ABSTRACT
Human noroviruses are the most common viral cause of acute gastroenteritis worldwide. Currently, there are no approved vaccines or specific therapeutics to treat the disease. Some obstacles delaying the development of a norovirus vaccine are: (i) the extreme diversity presented by noroviruses; (ii) our incomplete understanding of immunity to noroviruses; and (iii) the lack of a robust cell culture system or animal model for human noroviruses. Recent advances in in vitro cultivation of norovirus, novel approaches applied to viral genomics and immunity, and completion of vaccine trials and birth cohort studies have provided new information toward a better understanding of norovirus immunity. Here, we will discuss the complex relationship between norovirus diversity and correlates of protection for human noroviruses, and how this information could be used to guide the development of cross-protective vaccines.
Introduction
Noroviruses are small, icosahedral, non-enveloped, positive-sense, single-stranded RNA viruses that infect different mammal species. The human norovirus genome is ~7.5 kb in length and is divided into three open reading frames (ORF1-3). Upon entry into susceptible cells, the ORF1 is immediately translated as a polyprotein that is processed co- and post-translationally by the viral protease to yield six nonstructural (NS) proteins required for viral replication: NS1/2 (N-term), NS3 (helicase), NS4 (3A-like), NS5 (VPg), NS6 (protease), and NS7 (RNA-dependent RNA polymerase, RdRp). ORF2, which encodes the major capsid protein VP1, and ORF3, which encodes the minor capsid protein VP2, are translated from the subgenomic RNA. The viral capsid presents a T = 3 icosahedral structure consisting of 90 VP1 dimers, and an undetermined quantity of VP2 (, panel A).Citation1,Citation2 Heterologous expression of VP1 leads to the self-assembly of virus-like particles (VLPs), which are structurally- and antigenically similar to the native virion, although viruses and VLPs with different sizes and symmetries have also been described.Citation3–5 In the absence of a robust cell culture system for viral cultivation, VLPs have been instrumental in vaccine research.
Figure 1. Structure and variable antigenic sites of the human norovirus major capsid protein, VP1. (a) The norovirus capsid is composed of 90 dimers of VP1 arranged in a T = 3 icosahedral symmetry. (b) The VP1 protein is divided into the conserved Shell (S) and the variable Protruding (P) domains. The P domain is further divided into the P1 and P2 subdomains. The surface-exposed P2 subdomain is thought to dictate binding to the cellular attachment factors, histo-blood group antigen (HGBA) carbohydrates (highlighted in green), while the S domain forms the core of the viral particle.Citation2 (c) The known variable antigenic sites (A, C, D, E, and G) located on the surface of the P2 subdomain of GII.4 norovirus are highlighted. The structural models were rendered using UCSF Chimera (version 1.11.2) and the following Protein Data Bank (PDB) files: 1IHM and 2ZLE (Norwalk virus, GI.1) and 2OBS (VA387 virus, GII.4 Grimsby variant). (d) Residues mapping on the variable antigenic sites of GII.4 noroviruses. Changes on these sites correlate with the emergence of GII.4 variants. (e) Amino acid variation in the P domain of the VP1 protein and its correlation with GII.4 variant distribution was quantified with Shannon entropy (left) and adjusted Rand index (right). Dataset includes sequences collected from 1995 to 2016, as described in Tohma et al., 2019.Citation70 Entropy values were calculated using the Shannon Entropy-One tool, as implemented in Los Alamos National Laboratory (www.hiv.lanl.gov) for six major GII.4 variants. The boxplot shows mean and standard deviation from each antigenic and non-antigenic site of major variants and all-in sequences that included a maximum of 50 randomly subsampled strain/variant to reduce sampling bias (n = 474). The dotted line indicates the mean of entropy values from non-antigenic sites in the subsampled all-in dataset. Adjusted Rand index, in which higher index values indicate a higher degree of correlation between variant distribution and the amino acid variation, was calculated with the subsampled all-in dataset using R and presented in a dot plot. Each circle represents the index from each antigenic and non-antigenic site
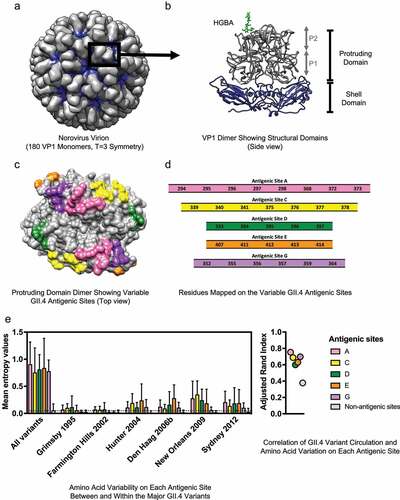
Upon infection, the VP1 protein is the major target of B-cell-mediated immune responses, and thus is the primary focus of vaccine development.Citation6,Citation7 The VP1 protein is structurally divided into the Shell (S) domain and the Protruding (P) domain (, panel B).Citation2 Since the S domain is highly conserved across different genotypes, most antigenic sites mapping on this domain are cross-reactive.Citation8,Citation9 Unfortunately, most data indicate that protective antibodies map to the variable P domain.Citation10–14 The P domain is further divided into the P1 and P2 subdomains. The P1 subdomain is partially conserved, while the P2 subdomain contains the highest sequence variability, including highly variable motifs that are involved in cellular attachment and antibody recognition (, panel B).Citation2 Both cross-reactive and neutralizing antibodies have been mapped to the P1 subdomain,Citation12,Citation15 opening opportunities to induce cross-protective responses.
Based on the genetic diversity of VP1, noroviruses are divided into 10 genogroups and almost 50 genotypes.Citation16 Human noroviruses are classified into at least five genogroups that differ by around 40–60% in their amino acid sequences. Genogroups are further subdivided into genotypes that vary between 20% and 40% within each genogroup.Citation17 Although humans can be infected by different noroviruses (>30 genotypes, mainly from Genogroups I and II), viruses from the GII.4 genotype are the major cause of large epidemics worldwide.Citation18,Citation19
Human noroviruses are the leading cause of viral gastroenteritis in the modern world, and are implicated in upwards of 200,000 deaths worldwide, primarily in children from developing countries.Citation20,Citation21 In healthy individuals, norovirus causes acute gastroenteritis (diarrhea and vomiting) that resolves within 24–48 hours, with virus shedding typically lasting between 2 and 8 weeks in the stool.Citation22 However, in vulnerable populations (like the elderly, malnourished children, or immunocompromised individuals), the length and severity of disease is increased. Specifically, in immunocompromised individuals, gastroenteritis symptoms and viral shedding can last months or years.Citation23 In addition to the disease burden, norovirus presents a major impact on the global economy, with around 4.2 USD billion in direct health-care costs and an additional 60.3 USD billion in indirect costs, i.e. loss of productivity due to absenteeism of work or morbidity.Citation24
Based on the natural history of the disease, the general consensus is that a vaccine is the most effective option to mitigate the burden of norovirus disease. Although delayed by major obstacles (i.e. genetic diversity, lack of understanding of immunity, and the absence of a robust cell culture system and animal models), steady progress has been made toward the development of a norovirus vaccine. In this review, we will examine the current knowledge on correlates of protection for human norovirus from the perspective of viral genetic and antigenic diversity, and how this information can be used to design potential cross-protective vaccines.
