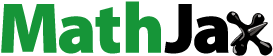
ABSTRACT
Childhood obesity and T2DM have shown a recent alarming increase due to important changes in global lifestyle and dietary habits, highlighting the need for urgent and novel solutions to improve global public health. Gut microbiota has been shown to be relevant in human health and its dysbiosis has been associated with MetS, a health condition linked to the onset of relevant diseases including T2DM. Even though there have been recent improvements in the understanding of gut microbiota–host interactions, pediatric gut microbiota has been poorly studied compared to adults. This review provides an overview of MetS and its relevance in school-age children, discusses gut microbiota and its possible association with this metabolic condition including relevant emerging gut microbiome-based interventions for its prevention and treatment, and outlines future challenges and perspectives in preventing microbiota dysbiosis from the early stages of life.
Introduction
Metabolic syndrome (MetS) has gained global relevance since it has shown to be strongly related to the onset of important maladies such as type-2 diabetes mellitus (T2DM) and cardiovascular diseases (CVD) and, nowadays, its prevalence in children and adolescents has been alarmingly increasing.Citation1 This metabolic condition is reversible and even preventable; therefore, early diagnosis and treatment are necessary to avoid future serious health complications and the premature onset of diseases. Recently, microbiome-based interventions are gaining popularity to treat and prevent metabolic disorders, as studies in mice suggest that there is a possible relationship between the gut microbiome and MetS.Citation2 Furthermore, research in humans has revealed discoveries regarding gut microbiota-host interactions, but most of it has been mainly conducted in adults due to constraints in ethical and practical considerations or even difficulties in acquiring samples from pediatric subjects, even though the gut microbiota of children have been shown to be more flexible to changes derived from environmental factors (i.e. diet) compared to adults, despite it being previously believed that the stabilization of gut microbial diversity occurred after the age of 3 years.Citation3
Metabolic syndrome and relevance of its study in pediatric subjects
Global childhood obesity had a dramatic 10-fold increase in the last four decades among children from 5 to 19 years old irrespective of socioeconomic status.Citation4 This is relevant since previous statistical studies in the Danish population have significantly correlated high body mass index (BMI) values in individuals from 7 years old to early adulthood with an increased risk of developing adult T2DM if this condition is maintained until puberty or later.Citation5 Moreover, it is predicted that 6–39% of pediatric subjects with obesity may also be suffering of MetS, presenting fluctuations in its prevalence depending on the applied diagnostic criteria since there are no standardized international criteria to diagnose this metabolic condition in children and adolescents.Citation6
MetS, also known as “Syndrome X”, is an acquired condition characterized by possessing at least three of the following cardio-metabolic abnormalities: high blood pressure, increased central adiposity, hyperglycemia, high triglyceride levels, and decreased high-density lipoprotein cholesterol (HDL-c) levels.Citation7 Its pathophysiology is a result of genetic predisposition, demographic background, and lifestyle factors such as sedentarism and diets rich in refined carbohydrates, sugars and fats.Citation8,Citation9 Notably, a relationship between gut microbiota and MetS was published a decade ago, where 16S rRNA sequencing determined that Toll-like receptor (TLR) 5 knock-out mice (T5KO) had gut microbial alterations linked with increased low-grade proinflammatory signaling that ended in MetS, validating it with gut microbiota transplantation from T5KO into wild-type (WT) germ-free mice, where all of the key features of MetS were developed after the procedure.Citation2
Bacteria are the most predominant microorganisms that inhabit the gut. Firmicutes, Bacteroidetes, Actinobacteria, Fusobacteria, Proteobacteria, and Verrucomicrobia are the most abundant phyla in humans, with 90% corresponding to Firmicutes and Bacteroidetes.Citation10 Moreover, the gut microbiota is shaped by multiple factors such as diet, drug treatments, and geographic region.Citation11–13 Previously, host genetics was believed to affect gut microbiota, but this remains unclear as no significant associations have been reported, suggesting that it may depend more on environmental factors.Citation14
Aging also seems to affect gut microbial communities. Studies in healthy Japanese subjects from 0 to 104 years old helped to classify them into two clusters: “adult-enriched” and “infant/elderly-enriched” gut microbiota. In children <20 years old, studies showed that the gut microbial maturation process was still taking place since alpha-diversity and abundance groups of some genera belonging to Lachnospiraceae, Bacteroides, and Bifidobacterium changed depending on the age. Interestingly, the categorization of young children fell into both “infant” and “adult” clusters despite their age, concluding that differences in the maturation process of humans also affect gut microbial composition.Citation15
Variations in the human gut microbial maturation process begin at the time of conception. Mode of birth and infant feeding seem to affect gut colonization, confirming that the maternal gut microbiota is important for a newborn.Citation16 Exhaustive studies have already been performed to characterize the human gut microbiota in individuals from 0 to 3 years old and in adulthood, but not in children from 3 to 18 years old due to difficulties obtaining samples or ethical/practical issues.Citation3 Few or no studies can be found related to the gut microbiota of school-age children and their associations with MetS and T2DM; meanwhile, overweight and obesity belong to the most studied metabolic disorders in this population [ near here].
