ABSTRACT
Prematurity coupled with the necessary clinical management of preterm (PT) infants introduces multiple factors that can interfere with microbial colonization. This study aimed to review the perinatal, physiological, pharmacological, dietary, and environmental factors associated with gut microbiota of PT infants. A total of 587 articles were retrieved from a search of multiple databases. Sixty studies were included in the review after removing duplicates and articles that did not meet the inclusion criteria. Review of this literature revealed that evidence converged on the effect of postnatal age, mode of delivery, use of antibiotics, and consumption of human milk in the composition of gut microbiota of PT infants. Less evidence was found for associations with race, sex, use of different fortifiers, macronutrients, and other medications. Future studies with rich metadata are needed to further explore the impact of the PT exposome on the development of the microbiota in this high-risk population.
Introduction
The early postpartum period is a critically important time for establishing the gut microbiota. Studies in full-term infants have shown that the characteristics of gut microbial communities are determined by multiple factors, including postnatal age, mode of delivery, diet, antibiotic exposure,Citation1 geographic location, and ethnicity.Citation2 The type of feeding (breastfeeding or formula feeding) and the introduction to solids are the most influential in shaping composition and function of the gut microbiota in the first year of life.Citation3,Citation4 By 2- to 3-years of age, the gut microbiota resembles an adult-like microbiota composition.Citation5 However, other factors, such as the exposure to antibiotics and hospitalization, can disrupt this trend.Citation3,Citation5
Preterm infants, born less than 37 weeks of gestation, experience many physiological, medical, dietary, and environmental challenges that can detrimentally affect their microbial colonization. The rates of PT birth by cesarean section (C-section) are around 31% worldwide,Citation6 and 64% in the USA.Citation7 These rates are higher than the prevalence of C-section delivery in full-term infants, which is about 21%.Citation8 Given their prematurity and compromised health status, PT infants can remain hospitalized in the neonatal intensive care unit (NICU) for an extended period of time after birth. The total length of stay varies depending on the growth and development of each infant. Infants born at an earlier gestational age (GA) and with lower birth weight spend more time in the NICU.Citation9 As part of their medical care, PT infants receive many medications that can influence the gut microbiota, particularly antibiotics. Another critical factor in the treatment of these infants is how they are fed. The goal of the dietary treatments is to optimize the infant’s growth by providing adequate calories, macronutrients, and micronutrients via parenteral or enteral routes.Citation10 To achieve the nutritional goals, PT infants can be fed different types of milk and fortifiers during the course of their hospitalization.Citation11 Taken together, these factors can profoundly influence the establishment of the gut microbiota of infants born preterm.
The way the microbiome develops in early life is critically important, as key mutualistic relationships exist between the host, bacterial communities, and their metabolites. Additionally, the microbiome shapes immune development,Citation12,Citation13 and is implicated in cognitive development.Citation14 If this homeostasis is altered by external factors, a dysbiosis in the gut ecosystem can occur, with a greater presence and abundance of pathogenic bacteria.Citation15 In PT infants, the gut microbial composition is often characterized as dysbiotic,Citation16 with slower acquisition and an overall lower prevalence of beneficial bacteria.Citation17 This dysbiosis appears to be associated with a higher risk of developing serious complications including sepsis, and necrotizing enterocolitis (NEC),Citation16,Citation18,Citation19 which can have detrimental long-term effects on the infant’s health, including disruption in neurodevelopment. Previous systematic reviews have investigated how various factors influence PT infants microbiome, including antibiotic use,Citation20 enteral feeding,Citation21 and the hospital environment.Citation22 However, these factors do not work in isolation, and no previous systematic review has attempted to capture the full complexity of factors shaping PT infants microbiome. Thus, the goal of this review was to review the literature available regarding the impact of perinatal, physiological, pharmacological, dietary, and environmental factors on the composition of the gut microbiota of PT infants. By holistically examining the multifactorial influences on colonization of PT infant’s gut, gaps in the literature will be identified, which will highlight the opportunities for novel interventions aiming to optimize the establishment of these bacterial communities of infants born preterm.
Methods
This systematic review was registered in the PROSPERO database (CRD42020131964) and was conducted according to the guidelines of the Preferred Reporting Items for Systematic Review and Meta-Analysis Protocols (PRISMA).Citation23
Data sources and search strategy
A systematic search was performed in four databases (PubMed/MEDLINE, Scopus, Web of Science, and the Cochrane Library) from May to July of 2019. The search terms included: “preterm infant”, “premature infant”, “extremely premature infant” “gut microbiome”, “gut microbiota”, “gastrointestinal microbiome”, “fecal microbiota”, “maternal health”, “gestational age”, “mode of delivery”, “C-section”, “cesarean section”, “immaturity”, “neonatal intensive care unit”, “NICU”, “hospital environment”, “hospitals”, “antibiotics”, “anti-bacterial agents”, “medication”, “parenteral nutrition”, “enteral nutrition”, “breastfeeding”, “human milk”, “mother’s milk”, “donor human milk”, “preterm formula”, “infant formula”, “probiotic”, “probiotics”, “prebiotic”, “prebiotics”, and “milk fortifier”.
Study selection
To be eligible, studies needed to be focused on the gut microbiota of PT infants, conducted in human subjects, and be a cross-sectional, longitudinal, or s clinical trial study. Articles were excluded if they were not in English, no full-text was available, and were published before 2009, when advanced sequencing technologies were not widely used.Citation24 After the literature search, all obtained articles were independently assessed by the two authors (MAL and AMD) to determine those to be included in the review. In the case of disagreements, a third author (SMD) resolved the discrepancies.
Data extraction
The information extracted from each study included: author, year of publication, geographic location of the study sample, study design, sample size, length of study, intervention or exposure, (if applicable), intervention characteristics (if applicable), control group (if applicable), gut microbiota assessment method, 16S rRNA variable region (if applicable), sequencing platform (if applicable), alpha diversity, beta diversity, taxonomy, other gut microbiota related outcomes, and clinical outcomes.
Quality of the evidence and risk of bias assessment
Eligible clinical trials were assessed using the Cochrane Collaboration Tool for assessing risk of bias (RoB2).Citation25 This tool assesses potential research biases in five domains: bias arising from the randomization process, bias due to deviations from intended interventions, bias due to missing outcome data, bias in measurement of the outcomes, and bias in selection of the reported result. From these domains, an overall risk of bias was assigned to each study. A study was considered as “low risk of bias” if it showed low risk an all five domains, “some concerns” if it raised concerns in at least one of the domains, and “high risk of bias” if a study was high risk of bias in at least one of the domains, or scored “some concerns” in more than one domain.Citation25 Cross-sectional or longitudinal studies were assessed using the Newcastle-Ottawa Scale (NOS) for observational studies.Citation26 This tool measures four domains, including participant selection, comparability, exposure, and outcome. The scoring is based on number of stars, cross-sectional studies could receive up to six stars, and longitudinal studies could score a maximum of nine stars.Citation26 All the selected articles were assessed by MAL and AMD.
Results
Study selection
A total of 587 articles were identified through the database search, and four articles were retrieved through cross-reference. After removing duplicates, 170 articles were initially screened by title and abstract. At this step, 99 articles were excluded based on the study design (n = 73), studies performed in animal models or in vitro (n = 4), scope of the study (n = 14), year of publication (n = 3), and no abstract availability (n = 5). In total, 71 articles underwent full-text review. In this step, 11 articles were removed due to text not being available in English (n = 2), no availability of full text (n = 2), or scope of the study (n = 7). As shown in (), a total of 60 articles were included in the qualitative synthesis.
Study characteristics
Characteristics of the 60 articles are presented in (). The average sample if the included studies was of 50 infants. Twenty five percent of the studies were clinical trials (n = 15), and 75% observational studies (n = 45). The most common treatments from the intervention studies were the supplementation of prebiotics or probiotics (n = 14). The determination methods of the gut microbiota of PT infants, summarized in Supplementary Table 1, included bacterial DNA sequencing, bacterial culture, denaturing, and temperature gradient gel electrophoresis (DGGE and TGGE), terminal restriction fragment length polymorphism (T-RFLP), pulsed-field gel electrophoresis (PFGE), fluorescent in situ hybridization (FISH), matrix assisted laser desorption/ionization time-of-flight mass spectrometry (MALDI-TOF) and microarrays. Most studies used next-generation sequencing (NGS) technologies targeting the V3-V4, V4, and V3-V5 regions of the 16S rRNA bacterial gene. The platforms used included Ion Torrent, Roche 454 GS FLX Titanium, and Illumina technologies.
Table 1. Characteristics of studies included in the systematic review
Quality of the evidence
The RoB2 tool, applied to clinical trials, showed that 4/15 studies (26.7%) scored “Some concerns” for the risk of bias. The primary source of bias, summarized in Supplementary Table 2, came from the randomization process,Citation27 deviation from the intended intervention,Citation28–30 and the measurement of the outcomes.Citation31 One study,Citation31 was found to have high risk of bias, and thus it was not included in the description of the results. Evaluation of cohort and case-control studies using NOS are shown in Supplementary Table 3 and Supplementary Table 4, respectively. Among the cohort studies, 39.4% had a score of nine stars (highest score possible. A score of six was obtained in 20 of the 33 cohort studies, because these studies consisted in only one group of PT infants. Therefore, these cohort studies had no score for the “selection of the non-exposed cohort” and “comparability of cohort on the bases of the design or analysis” sections. Lastly, all the case-control studies had an overall score of nine stars.
Factors affecting the gut microbiota of preterm infants
Perinatal factors
Pregnancy complications
Four observational studies, shown in (), reported the effect of premature rupture of membranes (PROM),Citation32–34 chorioamnionitis, which is the bacterial infection of the membranes of the placenta and amniotic fluid,Citation32,Citation35 prenatal antibiotics,Citation32 and antenatal steroidsCitation35 on the gut microbiota composition of PT infants. Infants from mothers who had PROM and/or chorioamnionitis (diagnosed and confirmed by placental pathology) during pregnancy had lower alpha diversity over time compared to those infants whose mothers did not develop these complications.Citation32 However, this association was significantly confounded by the use of antibiotics. Cong et al. found that PROM explained ~2% of the variation of the beta diversity from gut microbiota of PT infants.Citation33 Chernikova et al. described that, regardless of the use of antibiotics, PT infants exposed to prolonged PROM had higher abundances of Staphylococcus and Streptococcus across time; these infants also showed faster increase in the abundance of Enterobacter, and lower colonization with Clostridium over time.Citation32 In contrast, Zwittink et al. found no association between the gut microbiota composition of PT infants and the exposure to PROM.Citation34 Infants exposed to chorioamnionitis during gestation, had greater abundances of Serratia, Parabacteroides, and Bradyrhizobium independent of the use of antibiotics.Citation32 It is important to mention that Bradyrhizobium has been described as a common contaminant from NGS techniques which can be detected in samples with low microbial biomass.Citation36 Another observational study found that the relative abundance of Gammaproteobacteria was positively associated with antenatal steroids.Citation35 This same study demonstrated that PT infants showed two different gut bacterial community patterns described as clusters. Cluster 1 with low abundances of Gammaproteobacteria and Cluster 2 with high abundances of Gammaproteobacteria.Citation35 When PT infants from Cluster 2 were exposed to chorioamnionitis (diagnosed by clinical sings) during gestation, the abundances of Gammaproteobacteria were lower, whereas PT infants from Cluster 2 exposed to antenatal steroids had higher abundances of Gammaproteobacteria.Citation35
Table 2. Perinatal factors and gut microbiota composition of PT infants
Mode of delivery
A total of 21 studies reported associations between mode of delivery and the characteristics of the gut microbiota of PT infants, shown in (). One longitudinal study that followed PT infants from birth until discharge found that, over time, infants born via C-section had higher alpha diversity (Simpson diversity index) compared to vaginally delivered infants.Citation32 However, a large number of studies reported no associations between mode of delivery and alpha diversity.Citation37–42 Similarly, most of the studies found no differences in beta diversity by mode of delivery.Citation37,Citation40,Citation43–45 Only two observational studies reported that mode of delivery explained 1.93%Citation37 to 12%Citation41 of the variation of beta diversity of the fecal microbiota of PT infants. It is important to note that results from Butcher et al. came from PT infants exclusively fed mother’s own milk (MOM).Citation37 A cohort study analyzed the fecal microbiota composition of PT infants during early and late feeding stages, representing 1 to 2 weeks and 2 to 4 weeks after the introduction of enteral feeding, respectively. The authors found that mode of delivery was significantly associated with beta diversity (Unweighted UniFrac distances) only during the late feeding time points.Citation46
Thirteen publications reported significant modifications in the taxonomic profile of PT infants depending on mode of delivery. A case-control study looking at the effect of histamine-2 receptor (H-2) blocker, found that Proteobacteria abundance was significantly lower in vaginally-delivered infants compared to infants born via C-section.Citation47 Ho et al. reported that the abundance of Firmicutes was positively associated with birth via C-section.Citation35 At class level, this same study reported a positive association between Gammaproteobacteria abundances and vaginal delivery at ≤ 2 weeks of postnatal age. This difference was mainly attributed to PT infants belonging to a cluster of colonization characterized by high abundances of Gammaproteobacteria.Citation35 In accordance with this, La Rosa et al. found that the abundances of Gammaproteobacteria were negatively associated with C-section delivery only in infants born less than 26 weeks of GA.Citation48 It was also reported by two different authors that the abundance of Bacilli was greater in PT infants delivered via C-section.Citation37,Citation48 Furthermore, vaginal delivery was positively associated with the abundances of Bacteroides,Citation41,Citation44,Citation49–51 Parabacteroides,Citation44 Staphylococcus,Citation38 and Enterobacteriaceae,Citation52 and was negatively associated with the abundances of Enterobacter, Pantotea, Kluyvera, Erwinia, KlebsiellaCitation32 and Clostridium.Citation52 Differences between mode of delivery and gut microbiota composition seem to be more pronounced soon after birth, and diminish over postnatal time. A longitudinal study over the first 100 days of life of PT infants reported that during the first week after birth, vaginally delivered infants belonged to a bacterial cluster dominated by Escherichia, and infants born via C-section were more likely to associate with a cluster dominated by Klebsiella.Citation40 Although these differences remained similar during the first four consecutive weeks of postnatal age, after the fifth week, both groups (vaginally delivered and C-section) showed similar patterns of colonization.Citation40 Finally, a total of four studies found no differences in gut microbial composition and mode of delivery.Citation38,Citation53–55
Physiological factors
Ethnicity and sex
Few data exist regarding associations between ethnicity and sex and the gut microbial colonization of PT infants, as shown in (). A longitudinal observational study reported associations between race and the abundances of Firmicutes, and Gammaproteobacteria. The abundance of Firmicutes was positively associated with Latino ethnicity in PT infants with a colonization pattern low in Gammaproteobacteria. At ≤ 2 weeks postnatal age, Gammaproteobacteria abundance was positively associated with Latino ethnicity.Citation35
Table 3. Physiological factors and gut microbiota composition of PT infants
In terms of differences in microbiota by infant sex, Cong et al. showed that alpha diversity, measured by the Gini-Simpson diversity index, was positively associated with female sex.Citation33 As for beta diversity, sex explained 6% of the variance from the Bray-Curtis dissimilarity index.Citation33 In contrast, two publications found no differences in alpha diversity,Citation42 and beta diversityCitation34 associated with infant’s sex.