The correlates of protection for human noroviruses
A combination of both host genetic factors and immunological responses likely influences norovirus susceptibility, replication, and protection. One of the genetic factors contributing to norovirus susceptibility is the secretor status, which is associated with the presence of histo-blood group antigens (HBGAs) on the surface of epithelial cells. Although a definitive cellular receptor has not yet been identified for human noroviruses, HBGAs have been demonstrated to bind to the VP1 protein and facilitate attachment and/or entry into the cell (, panel B).Citation25–27
HBGAs are carbohydrates expressed on the surface of most epithelial cells and are the determinants of the ABO and Lewis blood group systems.Citation28 Biosynthesis of the different HBGAs is dependent upon multiple glycosyltransferase enzymes, which are responsible for the stepwise addition of monosaccharides onto a precursor carbohydrate molecule. Two of these glycosyltransferases, the fucosyltransferase 1 and 2 (FUT1 and FUT2) enzymes catalyze the addition of a fucose moiety onto the disaccharide precursor via α1,2 linkage, producing the H antigen, which can be further modified.Citation29 Mutations that inactivate the FUT2 gene result in the secretor negative phenotype in humans, which has been shown to provide resistance to infection by certain norovirus genotypes.Citation26,Citation30–33 Recently, stem-cell derived human intestinal enteroids (HIE) have been shown to be susceptible to some human norovirus strains.Citation34 Using HIE, it has been shown that the expression of a functional FUT2 enzyme in the HIE was necessary for attachment and infection of GI.1 and some GII genotypes,Citation27 confirming the relevance of genetic factors to norovirus susceptibility.
Due to the inherent ability of certain norovirus genotypes to bind to HBGA carbohydrates, a carbohydrate blockade assay has been developed in lieu of a cell culture-based neutralization assay. The carbohydrate blockade assay measures the ability of antibodies to block the binding of norovirus VLPs to the HBGA carbohydrates. Several studies have shown that high blockade antibody titers are correlated with disease protection.Citation35,Citation36 Importantly, it has been recently shown that carbohydrate blockade assays and neutralization in HIE present similar patterns in antibody reactivity.Citation11,Citation37 As the HIE culture system exhibits evident disadvantages, such as limited scalability to high-throughput and/or the inability of certain noroviruses to replicate in the cells, the blockade assays are a good alternative to determining the antigenic relationships between strains and to study antibody-mediated protection.Citation11,Citation12,Citation37,Citation38
While some animal models have been developed for human noroviruses,Citation14,Citation39–41 they either do not recapitulate disease or replication has been shown for only a limited number of strains. Thus, human challenge studies have facilitated the study of norovirus immunity. Early studies have shown short-term protection (4–14 weeks post initial challenge) against the homologous virus,Citation42,Citation43 and the lack of cross-protection between heterologous viruses, today identified as GI and GII viruses.Citation44 Protection from disease was associated with high levels of norovirus-specific antibodies before infection.Citation42,Citation43,Citation45,Citation46 A caveat of the early studies is that the viruses used for challenge were not titered,Citation43 so an overwhelming infectious dose, which does not represent natural conditions, could have masked the effect of immunity mounted by previous infections.Citation47
Challenge studies and analyses of natural infections have revealed that both serum and mucosal antibodies are involved in the immune response. An increase in norovirus-specific IgG and IgA has been reported after infection,Citation48 with higher titers of preexisting salivary IgA being correlated with protection.Citation33,Citation45 Interestingly, when compared to norovirus-specific IgG antibodies, a higher proportion of IgA antibodies blocked the binding of norovirus to carbohydrates and exhibited a more potent blockade titer.Citation49 IgAs were also shown to be superior to IgG in terms of murine norovirus (MNV) neutralization in vitro and protective function in vivo.Citation50 Simlarly, a higher percentage of serum IgA antibodies bound to the P domain, which contains neutralizing epitopes, compared to serum IgG, which equally recognized the P domain and the conserved S domain.Citation50 Additional studies are warranted to determine the role of IgA in virus neutralization. In addition to serum and salivary IgA antibodies, fecal IgA also are induced after infection.Citation33,Citation45,Citation51 Although preexisting levels of norovirus-specific fecal IgA were not correlated with protection, in individuals who developed gastroenteritis, fecal IgA levels were inversely correlated with peak viral load in the stool,Citation45 suggesting a role in the control of viral replication. These studies underline both serum and mucosal immunity as major players in the immune response against norovirus.
The impact of norovirus diversity on immunity
Although the genetic diversity of human noroviruses is well established, the antigenic diversity and its role in immunity, especially between genotypes, is less understood. A study that analyzed the cross-reactivity of hyperimmune sera produced from 26 norovirus VLPs, representing around 18 genotypes, showed a variable range of cross-reactivity between the different genotypes via ELISA.Citation52 However, the observed cross-reactivity is likely influenced by non-neutralizing antibodies targeting conserved regions of the VLPs, and thus may not truly represent the functional antigenic relationships between the different genotypes. Preliminary neutralization experiments in HIE and analyses of natural reinfection cases over time suggest that some norovirus genotypes are antigenically distinct from each other.Citation11,Citation17,Citation42,Citation53–55 Despite all these studies, extensive analyses of the functional (blockade/neutralizing) antibodies between all human norovirus genotypes have not been performed.
Another confounding factor is that contrasting evidence on the duration of immunity has been documented. While challenge studies suggest a short duration of protection,Citation42,Citation43 mathematical modeling based on norovirus community transmission suggests that immunity may last up to 9 years.Citation56 Moreover, studies have shown that individuals can be infected with multiple genotypes over the course of their lifetimes.Citation42,Citation51,Citation53–55,Citation57,Citation58 Whether these reinfection patterns are associated with short duration of immunity, genotype antigenic differences, biological properties of emerging viruses, or all of the above have not yet been fully determined.
Based on reinfection patterns recorded in longitudinal and birth cohort studies, and anecdotical reports, human noroviruses were recently clustered into genetically similar groups called “immunotypes,” where infection with a genotype precluded disease with genotypes within the same immunotype.Citation17 This symptomatic reinfection pattern suggests that multiple exposures over a lifetime could provide cross-protective immunity. Indeed, there are data supporting that the history of infection also influences the magnitude and breadth of the immune response. A rapid anamnestic response specific to the initial infecting genotype has been recorded in children and adults.Citation42,Citation51 Moreover, serum IgG and IgA collected from adults infected with norovirus were able to block the binding of VLPs from multiple genotypes to carbohydratesCitation59,Citation60 and immunization with norovirus VLP-based vaccine candidates induces antibodies against heterologous strains,Citation61,Citation62 probably by recalling antibodies specific to prior infections.Citation61 Whether cross-protective antibodies would develop in naïve individuals upon vaccination remains to be determined. Thus, there appears to be a multifaceted relationship between previous infections and cross-protective immune responses, and further studies are warranted to determine whether these genetically related viruses share similar antigenic properties that could result in enhanced cross-protection.
One exception to this pattern of reinfection was repeated infections with GII.4 viruses, the genotype responsible for the majority of cases in humans.Citation18,Citation19 GII.4 reinfections are likely due to the ever-changing nature of this genotype, which results in antigenically distinct variants emerging to cause outbreaks around the world every 2–8 years.Citation18,Citation19 In contrast, non-GII.4 noroviruses appear to be “static” in nature, such that strains that are detected decades apart remain similar at the amino acid level.Citation17 Although certain non-GII.4 noroviruses (GI.3, GII.1, GII.2, GII.6, GII.17, among others) can be subdivided into variants, individual viruses within each variant show limited diversification at the amino acid level.Citation63 Thus, the evolving nature of GII.4 noroviruses needs to be especially considered in vaccine design.
