ABSTRACT
The association of gut microbiota with gastrointestinal carcinogenesis has been heavily investigated since the recent advance in sequencing technology. Accumulating evidence has revealed the critical roles of commensal microbes in cancer progression. Given by its importance, emerging studies have focussed on targeting microbiota to ameliorate therapeutic effectiveness. It is now clear that the microbial community is closely related to the efficacy of chemotherapy, while the correlation of microbiota with immunotherapy is much less studied. Herein, we review the up-to-date findings on the influence of gut microbiota on three common immunotherapies including adoptive cell transfer, immune checkpoint blockade, and CpG-oligodeoxynucleotide therapy. We then explore three microbiota-targeted strategies that may improve treatment efficacy, involving dietary intervention, probiotics supplementation, and fecal microbiota transplantation.
Introduction
The human gastrointestinal tract harbor thousands of microbial species. For example, intestines consist of a dense community with around 1013 microbes mainly from phyla Bacteroidetes and Firmicutes;Citation1 while microbial abundance in the stomach is the least along the tract due to its extreme acidity with predominant expression of Firmicutes and Proteobacteria.Citation2 These microorganisms form a microbiota (referring to an ecological community of microbes that is found within a specific environment), which interacts with a variety of host cells to contribute physiological functions including nutrient metabolism and gut barrier regulation.Citation3,Citation4 In particular, the gut microbiota substantially contributes to immune homeostasis as exemplified by using germ-free animals (referring to animals raised in the strict sterile conditions that have no microbes living in/on them), which displayed impaired development of regulatory T cells (TReg) and poor growth of gut-associated lymphoid tissues.Citation3–5 Whereas the host immunity can, in turn, manipulate the microbial profile: secretary immunoglobulin-A (IgA) from gut plasma cells has reactivity to a broad spectrum of microbes, and these IgA could enhance translocation of selected commensals into lymphoid tissues to facilitate antigen presentation and regulate microbial diversity.Citation6–8
The immunity-microbiota crosstalk is continuously regulated in a healthy state. Yet, such equilibrium is readily affected by host genetic background and numerous environmental factors especially dietary intake.Citation9–11 Once the extrinsic force overpowers the intrinsic stability, dysbiosis, termed as a compositional and functional alteration in the microbiota, can occur.Citation12 With aid of the next-generation microbial sequencing, it is now well established that a shift in microbiota profile is greatly associated with cancer development and progression,Citation13,Citation14 and tremendous work has been conducted to decipher the underlying mechanism. Briefly, enriched microbe-associated molecular patterns (MAMPs; molecules that are found in/on microbes, e.g. flagellins, lipopolysaccharides (LPS), and can be recognized by recognition receptors of the innate immune system) trigger enhanced toll-like receptor (TLR)-mediated immune response which leads to inflammation. While persistent inflammation can exacerbate the imbalanced microbial community, thus forming a vicious loop and eventually resulting in gastrointestinal carcinogenesisCitation14–16 (an illustration on this interaction is shown in ).
Figure 1. Gut dysbiosis interacts with host immunity to induce chronic inflammation. Blue and red rods represent beneficial commensals and pathobionts respectively. TLR4 from innate immunity recognizes MAMPs (e.g. LPS, flagellins) of dysbiotic microbiota and leads to initiation of NF-κB-dependent downstream production of pro-inflammatory cytokines (e.g. IL-1, IL-6, IL-17, IL-23). The NF-κB signaling cascade can also be activated by microbial derivatives especially a group of metabolites known as bile acid. In addition, dysbiosis can increase gut barrier permeability to induce translocation of pathobionts and metabolites from the mucosa to bloodstream, and eventually into the hepatopancreatic ductal system. All these processes can cause persistent inflammation, which can further exaggerate the imbalanced microbial community, thus forming a vicious cycle and promoting carcinogenesis
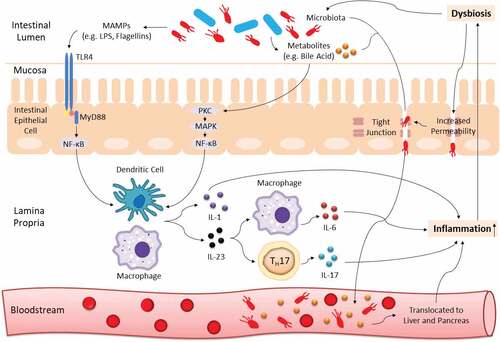
Given its pivotal role in gastrointestinal cancer, it is plausible to target the gut microbiota as a therapeutic strategy. Indeed, accumulated studies have illustrated that the commensal microbes can impact the efficacy of chemotherapy.Citation17 In comparison, evidence on how microbiota correlates with immunotherapy only emerges recently. Here we summarize and discuss the up-to-date findings on the association of gut microbiota with most-studied immunotherapies including adoptive cell transfer (ACT), immune checkpoint blockade, and CpG-oligodeoxynucleotide therapy. Also, in acknowledgment of a relatively deeper understanding of the association between microbiota and gastrointestinal cancer than other cancers, we highlight three microbiota-targeted strategies that may improve treatment efficacy against gastrointestinal cancer, involving dietary intervention, probiotics, and fecal microbiota transplantation (FMT).
Gut microbiota and immunotherapy
Resistance to traditional therapies including chemotherapy and radiotherapy is associated with high tumor recurrence and has been the key problem in curing cancer patients.Citation18 In the last decade a bloom of clinical trials has displayed the potential of immunotherapy in treating cancers, of which several FDA-approved strategies could provide long-lasting anticancer effects to patients who were unresponsive to conventional treatment. Meanwhile, apart from its role in carcinogenesis, the gut microbiota also demonstrates its influence across a range of cancer treatments.Citation19,Citation20 In the following section, we discuss the role of gut microbiota in three major immunotherapy approaches involving ACT, immune checkpoint blockade, and CpG-oligodeoxynucleotide therapy (summarized in ).
Table 1. Summary of the association of gut microbiota with host immunity to impact cancer immunotherapy
Adoptive immune cell transfer
ACT is a treatment approach to utilize autologous immune cells such as tumor-infiltrating lymphocytes (TILs) and cytotoxic T lymphocytes (CTLs) against cancer. It includes three steps: (1) isolating T cells from tumor tissues or peripheral blood vessels; (2) co-culturing with interleukin (IL)-2 to allow ex vivo expansion; and (3) reinfusion of extracted T cells back into the patients.Citation34,Citation35 Whereas most recent clinical trials involves genetically modified to enhance expression of antigen-specific T cell receptor (TCR) or chimeric antigen receptor (CAR) on extracted T cells, thereby triggering stronger anticancer immunological response after reinfusion to overcome immuno-resistant mechanisms of tumor cells (e.g. defective antigen processing).Citation34–36 ACT has shown a greater specificity than chemotherapy since autologous immune cells are used and could be genetically modified to recognize and target specific tumor antigens; thus, ACT is considered as a highly personalized cancer therapy.Citation37,Citation38 To date ACT especially CAR-T cell therapy has displayed remarkable success in eradicating hematologic malignanciesCitation36,Citation39 and metastatic melanoma.Citation40,Citation41 While the efficacy of ACT in gastrointestinal tumors including colorectal cancer (CRC),Citation42 hepatocellular carcinoma (HCC),Citation34 and esophageal cancerCitation43,Citation44 is limited, which may be attributed to poor trafficking to tumors, dysregulated TReg/effector T cell ratio, and immunosuppressive tumor microenvironment.Citation45,Citation46 Extensive work is ongoing to optimize ACT by modulating tumor-immune microenvironment (TIM),Citation46 modifying genetic engineering strategies,Citation47 or coupling with radiotherapy.Citation48 Arising trials are developing alternatives such as CAR-expressing nature killer (NK) cell therapy,Citation49 and combining dendritic cells and cytotoxic-induced killer cells to treat HCC and pancreatic cancer.Citation50–52
Gut Microbiota and Adoptive Immune Cell Transfer
The first evidence showing the correlation of gut microbiota with ACT efficacy was reported in 2007 when ACT plus total body irradiation (TBI; a form of lymphodepletion) were applied to a mouse model with a deficiency in a cluster of differentiation (Cd)-14 and Tlr-4.Citation22 Depleting microbiota by antibiotics or suppressing LPS signaling components impaired the function of adoptively transferred Cd8 + T cells and decreased the number of activated dendritic cells, resulting in reduced anticancer efficacy. Whereas LPS administration to TBI-treated microbiota-depleted mice could promote proliferation and function of reinfused T cells, and even be able to long-term cure mice with large tumors. Mechanically, TBI could induce microbial translocation particularly gram-negative LPS-producing bacteria into the mesenteric lymph nodes. These translocated microbes then initiate the Tlr4 pathway by expressing various Tlr4 agonists (e.g. LPS, peptidoglycan), leading to enhanced activation of dendritic cells with increased secretion of pro-inflammatory cytokines including Il-1β, Il-6, and tumor necrosis factor (Tnf)-α across the gut. Notably, LPS administration could improve anticancer response mediated by adoptively transferred Cd8 + T cells in TBI-untreated mice.
In 2018 Uribe-Herranz et al. applied ACT to tumor-bearing C57BL/6 mice fromtwo vendors (JAX and HAR) and found that tumor growth is almost completely abolished in HAR mice but not JAX mice.Citation21 The 16S rRNA sequencing revealed the difference in composition of fecal microbiota between JAX and HAR mice – a diverse range of Bacteroidetes taxa was present in HAR mice, while JAX mice was dominated by Bacteroidales S24-7, hence suggesting that ACT efficacy may correlate to several genera of Bacteroidetes including Bacteroides and Parabacteroides. An antibiotic vancomycin was then used to eliminate the gram-negative phylum Bacteroidetes in JAX and HAR mice. In terms of efficacy, no change was observed in HAR mice but tumor regression in JAX mice was greatly enhanced to match the impact of ACT on HAR mice without vancomycin treatment. Such amelioration was attributed to enhanced T helper cell (TH)-1-mediated immune response, and accumulation of peripheral Cd8α+ dendritic cells, resulting in increased expansion and activity of adoptively transferred T cells. Whilst no phenotypic change was observed under antibiotic treatments of neomycin and metronidazole, thus implicating the role of specific bacteria in mediating host response to ACT. Collectively, these findings suggest a potential way to enhance response to ACT by altering the gut microbiota, yet it remains elusive that which specific microbes are responsible for such improvement. An in-depth mechanistic study is, therefore, necessary to identify reliable microbial targets for modulating the ACT efficacy before proceeding to clinical trials.