Weight and growth
Four studies, summarized in (), reported differences in the gut microbial composition depending on weight and growth rate. Two observational studies found birth weight to be significantly associated with gut microbiota beta diversity of PT infants.Citation54,Citation56 Gregory et al. reported that, after birth, there were significant differences in beta diversity between PT infants with extremely low birth weight (ELBW, birth weight <1000 g) and PT infants with very low birth weight (VLBW, birth weight <1500 g).Citation57 This same study, observed differences in the taxonomic composition by birth weight. However, these differences were primarily observed in infants fed PT formula. Across time, the abundances of Lactobacillales were higher in ELBW infants compared to VLBW infants. In contrast, the abundance of Clostridiales and Enterobacteriales was greater in VLBW across time compared to ELBW.Citation57
A longitudinal study analyzed the association between growth and gut microbial colonization.Citation58 The authors compared PT infants that presented growth failure (weight below the 3rd percentile of the Fenton growth charts) at 40-weeks postmenstrual age and PT infants with appropriate growth. In the first nine weeks postnatal age, alpha diversity (Shannon diversity index) was lower in infants with growth failure.Citation58 Infants that had growth failure had higher abundances of Staphylococcaceae and Bacteroideceae during the first weeks postnatal age, but during the third and ninth week of life, PT infants had greater abundance of Enterobacteriaceae and Erysipelotrichaceae.Citation58 In the appropriate postnatal growth group, the authors found significant differences over time (1–9 weeks postnatal age) in bacteria of the family Bacillaceae, Streptococcaceae, Peptostreptococcaceae, Veillonellaceae, Lachnospira-ceae, Micrococcaceae, Tissierellaceae and Clostridiaceae.Citation58 Furthermore, this same group created a gut microbiota maturity index to investigate its association with growth. The final model of this maturity index included the following discriminatory bacteria: Lactobacillales, Peptostreptococcaceae, Clostridiaceaceae, Streptococcus, Staphylococcus, Veillonella, Enterococcus, Rahnella, Bifidobacterium, and Erwinia.Citation58 Even though the relative microbiota maturity index was positively correlated with postmenstrual age, infants with growth failure had significantly lower values of this index compared to infants with appropriate growth.Citation58
Birth gestational age, postnatal age, and corrected gestational age
A total of 28 studies, shown in (), reported differences in diversity and composition of PT infants gut microbiota based on GA at birth, postnatal age, and corrected GA. Two longitudinal studies reported significant associations between GA at birth and different diversity indices.Citation50,Citation59 Dahl et al. analyzed the gut microbiota composition of PT infants at three different time points: 10 days, 4 months, and 1 year after birth. The authors found that Shannon diversity index was positively associated with GA at birth during the first 10 days postnatal age, even after controlling for exposure to antibiotics.Citation59 Similar results were found by Chernikova et al. where after adjusting for postnatal age, antibiotic use, delivery mode and consumption of human milk, extremely PT infants (born <28 weeks GA) had significantly lower alpha diversity (measured by the Simpson diversity index) compared to very PT infants (born 28–32 weeks GA) and to moderate/late PT infants (born 32–37 weeks GA).Citation50 Whereas alpha diversity was similar between very and moderate/late PT infants.Citation50 Therefore, the authors created two groups of infants based on birth GA: infants born before 32 weeks GA, and those born ≥32 weeks GA. Infants born at a later age had higher Simpson diversity index compared to those born before 32 weeks of gestation.Citation50 A large number of longitudinal observational studies reported that alpha diversity, measured by different indices, increases with postnatal age.Citation35,Citation37,Citation38,Citation40,Citation50,Citation57,Citation60,Citation61
Eight studies reported the effect of birth GA and postnatal age on beta diversity. Two longitudinal studies explored gut microbial colonization of PT infants based on type of feeding during the first days of postnatal age.Citation33,Citation37 Results showed that GA at birth explained 1.28%Citation37 to 3%Citation33 of the variance of the Bray-Curtis dissimilarity index. The former came from infants exclusively fed MOM,Citation37 whereas the latter was independent of the feeding type.Citation33 Four different observational studies looked at gut microbiota development of PT infants, with a follow-up period of the first 21,Citation62 30,Citation43 or up to 60Citation42,Citation57 days of life. These studies found that postnatal age significantly associates with the community structure measured by UniFrac distances,Citation62 and the Bray-Curtis dissimilarity index.Citation42,Citation43,Citation57
Twenty-four studies included in this systematic review reported differences in taxonomic composition based on postnatal age. Mai et al. conducted a case-control study comparing PT infants with late onset sepsis to healthy PT infants.Citation63 At phylum level, the authors found that in healthy infants, there is a decrease in the abundances of Proteobacteria over time.Citation63 Evidence from multiple longitudinal studies suggests that during early time points after birth, there is an enrichment of Bacilli,Citation37,Citation48,Citation64 which then decreases over time.Citation35 This decrease in Bacilli coincides with an enrichment of Gammaproteobacteria,Citation35,Citation64 and Clostridia.Citation35,Citation37,Citation48,Citation64 In accordance, Gregory et al. showed that from 28–30 weeks of corrected GA, the gut microbiota is characterized by higher abundances of Bacillales and Lactobacillales.Citation57 Following this, there is a significant decrease in Lactobacillales, particularly in infants fed PT formula.Citation57 Around 31–33 weeks of corrected GA, in infants fed PT formula, there is a bloom of Enterobacteriales, and in infants fed PT formula plus MOM a bloom of Clostridiales.Citation57 At family level, authors reported that during early-life time points (<5 weeks postnatal age) there are higher abundances of Comamonadaceae,Citation65 and Gram-positive cocciCitation55 such as Staphylococcaceae.Citation58 Bacteria from the families of EnterobacteriaceaeCitation43,Citation55,Citation58,Citation65 and BifidobacteriaceaeCitation55 also increase their abundance over time.
At lower taxonomic rank, bacteria of the genera Staphylococcus,Citation38,Citation45,Citation66 and EnterococcusCitation38,Citation45,Citation66,Citation67 are the main colonizers of PT infants gut during the first weeks of life (<4 weeks postnatal age). Zwittink et al. showed that at three weeks postnatal age, the mean relative abundance of Staphylococcus and Enterococcus was higher in extremely PT (<28 weeks GA) infants compared to very and moderate/late (32–37 weeks GA) PT infants.Citation45 Following the first weeks of life, some studies report a decrease in the abundance of Staphylococcus,Citation32,Citation52,Citation62 Escherichia-Shigella,Citation32 Streptococcus,Citation52 and Parabacteroides.Citation50 Furthermore, there is a positive association between postnatal age and the presence and/or abundance of specific bacteria, including Anaerobiospirillum,Citation66 Haemophilus,Citation66 Veillonella,Citation32,Citation66 Lactobacillus,Citation50 Bacteroides,Citation51 Clostridia,Citation66 Serratia,Citation61,Citation66 Yersinia,Citation61,Citation66 Pseudomonas,Citation66 Klebsiella,Citation52 Granulicatella,Citation61,Citation66 Proteus,Citation66 Propionibacterium,Citation56 and Enterobacter.Citation38 Zhou et al. reported that in their study population, Enterobacter was a member of the core microbiota of PT infants over the first 60 days of postnatal age.Citation42 However, these results are still not consistent. Moles et al. found a decrease in the abundance of Propionibacterium from meconium samples to stool samples during the third week of life.Citation66 It is important to highlight that although studies report an increase in the abundance of Bifidobacterium over time,Citation38,Citation52,Citation65,Citation68 evidence converges in that the colonization with this obligate anaerobe is delayed in PT infants.Citation17,Citation55
The colonization pattern across time in PT infants is also affected by GA at birth. Chernikova et al. reported that infants born >32 weeks GA are colonized with greater abundances of Streptococcus and Bifidobacterium than those born ≤32 weeks GA.Citation50 At 6-weeks of postnatal age, infants born >32 weeks GA had a higher number of members from the genera Bacteroides and lower abundance of Parabacteroides.Citation50 This same study also reported that the abundance of Pantoea, a bacteria of the family Enterobacteriaceae, was higher in moderate/late PT infants (32–37 weeks GA), even after adjusting for other exposures including postnatal age.Citation50 Another longitudinal study found that at three weeks postnatal age, extremely PT infants (<28 weeks GA) had higher abundances of Enterobacter, whereas very PT infants (28–32 weeks GA) harbored higher abundances of Bifidobacterium.Citation45
Less data exists regarding the relationship between specific species and postnatal age. Two longitudinal studies analyzed the gut microbiota of PT infants at species level utilizing human intestinal tract chip analysisCitation61 or PCR-amplified 16S rRNA fragments.Citation66 There was a positive association between postnatal age and the abundance of Enterobacter aerogenes,Citation61 Bacteroides splachnicus,Citation66 Escherichia coli,Citation61 Clostridium difficille,Citation66 and Klebsiella pneumoniae.Citation61,Citation66 Whereas, over time, there was a decrease in Prevotella tannerae, Lactobacillus plantarum, Streptococcus intermedius, and Streptococcus mitis.Citation66
Pharmacological factors
Antibiotics
Twenty-two studies reported the effect of antibiotics on the gut bacterial communities of PT infants, presented in (). As expected, antimicrobial agents reduced the gut bacterial diversity.Citation32,Citation34,Citation42,Citation56,Citation60,Citation69,Citation70 Two observational studies determined that the duration of antibiotic exposure was significantly associated with the reduction in microbial diversity.Citation34,Citation71 The decrease in alpha diversity was similar in PT infants that were exposed to short antibiotic treatment, ranging from ≤ 3 daysCitation34 to ≤ 7 days,Citation71 or exposed to longer treatment (≥ 5 days or > 7 days).Citation34,Citation71 Furthermore, the reduction in alpha diversity seems to be only temporal. Several studies reported that a decrease in diversity indices like observed OTUs, Simpson, Shannon, Chao1, and phylogenetic diversity remains significant only within the first week after the use of antibiotics.Citation34,Citation42,Citation56,Citation70 In fact, diversity tends to recover after the cessation of antibiotic treatment.Citation32,Citation34 Nonetheless, some studies reported no effect of antibiotics in diversity metricsCitation38 or opposite resultsCitation41 than those previously described in this review. Wandro et al. conducted a longitudinal study of VLBW (<1500 g) infants and found decrease Shannon diversity index in PT infants with no record of antibiotic use.Citation41
Table 4. Pharmacological factors and gut microbiota composition of PT infants
Several studies found significant associations between the use of antibiotics and beta diversity of gut microbiota from PT infants.Citation33,Citation34,Citation43,Citation45 Cong et al. observed that antibiotic use within the first 48–72 hours after birth explained ~3% of the variation from the Bray-Curtis dissimilarity index.Citation33 Evidence from two studies conducted by Zwittink et al. described a strong association between antibiotic treatment and beta diversity.Citation34,Citation45 The duration of the use of antibiotics, whether it was less than three days or more than five days, explained 3.6% of the variation of the gut microbiota composition.Citation34 Furthermore, up to 25.6% of the variance of these bacterial communities was explained when more antibiotic-related factors were taken into consideration, such as duration and number of antibiotics that were administrated.Citation45
Changes in diversity induced by antimicrobial agents in PT infants subsequently influence taxonomic composition of the fecal microbiota, with some bacteria decreasing while others blooming. Specifically, there was a positive association between the exposure to antibiotics and the abundance of GammaproteobacteriaCitation37,Citation48,Citation64 and Betaproteobacteria,Citation71 while there was a negative association with bacteria from the class Clostridia.Citation48,Citation72 A study found that, at 30 days postnatal age, PT infants who were never exposed to antibiotics, had higher abundances of Bifidobacteriaceae, Streptococcaceae, Comamonadaceae, Staphylococcaceae, and unclassified Bacilli compared to infants that have been previously exposed to antibiotics.Citation65 Millar et al. reported that by each successive day of antibiotic usage in PT infants, there is 16% to 17% less chance of colonization with Veillonallaceae.Citation73 In accordance with this, another study reported that exposure to antibiotics reduces the presence of bacteria from the genus Veillonella.Citation53 Different studies reported a negative association between the exposure to antibiotics and the abundance of Enterobacteriaceae,Citation34 Lactobacillus,Citation53 Bifidobacterium,Citation34,Citation50,Citation71 and Bacteroides;Citation50 and a positive association between antibiotic use and the abundance of Enterococcus.Citation34 However, a study looking specifically at Bacteroides gut colonization in PT infants found no association between antibiotic use and the abundance of this bacteria.Citation51
