ABSTRACT
Colonization and maturation of the gut microbiota (GM) during early life is a landmark event that fundamentally influences the (early) immunity and later-life health of various mammals. This is a delicate, systematic process that is biologically actively regulated by infants and their mothers, where (secretory) IgA, an important regulator of microbes found in breast milk and generated actively by infants, may play a key role. By binding to microbes, IgA can inhibit or enhance their colonization, influence their gene expression, and regulate immune responses. IgA dysfunction during early life is associated with disrupted GM maturation and various microbe-related diseases, such as necrotizing enterocolitis and diarrhea, which can also have a lasting effect on GM and host health. This review discusses the process of early GM maturation and its interaction with immunity and the role of IgA (focusing on milk secretory IgA) in regulating this process. The possible application of this knowledge in promoting normal GM maturation processes and immune education has also been highlighted.
1. Introduction
Breast milk has long been considered the gold standard for infant food. However, many infants are partially or exclusively formula-fed for various reasons, such as the insufficiency of breast milk or disease prevention (for example, HIV and hepatitis B virus infection).Citation1,Citation2 In China’s Sichuang province, ~70% (n = 695) of infants received infant formula as their first food, increasing to 88% within 1 month.Citation2 In the USA, only approximately 43% (816 out of 1899) of infants were exclusively fed breast milk at 1 month of age.Citation3 Breast milk is significantly more than a nutritional source for infants. It also plays a profound role in shaping the infant gut microbiota (GM) and immune system.Citation4,Citation5 Exclusive formula feeding is associated with changes in the GM,Citation6 intestinal environment, and incidences of various diseases, such as necrotizing enterocolitis (NEC),Citation7 obesity, and allergies.Citation8 The primary differences between formula and breast milk include the lack of human milk oligosaccharides (HMOs), microbes, such as Lactobacillus and Bifidobacterium, and antimicrobial components, such as IgG and IgA in formula.Citation5,Citation9
HMOs have received considerable attention over the past few decades. Based on the results obtained from these studies, oligosaccharides, including galacto-oligosaccharides, fructo-oligosaccharides, and polydextrose, have been added to infant formulas to mimic HMOs. These additions close the gap between formula and breastfeeding to some degree, including a decrease in colonic pH and an increase in Bifidobacterium.Citation10 In addition to oligosaccharides, certain bacteria, or so-called probiotics, mainly members of Bifidobacterium and Lactobacillus spp., have been used in conjunction with formula, which is believed to benefit infant health. However, the results obtained from these studies are heterogeneous. While some have declared beneficial outcomes from these additions,Citation11,Citation12 others have found them to be ineffective or even deleterious.Citation13,Citation14 Numerous studies have shown that the efficacy of probiotic supplementation, such as in disease prevention (for example, NEC)Citation15,Citation16 and GM restoration,Citation11 can only be fulfilled in breast-feeding infants (i.e., in a breast feeding-dependent manner), indicating that certain ingredients in breast milk enhance the function of probiotics. Candidate ingredients include HMOs and secretory IgA (SIgA). However, Lactobacillus, the commonly used probiotic for infants,Citation11 cannot utilize HMOs. On the contrary, it has recently been discovered that SIgA determines the mucosal colonization of Lactobacillus,Citation17 suggesting a potential role of SIgA in regulating commensal colonization.
Indeed, gut SIgA has been characterized as a principal regulator of GM (specified below in Section 3).Citation18 IgA dysfunction can result in significant gut dysbiosis, including the expansion of (potential) pathogens and increased microbial encroachment on intestinal epithelial cells (IECs), which is implicated in the development of microbe- and inflammation-related diseases (for example, metabolic syndrome (MetS), NEC, and sepsis).Citation5,Citation19–21 A balanced interaction between the GM and immune system during early life has a lifelong effect on host health and depends on the regular establishment of GM, a process regulated by (S)IgA in maternal breast milk and the plasma and intestine of infants.Citation5,Citation22,Citation23 This specific role of IgA during early life has only been recognized recently. Full identification of properties and functions of IgA during this unique life stage can substantially aid the formation of a healthy GM and immune system in infants.
2. Establishment of the GM and its effects on immunity
The microbiota is found in various body parts, such as the skin, lungs, vagina, and oral cavity. However, microbiota in the gut exhibits the highest microbial loads with a crucial effect on the immunity and metabolism of the host and has received considerable attention over the past few years. Although some studies have demonstrated the existence of bacterial DNA and viable bacteria in the meconium,Citation24 placenta,Citation25 amniotic fluid,Citation26 and fetal intestinesCitation27 in humans, it is still believed that healthy, vaginally delivered infants obtain their first primary GM during birth from the fecal and vaginal material of their mothers.Citation28,Citation29 The bacterial microbiota, virome, and mycobiome of infant GM are significantly different from those of adult GM. These microbial components of infant GM change in delicate processes and mature at different timesCitation6,Citation30,Citation31 (). The correct assembly of these microbial compartments is pivotal for the proper development of early gut immunity, which can have a lifelong effect.Citation32
Figure 1. Normal maturation process of early gut microbiota in vaginally delivered, full-term, and breast-fed infants. For bacterial microbiota, the abundance of Proteobacteria is highest during the first few weeks of life, but decreases quickly over time. Actinobacteria, primarily certain Bifidobacterium strains, initially increase followed by a decrease, reaching its highest level of richness at around six months of age. The abundance of Bacteroidetes is low at birth but increases steadily during the first year of life. The overall proportion of Firmicutes does not change significantly, showing some fluctuations during maturation. Verrucomicrobia, mainly Akkermansia muciniphila, can be detected after one month of age, and its abundance increases over time. For viral microbiota, virus-like particles cannot be detected at birth but their richness increases quickly and reaches 109/g feces at four months of age, a level similar to that in adults. Furthermore, an increase in virus infecting human cells also becomes evident over time. For mycobiota, its α-diversity shows a slight increase over time, while its β-diversity remains mostly unchanged. However, the mycobiota composition changes over time, showing a shift from the early dominance of Debaryomyces hanseni to Saccharomyces cerevisiae at one year of age. Moreover, changing GM patterns can vary substantially among individuals and can be disrupted by various factors. Therefore, a precise time frame regarding the maturation of early GM compositions needs to be established
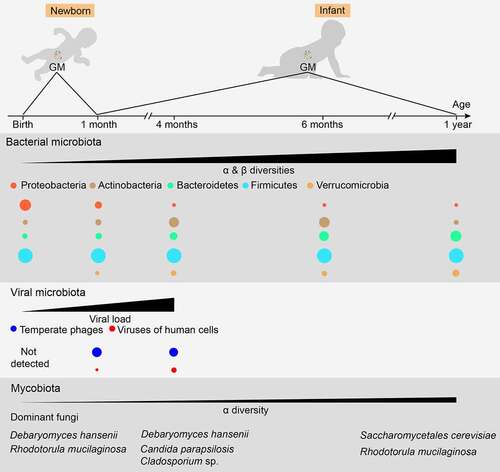
In full-term, breastfed, vaginally delivered healthy infants, the bacterial microbiota is primarily composed of aerobic or facultative anaerobic Proteobacteria and Firmicutes members, such as Escherichia/Shigella and Klebsiella, Enterococcus spp., and Lactobacillus during the first weeks of life.Citation6,Citation33 The abundance of these bacteria decreases rapidly over the next few months, along with the expansion of the anaerobic bacterial genera, Bifidobacterium, Clostridium, and Bacteroides. Then, Bifidobacterium declines, and adult-like bacterial microbiota is formed at 1–3 years of age. During homeostasis, this adult GM in the colon and feces is dominated by the anaerobic bacteria phyla Bacteroidetes and Firmicutes, accompanied by small proportions of Proteobacteria, Verrucomicrobia, and Actinobacteria.Citation34 A higher proportion of Proteobacteria has also been identified in the small intestine than in the colon.Citation35
The appropriate interaction between bacterial antigens and the gut immune system during early life imprints immunotolerance to gut commensals and dietary and autologous antigens. The disruption of this process can increase the disposition of immune-related diseases. For example, Escherichia coli-derived, but not B. dorei-derived, lipopolysaccharides (LPS) can elicit immune responses in primary human peripheral blood mononuclear cells and protect mice from the development of type 1 diabetes.Citation36 Finnish and Estonian infants, who have higher levels of Bacteroides in their GM during the first 3 years of life than Russian infants, displayed a higher prevalence of early-onset autoimmune diseases.Citation36 Similarly, the morbidities of atopy and wheezing at 1 year of age were negatively correlated with the fecal LPS levels at 3 months of age in Canadian infants, although the LPS properties were not characterized.Citation37 Interestingly, as discussed above, Escherichia forms a significant part of the GM during the first months of life, while Bacteroides increases much later. This seems to be a co-evolution between the host and bacterial GM rather than a coincidence.
Bacteriophages belonging to the gut virome, which infect and kill bacterial cells.Citation38 The human gut virome is highly dynamic during early life but stabilizes in adulthood.Citation39 Virus-like particles are relatively low in the meconium and early feces of healthy infants or cannot be detected by fluorescence staining. Their level increases dramatically and reaches 109/g feces within 1 month, which is similar to that found in adults.Citation30 During the first month of life, pioneer bacteria-specific bacteriophages, such as those of Bifidobacterium and Lactobacillus, dominate the infant gut virome, while the abundance of eukaryotic viruses that infect humans, such as Adenoviridae, Anelloviridae, Caliciviridae, and Picornaviridae, increased significantly at 4 months of age.Citation30,Citation38 In addition, the gut virome can influence the immune responses to bacteria and vice versa. Infecting germ-free (GF) or antibiotic-treated mice with murine norovirus protected them from intestinal injury and pathogenic bacterial infection,Citation40 while gram-negative bacteria can induce intestinal antiviral activity in a necrosis factor κB (NF-κB)-dependent manner.Citation41
Similar to gut virome, interactions among the bacterial microbiota, mycobiome, and gut immunity have been reported. Clusters IV and XIVa of Clostridia resist the colonization of Candida albicans via the hypoxia-inducible factor-1α-mediated generation of LL-37 in mice.Citation42 The administration of anti-fungal agents exaggerated dextran sulfate sodium (DSS)-induced colitis and house dust mite-induced allergic airway disease, along with bacterial dysbiosis, including a decline in Bacteroides and Clostridium and an increase in Streptococcus.Citation43 Unlike bacterial microbiota, fungal diversity changes moderately over time, with a slight increase in alpha-diversity while beta-diversity remains virtually unchanged.Citation44 A transformation from Debaryomyces hansenii to Saccharomyces cerevisiae was evident in Saccharomycetales during the first year of life.Citation44 Balanced mycobiota in adults mainly include Candida, Malassezia, and Saccharomyces.Citation45
Overall, the establishment of GM during early life is a dynamic process in which bacterial microbiota, mycobiota, viral microbiota, and the gut immune system interact with each other to shape the early gut. This process plays a crucial role in life-long health but can be disturbed by various modern medical practices, such as antibiotics and formula-feeding.Citation46 Furthermore, the competition between GM is relatively weak, while the plasticity is strong during this time, making it more practicable to actively build a GM that benefits health.Citation47 However, an understanding of the GM maturation process and controlling factors is necessary.
3. Role of IgA in the establishment of the GM and immunity
Considering the significant effect of an appropriately established GM on health, it is evolutionarily illogical that the host cannot actively regulate this process. Indeed, during pregnancy and lactation, the gut and vaginal microbiota of mothers change in an organized manner, including the expansion of commensals such as Bifidobacterium and Lactobacillus.Citation48,Citation49 These changes are essential for newborns to obtain a healthy initial GM at birth. In addition to programming the first GM, IgA secreted by mothers and infants may also play a role in GM maturationCitation50 ().