Immune checkpoint blockade
The aim of immune checkpoint blockade is to restore and strengthen the anticancer response by suppressing the intrinsic immuno-inhibitory pathways, which are commonly utilized by tumor cells to develop immune resistance.Citation35 Enormous efforts have been invested to exploit the efficacy of treating cancer patients with fully-humanized monoclonal antibodies against two of the most-studied immune checkpoint regulators – cytotoxic T lymphocyte-associated antigen-4 (CTLA-4) and programmed cell death protein-1 (PD-1) or its ligand PD-1-ligand 1 (PD-L1). Both CTLA-4 and PD-1 are TCRs belonging to the immunoglobulin superfamily,Citation53,Citation54 but they share different features and mechanisms in regulating host immunity (Supplementary Table 1). To date, some immune checkpoint inhibitors (ICIs) have received FDA approval including blockers of PD-1 (nivolumab, pembrolizumab, and cemiplimab), PD-L1 (atezolizumab, avelumab, and durvalumab), and CTLA-4 (ipilimumab) for treating cancers, particularly metastatic melanoma, and nonsmall-cell lung cancer (NSCLC; Supplementary Table 2). These ICIs can also be used against several gastrointestinal cancers involving HCC,Citation55,Citation60 gastric cancer,Citation57,Citation58 esophageal carcinoma,Citation59 and DNA mismatch repair-deficient or microsatellite instability-high (dMMR/MSI-H) CRCCitation56,Citation61 (). However, ICI therapy is frequently linked with immune-related adverse events (irAEs) such as colitis and pneumonitis.Citation63,Citation64 Arising evidence has now revealed the correlation between irAEs incidence and efficacy of ICI therapy.Citation65,Citation66 Together with high variation in therapeutic responsiveness (45–60% for patients with melanoma or MSI-H tumors; and 15–30% for patients with solid tumorsCitation66), it is thus critical to develop strategies to reduce the occurrence of irAEs and enhance treatment efficacy.
Table 2. FDA-approved immune checkpoint blockade against gastrointestinal cancer
Given that CTLA-4 and PD-1 regulate immune response through distinct mechanisms, a combination of anti-CTLA-4 and anti-PD-1/PD-L1 drugs were therefore proposed to improve patient outcomes. An early animal study revealed that anti-CTLA-4 antibodies could act synergistically with PD-1 blockade to increase effector T cell infiltration and allow continuous expansion of tumor-specific T cells, thereby shifting TIM from suppressive to inflammatory.Citation67 Currently, there is one FDA-approved combination – nivolumab (3 mg/kg) plus low-dose ipilimumab (1 mg/kg) for treating metastatic melanoma, renal cell carcinoma (RCC; Supplementary Table 2), and dMMR/MSI-H CRC (). Most joint-ICI therapies yield more positive results in clinical trials with less side effects when comparing with monotherapy except for pembrolizumab-ipilimumab combined treatment, which showed high toxicity in melanoma patients.Citation68,Citation69 In dMMR/MSI-H CRC, the objective response rate and 12-month overall survival in patients treated with both nivolumab and ipilimumab increased by 24% and 12%, respectively, when comparing with patients receiving nivolumab only (NCT02060188,Citation56,Citation62). Similar results were reported when applying joint-ICI therapy with an uncommon dosage (1 mg/kg of nivolumab plus 3 mg/kg of ipilimumab) on patients with chemotherapy-refractory gastroesophageal cancer, of which the objective response rate, and 12-month progression-free survival increased by 12% and 9%, respectively, together with 30% decrease in the incidence of grade 3–4 treatment-related adverse events (NCT01928394,Citation70). Other novel approaches to improve the safety and efficacy of ICI therapy include combination with neoantigen vaccines, chemotherapy, or other anticancer drugs, as well as modulating the gut microbiota profile.Citation68,Citation69 In addition, Conforti et al. conducted a dedicated meta-analysis on a total of 11,351 cancer patients from 20 clinical trials to decipher whether gender difference can influence immune checkpoint blockade.Citation71 Their findings revealed that although both ICI therapy significantly improves overall survival in patients of both sexes, the magnitude of this benefit is largely sex-dependent with men showing much greater efficacy than women. Extensive work is therefore needed to improve treatment outcomes for women or perhaps designing differential immunotherapeutic approaches between men and women.
Gut microbiota and immune checkpoint blockade – preclinical studies
CTLA-4 blockade
In 2015 Vétizou et al. found that a significant decrease in activated effector Cd4 + T cells and TILs are occurred in tumor-bearing mice treated with broad-spectrum antibiotics or housed in germ-free conditions, resulting in ineffective CTLA-4 blockade.Citation23 Reduction of Bacteroidales and Burkholderiales in the faces of these microbiota-depleted mice was identified. Notably, re-colonization of species from these two taxa including Bacteroides thetaiotaomicron, Bacteroides fragilis, and/or Burkholderia cepacia into microbiota-depleted mice rescued CTLA-4 blockade resistance by promoting TH1-mediated immune response and dendritic cell maturation in tumor-draining lymph nodes, meanwhile alleviating anti-CTLA-4-induced colitis. Adoptive transfer of B. fragilis-specific TH1 cells could also restore sensitivity to CTLA-4 blockade. In another study, vancomycin supplementation to mice before administration of anti-CTLA-4 antibodies and dextran sulfate sodium (a colitogen to model blockade-induced colitis) provoked a more severe and largely fatal form of the disease, implicating the role of gram-positive bacteria in mitigating CTLA-4 blockade-induced colitis.Citation31 Of note, oral gavage of a mixture of four gram-positive Bifidobacterium species could ameliorate the immunopathology associated with CTLA-4 blockade by upregulating T cell-mediated metabolic processing, thereby rescuing mice from vancomycin-induced dysbiosis.
PD-1/PD-L1 blockade
In 2015 Sivan et al. compared the growth of subcutaneous melanoma between C57BL/6 mice obtained from two vendors (JAX and TAC) which are known to differ in their commensal microbes.Citation25 They revealed that tumors in TAC mice grow faster and are less sensitive to anti-PD-L1 antibodies compared with JAX mice, and these differences were associated with lower intratumoral infiltration of Cd8 + T cells and weaker tumor-specific T cell response. When cohousing with JAX mice or administering feces from JAX mice, TAC mice acquired the phenotypes as observed in JAX mice with improved responsiveness to PD-L1 blockade to an extent similar to anti-PD-1-treated JAX mice. The 16S rRNA sequencing on fecal samples of JAX-fed TAC mice identified that Bifidobacterium species including Bifidobacterium adolescentis, Bifidobacterium breve, and Bifidobacterium longum showed the largest increase in abundance and strongest association with anticancer T cell response. Of note, oral gavage of a commercially available cocktail of Bifidobacterium spp. involving B. breve and B. longum to TAC mice was sufficient to improve dendritic cell-mediated immune responses (e.g. increased level of interferon-γ, accumulation of peripheral tumor-specific T cells, and intratumoral Cd8 + T cells), leading to ameliorated tumor control to the same extent as anti-PD-L1 antibodies, whereas combining both treatments almost abolished all tumor growth. Collectively, these data indicates that commensal Bifidobacterium could influence host anticancer immunity, thereby enhancing the efficacy of PD-1/PD-L1 blockade.
Gut microbiota and immune checkpoint blockade – microbial profiling studies
Numerous studies have utilized next-generation sequencing to investigate the correlation between gut microbiota and therapeutic response in patients treated with PD-1/PD-L1 blockade by comparing the diversity and composition of fecal microbiota in responders with nonresponders. Bacterial species enriched in responders and their corresponding mechanisms are listed in . Some of these species include B. longum, Collinsella aerofaciens, and Enterococcus faecium in metastatic melanoma ;Citation26,Citation29 and B. longum, Akkermansia muciniphila, and Prevotella corpri in NSCLC and RCC.Citation28,Citation30 To establish a cause–effect relationship between commensals and blockade efficacy, feces from responding patients were transplanted into tumor-bearing mice with microbiota depleted by either antibiotics or housing in germ-free condition.Citation26,Citation29,Citation30 The efficacy of PD-1/PD-L1 blockade was ameliorated in these recipient mice in relation to enhanced T cell response and anticancer immunity, whilst transplantation of feces from nonresponders failed to do so. Notably, administration of A. muciniphila to microbiota-depleted mice with fecal transplantation from nonresponders stimulated Il-12-dependent infiltration of Ccr-9+ Cxcr-3+ Cd4 + T cells into tumor beds, resulting in the restoration of the anticancer effect of PD-1 blockade.Citation30
In comparison, there were much fewer investigations on how the gut commensals influence response to ICI therapy in patients with gastrointestinal cancers. Zheng et al. prospectively analyzed the fecal samples of HCC patients receiving an anti-PD-1 drug camrelizumab.Citation27 Before treatment, the fecal microbiota in both responders and nonresponders was dominated by Bacteroidetes following by Firmicutes and Proteobacteria, which are in accordance with findings in healthy adults.Citation1 When treatment proceeded, Proteobacteria species including Escherichia coli and Klebsiella pneumoniae markedly increased in nonresponders, while microbial composition in responders remained stable. Subsequent analysis identified 20 significantly enriched species in responders and their associated functional pathways, including Bifidobacterium dentium, correlated with anti-inflammatory cellulose metabolism; four Lactobacillus species, correlated with reducing oxidative stress injury; and two Ruminococcaceae species and A. muciniphila, which were reported capable of improving anti-PD-1/PD-L1 efficacy,Citation29,Citation30 were correlated with multiple critical metabolisms. Overall, these findings illustrate that the gut commensals are closely related to patient responsiveness to ICI therapy. Targeting or modulating the microbiota to manipulate its composition could therefore be a potential clinical strategy to enhance therapeutic response. It is noteworthy that to date there is insufficient profiling work on revealing the correlation between microbiota and efficacy of joint-ICI immunotherapy.
Gut microbiota and immune checkpoint blockade – clinical studies
Antibiotic treatment
It is common for clinicians to prescribe broad-spectrum antibiotics to patients with prolonged immunosuppression to prevent opportunistic infections, and patients with immune checkpoint blockade-induced diarrhea accompanied by fever or leukocytosis.Citation72,Citation73 However, as antibiotics are well known to cause compositional alteration in the gut microbiota, several clinical trials were conducted to depict the impact of antibiotics on patients receiving ICI therapy. Derosa et al. performed the largest independent retrospective study by far to assess the effect of antibiotic treatment prior to the first dose of the anti-PD-1/PD-L1 drug in 360 patients with RCC (n = 121) or NSCLC (n = 139).Citation74 Shorter progression-free survival (PFS) and overall survival was observed in RCC patients exposed to antibiotic 30 or 60 d as well as NSCLC patients exposed to antibiotics 30 d before treatment initiation. Whereas in the retrospective study of Sen et al. involving 172 patients with RCC, NSCLC, melanoma, sarcoma, and gastrointestinal stromal tumors, antibiotics were applied to patients during (n = 54), 30 (n = 19) or 60 d (n = 14) prior to PD-1 and/or CTLA-4 blockade.Citation75 Contrastingly, neither PFS nor overall survival showed any difference between antibiotic-treated and untreated patients at all time points, except that a decrease in overall survival was observed in patients with the use of antibiotics 30 d before treatment. Similar results were obtained in another retrospective study involving 161 patients with gastroesophageal cancer.Citation76 To elucidate the heterogeneity among studies, Huang et al. conducted a dedicated meta-analysis to pool all data from 19 relevant publications comprising a total of 2,740 cancer patients.Citation77 Statistically significant reduction in PFS and overall survival were observed when comparing blockade-treated patients with the use of antibiotics to those without regardless of the cancer type, thus indicating the negative association between antibiotics and efficacy of ICI treatment.
Further evaluation on how the initiation time of antibiotic treatment impact patient outcomes was done by Pinato et al.Citation78 Broad-spectrum antibiotics were given to 29 and 68 patients (malignant melanoma or NSCLC) 30 d before or during anti-PD-1/PD-L1 therapy, respectively. Only pretreatment use of antibiotics but not concurrent use was associated with worse overall survival and a higher risk of primary disease refractory, suggesting that antibiotic application is still safe for patients who are undergoing ICI therapy. Nevertheless, these clinical work has revealed the proof-of-concept relationship between microbiota and antibiotics as demonstrated preclinically. Although the result has been controversial, it is obvious that antibiotics have no significant benefits or even worsens treatment responsiveness. Extensive work is thus required to ensure the safety and necessity before prescribing antibiotics to patients who would receive immune checkpoint blockade.