The VP1 antigenic topology of GII.4 norovirus has been extensively characterized. Bioinformatics and experimental analyses (i.e. comparing blockade responses of human sera collected from GII.4 outbreaks or hyperimmune sera produced in experimental animals against VLPs of representative GII.4 variantsCitation64,Citation65) conducted over the past decade have identified five variable antigenic sites that are involved in the chronological emergence of new variants. The precise residues on VP1 involved in each of those variable antigenic sites have been mapped using human and animal monoclonal antibodies (mAbs) (, panel C). Thus, antigenic site A consists of residues 294–298, 368, 372, 373; antigenic site C of 339–341, 375–378; antigenic site D of 393–397; antigenic site E of 407, 411–414; and antigenic site G of 352, 355–357, 359, 364 (, panel D).Citation66–70 Most of these sites are on the loops and/or outer surface of the P2 subdomain where they can interact with neutralizing antibodies and attachment factors. Notably, although the overall structure of the capsid is mostly conserved between the different variants of GII.4, the length varies among genotypes. Thus, careful interpretation of these data should be considered when extrapolating GII.4 antigenic sites to other genotypes.
While the antigenic sites present low levels of intra-variant diversification, most amino acid changes occur at the inter-variant level, suggesting that changes on these residues are important in differentiating the GII.4 variants (, panel E, left). Changes on these antigenic sites, particularly antigenic sites A, C, and G, also correlate with the circulation patterns of GII.4 variants (, panel E, right).Citation70 Notably, while mutations in single antigenic sites can abolish mAbs binding,Citation69,Citation70 large-scale antigenic studies suggest that multiple sites are required for antigenic diversification of GII.4.Citation38 Indeed, structural analyses identified mAbs with footprint spanning multiple antigenic sites, suggesting that the virus may need to change multiple sites to evade the host immune response.Citation13,Citation68,Citation71
The identification and manipulation of immunodominant epitopes are important factors in designing a vaccine that is able to induce functional, protective antibodies against a foreign antigen.Citation72 It was demonstrated that the emergence of the GII.4 New Orleans/2009 variant was associated with changes in the immunodominant antigenic site A, whereby ~40% of blockade antibodies from human outbreak sera were directed against this site.Citation69 Similarly, using polyclonal guinea pig sera, our group showed that antigenic sites A and G from the GII.4 Sydney/2012 variant were both important immunodominant blockade sites.Citation70 Whether these immunodominant sites can induce cross-protective antibodies against multiple strains or if these antibodies are variant-specific remains to be determined. For example, as antigenic site G is more conserved compared to the other major antigenic sites/motifs, antibodies targeting site G could potentially provide protection against multiple GII.4 variants. These immunodominant sites may potentially mask protective epitopes from the immune response as seen in other viruses (e.g. influenza and HIV).Citation73–75
Some studies have predicted additional epitopes that are dependent upon capsid conformation and assembly. Noroviruses, among other viruses, are thought to be dynamic in their structure, i.e. the capsid exists in multiple states. This phenomenon is termed “viral breathing” and is thought to regulate antibody access to epitopes,Citation76 such as the conserved antigenic site F (residues 327 and 404).Citation77,Citation78 In addition, although initial structural studies have determined that the norovirus capsid arranges with a T = 3 icosahedral symmetry, GII.4 norovirus can also assemble into larger particles with T = 4 icosahedral symmetry. These T = 4 particles are made up of 240 copies of VP1 that adopt different conformations that may present unique epitopes.Citation4,Citation5 Interestingly, the formation of T = 4 particles seems restricted to VLPs produced with the baculovirus expression system, as images of native GII.4 virions reveal the presence of particles of the expected size (~30 nm), as well as smaller particles (~18 nm) that are likely T = 1.Citation34 Thus, the role of VLPs with T = 4 icosahedral symmetry for vaccine design should be further explored.
In contrast to GII.4, the evolution and antigenicity of non-GII.4 noroviruses are less understood. Our group recently showed that hyperimmune guinea pig sera produced against various norovirus genotypes exhibited specific blockade and neutralizing titers against homologous genotypes but not against heterologous genotypes,Citation11 suggesting that a cross-protective vaccine would likely need multiple components. Vaccine design may also be complicated by the fact that, similar to GII.4, certain non-GII.4 genotypes (GII.17, GII.6, etc.) can be subdivided into variants.Citation17 Furthermore, although GII.4 is responsible for the majority of outbreaks, non-GII.4 noroviruses can also emerge and predominant in the population. In the winter season of 2014–2015, GII.17 unexpectedly overtook GII.4 to become the predominant genotype in several parts of East Asia.Citation79,Citation80 The epidemic GII.17 noroviruses were shown to be antigenically distinct from previous strains via carbohydrate blockade assays using human and hyperimmune mouse sera.Citation81 Similarly, GII.2 emerged and predominated in several countries in the winter of 2016–2017.Citation82–84 Thus, an ideal vaccine would need to protect against multiple genotypes.
Unlike GII.4 noroviruses, the antigenic sites of non-GII.4 genotypes have not been well defined. Exchanging the amino acid residues 393–396 from the GII.17/2015 outbreak strain to an archival GII.17/1978 strain altered the blockade activity of monoclonal and polyclonal antibodies, suggesting that these residues may be involved in defining the antigenicity of these GII.17 strains.Citation85 In contrast, the antigenicity of GII.2 variants across decades (1976–2010) appears to be similar, as sera collected from humans challenged with GII.2 SMV/1976 were able to block contemporary GII.2 VLPs, although to a lesser extent compared to the homotypic VLP.Citation86 Interestingly, mAbs produced against GII.2 SMV/1976 exhibited blockade titers against a panel of GII.2 strains spanning decades.Citation86 Thus, certain genotypes (e.g. GII.2) appear to be more stable in their antigenic evolution over time.
Current and prospective vaccines
As previously discussed, the extreme diversity of human noroviruses is one of the major hurdles in the development of cross-protective vaccines. Based on data gathered from challenge studies,Citation14,Citation44 current norovirus vaccine candidates are bivalent in formulation, consisting of a GI and a GII component. Two candidates are currently in clinical trials: (i) a VLP-based vaccine administered via the intramuscular route (developed by Takeda Pharmaceuticals) and (ii) an oral vaccine adenovirus vector expressing the VP1 gene (developed by Vaxart, Inc.). Takeda’s VLP-based vaccine candidate was well tolerated in healthy volunteers and induced humoral and mucosal immunity against both antigens, a GI.1 VLP and a consensus GII.4 VLP derived from three different GII.4 variants.Citation62,Citation87 Vaxart recently announced the results of a Phase 1b clinical trial to test the safety and immunogenicity of the vector-based, bivalent (GI.1 + GII.4) vaccine formulation.Citation88 The bivalent vaccine was well tolerated and induced robust IgA responses in the majority of vaccinees with no observed interference. Vaccination with monovalent vectors expressing GI.1 or GII.4 VP1 were also well tolerated and resulted in an increase in antibody titers against the antigens.Citation88,Citation89
In addition to the vaccine candidates in clinical trials, several investigational vaccines are currently being tested in preclinical studies. Many of these clinical and pre-clinical vaccine candidates have been reviewed (see ref.Citation47) and are summarized in . Briefly, a trivalent combination vaccine consisting of a GI.3 VLP, a GII.4 VLP, and a recombinant VP6 protein from rotavirus induced moderate levels of serum IgG and blockade antibodies in immunized mice against homologous and heterologous norovirus and rotavirus strains.Citation90,Citation91 The foundation of this particular vaccine is the recombinant VP6 rotavirus protein, which forms a tubular-like capsule and acts as an adjuvant to the norovirus VLPs.Citation91 Although norovirus VLPs are often produced using the baculovirus expression system,Citation62,Citation92 expression of the VP1 protein in a plant-based tobacco mosaic virus system also results in production of immunogenic VLPs.Citation93 A second adenovirus-vectored vaccine candidate expressing the VP1 of a single GII.4 strain induced mucosal immunity and a balanced cellular Th1/Th2 response in experimental animals.Citation94 In addition to adenovirus, Vesicular Stomatitis virus (VSV) is also used as a vector for expression of norovirus VP1. Immunization of mice with a single dose of recombinant (r) VSV-VP1 resulted in strong serum and mucosal responses, as well as the induction of norovirus-specific T-cells in some animals.Citation95 Finally, another vaccination strategy relies on the singular expression of the P domain of VP1; this results in the formation of highly immunogenic P-particles that are antigenically similar to whole VLPs and that can be used as an alternative to VLP vaccination.Citation96 A study on GII.4 P particles showed that hyperimmune sera in immunized animals was able to block the binding of the GII.4 VLP to carbohydrates, suggesting that the antibodies have potentially neutralizing activity.Citation97 Thus, many potential vaccine candidates utilizing a variety of approaches for human norovirus are being explored. However, it remains to be seen whether the vaccines induce broad protection against the other genotypes, some of which recently have emerged to cause large outbreaks in different countries (i.e. GII.17, GII.2) or in vulnerable populations (i.e. children, the elderly).