Table 1. Relevant gut microbiota studies conducted in pediatric subjects from different countries to unravel their relationship with metabolic disorders related with MetS
Studies in Dutch healthy children and adults demonstrated that the former possess a greater abundance of Bifidobacterium spp. and Bacteroidetes phylum (including the genera Bacteroides and Prevotella), while Firmicutes phylum was more predominant in adults (including the genera Eubacterium, Clostridium, Dorea, and Coprococcus), contradicting studies where a greater abundance of Firmicutes and lower Bacteroidetes levels were found in American healthy children aged from 7 to 12 years old, concluding that geographic origins may also impact gut microbiota-host interactions.Citation27,Citation28
Moreover, the pediatric gut microbiota has shown to be more flexible to environmental changes rather than that of adults, enriched with functions that may support host’s development meanwhile in adults possess more inflammatory, adiposity, and obesity functionality.Citation28 Studies in Mexicans between 6 and 11 years old showed that the gut microbiota of obese individuals may resemble that in adults: obese children showed a higher abundance of Firmicutes and Actinobacteria and lower abundance of Bacteroidetes compared to normal weight children, which was similar to results obtained from Dutch adults.Citation27,Citation29 Interestingly, other studies revealed an increased richness and variability in gut microbial communities of obese Mexican children with a higher abundance of Firmicutes, Proteobacteria and Actinobacteria and a lower abundance of Bacteroidetes detected in obese individuals and obese with MetS individuals compared to normal weighted patients, similar to results obtained by Nirmalkar et al. (2018) in children from the same country.Citation23,Citation29
Apparently, it is not just lifestyle that can affect gut microbiota, but also aging, geographic region, and individual variability in the human microbial maturation process must be considered before designing gut microbiome–based interventions to treat or prevent MetS. Now, knowing that the gut microbiota of children is more flexible, additional efforts must be made to study this population which is prompt to respond positively and rapidly to microbial changes that may reverse deleterious medical conditions before developing irreversible diseases.
Gut microbiota and its possible relationship with metabolic syndrome
Humans possess their own genome but also carry the gut microbiome, which affects their metabolism. Gut bacteria are involved in highly relevant activities that are crucial in the well-functioning of human’s metabolism [ near here], but alterations in their composition may cause negative medical conditions. “Microbial dysbiosis” is the most popular term used to refer to these disparities, even though it has been recently cataloged as ambiguous due to the lack of a consensual definition within the scientific community and a poor characterization of how a healthy gut microbiota actually looks like.Citation30 Mostly, it is used to refer to an increased abundance of harmful bacteria and a disturbance in beneficial microbial communities, which has shown to be possibly associated with obesity, hypertension, alterations in the nervous system, liver diseases, T2DM, and other maladies.Citation31 For this reason, in the present review, this term and “microbial alterations” will be applied interchangeably.
Figure 1. General overview of gut microbiota and its relationship with human metabolism according to recent findings retrieved from conventional techniques and “omic” technologies: A. The predominance of a hypoxic environment due to the presence of strict anaerobic bacteria and SCFA producers has been related with a healthy condition, promoting immune homeostasis, preserving the integrity of the intestinal barrier, and potentially being involved in the well-functioning of key organs and tissues that are relevant in the onset of MetS. B. Loss of the hypoxic environment due to an increased presence of facultative anaerobic bacterial communities and PAMP producers has been related to gut dysbiosis, increased intestinal permeability, and the triggering of proinflammatory activity, potentially causing a negative impact in the functionality of key organs and tissues that are involved in the development of MetS
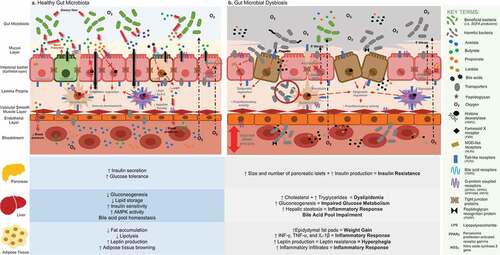
During the last few years, “omic” technologies have gained popularity for the study of gut microbial functional potential, taxonomy, and human metabolism.Citation32 The combination of metagenomics, transcriptomics, metabolomic, and proteomics has been useful for unraveling the relationship between microbiota and its host in multiple ways.Citation33,Citation34 However, conventional culture-based techniques are still needed as proof-of-concept, even though they can be labor-intensive and exclusively used with cultivable microorganisms.
Of note, the association of the gut microbiota with inflammation responses has shown to be highly relevant in the onset of MetS. Immune response and gut homeostasis seem to depend on gut microbiota–host bidirectional communication, which is mediated by the production and/or sensing of metabolites coming from the two involved.Citation35 However, alterations in the microbial composition of the gut also seem to have an effect on the human metabolome, causing positive and/or negative effects on health and disrupting metabolic activities [ near here]. Relevant bacterial products that have been related to MetS will be discussed herein.