Blockade-induced adverse events
ICI therapy could induce various irAEs such as colitis and hepatitis in the gastrointestinal tract.Citation63,Citation64 A prospective study in 2016 identified an increased fecal abundance of three Bacteroidetes families (Bacteroidaceae, Barnesiellaceae, and Rikenellaceae) in 24 ipilimumab-treated melanoma patients who did not develop blockade-induced colitis.Citation32 Later in 2019, a similar study also reported the enriched fecal abundance of Bacteroides and Parabacteroides from phylum Bacteroidetes in 18 pembrolizumab-treated lung cancer patients without the development of blockade-induced diarrhea.Citation79 In a retrospective study involving 826 patients with ICI therapy, the use of antibiotics no matter before or after treatment was associated with reduced occurrence and recurrence of irAEs.Citation80 Yet additional hospitalization and immunosuppressive treatment (e.g. corticosteroid supplementation) were more often needed for those receiving antibiotics after ICI therapy. Of note, antibiotics administrated at the onset of irAEs were correlated with enhanced severity and recurrence of irAEs. These clinical data thus illustrates that alteration in the microbial profile is associated with irAE incidence in patients treated with immune checkpoint blockade. Although antibiotics seem to be effective in preventing the onset of irAEs, depleted microbiota could meanwhile increase the occurrence of more severe irAEs.
In 2017 Chaput et al. accessed the composition of fecal microbiota at baseline and during ipilimumab treatment in a prospective cohort with 26 metastatic melanoma patients.Citation24 Patients whose baseline microbiota enriched with Ruminococcus and Faecalibacterium had longer PFS and overall survival, whilst the high proportion of Bacteroides was present in patients with poorer clinical benefits. Contrastingly patients with baseline enrichment of Firmicutes species (e.g. Clostridiales bacterium and Faecalibacterium prausnitzii) were more likely to develop blockade-induced colitis, while the fecal microbiota in colitis-free patients was dominated by Bacteroidetes (e.g. Prevotella and Bacteroides uniformis), which is in accordance with previous findings.Citation32,Citation79 Low baseline levels of systemic pro-inflammatory cytokines, CD4+, and CD8 + T cells, and a substantial increase in CD4 + T cells upon treatment initiation were observed in patients with Faecalibacterium-dominant fecal microbiota who showed long-term clinical benefits but a higher incidence of colitis. For patients with Bacteroides-dominant fecal microbiota who had a lower occurrence of colitis but instead with poorer therapeutic response, a much higher proportion of CD4 + T cells at baseline and no enhanced T cell induction after treatment start was observed, thus indicating that the microbial profile at baseline could predict patient outcome and toxicity to ICI therapy. Taken together, these findings demonstrate the correlation between microbiota and immune checkpoint blockade as well as its related adverse events. Given that modulating the commensals directly to ameliorate treatment efficacy is yet to show the convincing results in practice, targeting the microbiota composition and utilizing it as a prediction tool for the patient outcome may instead yield a promising direction.
Gut microbiota and CpG-oligodeoxynucleotide therapy
Unmethylated CpG dinucleotide motif originally exists in a bacterial genome. It is a MAMP that could trigger the host immunity to initiate TLR9/IL-1 R-mediated signaling cascade, leading to upregulation of pro-inflammatory cytokines, and activation of IRF and NF-κB downstream pathway.Citation81,Citation82 Synthetic oligodeoxynucleotides (ODNs) comprising of CpG motifs similar to those naturally found in bacteria but with less toxicity have been developed, and these CpG-ODNs could be recognized by myeloid and dendritic cells to stimulate immune activation.Citation83,Citation84 Several CpG-ODNs especially CpG-7909 has been applied in clinical trials.Citation85 However, unimpressive results were often revealed as monotherapy seemed to be insufficient to induce robust anticancer effect, which could be explained by distinct expressing patterns of TLR9 among patients, and overshadowing the immuno-stimulatory effect of CpG-ODNs by the immunosuppressive tumor microenvironment.Citation86 Meanwhile, large efforts have been invested to apply CpG-ODNs as an adjuvant of other treatments including chemotherapy and immunotherapy.Citation86,Citation87
Preclinically, intratumoural or peritumoral injection of CpG-ODNs plus ICIs increased the circulating levels and tumoral infiltration of effector Cd8 + T cells, thereby prolonging the survival of tumor-bearing blockade-resistant mice.Citation88,Citation89 In the study of Wang et al., two mouse models that mimic anti-PD-1-resistance as inpatients were developed.Citation90 A synergistic effect was observed when CpG-ODN SD-101 plus anti-PD-1 antibodies were injected intratumorally SD-101 altered TIM by promoting T cell infiltration and generation of multifunctional Cd8 + T cells, and subsequent PD-1 blockade led to further expansion of CpG-induced Cd8 + T cells differentiating into short-lived effector cells and long-lived memory precursors. These results thus provide a rationale for proceeding into trials with the use of this innovative CpG-ODN-immune checkpoint blockade-combined strategy.Citation86
In germ-free mice or antibiotic-treated mice, injection of CpG-ODNs and anti-IL-10 R antibodies failed to reduce subcutaneous tumor growth with shortened survival when compared to those without microbiota depletion.Citation33 Reduced secretion of pro-inflammatory cytokines (Il-1α, Il-1β) and lowered Cd45+ TILs-produced Tnf was occurred in these microbiota-depleted mice after injection. Ineffective treatment was also observed in Tlr4−/- mice, whilst the administration of Tlr4 agonist LPS could restore the responsiveness of myeloid cells toward treatment in wild-type mice with impaired microbiota. This indicates that gut microbiota could prime tumor-associated myeloid cells through Tlr4 activation to provoke Tlr9-dependent immune response upon CpG-ODN injection. Several fecal bacteria were correlated with CpG-ODN efficacy, of which gram-negative (e.g. Ruminococcus and Alistipes shahii) and gram-positive (e.g. Lactobacillus fermentum, Lactobacillus intestinalis, and Lactobacillus murinum) genera were positively and negatively correlated to CpG-ODN-induced Tnf production, respectively. Notably, when administrating A. shahii to microbiota-depleted CpG-ODN-treated mice, the ability of tumor-associated myeloid cells to produce Tnf was restored. In contrast, oral gavage of L. fermentum (well-established anti-inflammatory speciesCitation91,Citation92) attenuated anticancer response to CpG-ODNs in mice pretreated with antibiotics. Altogether it is now clear that the efficacy of CpG ODNs or other immunotherapies is closely related to the commensal microbiota. As different microbes could lead to opposing therapeutic responses, altering the microbial community by clinical interventions such as probiotics and FMT could be a feasible approach to further ameliorate the anticancer effect of cancer treatments.
Targeting gut microbiota as adjuvants of immunotherapy
Given its therapeutic potential, growing interest in targeting the gut microbiota to alleviate dysbiosis or associated inflammation, and utilizing it as adjuvants of immunotherapy have been arisen (relevant ongoing clinical trials are listed in ). Here we discuss three strategies involving dietary intervention, probiotics and FMT that aim to alter the microbial community to contribute greater clinical benefits to patients treated with immunotherapy, which is summarized in .
Table 3. Ongoing clinical studies on the role of microbiota in gastrointestinal cancer.a.
Figure 2. Targeting gut microbiota as adjuvants of cancer immunotherapy. Dietary intervention, probiotics and FMT are microbiota-targeted strategies that can ameliorate the efficacy of immunotherapy in 4 distinct mechanisms. a | As the microbiota composition is easily affected, utilizing these extrinsic strategies can restore the imbalanced microbial community to alleviate dysbiosis-associated pathology. b | The anticancer immunity in tumor microenvironment is inhibited to flavor tumor cell growth. By reconstructing the T cell repertoire, the suppressed host immunity can be provoked once again to fight against cancer. c | A diversity of immune cells (e.g. NK and dendritic cells) infiltrate from the circulation into the tumor to further contribute to killing of cancer cells. d | Apart from direct effects on the tumor, the anticancer immunity is stimulated by these strategies to increase or decrease production of anti-inflammatory or pro-inflammatory cytokines respectively, thereby alleviating persistent inflammation in cancer patients
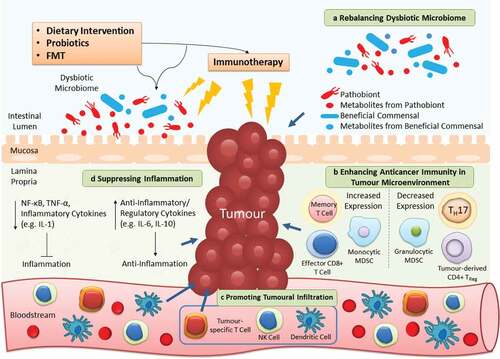
Dietary intervention
Long-term-imbalanced diet has been correlated with cancer, for instance, food intake with insufficient fiber and excessive proteins from red meats is adequate to promote CRC development.Citation93–95 Meanwhile, dietary nutraceuticals can prevent carcinogenesis as reported in thousands of work.Citation93,Citation95,Citation96 Recently utilizing nutraceuticals especially polyphenols (a group of natural plant-derived chemicals) to treat cancer has been emerged. For example, resveratrol in grapes could enhance anticancer immunity in tumor-bearing mice by making TIM unfavorable for tumor growth (e.g. promoting accumulation of effector Cd8 + T cells and monocytic myeloid-derived suppressor cells (MDSCs), and inhibiting the population of tumor-derived Cd4+ Cd25+ TReg and Cd8 + T cell-suppressing granulocytic MDSCsCitation97–99). When providing resveratrol to immunotherapy-treated tumor-bearing mice, complete tumor abolishment and metastasis retardation was observed without causing therapy-induced injury in normal epithelial cells.Citation100,Citation101 To date, there are no trials testing the efficacy of resveratrol-adjuvant immunotherapy, while short-term resveratrol administration (≤14 d) to CRC patients could reduce tumor cell proliferationCitation102 and induce apoptosis (NCT00920803,Citation103). Other polyphenols including flavonoids,Citation104 genistein,Citation105 and pomegranateCitation106 have shown convincing anticancer activities in preclinical but not in clinical studies, which is suggested due to the unrealistic amount of nutraceuticals required for exhibiting some effects in patients.Citation107 For instance, although curcumin is one of the most promising polyphenols as a potential adjuvant of CRC treatment, clinical use is often restricted due to its low water solubility and bioavailability.Citation107,Citation108 Several nano-formulations such as liposomes and micelles are thus developing to improve curcumin delivery into patients.Citation109
A high-fat low-protein/carbohydrate dietary combination known as the ketogenic diet is well established for its neuroprotective effect against several neurological disorders including epilepsy and Alzheimer’s disease.Citation110 Lowered glucose intake in a ketogenic diet can restrict tumor cell metabolism without affecting normal cells, as tumor cells rely on glucose as an energy source and are unable to metabolize ketone bodies.Citation110 Whereas normal cells can metabolize both; thus, avoiding tumors to obtain sufficient energy. A ketogenic diet can also influence the host immunity: it suppresses lactate production by glycolytic tumors, leading to the enhanced anticancer immune response by inhibiting lactate-mediated tumoral immunosuppression and MDSC expression in tumor-bearing mice.Citation111 To date, ketogenic diet intervention has shown varied anticancer efficacy in clinical trials,Citation112,Citation113 whereas combination with chemotherapy and radiotherapy has yielded more convincing results.Citation110 While the efficacy of combining a ketogenic diet with immunotherapy is yet to be determined.