Table 1. Current and prospective human norovirus vaccines
Overcoming diversity to develop cross-protective vaccines
One of the goals in vaccine development is the induction of cross-protective antibodies. Studies of the human antibody repertoire following vaccination with the bivalent VLP vaccine candidate revealed three classes of antibodies: (i) antibodies that bound a wide array of genotypes but did not exhibit blockade activity, (ii) antibodies that exhibited binding and blockade activity specifically against the GII.4 consensus VLP and historical GII.4 strains, and (iii) a single mAb – A1431 – that recognized and blocked/neutralized all tested GII.4 strains, including forthcoming variants.Citation12 A1431 was mapped to the cleft between the P1 and P2 subdomains of VP1, which consists of amino acids that are highly conserved within the multiple GII.4 variants. As this site did not overlap with the HBGA binding sites, the mechanism of blockade/neutralization is likely due to steric hindrance.Citation12 Likewise, mouse mAb 5B18 was mapped to a conserved region of the P1 subdomain located near the P1/Shell interface.Citation15,Citation98 Interestingly, the antibody-binding site was buried within the P1 domain and was only transiently exposed to the surface, perhaps due to the “viral breathing” phenomenon or VLP disassembly. Antibodies against such hidden sites may be involved in cross-protective immunity, and further research is warranted to understand how to elicit responses against such hidden epitopes.
Although blockade/neutralizing antibodies are thought to play a major role in protection, the potential role of non-neutralizing, cross-protective antibodies in immunity should not be disregarded. Non-neutralizing IgA antibodies against influenza A virus were recently shown to inhibit the release of multiple influenza A strains from infected cells.Citation99 Similarly, administration of cross-reactive, non-neutralizing antibodies protected mice from lethal influenza B virus challenge.Citation100 In contrast, IgG1 antibodies against influenza virus were shown to inhibit the cross-protective effect of IgG2 antibodies after vaccination in mice, suggesting that antibody isotypes play a major role in pathogen control.Citation101 Other mechanisms by which non-neutralizing antibodies enhance or antagonize the immune response have been reviewed.Citation102 Vaccine design should focus on targeting conserved, protective epitopes and inducing the proper Ig subtype, perhaps by manipulating the presentation of the antigen and/or by selecting adjuvants that will enhance the antibody repertoire as shown for influenza and human papilloma virus.Citation103,Citation104
In conclusion, the large diversity of human noroviruses presents a problem that must be overcome to develop a cross-protective vaccine. Historically, the lack of a robust cell culture system and suitable small animal model contributed to the difficulties in the study of human norovirus antigenicity and correlates of immune protection. Recently, several advances have been made, including the advent of a cell culture system that is susceptible to certain norovirus strainsCitation34 and the conclusion of early-phase vaccine trials,Citation87,Citation89 helping us to better understand the role of norovirus diversification in immunity. Future research that focuses on the synergy of duration of immunity, genotype antigenic differences, B- and T-cell immunodominance, and acquisition of biological properties that enhance replication and transmission will facilitate the development of effective control strategies against noroviruses.
Acknowledgments
We thank Joseph Kendra and Antonia Thomas for critical reading of the manuscript.
Disclosure statement
No potential conflict of interest was reported by the authors.
Additional information
Funding
References
- Thorne LG, Goodfellow IG. Norovirus gene expression and replication. J Gen Virol. 2014;95(Pt 2):278–13. doi:10.1099/vir.0.059634-0.
- Prasad BV, Hardy ME, Dokland T, Bella J, Rossmann MG, Estes MK. X-ray crystallographic structure of the Norwalk virus capsid. Science. 1999;286(5438):287–290. doi:10.1126/science.286.5438.287.
- Jiang X, Wang M, Graham DY, Estes MK. Expression, self-assembly, and antigenicity of the Norwalk virus capsid protein. J Virol. 1992;66(11):6527–6532. doi:10.1128/JVI.66.11.6527-6532.1992.
- Devant JM, Hofhaus G, Bhella D, Hansman GS. Heterologous expression of human norovirus GII.4 VP1 leads to assembly of T=4 virus-like particles. Antiviral Res. 2019;168:175–182. doi:10.1016/j.antiviral.2019.05.010.
- Jung J, Grant T, Thomas DR, Diehnelt CW, Grigorieff N, Joshua-Tor L. High-resolution cryo-EM structures of outbreak strain human norovirus shells reveal size variations. Proc Natl Acad Sci U S A. 2019;116(26):12828–12832. doi:10.1073/pnas.1903562116.
- Ajami NJ, Barry MA, Carrillo B, Muhaxhiri Z, Neill FH, Prasad BV, Opekun AR, Gilger MA, Graham DY, Atmar RL, et al. Antibody responses to norovirus genogroup GI.1 and GII.4 proteases in volunteers administered Norwalk virus. Clin Vaccine Immunol. 2012;19(12):1980–1983. doi:10.1128/CVI.00411-12.
- Atmar RL, Ramani S, Estes MK. Human noroviruses: recent advances in a 50-year history. Curr Opin Infect Dis. 2018;31(5):422–432. doi:10.1097/QCO.0000000000000476.
- Parra GI, Azure J, Fischer R, Bok K, Sandoval-Jaime C, Sosnovtsev SV, Sander P, Green KY, Jhaveri R. Identification of a broadly cross-reactive epitope in the inner shell of the norovirus capsid. PLoS One. 2013;8(6):e67592. doi:10.1371/journal.pone.0067592.
- Parra GI, Sosnovtsev SV, Abente EJ, Sandoval-Jaime C, Bok K, Dolan MA, Green KY. Mapping and modeling of a strain-specific epitope in the Norwalk virus capsid inner shell. Virology. 2016;492:232–241. doi:10.1016/j.virol.2016.02.019.
- Alvarado G, Ettayebi K, Atmar RL, Bombardi RG, Kose N, Estes MK, Crowe JE Jr. Human Monoclonal Antibodies That Neutralize Pandemic GII.4 Noroviruses. Gastroenterology. 2018;155(6):1898–1907. doi:10.1053/j.gastro.2018.08.039.