Short-chain fatty acids
Short-chain fatty acids (SCFAs) result from the fermentation of undigested carbohydrates and dietary fibers in the gastrointestinal tract. Acetate, propionate, and butyrate are the most abundant variants found in humans: acetate is the most abundant in the bloodstream, butyrate is mainly used by colonocytes as energy source, and propionate is mainly metabolized by the liver.Citation36,Citation37 They translocate into the circulatory system through carrier-mediated transporters and nonionic diffusion,Citation38 helping to reach multiple organs and affect their functionality. Humans also possess free fatty acid (i.e., GPR43 and GPR41) and G protein-coupled receptors (i.e., GPR109A and OLFR78) to sense them in the gut epithelial layer, immune cells, pancreatic cells, adipose tissue, blood vessels, etc.Citation9,Citation39
These fatty acids provide protection, integrity, and energy to gut epithelial cells,Citation37 but they can also regulate the production of peptide-YY (PYY) and glucagon like peptide-1 (GLP-1) in L-cells, gut hormones that regulate appetite, food intake, and glucose-induced insulin secretion and sensitivity.Citation40,Citation41 SCFAs also seem to modulate and maintain pressure homeostasis in blood vesselsCitation42 and regulate energy and glucose metabolism in adipose tissue and pancreatic cells, helping to reduce body weight, increase leptin levels, and improve insulin secretion.Citation37,Citation43
Moreover, they also have the ability to confer protection against pathogens and external threats, acting over immune cells.Citation39 Butyrate and propionate inhibit histone deacetylases (HDACs), which are involved in DNA epigenetics, promoting anti-inflammatory (i.e. IL-18 and IL-10) and decreasing levels of proinflammatory cytokines (i.e. TNF-α, IFN-γ, and IL-6), maintaining homeostasis.Citation37,Citation44 The main SCFA producers belong to families of the Firmicutes phylum, but families from Fusobacteria, Actinobacteria, Thermotogue, and Spirochetes also seem to be butyrate producers. However, there are other communities belonging to Bifidobacterium and Lactobacillus genera that are not natural butyrate producers but can acquire this ability with the help of cross-feeding commensal bacteria producers of lactate and acetate, such as Faecalibacterium, Eubacterium, and Roseburia.Citation45
Mitochondrial β-oxidation of butyrate is key for the host’s gut homeostasis, preserving its hypoxic environment.Citation46 Studies in mice revealed that colonic epithelial hypoxia promotes the dominance of obligate anaerobes in the gut, such as SCFA producers, avoiding the increase in facultative anaerobes belonging to Enterobacteriaceae (i.e. Salmonella enterica serovar Typhimurium and Escherichia coli) which are microbial signatures of epithelial dysfunction.Citation47,Citation48 Thus, the lower abundance of SCFAs producers and increased facultative anaerobes may be a potential feature of gut dysbiosis. When gut microbial alterations take place, activation of the intestinal immune system occurs. A decrease in SCFA producers promote the gut’s microbial preference of less favorable substrates (i.e., mucins or dietary and endogenous proteins), causing an increase in intestinal permeability and the lower secretion of protective mucus,Citation45 allowing the translocation of bacteria and harmful metabolites into the bloodstream.Citation48
Metagenomic studies in the Chinese population showed a lower abundance of several butyrate-producing bacteria belonging to Firmicutes in prediabetic (Pre-DM) and treatment-naïve T2DM (TN-T2D) subjects and a higher abundance of Enterobacteriaceae, mostly dominated by E. coli. Also, a lower abundance of A. muciniphila and C. bartlettii was detected in TN-T2D compared to control and Pre-DM groups,Citation49 concluding that hypoxic conditions may be relevant for gut homeostasis. Akkermansia muciniphila has previously been negatively correlated with overweight, obesity, untreated T2DM, and hypertension, while Clostridium bartlettii has been negatively associated with insulin resistance (IR).Citation50,Citation51 Of note, it is difficult to consider SCFAs as potential biomarkers of gut dysbiosis since 90–95% of these molecules produced in the colonic lumen are absorbed in the gut mucosa,Citation44 making their quantification difficult in human samples; however, that does not mean that their study is not relevant to determine more about gut microbiota–host interactions.
Pathogen-associated molecular patterns
Pathogen-associated molecular patterns (PAMPs) trigger responses in the host since the gut immune system responds to environmental changes and endogenous threats through the activation of pattern recognition receptors (PRRs). Toll-like receptors (TRLs) are a family of PRRs that sense not just a broad spectrum of microbial-associated (MAMPs) and damage-associated molecular patterns (DAMPs), but also PAMPs. TLR2, TLR4, and TLR5 interact the most with the human gut microbiota, since they are found in cellular membranes of gut epithelial and immune cells.Citation52,Citation53
Lipopolysaccharide (LPS) is a membrane component of gram-negative bacteria such as Bacteroidetes and Proteobacteria that induces chronic low-grade inflammation, promoting obesity and IR through the activation of TLRs, mainly TLR4.Citation54,Citation55 Studies found that mice fed a 4-week high-fat diet had increased levels of circulatory LPS, promoting inflammation in adipose tissue due to potential diabetogenic proinflammatory cytokines (i.e. IL-1, IL-6 and TNF-α), weight gain, and liver IR.Citation56
Moreover, flagellin is another component that activates TLRs (mainly TLR5) and induces the production of proinflammatory cytokines (i.e. TNF-α, IL-6, and IL-1β).Citation52 Increased levels of flagellated bacteria, such as communities belonging to γ-Proteobacteria (i.e. motile pathobiont E. coli strains) and Firmicutes, have been correlated with multiple inflammatory diseases where MetS is involved, since they upregulate motility-related gene expression that boosts gut microbial production of flagellin, which increases microbiota encroachment into the mucus layer and proinflammatory potential within the host.Citation57
Metagenomic studies in normal-weighted Mexican children (NW), obese (O), and obese with MetS (OMS) from 7 to 10 years old revealed the higher abundance of Firmicutes and lower abundance of Bacteroidetes in O and OMS compared with NW children. Furthermore, OMS subjects seem to possess a higher abundance of Proteobacteria. Interestingly, introducing metatranscriptomics to this work helped to detect a significant prevalence of membrane and surface proteins in the transcriptome of these children, suggesting that bacterial components can be related to the onset of chronic inflammation that is responsible for metabolic disorders.Citation23 Something similar was seen in Chinese adults, where a higher abundance of unique Proteobacteria (mainly from Escherichia, Citrobacter, and Enterobacter) was detected in prediabetic subjects. In this study, coupling metaproteomic approaches also helped to reveal that 90% of the genes and meta-proteins found were assigned to Firmicutes, Bacteroides, and Proteobacteria, which have already been seen to be the main producers of LPS and flagellins.Citation49 This is how both studies show the relevance of bacterial membrane components in the study of inflammatory diseases, with several of them having been confirmed to trigger host immune responses and their abundance being dependent on the microbial composition of the gut.
Finally, peptidoglycan (PG) is a PAMP that is present in the outer membrane of gram-positive and gram-negative bacteria. It can be recognized by TLRs (mainly TLR2), secreted PG recognition proteins (PGLRPs) produced by the host, and nucleotide-binding oligomerization domain (NOD)-like receptors (NLRs). Some NLRs possess the ability to create “inflammasomes” that trigger proinflammatory cytokines (i.e. IL-1β and IL-18) related to the pathogenesis of inflammation in cases of obesity and IR.Citation58,Citation59 Recent studies have shown associations between PG and MetS in adult Drosophila models, where a decrease in insulin signaling was observed due to the activation of PG-dependent NF-kB, together with alterations in gut microbial communities;Citation58 however, this still needs to be confirmed in humans.