Probiotics
Introducing exogenous probiotics with functional colonization can reward health benefits as exemplified by using probiotics to treat IBD.Citation114,Citation115 Regarding to cancer, apart from the well-known Lactobacillus rhamnosus GG which can inhibit expressions of inflammatory proteins NF-κB-p65, COX-2, and TNF-α to reduce colon tumor incidence,Citation116,Citation117 some Lactobacillus species also display immunomodulatory features to suppress carcinogenesis. Lactobacillus casei BL23 could improve immune response by reducing TReg level in mice with colitis-associated cancer;Citation118 and Lactobacillus plantarum prolonged survival of tumor-bearing mice by enhancing effector Cd8 + T cells functions, Cd4 + T cells differentiation, and NK cells intratumoural infiltration.Citation119 In a trial probiotics (Lactobacillus acidophilus NCFM and Bifidobacterium lactis BI-04) were given to 15 CRC patients (NCT03072641,Citation120). Enrichment of butyrate-producing bacteria (e.g. Clostridiales and Faecalibacterium) in both tumor and nontumor colonic mucosa, and reduction of CRC-associated genera including Fusobacterium and Peptostreptococcus in the fecal microbiota was identified. These findings reveal the capability of probiotics to relieve dysbiosis and ameliorate anticancer immunity, yet it remains elusive how such alteration in the microbial profile can benefit cancer patients.
Uprising interest in coupling cancer immunotherapy with probiotics has been emerged. In 2019 Zhuo et al. reported that administration of L. acidophilus lysates to carcinogen-treated mice partially restored CRC-associated dysbiosis (e.g. enrichment of Proteobacteria) and improved anti-CTLA-4 efficacy, which is attributed to decreased intratumoural populations of Cd4+ Cd25+ Foxp3+ TReg, and increased effector Cd8+ and memory T cells.Citation121 In other studies, oral gavage of four Bifidobacterium species or Lactobacillus reuteri could abrogate the onset of blockade-induced colitis via promoting T cell-mediated metabolismCitation31 or lowering expression of group 3 innate lymphocytesCitation122 respectively. E. coli Nissle 1917 supplementation to tumor-bearing mice also enhanced tumor-specific T cell infiltration and dendritic cell activation to relieve the immunosuppressive tumor microenvironment, thereby ameliorating the efficacy of galunisertib (an immunotherapy drug for transforming growth factor-β blockade but previously displayed poor clinical results).Citation123 Nevertheless, although probiotics have been widely popularized in the general public, there are conflicting clinical results for many probiotic strains and formulations with inadequate understandings about their impacts on host and interactions with the commensal microbiota.Citation124
Fecal microbiota transplantation
FMT is a therapy to deliver feces from healthy donors into the gastrointestinal tract of receivers via colonoscopy or oral administration to cure diseases by restoring the balance and functions of gut microbiota. FMT has been widely applied to treat recurrent Clostridium difficile infection with incredibly high response rates (≥90%),Citation125,Citation126 and it also shows its therapeutic potential against graft-versus-host disease, neuropsychiatric (e.g. depression and Parkinson’s disease) or other gut disorders (e.g. IBD and ulcerative colitis).Citation127,Citation128 Whereas to date evidence on using FMT to treat gastrointestinal cancer is vastly limited. In the study of Wong et al., the transfer of fecal samples from CRC patients into carcinogen-treated microbiota-depleted mice resulted in increased intestinal carcinogenesis,Citation129 yet whether acquiring feces from healthy individuals could suppress CRC progression requires testing. Additionally, restoration of gut microbial diversity (e.g. enrichment of Lactobacillus and butyrate-producing taxa) and decrease in intrahepatic lipid accumulation were observed in mice with high-fat-diet-induced steatohepatitis after acquiring feces from healthy mice, suggesting that FMT could mitigate the onset of nonalcoholic fatty liver disease-related HCC.Citation130
Similarly, coupling FMT with immunotherapy is mainly under preclinical investigation. Transplanting feces from responders of anti-PD-1 treatment into tumor-bearing mice with depleted microbiota could ameliorate PD-1/PD-L1 blockade efficacy.Citation26,Citation29,Citation30 Such improvement is attributed to altered T cell repertoire: enrichment of Cd45+ and effector Cd8 + T cells, and reduction of Cd11b+Cd11c+ MDSCs, RORγt+ TH17, and Cd4+ Foxp3+, and Cd4+ Il-17+ TReg, which is in line with clinical findings.Citation29 In 2018 FMT was pioneering employed to treat two patients with steroid- and immunosuppressive-refractory ICI blockade-induced colitis (NCT1928394 for the first case and NCT02113657 for the second caseCitation131). Both patients experienced complete remission upon ≤2 times of FMT with reduced inflammation and increased CD4+ FoxP3+ TReg-to-effector CD8 + T cells ratio in colonic mucosa. Microbial community reconstruction was observed in both patients with a notable enrichment of Bifidobacterium, which was previously illustrated its ability to abrogate CTLA-4-blockade-induced colitis in miceCitation31. Currently, there is one ongoing early-phase 1 trial to test whether FMT can improve immunotherapy efficacy in anti-PD-1-resistant/refractory gastrointestinal cancer patients (NCT04130763; ). Overall FMT has an excellent safety profile in treating nonmalignant disease even in immunocompromised patients.Citation132,Citation133 As for cancer, in 2019 Wardill et al. described the current limitations on utilizing FMT as supportive cancer therapy involving highly varied definition and delivery methods across the globe.Citation134 More importantly, it is impossible to standardize the approach due to the difficulty in defining “healthy” microbiota; thus, the risk of disease transmission should never be neglected, and perhaps transplantation of known beneficial microbes or probiotics would be a better alternative to FMT.
Current limitations and future directions
The popularization of next-generation sequencing has brought a tremendous breakthrough in deciphering the features of human gut microbiota in health and cancer states. Subsequent preclinical investigations have evaluated the mechanistic link between microbes and host immunity in carcinogenesis as exemplified by the discovery of F. nucleatum enrichment in metagenomic studies,Citation135 following by animal work to illustrate its immunosuppressive feature to promote CRC.Citation136,Citation137 Numerous current findings have displayed that microbes can affect anticancer immunity. Yet, these works mostly focuses on elucidating the role of specific species instead of a microbial community in the cancer-immunity crosstalk. To date how the altered microbiota as observed in gastrointestinal cancer patients is correlated with the suppressed immune system is vastly unknown, and in fact this is in line with one of the key limitations in the majority of microbial profiling publications – descriptive findings are mostly provided without in-depth explanation. Indeed, it is now generally accepted that microbiota in cancer patients is distinct from noncancer individuals, but the mechanistic correlation between such compositional difference and cancer progression remains massively unclear. Hence, more efforts are suggested to link sequencing data with the extensive investigation when conducting microbiota research. For example, an ongoing clinical trial aims to determine the association of gut microbiota with neutrophils intratumoral infiltration in CRC (NCT03841799; ).
There are several more unaddressed issues regarding to the study on gut microbiota. For instance, Walter et al. in 2020 reported the unrealistic high rate of pathologies being transferred from humans to rodents among publications (95%) and expressed their concerns as these findings may overstate the roles of microbiota in human diseases.Citation138 They, therefore, recommended that a more rigorous experimental approach is required worldwide to avoid false concepts. In 2019 the International Cancer Microbiome Consortium (ICMC) released an expert consensus on the role of the microbiome in cancer initiation with the highlight of five pivotal questions (description in ) and suggesting the necessity of conducting large interventional cohort studies with integrative analysis with other oncological research projects to comprehensively reveal the microbe-associated cancer-related pathologies (e.g. genotoxicity, suppressed immunity, and altered metabolism).Citation139
Table 4. Summary of the consensus statement of the International Cancer Microbiome Consortium.Citation139.
It is now clear that gut microbiota has an unneglectable role in influencing cancer immunotherapy. Such discovery has provided solid fundamentals to ameliorate treatment efficacy by modulating the microbial profile in patients via distinct approaches such as probiotic supplementation and FMT. Yet again, the lack of understanding of the mechanistic crosstalk between host immunity and specific microbes or overall microbial community has limited the progress of the clinical investigation. To increase the translational potential of microbiota-targeted therapies, ICMC pinpointed the importance of formulating standardized guidelines with enhanced transparency to facilitate reproducibility when presenting “meta-omics” data in the academia.Citation139 Additionally, combining microbiota research with other novel technologies may offer new findings to connect with scientific areas that have been well studied. For example, the patient-derived organoid model is a newly developed and robust in vitro system, which has shown great potential in predicting treatment outcomes due to the complete preservation of phenotypes and genotypes of their originated tumors.Citation141 A study in 2020 performed whole-genome sequencing on human CRC organoids with exposure to genotoxic pks+ E. coli to reveal its related oncogenic mutational signatures, thus illustrating the use of organoids to depict the microbe-associated carcinogenesis at the genomic level.Citation142
Furthermore, it has been increasingly documented that metabolites (referring to the intermediate end products during microbe-mediated metabolism) have distinct roles in cancer progression. For example, a class of metabolites known as bile acids has demonstrated its cancer-promoting properties under dysbiotic condition.Citation143 Alterations in microbiota composition could increase the level of deoxycholic acid (a type of bile acid known for causing DNA damageCitation144) in the enterohepatic circulation, leading to disruption in the gut barrier and further promoting intestinalCitation145 or liver carcinogenesis.Citation146 Of note, evidence on how metabolites could influence immunotherapy had been insufficient until recently, by which a study in 2020 identified that a metabolite called inosine (produced by Bifidobacterium pseudolongum) could enhance response to immune checkpoint blockade in mice through activating anticancer T cells.Citation147 In addition to bacteria, accumulating publications have displayed the importance of gut viral, fungal, and archaeal microbiota in gastrointestinal cancers including CRC.Citation148–150 Yet, it is noteworthy that to date there is a lack of investigation on their roles in cancer treatment, and hence future work (e.g. shotgun metagenomic sequencing, which allows cross-domain profiling with greater taxonomy resolution and genomic coverage than conventional 16S rRNA sequencing) is suggested to evaluate whether nonbacterial microbiota can also affect the efficacy of immunotherapy.
Conclusion
Coupling the modern sequencing technology with the mechanistic investigation has aided the identification of novel interaction between the host immunity and microbial community in health and cancer states. Here we highlight the importance of gut commensals on modulating the efficacy of cancer immunotherapy. Notably, strategies that target microbiota as a cancer monotherapy or an adjuvant of first-line treatment have now been heavily studied. In summary, even though the knowledge gap between gut microbiota and gastrointestinal cancer is narrowing, enormous efforts are undoubtedly needed to fully unravel the underlying mechanisms, thereby potentiating the application of microbiota-targeted therapies in clinical practice.
Abbreviations
Disclosure of interest
The authors report no conflict of interest.
Author contributions
HCHL researched data for the article, contributed substantially to the discussion of content, designed the figures, and wrote the manuscript. JJYS reviewed/edited the manuscript before submission. JY made a substantial contribution to the discussion of content, edited the figures, and reviewed/edited the manuscript before submission. All authors approved the final version of the manuscript.