Importantly, microbiota modifications caused by antibiotics might depend on the type of antibiotic used. Gibson et al. assessed the effect of different antibiotics, including meropenem, cefotaxime, ticarcillin/clavulanate, ampicillin, vancomycin, and gentamicin. They reported an increase in Staphylococcus epidermis after the use of meropenem (beta-lactamase inhibitor), Klebsiella pneumoniae after the use of Ticarcillin-Clavulanate (combined extended-spectrum penicillin with a beta-lactamase inhibitor), and Escherichia coli with the use of cefotaxime, a broad spectrum cephalosporin antibiotic.Citation60 All of these medications exert their antibiotic effects by affecting cell wall synthesis, or by causing cell death. A similar study conducted by Zhu et al. reported an increase in bacteria of the phylum Bacteroides and Actinobacteria with the use of penicillin-moxalactam (an oxacephem antibiotic usually grouped with the cephalosporins) and with the use of piperacillin-tazobactam (penicillin with a beta-lactamase inhibitor).Citation70 With penicillin-moxalactam, PT infants had greater abundances of Sphingomonas, Bacteroides, and Lactobacillus, and a decrease in Clostridium.Citation70 Korpela and collaborators found a decrease in Bifidobacterium abundances when an antibiotic of the class aminoglycosides or vancomycin were used.Citation38 This same study also described that when antibiotics of the class of aminoglycosides were administered, the abundance of Enterococcus decreased. In contrast, when vancomycin was used, the presence of Enterococcus was higher.Citation38 Although the taxonomic modifications were significant, these were only temporal, and the microbiota structure recovered within days after the cessation of antibiotic treatment.Citation38
Even though antibiotics are used to treat or reduce the presence of pathogenic bacteria in PT infants, their efficacy could be blunted by the presence of antibiotic-resistant bacteria. Moles et al. evaluated the gut colonization of PT infants by antibiotic-resistant bacteria during the first week of life and at 2-years of age.Citation74 Bacteria isolates obtained from stool samples were assessed for antibiotic susceptibility using agar dilution assays. This assay consists in platting the isolates in agar medium with antibiotics and measuring the diameters of the colonies that were exposed to the antibiotic.Citation75 The authors also performed bacteria identification at species level using MALDI-TOF spectrometry. In the early postpartum period, PT infants were colonized by a number of antibiotic-resistant bacteria including Enterococcus faecalis, Enterococcus faecium, Staphylococcus aureus, Escherichia coli, and Klebsiella pneumonia.Citation74 However, by 2-years of age, these same bacteria showed antibiotic susceptibility.Citation74
Other medications
Only a few studies have evaluated the effect of medications other than antibiotics on the gut colonization of PT infants, as shown in (). Gupta et al. conducted a case-control/cross-sectional study of infants who received H2-blockers vs. infants who did not received this medication.Citation47 There was a decrease in the alpha diversity (measured by Shannon diversity index) in PT infants that were exposed to H2-blockers compared to those not exposed to this medication.Citation47 Taxonomically, after the administration of H2-blockers, there was a significant decrease in Firmicutes accompanied by an increase of Proteobacteria. At lower taxonomic ranks, infants that were exposed to H2-blockers had increased abundance of Gammaproteobacteria and Enterobacteriaceae.Citation47 Additionally, an observational study found a positive association between the use of H2-blockers and the abundance of Bifidobacterium at a later time point of the follow-up period (>33 weeks postmenstrual age).Citation64
Dietary factors
Macronutrients
Several studies, summarized in (), have reported modifications of the gut microbiota of PT infants based on macronutrient composition, type of milk consumed, and use of fortifiers. A longitudinal study following PT infants during the hospitalization period in the NICU found that the ratio of grams of enteral lipids to total calories (g/kcal) was positively associated with the abundance of Actinobacteria, the ratio of enteral protein (g/kcal) with Firmicutes abundance, and ratio enteral carbohydrate (g/kcal) with abundance of Actinobacteria, Proteobacteria, and Firmicutes.Citation64 At 33-weeks postmenstrual age, there was an increase of Bifidobacterium abundance associated with greater ratio of enteral lipid intake whereas, and higher ratio of enteral protein intake was associated with reduced Bifidobacterium.Citation64
Table 5. Dietary factors and gut microbiota composition of PT infants
Younge et al. conducted a randomized controlled trial (RCT) to test the effect of enteral supplementation of high-fat polyunsaturated fatty acid (HF-PUFA) from fish oil and safflower oil on the gut microbiota of PT infants.Citation76 There were no differences in the first week after supplementation with HF-PUFA, but over time, alpha diversity (measured by Shannon and inverse Simpson indices) was higher in infants that received HF-PUFA supplementation.Citation76 At phylum level, those receiving the HF-PUFA intervention had a lower abundance of Proteobacteria and higher abundance of Actinobacteria than those without the intervention.Citation76 There were further differences at the genus level, and these differences were categorized as early (1–9 weeks), mid (2–9 weeks), or late (4–9 weeks) changes after treatment initiation. Some of the early changes were a decrease in Escherichia-Shigella and Salmonella in the HF-PUFA, and an increase in the abundance of Corynebacterium and Geobacillus. At 2–9 weeks after initiation of HF-PUFA, supplementation, there was a significant increase in the relative abundance of Erwinia and decreases in Serratia, Pantoea, Clostridium, Tatumella, and Streptococcus. Lastly, a reduction in fecal Cedecea and Citrobacter in the HF-PUFA group was reported as a late change.Citation76
Milk and fortifiers
Twenty studies reported associations related to milk and/or fortifier administration to PT infants and the structure of their gut microbiota, shown in (). One study reported an increase in the alpha diversity with the consumption of exclusively MOM, compared to donor human milk (DHM), PT formula or the combination of two different types of milk (MOM + DHM, MOM + PT formula, or DHM + PT formula).Citation33 Gibson et al described an increase in species richness with the consumption of human milk (MOM, DHM or the combination of both).Citation60 Another study reported that the combination of MOM with a bovine milk-based fortifier, two to four weeks after the introduction of enteral feeds, significantly increased alpha diversity compared to PT infants fed PT formula alone or in combination with MOM.Citation46 Underwood et al. found a lower Shannon diversity index in infants fed PT formula compared to those fed MOM.Citation30 Additionally, one study reported that introduction of PT formula before 10 days postnatal age was positively associated with Shannon diversity index.Citation59 Nonetheless, some studies found no differences in alpha diversity based on the type of diet, whether it was human milk (MOM and/or DHM),Citation41,Citation50,Citation77 PT formula,Citation77 the combination of human milk and PT formula,Citation41 or the supplementation of human milk with a bovine milk-based fortifier.Citation33
Six different studies described the effect of milk and fortifiers on beta diversity. There was a positive association between feeding type (MOM, DHM, and PT formula) and beta diversity, measured by Bray-Curtis dissimilarity indexCitation57,Citation78 and UniFrac distances.Citation78 Another study comparing only human milk vs. PT formula found an association between these feeding exposures and the gut microbiota structure.Citation79 Cong et al. compared the effect of different types of milks and found that up to 11% of the variance in the Bray-Curtis dissimilarity index could be explained by the feeding type.Citation33 However, some studies found no association between human milk consumption and the gut microbiota beta diversity of infants born preterm.Citation34,Citation45
Taxonomically, studies found a positive association between the abundance of Proteobacteria with MOM consumptionCitation59 and the fortification with a bovine milk-based fortifier,Citation46 as well as a negative association between MOM consumption and the abundance of Firmicutes.Citation59 A study conducted by La Rosa et al. reported that across time, there is a linear relationship between the abundance of Gammaproteobacteria and MOM consumption.Citation48 et al. found a decrease in the abundance of Lactobacillus with exposure to human milk (MOM and/or DHM).Citation50 Butcher et al. followed PT infants that were exclusively fed MOM and identified that these infants were mainly colonized by Bacilli, Clostridia, and Gammaproteobacteria, with very low levels of Bifidobacterium.Citation37 In contrast, different studies showed that exposure to MOM was associated with greater abundances of bacteria of the class Lactobacillales,Citation33 Bacillales,Citation33 Bifidobacteriales,Citation33 and Clostridiales,Citation33,Citation57 and higher abundance of the genera Bifidobacterium, Acinetobacter and Haemophilus.Citation78 One study compared the gut colonization specifically by species of the genus Staphylococcus in PT infants exclusively fed MOM.Citation80 Results showed that infants fed MOM had a lower presence of Staphylococcus aureus, Staphylococcus hominis, and Staphylococcus lugdunesis compared to full-term infants.Citation80 Some of these differences could be attributed to the composition of human milk. Underwood et al. reported that PT infants consuming MOM of secretor mothers (expressing 2′-fucosyltransferase) that produce milk containing the human milk oligosaccharides (HMOs), 2′-fucosyllactose and lactodifucotetraose, had a lower abundance of Gammaproteobacteria and higher abundance of Lactobacillaceae.Citation81 Another observational study analyzed the gut microbial composition of PT infants and of the milk they were fed.Citation68 The authors reported that when infants consumed MOM with a high abundance of Staphylococcus, they harbored a gut microbiota rich in Staphylococcus.Citation68 In contrast, infants that consumed MOM high in Rothia, Enterococcus, and Streptococcus developed a gut microbiota with higher abundances of Bifidobacterium.Citation68
Studies also reported differences in the gut microbiota based on DHM and PT formula consumption. Preterm infants that were fed DHM had higher abundances of Enterobacteriales,Citation33 Staphylococcus, Clostridium, Coprococcus, Aggregatibacter, and Lactobacillus.Citation78 If infants were exclusively fed PT formula, they had a greater abundance of Lactobacillales,Citation57 Enterobacteriales,Citation33,Citation57 and ClostridialesCitation57 compared to those fed human milk. At genus level, PT formula consumption was positively associated with the abundance of Blautia, Streptococcus, Acidaminococcus, Rothia, Dorea,Citation78 Terrisporobacter and Peptoclostridium.Citation46 An observational study concluded that the best discriminators of the gut microbiota of PT infants fed MOM were bacteria of the genus Citrobacter, Clostridium, Ruminococcus, and Negativicoccus, whereas the best discriminators of infants consuming PT formula were Streptococcus, Bacillus, and Anaerococcus.Citation57
Although there are differences in gut microbiota composition depending on the type of milk consumed, PT infants are likely to be fed more than one type of milk at a time. As previously mentioned, a study evaluating different feeding patterns found that when infants were fed DHM and/or PT formula, they had increased levels of Enterobacteriales compared to other feeding groups and combinations.Citation33 Infants consuming MOM in combination with PT formula, had the highest enrichment of Bifidobacteriales.Citation33 Furthermore, studies also observed differences in the gut microbiota depending on the type of fortification to human milk. Cai et al. described a decrease in Veillonella with a bovine milk fortifier in infants consuming MOM.Citation46 Another study found that PT infants fed MOM with human milk-based fortifier had a higher abundance of Gammaproteobacteria and a lower abundance of Bacillales.Citation28 Additionally, an increase in Bifidobacterium was observed if PT infants were fed MOM with the addition of a human milk-based fortifier.Citation28 In contrast, Butcher et al. did not find changes in the gut microbiota of PT infants when they received MOM fortified with a bovine milk-based fortifier.Citation37
Prebiotics and probiotics
Fourteen studies described the effect of prebiotics or probiotics in the gut microbiota of PT infants, presented in (). These studies were focused on supplementation with prebiotics,Citation28,Citation69,Citation82,Citation83 probiotics,Citation27,Citation29,Citation30,Citation44,Citation84–86 or both.Citation87 The prebiotics tested on PT infants were fructooligosaccharides (FOS) from clover honey,Citation82 galactooligosaccharides (GOS) + FOS,Citation83 GOS vs. HMOs,Citation28 or GOS + FOS + acidic oligosaccharides (AOS).Citation69 Publications related to prebiotic supplementation did not report differences in alpha or beta diversity subsequent to supplementation. However, several taxonomic differences were found with prebiotic supplementation. When PT infants received FOS alone, there was a decrease in Enterobacteriaceae and an increase in Bifidobacterium and Lactobacillus.Citation88 With the supplementation of GOS + FOS, the abundance of Lactobacillus increased over time; however, the abundance of Lactobacillus was not significantly different than the control group.Citation83 Despite the changes mentioned above, Westerbeek et al. did not find any significant changes in the gut colonization of PT infants after intervention with a mixture of GOS + FOS + AOS.Citation69 Underwood et al. reported an increase in Clostridia with increasing doses of either GOS or HMOs added to PT formula compared to PT formula without these prebiotics.Citation28