Figure 2. The role of milk SIgA in regulating gut microbiota maturation. IgA+ plasma cells (PCs) produce milk SIgA in the mammary gland, which originates from the gut and is educated by gut microbiota (GM). Milk SIgA is the primary (exclusive) source of intestinal SIgA for breastfeeding infants. SIgA in the intestine can bind to specific pathogens, promoting their clearance via aggregation. The binding of SIgA to certain commensals, such as Lactobacillus, can enhance their mucosal colonization. A lack in milk SIgA can lead to the over-enrichment of pathogens and delayed GM maturation, which is associated with the development of various microbe- and immunity-related diseases during infancy (for example, NEC and IBD) and in later life (for example, obesity, allergies, and autoimmune diseases). NEC, necrotizing enterocolitis; IBD, inflammatory bowel disease
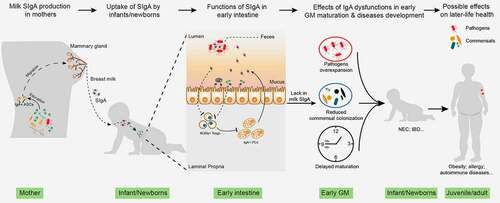
In humans, IgA is the most abundant immunoglobulin isotype secreted into the gut, accounting for three-quarters of the immunoglobulin generated daily at 3–5 g/d.Citation51 Naïve B cell precursors expressing IgM and IgD acquire the ability to produce IgA after undergoing IgA class switch recombination (CSR).Citation52 Based on the type of antigen and the site of B cell activation, IgA induction has been defined as T cell-dependent (TD) and -independent (TI) pathways. The small intestinal lamina propria (LP) harbors the largest (~80%) IgA+ PC population, while its abundance in colonic LP is small.Citation35 Small populations of IgA+ PCs can also be detected in extraintestinal tissues, including the lungs, salivary glands, lactating mammary glands, liver, and bone marrow.Citation53 In mucosal tissues, IgA is released together with a fraction of the pIgR, named secretory component (SC), through proteolytic cleavage, resulting in the formation of SIgA.
Gut dysbiosis has been widely reported in mice with IgA-related gene deficiency. RAG-deficient mice cannot generate IgA because of the lack of mature lymphocytes, which exhibit reduced GM diversity and increased intragroup differences in GM composition.Citation54,Citation55 Similarly, a reduction in bacterial diversity has been reported in Ighm−/− (B cell-deficient) mice compared to that in their wild type (WT) and Ighm+/− littermates.Citation54 Nonetheless, other immunoglobulin isotypes such as IgMCitation35 may also contribute to the observed alteration in GM because of the complete deficiency in B cell function in these two mouse strains. Other studies using IgA- or SIgA-specific-deficient mice further highlighted the important role of (S)IgA in regulating GM composition (specified below in section 3.1.1).Citation22,Citation23 Consistent with this, GF mice with a higher abundance and diversity of IgA exhibit a greater ability to diversify the GM after transfer.Citation56 Which type of IgA response, TD or TI, dominates GM homeostasis? Contradicting evidences exist on this topic. IgA produced in T cell-deficient mice can bind to a similar group of GM as produced by WT mice,Citation35 indicating that TD IgA is dispensable for the maintenance of GM homeostasis. However, mice exhibiting T cell dysfunctions, such as Cd3e−/−, Pdcd1−/−, and T-Myd88−/− mice, display different GMs compared to WT mice, including a decrease in bacterial diversity and an increase in the abundance of Gammaproteobacteria members Enterobacteriaceae and Desulfovibrionaceae.Citation21,Citation54,Citation57 These results show that although TD and TI IgA bind to a similar group of GM, the outcomes vary owing to different IgA repertoire properties. Consistent with this, mice carrying a knock-in mutation of Aicda can still support IgA-generating CSR but not somatic hypermutation (SHM), which shows changes in the composition of their microbiota.Citation58
Alteration in the intestinal IgA response to specific bacterial antigens can also change GM composition.Citation59,Citation60 Immunization of WT mice with Salmonella-derived flagellin increased intestinal flagellin-specific SIgA levels, leading to a decrease in flagellar bacteria, mainly Proteobacteria members.Citation60 In contrast, in Tlr5−/- mice, there is a lack of immune response to flagellin because of the deficiency in Toll-like receptor 5 (TLR5), a main receptor of flagellin, resulting in an increase in flagellar bacteria.Citation59 Collectively, these results demonstrate an important role for IgA in regulating GM.
IgA deficiency is defined as a serum IgA level below minus two standard deviations of the average population-level normalized for age (partial) or as a serum IgA level lower than 0.07 g/L (complete).Citation61 In children of all ages, the prevalence of IgA deficiency in healthy individuals, ranging from 1/170 to 1/400, is lower than that in those with recurrent respiratory tract infections, which ranges from 1/4 to 1/65.Citation61 Furthermore, 46% of children exhibiting complete IgA deficiency presented with recurrent infections before 1 year of age and 74% before 5 years of age.Citation62 These observations indicate a possible role of IgA in controlling microbes during early life. In addition to recurrent infections, children with IgA deficiency are more susceptible to developing other diseases, such as allergic and autoimmune diseases.Citation63–65
3.1 Breast milk SIgA
Newborns cannot actively generate intestinal SIgA (specified below in the section Intestinal SIgA), rendering the milk SIgA from mothers the exclusive source of intestinal SIgA. IgA is the primary immunoglobulin isotype in breast milk, at a concentration of 15 g/L in colostrum and 1 g/L in mature milk, providing a daily level of 0.5–1.0 g IgA for infants.Citation66 Although a small number of antibody-secreting cells (ASCs) can be detected in breast milk, SIgA is mainly produced by PCs in the basolateral region of the mammary glands and secreted into the milk by transcytosis via epithelial cells.Citation67 The IgA+ PCs in the mammary gland increase dramatically during pregnancy and lactation and wane after lactation ceases.Citation50 They are believed to originate from the intestine mainly because (1) signaling molecules orchestrating the homing of IgA+ PCs to the mammary gland are the same as those in the intestine,Citation68 (2) the IgA+ PCs and IgA repertoires in the mammary gland are similar to those in the intestine,Citation69 and (3) radioactively labeled intestinal B cells migrate to the mammary gland at a frequency similar to the spleen and bone marrow during pregnancy.Citation4
3.1.1 Immune exclusion
An important function of SIgA in the intestine is to enhance the elimination of opportunistic pathogens via agglutination, a function known as immune exclusionCitation70 (). A recent study has shown that high-avidity intestinal SIgA can bind to the daughter cells of certain intestinal pathogens, such as Salmonella enterica subspecies enterica serovar Typhimurium and E. coli strains CFT073 and 8178, resulting in incomplete binary fission and the formation of clumps. This enhanced growth is effective at all realistic pathogen densities, promoting the elimination of low-density pathogens in the gut.Citation71 Although this mechanism was not verified for Clostridium difficile, infants aged between 2.9 and 5.3 months with higher fecal SIgA levels show lower colonization of C. difficile.Citation72
Proteobacteria accounts for a significant proportion of IgA+ bacteria in fecal and intestinal samples.Citation18 Similarly, in humans, Proteobacteria is abundant during the first days of life in mice but decreases rapidly.Citation22 Despite being influenced by the gut oxygen content, this process is also controlled by SIgA in the gut. Persistent colonization of Gammaproteobacteria was evident in IgA-deficient (Igha−/−) mice, resulting in higher susceptibility to intestinal inflammation in neonatal and developed gut.Citation22 Notably, although not confirmed by the study, this IgA-mediated inhibition of Gammaproteobacteria could be attributed to SIgA in the milk since young mice cannot actively generate intestinal SIgA until weaning.Citation23 Another study using milk SIgA-deficient (Pigr−/−) mice further demonstrated the pivotal role of milk SIgA in shaping early and adult GMs.Citation23 Rogiera et al. found that the GM of both weanling (21 days) and adult (70 days) mice that received no maternal SIgA from their nursing mothers during lactation were different from those of mice that did, including an expansion of Pasteurellaceae, belonging to Gammaproteobacteria.Citation23 Similar to the results obtained for Igha−/− mice, deficiency in maternal SIgA also increased the susceptibility to chemically induced colonic injury in adulthood.Citation23
3.1.2 Disease prevention
Many common intestinal (potential) pathogens, such as Salmonella, Klebsiella, and Escherichia, are Gammaproteobacteria members. Persistent high colonization of Proteobacteria members is a typical characteristic of early gut dysbiosis and is associated with various diseases, such as late-onset sepsis (LOS) and NEC.Citation73–75
NEC is an inflammatory mucosal disease with high mortality that affects a significant proportion of preterm infants with a gestational age of less than 33 weeks. The pathogenesis of NEC has not been well defined, but gut dysbiosis, mainly the overexpansion of Gammaproteobacteria, is usually observed before the onset of NEC and is believed to be involved in its development.Citation74 As a main controller of the gut Proteobacteria members,Citation22,Citation23 SIgA may play a key role in controlling the development of NEC in preterm infants. Indeed, a recent study investigated the role of milk SIgA in the development of NEC and found a decrease in IgA-bound bacteria and an increase in the Gammaproteobacteria member Enterobacteriaceae in the IgA-unbound fraction of infants who developed NEC.Citation5 This indicated an impaired ability of IgA to bind to Gammaproteobacteria in these infants. Igha−/− mice that received no passive SIgA during suckling exhibited NEC development rates that were similar to those of formula-fed mice, while the incidence of NEC in WT control mice was lower, further demonstrating the crucial role of IgA in NEC development.Citation5 In accordance, the NEC incidence was substantially lower in breastfed infants than in those who were formula-fed.Citation76 Similarly, prenatal stress reduced the IgA levels in mice, resulting in GM alteration in 2-week-old neonatal mice while increasing their susceptibility to experimental NEC.Citation77
Meanwhile, it also should be noticed that other mechanism(s) may be also involved in the regulation of disease development by milk SIgA, for example, through the direct regulation of gut immunity (specified below in Section 3.1.5).
3.1.3 Enhancement of mucosal colonization
Besides pathogens, IgA can bind to various commensals, such as lactobacilli, some Clostridia species, and Prevotella.Citation78 However, as mentioned above, IgA binding can lead to different outcomes because of the discrepancy in IgA properties. In fact, contrary to enhancing the clearance of pathogens by binding to them, IgA binding promotes mucosal colonization and adhesion to the IECs of certain commensals such as Lactobacillus and Bifidobacterium,Citation17,Citation79 which may be crucial for the early colonization of commensals and GM maturation. IgA-coated Lactobacillus can colonize the mucous in a glycan-dependent manner.Citation17 Disassociation from SIgA or cleavage of glycan side chains of SIgA using a promiscuous N-glycosidase disables the mucosal colonization of Lactobacillus,Citation17 highlighting the indispensable role of SIgA in mediating the mucosal colonization of Lactobacillus. Similar to Lactobacillus, an in vitro study using Caco-2 cells demonstrated that SIgA can enhance the adhesion of Bifidobacterium to cultured epithelial cells.Citation79 These results are also in line with the above-mentioned observations that the efficiency of probiotics supplementation is dependent on breastfeeding.Citation11,Citation15,Citation16 In addition, deep colonization of these commensal bacteria within the mucus with the help of SIgA protects them from the predation of bacteriophages whose titer in the mucous is lower than that in the lumen.Citation80
Therefore, this unique niche provided by the intestinal SIgA to certain commensals may affect the maturation of early GM. Indeed, children with malnutrition exhibit an overabundance of pathogens, including Haemophilus, Campylobacter, and Escherichia/Shigella, belonging to Proteobacteria.Citation81 This is associated with disordered IgA responses to GM, mainly an increase in IgA recognition of pathogens and a decrease in IgA recognition of commensals such as Verrucomicrobiaceae and Bacteroidaceae.Citation82 This impaired IgA recognition of commensals in children with malnutrition is associated with decreased GM diversity and stunted GM maturation.Citation83 Moreover, the children involved in these studies ranged in age from 6 months to over 5 years and were not all breastfed. Therefore, intestinal SIgA actively generated by children is also involved in regulating early GM maturation.