Supplemental Material
Download MS Word (41.3 KB)Acknowledgments
Thanks to Hye Won Lee from the Department of Internal Medicine, Institute of Gastroenterology, Yonsei University College of Medicine, Korea, for her opinions on the clinical aspects.
Supplementary material
Supplemental data for this article can be accessed on the publisher’s website.
References
- Human Microbiome Project Consortium. Structure, function and diversity of the healthy human microbiome. Nature. 2012;486(7402):207–21. doi:10.1038/nature11234.
- Lama SY, Yu J, Wong SH, Peppelenboscha MP, Fuhler GM. The gastrointestinal microbiota and its role in oncogenesis. Best Pract Res Clin Gastroenterol. 2017;31(6):607–618. doi:10.1016/j.bpg.2017.09.010.
- Lozupone CA, Stombaugh J, Gordon J, Jansson JK, Knight R. Diversity, stability and resilience of the human gut microbiota. Nature. 2012;489(7415):220–230. doi:10.1038/nature11550.
- Lloyd-Price J, Mahurkar A, Rahnavard G, Crabtree J, Orvis J, Hall AB, Brady A, Creasy HH, McCracken C, Giglio MG, et al. Strains, functions and dynamics in the expanded Human Microbiome Project. Nature. 2017;550(7674):61–66. doi:10.1038/nature23889.
- Round JL, Mazmanian SK. The gut microbiota shapes intestinal immune responses during health and disease. Nat Rev Immunol. 2009;9(5):313–323. doi:10.1038/nri2515.
- Fransen F, Zagato E, Mazzini E, Fosso B, Manzari C, El Aidy S, Chiavelli A, D’Erchia AM, Sethi MK, Pabst O, et al. BALB/c and C57BL/6 mice differ in polyreactive iga abundance, which impacts the generation of antigen-specific iga and microbiota diversity. Immunity. 2015;43(3):527–540. doi:10.1016/j.immuni.2015.08.011.
- Bunker JJ, Erickson SA, Flynn TM, Henry C, Koval JC, Meisel M, Jabri B, Antonopoulos DA, Wilson PC, Bendelac A. Natural polyreactive IgA antibodies coat the intestinal microbiota. Science. 2017;358(6361):6619. doi:10.1126/science.aan6619.
- Nakajima A, Vogelzang A, Maruya M, Miyajima M, Murata M, Son A, Kuwahara T, Tsuruyama T, Yamada S, Matsuura M, et al. IgA regulates the composition and metabolic function of gut microbiota by promoting symbiosis between bacteria. J Exp Med. 2018;215(8):2019–2034. doi:10.1084/jem.20180427.
- Le Chatelier E, Nielsen T, Qin J, Prifti E, Hildebrand F, Falony G, Almeida M, Arumugam M, Batto JM, Kennedy S, et al. Richness of human gut microbiome correlates with metabolic markers. Nature. 2013;500(7464):541–546. doi:10.1038/nature12506.
- Cotillard A, Kennedy SP, Kong LC, Prifti E, Pons N, Le Chatelier E, Almeida M, Quinquis B, Levenez F, Galleron N, et al. Dietary intervention impact on gut microbial gene richness. Nature. 2013;500(7464):585–588. doi:10.1038/nature12480.
- Rothschild D, Weissbrod O, Barkan E, Kurilshikov A, Korem T, Zeevi D, Costea PI, Godneva A, Kalka IN, Bar N, et al. Environment dominates over host genetics in shaping human gut microbiota. Nature. 2018;555(7695):210–215. doi:10.1038/nature25973.
- Levy M, Kolodziejczyk AA, Thaiss CA, Elinav E. Dysbiosis and the immune system. Nat Rev Immunol. 2017;17(4):219–232. doi:10.1038/nri.2017.7.
- Lloyd-Price J, Abu-Ali G, Huttenhower C. The healthy human microbiome. Genome Med. 2016;8(1):51. doi:10.1186/s13073-016-0307-y.
- Wong SH, Yu J. Gut microbiota in colorectal cancer: mechanisms of action and clinical applications. Nat Rev Gastroenterol Hepatol. 2019;16:690–704.
- Zeng MY, Inohara N, Nuñez G. Mechanisms of inflammation-driven bacterial dysbiosis in the gut. Mucosal Immunol. 2017;10(1):18–26. doi:10.1038/mi.2016.75.
- Chen J, Pitmon E, Wang K. Microbiome, inflammation and colorectal cancer. Semin Immunol. 2017;32:43–53. doi:10.1016/j.smim.2017.09.006.
- Alexander JL, Wilson ID, Teare J, Marchesi JR, Nicholson JK, Kinross JM. Gut microbiota modulation of chemotherapy efficacy and toxicity. Nat Rev Gastroenterol Hepatol. 2017;14(6):356–365. doi:10.1038/nrgastro.2017.20.
- Holohan C, Van Schaeybroeck S, Longley DB, Johnston PG. Cancer drug resistance: an evolving paradigm. Nat Rev Cancer. 2013;13(10):714–726. doi:10.1038/nrc3599.
- Roy S, Trinchieri G. Microbiota: a key orchestrator of cancer therapy. Nat Rev Cancer. 2017;17:271–285.
- Helmink BA, Khan MAW, Hermann A, Gopalakrishnan V, Wargo JA. The microbiome, cancer, and cancer therapy. Nat Med. 2019;25(3):377–388. doi:10.1038/s41591-019-0377-7.
- Uribe-Herranz M, Bittinger K, Rafail S, Guedan S, Pierini S, Tanes C, Ganetsky A, Morgan MA, Gill S, Tanyi JL, et al. Gut microbiota modulates adoptive cell therapy via CD8α dendritic cells and IL-12. JCI Insight. 2018;3(4):94952. doi:10.1172/jci.insight.94952.
- Paulos CM, Wrzesinski C, Kaiser A, Hinrichs CS, Chieppa M, Cassard L, Palmer DC, Boni A, Muranski P, Yu Z, et al. Microbial translocation augments the function of adoptively transferred self/tumor-specific CD8+ T cells via TLR4 signaling. J Clin Invest. 2007;117(8):2197–2204. doi:10.1172/JCI32205.
- Vétizou M, Pitt JM, Daillère R, Lepage P, Waldschmitt N, Flament C, Rusakiewicz S, Routy B, Roberti MP, Duong CP, et al. Anticancer immunotherapy by CTLA-4 blockade relies on the gut microbiota. Science. 2015;350(6264):1079–1084. doi:10.1126/science.aad1329.
- Chaput N, Lepage P, Coutzac C, Soularue E, Le Roux K, Monot C, Boselli L, Routier E, Cassard L, Collins M, et al. Baseline gut microbiota predicts clinical response and colitis in metastatic melanoma patients treated with ipilimumab. Ann Oncol. 2017;28(6):1368–1379. doi:10.1093/annonc/mdx108.
- Sivan A, Corrales L, Hubert N, Williams JB, Aquino-Michaels K, Earley ZM, Benyamin FW, Lei YM, Jabri B, Alegre ML, et al. Commensal Bifidobacterium promotes antitumor immunity and facilitates anti-PD-L1 efficacy. Science. 2015;350(6264):1084–1109. doi:10.1126/science.aac4255.
- Matson V, Fessler J, Bao R, Chongsuwat T, Zha Y, Alegre ML, Luke JJ, Gajewski TF. The commensal microbiome is associated with anti-PD-1 efficacy in metastatic melanoma patients. Science. 2018;359(6371):104–108. doi:10.1126/science.aao3290.
- Zheng Y, Wang T, Tu X, Huang Y, Zhang H, Tan D, Jiang W, Cai S, Zhao P, Song R, et al. Gut microbiome affects the response to anti-PD-1 immunotherapy in patients with hepatocellular carcinoma. J Immunother Cancer. 2019;7(1):193. doi:10.1186/s40425-019-0650-9.
- Jin Y, Dong H, Xia L, Yang Y, Zhu Y, Shen Y, Zheng H, Yao C, Wang Y, Lu S. The diversity of gut microbiome is associated with favorable responses to anti-programmed death 1 immunotherapy in chinese patients with NSCLC. J Thorac Oncol. 2019;14(8):1378–1389. doi:10.1016/j.jtho.2019.04.007.
- Gopalakrishnan V, Spencer CN, Nezi L, Reuben A, Andrews MC, Karpinets TV, Prieto PA, Vicente D, Hoffman K, Wei SC. Gut microbiome modulates response to anti-PD-1 immunotherapy in melanoma patients. Science. 2018;359(6371):97–103. doi:10.1126/science.aan4236.
- Routy B, Le Chatelier E, Derosa L, Duong CPM, Alou MT, Daillère R, Fluckiger A, Messaoudene M, Rauber C, Roberti MP, et al. Gut microbiome influences efficacy of PD-1-based immunotherapy against epithelial tumors. Science. 2018;359(6371):91–97. doi:10.1126/science.aan3706.
- Wang F, Yin Q, Chen L, Davis MM. Bifidobacterium can mitigate intestinal immunopathology in the context of CTLA-4 blockade. Proc Natl Acad Sci U S A. 2018;115(1):157–161. doi:10.1073/pnas.1712901115.
- Dubin K, Callahan MK, Ren B, Khanin R, Viale A, Ling L, No D, Gobourne A, Littmann E, Huttenhower C, et al. Intestinal microbiome analyses identify melanoma patients at risk for checkpoint-blockade-induced colitis. Nat Commun. 2016;7:10391.
- Iida N, Dzutsev A, Stewart CA, Smith L, Bouladoux N, Weingarten RA, Molina DA, Salcedo R, Back T, Cramer S, et al. Commensal bacteria control cancer response to therapy by modulating the tumor microenvironment. Science. 2013;342(6161):967–970. doi:10.1126/science.1240527.
- Zhang R, Zhang Z, Liu Z, Wei D, Wu X, Bian H, Chen Z. Adoptive cell transfer therapy for hepatocellular carcinoma. Front Med. 2019;13(1):3–11. doi:10.1007/s11684-019-0684-x.
- Christofi T, Baritaki S, Falzone L, Libra M, Zaravinos A. Current Perspectives in Cancer Immunotherapy. Cancers (Basel). 2019;11(10):E1472. doi:10.3390/cancers11101472.
- Coscia M, Vitale C, Cerrano M, Maffini E, Giaccone L, Boccadoro M, Bruno B. Adoptive immunotherapy with CAR modified T cells in cancer: current landscape and future perspectives. Front Biosci (Landmark Ed). 2019;24(7):1284–1315. doi:10.2741/4780.
- Rosenberg SA, Restifo NP. Adoptive cell transfer as personalized immunotherapy for human cancer. Science. 2015;348(6230):62–68. doi:10.1126/science.aaa4967.
- Gross G, Eshhar Z. Therapeutic potential of T cell chimeric antigen receptors (CARs) in cancer treatment: counteracting off-tumor toxicities for safe CAR T cell therapy. Annu Rev Pharmacol Toxicol. 2016;56(1):59–83. doi:10.1146/annurev-pharmtox-010814-124844.
- Holstein SA, Lunning MACAR. T-cell therapy in hematologic malignancies: a voyage in progress. Clin Pharmacol Ther. 2019;107(1):112–122. doi:10.1002/cpt.1674.
- Merhavi-Shoham E, Itzhaki O, Markel G, Schachter J, Besser MJ. Adoptive cell therapy for metastatic melanoma. Cancer J. 2017;23(1):48–53. doi:10.1097/PPO.0000000000000240.