Bile acids
Bile acids (BAs) are products from hepatic cholesterol metabolism that help to absorb dietary lipids and vitamins.Citation60 Primary bile acids (PBAs) and secondary bile acids (SBAs) are also involved in the activation of intestinal nuclear farnesoid X receptor (FXR) which has a crosstalk interaction with G protein-coupled bile acid receptor (TGR5).Citation61,Citation62 FXR is more involved in GLP-1 secretion, helping to improve glucose and insulin sensitivity, while TGR5 is more focused on the browning process, energy metabolism, and decreasing obesity in adipose tissue.Citation63
The gut microbiota seems to be involved in BA metabolism since the deconjugation of PBAs in the small intestine is performed by bacterial bile salt hydrolases (BSH) from a wide variety of gram-positive (i.e., Bifidobacterium, Clostridium, Lactobacillus, Enterococcus, and Acetatifactor) and gram-negative bacteria (i.e., Bacteroides acidifaciens). Moreover, bacterial hydroxysteroid dehydrogenase (HSDH) from specific bacteria belonging to Bacteroides, Clostridium, Lactobacillus, Eubacterium, and Escherichia genera convert PBAs into SBAs, where cholic acid (CA) is converted into lithocholic acid (LCA) and chenodeoxycholic acid (CDCA) into deoxycholic acid (DCA) for their reabsorption and return to the liver.Citation64 During the bacterial processing of PBAs, BAs also exert antimicrobial properties due to their detergent effect on bacterial cell membranes, inducers of DNA damage, and disruptors of protein structures that may also affect gut microbiota.Citation65
Alterations in the BA pool have been related to colon cancer, inflammatory bowel disease, cholestatic liver disease, and MetS. When gut microbial alterations occur, the BA pool becomes dysregulated, acquiring an increased proinflammatory and cytotoxic potential that may affect the liver.Citation62 Metagenomic studies using mice cecal content showed that the abundance of Acetatifactor, belonging to the Clostridium cluster XIV, and Bacteroides depends on the activation of FXR. Both bacterial communities possess high BSH and 7α- and 7β-dehydroxylase activity that help in the production of LCA, which is involved in the activation of TGR5 signaling.Citation65
Metagenomic studies in Italian NW and O children showed an increased depletion of Bacteroides spp. in O subjects and a negative correlation with BMI z-score, supporting the idea that this genus is relevant in bile acid homeostasis.Citation19 Furthermore, metagenomic and metabolomic studies in Japanese adults proved what was previously found in mice: Clostridium cluster XIVa (i.e. C. scindens, C. hylemonae, Ruminococcus gnavus, and Peptostreptococcus productus) was lower in subjects with inflammatory diseases, specifically with Crohn’s disease and ulcerative colitis, compared to the control group and measurements of BAs in serum and feces showed that this cluster was probably the higher contributor of 7α-dehydroxylation of BAs to produce SBAs (LCA and DCA) and significant in 7β-dehydrogenation of BAs.Citation66
All of the above-mentioned facts highlight the relevance of FXR-TGR5 crosstalk mediated by the BA pool in the shaping of the gut microbiota and the importance of SBA producers in bile acid metabolism, glucose, and energy homeostasis. However, further research needs to be performed to understand the role of BAs in MetS, and it is necessary to study the effect of BA metabolism in the gut microbiota of children and compare it with that of adults. It has already been reported that the alternative or “acidic” pathway to produce PBAs in the liver seems to be preferred by human metabolism during childhood compared to the classic or “neutral” pathway that is more common in adults,Citation64 something that may affect the maturation process of gut microbiota through life.
Colonic gases
Hydrogen (H2) and carbon dioxide (CO2) are the major byproducts of microbial anaerobic fermentation, which can be released to the intestinal environment or taken by other bacteria.Citation62 For example, H2 can be used by bacteria to produce sulfide, acetate, and methane. The main bacterial producers of H2 belong to the Firmicutes and Bacteroidetes phyla (i.e., Roseburia, Ruminococcus, Bacteroides, Clostridum, and Eubacterium) and CO2 is produced by bacteria such as Clostridium species (i.e., C. sporogenes, C. butyricum, and C. perfringens), which also have the ability to produce both gases.Citation65,Citation67
Methanogens, which are mainly composed of archaea, use both gases to produce methane (CH4). Methanobrevibacter smithii is the only methanogen known to live within the human gut, but Methanosphaera stadtmanae can also sometimes be observed.Citation68 M. smithii has been related to increased BMI and impaired glucose intolerance in children and adults, respectively, but studies are still inconclusive since others report lower levels of this archaea in obese subjects.Citation69–71
Sulfate-reducing bacteria also use H2 to produce hydrogen sulfide (H2S). Desulfovibrio spp. is the most highly active species and greatest affinity bacteria for H2. Since both sulfate‐reducing bacteria and methanogens from the gut compete for the same pool of H2, the availability of sulfate for H2S production is the bottleneck for this preference.Citation65,Citation67 Desulfovibrio piger has been associated with abundance of Collinsella aerofaciens, an Actinobacterium that conducts sugar fermentation removing H2, lactate, and formate. This is relevant since C. aerofaciens has been linked with BA metabolism, the regulation of blood cholesterol, the production of SCFAs, and gut homeostasis.Citation72,Citation73 However, production of H2S in the gastrointestinal tract seem to also be related in arterial blood pressure homeostasis and provide cardioprotective effects, but mechanisms are still unclear.Citation72 Of note, a higher concentration of H2S seems to be relevant for liver and adipose tissue since it has been shown to promote the regulation of insulin sensitivity, stimulate hepatic gluconeogenesis and glycogenolysis, inhibit glucose and glycogen storage usage, and regulate lipolysis, inflammation, and adipokine production.Citation74