The probiotics that were supplemented in the studies included in this review include: Infloran® (Lactobacillus acidophilus + Bifidobacterium bifidum),Citation27,Citation53 a mixture of Bifidobacterium breve + Bifidobacterium longum subsp. infantis + Bifidobacterium longum subsp. Longum,Citation84 single strain administration of Bifidobacterium breve,Citation73,Citation84 Bifidobacterium longum BB536 + Lactobacillus rhamnosus GG,Citation86 Lactobacillus rhamnosus,Citation85 Bifidobacterium breve M16-B,Citation29 Bifidobacterium longum subsp. infantis or Bifidobacterium animals subsp. lactis,Citation30 or Dierol® (Saccharomyces boulardii).Citation44 After supplementation with Infloran®, there was an increase in the alpha diversity, measured by Shannon diversity index.Citation27 Both publications supplementing Infloran® to PT infants reported an increase in Lactobacillus and Bifidobacterium after treatment.Citation27,Citation53 Additionally, in PT infants supplemented with Infloran®, there was an increase Escherichia, along with a decrease in Veillonella, and Streptococcus at 28 days postnatal age.Citation53 The treatment with B. breve showed no impact on the alpha diversity (measured by Simpson diversity index) after supplementation.Citation73 Administration of B. breve alone or in with B. infants + B. longum increased the abundances of Bifidobacterium in the gut of PT infants.Citation84 When infants received B. longum + L. rhamnosus there was an increase in Bifidobacterium as well as in Lactobacillus.Citation86 Underwood et al. found an increase in the Shannon diversity index when formula-fed PT infants received B. animalis subsp. lactis.Citation30 This study also found an increase in Bifidobacterium after supplementation with B. longum subsp infantis in infants consuming PT formula; however, this increase was even greater in PT infants receiving the supplementation while consuming MOM.Citation30 There was no dose-response in Bifidobacterium abundance in the group supplemented with B. animalis subs lactis.Citation30 Overall, the supplementation with probiotics decreased the presence of Proteobacteria,Citation30 Enterobacteriaceae,Citation84 and Clostridium histolyticum.Citation85 A study found that after supplementation with B. breve, the gut microbiota of some PT infants had less than 6% abundance of Bifidobacterium; these infants were described as non-responders.Citation29 Additionally, these infants actually had increased abundances of Enterobacteriaceae and Clostridiaceae.Citation29 After supplementation with Dierol®, there was a decrease in the alpha diversity (measured by Simpson diversity index) overtime. However, this reduction was not significantly different than the one showed in the placebo group.Citation44 The authors found no association between probiotic supplementation with Dierol® and the gut microbiota beta diversity. Dierol® supplementation was associated with an increase in Veillonella, Clostridium, and Bifidobacterium, as well as a decrease in Enterococcus and Pseudomonas.Citation44
One study aimed to analyze the effect of the combination of prebiotic and probiotics. Preterm infants were exposed to Culturelle® (L. rhamnosus GG + inulin) or to ProBioPlus DDS® (L. acidophilus + B. longum + B. bifidum + B. infantis + inulin). Over four weeks of supplementation, infants exposed to Culturelle® showed a decrease in Gram-negative bacteria, whereas supplementation with ProBioPlus DDS® significantly increased Bifidobacterium and Lactobacillus over the same 4-week period.Citation87
Environmental factors
NICU environment
Recently, studies have aimed to analyze the effect of the hospital and NICU environment on the gut colonization of PT infants. Four studies, summarized in (), reported associations related to the gut microbiota and the hospitalization period. Tauchi et al. conducted a longitudinal observational study that followed PT infants during their time spent in the NICU.Citation55 Results from this study showed that there was a positive association between the abundance of Bifidobacteriaceae and the infant transition from an incubator to an open bed.Citation55 On the other hand, La Rosa et al. performed a similar analysis where they concluded that there was no association between the gut microbiota composition of infants that were housed in a single room or an open room with multiple subjects.Citation48
Table 6. Environmental factors and gut microbiota composition of PT infants
Two studies conducted by Brooks and collaborators compared the characteristics of the gut microbiota of PT infants with the room environment at the NICU.Citation89,Citation90 These studies consisted of collecting stool samples from the infants as wells as medical equipment and surface samples from the NICU room they were housed. These surface samples consisted of the most frequently touched surfaces in the NICU: medical equipment, floors, sinks, computer equipment, counters, coolers, ceilings, and cell phones. An overlap between specific bacteria strains present in the infant’s gut and the NICU surfaces was found, specifically for Staphylococcus and Enterococcus faecalis.Citation89 When they analyzed specific items, they found that the tubing system had the highest abundance of bacteria colonizing the infant’s gut, and the electronics had the lowest abundance.Citation89 The other study conducted by the same group, found similar results. Up to twelve bacterial species were shared between the microbiota of the infant’s gut and NICU surfaces.Citation90 The species that were more common to overlap between the NICU surfaces and the infant’s gut were Enterococcus faecalis, Staphylococcus epidermis, Klebsiella pneumoniae, Propionibacterium avidu, Escherichia coli, Pseudomonas aeruginosa, and to a lesser extent Staphylococcus aureus, Serratia marcescens, Rothia mucilaginosa, Citrobacter freundii, Streptococcus agalactieae and Prevotella bivia.Citation90 Interestingly, although Clostridia is a common colonizer of PT infants gut, this bacteria was rarely found in the NICU room surfaces.Citation90
Discussion
The goal of this review was to investigate the perinatal, physiological, dietary, pharmacological, and environmental factors that influence the establishment of the gut bacterial communities in PT infants. A total of 60 publications met the inclusion criteria, reporting changes in alpha diversity, beta diversity, and taxonomic composition of the gut microbiota in response to the various physiological and environmental parameters experienced by PT infants (). Nutritional inputs (milk and fortifiers) constituted the largest component of the evidence base.
Figure 2. Multifactorial colonization of the preterm gut
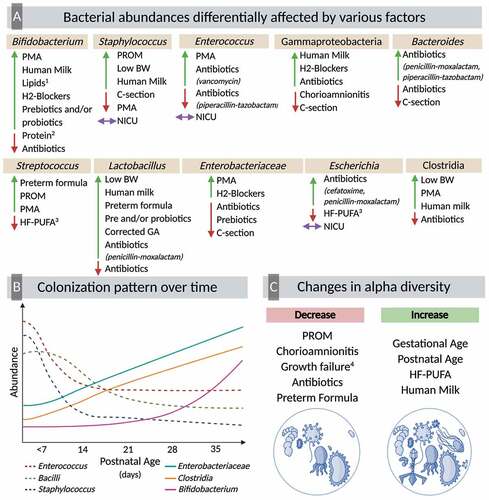
Modifications in the gut microbiota of PT infants could begin during pregnancy and delivery. The prevailing paradigm in obstetrics has been the sterile womb hypothesis. However, studies have identified the presence of bacteria in the amniotic fluid, placenta, umbilical cord,Citation91,Citation92 and meconium of PT,Citation93 and full-term infants.Citation94 This suggests that colonization of the gastrointestinal tract begins in utero.Citation95 However, several groups have brought into question whether the detected microbes represent microbial contamination.Citation96,Citation97 Two recent studies using microbial culture, qPCR, and DNA sequencing found a lack of evidence for microbes in placental or fetal tissue of rhesus monkeysCitation98 or mice.Citation99 Nonetheless, PT infants are often exposed to pregnancy-related complications, such as PROM and chorioamnionitis, which can induce PT delivery. These complications were associated with decreased diversity and increased abundance of Gammaproteobacteria, Staphylococcus, Streptococcus, Serratia, and Parabacteroides.Citation32,Citation33,Citation35
In full-term infants, significant differences in fecal microbiota have been reported depending on the mode of delivery,Citation100 and these modifications can persist up to one year postpartum.Citation101 Many studies in this review reported differences in the structure of the gut microbiota of PT infants depending on delivery mode. Although there was marked variability in the findings related to alpha diversity and beta-diversity, taxonomically, there was a more consistent trend. Vaginal delivery was consistently associated with the presence of Bacteroides.Citation44,Citation49–51 This observation is in accordance with previous reports showing that full-term infants born via C-section have low Bacteroides abundance.Citation102 This increase in abundance of Bacteroides in vaginally-delivered infants might be attributed to maternal’s fecal microbiota rather that the vaginal microbiota.Citation103 The lack of consistency in the results could attributed to the high prevalence of C-section deliveries in PT infants (31%-64%).Citation6,Citation7 Thus, further research is needed to clarify the results.
Different physiological factors were explored in this review, including ethnicity, sex, weight, and age. Few studies reported the effect of genetic factors like ethnicity and sex affecting the gut microbiota of PT infants.Citation33–35,Citation42 There was not a significant trend that could be drawn from these results. Although previous literature has reported possible differences in the gut microbiota associated with sexCitation104 and ethnicity,Citation105 insufficient data exists in newborns. There is a strong relationship between PT birth and low birth weight which can affect the fecal microbiota composition. Low birth weight appeared to be associated with higher abundances of Lactobacillales and Clostridiales.Citation57 However, these results could be in part explained by the feeding regimen these infants were exposed to, since these findings came from PT infants fed PT formula. In fact, evidence has shown that there are higher abundances of Clostridium and Lactobacillus in full-term infants fed formula.Citation106 In addition to low birth weight, postnatal growth failure is a common feature in PT infants. A study reported the association between diversity, microbiota maturity and growth failure.Citation58 A low microbiota-for-age Z-score was found to be prevalent in PT infants.Citation58 In children, the microbiota-for-age metric has been linked to modifications in the taxonomical composition related to malnutrition.Citation107
In PT infants, both GA at birth and postnatal age are associated with modifications in the structure of the gastrointestinal microbiota. Throughout the literature review, there was consistent evidence of an increase in diversity with greater GA at birth and postnatal age. The most notable changes over time were a decrease in Enterococcus, Bacilli and Staphylococcus and an increase in Enterobacteriaceae, Clostridia and Bifidobacterium. It is important to highlight that the colonization with Bifidobacterium appears to be delayed in PT infants compared to full-term infants.Citation55 Studies in full-term infants have shown that the gut bacterial communities are characterized by low diversity after birth which increases over time and is influenced by dietary factors such as breastfeeding and weaning.Citation108 Immediately after birth, the primary gut colonizers are facultative anaerobic bacteria, which reduce the oxygen content of the gut to allow for the subsequent colonization with obligate anaerobes.Citation109 Clostridium is a strict anaerobe, and some of its members, particularly those from Clostridium cluster 1, are associated with prematurity and NEC.Citation110 In contrast, the late acquisition of Bifidobacterium in PT infants, another strict anaerobe, could be attributed to the lower exposure to human milk compared to full-term infants. The colonization with Bifidobacterium has been significantly associated with breastfeeding and human milk consumption in newborns.Citation111
The strong relationship between diet and fecal microbiota composition is a well-known fact. Human milk is the gold standard for infant nutrition and plays an essential role in the gut bacterial colonization. The review of the literature showed that in PT infants, this is not an exception. Overall, the consumption of human milk, particularly MOM was associated with greater presence of BifidobacteriumCitation33,Citation68,Citation78 and Staphylococcus.Citation68,Citation78 This association could be explained in part to the microbiota composition of human milk. Studies have found that the microbiota composition of human milk is rich primarily in StaphylococcusCitation112,Citation113 and Bifidobacterium.Citation112 Nonetheless, there was no consistency across studies between types of milk (human milk [MOM or DHM] or PT formula) and taxonomic composition of the fecal microbiota of PT infants. This could be explained, in part, by the variety of feeding strategies PT infants are exposed to during the hospitalization period. Rates of breastfeeding are lower in PT infants compared to full-term infants;Citation114 mothers that deliver prematurely may have little or no milk production caused by immaturity in the mammary gland, illness, or stress.Citation114 In the case that PT infants do not receive their MOM, they will be fed DHM or PT formula. Differences in the composition of DHM and PT formula could result in very different gastrointestinal colonization patterns in PT infants. Furthermore, if PT infants are fed MOM or DHM, this will be supplemented with a milk fortifier (human milk-based or bovine milk-based) to achieve adequate nutritional composition and meet the newborn’s needs.Citation115 These fortifiers likely further alter gut microbiota composition. With these diverse feeding possibilities, significant and consistent changes will be less likely to be found. For instance, although Bifidobacterium is associated with human milk consumption, one study reported a decrease of this bacteria when PT infants were exposed to MOM fortified with a bovine milk fortifier.Citation28 This underlines the importance of studies analyzing the gut microbiota taking into account and reporting detailed information regarding the infant’s diet. Additionally, the majority of studies from this literature review were related to dietary factors focused on human milk and/or fortifiers, and very few considered the effect of macronutrients.Citation64,Citation76 Results from two studies showed that the protein content, lipid content,Citation64 and lipid supplementationCitation76 of the diet are associated with the fecal microbiota of PT infants, but more well controlled RCT are needed to further explore these conclusions.