- Dafni U, Michielin O, Lluesma SM, Tsourti Z, Polydoropoulou V, Karlis D, Besser MJ, Haanen J, Svane IM, Ohashi PS, et al. Efficacy of adoptive therapy with tumor-infiltrating lymphocytes and recombinant interleukin-2 in advanced cutaneous melanoma: a systematic review and meta-analysis. Ann Oncol. 2019;30(12):1902–1913. doi:10.1093/annonc/mdz398.
- Fan J, Shang D, Han B, Song J, Chen H, Yang JM. Adoptive cell transfer: is it a promising immunotherapy for colorectal cancer? Theranostics. 2018;8(20):5784–5800. doi:10.7150/thno.29035.
- Zhao Q, Yu J, Meng X. A good start of immunotherapy in esophageal cancer. Cancer Med. 2019;8(10):4519–4526. doi:10.1002/cam4.2336.
- Tanaka T, Nakamura J, Noshiro H. Promising immunotherapies for esophageal cancer. Expert Opin Biol Ther. 2017;17(6):723–733. doi:10.1080/14712598.2017.1315404.
- Newick K, O’Brien S, Moon E, Albelda SM, Cell CART. Therapy for solid tumors. Annu Rev Med. 2017;68(1] has been updated.):139–152. doi:10.1146/annurev-med-062315-120245.
- Yeku O, Li X, Brentjens RJ. Adoptive T-cell therapy for solid tumors. Am Soc Clin Oncol Educ Book. 2017;37:193–204. doi:10.14694/EDBK_180328.
- DeRenzo C, Gottschalk S. Genetic modification strategies to enhance CAR T cell persistence for patients with solid tumors. Front Immunol. 2019;10:218. doi:10.3389/fimmu.2019.00218.
- Minn I, Rowe SP, Pomper MG, Enhancing CAR. T-cell therapy through cellular imaging and radiotherapy. Lancet Oncol. 2019;20(8):e443–e451. doi:10.1016/S1470-2045(19)30461-9.
- Kloess S, Kretschmer A, Stahl L, Fricke S, Koehl UCAR. Expressing natural killer cells for cancer retargeting. Transfus Med Hemother. 2019;46(1):4–13. doi:10.1159/000495771.
- Cao J, Kong FH, Liu X, Wang XB. Immunotherapy with dendritic cells and cytokine-induced killer cells for hepatocellular carcinoma: A meta-analysis. World J Gastroenterol. 2019;25(27):3649–3663. doi:10.3748/wjg.v25.i27.3649.
- Liu YL, Yang LX, Zhang F, Tang BS, Zhao LT, Zhu JR, Jin QY, Wang RX, Li YM. Clinical effect and safety of dendritic cell-cytokine-induced killer cell immunotherapy for pancreatic cancer: a systematic review and meta-analysis. Cytotherapy. 2019;21(10):1064–1080. doi:10.1016/j.jcyt.2019.07.006.
- Garofano F, Gonzalez-Carmona MA, Skowasch D, Schmidt-Wolf R, Abramian A, Hauser S, Strassburg CP, Schmidt-Wolf IGH. Clinical trials with combination of cytokine-induced killer cells and dendritic cells for cancer therapy. Int J Mol Sci. 2019;20(17):E4307. doi:10.3390/ijms20174307.
- Brunet JF, Denizot F, Luciani MF, Roux-Dosseto M, Suzan M, Mattei MG, Golstein P. A new member of the immunoglobulin superfamily--CTLA-4. Nature. 1987;328(6127):267–270. doi:10.1038/328267a0.
- Ishida Y, Agata Y, Shibahara K, Honjo T. Induced expression of PD-1, a novel member of the immunoglobulin gene superfamily, upon programmed cell death. Embo J. 1992;11(11):3887–3895. doi:10.1002/j.1460-2075.1992.tb05481.x.
- El-Khoueiry AB, Sangro B, Yau T, Crocenzi TS, Kudo M, Hsu C, Kim TY, Choo SP, Trojan J, Rd WTH, et al. Nivolumab in patients with advanced hepatocellular carcinoma (CheckMate 040): an open-label, non-comparative, phase 1/2 dose escalation and expansion trial. Lancet. 2017;389(10088):2492–2502. doi:10.1016/S0140-6736(17)31046-2.
- Overman MJ, McDermott R, Leach JL, Lonardi S, Lenz HJ, Morse MA, Desai J, Hill A, Axelson M, Moss RA, et al. Nivolumab in patients with metastatic DNA mismatch repair-deficient or microsatellite instability-high colorectal cancer (CheckMate 142): an open-label, multicentre, phase 2 study. Lancet Oncol. 2017;18(9):1182–1191. doi:10.1016/S1470-2045(17)30422-9.
- Fuchs CS, Doi T, Jang RW, Muro K, Satoh T, Machado M, Sun W, Jalal SI, Shah MA, Metges JP, et al. Safety and efficacy of pembrolizumab monotherapy in patients with previously treated advanced gastric and gastroesophageal junction cancer: phase 2 clinical KEYNOTE-059 Trial. JAMA Oncol. 2018;4(5):e180013. doi:10.1001/jamaoncol.2018.0013.
- Shitara K, Özgüroğlu M, Bang YJ, Di Bartolomeo M, Mandalà M, Ryu MH, Fornaro L, Olesiński T, Caglevic C, Chung HC, et al. Pembrolizumab versus paclitaxel for previously treated, advanced gastric or gastro-oesophageal junction cancer (KEYNOTE-061): a randomised, open-label, controlled, phase 3 trial. Lancet. 2018;392(10142):123–133. doi:10.1016/S0140-6736(18)31257-1.
- Kato K, Shah MA, Enzinger P, Bennouna J, Shen L, Adenis A, Sun JM, Cho BC, Özgüroğlu M, Kojima T, et al. KEYNOTE-590: phase III study of first-line chemotherapy with or without pembrolizumab for advanced esophageal cancer. Future Oncol. 2019;15(10):1057–1066. doi:10.2217/fon-2018-0609.
- Zhu AX, Finn RS, Edeline J, Cattan S, Ogasawara S, Palmer D, Verslype C, Zagonel V, Fartoux L, Vogel A, et al. Pembrolizumab in patients with advanced hepatocellular carcinoma previously treated with sorafenib (KEYNOTE-224): a non-randomised, open-label phase 2 trial. Lancet Oncol. 2018;19(7):940–952. doi:10.1016/S1470-2045(18)30351-6.
- O’Neil BH, Wallmark JM, Lorente D, Elez E, Raimbourg J, Gomez-Roca C, Ejadi S, Piha-Paul SA, Stein MN, Abdul Razak AR, et al. Safety and antitumor activity of the anti-PD-1 antibody pembrolizumab in patients with advanced colorectal carcinoma. PLoS One. 2017;12(12):e0189848. doi:10.1371/journal.pone.0189848.
- Overman MJ, Lonardi S, Wong KYM, Lenz HJ, Gelsomino F, Aglietta M, Morse MA, Van Cutsem E, McDermott R, Hill A, et al. Durable clinical benefit with nivolumab plus ipilimumab in DNA mismatch repair-deficient/microsatellite instability-high metastatic colorectal cancer. J Clin Oncol. 2018;36(8):773–779. doi:10.1200/JCO.2017.76.9901.
- Khoja L, Day D, Wei-Wu Chen T, Siu LL, Hansen AR. Tumour- and class-specific patterns of immune-related adverse events of immune checkpoint inhibitors: a systematic review. Ann Oncol. 2017;28(10):2377–2385. doi:10.1093/annonc/mdx286.
- Ji HH, Tang XW, Dong Z, Song L, Jia YT. Adverse event profiles of Anti-CTLA-4 and Anti-PD-1 monoclonal antibodies alone or in combination: analysis of spontaneous reports submitted to FAERS. Clin Drug Investig. 2019;39(3):319–330. doi:10.1007/s40261-018-0735-0.
- Michot JM, Bigenwald C, Champiat S, Collins M, Carbonnel F, Postel-Vinay S, Berdelou A, Varga A, Bahleda R, Hollebecque A, et al. Immune-related adverse events with immune checkpoint blockade: a comprehensive review. Eur J Cancer. 2016;54:139–148. doi:10.1016/j.ejca.2015.11.016.
- Das S, Johnson DB. Immune-related adverse events and anti-tumor efficacy of immune checkpoint inhibitors. J Immunother Cancer. 2019;7(1):306. doi:10.1186/s40425-019-0805-8.
- Curran MA, Montalvo W, Yagita H, Allison JPPD. 1 and CTLA-4 combination blockade expands infiltrating T cells and reduces regulatory T and myeloid cells within B16 melanoma tumors. Proc Natl Acad Sci U S A. 2010;107(9):4275–4280. doi:10.1073/pnas.0915174107.
- Szostak B, Machaj F, Rosik J, Pawlik A. CTLA4 antagonists in phase I and phase II clinical trials, current status and future perspectives for cancer therapy. Expert Opin Investig Drugs. 2019;28(2):149–159. doi:10.1080/13543784.2019.1559297.
- Jiang Y, Chen M, Nie H, Yuan YPD. 1 and PD-L1 in cancer immunotherapy: clinical implications and future considerations. Hum Vaccin Immunother. 2019;15(5):1111–1122. doi:10.1080/21645515.2019.1571892.
- Janjigian YY, Bendell J, Calvo E, Kim JW, Ascierto PA, Sharma P, Ott PA, Peltola K, Jaeger D, Evans J, et al. CheckMate-032 study: efficacy and safety of nivolumab and nivolumab plus ipilimumab in patients with metastatic esophagogastric cancer. J Clin Oncol. 2018;36(28):2836–2844. doi:10.1200/JCO.2017.76.6212.
- Conforti F, Pala L, Bagnardi V, De Pas T, Martinetti M, Viale G, Gelber RD, Goldhirsch A. Cancer immunotherapy efficacy and patients’ sex: a systematic review and meta-analysis. Lancet Oncol. 2018;19(6):737–746. doi:10.1016/S1470-2045(18)30261-4.
- Villadolid J, Amin A. Immune checkpoint inhibitors in clinical practice: update on management of immune-srelated toxicities. Transl Lung Cancer Res. 2015;4(5):560–575. doi:10.3978/j..2218-6751.2015.06.06.
- Champiat S, Lambotte O, Barreau E, Belkhir R, Berdelou A, Carbonnel F, Cauquil C, Chanson P, Collins M, Durrbach A, et al. Management of immune checkpoint blockade dysimmune toxicities: a collaborative position paper. Ann Oncol. 2016;27(4):559–574.
- Derosa L, Hellmann MD, Spaziano M, Halpenny D, Fidelle M, Rizvi H, Long N, Plodkowski AJ, Arbour KC, Chaft JE, et al. Negative association of antibiotics on clinical activity of immune checkpoint inhibitors in patients with advanced renal cell and non-small-cell lung cancer. Ann Oncol. 2018;29(6):1437–1444. doi:10.1093/annonc/mdy103.
- Sen S, Carmagnani Pestana R, Hess K, Viola GM, Subbiah V. Impact of antibiotic use on survival in patients with advanced cancers treated on immune checkpoint inhibitor phase I clinical trials. Ann Oncol. 2018;29(12):2396–2398. doi:10.1093/annonc/mdy453.