3.1.4 Regulation of viral and fungal colonization
The direct binding of SIgA to viruses in the intestine has not been widely documented despite the vaccine-elicited IgA response to specific viruses, such as rotavirus.Citation84 Therefore, the direct and overall effects of intestinal SIgA from milk or actively generated by the infants on early viral colonization have not been determined and may be minimal. However, milk SIgA may indirectly affect early viral colonization by regulating the bacterial microbiota in the intestine.Citation30 As mentioned above, SIgA can enhance the mucosal colonization of Lactobacillus, a common commensal significantly abundant in the early gut and milk.Citation17 Similarly, the richness of Bifidobacterium, another abundant commensal in the early gut, is positively associated with fecal SIgA levels in young children (from birth to 18 months of age).Citation85 Consistent with this, 4-month-old breast-fed infants show an increase in the temperate bacteriophages of Lactobacillus and Bifidobacterium, which is accompanied with a decrease in viruses that can infect human cells compared to exclusively formula-fed infants.Citation30 Moreover, besides SIgA, some other ingredients present in the breast milk, such as oligosaccharides, bacteria, and IgG, can regulate viral colonizationCitation9,Citation86 therefore, the role of these ingredients in regulating gut virome in breast-feeding infants cannot be ruled out.
On the one hand, the oral colonization of commensal C. albicans in mice induced the generation of cross-specific SIgA that binds to both the bacteria and fungi in saliva.Citation87 The binding of pathogenic C. albicans strain SC5314, by this commensal-induced SIgA prevented their adhesion to and invasion of oral epithelial cells.Citation87 Similarly, S. boulardii can induce the generation of SIgA against C. difficile toxin A in the intestine of mice.Citation88 On the other hand, bacterial colonization can also induce the production of antifungal SIgA. One month of L. casei and B. breve consumption caused an increase in the salivary anti-Candida SIgA levels in elderly humans, accompanied with a slight but significant reduction in the fungal load.Citation89 Consequently, SIgA may also mediate the interaction between bacteria and fungi in the mucosa. However, studies investigating the influence of SIgA in the intestine on the overall gut microbiota in adults and its succession during early life, as well as the effect on disease development, are exceedingly rare. This merits further determination since mycobiota are involved in the development of various diseases (for example, Crohn’s disease, reviewed in detail in Ref.Citation90) and can interact closely with IgA.
3.1.5 The effect on immune education
By inhibiting the colonization of (potential) pathogens, such as Gammaproteobacteria members and C. difficile, the intestinal SIgA from milk may contribute to the management of inflammatory responses to these bacteria and imprint anti-inflammatory properties in the gut immune system.Citation91 In addition, the binding of microbial antigens by SIgA can enhance the recognition of these antigens through microfold cells on the PPs and improve the immune responses to these antigens.Citation56 The clearance of pathogens, colonization, and interaction with certain commensals (partially) mediated by SIgA during early life can enhance the continued generation of Tregs.Citation92,Citation93 Furthermore, early life infection or a lack of interaction with commensals are associated with a reduction in Tregs and increased susceptibility to inflammation and colitis in later life.Citation92,Citation94,Citation95
These mechanisms indicate that intestinal SIgA is associated with the generation of Tregs and immune tolerance during early life.Citation92,Citation94,Citation95 However, a recent study showed that the levels of colonic RORγ+ Tregs are set up in early life and are negatively correlated with milk SIgA levels 4. C57BL/6 J (B6) mice displayed a significantly higher proportion of colonic RORγ+ Tregs than BALB/c mice. This depends on their nursing genotype rather than birth mothers, since mice born to B6 dams but nursed by BALB/c mice show similar levels of colonic RORγ+ Treg than BALB/c mice, and vice versa. This nursing-mediated alteration of RORγ+ Treg is early life- and GM- dependent and is stable to various disruptions.Citation4
The abundance of IgA+ PCs in the colon and small intestine was higher in adult BALB/c mice than in B6 mice, while the same applies to fecal and serum IgA levels. Although no significant difference was apparent between the mammary IgA+ PCs of the two genotypes, the IgA levels in breast milk were higher in BALB/c mice.Citation4 Pups nursed by BALB/c mice, independent of their birth mothers, show higher fecal IgA levels and IgA+ bacterial proportions, which can also be transmitted through generations.Citation4 Furthermore, the colonic RORγ+ Treg level in mice nursed by Igha−/− BALB/c mice was higher than that in mice nursed by WT BALB/c mice, independent of their birth mother. A deficiency in RORγ+ Treg leads to increased IgA+ bacterial levels in feces.Citation4
These results indicate a double-negative feedback loop between IgA and colonic RORγ+ Tregs. However, Treg is generally thought to be a positive regulator of IgA generation.Citation18 Then, why do high colonic RORγ+ Tregs reduce IgA productionCitation4? Mice with Treg cell-specific c-Maf deficiency (MafΔTreg) display a decrease in IL-10 production but an increase in serum and fecal IgA, as well as substantially elevated fecal IgA+ bacterial levels. Similar results have been obtained from Il-10ΔTreg mice, suggesting that IL-10 mediates the Treg-induced inhibition of IgA production.Citation96 However, IL-10 is essential for the generation of the IgA CSR.Citation18 How does a reduction in IL-10 enhance IgA responsesCitation96? A possible explanation is that a decrease in IL-10 enhances the gut immune response to GM, leading to an increase in other factors related to IgA generation, such as IL-6, resulting in increased IgA production.Citation70 Although it does not generate inflammatory factors, such as NF-κB and TNF-α, the IgA response per se is a unique inflammatory response (type 3 inflammation, pathogen clearance) to microbes.Citation97 Consistently, the colonization of microbes in GF mice that generate low-level intestinal SIgA leads to a rapid expansion of the intestinal IgA+ PCs and an increase in IgA production.Citation98 MafΔTreg mice also exhibited high levels of intestinal IL-17A–IL-22-producing TH17 cells, demonstrating an enhanced immune response to GM.Citation96
How can IgA continuously influence the RORγ+ Treg level in the colon? Possible mechanisms include the following: (1) IgA binding prevents the encroachment of GM. (2) IgA binding decreases the expression of virulent GM genes. (3) IgA enhances the clearance of GM and inhibits the translocation of their metabolites.Citation99 All these mechanisms inhibit the interaction between GM and the host, reducing the immune responses of the host to GM. However, this is inconsistent with observations that IgA-binding promotes the recognition of noninvasive S. Typhimurium and E. coli and the generation of antigen-specific CD4+ cells and IgA,Citation56 which may be attributed to the different life stages of the mice used in these studies.
An increase in the RORγ+ Treg levels in B6-fostered mice induced by low milk SIgA results in increased intestinal injury and bacterial burdens in the gut when challenged by the mouse pathogen Citrobacter rodentium.Citation4 Furthermore, high RORγ+ Treg proportions are associated with lower susceptibility to colitis, cancer, and allergies, suggesting the importance of a balanced trait.Citation4
In summary, as the primary source of SIgA in the early gut, milk SIgA is essential in regulating GM maturation and immune education. Abnormal acquisition of SIgA from milk leads to changes in the patterns of the bacterial, viral, and fungal successions, which is associated with the development of various microbe- and immunity-related diseases.Citation5,Citation22,Citation23 Milk SIgA can also directly affect the development of gut immune compartments, primarily colonic RORγ+ Tregs, influencing the immune responses to commensals and disease disposition in later life.Citation4 Consequently, a lack of SIgA during early life could be a primary reason for the increased susceptibility to the development of various diseases, including obesity, inflammatory bowel disease (IBD), and NEC, as well as allergic and autoimmune diseases, in exclusively formula-fed infants.Citation5,Citation100–102
3.2 Intestinal SIgA
The intestinal IgA+ PCs in human infants can only be readily detected after ~1 month of age, the abundance of which increases steadily with time, approaching (but do not reach) adult gut levels at 2 years of age.Citation103 Consistently, IgA+ bacteria in the feces of exclusively formula-fed infants was evident until ~30 days of age.Citation5 As in humans, mice begin to actively generate intestinal SIgA after weaning (at approximately 21 days of age). Young mice lacking maternal antibodies from the milk generate active intestinal SIgA in advance.Citation23,Citation104 Early GM significantly shapes the development of intestinal B cells and has an extended impact on the intestinal SIgA repertoire, which, unlike milk SIgA, can continuously affect intestinal homeostasis.Citation105,Citation106
In monocolonized GF mice, increased bacterial exposure causes a decrease in the diversity of intestinal SIgA repertoire.Citation105 This corresponds with the observation that intestinal IgA response to abundant commensals leads to the generation of monoclonal IgA in the intestinal SIgA repertoire.Citation69 The encountering order also affects the intestinal IgA response to microbes. Colonization of a second bacterium, C. orbiscindens, dampens the gut IgA response to the originally colonized bacteria, E. coli strain HA107,Citation105 promoting the reaction to newly encountered (potential) pathogens.Citation18 Moreover, the gut IgA response to specific microbial antigens, such as flagellin, can be long-lasting after transient exposure,Citation60,Citation107 which is especially significant in early life.Citation106
Dysregulation of the active intestinal IgA response in the early gut is correlated with the development of allergic diseases.Citation108 Dzidic et al. investigated of the correlation between the SIgA binding of the GM at 12 months of age, when the generation of active gut SIgA is fairly abundant,Citation103 and the development of allergic manifestations at 7 years of age.Citation108 They found that children with allergic and asthmatic symptoms showed an altered SIgA recognition pattern of gut bacteria, including a substantial loss of SIgA binding to Escherichia/Shigella, at 12 months of age.Citation108
The maturation of active intestinal SIgA generation in infants (young children) is relatively slow but can be accelerated by weaning. Therefore, it seems that intestinal SIgA acts as a slowly starting relay of milk SIgA during early life, which could be influenced by milk SIgA.Citation22,Citation23
3.3 Serum IgA
Contrary to milk and intestinal SIgA, serum IgA actively generated by newborns can be detected in small amounts at birth. Most serum IgA is polymeric during infancyCitation109,Citation110 and is produced by IgA+ B cells derived from bone marrow. However, in vitro studies showed that serum IgA could bind to a GM group, including Proteobacteria, similar to milk and intestinal SIgA,Citation111,Citation112 indicating a correlation between bone marrow- and intestinally derived IgA+ PCs. Indeed, during homeostasis, the generation of serum IgA and bone marrow IgA+ PCs can be significantly enhanced by the enrichment of gut Proteobacteria.Citation111 Directly monitoring intestinal B cell migration by photoconverting intestinal tissues also demonstrated a considerable transfer of intestinal IgA+ PCs to the bone marrow.Citation4 This GM-boosted serum IgA production protects mice from polymicrobial sepsis during intestinal injury.Citation111 Similarly, the abundance of Clostridium cluster XI and Proteobacteria is positively correlated with the generation of serum IgA against rotavirus in 6-week-old infants after vaccine treatment.Citation113 Therefore, although serum IgA may not directly influence GM maturation, it plays a pivotal role in protecting against gut-derived infections. Based on these observations, serum IgA may complement milk and intestinal SIgA to protect against gut-derived antigens.
3.4 Role of IgG and IgM in milk
Breast milk contains considerable amounts of IgG (~0.05 g/L) and (S)IgM (~0.014 g/L),Citation114 although at lower levels than (S)IgA, which can influence both GM and immunity in the infant gut.Citation9, 104,Citation115 Infecting or intraperitoneally immunizing dams twice with heat-inactivated mouse pathogen, C. rodentium, 2 weeks before mating confers protection to their offspring against oral C. rodentium challenge at 18 days of age in milk IgG, but not in an IgA- and IgM-dependent manner. This is associated with the virulence recognition factors encoded within the locus of enterocyte effacement pathogenicity island via IgG.Citation115 In addition to this pathogen-induced antigen-specific IgG, natural (polyreactive) IgG generated in specific pathogen-free dams or dams colonized with specific Enterobacteriaceae members can protect their offspring against the oral challenge of enterotoxigenic E. coli at 6–7 days of age.Citation9 These results indicate a significant similarity between IgG and IgA. Both the antigen-specific IgA and IgG responses can be elicited by certain pathogens, while IgA and IgG with polyreactivity (especially against Proteobacteria members) are induced by some commensals.Citation9,Citation112,Citation115 Moreover, similar to milk (S)IgA, which inhibits the generation of RORγ+ Tregs, milk IgG coordinated with IgA can dampen T cell responses in the neonatal gut in a TLR-dependent but Tcell-independent manner 104. However, regardless of these similarities, IgG binds to a smaller fraction and different set of bacteria than IgA in young children.Citation85
In contrast, IgM and IgA bind an exceedingly similar subset (mainly Bifidobacterium and Enterobacteriaceae members) of GM in young children.Citation85 Considering the low levels of gut (S)IgM, it may supplement (S)IgA. Indeed, IgA deficiency usually leads to a substantial increase in the intestinal IgM+ ASC and IgM levels in humans.Citation116 Only those with compensatory IgM secretion show a normal GM compared to IgA-sufficient individuals.Citation117 In summary, it appears that the most abundant antibody in breast milk, SIgA, plays a fundamental role in regulating GM and immunity in the neonatal gut. Furthermore, IgG is crucial for preventing specific pathogens, while (S)IgM is more complementary to (S)IgA.Citation85,Citation116,Citation117
4. Possible applications for early-life GM regulation via IgA
4.1 Demands for the enhancement of early-life IgA functionality
In which situations is it necessary and possible to enhance the IgA functionality to ensure a balanced maturation of the GM and immunity? Exclusively formula-fed infants may be considered first because they do not receive passive IgA through breastfeeding. Second, as the most prevalent primary immunodeficiency, IgA deficiency in children is implicated in the development of various diseases, primarily recurrent infections, as well as allegoric and autoimmune diseases, where impaired regulation of the GM by SIgA during early life may play an important role.Citation61 Third, other factors, including antibiotic exposure, birth mode, gestational age, and hospitalization, can disturb early GM, where the supplementation of additional SIgA may help to decrease the effect of these factors on normal GM establishment.
