conflicts of interest were disclosed.
Supplemental Material
Download TIFF Image (5.8 MB)Supplementary Material
Supplemental data for this article can be accessed on the publisher’s website.
Additional information
Funding
References
- Leung C, Rivera L, Furness JB, Angus PW. The role of the gut microbiota in NAFLD. Nat Rev Gastroenterol Hepatol. 2016;13(7):412. doi:10.1038/nrgastro.2016.85.
- Geva-Zatorsky N, Sefik E, Kua L, Pasman L, Tan TG, Ortiz-Lopez A, Yanortsang TB, Yang L, Jupp R, Mathis D, et al. Mining the human gut microbiota for immunomodulatory organisms. Cell. 2017;168(5):928–943.e11. doi:10.1016/j.cell.2017.01.022.
- Tamburini S, Nan S, Han CW, Clemente JC. The microbiome in early life: implications for health outcomes. Nat Med. 2016;22(7):713–722. doi:10.1038/nm.4142.
- Wankhade UD, Zhong Y, Kang P, Alfaro M, Chintapalli SV, Thakali KM, Shankar K. Enhanced offspring predisposition to steatohepatitis with maternal high-fat diet is associated with epigenetic and microbiome alterations. PLoS One. 2017;12(4):e0175675. doi:10.1371/journal.pone.0175675.
- Suez J, Korem T, Zeevi D, Zilberman-Schapira G, Thaiss CA, Maza O, Israeli D, Zmora N, Gilad S, Weinberger A, et al. Artificial sweeteners induce glucose intolerance by altering the gut microbiota. Nature. 2014;70(7521):181–186. doi:10.1038/nature13793.
- Swithers SE. Artificial sweeteners produce the counterintuitive effect of inducing metabolic derangements. Trends Endocrinol Metab Tem. 2013;24(9):431–441. doi:10.1016/j.tem.2013.05.005.
- Uebanso T, Ai O, Kitayama R, Yoshimoto A, Nakahashi M, Shimohata T, Mawatari K, Takahashi A. Effects of low-dose non-caloric sweetener consumption on gut microbiota in mice. Nutrients. 2017;9(6):560. doi:10.3390/nu9060560.
- Ruiz-Ojeda FJ, Plaza-Díaz J, Sáez-Lara MJ, Gil A. Effects of sweeteners on the gut microbiota: a review of experimental studies and clinical trials. Adv Nutr. 2019;10(suppl_1):S31–S48. doi:10.1093/advances/nmy037.
- Bian X, Chi L, Gao B, Tu P, Ru H, Lu K. Gut microbiome response to sucralose and its potential role in inducing liver inflammation in mice. Front Physiol. 2017;8:487. doi:10.3389/fphys.2017.00487.
- Dhurandhar D, Bharihoke V, Kalra S. A histological assessment of effects of sucralose on liver of albino rats. Morphologie. 2018;102(338):197–204. doi:10.1016/j.morpho.2018.07.003.
- Liu CW, Chi L, Tu P, Xue J, Ru H, Lu K. Quantitative proteomics reveals systematic dysregulations of liver protein metabolism in sucralose-treated mice. J Proteomics. 2019;196:1–10. doi:10.1016/j.jprot.2019.01.011.
- Olivier-Van Stichelen S, Rother KI, Hanover JA. Maternal exposure to non-nutritive sweeteners impacts progeny’s metabolism and microbiome. Front Microbiol. 2019;10:1360. doi:10.3389/fmicb.2019.01360.
- Sangild PT. Gut responses to enteral nutrition in preterm infants and animals. Exp Biol Med. 2006;231(11):1695–1711. doi:10.1177/153537020623101106.
- Innis SM, Dai C, Wu X, Buchan AM, Jacobson K. Perinatal lipid nutrition alters early intestinal development and programs the response to experimental colitis in young adult rats. Am J Physiol Gastrointest Liver Physiol. 2010;299(6):G1376. doi:10.1152/ajpgi.00258.2010.
- Dai X, Wang B. Role of gut barrier function in the pathogenesis of nonalcoholic fatty liver disease. Gastroenterol Res Pract. 2015;2015:1–6. doi:10.1155/2015/287348.
- Anderson JM, Van Itallie CM. Tight junctions and the molecular basis for regulation of paracellular permeability. Am J Physiol. 1995;269:467–475.