- Ford-Siltz LA, Wales S, Tohma K, Gao Y, Parra GI. Genotype-specific neutralization of norovirus is mediated by antibodies against the protruding domain of the major capsid protein. J Infect Dis. 2020. doi:10.1093/infdis/jiaa116.
- Lindesmith LC, McDaniel JR, Changela A, Verardi R, Kerr SA, Costantini V, Brewer-Jensen PD, Mallory ML, Voss WN, Boutz DR, et al. Sera antibody repertoire analyses reveal mechanisms of broad and pandemic strain neutralizing responses after human norovirus vaccination. Immunity. 2019;50(6):1530–1541 e8. doi:10.1016/j.immuni.2019.05.007.
- Koromyslova AD, Morozov VA, Hefele L, Hansman GS. Human norovirus neutralized by a monoclonal antibody targeting the histo-blood group antigen pocket. J Virol. 2019;93(5):e02174-18.
- Bok K, Parra GI, Mitra T, Abente E, Shaver CK, Boon D, Engle R, Yu C, Kapikian AZ, Sosnovtsev SV, et al. Chimpanzees as an animal model for human norovirus infection and vaccine development. Proc Natl Acad Sci U S A. 2011;108(1):325–330. doi:10.1073/pnas.1014577107.
- Hansman GS, Taylor DW, McLellan JS, Smith TJ, Georgiev I, Tame JR, Park SY, Yamazaki M, Gondaira F, Miki M, et al. Structural basis for broad detection of genogroup II noroviruses by a monoclonal antibody that binds to a site occluded in the viral particle. J Virol. 2012;86(7):3635–3646. doi:10.1128/JVI.06868-11.
- Chhabra P, De Graaf M, Parra GI, Chan MC, Green K, Martella V, Wang Q, White PA, Katayama K, Vennema H, et al. Updated classification of norovirus genogroups and genotypes. J Gen Virol. 2019;100(10):1393–1406. doi:10.1099/jgv.0.001318.
- Parra GI, Squires RB, Karangwa CK, Johnson JA, Lepore CJ, Sosnovtsev SV, Green KY, Sestak K. Static and evolving norovirus genotypes: implications for epidemiology and immunity. PLoS Pathog. 2017;13(1):e1006136. doi:10.1371/journal.ppat.1006136.
- Parra GI. Emergence of norovirus strains: a tale of two genes. Virus Evol. 2019;5(2):vez048. doi:10.1093/ve/vez048.
- Siebenga JJ, Vennema H, Zheng DP, Vinje J, Lee BE, Pang XL, Ho EC, Lim W, Choudekar A, Broor S, et al. Norovirus illness is a global problem: emergence and spread of norovirus GII.4 variants, 2001-2007. J Infect Dis. 2009;200(5):802–812. doi:10.1086/605127.
- Lanata CF, Fischer-Walker CL, Olascoaga AC, Torres CX, Aryee MJ, Black RE. Child health epidemiology reference group of the World Health, O., Unicef, Global causes of diarrheal disease mortality in children <5 years of age: a systematic review. PLoS One. 2013;8:e72788.
- Lopman BA, Steele D, Kirkwood CD, Parashar UD. The vast and varied global burden of norovirus: prospects for prevention and control. PLoS Med. 2016;13(4):e1001999. doi:10.1371/journal.pmed.1001999.
- Atmar RL, Opekun AR, Gilger MA, Estes MK, Crawford SE, Neill FH, Graham DY. Norwalk virus shedding after experimental human infection. Emerg Infect Dis. 2008;14(10):1553–1557. doi:10.3201/eid1410.080117.
- Bok K, Green KY. Norovirus gastroenteritis in immunocompromised patients. N Engl J Med. 2012;367(22):2126–2132. doi:10.1056/NEJMra1207742.
- Bartsch SM, Lopman BA, Ozawa S, Hall AJ, Lee BY, Olson DR. Global economic burden of norovirus gastroenteritis. PLoS One. 2016;11(4):e0151219. doi:10.1371/journal.pone.0151219.
- Rockx BH, Vennema H, Hoebe CJ, Duizer E, Koopmans MP. Association of histo-blood group antigens and susceptibility to norovirus infections. J Infect Dis. 2005;191(5):749–754. doi:10.1086/427779.
- Le Pendu J, Ruvoen-Clouet N. Fondness for sugars of enteric viruses confronts them with human glycans genetic diversity. Hum Genet. 2019;139(6–7):903–910. doi:10.1007/s00439-019-02090-w.
- Haga K, Ettayebi K, Tenge VR, Karandikar UC, Lewis MA, Lin SC, Neill FH, Ayyar BV, Zeng XL, Larson G, et al. Genetic manipulation of human intestinal enteroids demonstrates the necessity of a functional fucosyltransferase 2 gene for secretor-dependent human norovirus infection. mBio. 2020;11(2). doi:10.1128/mBio.00251-20.
- Ravn V, Dabelsteen E. Tissue distribution of histo-blood group antigens. APMIS. 2000;108(1):1–28. doi:10.1034/j.1600-0463.2000.d01-1.x.
- Marionneau S, Cailleau-Thomas A, Rocher J, Le Moullac-Vaidye B, Ruvoen N, Clement M, Le Pendu J. ABH and Lewis histo-blood group antigens, a model for the meaning of oligosaccharide diversity in the face of a changing world. Biochimie. 2001;83(7):565–573. doi:10.1016/S0300-9084(01)01321-9.
- Thorven M, Grahn A, Hedlund KO, Johansson H, Wahlfrid C, Larson G, Svensson L. A homozygous nonsense mutation (428G–>A) in the human secretor (FUT2) gene provides resistance to symptomatic norovirus (GGII) infections. J Virol. 2005;79(24):15351–15355. doi:10.1128/JVI.79.24.15351-15355.2005.
- Larsson MM, Rydell GE, Grahn A, Rodriguez-Diaz J, Akerlind B, Hutson AM, Estes MK, Larson G, Svensson L. Antibody prevalence and titer to norovirus (genogroup II) correlate with secretor (FUT2) but not with ABO phenotype or Lewis (FUT3) genotype. J Infect Dis. 2006;194(10):1422–1427. doi:10.1086/508430.
- Frenck R, Bernstein DI, Xia M, Huang P, Zhong W, Parker S, Dickey M, McNeal M, Jiang X. Predicting susceptibility to norovirus GII.4 by use of a challenge model involving humans. J Infect Dis. 2012;206(9):1386–1393. doi:10.1093/infdis/jis514.
- Lindesmith L, Moe C, Marionneau S, Ruvoen N, Jiang X, Lindblad L, Stewart P, LePendu J, Baric R. Human susceptibility and resistance to Norwalk virus infection. Nat Med. 2003;9(5):548–553. doi:10.1038/nm860.
- Ettayebi K, Crawford SE, Murakami K, Broughman JR, Karandikar U, Tenge VR, Neill FH, Blutt SE, Zeng XL, Qu L, et al. Replication of human noroviruses in stem cell-derived human enteroids. Science. 2016;353(6306):1387–1393. doi:10.1126/science.aaf5211.
- Atmar RL, Bernstein DI, Lyon GM, Treanor JJ, Al-Ibrahim MS, Graham DY, Vinje J, Jiang X, Gregoricus N, Frenck RW, et al. Serological correlates of protection against a gii.4 norovirus. Clin Vaccine Immunol. 2015;22(8):923–929. doi:10.1128/CVI.00196-15.