Prolonged antibiotic therapy (≥ 5 days) is usually employed to prevent group B Streptococcus-induced early-onset sepsis, while it is associated with the development of LOS.Citation118 Infections caused by Gammaproteobacteria members E. coli and K. pneumonia, Staphylococcus spp., and fungi, such as Candida spp., which is commonly seen after antibiotic administration, is implicated in the development of LOS in preterm infants.Citation75,Citation119,Citation120 Therefore, antibiotic-induced dysbiosis may contribute to the development of LOS. Since SIgA in the gut is closely related to the clearance of Gammaproteobacteria,Citation22 the enhancement of IgA function may contribute to the management of LOS. In addition to LOS, as discussed above, preterm infants are at risk of developing NEC, a deadly disease associated with exclusive formula-feeding and deficient IgA response, which may be prevented by enhancing IgA function.Citation5
Although infants are more tolerant to the colonization of C. difficile, possibly because they lack the receptors for C. difficile toxins during infancy, it can cause diarrhea and increase susceptibility to C. difficile infection in adulthood.Citation121 Prolonged stay in a neonatal intensive care unit is associated with an increase in the colonization of C. difficile in preterm infants,Citation122 while a higher fecal SIgA level and breastfeeding are negatively associated with its colonization,Citation72,Citation121 indicating the potential role of SIgA in preventing C. difficile colonization.
The main difference in the GM of infants delivered via C-section is a decrease in Bifidobacterium, Lactobacillus, and bacterial diversity. Supplementation with mixed probiotics, including B. breve Bb99, Propionibacterium freudenreichii subsp. hermanii JS, L. rhamnosus Lc705, and Lactobacillus rhamnosus GG (LGG) restores C-section-induced dysbiosis, including an increase in Bifidobacterium and a reduction in Proteobacteria in breastfed but not formula-fed 3-month-old infants.Citation11 Investigation on the effect of probiotic supplementation on NEC using multiple strains of Lactobacillus, Bifidobacterium, and Streptococcus indicated a breastfeeding-dependent decrease in the morbidity and mortality of NEC.Citation15,Citation16 Similar to probiotics, the effect of fecal microbiota transplantation in restoring C-section-induced dysbiosis in newborns may also depend on breastfeeding.Citation123 Although the effect of other possible ingredients in breast milk cannot be excluded, the milk SIgA-mediated enhancement of the colonization of these probiotics (commensals) could be essential, as discussed above.Citation17 Furthermore, it indicates that SIgA supplementation would be beneficial when attempting to restore an imbalanced GM in infants when SIgA from milk is not accessible.
4.2 Strategies to enhance intestinal IgA function in early life
As infants begin to actively generate their own intestinal SIgA after approximately 1 month of age, the capacity to directly enhance local IgA generation is feasible (). Probiotics, mainly various Bifidobacterium and Lactobacillus strains, are well-known for their ability to induce SIgA production.Citation124 Supplementation of Bifidobacterium and Lactobacillus in infants increases fecal SIgA levels while reducing fecal pH and calprotectin, an inflammatory marker.Citation125,Citation126 In addition to the direct supplementation of probiotics, infants exclusively fed the L. paracasei CBA L74-fermented formula from birth exhibited a significantly higher fecal SIgA level at 3 months of age than those fed a standard formula independent of delivery modes.Citation127 Similar results were observed in young children (33 ± 9 months of age), accompanied with a reduction in common infectious diseases in these children.Citation128 These studies indicate that probiotic-derived metabolites may be essential for improving SIgA production. Indeed, supplementation of heat-killed probiotics or p40, an LGG-derived protein, promoted SIgA production.Citation125,Citation129 This provides a new avenue for enhancing local IgA function that is safer than live probiotics because certain strains of probiotics, such as LGG and L. acidophilus, can translocate into the circulation system of patients in intensive care units, contributing to bacteremia, especially in those aged less than 4 years.Citation13
Figure 3. Possible application of IgA to enhance normal gut microbiota maturation. before direct iga supplementation, the “core microbes” must first be identified by analyzing the gut microbiota (GM) properties in pregnant women based on large-scale sequencing. This core microbiota is then used to immunize cows or IgA-producing antibody-secreting cells (ASCs) (or through other possible methods) and obtain IgA. Next, a comparison between the repertoires of this IgA and milk SIgA is also required. For enhancing local IgA functionality, the possible approaches include the supplementation of specific commensals, probiotics, prebiotics, and unique vaccines derived from pathogens
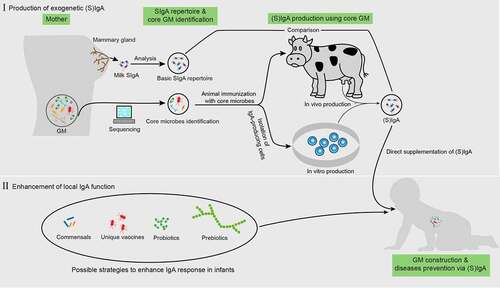
In addition to these traditional probiotics, certain commensals reportedly induce intestinal IgA responses in mice and cows.Citation130,Citation131 Yang et al. reported that 11 B. ovatus strains isolated from humans could strongly induce IgA production in mice.Citation130 Notably, this effect does not depend on the colonizing order of these bacteria since the transfer of a multiplex cocktail of these bacterial strains significantly and consistently elevated IgA production in humanized mice.Citation130 Moreover, all these bacterial strains were isolated from humans, which are commensals but not (opportunistic) pathogens, suggesting that these bacteria are relatively safe when applied to humans.Citation130 Supplementation of the fungus, S. cerevisiae boulardii CNCM I-1079, increased the ileal and colonic SIgA and IgA+ PCs in newborn dairy calves at 7 days of age, accompanied with Lactobacillus spp. enrichment in the jejunum, corresponding with the ability of IgA to enhance Lactobacillus colonization.Citation17,Citation131
Dietary fiber-derived short-chain fatty acids can enhance IgA generation in mice by promoting energy production and mRNA expression of genes related to B cell differentiation.Citation132 Similarly, 26 weeks of oligosaccharide supplementation in infants from birth resulted in a significant increase in fecal SIgA independent of feeding modes.Citation133 As mentioned above, vitamin A (VA)-derived RA is essential for IgA CSR, while VA deficiency leads to a lack of IgA-secreting cells in the small intestines of mice.Citation134 In addition, an altered GM with decreased bacterial diversity and an increase in the abundance of Proteobacteria members in mice and children were evident.Citation135,Citation136 On the contrary, dietary VA supplementation in 0.5–3.0-year-old children elevated serum IgA levels, decreased diarrhea, and respiratory disease incidences during treatment.Citation137
In addition to enhancing the overall IgA response, as mentioned above, it is feasible to strengthen the IgA response against specific microbial antigens. Several immunizations with flagellin derived from Proteobacteria and Salmonella induced a persistent IgA response in mice, including increased serum and intestinal (S)IgA.Citation60 This flagellin immunization enhanced IECs and GM separation, reduced fecal flagellin levels, and protected mice from diet-induced MetS and chemically induced colitis while decreasing flagellin-producing Proteobacteria, Desulfovibrionaceae, in a TD manner.Citation60 In contrast, an impaired IgA response to flagellin/Proteobacteria resulted in persistent overexpansion of Proteobacteria members in both newborn and adult mice and an increase in susceptibility to MetS and intestinal injury as discussed above.Citation20,Citation22 Therefore, although the safety of flagellin immunization needs further careful determination, this may be a viable approach for controlling the overexpansion of Proteobacteria, a common dysbiosis related to the development of various diseases (for example, NEC, sepsis, malnutrition, and pediatric Crohn’s disease)Citation5,Citation75,Citation82,Citation138 in infants.
4.3 Direct supplementation with IgA
The strength of the active IgA repose in infants can be impracticable in some cases, such as in children with IgA-related gene deficiency or those less than 1 month of age. Therefore, direct supplementation of IgA in infants may be a better choice ().
The main purpose of IgA supplementation in infants is to ensure the normal maturation of their GM. Therefore, two key questions require answers: (1) What is a normal GM maturation process? (2) What is a normal IgA repertoire (i.e., the IgA repertoire that supports normal GM maturation)? As mentioned above, the maturation of early GM can be completed as a process from simple to complex composition and from aerobic/facultative anaerobic to anaerobic bacteria.Citation18 However, until now, a precise and overall determination of the composition-time changing schedule of normal GM maturation process, if it exists, has not been well elucidated at the human species level. The determination of this schedule is essential to guide the orchestration of this maturation process. Not only the amount but also the properties of IgA provided to infants affect GM maturation. As discussed above, mice carrying a knock-in mutation of Aicda exhibit a defect in SHM, but the IgA-generating CSR is not affected, which is accompanied with altered GM composition.Citation58 In addition, a high-fat diet induces changes in IgA repertoire in the spleen and intestine, including an increase in the unmutated IgA proportion, in mice.Citation139 Although milk SIgA was not evaluated, considering the gut origin of mammary IgA+ PCs,Citation4 it is reasonable to expect a change in the milk SIgA repertoire. Obese mothers display normal milk SIgA levels,Citation140 and the bacterial composition in the first neonatal stool (after meconium) of their infants delivered via C-section is also similar to that of infants born to mothers with normal body weight.Citation141 However, following the maturation of the GM, young children (about half and most of which were delivered via C-section and are breastfeeding, respectively) born to mothers with obesity showed a different GM composition and exhibited increased susceptibility to obesity compared to those born to mothers with normal body weight,Citation142,Citation143 which may be partially attributed to the differences in milk SIgA properties. Therefore, characterizing a basic IgA repertoire that supports normal GM maturation and a core microbial composition capable of inducing this basic IgA repertoire is essential for possible future applications.
How can this basic IgA repertoire and core microbial composition be identified? Direct investigation of the common properties of the IgA repertoire in human breast milk based on large-scale IgA sequencing seems to be a possible approach. However, the IgA repertoire varies significantly among individuals,Citation144 making it challenging to summarize commonalities, especially in populations or even at the species level. In addition, during homeostasis, polyreactivity represents the primary selective pressure during B cell maturation in the germinal centers, leading to the binding of a similar group of microbes among individuals.Citation82 These phenomena indicate that there is possibly no need to precisely determine the sequencing properties of the basic IgA repertoire. Instead, identifying the core microbial composition that shapes this basic IgA repertoire seems to be more practicable 105. During the late stage of pregnancy changes, the GM of women changes regularly, including an increase in Proteobacteria and Actinobacteria members, such as Bifidobacteria and Enterobacteriaceae.Citation48 Proteobacteria members can induce significant IgA responses,Citation111 suggesting a possible essential role of Proteobacteria in regulating the milk SIgA repertoire, which, in conjunction with the role of other GM taxa, warrants further investigation.