Finally, acetogenesis also requires H2 as a substrate, contributing positively to energy harvest. Firmicutes phylum has been shown to be the largest group of characterized gut acetogens in several mammals.Citation67,Citation68 Blautia hydrogenotrophica and Ruminococcus bromii are some examples of bacteria involved in formate uptake to produce acetate.Citation75 Metagenomics coupled with metabolomic and transcriptomic approaches concluded that human obese subjects with IR possessed a lower relative abundance of Firmicutes, while Bacteroidetes and Proteobacteria were increased compared to insulin-sensitive individuals. Firmicutes, specifically belonging to the Ruminococcaceae family, were positively correlated with subcutaneous adipose tissue, plasma acetate, and insulin sensitivity in brown adipocytes,Citation76 inferring their relevance in obesity and MetS. Moreover, studies in Mexican children showed that Ruminococcaceae UCG-002 was present in normal weighted individuals and appeared to be negatively associated with obesity but positively associated with fasting plasma insulin, highlighting the relevance of studying the role of the gut microbiota in human intermediate metabolism.Citation77
Branched-chain and aromatic amino acids
Branched-chain amino acids (BCAAs) have been associated with metabolic features related to MetS, the onset of T2DM, and CVDs. They are composed of leucine, isoleucine, and valine, which cannot be synthesized by humans but can be obtained from exogenous sources such as the diet.Citation78 It is believed that increased circulatory BCAA levels may be involved in the accumulation of incompletely oxidized fatty acids and glucose, impaired response to insulin, alterations in glucose homeostasis, and mitochondrial stress.Citation78,Citation79
Metabolomic and metagenomic studies in Danish adults found bacterial species with an enriched potential of BCAA biosynthesis and decreased BCAA bacterial inward transportation. Prevotella copri and Bacteroides vulgatus were the main bacterial species associated with IR, while Butyrivibrio crossotum and Eubacterium siraeum were negatively linked to this condition. Forty-one microbial functional modules were detected and correlated with IR and MetS phenotypes, mostly belonging to vitamin and cofactor biosynthesis, and BCAA, LPS, and transport systems. Methanogenesis, the inward transport of BCAAs, pyruvate oxidation and transport systems were more negatively associated with IR and Homeostatic Model Assessment for IR (HOMA-IR).Citation80
Moreover, metabolomic approaches in children detected high BCAA levels in Austrian subjects from 9 to 19 years old diagnosed with severe obesity, being positively correlated with liver fat content. Interestingly, liver fat content was also positively correlated with acylcarnitines C3-, C4-, and C5- which are BCAA degradation by-products, confirming the potential role of BCAAs in metabolic disparities.Citation81
On the other hand, aromatic amino acids (AAAs) such as tryptophan, phenylalanine and tyrosine have also been correlated with IR, T2DM, and CVDs. Studies in Chinese obese subjects showed high levels of circulatory BCAAs and AAAs coupled with the higher gut microbial capacity for producing them. AAAs showed a positive correlation with HOMA-IR, hyperlipidemia, inflammatory circulatory factors, and hyperglycemia. Additionally, B. thetaiotaomicron and B. ovatus were found to take and ferment AAAs, concluding that a depletion of Bacteroides spp. in obese subjects may potentially cause increased levels of AAAs.Citation82
Additionally, the production of deleterious microbial metabolites has also been related to several AAAs. Phenylalanine and tyrosine have been reported to be taken by certain gut microbial communities to produce phenolic end compounds such as phenol and p-cresol previously linked with cytotoxicity, genotoxicity, promoters of paracellular permeability and the reduction of endothelial barrier function in vitro, etc. Bacteria belonging to Enterococcaceae, Clostridiaceae, Staphylococcaceae, and Enterobacteriaceae have been shown to be predominant phenol-producers, while p-cresol has been found to be produced by certain Clostridium species, Bacteroidaceae, Bifidobacteriaceae, Eubacteriaceae, Lachnospiraceae, Porphyromonadaceae, Ruminococcaceae, Veillonellaceae and Fusobacteriaceae.Citation83,Citation84
Finally, in a 7.5-year longitudinal study from childhood to early adulthood in nondiabetic Finnish girls, serum concentrations of BCAAs and AAAs showed the strongest correlation with HOMA-IR. They concluded that IR starts to develop in early puberty and reached its peak in mid-puberty probably due to high metabolic and hormonal changes.Citation85 Gut microbiota was not analyzed, but it denotes the significance of researching these molecules in school-age children since gut microbial maturation may also be influenced by metabolic and hormonal alterations that are characteristic of puberty.