- Le Chatelier E, Nielsen T, Qin J, Prifti E, Hildebrand F, Falony G, Almeida M, Arumugam M, Batto JM, Kennedy S, et al. Richness of human gut microbiome correlates with metabolic markers. Nature. 2013;500:541–546.
- Wu TR, Lin CS, Chang CJ, Lin TL, Martel J, Ko YF, Ojcius DM, Lu CC, Young JD, Lai HC. et al. Gut commensal Parabacteroides goldsteinii plays a predominant role in the anti-obesity effects of polysaccharides isolated from Hirsutella sinensis. Gut. 2018;68:248–262. gutjnl-2017-315458.
- Yamei Y, Yujia P, Jilong T, Mi J, Lu L, Li X, Ran L, Zeng X, Cao Y. Effects of anthocyanins from the fruit of Lycium ruthenicum Murray on intestinal microbiota. J Funct Foods. 2018;48:533–541. doi:10.1016/j.jff.2018.07.053.
- Turnbaugh PJ, Ley RE, Mahowald MA, Magrini V, Mardis ER, Gordon JI. An obesity-associated gut microbiome with increased capacity for energy harvest. Nature. 2006;444(7122):1027–1031. doi:10.1038/nature05414.
- Kostic AD, Xavier RJ, Gevers D. The microbiome in inflammatory bowel disease: current status and the future ahead. Gastroenterology. 2014;146(6):1489–1499. doi:10.1053/j.gastro.2014.02.009.
- Zhang C, Zhang M, Pang X, Zhao Y, Wang L, Zhao L. Structural resilience of the gut microbiota in adult mice under high-fat dietary perturbations. Isme J. 2012;6(10):1848–1857. doi:10.1038/ismej.2012.27.
- Willing BP, Dicksved J, Halfvarson J, Andersson AF, Lucio M, Zheng Z, Järnerot G, Tysk C, Jansson JK, Engstrand L, et al. A pyrosequencing study in twins shows that gastrointestinal microbial profiles vary with inflammatory bowel disease phenotypes. Gastroenterology. 2011;139(6):1844–1854.e1. doi:10.1053/j.gastro.2010.08.049.
- Kang C, Wang B, Kaliannan K, Wang X, Lang H, Hui S, Huang L, Zhang Y, Zhou M, Chen M, et al. Gut microbiota mediates the protective effects of dietary capsaicin against chronic low-grade inflammation and associated obesity induced by high-fat diet. MBio. 2017;8(3):e00470–17. doi:10.1128/mBio.00470-17.
- Porras D, Nistal E, Martínez-Flórez S, Pisonero-Vaquero S, Olcoz JL, Jover R, González-Gallego J, García-Mediavilla MV, Sánchez-Campos S. Protective effect of quercetin on high-fat diet-induced non-alcoholic fatty liver disease in mice is mediated by modulating intestinal microbiota imbalance and related gut-liver axis activation. Free Radic Biol Med. 2016;102:188–202. doi:10.1016/j.freeradbiomed.2016.11.037.
- Clausen MR, Mortensen PB. Kinetic studies on colonocyte metabolism of short chain fatty acids and glucose in ulcerative colitis. Gut. 1995;37(5):684. doi:10.1136/gut.37.5.684.
- Chu H, Duan Y, Yang L, Schnabl B. Small metabolites, possible big changes: a microbiota-centered view of non-alcoholic fatty liver disease. Gut. 2019;68(2):359–370. doi:10.1136/gutjnl-2018-316307.
- Belkaid Y, Hand TW. Role of the microbiota in immunity and inflammation. Cell. 2014;157(1):121–141. doi:10.1016/j.cell.2014.03.011.
- Nash MJ, Frank DN, Friedman JE. Early microbes modify immune system development and metabolic homeostasis—the “Restaurant” hypothesis revisited. Front Endocrinol (Lausanne). 2017;8:349. doi:10.3389/fendo.2017.00349.
- Kim B, Park K, Ji Y, Park S, Holzapfel W, Hyun C. Protective effects of Lactobacillus rhamnosus GG against dyslipidemia in high-fat diet-induced obese mice. Biochem Biophys Res Commun. 2016;473(2):530–536. doi:10.1016/j.bbrc.2016.03.107.
- Wesolowski SR, Kasmi KCE, Jonscher KR, Friedman JE. Developmental origins of NAFLD: a womb with a clue. Nat Rev Gastroenterol Hepatol. 2016;14(2):81. doi:10.1038/nrgastro.2016.160.