Another possible application is to inhibit the colonization and (or) promote the clearance of (potential) pathogens in infants using IgA against specific microbial antigens. Similar to the robust induction of anti-flagellin IgA via flagellin immunization in mice, repeated immunization of dietary cows with formaldehyde-inactivated C. difficile and partly disabled toxins A and B derived from C. difficile substantially induced an IgA response against C. difficile, increasing total milk SIgA and SIgA-against C. difficile.Citation145 Infants who were fed formula supplemented with immunoglobulins concentrated from the milk of cows immunized with E. coli showed a decreased incidence of diarrhea.Citation146 Although the potential roles of IgM and IgG cannot be excluded (specified below), E. coli-specific SIgA may play a central role,Citation5,Citation145 also providing a possible approach for the quantity of basic and specific antigen-targeted IgA produced.
The in vitro production of monoclonal or polyclonal IgA using specific antigen-selected B cells can be an avenue for generating highly pure IgA.Citation112 However, IgA generated using this method may require an additional combination of the SC, which is essential for the resistance to gastrointestinal digestion and the anchoring of IgA to mucus.Citation67
5. Conclusions and perspectives
During the extended process of co-evolution between GM and mammals, the host has developed a GM-dependent pattern of early-life immune education. This pattern is exceedingly stable throughout the evolution process, which is challenged by various modern medical and nutritional technologies such as C-section, antibiotic exposure, and formula feeding.Citation46 The disturbance of this pattern may be one reason for the increased incidence of various inflammatory diseases, such as allergies and obesity.Citation46,Citation147 As a key regulator of GM, IgA influences immune education indirectly by regulating GM maturation and directly affects the immune response.Citation4 Disruption of IgA function, including both passive SIgA from breast milk and the local intestinal IgA response, may be essential in impairing GM maturation and immune education processes. Recent studies have shown that IgA is implicated in GM maturationCitation22,Citation23 and the development of certain diseases such as NEC and malnutrition during early life,Citation5,Citation81,Citation82 indicating a potential role of IgA in shaping a healthy GM and disease prevention. However, studies regarding the role of IgA in early GM and immunity are still relatively lacking. More studies are warranted to further investigate the underlying mechanism(s) and find more possible implications of IgA in disease development. For example, in addition to NEC, disordered interactions between IgA and GM have been implicated in other diseases, including obesity,Citation19,Citation60 IBD,Citation78 and sepsis.Citation111 Although these results were obtained from adult mice or humans, similar patterns may exist in early life. In addition, milk SIgA has been reported to persistently inhibit the generation of intestinal RORγ+ Tregs in a GM- and early life-dependent manner,Citation4 and its influence on the development of diseases, such as IBD, merits further investigation.
In addition, although evidence is relatively scarce,Citation127,Citation138,Citation145 direct supplementation of SIgA or enhancement of the local intestinal IgA response may be possible avenues to regulate the early life GM maturation process and prevent gut dysbiosis-related diseases. The primary challenges of IgA supplementation in infants include preparation limitations, safety, practicability, and efficacy. IgA preparation can be achieved either in vivo using cows immunized with specific pathogens or colonized with core microbesCitation145 or in vitro using specific antigen-selected ASCs.Citation112 Efficacy is the foundation of this study. Although some studies have verified the efficiency of IgA supplementation in disease prevention,Citation146 thus far, none have evaluated its effect on GM maturation, which may be one of the central aspects for future application, as discussed above. Although a full investigation of the efficacy and safety of IgA supplementation (including IgA preparation strategies) in shaping early GM and disease prevention is difficult, it is relatively easy to preliminarily verify using IgA (Igha−/-)- and SIgA (Pigr−/-)-deficient mice, in these cases, SIgA can be prepared from the feces of WT dams.Citation148
Authors’ contributions
JG prepared the first draft and CR, XH, YY, WH, and JZ edited and approved the final manuscript.
Competing interests
The authors declare that they have no competing interests.
Additional information
Funding
References
- Leroy V, Sakarovitch C, Viho I, Becquet R, Ekouevi DK, Bequet L, Rouet F, Dabis F, Timite-Konan M, Group ADPS. Acceptability of formula-feeding to prevent HIV postnatal transmission, Abidjan, Cote d’Ivoire: ANRS 1201/1202 Ditrame Plus Study. Jaids Journal of Acquired Immune Deficiency Syndromes. 2007;44(1):77–21. doi:10.1097/01.qai.0000243115.37035.97.
- Tang L, Binns CW, Lee AH. Infant formula crisis in China: a cohort study in Sichuan province. J Health Popul Nutr. 2015;33:117.
- Karmaus W, Soto-Ramírez N, Zhang H. Infant feeding pattern in the first six months of age in USA: a follow-up study. Int Breastfeed J. 2017;12(1):48. doi:10.1186/s13006-017-0139-4.
- Ramanan D, Sefik E, Galván-Peña S, Wu M, Yang L, Yang Z, Kostic A, Golovkina TV, Kasper DL, Mathis D. An immunologic mode of multigenerational transmission governs a gut Treg setpoint. Cell. 2020. doi:10.1016/j.cell.2020.04.030.
- Gopalakrishna KP, Macadangdang BR, Rogers MB, Tometich JT, Firek BA, Baker R, Ji J, Burr AH, Ma C, Good M. Maternal IgA protects against the development of necrotizing enterocolitis in preterm infants. Nat Med. 2019;25(7):1110–1115. doi:10.1038/s41591-019-0480-9.
- Bäckhed F, Roswall J, Peng Y, Feng Q, Jia H, Kovatcheva-Datchary P, Li Y, Xia Y, Xie H, Zhong H. Dynamics and stabilization of the human gut microbiome during the first year of life. Cell Host Microbe. 2015;17(5):690–703. doi:10.1016/j.chom.2015.04.004.
- Raba AA, O’Sullivan A, Semberova J, Martin A, Miletin J. Are antibiotics a risk factor for the development of necrotizing enterocolitis—case-control retrospective study. Eur J Pediatr. 2019;178(6):923–928. doi:10.1007/s00431-019-03373-0.
- Melnik BC. The potential mechanistic link between allergy and obesity development and infant formula feeding. Allergy, Asthma & Clinical Immunology. 2014;10(1):37. doi:10.1186/1710-1492-10-37.
- Zheng W, Zhao W, Wu M, Song X, Caro F, Sun X, Gazzaniga F, Stefanetti G, Oh S, Mekalanos JJ. Microbiota-targeted maternal antibodies protect neonates from enteric infection. Nature. 2020;577(7791):543–548. doi:10.1038/s41586-019-1898-4.
- Knol J, Scholtens P, Kafka C, Steenbakkers J, Gro S, Helm K, Klarczyk M, Schöpfer H, Böckler H-M, Wells J. Colon microflora in infants fed formula with galacto-and fructo-oligosaccharides: more like breast-fed infants. J Pediatr Gastroenterol Nutr. 2005;40(1):36–42. doi:10.1097/00005176-200501000-00007.
- Korpela K, Salonen A, Vepsalainen O, Suomalainen M, Kolmeder C, Varjosalo M, Miettinen S, Kukkonen K, Savilahti E, Kuitunen M, et al. Probiotic supplementation restores normal microbiota composition and function in antibiotic-treated and in caesarean-born infants. Microbiome. 2018;6(1):182. doi:10.1186/s40168-018-0567-4.
- Paul HA, Collins KH, Nicolucci AC, Urbanski SJ, Hart DA, Vogel HJ, Reimer RA. Maternal prebiotic supplementation reduces fatty liver development in offspring through altered microbial and metabolomic profiles in rats. Faseb J. 2019;33(4):5153–5167.
- Yelin I, Flett KB, Merakou C, Mehrotra P, Stam J, Snesrud E, Hinkle M, Lesho E, McGann P, McAdam AJ. Genomic and epidemiological evidence of bacterial transmission from probiotic capsule to blood in ICU patients. Nat Med. 2019;25(11):1728–1732. doi:10.1038/s41591-019-0626-9.
- Freedman SB, Williamson-Urquhart S, Farion KJ, Gouin S, Willan AR, Poonai N, Hurley K, Sherman PM, Finkelstein Y, Lee BE. Multicenter trial of a combination probiotic for children with gastroenteritis. New England Journal of Medicine. 2018;379(21):2015–2026. doi:10.1056/NEJMoa1802597.
- Repa A, Thanhaeuser M, Endress D, Weber M, Kreissl A, Binder C, Berger A, Haiden N. Probiotics (Lactobacillus acidophilus and Bifidobacterium bifidum) prevent NEC in VLBW infants fed breast milk but not formula. Pediatr Res. 2015;77(2):381–388. doi:10.1038/pr.2014.192.
- Chang H-Y, Chen J-H, Chang J-H, Lin H-C, Lin C-Y, Peng -C-C. Multiple strains probiotics appear to be the most effective probiotics in the prevention of necrotizing enterocolitis and mortality: an updated meta-analysis. PLoS One. 2017;12(2):e0171579. doi:10.1371/journal.pone.0171579.
- Huus KE, Bauer KC, Brown EM, Bozorgmehr T, Woodward SE, Serapio-Palacios A, Boutin RCT, Petersen C, Finlay BB. Commensal Bacteria Modulate Immunoglobulin A Binding in Response to Host Nutrition. Cell Host Microbe. 2020;27(6):909–921 e5. doi:10.1016/j.chom.2020.03.012.
- Guo J, Huang W HX, You Y, Jicheng Z. Interaction between IgA and gut microbiota and its role in controlling metabolic syndrome. Obesity Reviews. 2020;22(4):1–15.
- Luck H, Khan S, Kim JH, Copeland JK, Revelo XS, Tsai S, Chakraborty M, Cheng K, Chan YT, Nøhr MK. Gut-associated IgA+ immune cells regulate obesity-related insulin resistance. Nat Commun. 2019;10(1):1–17. doi:10.1038/s41467-019-11370-y.
- Petersen C, Bell R, Klag KA, Lee S-H, Soto R, Ghazaryan A, Buhrke K, Ekiz HA, Ost KS, Boudina S. T cell–mediated regulation of the microbiota protects against obesity. Science. 2019;365(6451):eaat9351. doi:10.1126/science.aat9351.
- Kubinak JL, Petersen C, Stephens WZ, Soto R, Bake E, O’Connell RM, Round JL. MyD88 signaling in T cells directs IgA-mediated control of the microbiota to promote health. Cell Host Microbe. 2015;17(2):153–163. doi:10.1016/j.chom.2014.12.009.
- Mirpuri J, Raetz M, Sturge CR, Wilhelm CL, Benson A, Savani RC, Hooper LV, Yarovinsky F. Proteobacteria-specific IgA regulates maturation of the intestinal microbiota. Gut Microbes. 2014;5(1):28–39. doi:10.4161/gmic.26489.
- Rogier EW, Frantz AL, Bruno ME, Wedlund L, Cohen DA, Stromberg AJ, Kaetzel CS. Secretory antibodies in breast milk promote long-term intestinal homeostasis by regulating the gut microbiota and host gene expression. Proceedings of the National Academy of Sciences. 2014;111(8):3074–3079. doi:10.1073/pnas.1315792111.
- Younge N, McCann JR, Ballard J, Plunkett C, Akhtar S, Araújo-Pérez F, Murtha A, Brandon D, Seed PC. Fetal exposure to the maternal microbiota in humans and mice. JCI Insight. 2019;4(19):19. doi:10.1172/jci.insight.127806.
- Aagaard K, Ma J, Antony KM, Ganu R, Petrosino J, Versalovic J. The placenta harbors a unique microbiome. Sci Transl Med. 2014;6(237):237ra65–237ra65. doi:10.1126/scitranslmed.3008599.
- Collado MC, Rautava S, Aakko J, Isolauri E, Salminen S. Human gut colonisation may be initiated in utero by distinct microbial communities in the placenta and amniotic fluid. Sci Rep. 2016;6(1):1–13. doi:10.1038/srep23129.
- Rackaityte E, Halkias J, Fukui E, Mendoza V, Hayzelden C, Crawford E, Fujimura K, Burt T, Lynch S. Viable bacterial colonization is highly limited in the human intestine in utero. Nat Med. 2020;26(4):599–607. doi:10.1038/s41591-020-0761-3.
- De Goffau MC, Lager S, Sovio U, Gaccioli F, Cook E, Peacock SJ, Parkhill J, Charnock-Jones DS, Smith GC. Human placenta has no microbiome but can contain potential pathogens. Nature. 2019;572(7769):329–334. doi:10.1038/s41586-019-1451-5.