Gut microbiome-based interventions for the prevention and treatment of disorders related to metabolic syndrome
Prebiotics are gaining popularity since they serve as food for beneficial gut microbes, having a positive impact on human health. Studies in randomly selected obese, overweight, and healthy Canadian children from 7 to 12 years old showed the role of oligofructose-enriched inulin (OI) in the behavior and composition of the gut microbiota. After 16 week-consumption, subjects showed a significant reduction in body weight, body fat, and trunk fat. Moreover, they showed a relevant reduction of IL-6 levels and serum triglycerides compared to the placebo group. In addition, Bifidobacterium spp. (linked with normal-weight conditions) was increased and Bacteroides vulgatus (linked to IR) was decreased compared with the control group.Citation86
The discovery, characterization, and use of microorganisms that seem to be associated with health have also recently gained interest; for example, probiotics, which have already demonstrated interesting benefits in school-age children. A study conducted in Malaysian children from 7 to 10 years old with NW and O conditions that consumed a fermented probiotic drink enriched with Lactobacillus casei Shirota for 4 weeks showed significant increases in Lactobacillus and Bifidobacterium abundance compared to in the control groups. Additionally, fecal SCFA levels were altered since total SCFAs and propionic acid had a significant increase in NW and O after consuming the beverage compared to levels reported at the beginning of the intervention.Citation87 Moreover, studies in Japanese children from 5 to 10 years old given a probiotic drink enriched with the same microorganism had similar results after 6 months of consumption. They had an increase in Lactobacillus and Bifidobacterium but also a significant decrease in Enterobacteriaceae, Staphylococcus and Clostridium perfringens communities. However, these changes appeared to cease 6 months later when individuals arrested its consumption, concluding that the regular use of probiotics may help in the restoration of the gut microbiota.Citation88
Furthermore, dietary assessment may be helpful in the evaluation of diet- and nutrition-associated variations and their impact on gut microbial abundance and variability. A research in Filipino pediatric subjects with NW and O conditions was conducted to determine how differences in macronutrients, dietary fiber, and total energy intakes affect their gut environment and microbial communities. In the end, there were no significant differences in these dietetic components in their gut microbiota diversity and structure, since both study groups were shown to follow a mixture of traditional and Western nutritional patterns, deriving inconclusive results. However, the relative abundance of Bifidobacterium, Turicibacter and Clostridiaceae 1 was statistically higher in NW individuals compared to O subjects, and Lachnospira, Erysipelotrichaceae UCG-003 and Peptostreptococcaceae genera were statistically higher in O subjects with higher dietary fiber consumption compared to those who consume it in lower quantities.Citation89 Despite these inconclusive results, dietary assessment may be promising if inclusion/exclusion criteria are well established to work with more standardized study groups.
Finally, it is important to note that there have been no studies conducted within pediatric population to treat or prevent MetS, but they scarcely exist in adult subjects. A study reported in overweight/obese insulin-resistant adults supplemented with pasteurized Akkermansia muciniphila for 3 months showed a significant improvement in insulin sensitivity, and a decrease in plasma total cholesterol and insulinemia compared to the placebo group, with no significant alterations in gut microbiome structure. Moreover, it also showed a decrease in body weight, fat mass content, and hip circumference, but with no statistical significance.Citation50
Challenges and future perspectives
Studying pediatric subjects is a hard task due to the complexity of retrieving samples and considering variations in their hormonal and gut microbial maturation processes since the time of conception. However, knowing more about gut microbial flexibility and apparent enriched functionality that supports the host’s development may help to reveal interesting insights regarding gut microbiota and its possible association with MetS from the early stages of life. “Omic” technologies have helped during the last years to study gut microbiome–host interactions with the use of noninvasive biological samples but they cannot completely replace conventional techniques conducted in vitro and/or in vivo using animal models since they are still needed as proof-of-concept.
Some studies have shown that the gut microbiota of obese children may resemble that in adults, but this needs to be studied in more detail. Variations among both groups are important since diet, lifestyle, and aging have been shown to probably be involved in the shaping of the gut microbiota. Of note, there are other unknown and known features, such as genetics, drug consumption, and demographic background that may also be involved in gut microbial alterations, adding greater difficulty to its study. Moreover, increasing population-based cohort studies on gut microbiota composition found in countries with high rates of childhood obesity and MetS may also help to learn more about these microbial communities and their role in human metabolism at different stages of life, with greater accuracy, less variability, and the possibility of considering hormonal fluctuations that could affect these interactions.
Furthermore, interactions, even among microbial communities, are barely studied and must be considered in future research, since viruses, archaea, and fungi also inhabit the human gut. For all of the aforementioned, it is concluded that there is still a lot of work to do regarding the role of gut microbiota in MetS and other cardiometabolic conditions, but mysteries must be unraveled one step at a time to develop future and effective microbiome-based interventions that may help to improve the quality of life of children and adolescents that are now exposed to the increasing prevalence of obesity and MetS. The gut microbiota-brain axis was not reviewed here, but it should also not be disregarded since it has been shown to have an important role in human mechanisms of disease.
Acronyms
AAA – aromatic amino acid; BA – bile acid; BMI – body mass index; BCAA – branched-chain amino acid; BSH – bile salt hydrolase; CO2 – carbon dioxide; FXR – nuclear farnesoid X receptor; H2 – hydrogen; H2S – hydrogen sulfide; HOMA-IR – homeostatic model assessment for insulin resistance; IR – insulin resistance; LPS – lipopolysaccharide; MetS – metabolic syndrome; PAMP – Pathogen-associated molecular pattern; PBA – primary bile acid; PG – peptidoglycan; NOD – nucleotide-binding oligomerization domain; NLR – NOD-like receptor; NW – normal weight; O – obese; SBA – secondary bile acid; SCFA – short-chain fatty acid; TGR5 – G protein-coupled bile acid receptor; TLR – toll-like receptor; T2DM – type-2 diabetes mellitus.
Acknowledgments
The authors would like to thank CONACyT Mexican National Council for Research and Technology (AKCS CVU No. 875797) and Tecnologico de Monterrey for academic scholarship.
Disclosure statement
No potential conflict of interest was reported by the author(s).
References
- Saklayen MG. The global epidemic of the metabolic syndrome. Curr Hypertens Rep. 2018;20:12. doi:10.1007/s11906-018-0812-z.
- Vijay-Kumar M, Aitken JD, Carvalho FA, Cullender TC, Mwangi S, Srinivasan S, Sitaraman SV, Knight R, Ley RE, Gewirtz AT, et al. Altered Gut microbiota in mice lacking toll-like receptor 5. Science. 2010;328:228–15. doi:10.1126/science.1179721.
- Derrien M, Alvarez AS, de Vos WM. The Gut microbiota in the first decade of life. Trends Microbiol. 2019;27:997–1010. doi:10.1016/j.tim.2019.08.001.