- Greally M, Chou JF, Chatila WK, Margolis M, Capanu M, Hechtman JF, Tuvy Y, Kundra R, Daian F, Ladanyi M, et al. Clinical and molecular predictors of response to immune checkpoint inhibitors in patients with advanced esophagogastric cancer. Clin Cancer Res. 2019;25(20):6160–6169. doi:10.1158/1078-0432.CCR-18-3603.
- Huang XZ, Gao P, Song YX, Xu Y, Sun JX, Chen XW, Zhao JH, Wang ZN. Antibiotic use and the efficacy of immune checkpoint inhibitors in cancer patients: a pooled analysis of 2740 cancer patients. Oncoimmunology. 2019;8(12):e1665973. doi:10.1080/2162402X.2019.1665973.
- Pinato DJ, Howlett S, Ottaviani D, Urus H, Patel A, Mineo T, Brock C, Power D, Hatcher O, Falconer A, et al. Association of prior antibiotic treatment with survival and response to immune checkpoint inhibitor therapy in patients with cancer. JAMA Oncol. 2019;5(12):1774–1778.
- Liu T, Xiong Q, Li L, Hu Y. Intestinal microbiota predicts lung cancer patients at risk of immune-related diarrhea. Immunotherapy. 2019;11(5):385–396. doi:10.2217/imt-2018-0144.
- Abu-Sbeih H, Herrera LN, Tang T, Altan M, Chaftari AP, Okhuysen PC, Jenq RR, Wang Y. Impact of antibiotic therapy on the development and response to treatment of immune checkpoint inhibitor-mediated diarrhea and colitis. J Immunother Cancer. 2019;7(1):242. doi:10.1186/s40425-019-0714-x.
- Hemmi H, Takeuchi O, Kawai T, Kaisho T, Sato S, Sanjo H, Matsumoto M, Hoshino K, Wagner H, Takeda K, et al. A Toll-like receptor recognizes bacterial DNA. Nature. 2000;408(6813):740–745. doi:10.1038/35047123.
- Klinman DM. Immunotherapeutic uses of CpG oligodeoxynucleotides. Nat Rev Immunl. 2004;4(4):249–258. doi:10.1038/nri1329.
- Krieg AM, Yi AK, Matson S, Waldschmidt TJ, Bishop GA, Teasdale R, Koretzky GA, Klinman DM. CpG motifs in bacterial DNA trigger direct B-cell activation. Nature. 1995;374(6522):546–549. doi:10.1038/374546a0.
- Häcker H, Vabulas RM, Takeuchi O, Hoshino K, Akira S, Wagner H. Immune cell activation by bacterial CpG-DNA through myeloid differentiation marker 88 and tumor necrosis factor receptor-associated factor (TRAF)6. J Exp Med. 2000;192(4):595–600. doi:10.1084/jem.192.4.595.
- Murad YM, Clay TM, Lyerly HK, Morse MACPG. 7909 (PF-3512676, ProMune): toll-like receptor-9 agonist in cancer therapy. Expert Opin Biol Ther. 2007;7(8):1257–1266. doi:10.1517/14712598.7.8.1257.
- Adamus T, Kortylewski M. The revival of CpG oligonucleotide-based cancer immunotherapies. Contemp Oncol (Pozn). 2018;22(1A):56–60. doi:10.5114/wo.2018.73887.
- Voeller J, Erbe AK, Slowinski J, Rasmussen K, Carlson PM, Hoefges A, VandenHeuvel S, Stuckwisch A, Wang X, Gillies SD, et al. Combined innate and adaptive immunotherapy overcomes resistance of immunologically cold syngeneic murine neuroblastoma to checkpoint inhibition. J Immunother Cancer. 2019;7(1):344. doi:10.1186/s40425-019-0823-6.
- Mangsbo SM, Sandin LC, Anger K, Korman AJ, Loskog A, Tötterman TH. Enhanced tumor eradication by combining CTLA-4 or PD-1 blockade with CpG therapy. J Immunother. 2010;33(3):225–235. doi:10.1097/CJI.0b013e3181c01fcb.
- Reilley MJ, Morrow B, Ager CR, Liu A, Hong DS, Curran MA. TLR9 activation cooperates with T cell checkpoint blockade to regress poorly immunogenic melanoma. J Immunother Cancer. 2019;7(1):323. doi:10.1186/s40425-019-0811-x.
- Wang S, Campos J, Gallotta M, Gong M, Crain C, Naik E, Coffman RL, Guiducci C. Intratumoral injection of a CpG oligonucleotide reverts resistance to PD-1 blockade by expanding multifunctional CD8+ T cells. Proc Natl Acad Sci U S A. 2016;113(46):E7240–E7249. doi:10.1073/pnas.1608555113.
- Rodríguez-Nogales A, Algieri F, Garrido-Mesa J, Vezza T, Utrilla MP, Chueca N, Garcia F, Olivares M, Rodríguez-Cabezas ME, Gálvez J. Differential intestinal anti-inflammatory effects of Lactobacillus fermentum and Lactobacillus salivarius in DSS mouse colitis: impact on microRNAs expression and microbiota composition. Mol Nutr Food Res. 2017;61(11):11. doi:10.1002/mnfr.201700144.
- Jang YJ, Kim WK, Han DH, Lee K, Ko G. Lactobacillus fermentum species ameliorate dextran sulfate sodium-induced colitis by regulating the immune response and altering gut microbiota. Gut Microbes. 2019;10(6):696–711. doi:10.1080/19490976.2019.1589281.
- O’Keefe SJ. Diet, microorganisms and their metabolites, and colon cancer. Nat Rev Gastroenterol Hepatol. 2016;13(12):691–706. doi:10.1038/nrgastro.2016.165.
- Louis P, Hold GL, Flint HJ. The gut microbiota, bacterial metabolites and colorectal cancer. Nat Rev Microbiol. 2014;12(10):661–672. doi:10.1038/nrmicro3344.
- Yang J, Yu J. The association of diet, gut microbiota and colorectal cancer: what we eat may imply what we get. Protein Cell. 2018;9(5):474–487. doi:10.1007/s13238-018-0543-6.
- Costea T, Hudiță A, Ciolac OA, Gălățeanu B, Ginghină O, Costache M, Ganea C, Mocanu MM. Chemoprevention of colorectal cancer by dietary compounds. Int J Mol Sci. 2018;19(12):3787. doi:10.3390/ijms19123787.
- Yang Y, Paik JH, Cho D, Cho JA, Kim CW. Resveratrol induces the suppression of tumor-derived CD4+CD25+ regulatory T cells. Int Immunopharmacol. 2008;8(4):542–547. doi:10.1016/j.intimp.2007.12.006.
- Chen L, Yang S, Liao W, Xiong Y. Modification of antitumor immunity and tumor microenvironment by resveratrol in mouse renal tumor model. Cell Biochem Biophys. 2015;72(2):617–625. doi:10.1007/s12013-015-0513-z.
- Zhao Y, Shao Q, Zhu H, Xu H, Long W, Yu B, Zhou L, Xu H, Wu Y, Su Z. Resveratrol ameliorates Lewis lung carcinoma-bearing mice development, decreases granulocytic myeloid-derived suppressor cell accumulation and impairs its suppressive ability. Cancer Sci. 2018;109(9):2677–2686. doi:10.1111/cas.13720.
- Soto BL, Hank JA, Van De Voort TJ, Subramanian L, Polans AS, Rakhmilevich AL, Yang RK, Seo S, Kim K, Reisfeld RA, et al. The anti-tumor effect of resveratrol alone or in combination with immunotherapy in a neuroblastoma model. Cancer Immunol Immunother. 2011;60(5):731–738. doi:10.1007/s00262-011-0971-0.
- Guan H, Singh NP, Singh UP, Nagarkatti PS, Nagarkatti M. Resveratrol prevents endothelial cells injury in high-dose interleukin-2 therapy against melanoma. PLoS One. 2012;7(4):e35650. doi:10.1371/journal.pone.0035650.
- Patel KR, Brown VA, Jones DJ, Britton RG, Hemingway D, Miller AS, West KP, Booth TD, Perloff M, Crowell JA, et al. Clinical pharmacology of resveratrol and its metabolites in colorectal cancer patients. Cancer Res. 2010;70(19):7392–7399. doi:10.1158/0008-5472.CAN-10-2027.
- Howells LM, Berry DP, Elliott PJ, Jacobson EW, Hoffmann E, Hegarty B, Brown K, Steward WP, Gescher AJ. Phase I randomized, double-blind pilot study of micronized resveratrol (SRT501) in patients with hepatic metastases--safety, pharmacokinetics, and pharmacodynamics. Cancer Prev Res (Phila). 2011;4(9):1419–1425. doi:10.1158/1940-6207.CAPR-11-0148.
- Feng X, Li Y, Brobbey Oppong M, Qiu F. Insights into the intestinal bacterial metabolism of flavonoids and the bioactivities of their microbe-derived ring cleavage metabolites. Drug Metab Rev. 2018;50(3):343–356. doi:10.1080/03602532.2018.1485691.
- Qin J, Teng J, Zhu Z, Chen J, Huang WJ. Genistein induces activation of the mitochondrial apoptosis pathway by inhibiting phosphorylation of Akt in colorectal cancer cells. Pharm Biol. 2016;54(1):74–79. doi:10.3109/13880209.2015.1014921.
- Sharma P, McClees SF, Afaq F. Pomegranate for prevention and treatment of cancer: an update. Molecules. 2017;22(1):177. doi:10.3390/molecules22010177.
- Núñez-Sánchez MA, González-Sarrías A, Romo-Vaquero M, García-Villalba R, Selma MV, Tomás-Barberán FA, García-Conesa MT, Espín JC. Dietary phenolics against colorectal cancer--From promising preclinical results to poor translation into clinical trials: pitfalls and future needs. Mol Nutr Food Res. 2015;59(7):1274–1291. doi:10.1002/mnfr.201400866.
- Jalili-Nik M, Soltani A, Moussavi S, Ghayour-Mobarhan M, Ferns GA, Hassanian SM, Avan A. Current status and future prospective of Curcumin as a potential therapeutic agent in the treatment of colorectal cancer. J Cell Physiol. 2018;233(9):6337–6345. doi:10.1002/jcp.26368.
- Wong KE, Ngai SC, Chan KG, Lee LH, Goh BH, Chuah LH. Curcumin nanoformulations for colorectal cancer: a review. Front Pharmacol. 2019;10:152. doi:10.3389/fphar.2019.00152.
- Woolf EC, Syed N, Scheck AC. Tumor metabolism, the ketogenic diet and β-hydroxybutyrate: novel approaches to adjuvant brain tumor therapy. Front Mol Neurosci. 2016;9:122.
- Husain Z, Huang Y, Seth P, Sukhatme VP. Tumor-derived lactate modifies antitumor immune response: effect on myeloid-derived suppressor cells and NK cells. J Immunol. 2013;191(3):1486–1495. doi:10.4049/jimmunol.1202702.
- Schmidt M, Pfetzer N, Schwab M, Strauss I, Kämmerer U. Effects of a ketogenic diet on the quality of life in 16 patients with advanced cancer: A pilot trial. Nutr Metab (Lond). 2011;8(1):54. doi:10.1186/1743-7075-8-54.
- Sremanakova J. Sowerbutts AM1, Burden S. A Systematic Review of the Use of Ketogenic Diets in Adult Patients with Cancer. J Hum Nutr Diet. 2018;31:793–802.