Several studies from this review reported changes in the gut microbiota with the consumption of prebiotics and probiotics, whether used separately or in combination. The most commonly prebiotics administrated were FOS and GOS, and the most common probiotics were B. breve, B. longum, L. acidophilus, and L. rhamnosus. Most of the significant modifications in the gut microbiota of PT infants were observed with the use of probiotics. As expected, there was a noticeable increase in Bifidobacterium and Lactobacillus in the infant’s gut with the use of probiotics. Interestingly, the use of probiotics together with human milk (particularly MOM) had an additive effect in increasing Bifidobacterium abundance.Citation30 Human milk is rich in HMOs,Citation116 which are indigestible carbohydrates that are utilized by members of the genera Bifidobacterium.Citation117 This could explain the differences between PT formula-fed and PT infants exposed to MOM. The use of prebiotics and/or probiotics has shown to decrease colic episodes, decrease fecal pH, improve feeding tolerance and gastric motility, and reduce the risk of allergies.Citation118 Extensive research has shown the beneficial effect of probiotics therapy in the reduction of NEC and death in infants born preterm.Citation119 However, evidence is still lacking regarding the short- and long-term effects that these probiotics have in the fecal microbiota in PT infants.
The use of antibiotics and the effect they have on the gut microbiota was widely reported across the literature. During hospitalization in the NICU, PT infants are exposed to a variety of medications and antibiotics. It has been reported that up to 89% of preterm infants received antibiotics after birth.Citation120 Across the literature, studies found modifications in the diversity and the taxonomical composition of the gut microbiota populations in PT infants after the exposure to antibiotics. Even though antibiotics are prescribed to reduce the number of pathogenic bacteria, the literature review showed this comes accompanied by a decrease in beneficial commensal bacteria like Bifidobacterium. The shifts in the overall structure of the gut microbiota are important for the host’s health in the sense that they could cause perturbations in the innate and adaptive immune system.Citation121 This is something particularly significant for infants in a fragile state such as PT infants. Moreover, shifts in the gut microbiota appeared to be temporal. This goes in accordance with previous reports showing the transient modifications in the gut microbiota caused by antibiotics exposure, albeit the alteration in the immune system can still occur.Citation122 Furthermore, antibiotic-associated alterations in the gut microbiota seem to be dependent on the type of antibiotic. One study reported opposite effects in the abundance of Enterococcus; this bacteria decreased with aminoglycoside and increased with vancomycin.Citation38 Although vancomycin is used to treat gastrointestinal infections, vancomycin-resistant Enterococcus is common nowadays and can be the cause of serious infections in older population.Citation123 Antibiotic-resistant bacteria infections have become a public health concern, and data have shown that infants can be colonized with antibiotic-resistant bacteria early in life.Citation124 This colonization with antibiotic-resistant bacteria could be coming from environmental, dietary, or maternal factors.Citation124 Further exploration of these associations should be conducted to understand the shifts in the bacteria communities of the PT gut. This review also aimed to describe the effect of all commonly administered medications on the gut microbiota composition of PT infants. However, only reports on antibiotics and H2-blockers were found and included in the results. Since PT infants are routinely exposed to a variety of medications during their stay in the NICU, which may modulate the microbiota composition and/or function, additional research that investigates the impact of these other medications is warranted.
Finally, we considered the relationship that exists between the living environment and the gut microbiota. Although the evidence is scarce, two different studies demonstrated associations between the housing environment (incubator or bed, and single vs. open rooms) with the structure of the gut microbiota of PT infants.Citation48,Citation55 Only one study found an effect between the infant transition from an incubator to an open bed and colonization with Bifidobacteriaceae.Citation55 Two different studies, reported how the same bacteria strains were colonizing both the PT gut and many surfaces from the NICU.Citation89,Citation90 These similarities between housing environment and the microbiota from different parts of the human body have been previously studied. It has been hypothesized that humans might serve as vectors among multiple room surfaces, and thus, the colonization can be bi-directional.Citation125 Hospital-acquired infections are strongly associated with the diversity of microorganisms found in this environment.Citation126 This is particularly relevant for PT infants, since these infants are more likely to spend extended periods of time in the NICU. In this environment, a variety of surfaces could serve as sources of microorganisms, including incubators, ventilators, warmers, electronic equipment, as well as health care providers.Citation22
This review considers the multifactorial colonization of the PT gut, however, there are some limitations worth mentioning. One limitation of this review is the heterogeneity in the methods for the assessment and analysis of the gut microbiota across studies. As shown Supplementary Table 1, the most common method used was NGS (47 studies); from these studies, three performed whole genome sequencing, whereas the rest used 16S rRNA sequencing. Other studies used bacterial culture, molecular methods and other non-sequencing methods such as qPCR, DGGE, TGGE, T-RFLP, PFGE, FISH, MALDI-TOF, and microarrays. The main limitation of bacterial culture and non-sequencing methods is an imprecise characterization of the microbiota diversity.Citation127 Also, studies that utilize these methods usually target specific bacteria to answer specific questions, rather than assessing the gut microbiota in a broader way.Citation127,Citation128 From the studies that used NGS, there were also differences on the platforms used: pyrosequencing, Illumina dye sequencing or pH-mediated sequencing. The main differences between these techniques are related to read length, reads per run, and reads retained after filtering; where platforms like Illumina will yield more reads and longer reads than the other two platforms.Citation129 Thus, this variation in gut microbiota assessment could create biases in the results that have been reported in this review.
Lastly, the available literature regarding the gut microbiota of PT infants relied predominantly on observational studies with very few clinical trials, suggesting the need for more intervention RCTs with adequate power and sample size calculations. After birth, PT infants are at a fragile stage and require a variety of medical interventions. The exposure to different dietary and pharmacological factors will depend on the health status of the infant; this makes it challenging to conduct RCTs in this population. From the review of the literature, almost all the studies of prebiotics and/or probiotics supplementation were clinical trials. These microbiome modulating strategies have been widely used in PT infants and recently, the European Society for Pediatric Gastroenterology Hepatology and Nutrition (ESPGHAN) recommended additional RCT to study the effect of probiotics in infants born preterm.Citation130
Conclusions and future directions
Results from this literature review about the multifactorial colonization in the PT infant gut highlights how multiple factors and different exposures can differently modify the abundance or presence of bacteria from the same genera or class, as shown in (). Although changes in numerous bacteria were found across perinatal, physiological, dietary, pharmacological, and environmental factors, some bacteria consistently showed differences across the mentioned factors. These bacteria included Bifidobacterium, Staphylococcus, Enterococcus, Gammaproteobacteria, Bacteroides, Streptococcus, Lactobacillus, Enterobacteriaceae, Escherichia and Clostridia. The results of this systematic review also illustrate the variability in some of the associations that have been reported with the gut microbiota, which highlights the need of more comprehensive studies analyzing the effect of mode of delivery, sex, type of milk consumed, use of fortifiers, and use of medications on the composition of the gut microbiota of PT infants. Infants born preterm most likely will be affected by multiple conditions at the same time including C-section delivery, antibiotics exposure, low birth weight, and different feeding regimes. With the rapid advancement in sequencing technologies, such as long-read 16S rRNA sequencing that allow for a deeper resolution of the gut microbiome, coupled with the use of more sophisticated computational tools, future biomedical research should aim to integrate multiple biological inputs, seeking to understand complex systems such as the gut microbiota of PT infants. From a systems biology perspective, this would encompass studying the associations between bacterial genome, infant’s metabolome, immune markers, clinical status, dietary factors, and the effect on the infant health outcomes. Robust associations support the need for prospective RCTs to utilize modifiable factors, such as diet, to mitigate the adverse effects of non-modifiable factors, including low GA or low birth weight, to help prevent or ameliorate detrimental complications associated with the common dysbiosis associated with PT birth.
Supplemental Material
Download MS Word (159.9 KB)Supplementary material
Supplemental data for this article can be accessed on the publisher’s website.
Additional information
Funding
References
- Hasan N, Yang H. Factors affecting the composition of the gut microbiota, and its modulation. PeerJ [Internet]. 2019;7:e7502. https://pubmed.ncbi.nlm.nih.gov/31440436
- Deschasaux M, Bouter KE, Prodan A, Levin E, Groen AK, Herrema H, Tremaroli V, Bakker GJ, Attaye I, Pinto-Sietsma S-J, et al. Depicting the composition of gut microbiota in a population with varied ethnic origins but shared geography. Nat Med [Internet]. 2018;24(10):1526–33. doi:10.1038/s41591-018-0160-1.
- Stinson LF, Payne MS, Keelan JA, Critical A. Review of the bacterial baptism hypothesis and the impact of cesarean delivery on the infant microbiome. Front Med [Internet]. 2018;5:135. doi:10.3389/fmed.2018.00135.
- Stewart CJ, Ajami NJ, O’Brien JL, Hutchinson DS, Smith DP, Wong MC, Ross MC, Lloyd RE, Doddapaneni H, Metcalf GA, et al. Temporal development of the gut microbiome in early childhood from the TEDDY study. Nature [Internet]. 2018;562(7728):583–588. doi:10.1038/s41586-018-0617-x.
- Rinninella E, Raoul P, Cintoni M, Franceschi F, Miggiano G, Gasbarrini A, Mele M. What is the healthy gut microbiota composition? A changing ecosystem across age, environment, diet, and diseases. Microorganisms [Internet]. 2019;7(1):14. doi:10.3390/microorganisms7010014.
- Thanh BYL, Lumbiganon P, Pattanittum P, Laopaiboon M, Vogel JP, Oladapo OT, Pileggi-Castro C, Mori R, Jayaratne K, Qureshi Z, et al. Mode of delivery and pregnancy outcomes in preterm birth: a secondary analysis of the WHO Global and Multi-country Surveys. Sci Rep [Internet]. 2019;9(1):15556. doi:10.1038/s41598-019-52015-w.
- Stoll BJ, Hansen NI, Bell EF, Walsh MC, Carlo WA, Shankaran S, Laptook AR, Sanchez PJ, Van Meurs KP, Wyckoff M, et al. Trends in care practices, morbidity, and mortality of extremely preterm Neonates, 1993-2012. JAMA. 2015;314(10):1039–1051. doi:10.1001/jama.2015.10244.
- Boerma T, Ronsmans C, Melesse DY, Barros AJD, Barros FC, Juan L, Moller A-B, Say L, Hosseinpoor AR, Yi M, et al. Global epidemiology of use of and disparities in caesarean sections. Lancet (London, England). 2018;392(10155):1341–1348. doi:10.1016/S0140-6736(18)31928-7.
- Morrow CB, McGrath-Morrow SA, Collaco JM. Predictors of length of stay for initial hospitalization in infants with bronchopulmonary dysplasia. J Perinatol [Internet]. 2018;38(9):1258–1265. doi:10.1038/s41372-018-0142-7.
- Hay WW. Nutritional support strategies for the preterm infant in the neonatal intensive care unit. Pediatr Gastroenterol Hepatol Nutr [Internet]. 2018;21(4):234. doi:10.5223/pghn.2018.21.4.234.
- Su B-H. Optimizing nutrition in preterm infants. Pediatr Neonatol [Internet]. 2014;55(1):5–13. doi:10.1016/j.pedneo.2013.07.003.