- Reeck A, Kavanagh O, Estes MK, Opekun AR, Gilger MA, Graham DY, Atmar RL. Serological correlate of protection against norovirus-induced gastroenteritis. J Infect Dis. 2010;202(8):1212–1218. doi:10.1086/656364.
- Atmar RL, Ettayebi K, Ayyar BV, Neill FH, Braun RP, Ramani S, Estes MK. Comparison of Microneutralization and Histo-Blood Group Antigen-Blocking Assays for Functional Norovirus Antibody Detection. J Infect Dis. 2020;221(5):739–743. doi:10.1093/infdis/jiz526.
- Kendra JA, Tohma K, Ford-Siltz LA, Lepore CJ, Parra GI. Antigenic cartography reveals complexities of the genetic determinants that lead to antigenic differences among pandemic GII.4 noroviruses. Proc Natl Acad Sci U S A. 2021;118(11).e2015874118.
- Cheetham S, Souza M, Meulia T, Grimes S, Han MG, Saif LJ. Pathogenesis of a genogroup II human norovirus in gnotobiotic pigs. J Virol. 2006;80(21):10372–10381. doi:10.1128/JVI.00809-06.
- Han MG, Cheetham S, Azevedo M, Thomas C, Saif LJ. Immune responses to bovine norovirus-like particles with various adjuvants and analysis of protection in gnotobiotic calves. Vaccine. 2006;24(3):317–326. doi:10.1016/j.vaccine.2005.07.071.
- Taube S, Kolawole AO, Hohne M, Wilkinson JE, Handley SA, Perry JW, Thackray LB, Akkina R, Wobus CE, Dermody TS. A mouse model for human norovirus. mBio. 2013;4(4). doi:10.1128/mBio.00450-13.
- Karangwa CK, Parra GI, Bok K, Johnson JA, Levenson EA, Green KY. Sequential Gastroenteritis Outbreaks in a Single Year Caused by Norovirus Genotypes GII.2 and GII.6 in an Institutional Setting. Open Forum Infect Dis. 2017;4(4):ofx236. doi:10.1093/ofid/ofx236.
- Parrino TA, Schreiber DS, Trier JS, Kapikian AZ, Blacklow NR. Clinical immunity in acute gastroenteritis caused by Norwalk agent. N Engl J Med. 1977;297(2):86–89. doi:10.1056/NEJM197707142970204.
- Wyatt RG, Dolin R, Blacklow NR, DuPont HL, Buscho RF, Thornhill TS, Kapikian AZ, Chanock RM. Comparison of three agents of acute infectious nonbacterial gastroenteritis by cross-challenge in volunteers. J Infect Dis. 1974;129(6):709–714. doi:10.1093/infdis/129.6.709.
- Ramani S, Neill FH, Opekun AR, Gilger MA, Graham DY, Estes MK, Atmar RL. Mucosal and cellular immune responses to Norwalk Virus. J Infect Dis. 2015;212(3):397–405. doi:10.1093/infdis/jiv053.
- Atmar RL, Bernstein DI, Harro CD, Al-Ibrahim MS, Chen WH, Ferreira J, Estes MK, Graham DY, Opekun AR, Richardson C, et al. Norovirus vaccine against experimental human Norwalk Virus illness. N Engl J Med. 2011;365(23):2178–2187. doi:10.1056/NEJMoa1101245.
- Cortes-Penfield NW, Ramani S, Estes MK, Atmar RL. Prospects and challenges in the development of a Norovirus vaccine. Clin Ther. 2017;39(8):1537–1549. doi:10.1016/j.clinthera.2017.07.002.
- Costantini VP, Cooper EM, Hardaker HL, Lee LE, DeBess EE, Cieslak PR, Hall AJ, Vinje J. Humoral and mucosal immune responses to human norovirus in the elderly. J Infect Dis. 2020;221(11):1864–1874. doi:10.1093/infdis/jiaa021.
- Sapparapu G, Czako R, Alvarado G, Shanker S, Prasad BV, Atmar RL, Estes MK, Crowe JE Jr. Frequent use of the IgA Isotype in human b cells encoding potent norovirus-specific monoclonal antibodies that block HBGA binding. PLoS Pathog. 2016;12(6):e1005719. doi:10.1371/journal.ppat.1005719.
- Onodera T, Hashi K, Shukla RK, Miki M, Takai-Todaka R, Fujimoto A, Kuraoka M, Miyoshi T, Kobayashi K, Hasegawa H, et al. Immune-focusing properties of virus-like particles improve protective IgA responses. J Immunol. 2019;203(12):3282–3292. doi:10.4049/jimmunol.1900481.
- Parra GI, Green KY. Sequential gastroenteritis episodes caused by 2 norovirus genotypes. Emerg Infect Dis. 2014;20(6):1016–1018. doi:10.3201/eid2006.131627.
- Hansman GS, Natori K, Shirato-Horikoshi H, Ogawa S, Oka T, Katayama K, Tanaka T, Miyoshi T, Sakae K, Kobayashi S, et al. Genetic and antigenic diversity among noroviruses. J Gen Virol. 2006;87(Pt 4):909–919. doi:10.1099/vir.0.81532-0.
- Sakon N, Yamazaki K, Nakata K, Kanbayashi D, Yoda T, Mantani M, Kase T, Takahashi K, Komano J. Impact of genotype-specific herd immunity on the circulatory dynamism of norovirus: a 10-year longitudinal study of viral acute gastroenteritis. J Infect Dis. 2015;211(6):879–888. doi:10.1093/infdis/jiu496.
- Saito M, Goel-Apaza S, Espetia S, Velasquez D, Cabrera L, Loli S, Crabtree JE, Black RE, Kosek M, Checkley W, et al. Multiple norovirus infections in a birth cohort in a Peruvian Periurban community. Clin Infect Dis. 2014;58(4):483–491. doi:10.1093/cid/cit763.
- Nelson MI, Mahfuz M, Chhabra P, Haque R, Seidman JC, Hossain I, McGrath M, Ahmed AMS, Knobler S, Vinje J, et al. Genetic diversity of noroviruses circulating in a pediatric cohort in Bangladesh. J Infect Dis. 2018;218(12):1937–1942. doi:10.1093/infdis/jiy454.
- Simmons K, Gambhir M, Leon J, Lopman B. Duration of immunity to norovirus gastroenteritis. Emerg Infect Dis. 2013;19(8):1260–1267. doi:10.3201/eid1908.130472.
- Chhabra P, Rouhani S, Browne H, Yori PP, Salas MS, Olortegui MP, Moulton LH, Kosek MN, Vinje J. Homotypic and heterotypic protection and risk of re-infection following natural norovirus infection in a highly endemic setting. Clin Infect Dis. 2021;72(2):222–229. doi:10.1093/cid/ciaa019.
- Lopman BA, Trivedi T, Vicuna Y, Costantini V, Collins N, Gregoricus N, Parashar U, Sandoval C, Broncano N, Vaca M, et al. Norovirus infection and disease in an ecuadorian birth cohort: association of certain norovirus genotypes with host FUT2 secretor status. J Infect Dis. 2015;211(11):1813–1821. doi:10.1093/infdis/jiu672.
- Lindesmith LC, Beltramello M, Swanstrom J, Jones TA, Corti D, Lanzavecchia A, Baric RS. Serum immunoglobulin A cross-strain blockade of human noroviruses. Open Forum Infect Dis. 2015;2(3):ofv084. doi:10.1093/ofid/ofv084.