- Soderborg TK, Clark SE, Mulligan CE, Janssen RC, Babcock L, Ir D, Young B, Krebs N, Lemas DJ, Johnson LK, et al. The gut microbiota in infants of obese mothers increases inflammation and susceptibility to NAFLD. Nat Commun. 2018;9(1):4462. doi:10.1038/s41467-018-06929-0.
- Moser VC, Mcdaniel KL, Woolard EA, Phillips PM, Franklin JN, Gordon CJ. Impacts of maternal diet and exercise on offspring behavior and body weights. Neurotoxicol Teratol. 2017;63:S0892036217300478. doi:10.1016/j.ntt.2017.07.002.
- Chia AR, Chen LW, Lai JS, Wong CH, Neelakantan N, Van dam RM, Chong MFF. Maternal dietary patterns and birth outcomes: a systematic review and meta-analysis. Adv Nutr. 2019;10(4):685–695. doi:10.1093/advances/nmy123.
- Abou-Donia M, El-Masry E, Abdel-Rahman A, Mclendon R, Schiffman S. Splenda alters gut microflora and increases intestinal P-Glycoprotein and cytochrome P-450 in male rats. J Toxicol Environ Health. 2007;71(21):1415–1429. doi:10.1080/15287390802328630.
- King V, Dakin RS, Liu L, Hadoke PWF, Walker BR, Seckl JR, Norman JE, Drake AJ. Maternal obesity has little effect on the immediate offspring but impacts on the next generation. Endocrinology. 2013;154(7):2514–2524. doi:10.1210/en.2013-1013.
- Mulligan CM, Friedman JE. Maternal modifiers of the infant gut microbiota: metabolic consequences. J Endocrinol. 2017;235(1):JOE-17-0303. doi:10.1530/JOE-17-0303.
- Noah TK, Donahue B, Shroyer NF. Intestinal development and differentiation. Exp Cell Res. 2011;317(19):2702–2710. doi:10.1016/j.yexcr.2011.09.006.
- Lillycrop KA, Burdge GC. Epigenetic mechanisms linking early nutrition to long term health. Best Pract Res Clin Endocrinol Metab. 2012;26(5):667–676. doi:10.1016/j.beem.2012.03.009.
- Liu P, Che L, Yang Z, Feng B, Che L, Xu S, Lin Y, Fang Z, Li J, Wu D, et al. A maternal high-energy diet promotes intestinal development and intrauterine growth of offspring. Nutrients. 2016;8(5):258. doi:10.3390/nu8050258.
- Fang Y, Liu L, Cao H, Moore DJ, Washington MK, Wang B, Peek RM, Acra SA, Polk DB. Neonatal colonization of mice with LGG promotes intestinal development and decreases susceptibility to colitis in adulthood. Mucosal Immunol. 2016;10(1):117–127. doi:10.1038/mi.2016.43.
- Nicholson JK, Holmes E, Kinross J, Burcelin R, Gibson G, Jia W, Pettersson S. Host-gut microbiota metabolic interactions. Science. 2012;336(6086):1262–1267. doi:10.1126/science.1223813.
- Ferretti P, Pasolli E, Tett A, Asnicar F, Gorfer V, Fedi S, Armanini F, Truong DT, Manara S, Zolfo M, et al. Mother-to-infant microbial transmission from different body sites shapes the developing infant gut microbiome. Cell Host Microbe. 2018;24(1):133–145.e5. doi:10.1016/j.chom.2018.06.005.
- Omran A, Ahearn G, Bowers D, Swenson J, Coughlin C. Metabolic effects of sucralose on environmental bacteria. J Toxicol. 2013 Dec 3 2013;2013(4):372986. doi:10.1155/2013/372986.
- Carmen CM, Samuli R, Juhani A, Erika I, Seppo S. Human gut colonisation may be initiated in Utero by distinct microbial communities in the placenta and amniotic fluid. Sci Rep. 2016;6(23129):23129. doi:10.1038/srep23129.
- Cox LM, Yamanishi S, Sohn J, Alekseyenko A, Leung J, Cho I, Kim S, Li H, Gao Z, Mahana D, et al. Altering the intestinal microbiota during a critical developmental window has lasting metabolic consequences. Cell. 2014;158(4):705–721. doi:10.1016/j.cell.2014.05.052.