- Celiberto LS, Bedani R, Rossi EA, Cavallini DC. Probiotics: the scientific evidence in the context of inflammatory bowel disease. Crit Rev Food Sci Nutr. 2017;57(9):1759–1768. doi:10.1080/10408398.2014.941457.
- Derwa Y, Gracie DJ, Hamlin PJ, Ford AC. Systematic review with meta-analysis: the efficacy of probiotics in inflammatory bowel disease. Aliment Pharmacol Ther. 2017;46(4):389–400. doi:10.1111/apt.14203.
- Gamallat Y, Meyiah A, Kuugbee ED, Hago AM, Chiwala G, Awadasseid A, Bamba D, Zhang X, Shang X, Luo F, et al. Lactobacillus rhamnosus induced epithelial cell apoptosis, ameliorates inflammation and prevents colon cancer development in an animal model. Biomed Pharmacother. 2016;83:536–541. doi:10.1016/j.biopha.2016.07.001.
- Banna GL, Torino F, Marletta F, Santagati M, Salemi R, Cannarozzo E, Falzone L, Ferraù F, Libra M. Lactobacillus rhamnosus GG: an overview to explore the rationale of its use in cancer. Front Pharmacol. 2017;8:603. doi:10.3389/fphar.2017.00603.
- Jacouton E, Chain F, Sokol H, Langella P, Bermúdez-Humarán LG. Probiotic strain lactobacillus casei BL23 prevents colitis-associated colorectal cancer. Front Immunol. 2017;8:1553. doi:10.3389/fimmu.2017.01553.
- Hu J, Wang C, Ye L, Yang W, Huang H, Meng F, Shi S, Ding Z. Anti-tumour immune effect of oral administration of Lactobacillus plantarum to CT26 tumour-bearing mice. J Biosci. 2015;40(2):269–279. doi:10.1007/s12038-015-9518-4.
- Hibberd AA, Lyra A, Ouwehand AC, Rolny P, Lindegren H, Cedgård L, Wettergren Y. Intestinal microbiota is altered in patients with colon cancer and modified by probiotic intervention. BMJ Open Gastroenterol. 2017;4(1):e000145. doi:10.1136/bmjgast-2017-000145.
- Zhuo Q, Yu B, Zhou J, Zhang J, Zhang R, Xie J, Wang Q, Zhao S. Lysates of Lactobacillus acidophilus combined with CTLA-4-blocking antibodies enhance antitumor immunity in a mouse colon cancer model. Sci Rep. 2019;9(1):20128. doi:10.1038/s41598-019-56661-y.
- Wang T, Zheng N, Luo Q, Jiang L, He B, Yuan X, Shen L. Probiotics lactobacillus reuteri abrogates immune checkpoint blockade-associated colitis by inhibiting group 3 innate lymphoid cells. Front Immunol. 2019;10:1235. doi:10.3389/fimmu.2019.01235.
- Shi L, Sheng J, Wang M, Luo H, Zhu J, Zhang B, Liu Z, Yang X. Combination therapy of TGF-β blockade and commensal-derived probiotics provides enhanced antitumor immune response and tumor suppression. Theranostics. 2019;9(14):4115–4129. doi:10.7150/thno.35131.
- Suez J, Zmora N, Segal E, Elinav E. The pros, cons, and many unknowns of probiotics. Nat Med. 2019;25:716–729.
- Gough E, Shaikh H, Manges AR. Systematic review of intestinal microbiota transplantation (fecal bacteriotherapy) for recurrent Clostridium difficile infection. Clin Infect Dis. 2011;53(10):994–1002. doi:10.1093/cid/cir632.
- McDonald LC, Gerding DN, Johnson S, Bakken JS, Carroll KC, Coffin SE, Dubberke ER, Garey KW, Gould CV, Kelly C, et al. Clinical practice guidelines for clostridium difficile infection in adults and children: 2017 update by the infectious diseases society of America (IDSA) and society for healthcare epidemiology of America (SHEA). Clin Infect Dis. 2018;66(7):987–994. doi:10.1093/cid/ciy149.
- Wortelboer K, Nieuwdorp M, Herrema H. Fecal microbiota transplantation beyond Clostridioides difficile infections. EBioMedicine. 2019;44:716–729. doi:10.1016/j.ebiom.2019.05.066.
- Antushevich H. Fecal microbiota transplantation in disease therapy. Clin Chim Acta. 2020;503:90–98. doi:10.1016/j.cca.2019.12.010.
- Wong SH, Zhao L, Zhang X, Nakatsu G, Han J, Xu W, Xiao X, Kwong TNY, Tsoi H, Wu WKK, et al. Gavage of fecal samples from patients with colorectal cancer promotes intestinal carcinogenesis in germ-free and conventional mice. Gastroenterology. 2017;153(6):1621–1633.e6. doi:10.1053/j.gastro.2017.08.022.
- Zhou D, Pan Q, Shen F, Cao HX, Ding WJ, Chen YW, Fan JG. Total fecal microbiota transplantation alleviates high-fat diet-induced steatohepatitis in mice via beneficial regulation of gut microbiota. Sci Rep. 2017;7(1):1529. doi:10.1038/s41598-017-01751-y.
- Wang Y, Wiesnoski DH, Helmink BA, Gopalakrishnan V, Choi K, DuPont HL, Jiang ZD, Abu-Sbeih H, Sanchez CA, Chang CC, et al. Fecal microbiota transplantation for refractory immune checkpoint inhibitor-associated colitis. Nat Med. 2018;24(12):1804–1808. doi:10.1038/s41591-018-0238-9.
- Kelly CR, Ihunnah C, Fischer M, Khoruts A, Surawicz C, Afzali A, Aroniadis O, Barto A, Borody T, Giovanelli A, et al. Fecal microbiota transplant for treatment of Clostridium difficile infection in immunocompromised patients. Am J Gastroenterol. 2014;109(7):1065–1071. doi:10.1038/ajg.2014.133.
- Shogbesan O, Poudel DR, Victor S, Jehangir A, Fadahunsi O, Shogbesan G, Donato A. A systematic review of the efficacy and safety of fecal microbiota transplant for clostridium difficile infection in immunocompromised patients. Can J Gastroenterol Hepatol. 2018;2018:1394379. doi:10.1155/2018/1394379.
- Wardill HR, Secombe KR, Bryant RV, Hazenberg MD, Costello SP. Adjunctive fecal microbiota transplantation in supportive oncology: emerging indications and considerations in immunocompromised patients. EBioMedicine. 2019;44:730–740. doi:10.1016/j.ebiom.2019.03.070.
- Kostic AD, Gevers D, Pedamallu CS, Michaud M, Duke F, Earl AM, Ojesina AI, Jung J, Bass AJ, Tabernero J, et al. Genomic analysis identifies association of Fusobacterium with colorectal carcinoma. Genome Res. 2012;22(2):292–298. doi:10.1101/gr.126573.111.
- Rubinstein MR, Wang X, Liu W, Hao Y, Cai G, Han YW. Fusobacterium nucleatum promotes colorectal carcinogenesis by modulating E-cadherin/β-catenin signaling via its FadA adhesin. Cell Host Microbe. 2013;14(2):195–206. doi:10.1016/j.chom.2013.07.012.
- Gur C, Ibrahim Y, Isaacson B, Yamin R, Abed J, Gamliel M, Enk J, Bar-On Y, Stanietsky-Kaynan N, Coppenhagen-Glazer S, et al. Binding of the Fap2 protein of Fusobacterium nucleatum to human inhibitory receptor TIGIT protects tumors from immune cell attack. Immunity. 2015;42(2):344–355. doi:10.1016/j.immuni.2015.01.010.
- Walter J, Armet AM, Finlay BB, Shanahan F. Establishing or exaggerating causality for the gut microbiome: lessons from human microbiota-associated rodents. Cell. 2020;180(2):221–232. doi:10.1016/j.cell.2019.12.025.
- Scott AJ, Alexander JL, Merrifield CA, Cunningham D, Jobin C, Brown R, Alverdy J, O’Keefe SJ, Gaskins HR, Teare J, et al. International Cancer Microbiome Consortium consensus statement on the role of the human microbiome in carcinogenesis. Gut. 2019;68(9):1624–1632. doi:10.1136/gutjnl-2019-318556.
- Tjalsma H, Boleij A, Marchesi JR, Dutilh BE. A bacterial driver-passenger model for colorectal cancer: beyond the usual suspects. Nat Rev Microbiol. 2012;10(8):575–582. doi:10.1038/nrmicro2819.
- Lau HCH, Kranenburg O, Xiao H, Yu J. Organoid models of gastrointestinal cancers in basic and translational research. Nat Rev Gastroenterol Hepatol. 2020;17(4):203–222. doi:10.1038/s41575-019-0255-2.
- Pleguezuelos-Manzano C, Puschhof J, Rosendahl Huber A, van Hoeck A, Wood HM, Nomburg J, Gurjao C, Manders F, Dalmasso G, Stege PB, et al. Mutational signature in colorectal cancer caused by genotoxic pks+ E. Coli Nature. 2020;580(7802):269–273. doi:10.1038/s41586-020-2080-8.
- Jia W, Xie G, Jia W. Bile acid-microbiota crosstalk in gastrointestinal inflammation and carcinogenesis. Nat Rev Gastroenterol Hepatol. 2018;15:111–128.
- Bernstein C, Holubec H, Bhattacharyya AK, Nguyen H, Payne CM, Zaitlin B, Bernstein H. Carcinogenicity of deoxycholate, a secondary bile acid. Arch Toxicol. 2011;85(8):863–871. doi:10.1007/s00204-011-0648-7.
- Liu L, Dong W, Wang S, Zhang Y, Liu T, Xie R, Wang B, Cao H. Deoxycholic acid disrupts the intestinal mucosal barrier and promotes intestinal tumorigenesis. Food Funct. 2018;9(11):5588–5597. doi:10.1039/C8FO01143E.
- Yoshimoto S, Loo TM, Atarashi K, Kanda H, Sato S, Oyadomari S, Iwakura Y, Oshima K, Morita H, Hattori M, et al. Obesity-induced gut microbial metabolite promotes liver cancer through senescence secretome. Nature. 2013;499(7456):97–101. doi:10.1038/nature12347.
- Mager LF, Burkhard R, Pett N, Cooke NCA, Brown K, Ramay H, Paik S, Stagg J, Groves RA, Gallo M, et al. Microbiome-derived inosine modulates response to checkpoint inhibitor immunotherapy. Science. 2020;369(6510):1481–1489. doi:10.1126/science.abc3421.
- Nakatsu G, Zhou H, Wu WKK, Wong SH, Coker OO, Dai Z, Li X, Szeto CH, Sugimura N, Lam TY, et al. Alterations in enteric virome are associated with colorectal cancer and survival outcomes. Gastroenterology. 2018;155(2):529–541.e5. doi:10.1053/j.gastro.2018.04.018.
- Coker OO, Nakatsu G, Dai RZ, Wu WKK, Wong SH, Ng SC, Chan FKL, Sung JJY, Yu J. Enteric fungal microbiota dysbiosis and ecological alterations in colorectal cancer. Gut. 2019;68(4):654–662. doi:10.1136/gutjnl-2018-317178.
- Coker OO, Wu WKK, Wong SH, Sung JJY, Yu J. Altered gut archaea composition and interaction with bacteria are associated with colorectal cancer. Gastroenterology. 2020;159(4):1459–1470.e5. doi:10.1053/j.gastro.2020.06.042.