- Gagliardi A, Totino V, Cacciotti F, Iebba V, Neroni B, Bonfiglio G, Trancassini M, Passariello C, Pantanella F, Schippa S. Rebuilding the gut microbiota ecosystem. Int J Environ Res Public Health [Internet]. 2018;15(8):1679. doi:10.3390/ijerph15081679.
- Schwartz S, Friedberg I, Ivanov IV, Davidson LA, Goldsby JS, Dahl DB, Herman D, Wang M, Donovan SM, Chapkin R. A metagenomic study of diet-dependent interaction between gut microbiota and host in infants reveals differences in immune response. Genome Biol [Internet]. 2012;13(4):R32. doi:10.1186/gb-2012-13-4-r32.
- Lu J, Claud EC. Connection between gut microbiome and brain development in preterm infants. Dev Psychobiol [Internet]. 2019;61(5):739–751. doi:10.1002/dev.21806.
- Carding S, Verbeke K, Vipond DT, Corfe BM, Owen LJ. Dysbiosis of the gut microbiota in disease. Microb Ecol Heal Dis [Internet]. 2015;26:26191. http://www.microbecolhealthdis.net/index.php/mehd/article/view/26191
- Collado MC, Cernada M, Neu J, Pérez-Martínez G, Gormaz M, Vento M. Factors influencing gastrointestinal tract and microbiota immune interaction in preterm infants. Pediatr Res. 2015;77(6):726–731. doi:10.1038/pr.2015.54.
- Forsgren M, Isolauri E, Salminen S, Rautava S. Late preterm birth has direct and indirect effects on infant gut microbiota development during the first six months of life. Acta Paediatr [Internet]. 2017;[ accesssed 2019 May 6]. 106(7):1103–1109. doi:10.1111/apa.13837
- Tirone C, Pezza L, Paladini A, Tana M, Aurilia C, Lio A, D’Ippolito S, Tersigni C, Posteraro B, Sanguinetti M, et al. Gut and lung microbiota in preterm infants: immunological modulation and implication in neonatal outcomes. Front Immunol [Internet] 2019;10:2910. doi:10.3389/fimmu.2019.02910.
- Niemarkt HJ, De Meij TG, van Ganzewinkel C, de Boer NKH, Andriessen P, Hütten MC, Kramer BW. Necrotizing enterocolitis, gut microbiota, and brain development: role of the brain-gut axis. Neonatology. [Internet] 2019; accessed 2020 Apr 20]; 115(4):423–431. doi:10.1159/000497420
- Dierikx TH, Visser DH, Benninga MA, van Kaam AHLC, de Boer NKH, de Vries R, van Limbergen J, de Meij TGJ. The influence of prenatal and intrapartum antibiotics on intestinal microbiota colonisation in infants: A systematic review. J Infect [Internet]. 2020;81(2):190–204. doi:10.1016/j.jinf.2020.05.002.
- Xu W, Judge MP, Maas K, Hussain N, McGrath JM, Henderson WA, Cong X. Systematic Review of the Effect of Enteral Feeding on Gut Microbiota in Preterm Infants. J Obstet Gynecol Neonatal Nurs [Internet]. 2018; accessed 2019 May 6]; 47(3):451–463. doi:10.1016/j.jogn.2017.08.009
- Hartz LE, Bradshaw W, Brandon DH. Potential NICU Environmental Influences on the Neonate’s Microbiome. Adv Neonatal Care [Internet]. 2015;15:324–335. doi:10.1097/ANC.0000000000000220.
- Moher D, Shamseer L, Clarke M, Ghersi D, Liberati A, Petticrew M, Shekelle P, Stewart LA. Preferred reporting items for systematic review and meta-analysis protocols (PRISMA-P) 2015 statement. Syst Rev [Internet]. 2015;4(1):1. doi:10.1186/2046-4053-4-1.
- Huang X, Fan X, Ying J, Chen S. Emerging trends and research foci in gastrointestinal microbiome. J Transl Med [Internet]. 2019;17(1):67. doi:10.1186/s12967-019-1810-x.
- Sterne JAC, Savović J, Page MJ, Elbers RG, Blencowe NS, Boutron I, Cates CJ, Cheng H-Y, Corbett MS, Eldridge SM, et al. RoB 2: a revised tool for assessing risk of bias in randomised trials. BMJ [Internet]. 2019;366:l4898. doi:10.1136/bmj.l4898.
- Wells G. The Newcastle-Ottawa Scale (NOS) for assessing the quality of nonrandomised studies in meta-analyses [Internet]. [accessed 2019 May 6]: http://www.ohri.ca/programs/clinical_epidemiology/oxford.asp
- Abdulkadir B, Nelson A, Skeath T, Marrs ECL, Perry JD, Cummings SP, Embleton ND, Berrington JE, Stewart CJ. Routine use of probiotics in preterm infants: longitudinal impact on the microbiome and metabolome. Neonatology [Internet]. 2016; accessed 2019 May 6]; 109(4):239–247. doi:10.1159/000442936
- Underwood MA, Kalanetra KM, Bokulich NA, Mirmiran M, Barile D, Tancredi DJ, German JB, Lebrilla CB, Mills DA. Prebiotic oligosaccharides in premature infants. J Pediatr Gastroenterol Nutr [Internet]. 2014;58(3):352–360. doi:10.1097/MPG.0000000000000211.
- Underwood MA, Davis JCC, Kalanetra KM, Gehlot S, Patole S, Tancredi DJ, Mills DA, Lebrilla CB, Simmer K. Digestion of human milk oligosaccharides by bifidobacterium breve in the premature infant. J Pediatr Gastroenterol Nutr [Internet]. 2017; accessed 2019 May 6]; 65(4): 449–455. doi:10.1097/MPG.0000000000001590
- Underwood MA, Kalanetra KM, Bokulich NA, Lewis ZT, Mirmiran M, Tancredi DJ, Mills DAA. Comparison of two probiotic strains of bifidobacteria in premature infants. J Pediatr [Internet]. 2013;163(6):1585–1591.e9. doi:10.1016/j.jpeds.2013.07.017.
- Sherman MP, Sherman J, Arcinue R, Niklas V Randomized control trial of human recombinant lactoferrin: a substudy reveals effects on the fecal microbiome of very low birth weight infants. J Pediatr [Internet]. 2016 [ accessed 2019 May 6]:173:S37–42. doi:10.1016/j.jpeds.2016.02.074
- Chernikova DA, Koestler DC, Hoen AG, Housman ML, Hibberd PL, Moore JH, Morrison HG, Sogin ML, Zain-ul-abideen M, Madan JC. Fetal exposures and perinatal influences on the stool microbiota of premature infants. J Matern Neonatal Med [Internet]. 2016; accessed 2019 May 6]; 29(1):99–105. doi:10.3109/14767058.2014.987748
- Cong X, Judge M, Xu W, Diallo A, Janton S, Brownell EA, Maas K, Graf J. Influence of feeding type on gut microbiome development in hospitalized preterm infants. Nurs Res [Internet]. 2017;66(2):123–133. doi:10.1097/NNR.0000000000000208.
- Zwittink RD, Renes IB, van Lingen RA, van Zoeren-grobben D, Konstanti P, Norbruis OF, Martin R, Groot Jebbink LJM, Knol J, Belzer C. Association between duration of intravenous antibiotic administration and early-life microbiota development in late-preterm infants. Eur J Clin Microbiol Infect Dis [Internet]. 2018; accessed 2019 May 6]; 37(3):475–483. doi:10.1007/s10096-018-3193-y
- Ho TTB, Groer MW, Kane B, Yee AL, Torres BA, Gilbert JA, Maheshwari A. Dichotomous development of the gut microbiome in preterm infants. Microbiome [Internet]. 2018; accessed 2019 May 6]; 6(1):157. doi:10.1186/s40168-018-0547-8
- Laurence M, Hatzis C, Brash DE Common contaminants in next-generation sequencing that hinder discovery of low-abundance microbes. PLoS One [Internet] 2014 [ accessed 2020 Aug 10]; 9(5):e97876. doi:10.1371/journal.pone.0097876
- Butcher J, Unger S, Li J, Bando N, Romain G, Francis J, Mottawea W, Mack D, Stintzi A, O’Connor DL. Independent of birth mode or gestational age, very-low-birth-weight infants fed their mothers’ milk rapidly develop personalized microbiotas low in bifidobacterium. J Nutr [Internet]. 2018; accessed 2019 May 6]; 148(3):326–335. doi:10.1093/jn/nxx071
- Korpela K, Blakstad EW, Moltu SJ, Strømmen K, Nakstad B, Rønnestad AE, Brække K, Iversen PO, Drevon CA, de Vos W. Intestinal microbiota development and gestational age in preterm neonates. Sci Rep [Internet] 2018; accessed 2019 May 6]; 8(1):2453. doi:10.1038/s41598-018-20827-x
- Mshvildadze M, Neu J, Mai V. Intestinal microbiota development in the premature neonate: establishment of a lasting commensal relationship? Nutr Rev [Internet] 2008; accessed 2019 May 6]; 66(11):658–663. doi:10.1111/j.1753-4887.2008.00119.x
- Stewart CJ, Embleton ND, Clements E, Luna PN, Smith DP, Fofanova TY, Nelson A, Taylor G, Orr CH, Petrosino JF, et al. Cesarean or vaginal birth does not impact the longitudinal development of the gut microbiome in a cohort of exclusively preterm infants. Front Microbiol [Internet] 2017;8:1008. doi:10.3389/fmicb.2017.01008.
- Wandro S, Osborne S, Enriquez C, Bixby C, Arrieta A, Whiteson K The microbiome and metabolome of preterm infant stool are personalized and not driven by health outcomes, including necrotizing enterocolitis and late-onset sepsis. mSphere [Internet]. 2018; 3(3) doi:10.1128/mSphere.00104-18
- Zhou Y, Shan G, Sodergren E, Weinstock G, Walker WA, Gregory KE. Longitudinal analysis of the premature infant intestinal microbiome prior to necrotizing enterocolitis: a case-control study. PLoS One [Internet]. 2015;10(3):e0118632. doi:10.1371/journal.pone.0118632.
- Patel AL, Mutlu EA, Sun Y, Koenig L, Green S, Jakubowicz A, Mryan J, Engen P, Fogg L, Chen AL, et al. Longitudinal survey of microbiota in hospitalized preterm very-low-birth-weight infants. J Pediatr Gastroenterol Nutr [Internet] 2016 [ accessed 2019 May 6]; 62(2):292–303. doi:10.1097/MPG.0000000000000913
- Zeber-Lubecka N, Kulecka M, Ambrozkiewicz F, Paziewska A, Lechowicz M, Konopka E, Majewska U, Borszewska-Kornacka M, Mikula M, Cukrowska B, et al. Effect of Saccharomyces boulardii and mode of delivery on the early development of the gut microbial community in preterm infants. PLoS One [Internet] 2016 [ accessed 2019 May 6]; 11(2):e0150306. doi:10.1371/journal.pone.0150306
- Zwittink RD, van Zoeren-grobben D, Martin R, van Lingen RA, Groot Jebbink LJ, Boeren S, Renes IB, van Elburg RM, Belzer C, Knol J. Metaproteomics reveals functional differences in intestinal microbiota development of preterm infants. Mol Cell Proteomics [Internet]. 2017; accessed 2019 May 6]; 16(9):1610–1620. doi:10.1074/mcp.RA117.000102
- Cai C, Zhang Z, Morales M, Wang Y, Khafipour E, Friel J. Feeding practice influences gut microbiome composition in very low birth weight preterm infants and the association with oxidative stress: A prospective cohort study. Free Radic Biol Med [Internet]. 2019;142:146–154. doi:10.1016/j.freeradbiomed.2019.02.032.
- Gupta RW, Tran L, Norori J, Ferris MJ, Eren AM, Taylor CM, Dowd SE, Penn D. Histamine-2 receptor blockers alter the fecal microbiota in premature infants. J Pediatr Gastroenterol Nutr. [Internet] 2013;56(4):397–400. doi:10.1097/MPG.0b013e318282a8c2.
- La Rosa PS, Warner BB, Zhou Y, Weinstock GM, Sodergren E, Hall-Moore CM, Stevens HJ, Bennett WE, Shaikh N, Linneman LA, et al. Patterned progression of bacterial populations in the premature infant gut. Proc Natl Acad Sci [Internet] 2014 [ accessed 2019 May 6]; 111(34):12522–12527. doi:10.1073/pnas.1409497111
- Arboleya S, Martinez-Camblor P, Solís G, Suárez M, Fernández N, de Los Reyes-gavilán CG. Gueimonde M. Intestinal microbiota and weight-gain in preterm neonates. Front Microbiol [Internet]. 2017;8. http://journal.frontiersin.org/article/10.3389/fmicb.2017.00183/full.