- Hill DA, Artis D. Intestinal bacteria and the regulation of immune cell homeostasis. Annu Rev Immunol. 2010;28(28):623–667. doi:10.1146/annurev-immunol-030409-101330.
- Gensollen T, Iyer SS, Kasper DL, Blumberg RS. How colonization by microbiota in early life shapes the immune system. Science. 2016;352(6285):539–544. doi:10.1126/science.aad9378.
- Geuking MB, Cahenzli J, Lawson MA, Ng DK, Slack E, Hapfelmeier S, McCoy K, Macpherson A. Intestinal bacterial colonization induces mutualistic regulatory T cell responses. Immunity. 2011;34(6):794–806. doi:10.1016/j.immuni.2011.03.021.
- Tanoue T, Atarashi K, Honda K. Development and maintenance of intestinal regulatory T cells. Nat Rev Immunol. 2016;16(5):5. doi:10.1038/nri.2016.36.
- Atarashi K, Tanoue T, Shima T, Imaoka A, Kuwahara T, Momose Y, Cheng G, Yamasaki S, Saito T, Ohba Y, et al. Induction of colonic regulatory T cells by indigenous clostridium species. Science. 2011;331(6015):337–341. doi:10.1126/science.1198469.
- Gonçalves P, Araújo JR, Di SJ. A cross-talk between microbiota-derived short-chain fatty acids and the host mucosal immune system regulates intestinal homeostasis and inflammatory bowel disease. Inflamm Bowel Dis. 2018;24:558.
- Nakajima A, Kaga N, Nakanishi Y, Ohno H, Miyamoto J, Kimura I, Hori S, Sasaki T, Hiramatsu K, Okumura K, et al. Maternal high fiber diet during pregnancy and lactation influences regulatory T cell differentiation in offspring in mice. J Immunol. 2017;199(10):3516–3524. doi:10.4049/jimmunol.1700248.
- Gao X, Cao Q, Cheng Y, Zhao D, Wang Z, Yang H, Wu Q, You L, Wang Y, Lin Y, et al. Chronic stress promotes colitis by disturbing the gut microbiota and triggering immune system response. Proc Natl Acad Sci. 2018;115(13):201720696. doi:10.1073/pnas.1720696115.
- Park J, Kim M, Kang SG, Jannasch AH, Cooper B, Patterson J, Kim CH. Short-chain fatty acids induce both effector and regulatory T cells by suppression of histone deacetylases and regulation of the mTOR-S6K pathway. Mucosal Immunol. 2015;8(1):80–93. doi:10.1038/mi.2014.44.
- Chang PV, Hao L, Offermanns S, Medzhitov R. The microbial metabolite butyrate regulates intestinal macrophage function via histone deacetylase inhibition. Proc Natl Acad Sci U S A. 2014;111(6):2247–2252. doi:10.1073/pnas.1322269111.
- Gomez de Agüero M, Ganal-Vonarburg SC, Fuhrer T, Rupp S, Uchimura Y, Li H, Steinert A, Heikenwalder M, Hapfelmeier S, Sauer U, et al. The maternal microbiota drives early postnatal innate immune development. Science. 2016;351(6279):1296–1302. doi:10.1126/science.aad2571.
- Nash MJ, Frank DN, Friedman JE. Early microbes modify immune system development and metabolic homeostasis-the “Restaurant” hypothesis revisited. Front Endocrinol (Lausanne). 2017;8:349. doi:10.3389/fendo.2017.00349.
- Tilg H, Zmora N, Adolph TE, Elinav E. The intestinal microbiota fuelling metabolic inflammation. Nat Rev Immunol. 2020;20(1):40–54. doi:10.1038/s41577-019-0198-4.
- Peng L, Li ZR, Green RS, Holzman IR, Lin J. Butyrate enhances the intestinal barrier by facilitating tight junction assembly via activation of AMP-activated protein kinase in Caco-2 cell monolayers. J Nutr. 2009;139(9):1619–1625. doi:10.3945/jn.109.104638.
- Zhou D, Pan Q, Xin FZ, Zhang R-N, He C-X, Chen G-Y, Liu C, Chen Y-W, Fan J-G. Sodium butyrate attenuates high-fat diet-induced steatohepatitis in mice by improving gut microbiota and gastrointestinal barrier. World J Gastroenterol. 2017;23(1):60–75. doi:10.3748/wjg.v23.i1.60.