- Leiby JS, McCormick K, Sherrill-Mix S, Clarke EL, Kessler LR, Taylor LJ, Hofstaedter CE, Roche AM, Mattei LM, Bittinger K. Lack of detection of a human placenta microbiome in samples from preterm and term deliveries. Microbiome. 2018;6(1):1–11. doi:10.1186/s40168-018-0575-4.
- Liang G, Zhao C, Zhang H, Mattei L, Sherrill-Mix S, Bittinger K, Kessler LR, Wu GD, Baldassano RN, DeRusso P. The stepwise assembly of the neonatal virome is modulated by breastfeeding. Nature. 2020;581(7809):470–474.
- Wampach L, Heintz-Buschart A, Hogan A, Muller EE, Narayanasamy S, Laczny CC, Hugerth LW, Bindl L, Bottu J, Andersson AF. Colonization and succession within the human gut microbiome by archaea, bacteria, and microeukaryotes during the first year of life. Front Microbiol. 2017;8:738. doi:10.3389/fmicb.2017.00738.
- Gensollen T, Iyer SS, Kasper DL, Blumberg RS. How colonization by microbiota in early life shapes the immune system. Science. 2016;352(6285):539–544. doi:10.1126/science.aad9378.
- Akagawa S, Tsuji S, Onuma C, Akagawa Y, Yamaguchi T, Yamagishi M, Yamanouchi S, Kimata T, Sekiya, S.-i, et al. Effect of delivery mode and nutrition on gut microbiota in neonates. Ann Nutr Metab. 2019;74(2):132–139. doi:10.1159/000496427.
- Faith JJ, Guruge JL, Charbonneau M, Subramanian S, Seedorf H, Goodman AL, Clemente JC, Knight R, Heath AC, Leibel RL, et al. The long-term stability of the human gut microbiota. Science. 2013;341(6141):1237439. doi:10.1126/science.1237439.
- Bunker JJ, Flynn TM, Koval JC, Shaw DG, Meisel M, McDonald BD, Ishizuka IE, Dent AL, Wilson PC, Jabri B. Innate and adaptive humoral responses coat distinct commensal bacteria with immunoglobulin A. Immunity. 2015;43(3):541–553. doi:10.1016/j.immuni.2015.08.007.
- Vatanen T, Kostic A. D. d’Hennezel, E ; Siljander, H ; Franzosa, E A ; Yassour, M ; Kolde, R ; Vlamakis, H ; Arthur, T D ; Hämäläinen, A -m, Variation in microbiome LPS immunogenicity contributes to autoimmunity in humans Cell. 2016;165:842–853.
- Arrieta M-C, Stiemsma LT, Dimitriu PA, Thorson L, Russell S, Yurist-Doutsch S, Kuzeljevic B, Gold MJ, Britton HM, Lefebvre DL. Early infancy microbial and metabolic alterations affect risk of childhood asthma. Sci Transl Med. 2015;7(307):307ra152–307ra152. doi:10.1126/scitranslmed.aab2271.
- Lim ES, Zhou Y, Zhao G, Bauer IK, Droit L, Ndao IM, Warner BB, Tarr PI, Wang D, Holtz LR. Early life dynamics of the human gut virome and bacterial microbiome in infants. Nat Med. 2015;21(10):1228. doi:10.1038/nm.3950.
- Cadwell K. The virome in host health and disease. Immunity. 2015;42(5):805–813. doi:10.1016/j.immuni.2015.05.003.
- Kernbauer E, Ding Y, Cadwell K. An enteric virus can replace the beneficial function of commensal bacteria. Nature. 2014;516(7529):94–98. doi:10.1038/nature13960.
- Sansone CL, Cohen J, Yasunaga A, Xu J, Osborn G, Subramanian H, Gold B, Buchon N, Cherry S. Microbiota-dependent priming of antiviral intestinal immunity in Drosophila. Cell Host Microbe. 2015;18(5):571–581. doi:10.1016/j.chom.2015.10.010.
- Fan D, Coughlin LA, Neubauer MM, Kim J, Kim MS, Zhan X, Simms-Waldrip TR, Xie Y, Hooper LV, Koh AY. Activation of HIF-1α and LL-37 by commensal bacteria inhibits Candida albicans colonization. Nat Med. 2015;21(7):808. doi:10.1038/nm.3871.
- Wheeler ML, Limon JJ, Bar AS, Leal CA, Gargus M, Tang J, Brown J, Funari VA, Wang HL, Crother TR, et al. Immunological Consequences of Intestinal Fungal Dysbiosis. Cell Host Microbe. 2016;19(6):865–873. doi:10.1016/j.chom.2016.05.003.
- Schei K, Avershina E, Øien T, Rudi K, Follestad T, Salamati S, Ødegård RA. Early gut mycobiota and mother-offspring transfer. Microbiome. 2017;5(1):107. doi:10.1186/s40168-017-0319-x.
- Nash AK, Auchtung TA, Wong MC, Smith DP, Gesell JR, Ross MC, Stewart CJ, Metcalf GA, Muzny DM, Gibbs RA. The gut mycobiome of the Human Microbiome Project healthy cohort. Microbiome. 2017;5(1):1–13. doi:10.1186/s40168-017-0373-4.
- Renz H, Skevaki C. Early life microbial exposures and allergy risks: opportunities for prevention. Nat Rev Immunol. 2020;20(1):1–15. doi:10.1038/s41577-019-0258-9.
- Kubinak JL, Round JL. Do antibodies select a healthy microbiota? Nat Rev Immunol. 2016;16(12):767–774. doi:10.1038/nri.2016.114.
- Koren O, Goodrich JK, Cullender TC, Spor A, Laitinen K, Bäckhed HK, Gonzalez A, Werner JJ, Angenent LT, Knight R. Host remodeling of the gut microbiome and metabolic changes during pregnancy. Cell. 2012;150(3):470–480. doi:10.1016/j.cell.2012.07.008.
- Serrano MG, Parikh HI, Brooks JP, Edwards DJ, Arodz TJ, Edupuganti L, Huang B, Girerd PH, Bokhari YA, Bradley SP. Racioethnic diversity in the dynamics of the vaginal microbiome during pregnancy. Nat Med. 2019;25(6):1001–1011. doi:10.1038/s41591-019-0465-8.
- Weisz-Carrington P, Roux ME, Lamm ME. Plasma cells and epithelial immunoglobulins in the mouse mammary gland during pregnancy and lactation. The Journal of Immunology. 1977;119:1306–1309.
- Macpherson AJ, Geuking MB, McCoy KD. Homeland security: igA immunity at the frontiers of the body. Trends Immunol. 2012;33(4):160–167. doi:10.1016/j.it.2012.02.002.
- Chaudhuri J, Alt FW. Class-switch recombination: interplay of transcription, DNA deamination and DNA repair. Nat Rev Immunol. 2004;4(7):541–552. doi:10.1038/nri1395.
- Bunker JJ, Bendelac A. IgA Responses to Microbiota. Immunity. 2018;49(2):211–224. doi:10.1016/j.immuni.2018.08.011.
- Kawamoto S, Maruya M, Kato LM, Suda W, Atarashi K, Doi Y, Tsutsui Y, Qin H, Honda K, Okada T. Foxp3+ T cells regulate immunoglobulin a selection and facilitate diversification of bacterial species responsible for immune homeostasis. Immunity. 2014;41(1):152–165. doi:10.1016/j.immuni.2014.05.016.
- Reikvam DH, Derrien M, Islam R, Erofeev A, Grcic V, Sandvik A, Gaustad P, Meza‐Zepeda LA, Jahnsen FL, Smidt H. Epithelial‐microbial crosstalk in polymeric Ig receptor deficient mice. Eur J Immunol. 2012;42(11):2959–2970. doi:10.1002/eji.201242543.
- Fransen F, Zagato E, Mazzini E, Fosso B, Manzari C, El Aidy S, Chiavelli A, D’Erchia AM, Sethi MK, Pabst O. BALB/c and C57BL/6 mice differ in polyreactive IgA abundance, which impacts the generation of antigen-specific IgA and microbiota diversity. Immunity. 2015;43(3):527–540. doi:10.1016/j.immuni.2015.08.011.
- Kawamoto S, Tran TH, Maruya M, Suzuki K, Doi Y, Tsutsui Y, Kato LM, Fagarasan S. The inhibitory receptor PD-1 regulates IgA selection and bacterial composition in the gut. Science. 2012;336(6080):485–489. doi:10.1126/science.1217718.
- Wei M, Shinkura R, Doi Y, Maruya M, Fagarasan S, Honjo T. Mice carrying a knock-in mutation of Aicda resulting in a defect in somatic hypermutation have impaired gut homeostasis and compromised mucosal defense. Nat Immunol. 2011;12(3):264–270. doi:10.1038/ni.1991.
- Cullender TC, Chassaing B, Janzon A, Kumar K, Muller CE, Werner JJ, Angenent LT, Bell ME, Hay AG, Peterson DA. Innate and adaptive immunity interact to quench microbiome flagellar motility in the gut. Cell Host Microbe. 2013;14(5):571–581. doi:10.1016/j.chom.2013.10.009.
- Tran HQ, Ley RE, Gewirtz AT, Chassaing B. Flagellin-elicited adaptive immunity suppresses flagellated microbiota and vaccinates against chronic inflammatory diseases. Nat Commun. 2019;10(1):5650. doi:10.1038/s41467-019-13538-y.
- Koenen M, Van Montfrans J, Sanders E, Bogaert D, Verhagen L. Immunoglobulin A deficiency in children, an undervalued clinical issue. Clinical Immunology. 2019;209:108293. doi:10.1016/j.clim.2019.108293.
- Burgio G, Duse M, Monafo V, Ascione A, Nespoli L. Selective IgA deficiency: clinical and immunological evaluation of 50 pediatric patients. Eur J Pediatr. 1980;133(2):101–106. doi:10.1007/BF00441577.
- Shkalim V, Monselize Y, Segal N, Zan-Bar I, Hoffer V, Garty BZ. Selective IgA deficiency in children in Israel. J Clin Immunol. 2010;30(5):761–765. doi:10.1007/s10875-010-9438-x.
- Janzi M, Kull I, Sjöberg R, Wan J, Melén E, Bayat N, Östblom E, Pan-Hammarström Q, Nilsson P, Hammarström L. Selective IgA deficiency in early life: association to infections and allergic diseases during childhood. Clinical Immunology. 2009;133(1):78–85. doi:10.1016/j.clim.2009.05.014.
- Ludvigsson JF, Neovius M, Hammarström L. Association between IgA deficiency & other autoimmune conditions: a population-based matched cohort study. J Clin Immunol. 2014;34(4):444–451. doi:10.1007/s10875-014-0009-4.
- Hanson LA. Breastfeeding provides passive and likely long-lasting active immunity. Annals of Allergy, Asthma & Immunology. 1998;81(6):523–537. doi:10.1016/S1081-1206(10)62704-4.
- Dunne-Castagna VP, Mills DA, Lönnerdal B. Effects of milk secretory immunoglobulin A on the commensal microbiota. Milk, Mucosal Immunity, and the Microbiome: Impact on the Neonate, Karger Publishers. 2020;94:1–11.
- Morteau O, Gerard C, Lu B, Ghiran S, Rits M, Fujiwara Y, Law Y, Distelhorst K, Nielsen EM, Hill ED. An indispensable role for the chemokine receptor CCR10 in IgA antibody-secreting cell accumulation. The Journal of Immunology. 2008;181(9):6309–6315. doi:10.4049/jimmunol.181.9.6309.
- Lindner C, Thomsen I, Wahl B, Ugur M, Sethi MK, Friedrichsen M, Smoczek A, Ott S, Baumann U, Suerbaum S. Diversification of memory B cells drives the continuous adaptation of secretory antibodies to gut microbiota. Nat Immunol. 2015;16(8):880–888. doi:10.1038/ni.3213.
- Pabst O. New concepts in the generation and functions of IgA. Nat Rev Immunol. 2012;12(12):821–832. doi:10.1038/nri3322.