- Chernikova DA, Madan JC, Housman ML, Zain-ul-abideen M, Lundgren SN, Morrison HG, Sogin ML, Williams SM, Moore JH, Karagas MR, et al. The premature infant gut microbiome during the first 6 weeks of life differs based on gestational maturity at birth. Pediatr Res [Internet]. 2018;84(1):71–79. doi:10.1038/s41390-018-0022-z.
- Gregory KE, LaPlante RD, Shan G, Kumar DV, Gregas M. Mode of birth influences preterm infant intestinal colonization with bacteroides over the early neonatal period. Adv Neonatal Care [Internet]. 2015; accessed 2019 May 6]; 15(6):386–393. doi:10.1097/ANC.0000000000000237
- Sim K, Shaw AG, Randell P, Cox MJ, McClure ZE, Li M-S, Haddad M, Langford PR, Cookson WOCM, Moffatt MF, et al. Dysbiosis Anticipating Necrotizing Enterocolitis in Very Premature Infants. Clin Infect Dis. [Internet] 2015;60(3):389–397. doi:10.1093/cid/ciu822.
- Esaiassen E, Hjerde E, Cavanagh JP, Pedersen T, Andresen JH, Rettedal SI, Støen R, Nakstad B, Willassen NP, Klingenberg C Effects of probiotic supplementation on the gut microbiota and antibiotic resistome development in preterm infants. Front Pediatr [Internet] 2018 [ accessed 2019 May 6]; 6. doi:10.3389/fped.2018.00347
- Ravi A, Estensmo ELF, Abée-Lund TML, Foley SL, Allgaier B, Martin CR, Claud EC, Rudi K. Association of the gut microbiota mobilome with hospital location and birth weight in preterm infants. Pediatr Res. [Internet] 2017; accessed 2019 May 6]; 82(5):829–838. doi:10.1038/pr.2017.146
- Tauchi H, Yahagi K, Yamauchi T, Hara T, Yamaoka R, Tsukuda N, Watanabe Y, Tajima S, Ochi F, Iwata H, et al. Gut microbiota development of preterm infants hospitalised in intensive care units. Benef Microbes. [Internet] 2019;10(6):641–651. doi:10.3920/BM2019.0003.
- Brown CT, Xiong W, Olm MR, Thomas BC, Baker R, Firek B, Morowitz MJ, Hettich RL, Banfield JF.Hospitalized premature infants are colonized by related bacterial strains with distinct proteomic profiles. MBio. [Internet] 2018;9(2). doi:10.1128/mBio.00441-18.
- Gregory KE, Samuel BS, Houghteling P, Shan G, Ausubel FM, Sadreyev RI, Walker WA. Influence of maternal breast milk ingestion on acquisition of the intestinal microbiome in preterm infants. Microbiome. [Internet] 2016; accessed 2019 May 6]; 4(1):68. doi:10.1186/s40168-016-0214-x
- Younge NE, Newgard CB, Cotten CM, Goldberg RN, Muehlbauer MJ, Bain JR, Stevens RD, O’Connell TM, Rawls JF, Seed PC, et al. Disrupted maturation of the microbiota and metabolome among extremely preterm infants with postnatal growth failure. Sci Rep. [Internet] 2019;9(1):8167. doi:10.1038/s41598-019-44547-y.
- Dahl C, Stigum H, Valeur J, Iszatt N, Lenters V, Peddada S, Bjørnholt JV, Midtvedt T, Mandal S, Eggesbø M. Preterm infants have distinct microbiomes not explained by mode of delivery, breastfeeding duration or antibiotic exposure. Int J Epidemiol. [Internet] 2018;47(5):1658–1669. doi:10.1093/ije/dyy064.
- Gibson MK, Wang B, Ahmadi S, Burnham C-AD, Tarr PI, Warner BB, Dantas G. Developmental dynamics of the preterm infant gut microbiota and antibiotic resistome. Nat Microbiol. [Internet] 2016; accessed 2019 May 6]; 1(4):16024. doi:10.1038/nmicrobiol.2016.24
- Gómez M, Moles L, Espinosa-Martos I, Bustos G, de Vos W, Fernández L, Rodríguez J, Fuentes S, Jiménez E. Bacteriological and immunological profiling of meconium and fecal samples from preterm infants: a two-year follow-up study. Nutrients. [Internet] 2017; accessed 2019 May 6]; 9(12):1293. doi:10.3390/nu9121293
- Costello EK, Carlisle EM, Bik EM, Morowitz MJ, Relman DA Microbiome assembly across multiple body sites in low-birthweight infants. MBio [Internet] 2013 [ accessed 2019 May 6]; 4(6). doi:10.1128/mBio.00782-13
- Mai V, Torrazza RM, Ukhanova M, Wang X, Sun Y, Li N, Shuster J, Sharma R, Hudak ML, Neu J. Distortions in development of intestinal microbiota associated with late onset sepsis in preterm infants. PLoS One. [Internet] 2013;8(1):e52876. doi:10.1371/journal.pone.0052876.
- Grier A, Qiu X, Bandyopadhyay S, Holden-Wiltse J, Kessler HA, Gill AL, Hamilton B, Huyck H, Misra S, Mariani TJ, et al. Impact of prematurity and nutrition on the developing gut microbiome and preterm infant growth. Microbiome [ Internet] 2017 [ accessed 2019 May 6]; 5(1):158. doi:10.1186/s40168-017-0377-0
- Arboleya S, Sánchez B, Milani C, Duranti S, Solís G, Fernández N, de Los Reyes-gavilán CG, Ventura M, Margolles A, Gueimonde M. Intestinal microbiota development in preterm neonates and effect of perinatal antibiotics. J Pediatr. [Internet] 2015;166(3):538–544. doi:10.1016/j.jpeds.2014.09.041.
- Moles L, Gómez M, Heilig H, Bustos G, Fuentes S, de Vos W, Fernández L, Rodríguez JM, Jiménez E. Bacterial diversity in meconium of preterm neonates and evolution of their fecal microbiota during the first month of life. PLoS One. [Internet] 2013;8(6):e66986. doi:10.1371/journal.pone.0066986.
- Normann E, Fahlén A, Engstrand L, Lilja HE. Intestinal microbial profiles in extremely preterm infants with and without necrotizing enterocolitis. Acta Paediatr. [Internet] 2013;102(2):129–136. doi:10.1111/apa.12059.
- Biagi E, Aceti A, Quercia S, Beghetti I, Rampelli S, Turroni S, Soverini M, Zambrini AV, Faldella G, Candela M, et al. Microbial community dynamics in mother’s milk and infant’s mouth and gut in moderately preterm infants. Front Microbiol. [Internet] 2018;9:2512. doi:10.3389/fmicb.2018.02512.
- Westerbeek EAM, Slump RA, Lafeber HN, Knol J, Georgi G, Fetter WPF, Elburg RM. The effect of enteral supplementation of specific neutral and acidic oligosaccharides on the faecal microbiota and intestinal microenvironment in preterm infants. Eur J Clin Microbiol Infect Dis. [Internet] 2013;32(2):269–276. doi:10.1007/s10096-012-1739-y.
- Zhu D, Xiao S, Yu J, Ai Q, He Y, Cheng C, Zhang Y, Pan Y. Effects of one-week empirical antibiotic therapy on the early development of gut microbiota and metabolites in preterm infants. Sci Rep. [Internet] 2017; accessed 2019 May 6]; 7(1):8025 doi:10.1038/s41598-017-08530-9
- Zou Z-H, Liu D, Li H-D, Zhu D-P, He Y, Hou T, Yu J-L. Prenatal and postnatal antibiotic exposure influences the gut microbiota of preterm infants in neonatal intensive care units. Ann Clin Microbiol Antimicrob. [Internet] 2018; accessed 2019 May 6]; 17(1):9. doi:10.1186/s12941-018-0264-y
- Butel M-J, Suau A, Campeotto F, Magne F, Aires J, Ferraris L, Kalach N, Leroux B, Dupont C. Conditions of bifidobacterial colonization in preterm infants: a prospective analysis. J Pediatr Gastroenterol Nutr. [Internet] 2007;44(5):577–582. doi:10.1097/MPG.0b013e3180406b20.
- Millar M, Seale J, Greenland M, Hardy P, Juszczak E, Wilks M, Panton N, Costeloe K, Wade WG The microbiome of infants recruited to a randomised placebo-controlled probiotic trial (PiPS Trial). EBioMedicine [Internet] 2017 [ accessed 2019 May 6]; 20:255–262. doi:10.1016/j.ebiom.2017.05.019
- Moles L, Gómez M, Jiménez E, Fernández L, Bustos G, Chaves F, Cantón R, Rodríguez JM, Del Campo R Preterm infant gut colonization in the neonatal ICU and complete restoration 2 years later. Clin Microbiol Infect [Internet] 2015 [ accessed 2019 May 6]; 21(10):936.e1-936.e10. doi:10.1016/j.cmi.2015.06.003
- Syal K, Mo M, Yu H, Iriya R, Jing W, Guodong S, Wang S, Grys TE, Haydel SE, Tao N. Current and emerging techniques for antibiotic susceptibility tests. Theranostics. [Internet] 2017;7(7):1795–1805. doi:10.7150/thno.19217.
- Younge N, Yang Q, Seed PC Enteral high fat-polyunsaturated fatty acid blend alters the pathogen composition of the intestinal microbiome in premature infants with an enterostomy. J Pediatr [Internet] 2017 [ accessed 2019 May 6]; 181:93–101.e6. doi:10.1016/j.jpeds.2016.10.053
- Mshvildadze M, Neu J, Shuster J, Theriaque D, Li N, Mai V. Intestinal microbial ecology in premature infants assessed with non–culture-based techniques. J Pediatr. [Internet] 2010;156(1):20–25. doi:10.1016/j.jpeds.2009.06.063.
- Parra-Llorca A, Gormaz M, Alcántara C, Cernada M, Nuñez-Ramiro A, Vento M, Collado MC. Preterm gut microbiome depending on feeding type: significance of donor human milk. Front Microbiol. [Internet] 2018;9:1376. doi:10.3389/fmicb.2018.01376.
- Poroyko V, Morowitz M, Bell T, Ulanov A, Wang M, Donovan S, Bao N, Gu S, Hong L, Alverdy JC, et al. Diet creates metabolic niches in the “immature gut” that shape microbial communities. Nutr Hosp. 2011;26(6):1283–1295. doi:10.1590/S0212-16112011000600015.
- Soeorg H, Metsvaht T, Eelmäe I, Merila M, Treumuth S, Huik K, Jürna-Ellam M, Ilmoja M-L, Lutsar I. The role of breast milk in the colonization of neonatal gut and skin with coagulase-negative staphylococci. Pediatr Res. [Internet] 2017; accessed 2019 May 6]; 82(5):759–767. doi:10.1038/pr.2017.150
- Underwood MA, Gaerlan S, De Leoz MLA, Dimapasoc L, Kalanetra KM, Lemay DG, German JB, Mills DA, Lebrilla CB. Human milk oligosaccharides in premature infants: absorption, excretion, and influence on the intestinal microbiota. Pediatr Res. [Internet] 2015; accessed 2019 May 6]; 78(6):670–677. doi:10.1038/pr.2015.162
- Aly H, Said RN, Wali IE, Elwakkad A, Soliman Y, Awad AR, Shawky MA, Alam MSA, Mohamed MA. Medically Graded Honey Supplementation Formula to Preterm Infants as a Prebiotic. J Pediatr Gastroenterol Nutr. [Internet] 2017; accessed 2019 May 6]; 64(6):966–970. doi:10.1097/MPG.0000000000001597
- Armanian A-M, Sadeghnia A, Hoseinzadeh M, Mirlohi M, Feizi A, Salehimehr N, Torkan M, Shirani Z. The effect of neutral oligosaccharides on fecal microbiota in premature infants fed exclusively with breast milk: A randomized clinical trial. J Res Pharm Pract. [Internet] 2016;5(1):27. doi:10.4103/2279-042X.176558.
- Ishizeki S, Sugita M, Takata M, Yaeshima T. Effect of administration of bifidobacteria on intestinal microbiota in low-birth-weight infants and transition of administered bifidobacteria: A comparison between one-species and three-species administration. Anaerobe. [Internet] 2013;23:38–44. doi:10.1016/j.anaerobe.2013.08.002.
- Pärtty A, Luoto R, Kalliomäki M, Salminen S, Isolauri E. Effects of early prebiotic and probiotic supplementation on development of gut microbiota and fussing and crying in preterm infants: a randomized, double-blind, placebo-controlled trial. J Pediatr. [Internet] 2013;163(5):1272–1277.e2. doi:10.1016/j.jpeds.2013.05.035.