- Endo H, Niioka M, Kobayashi N, Tanaka M, Watanabe T. Butyrate-producing probiotics reduce nonalcoholic fatty liver disease progression in rats: new insight into the probiotics for the gut-liver axis. PLoS One. 2013;8(5):e63388. doi:10.1371/journal.pone.0063388.
- Wieland A, Frank DN, Harnke B, Bambha K. Systematic review: microbial dysbiosis and nonalcoholic fatty liver disease. Aliment Pharmacol Ther. 2015;42(9):1051–1063. doi:10.1111/apt.13376.
- Schnabl B, Brenner DA. Interactions between the intestinal microbiome and liver diseases. Gastroenterology. 2014;146(6):1513–1524. doi:10.1053/j.gastro.2014.01.020.
- Sellmann C, Priebs J, Landmann M, Degen C, Engstler AJ, Jin CJ, Gärttner S, Spruss A, Huber O, Bergheim I, et al. Diets rich in fructose, fat or fructose and fat alter intestinal barrier function and lead to the development of nonalcoholic fatty liver disease over time. J Nutr Biochem. 2015;26(11):1183–1192. doi:10.1016/j.jnutbio.2015.05.011.
- Li Y, Liu T, Yan C, Xie R, Guo Z, Wang S, Zhang Y, Li Z, Wang B, Cao H. Diammonium glycyrrhizinate protects against non-alcoholic fatty liver disease in mice through modulation of gut microbiota and restoring intestinal barrier. Mol Pharm. 2018;15:3860–3870. acs.molpharmaceut.8b00347.
- Xie R, Sun Y, Wu J, Huang S, Jin G, Guo Z, Zhang Y, Liu T, Liu X, Cao X, et al. Maternal high fat diet alters gut microbiota of offspring and exacerbates DSS-induced colitis in adulthood. Front Immunol. 2018;9:2608.
- Rau M, Rehman A, Dittrich M, Groen AK, Hermanns HM, Seyfried F, Beyersdorf N, Dandekar T, Rosenstiel P, Geier A, et al. Fecal SCFAs and SCFA-producing bacteria in gut microbiome of human NAFLD as a putative link to systemic T-cell activation and advanced disease. United Eur Gastroenterol J. 2018;6(10):1496–1507. doi:10.1177/2050640618804444.
- Wang L, Zhang J, Guo Z, Kwok L, Ma C, Zhang W, Lv Q, Huang W, Zhang H. Effect of oral consumption of probiotic lactobacillus planatarum P-8 on fecal microbiota, SIgA, SCFAs, and TBAs of adults of different ages. Nutrition. 2014;30(7–8):776–783.e1. doi:10.1016/j.nut.2013.11.018.
- Cassir N, Benamar S, La Scola B. Clostridium butyricum: from beneficial to a new emerging pathogen. Clin Microbiol Infect. 2016;22(1):37–45. doi:10.1016/j.cmi.2015.10.014.
- Seki H, Shiohara M, Matsumura T, Miyagawa N, Tanaka M, Komiyama A, Kurata S. Prevention of antibiotic-associated diarrhea in children by Clostridium butyricum MIYAIRI. Pediatr Int. 2003;45(1):86–90. doi:10.1046/j.1442-200X.2003.01671.x.
- Mo S, Kim BS, Yun SJ, Lee JJ, Yoon SH, Oh CH. Genome sequencing of clostridium butyricum DKU-01, isolated from infant feces. Gut Pathog. 2015;7(1):8. doi:10.1186/s13099-015-0055-3.
- Yasueda A, Mizushima T, Nezu R, Sumi R, Tanaka M, Nishimura J, Kai Y, Hirota M, Osawa H, Nakajima K, et al. The effect of Clostridium butyricum MIYAIRI on the prevention of pouchitis and alteration of the microbiota profile in patients with ulcerative colitis. Surg Today. 2016;46(8):939–949. doi:10.1007/s00595-015-1261-9.
- Chen D, Jin D, Huang S, Wu J, Xu M, Liu T, Dong W, Liu X, Wang S, Zhong W, et al. Clostridium butyricum, a butyrate-producing probiotic, inhibits intestinal tumor development through modulating Wnt signaling and gut microbiota. Cancer Lett. 2020;469:456–467. doi:10.1016/j.canlet.2019.11.019.