- Garwood P, Chaib F, Brogan C. Tenfold increase in childhood and adolescent obesity in four decades: new study by Imperial College London and WHO. London (UK): World Health Organization; 2017 Oct 11 [accessed 2021 Jan 1]. https://www.who.int/news/item/11-10-2017-tenfold-increase-in-childhood-and-adolescent-obesity-in-four-decades-new-study-by-imperial-college-london-and-who.
- Bjerregaard LG, Jensen BW, Ängquist L, Osler M, TIA S, Baker JL. Change in overweight from childhood to early adulthood and risk of Type 2 diabetes. N Engl J Med. 2018;378:1302–1312. doi:10.1056/NEJMoa1713231.
- Weihe P, Weihrauch-Blüher S. Metabolic syndrome in children and adolescents: diagnostic criteria, therapeutic options and perspectives. Curr Obes Rep. 2019;8:472–479. doi:10.1007/s13679-019-00357-x.
- Magge SN, Goodman E, Armstrong SC. The metabolic syndrome in children and Adolescents: shifting the focus to cardiometabolic risk factor clustering. Pediatr. 2017:140. doi:10.1542/peds.2017-1603.
- Ranasinghe P, Mathangasinghe Y, Jayawardena R, Hills AP, Misra A. Prevalence and trends of metabolic syndrome among adults in the Asia-pacific region: a systematic review. BMC Public Health. 2017;17(1):101. doi:10.1186/s12889-017-4041-1.
- de Groot PF, Frissen MN, de Clercq NC, Nieuwdorp M. Fecal microbiota transplantation in metabolic syndrome: history, present and future. Gut Microbes. 2017;8(3):253–267. doi:10.1080/19490976.2017.1293224.
- Sircana A, Framarin L, Leone N, Berrutti M, Castellino F, Parente R, De Michieli F, Paschetta E, Musso G. Altered Gut Microbiota in Type 2 Diabetes: just a Coincidence? Curr Diab Rep. 2018;18:98. doi:10.1007/s11892-018-1057-6.
- Deschasaux M, Bouter KE, Prodan A, Levin E, Groen AK, Herrema H, Tremaroli V, Bakker GJ, Attaye I, Pinto-Sietsma SJ, et al. Depicting the composition of gut microbiota in a population with varied ethnic origins but shared geography. Nat Med. 2018;24(10):1526–1531. doi:10.1038/s41591-018-0160-1.
- Wu H, Esteve E, Tremaroli V, Khan MT, Caesar R, Mannerås-Holm L, Ståhlman M, Olsson LM, Serino M, Planas-Fèlix M, et al. Metformin alters the gut microbiome of individuals with treatment-naive type 2 diabetes, contributing to the therapeutic effects of the drug. Nat Med. 2017;23(7):850–858. doi:10.1038/nm.4345.
- Zhao L, Zhang F, Ding X, Wu G, Lam YY, Wang X, Fu H, Xue X, Lu C, Ma J, et al. Gut bacteria selectively promoted by dietary fibers alleviate type 2 diabetes. Science. 2018;359(6380):1151–1156. doi:10.1126/science.aao5774.
- Rothschild D, Weissbrod O, Barkan E, Kurilshikov A, Korem T, Zeevi D, Costea PI, Godneva A, Kalka IN, Bar N, et al. Environment dominates over host genetics in shaping human gut microbiota. Nature. 2018;555:210–215. doi:10.1038/nature25973.
- Odamaki T, Kato K, Sugahara H, Hashikura N, Takahashi S, Xiao J, Abe F, Osawa R. Age-related changes in gut microbiota composition from newborn to centenarian: a cross-sectional study. BMC Microbiol. 2016;16:90. doi:10.1186/s12866-016-0708-5.
- Liu Y, Qin S, Song Y, Feng Y, Lv N, Xue Y, Liu F, Wang S, Zhu B, Ma J, et al. The Perturbation of Infant Gut Microbiota Caused by Cesarean Delivery Is Partially Restored by Exclusive Breastfeeding. Front Microbiol. 2019;10:598. doi:10.3389/fmicb.2019.00598.
- Payne AN, Chassard C, Zimmermann M, Müller P, Stinca S, Lacroix C. The metabolic activity of gut microbiota in obese children is increased compared with normal-weight children and exhibits more exhaustive substrate utilization. Nutr Diabetes. 2011;1:e12. doi:10.1038/nutd.2011.8.
- Xu P, Li M, Zhang J, Zhang T. Correlation of intestinal microbiota with overweight and obesity in Kazakh school children. BMC Microbiol. 2012;12(1):283. doi:10.1186/1471-2180-12-283.
- Riva A, Borgo F, Lassandro C, Verduci E, Morace G, Borghi E, Berry D. Pediatric obesity is associated with an altered gut microbiota and discordant shifts in Firmicutes populations. Environ Microbiol. 2017;19:95–105. doi:10.1111/1462-2920.13463.
- Méndez-Salazar EO, Ortiz-López MG, Granados-Silvestre M, Palacios-González B, Menjivar M. Altered Gut Microbiota and Compositional Changes in Firmicutes and Proteobacteria in Mexican Undernourished and Obese Children. Front Microbiol. 2018;9:2494. doi:10.1007/s00394-017-1445-8.
- Bai J, Hu Y, Bruner DW. Composition of gut microbiota and its association with body mass index and lifestyle factors in a cohort of 7-18 years old children from the American Gut Project. Pediatr Obes. 2019;14(4):e12480. doi:10.1111/ijpo.12480.
- Zhao Y, Zhou J, Liu J, Wang Z, Chen M, Zhou S. Metagenome of Gut Microbiota of Children With Nonalcoholic Fatty Liver Disease. Front Pediatr. 2019;7:518. doi:10.3389/fped.2019.00518.