- Rougé C, Piloquet H, Butel M-J, Berger B, Rochat F, Ferraris L, Des Robert C, Legrand A, de la Cochetière M-F, N’Guyen J-M, et al. Oral supplementation with probiotics in very-low-birth-weight preterm infants: a randomized, double-blind, placebo-controlled trial. Am J Clin Nutr [Internet] 2009 [ accessed 2019 May 6]; 89(6):1828–1835. doi:10.3945/ajcn.2008.26919
- Underwood MA, Salzman NH, Bennett SH, Barman M, Mills DA, Marcobal A, Tancredi DJ, Bevins CL, Sherman MP. A randomized placebo-controlled comparison of 2 prebiotic/probiotic combinations in preterm infants: impact on weight gain, intestinal microbiota, and fecal short-chain fatty acids. J Pediatr Gastroenterol Nutr. [Internet] 2009;48(2):216–225. doi:10.1097/MPG.0b013e31818de195.
- Rozé J-C, Ancel P-Y, Lepage P, Martin-Marchand L, Al Nabhani Z, Delannoy J, Picaud J-C, Lapillonne A, Aires J, Durox M, et al. Nutritional strategies and gut microbiota composition as risk factors for necrotizing enterocolitis in very-preterm infants. Am J Clin Nutr [Internet] 2017 [ accessed 2019 May 6]; 106(3):821–830. doi:10.3945/ajcn.117.152967
- Brooks B, Firek BA, Miller CS, Sharon I, Thomas BC, Baker R, Morowitz MJ, Banfield JF. Microbes in the neonatal intensive care unit resemble those found in the gut of premature infants. Microbiome. 2014 [Internet];2(1):1. doi:10.1186/2049-2618-2-1.
- Brooks B, Olm MR, Firek BA, Baker R, Thomas BC, Morowitz MJ, Banfield JF. Strain-resolved analysis of hospital rooms and infants reveals overlap between the human and room microbiome. Nat Commun. [Internet] 2017; accessed 2019 May 6]; 8(1):1814. doi:10.1038/s41467-017-02018-w
- Aagaard K, Ma J, Antony KM, Ganu R, Petrosino J, Versalovic J. The Placenta Harbors a Unique Microbiome. Sci Transl Med. [Internet] 2014;6(237):237ra65–237ra65. doi:10.1126/scitranslmed.3008599.
- Jiménez E, Fernández L, Marín ML, Martín R, Odriozola JM, Nueno-Palop C, Narbad A, Olivares M, Xaus J, Rodríguez JM. Isolation of commensal bacteria from umbilical cord blood of healthy neonates born by cesarean section. Curr Microbiol. [Internet] 2005;51(4):270–274. doi:10.1007/s00284-005-0020-3.
- Morais J, Marques C, Teixeira D, Durão C, Faria A, Brito S, Cardoso M, Macedo I, Pereira E, Tomé T, et al. Extremely preterm neonates have more Lactobacillus in meconium than very preterm neonates – the in utero microbial colonization hypothesis. Gut Microbes. [Internet]. 2020. 1–9. https://www.tandfonline.com/doi/full/10.1080/19490976.2020.1785804
- Collado MC, Rautava S, Aakko J, Isolauri E, Salminen S. Human gut colonisation may be initiated in utero by distinct microbial communities in the placenta and amniotic fluid. Sci Rep. [Internet] 2016;6(1):23129. doi:10.1038/srep23129.
- Walker RW, Clemente JC, Peter I, Loos RJF. The prenatal gut microbiome: are we colonized with bacteria in utero ? Pediatr Obes. [Internet] 2017;12:3–17. http://doi.wiley.com/10.1111/ijpo.12217
- Glassing A, Dowd SE, Galandiuk S, Davis B, Chiodini RJ. Inherent bacterial DNA contamination of extraction and sequencing reagents may affect interpretation of microbiota in low bacterial biomass samples. Gut Pathog. [Internet] 2016;8(1):24. doi:10.1186/s13099-016-0103-7.
- Perez-Muñoz ME, Arrieta M-C, Ramer-Tait AE, Walter J. A critical assessment of the “sterile womb” and “in utero colonization” hypotheses: implications for research on the pioneer infant microbiome. Microbiome. [Internet] 2017;5(1):48. doi:10.1186/s40168-017-0268-4.
- Theis KR, Romero R, Winters AD, Jobe AH, Gomez-Lopez N. Lack of evidence for microbiota in the placental and fetal tissues of rhesus macaques. mSphere. [Internet] 2020;5(3):e00210–20. doi:10.1128/mSphere.00210-20.
- Theis KR, Romero R, Greenberg JM, Winters AD, Garcia-Flores V, Motomura K, Ahmad MM, Galaz J, Arenas-Hernandez M, Gomez-Lopez N. No consistent evidence for microbiota in murine placental and fetal tissues. mSphere. [Internet] 2020;5(1):e00933–19. doi:10.1128/mSphere.00933-19.
- Bäckhed F, Roswall J, Peng Y, Feng Q, Jia H, Kovatcheva-Datchary P, Li Y, Xia Y, Xie H, Zhong H, et al. Dynamics and stabilization of the human gut microbiome during the first year of life. Cell Host Microbe. [Internet] 2015;17(5):690–703. doi:10.1016/j.chom.2015.04.004.
- Rutayisire E, Huang K, Liu Y, Tao F. The mode of delivery affects the diversity and colonization pattern of the gut microbiota during the first year of infants’ life: a systematic review. BMC Gastroenterol. [Internet] 2016;16(1):86. doi:10.1186/s12876-016-0498-0.
- Shao Y, Forster SC, Tsaliki E, Vervier K, Strang A, Simpson N, Kumar N, Stares MD, Rodger A, Brocklehurst P, et al. Stunted microbiota and opportunistic pathogen colonization in caesarean-section birth. Nature. [Internet] 2019;574(7776):117–121. doi:10.1038/s41586-019-1560-1.
- Mitchell CM, Mazzoni C, Hogstrom L, Bryant A, Bergerat A, Cher A, Pochan S, Herman P, Carrigan M, Sharp K, et al. Delivery mode affects stability of early infant gut microbiota. Cell Reports Med. 2020;1(9):100156. doi:10.1016/j.xcrm.2020.100156.
- Kim YS, Unno T, Kim B-Y, Park M-S. Sex differences in gut microbiota. World J Mens Health. [Internet] 2020;38(1):48. doi:10.5534/wjmh.190009.
- McBurney MI, Davis C, Fraser CM, Schneeman BO, Huttenhower C, Verbeke K, Walter J, Latulippe ME. Establishing what constitutes a healthy human gut microbiome: state of the science, regulatory considerations, and future directions. J Nutr. [Internet] 2019;149(11):1882–1895. doi:10.1093/jn/nxz154.
- Gritz EC, Bhandari V. The human neonatal gut microbiome: a brief review. Front Pediatr. [Internet] 2015;3:17. http://www.frontiersin.org/Neonatology/10.3389/fped.2015.00017/abstract
- Subramanian S, Huq S, Yatsunenko T, Haque R, Mahfuz M, Alam MA, Benezra A, DeStefano J, Meier MF, Muegge BD, et al. Persistent gut microbiota immaturity in malnourished Bangladeshi children. Nature. [Internet] 2014;510(7505):417–421. doi:10.1038/nature13421.
- Robertson RC, Manges AR, Finlay BB, Prendergast AJ. The human microbiome and child growth – First 1000 days and beyond. Trends Microbiol. 2019 [Internet];27(2):131–147. doi:10.1016/j.tim.2018.09.008.
- Penders J, Thijs C, Vink C, Stelma FF, Snijders B, Kummeling I, van den Brandt PA, Stobberingh EE. Factors influencing the composition of the intestinal microbiota in early infancy. Pediatrics. [Internet] 2006;118(2):511–521. doi:10.1542/peds.2005-2824.
- Schönherr-Hellec S, Aires J. Clostridia and necrotizing enterocolitis in preterm neonates. Anaerobe. [Internet] 2019;58:6–12. doi:10.1016/j.anaerobe.2019.04.005.
- Davis EC, Dinsmoor AM, Wang M, Donovan SM. Microbiome composition in pediatric populations from birth to adolescence: impact of diet and prebiotic and probiotic interventions. Dig Dis Sci. [Internet] 2020;65(3):706–722. doi:10.1007/s10620-020-06092-x.
- Zimmermann P, Curtis N. Breast milk microbiota: A review of the factors that influence composition. J Infect. [Internet] 2020;81(1):17–47. doi:10.1016/j.jinf.2020.01.023.
- Sakwinska O, Bosco N. Host microbe interactions in the lactating mammary gland. Front Microbiol. [Internet] 2019;10:1863. doi:10.3389/fmicb.2019.01863.
- Bonet M, Blondel B, Agostino R, Combier E, Maier RF, Cuttini M, Khoshnood B, Zeitlin J. Variations in breastfeeding rates for very preterm infants between regions and neonatal units in Europe: results from the MOSAIC cohort. Arch Dis Child - Fetal Neonatal Ed. [Internet] 2011;96(6):F450–2. doi:10.1136/adc.2009.179564.
- Gartner LM, Morton J, Lawrence RA, Naylor AJ, O’Hare D, Schanler RJ, Eidelman AI. Breastfeeding and the use of human milk. Pediatrics. [Internet] 2005;115:496–506. http://pediatrics.aappublications.org/cgi/doi/10.1542/peds.2004–2491
- Boquien C-Y. Human Milk: an Ideal Food for Nutrition of Preterm Newborn. Front Pediatr. [Internet] 2018;6:295. doi:10.3389/fped.2018.00295.
- Underwood MA, German JB, Lebrilla CB, Mills DA. Bifidobacterium longum subspecies infantis: champion colonizer of the infant gut. Pediatr Res. [Internet] 2015; accessed 2019 May 6]; 77(1–2):229–235. doi:10.1038/pr.2014.156
- Sohn K, Underwood MA. Prenatal and postnatal administration of prebiotics and probiotics. Semin Fetal Neonatal Med. [Internet] 2017; accessed 2019 May 13]; 22(5):284–289. doi:10.1016/j.siny.2017.07.002
- Sawh SC, Deshpande S, Jansen S, Reynaert CJ, Jones PM. Prevention of necrotizing enterocolitis with probiotics: a systematic review and meta-analysis. PeerJ. [Internet] 2016;4:e2429. doi:10.7717/peerj.2429.
- Hsieh MH, Versalovic J. The human microbiome and probiotics: implications for pediatrics. Curr Probl Pediatr Adolesc Health Care. [Internet] 2008;38(10):309–327. doi:10.1016/j.cppeds.2008.09.001.
- Francino MP. Antibiotics and the human gut microbiome: dysbioses and accumulation of resistances. Front Microbiol. [Internet] 2016;6:1543. doi:10.3389/fmicb.2015.01543.
- Zeissig S, Blumberg RS. Life at the beginning: perturbation of the microbiota by antibiotics in early life and its role in health and disease. Nat Immunol. [Internet] 2014;15(4):307–310. doi:10.1038/ni.2847.
- Levitus M, Rewane APT. Vancomycin-Resistant Enterococci (VRE). [Internet] Stat Pearls accessed 2020 Jun 16]; 2020;https://www.ncbi.nlm.nih.gov/books/NBK513233/.
- Zhang L, Kinkelaar D, Huang Y, Li Y, Li X, Wang HH. Acquired antibiotic resistance: are we born with it? Appl Environ Microbiol. [Internet] 2011;77(20):7134–7141. doi:10.1128/AEM.05087-11.
- Kelley ST, Gilbert JA. Studying the microbiology of the indoor environment. Genome Biol. [Internet] 2013;14(2):202. doi:10.1186/gb-2013-14-2-202.
- Rampelotto PH, Sereia AFR, de Oliveira LFV, Margis R. Exploring the Hospital Microbiome by High-Resolution 16S rRNA Profiling. Int J Mol Sci. [Internet] 2019;20(12):3099. doi:10.3390/ijms20123099.
- Costa M, Weese JS. Methods and basic concepts for microbiota assessment. Vet J. [Internet] 2019;249:10–15. doi:10.1016/j.tvjl.2019.05.005.
- Allaband C, McDonald D, Vázquez-Baeza Y, Minich JJ, Tripathi A, Brenner DA, Loomba R, Smarr L, Sandborn WJ, Schnabl B, et al. Microbiome 101: studying, analyzing, and interpreting gut microbiome data for clinicians. Clin Gastroenterol Hepatol [Internet] 2019; 17(2)::218–230. doi:10.1016/j.cgh.2018.09.017.
- Allali I, Arnold JW, Roach J, Cadenas MB, Butz N, Hassan HM, Koci M, Ballou A, Mendoza M, Ali R, et al. A comparison of sequencing platforms and bioinformatics pipelines for compositional analysis of the gut microbiome. BMC Microbiol. 2017;17(1):194. doi:10.1186/s12866-017-1101-8.
- van den Akker CHP, van Goudoever JB, Shamir R, Domellöf M, Embleton ND, Hojsak I, Lapillonne A, Mihatsch WA, Berni Canani R, Bronsky J, et al. Probiotics and preterm infants. J Pediatr Gastroenterol Nutr [Internet] 2020 [ accessed 2020 Jun 16]; 70(5):664–680. doi:10.1097/MPG.0000000000002655