- Lindesmith L, Moe C, Lependu J, Frelinger JA, Treanor J, Baric RS. Cellular and humoral immunity following Snow Mountain virus challenge. J Virol. 2005;79(5):2900–2909. doi:10.1128/JVI.79.5.2900-2909.2005.
- Lindesmith LC, Ferris MT, Mullan CW, Ferreira J, Debbink K, Swanstrom J, Richardson C, Goodwin RR, Baehner F, Mendelman PM, et al. Broad blockade antibody responses in human volunteers after immunization with a multivalent norovirus VLP candidate vaccine: immunological analyses from a phase I clinical trial. PLoS Med. 2015;12(3):e1001807. doi:10.1371/journal.pmed.1001807.
- Parra GI, Bok K, Taylor R, Haynes JR, Sosnovtsev SV, Richardson C, Green KY. Immunogenicity and specificity of norovirus Consensus GII.4 virus-like particles in monovalent and bivalent vaccine formulations. Vaccine. 2012;30(24):3580–3586. doi:10.1016/j.vaccine.2012.03.050.
- Tohma K, Lepore CJ, Ford-Siltz LA, Parra GI. Evolutionary dynamics of non-GII genotype 4 (GII.4) noroviruses reveal limited and independent diversification of variants. J Gen Virol. 2018;99(8):1027–1035. doi:10.1099/jgv.0.001088.
- Lindesmith LC, Donaldson EF, Lobue AD, Cannon JL, Zheng DP, Vinje J, Baric RS. Mechanisms of GII.4 norovirus persistence in human populations. PLoS Med. 2008;5(2):e31. doi:10.1371/journal.pmed.0050031.
- Debbink K, Lindesmith LC, Donaldson EF, Costantini V, Beltramello M, Corti D, Swanstrom J, Lanzavecchia A, Vinje J, Baric RS. Emergence of new pandemic GII.4 Sydney norovirus strain correlates with escape from herd immunity. J Infect Dis. 2013;208:1877–1887.
- Lindesmith LC, Beltramello M, Donaldson EF, Corti D, Swanstrom J, Debbink K, Lanzavecchia A, Baric RS. Immunogenetic mechanisms driving norovirus GII.4 antigenic variation. PLoS Pathog. 2012;8:e1002705.
- Debbink K, Donaldson EF, Lindesmith LC, Baric RS. Genetic mapping of a highly variable norovirus GII.4 blockade epitope: potential role in escape from human herd immunity. J Virol. 2012;86(2):1214–1226. doi:10.1128/JVI.06189-11.
- Parra GI, Abente EJ, Sandoval-Jaime C, Sosnovtsev SV, Bok K, Green KY. Multiple antigenic sites are involved in blocking the interaction of GII.4 norovirus capsid with ABH histo-blood group antigens. J Virol. 2012;86(13):7414–7426. doi:10.1128/JVI.06729-11.
- Lindesmith LC, Costantini V, Swanstrom J, Debbink K, Donaldson EF, Vinje J, Baric RS. Emergence of a norovirus GII.4 strain correlates with changes in evolving blockade epitopes. J Virol. 2013;87(5):2803–2813. doi:10.1128/JVI.03106-12.
- Tohma K, Lepore CJ, Gao Y, Ford-Siltz LA, Parra GI, Baric RS, Estes MK. Population Genomics of GII.4 Noroviruses Reveal Complex Diversification and New Antigenic Sites Involved in the Emergence of Pandemic Strains. MBio. 2019;10(5). doi:10.1128/mBio.02202-19.
- Shanker S, Czako R, Sapparapu G, Alvarado G, Viskovska M, Sankaran B, Atmar RL, Crowe JE Jr., Estes MK, Prasad BV. Structural basis for norovirus neutralization by an HBGA blocking human IgA antibody. Proc Natl Acad Sci U S A. 2016;113(40):E5830–E5837. doi:10.1073/pnas.1609990113.
- Angeletti D, Yewdell JW. Understanding and Manipulating Viral Immunity: antibody Immunodominance Enters Center Stage. Trends Immunol. 2018;39(7):549–561. doi:10.1016/j.it.2018.04.008.
- Eggink D, Goff PH, Palese P. Guiding the immune response against influenza virus hemagglutinin toward the conserved stalk domain by hyperglycosylation of the globular head domain. J Virol. 2014;88(1):699–704. doi:10.1128/JVI.02608-13.
- McGuire AT, Dreyer AM, Carbonetti S, Lippy A, Glenn J, Scheid JF, Mouquet H, Stamatatos L. HIV antibodies. Antigen modification regulates competition of broad and narrow neutralizing HIV antibodies. Science. 2014;346(6215):1380–1383. doi:10.1126/science.1259206.
- Garrity RR, Rimmelzwaan G, Minassian A, Tsai WP, Lin G, De Jong JJ, Goudsmit J, Nara PL. Refocusing neutralizing antibody response by targeted dampening of an immunodominant epitope. J Immunol. 1997;159:279–289.
- Lok SM, Kostyuchenko V, Nybakken GE, Holdaway HA, Battisti AJ, Sukupolvi-Petty S, Sedlak D, Fremont DH, Chipman PR, Roehrig JT, et al. Binding of a neutralizing antibody to dengue virus alters the arrangement of surface glycoproteins. Nat Struct Mol Biol. 2008;15(3):312–317. doi:10.1038/nsmb.1382.
- Lindesmith LC, Mallory ML, Debbink K, Donaldson EF, Brewer-Jensen PD, Swann EW, Sheahan TP, Graham RL, Beltramello M, Corti D, et al. Conformational occlusion of blockade antibody epitopes, a novel mechanism of gii.4 human norovirus immune evasion. mSphere. 2018;3(1). doi:10.1128/mSphere.00518-17.
- Lindesmith LC, Donaldson EF, Beltramello M, Pintus S, Corti D, Swanstrom J, Debbink K, Jones TA, Lanzavecchia A, Baric RS. Particle conformation regulates antibody access to a conserved GII.4 norovirus blockade epitope. J Virol. 2014;88(16):8826–8842. doi:10.1128/JVI.01192-14.
- Chan MC, Lee N, Hung TN, Kwok K, Cheung K, Tin EK, Lai RW, Nelson EA, Leung TF, Chan PK. Rapid emergence and predominance of a broadly recognizing and fast-evolving norovirus GII.17 variant in late 2014. Nat Commun. 2015;6(1):10061. doi:10.1038/ncomms10061.
- Matsushima Y, Ishikawa M, Shimizu T, Komane A, Kasuo S, Shinohara M, Nagasawa K, Kimura H, Ryo A, Okabe N, et al. Genetic analyses of GII.17 norovirus strains in diarrheal disease outbreaks from December 2014 to March 2015 in Japan reveal a novel polymerase sequence and amino acid substitutions in the capsid region. Euro Surveill. 2015;20(26). doi:10.2807/1560-7917.ES2015.20.26.21173.
- Dai YC, Xia M, Huang Q, Tan M, Qin L, Zhuang YL, Long Y, Li JD, Jiang X, Zhang XF. Characterization of antigenic relatedness between GII.4 and GII.17 noroviruses by use of serum samples from norovirus-infected patients. J Clin Microbiol. 2017;55(12):3366–3373. doi:10.1128/JCM.00865-17.
- Ao Y, Wang J, Ling H, He Y, Dong X, Wang X, Peng J, Zhang H, Jin M, Duan Z. Norovirus GII.P16/GII.2-associated gastroenteritis, China, 2016. Emerg Infect Dis. 2017;23(7):1172–1175. doi:10.3201/eid2307.170034.