- Moor K, Diard M, Sellin ME, Felmy B, Wotzka SY, Toska A, Bakkeren E, Arnoldini M, Bansept F, Dal C, et al. protects the intestine by enchaining growing bacteria. Nature. 2017;544(7651):498–502. doi:10.1038/nature22058.
- Bridgman SL, Konya T, Azad MB, Guttman DS, Sears MR, Becker AB, Turvey SE, Mandhane PJ, Subbarao P, Scott JA. High fecal IgA is associated with reduced Clostridium difficile colonization in infants. Microbes and Infection. 2016;18(9):543–549. doi:10.1016/j.micinf.2016.05.001.
- Heida F, Harmsen H, Timmer A, Kooi E, Bos A, Hulscher J. Identification of bacterial invasion in necrotizing enterocolitis specimens using fluorescent in situ hybridization. Journal of Perinatology. 2017;37(1):67–72. doi:10.1038/jp.2016.165.
- Pammi M, Cope J, Tarr PI, Warner BB, Morrow AL, Mai V, Gregory KE, Kroll JS, McMurtry V, Ferris MJ. Intestinal dysbiosis in preterm infants preceding necrotizing enterocolitis: a systematic review and meta-analysis. Microbiome. 2017;5(1):31. doi:10.1186/s40168-017-0248-8.
- Dong X-D, Li X-R, Luan -J-J, Liu X-F, Peng J, Luo -Y-Y, Liu C-J. Bacterial communities in neonatal feces are similar to mothers’ placentae. Canadian Journal of Infectious Diseases and Medical Microbiology. 2015;26(2):90–94.
- Cortez J, Makker K, Kraemer D, Neu J, Sharma R, Hudak M. Maternal milk feedings reduce sepsis, necrotizing enterocolitis and improve outcomes of premature infants. Journal of Perinatology. 2018;38(1):71–74. doi:10.1038/jp.2017.149.
- Brawner KM, Yeramilli VA, Kennedy BA, Patel RK, Martin CA. Prenatal stress increases IgA coating of offspring microbiota and exacerbates necrotizing enterocolitis-like injury in a sex-dependent manner. Brain Behav Immun. 2020;89:291–299. doi:10.1016/j.bbi.2020.07.008.
- Palm NW, De Zoete MR, Cullen TW, Barry NA, Stefanowski J, Hao L, Degnan PH, Hu J, Peter I, Zhang W, et al. Immunoglobulin A coating identifies colitogenic bacteria in inflammatory bowel disease. Cell. 2014;158(5):1000–1010. doi:10.1016/j.cell.2014.08.006.
- Mathias A, Duc M, Favre L, Benyacoub J, Blum S, Corthésy B. Potentiation of polarized intestinal Caco-2 cell responsiveness to probiotics complexed with secretory IgA. Journal of Biological Chemistry. 2010;285(44):33906–33913. doi:10.1074/jbc.M110.135111.
- Lourenço M, Chaffringeon L, Lamy-Besnier Q, Pédron T, Campagne P, Eberl C, Bérard M, Stecher B, Debarbieux L, De Sordi L. The spatial heterogeneity of the gut limits predation and fosters coexistence of bacteria and bacteriophages. Cell Host Microbe. 2020;28(3). doi:10.1016/j.chom.2020.06.002.
- Huus KE, Rodriguez-Pozo A, Kapel N, Nestoret A, Habib A, Dede M, Manges A, Collard JM, Sansonetti PJ, Vonaesch P, et al. Immunoglobulin recognition of fecal bacteria in stunted and non-stunted children: findings from the Afribiota study. Microbiome. 2020;8(1):113. doi:10.1186/s40168-020-00890-1.
- Kau AL, Planer JD, Liu J, Rao S, Yatsunenko T, Trehan I, Manary MJ, Liu T-C, Stappenbeck TS, Maleta KM. Functional characterization of IgA-targeted bacterial taxa from undernourished Malawian children that produce diet-dependent enteropathy. Sci Transl Med. 2015;7(276):276ra24–276ra24. doi:10.1126/scitranslmed.aaa4877.
- Subramanian S, Huq S, Yatsunenko T, Haque R, Mahfuz M, Alam MA, Benezra A, DeStefano J, Meier MF, Muegge BD. Persistent gut microbiota immaturity in malnourished Bangladeshi children. Nature. 2014;510(7505):417–421. doi:10.1038/nature13421.
- Nakawesi J, This S, Hütter J, Boucard-Jourdin M, Barateau V, Muleta KG, Gooday L, Thomsen KF, López AG, Ulmert I. αvβ8 integrin-expression by BATF3-dependent dendritic cells facilitates early IgA responses to Rotavirus. Mucosal Immunol. 2020;14(1):53–67.
- Janzon A, Goodrich JK, Koren O, Waters JL, Ley RE, Group TS. Interactions between the Gut Microbiome and Mucosal Immunoglobulins A, M, and G in the Developing Infant Gut. Msystems. 2019;4(6):6. doi:10.1128/mSystems.00612-19.
- Akkerman R, Faas MM, De Vos P. Non-digestible carbohydrates in infant formula as substitution for human milk oligosaccharide functions: effects on microbiota and gut maturation. Crit Rev Food Sci Nutr. 2019;59(9):1486–1497. doi:10.1080/10408398.2017.1414030.
- Millet N, Solis NV, Swidergall M. Mucosal IgA prevents commensal Candida albicans dysbiosis in the oral cavity. Front Immunol. 2020;11:2448. doi:10.3389/fimmu.2020.555363.
- Qamar A, Aboudola S, Warny M, Michetti P, Pothoulakis C, LaMont JT, Kelly CP. Saccharomyces boulardii stimulates intestinal immunoglobulin a immune response to clostridium difficiletoxin a in mice. Infect Immun. 2001;69(4):2762–2765. doi:10.1128/IAI.69.4.2762-2765.2001.
- Mendonça FHBP, Santos SSFD, Faria IDSD, Gonçalves E Silva CR, Jorge AOC, Leão MVP. Effects of probiotic bacteria on Candida presence and IgA anti-Candida in the oral cavity of elderly. Braz Dent J. 2012;23(5):534–538. doi:10.1590/S0103-64402012000500011.
- Mukherjee PK, Sendid B, Hoarau G, Colombel J-F, Poulain D, Ghannoum MA. Mycobiota in gastrointestinal diseases. Nat Rev Gastroenterol Hepatol. 2015;12(2):77. doi:10.1038/nrgastro.2014.188.
- Tamburini S, Shen N, Wu HC, Clemente JC. The microbiome in early life: implications for health outcomes. Nat Med. 2016;22(7):713–722. doi:10.1038/nm.4142.
- Knoop KA, Gustafsson JK, McDonald KG, Kulkarni DH, Coughlin PE, McCrate S, Kim D, Hsieh C-S, Hogan SP, Elson CO. Microbial antigen encounter during a preweaning interval is critical for tolerance to gut bacteria. Science Immunology. 2017;2(18):eaao1314. doi:10.1126/sciimmunol.aao1314.
- Smith PM, Howitt MR, Panikov N, Michaud M, Gallini CA, Bohlooly-Y M, Glickman JN, Garrett WS. The microbial metabolites, short-chain fatty acids, regulate colonic Treg cell homeostasis. Science. 2013;341(6145):569–573. doi:10.1126/science.1241165.
- Li D-K, Chen H, Ferber J, Odouli R. Infection and antibiotic use in infancy and risk of childhood obesity: a longitudinal birth cohort study. The Lancet Diabetes & Endocrinology. 2017;5(1):18–25. doi:10.1016/S2213-8587(16)30281-9.
- Krishnamoorthy N, Khare A, Oriss TB, Raundhal M, Morse C, Yarlagadda M, Wenzel SE, Moore ML, Peebles RS, Ray A. Early infection with respiratory syncytial virus impairs regulatory T cell function and increases susceptibility to allergic asthma. Nat Med. 2012;18(10):1525–1530. doi:10.1038/nm.2896.
- Neumann C, Blume J, Roy U, Teh PP, Vasanthakumar A, Beller A, Liao Y, Heinrich F, Arenzana TL, Hackney JA. c-Maf-dependent T reg cell control of intestinal TH 17 cells and IgA establishes host–microbiota homeostasis. Nat Immunol. 2019;20(4):471–481. doi:10.1038/s41590-019-0316-2.
- Zimmermann J, Macpherson AJ. Breast Milk Modulates Transgenerational Immune Inheritance. Cell. 2020;181(6):1202–1204. doi:10.1016/j.cell.2020.05.030.
- Macpherson AJ, Gatto D, Sainsbury E, Harriman GR, Hengartner H, Zinkernagel RM. A primitive T cell-independent mechanism of intestinal mucosal IgA responses to commensal bacteria. Science. 2000;288(5474):2222–2226. doi:10.1126/science.288.5474.2222.
- Uchimura Y, Fuhrer T, Li H, Lawson MA, Zimmermann M, Yilmaz B, Zindel J, Ronchi F, Sorribas M, Hapfelmeier S. Antibodies set boundaries limiting microbial metabolite penetration and the resultant mammalian host response. Immunity. 2018;49(3):545–559. e5. doi:10.1016/j.immuni.2018.08.004.
- Xu L, Lochhead P, Ko Y, Claggett B, Leong RW, Ananthakrishnan AN. Systematic review with meta‐analysis: breastfeeding and the risk of Crohn’s disease and ulcerative colitis. Aliment Pharmacol Ther. 2017;46(9):780–789. doi:10.1111/apt.14291.
- Carbone F, Montecucco F, Sahebkar A. Current and emerging treatments for neonatal sepsis. Expert Opin Pharmacother. 2020;21(5):549–556. doi:10.1080/14656566.2020.1721464.
- Patelarou E, Girvalaki C, Brokalaki H, Patelarou A, Androulaki Z, Vardavas C. Current evidence on the associations of breastfeeding, infant formula, and cow’s milk introduction with type 1 diabetes mellitus: a systematic review. Nutr Rev. 2012;70(9):509–519. doi:10.1111/j.1753-4887.2012.00513.x.
- Gustafson CE, Higbee D, Yeckes AR, Wilson CC, De Zoeten EF, Jedlicka P, Janoff EN. Limited expression of APRIL and its receptors prior to intestinal IgA plasma cell development during human infancy. Mucosal Immunol. 2014;7(3):467–477. doi:10.1038/mi.2013.64.
- Koch MA, Reiner GL, Lugo KA, Kreuk LS, Stanbery AG, Ansaldo E, Seher TD, Ludington WB, Barton GM, IgG M. IgA antibodies dampen mucosal T helper cell responses in early life. Cell. 2016;165(4):827–841. doi:10.1016/j.cell.2016.04.055.
- Li H, Limenitakis JP, Greiff V, Yilmaz B, Schären O, Urbaniak C, Zünd M, Lawson MA, Young ID, Rupp S. Mucosal or systemic microbiota exposures shape the B cell repertoire. Nature. 2020;584(7820):274–278. doi:10.1038/s41586-020-2564-6.
- New JS, Dizon BL, Fucile CF, Rosenberg AF, Kearney JF, King RG. Neonatal exposure to commensal-bacteria-derived antigens directs polysaccharide-specific B-1 B cell repertoire development. Immunity. 2020;53(1):172–186. e6. doi:10.1016/j.immuni.2020.06.006.
- Hapfelmeier S, Lawson MA, Slack E, Kirundi JK, Stoel M, Heikenwalder M, Cahenzli J, Velykoredko Y, Balmer ML, Endt K. Reversible microbial colonization of germ-free mice reveals the dynamics of IgA immune responses. Science. 2010;328(5986):1705–1709. doi:10.1126/science.1188454.
- Dzidic M, Abrahamsson TR, Artacho A, Björkstén B, Collado MC, Mira A, Jenmalm MC. Aberrant IgA responses to the gut microbiota during infancy precede asthma and allergy development. Journal of Allergy and Clinical Immunology. 2017;139(3):1017–1025. e14. doi:10.1016/j.jaci.2016.06.047.
- Ogawa J, Sasahara A, Yoshida T, Sira MM, Futatani T, Kanegane H, Miyawaki T. Role of transforming growth factor-β in breast milk for initiation of IgA production in newborn infants. Early Hum Dev. 2004;77(1–2):67–75. doi:10.1016/j.earlhumdev.2004.01.005.