- Lu J, Fang L, Sun L, Zeng H, Li Y, Zheng H, Wu S, Yang F, Song T, Lin J, et al. Association of GII.P16-GII.2 recombinant norovirus strain with increased norovirus outbreaks, Guangdong, China, 2016. Emerg Infect Dis. 2017;23(7):1188–1190. doi:10.3201/eid2307.170333.
- Niendorf S, Jacobsen S, Faber M, Eis-Hubinger AM, Hofmann J, Zimmermann O, Hohne M, Bock CT. Steep rise in norovirus cases and emergence of a new recombinant strain GII.P16-GII.2, Germany, winter 2016. Euro Surveill. 2017;22(4). doi:10.2807/1560-7917.ES.2017.22.4.30447.
- Lindesmith LC, Kocher JF, Donaldson EF, Debbink K, Mallory ML, Swann EW, Brewer-Jensen PD, Baric RS. Emergence of novel human norovirus GII.17 strains correlates with changes in blockade antibody epitopes. J Infect Dis. 2017;216(10):1227–1234. doi:10.1093/infdis/jix385.
- Swanstrom J, Lindesmith LC, Donaldson EF, Yount B, Baric RS. Characterization of blockade antibody responses in GII.2.1976 Snow Mountain virus-infected subjects. J Virol. 2014;88(2):829–837. doi:10.1128/JVI.02793-13.
- Atmar RL, Cramer JP, Baehner F, Han C, Borkowski A, Mendelman PM. An exploratory study of the salivary immunoglobulin a responses to 1 dose of a norovirus virus-like particle candidate vaccine in healthy adults. J Infect Dis. 2019;219(3):410–414. doi:10.1093/infdis/jiy529.
- Vaxart’s Tableted Oral Bivalent Norovirus Vaccine Meets Primary and Secondary Endpoints in Phase1b Study. 2019 September 25, [ cited 2020 August 12]; https://investors.vaxart.com/news-releases/news-release-details/vaxarts-tableted-oral-bivalent-norovirus-vaccine-meets-primary.
- Kim L, Liebowitz D, Lin K, Kasparek K, Pasetti MF, Garg SJ, Gottlieb K, Trager G, Tucker SN. Safety and immunogenicity of an oral tablet norovirus vaccine, a phase I randomized, placebo-controlled trial. JCI Insight. 2018;3(13). doi:10.1172/jci.insight.121077.
- Blazevic V, Malm M, Arinobu D, Lappalainen S, Vesikari T. Rotavirus capsid VP6 protein acts as an adjuvant in vivo for norovirus virus-like particles in a combination vaccine. Hum Vaccin Immunother. 2016;12(3):740–748. doi:10.1080/21645515.2015.1099772.
- Tamminen K, Lappalainen S, Huhti L, Vesikari T, Blazevic V, Zhou P. Trivalent combination vaccine induces broad heterologous immune responses to norovirus and rotavirus in mice. PLoS One. 2013;8(7):e70409. doi:10.1371/journal.pone.0070409.
- Blazevic V, Lappalainen S, Nurminen K, Huhti L, Vesikari T. Norovirus VLPs and rotavirus VP6 protein as combined vaccine for childhood gastroenteritis. Vaccine. 2011;29(45):8126–8133. doi:10.1016/j.vaccine.2011.08.026.
- Santi L, Batchelor L, Huang Z, Hjelm B, Kilbourne J, Arntzen CJ, Chen Q, Mason HS. An efficient plant viral expression system generating orally immunogenic Norwalk virus-like particles. Vaccine. 2008;26(15):1846–1854. doi:10.1016/j.vaccine.2008.01.053.
- Guo L, Wang J, Zhou H, Si H, Wang M, Song J, Han B, Shu Y, Ren L, Qu J, et al. Intranasal administration of a recombinant adenovirus expressing the norovirus capsid protein stimulates specific humoral, mucosal, and cellular immune responses in mice. Vaccine. 2008;26(4):460–468. doi:10.1016/j.vaccine.2007.11.039.
- Ma Y, Li J. Vesicular stomatitis virus as a vector to deliver virus-like particles of human norovirus: a new vaccine candidate against an important noncultivable virus. J Virol. 2011;85(6):2942–2952. doi:10.1128/JVI.02332-10.
- Tan M, Fang P, Chachiyo T, Xia M, Huang P, Fang Z, Jiang W, Jiang X. Noroviral P particle: structure, function and applications in virus-host interaction. Virology. 2008;382(1):115–123. doi:10.1016/j.virol.2008.08.047.
- Su W, Gao J, Zang Y, Wu H, Wang L, Hu H, Yu X, Kong W, Jiang C. Production, characterization and immunogenicity of P particles derived from norovirus GII.4 genotype 2004 variant. Acta Virol. 2015;59(1):33–39. doi:10.4149/av_2015_01_33.
- Shiota T, Okame M, Takanashi S, Khamrin P, Takagi M, Satou K, Masuoka Y, Yagyu F, Shimizu Y, Kohno H, et al. Characterization of a broadly reactive monoclonal antibody against norovirus genogroups I and II: recognition of a novel conformational epitope. J Virol. 2007;81(22):12298–12306. doi:10.1128/JVI.00891-07.
- Okuya K, Yoshida R, Manzoor R, Saito S, Suzuki T, Sasaki M, Saito T, Kida Y, Mori-Kajihara A, Kondoh T, et al. A potential role of non-neutralizing IgA antibodies in cross-protective immunity against influenza A viruses of multiple hemagglutinin subtypes. J Virol. 2020;94(12). doi:10.1128/JVI.00408-20.
- Asthagiri Arunkumar G, Ioannou A, Wohlbold TJ, Meade P, Aslam S, Amanat F, Ayllon J, Garcia-Sastre A, Krammer F, Schultz-Cherry S. Broadly Cross-Reactive, Nonneutralizing Antibodies against Influenza B Virus Hemagglutinin Demonstrate Effector Function-Dependent Protection against Lethal Viral Challenge in Mice. J Virol. 2019;93(6). doi:10.1128/JVI.01696-18.
- Shibuya M, Aoshi T, Kuroda E, Yoshioka Y, Schultz-Cherry S. Murine cross-reactive non-neutralizing polyclonal IgG1 antibodies induced by influenza vaccine inhibit the cross-protective effect of IgG2 against heterologous virus in mice. J Virol. 2020;94(12). doi:10.1128/JVI.00323-20.
- Lu LL, Suscovich TJ, Fortune SM, Alter G. Beyond binding: antibody effector functions in infectious diseases. Nat Rev Immunol. 2018;18(1):46–61. doi:10.1038/nri.2017.106.
- Bhide Y, Dong W, Gribonika I, Voshart D, Meijerhof T, De Vries-idema J, Norley S, Guilfoyle K, Skeldon S, Engelhardt OG, et al. Cross-protective potential and protection-relevant immune mechanisms of whole inactivated influenza virus vaccines are determined by adjuvants and route of immunization. Front Immunol. 2019;10:646. doi:10.3389/fimmu.2019.00646.
- Giannini SL, Hanon E, Moris P, Van Mechelen M, Morel S, Dessy F, Fourneau MA, Colau B, Suzich J, Losonksy G, et al. Enhanced humoral and memory B cellular immunity using HPV16/18 L1 VLP vaccine formulated with the MPL/aluminium salt combination (AS04) compared to aluminium salt only. Vaccine. 2006;24(33–34):5937–5949. doi:10.1016/j.vaccine.2006.06.005.