- Weemaes C, Klasen I, Göertz J, Beldhuis‐Valkis M, Olafsson O, Haraldsson A. Development of immunoglobulin A in infancy and childhood. Scand J Immunol. 2003;58(6):642–648. doi:10.1111/j.1365-3083.2003.01344.x.
- Wilmore JR, Gaudette BT, Atria DG, Hashemi T, Jones DD, Gardner CA, Cole SD, Misic AM, Beiting DP, Allman D. Commensal microbes induce serum IgA responses that protect against polymicrobial sepsis. Cell Host Microbe. 2018;23(3):302–311. e3. doi:10.1016/j.chom.2018.01.005.
- Bunker JJ, Erickson SA, Flynn TM, Henry C, Koval JC, Meisel M, Jabri B, Antonopoulos DA, Wilson PC, Bendelac A. Natural polyreactive IgA antibodies coat the intestinal microbiota. Science. 2017;358(6361):6361. doi:10.1126/science.aan6619.
- Harris V, Ali A, Fuentes S, Korpela K, Kazi M, Tate J, Parashar U, Wiersinga WJ, Giaquinto C, De Weerth C. Rotavirus vaccine response correlates with the infant gut microbiota composition in Pakistan. Gut Microbes. 2018;9(2):93–101. doi:10.1080/19490976.2017.1376162.
- Koenig Á, Diniz E, Barbosa MDA, Vaz SFC, F. A. C. Immunologic factors in human milk: the effects of gestational age and pasteurization. Journal of Human Lactation. 2005;21(4):439–443. doi:10.1177/0890334405280652.
- Caballero-Flores G, Sakamoto K, Zeng MY, Wang Y, Hakim J, Matus-Acuña V, Inohara N, Núñez G. Maternal immunization confers protection to the offspring against an attaching and effacing pathogen through delivery of IgG in breast milk. Cell Host Microbe. 2019;25(2):313–323. e4. doi:10.1016/j.chom.2018.12.015.
- Engström P, Hammarström L, Söder P, Smith C. Immunoglobulin levels in saliva in individuals with selective IgA deficiency: compensatory IgM secretion and its correlation with HLA and susceptibility to infections. J Clin Immunol. 1989;9(4):279–286. doi:10.1007/BF00918659.
- Catanzaro JR, Strauss JD, Bielecka A, Porto AF, Lobo FM, Urban A, Schofield WB, Palm NW. IgA-deficient humans exhibit gut microbiota dysbiosis despite secretion of compensatory IgM. Sci Rep. 2019;9(1):1–10. doi:10.1038/s41598-019-49923-2.
- Kuppala VS, Meinzen-Derr J, Morrow AL, Schibler KR. Prolonged initial empirical antibiotic treatment is associated with adverse outcomes in premature infants. J Pediatr. 2011;159(5):720–725. doi:10.1016/j.jpeds.2011.05.033.
- Ward TL, Knights D, Gale CA. Infant fungal communities: current knowledge and research opportunities. BMC Med. 2017;15(1):30. doi:10.1186/s12916-017-0802-z.
- Coker M, Hoen A, Dade E, Lundgren S, Li Z, Wong A, Zens M, Palys T, Morrison H, Sogin M. Specific class of intrapartum antibiotics relates to maturation of the infant gut microbiota: a prospective cohort study. BJOG: An International Journal of Obstetrics & Gynaecology. 2020;127(2):217–227. doi:10.1111/1471-0528.15799.
- Stoesser N, Eyre DW, Quan TP, Godwin H, Pill G, Mbuvi E, Vaughan A, Griffiths D, Martin J, Fawley W. Epidemiology of Clostridium difficile in infants in Oxfordshire, UK: risk factors for colonization and carriage, and genetic overlap with regional Cdifficile infection strains. PloS One. 2017;12(8):e0182307. doi:10.1371/journal.pone.0182307.
- Ferraris L, Couturier J, Eckert C, Delannoy J, Barbut F, Butel M-J, Aires J. Carriage and colonization of C difficile in preterm neonates: a longitudinal prospective study. PloS One. 2019;14(2):e0212568. doi:10.1371/journal.pone.0212568.
- Korpela K, Helve O, Kolho K-L, Saisto T, Skogberg K, Dikareva E, Stefanovic V, Salonen A, Andersson S, De Vos WM. Maternal Fecal Microbiota Transplantation in Cesarean-Born Infants Rapidly Restores Normal Gut Microbial Development: a Proof-of-Concept Study. Cell. 2020;2. doi:10.1016/j.cell.2020.08.047.
- Plaza-Diaz J, Ruiz-Ojeda FJ, Gil-Campos M, Gil A. Mechanisms of action of probiotics. Advances in Nutrition. 2019;10(suppl_1):S49–S66. doi:10.1093/advances/nmy063.
- Tanaka K, Tsukahara T, Yanagi T, Nakahara S, Furukawa O, Tsutsui H, Koshida S. Bifidobacterium bifidum OLB6378 simultaneously enhances systemic and mucosal humoral immunity in low birth weight infants: a non-randomized study. Nutrients. 2017;9(3):195. doi:10.3390/nu9030195.
- Mohan R, Koebnick C, Schildt J, Mueller M, Radke M, Blaut M. Effects of Bifidobacterium lactis Bb12 supplementation on body weight, fecal pH, acetate, lactate, calprotectin, and IgA in preterm infants. Pediatr Res. 2008;64(4):418–422. doi:10.1203/PDR.0b013e318181b7fa.
- Roggero P, Liotto N, Pozzi C, Braga D, Troisi J, Menis C, Giannì ML, Canani RB, Paparo L, Nocerino R. Analysis of immune, microbiota and metabolome maturation in infants in a clinical trial of Lactobacillus paracasei CBA L74-fermented formula. Nat Commun. 2020;11(1):1–13. doi:10.1038/s41467-020-16582-1.
- Corsello G, Carta M, Marinello R, Picca M, De Marco G, Micillo M, Ferrara D, Vigneri P, Cecere G, Ferri P, et al. Preventive Effect of Cow’s Milk Fermented with Lactobacillus paracasei CBA L74 on Common Infectious Diseases in Children: a Multicenter Randomized Controlled Trial. Nutrients. 2017;9(7):7. doi:10.3390/nu9070669.
- Wang Y, Liu L, Moore DJ, Shen X, Peek R, Acra SA, Li H, Ren X, Polk DB, Yan F. An LGG-derived protein promotes IgA production through upregulation of APRIL expression in intestinal epithelial cells. Mucosal Immunol. 2017;10(2):373–384. doi:10.1038/mi.2016.57.
- Yang C, Mogno I, Contijoch EJ, Borgerding JN, Aggarwala V, Li Z, Siu S, Grasset EK, Helmus DS, Dubinsky MC. Fecal iga levels are determined by strain-level differences in bacteroides ovatus and are modifiable by gut microbiota manipulation. Cell Host Microbe. 2020;3. doi:10.1016/j.chom.2020.01.016.
- Villot C, Chen Y, Pedgerachny K, Chaucheyras-Durand F, Chevaux E, Skidmore A, Guan L, Steele M. Early supplementation of Saccharomyces cerevisiae boulardii CNCM I-1079 in newborn dairy calves increases IgA production in the intestine at 1 week of age. J Dairy Sci. 2020;103(9):8615–8628. doi:10.3168/jds.2020-18274.
- Kim M, Qie Y, Park J, Kim CH. Gut microbial metabolites fuel host antibody responses. Cell Host Microbe. 2016;20(2):202–214. doi:10.1016/j.chom.2016.07.001.
- Scholtens PA, Alliet P, Raes M, Alles MS, Kroes H, Boehm G, Knippels LM, Knol J, Vandenplas Y. Fecal secretory immunoglobulin A is increased in healthy infants who receive a formula with short-chain galacto-oligosaccharides and long-chain fructo-oligosaccharides. J Nutr. 2008;138(6):1141–1147. doi:10.1093/jn/138.6.1141.
- Mora JR, Iwata M, Eksteen B, Song S-Y, Junt T, Senman B, Otipoby KL, Yokota A, Takeuchi H, Ricciardi-Castagnoli P. Generation of gut-homing IgA-secreting B cells by intestinal dendritic cells. Science. 2006;314(5802):1157–1160. doi:10.1126/science.1132742.
- Lv Z, Wang Y, Yang T, Zhan X, Li Z, Hu H, Li T, Chen J. Vitamin A deficiency impacts the structural segregation of gut microbiota in children with persistent diarrhea. J Clin Biochem Nutr. 2016;59(2):113–121.
- Xiao L, Chen B, Feng D, Yang T, Li T, Chen J. TLR4 may be involved in the regulation of colonic mucosal microbiota by vitamin a. Front Microbiol. 2019;10:268. doi:10.3389/fmicb.2019.00268.
- Lie C, Ying C, Wang E, Brun T, Geissler C. Impact of large-dose vitamin A supplementation on childhood diarrhoea, respiratory disease and growth. Eur J Clin Nutr. 1993;47:88–96.
- Brusaferro A, Cavalli E, Farinelli E, Cozzali R, Principi N, Esposito S. Gut dysbiosis and paediatric Crohn’s disease. Journal of Infection. 2019;78(1):1–7. doi:10.1016/j.jinf.2018.10.005.
- Pham T, Chng M, Roskin K, Jackson K, Nguyen K, Glanville J, Lee J, Engleman E, Boyd S. High-fat diet induces systemic B-cell repertoire changes associated with insulin resistance. Mucosal Immunol. 2017;10(6):1468–1479. doi:10.1038/mi.2017.25.
- Bachour P, Yafawi R, Jaber F, Choueiri E, Abdel-Razzak Z. Effects of smoking, mother’s age, body mass index, and parity number on lipid, protein, and secretory immunoglobulin A concentrations of human milk. Breastfeeding Medicine. 2012;7(3):179–188. doi:10.1089/bfm.2011.0038.
- Mueller NT, Shin H, Pizoni A, Werlang IC, Matte U, Goldani MZ, Goldani HA, Dominguez-Bello MG. Birth mode-dependent association between pre-pregnancy maternal weight status and the neonatal intestinal microbiome. Sci Rep. 2016;6(1):1–9. doi:10.1038/srep23133.
- Galley JD, Bailey M, Kamp Dush C, Schoppe-Sullivan S, Christian LM. Maternal obesity is associated with alterations in the gut microbiome in toddlers. PLoS One. 2014;9(11):e113026. doi:10.1371/journal.pone.0113026.
- Tun HM, Bridgman SL, Chari R, Field CJ, Guttman DS, Becker AB, Mandhane PJ, Turvey SE, Subbarao P, Sears MR, et al. Canadian healthy infant longitudinal development study, I., roles of birth mode and infant gut microbiota in intergenerational transmission of overweight and obesity from mother to offspring. JAMA Pediatr. 2018;172(4):368–377. doi:10.1001/jamapediatrics.2017.5535.
- Lindner C, Wahl B, Föhse L, Suerbaum S, Macpherson AJ, Prinz I, Pabst O. Age, microbiota, and T cells shape diverse individual IgA repertoires in the intestine. J Exp Med. 2012;209(2):365–377. doi:10.1084/jem.20111980.
- Schmautz C, Hillreiner M, Ballweg I, Pfaffl MW, Kliem H. Stimulated enrichment of Clostridium difficile specific IgA in mature cow’s milk. PloS One. 2018;13(4):e0195275. doi:10.1371/journal.pone.0195275.
- Tawfeek HI, Najim NH, Al-Mashikhi S. Efficacy of an infant formula containing anti-Escherichia coli colostral antibodies from hyperimmunized cows in preventing diarrhea in infants and children: a field trial. International Journal of Infectious Diseases. 2003;7(2):120–128. doi:10.1016/S1201-9712(03)90007-5.
- Cox LM, Blaser MJ. Antibiotics in early life and obesity. Nat Rev Endocrinol. 2015;11(3):182–190. doi:10.1038/nrendo.2014.210.
- Donaldson G, Ladinsky M, Yu K, Sanders J, Yoo B, Chou W-C, Conner M, Earl A, Knight R, Bjorkman P. Gut microbiota utilize immunoglobulin A for mucosal colonization. Science. 2018;360(6390):795–800. doi:10.1126/science.aaq0926.