- Gallardo-Becerra L, Cornejo-Granados F, García-López R, Valdez-Lara A, Bikel S, Canizales-Quinteros S, López-Contreras BE, Mendoza-Vargas A, Nielsen H, Ochoa-Leyva A. Metatranscriptomic analysis to define the Secrebiome, and 16S rRNA profiling of the gut microbiome in obesity and metabolic syndrome of Mexican children. Microb Cell Fact. 2020:19,61. doi:10.1186/s12934-020-01319-y.
- Quiroga R, Nistal E, Estébanez B, Porras D, Juárez-Fernández M, Martínez-Flórez S, García-Mediavilla MV, de Paz JA, González-Gallego J, Sánchez-Campos S, et al. Exercise training modulates the gut microbiota profile and impairs inflammatory signaling pathways in obese children. Exp Mol Med. 2020;52(7):1048–1061. doi:10.1111/1462-2920.13463.
- Chen X, Sun H, Jiang F, Shen Y, Li X, Hu X, Shen X, Wei P. Alteration of the gut microbiota associated with childhood obesity by 16S rRNA gene sequencing. PeerJ. 2020;8:e8317. doi:10.7717/peerj.8317.
- Cho KY. Lifestyle modifications result in alterations in the gut microbiota in obese children. BMC Microbiol. 2021;21(1):10. doi:10.1002/1873-3468.13064.
- Zhong H, Penders J, Shi Z, Ren H, Cai K, Fang C, Ding Q, Thijs C, EE B, Stehouwer CDA, et al. Impact of early events and lifestyle on the gut microbiota and metabolic phenotypes in young school-age children. Microbiome. 2019;7:2. doi:10.1186/s40168-018-0608-z.
- Hollister EB, Riehle K, Luna RA, Weidler EM, Rubio-Gonzales M, Mistretta TA, Raza S, Doddapaneni HV, Metcalf GA, Muzny DM, et al. Structure and function of the healthy pre-adolescent pediatric gut microbiome. Microbiome. 2015;3:36. doi:10.1186/s40168-015-0101-x.
- Nirmalkar K, Murugesan S, Pizano-Zárate ML, Villalobos-Flores LE, García-González C, Morales-Hernández RM, Nuñez-Hernández JA, Hernández-Quiroz F, Romero-Figueroa M, del S, et al. Gut microbiota and endothelial dysfunction markers in obese mexican children and adolescents. Nutrients. 2018; 10: doi:10.3390/nu10122009.
- Brüssow H. Problems with the concept of gut microbiota dysbiosis. Microb Biotechnol. 2020;13(2):423–434. doi:10.1111/1751-7915.13479.
- Velmurugan G, Dinakaran V, Rajendhran J, Swaminathan K. Blood microbiota and circulating microbial metabolites in diabetes and cardiovascular disease. Trends Endocrinol Metab. 2020;31:835–847. doi:10.1016/j.tem.2020.01.013.
- Segal JP, Mullish BH, Quraishi MN, Acharjee A, Williams HRT, Iqbal T, Hart AL, Marchesi JR. The application of omics techniques to understand the role of the gut microbiota in inflammatory bowel disease. Therap Adv Gastroenterol. 2019:12. doi:10.1177/1756284818822250.
- Quince C, Walker AW, Simpson JT, Loman NJ, Segata N. Shotgun metagenomics, from sampling to analysis. Nat Biotechnol. 2017;35:833–844. doi:10.1038/nbt.3935.
- Wang X, Zhang A, Miao J, Sun H, Yan G, Wu F, Wang X. Gut microbiota as important modulator of metabolism in health and disease. RSC Adv. 2018;8:42380–42389. doi:10.1039/C8RA08094A.
- Burgueño JF, Abreu MT. Epithelial Toll-like receptors and their role in gut homeostasis and disease. Nat Rev Gastroenterol Hepatol. 2020;17:263–278. doi:10.1038/s41575-019-0261-4.
- Pingitore A, Gonzalez-Abuin N, Ruz-Maldonado I, Huang GC, Frost G, Persaud SJ. Short chain fatty acids stimulate insulin secretion and reduce apoptosis in mouse and human islets in vitro: role of free fatty acid receptor 2. Diabetes Obes Metab. 2019;21:330–339. doi:10.1111/dom.13529.
- Koh A, Vadder FD, Kovatcheva-Datchary P, From Dietary BF. Fiber to host physiology: short-chain fatty acids as key bacterial metabolites. Cell. 2016;165:1332–1345. doi:10.1016/j.cell.2016.05.041.
- Sivaprakasam S, Bhutia YD, Yang S, Ganapathy V. Short-chain fatty acid transporters: role in colonic homeostasis. Compr Physiol. 2017;8:299–314. doi:10.1002/cphy.c170014.
- Li M, van Esch BCAM, Wagenaar GTM, Garssen J, Folkerts G, Henricks PAJ. Pro- and anti-inflammatory effects of short chain fatty acids on immune and endothelial cells. Eur J Pharmacol. 2018;831:52–59. doi:10.1016/j.ejphar.2018.05.003.
- Christiansen CB, Gabe MBN, Svendsen B, Dragsted LO, Rosenkilde MM, Holst JJ. The impact of short-chain fatty acids on GLP-1 and PYY secretion from the isolated perfused rat colon. Am J of Physiol Gastrointest Liver Physiol. 2018;315:G53–G65. doi:10.1152/ajpgi.00346.2017.
- Kuhre RE, Christiansen CB, Saltiel MY, Albrechtsen NJW, Holst JJ. On the relationship between glucose absorption and glucose-stimulated secretion of GLP-1, neurotensin, and PYY from different intestinal segments in the rat. Physiol Rep. 2017;5:e13507. doi:10.14814/phy2.13507.
- Natarajan N, Hori D, Flavahan S, Steppan J, Flavahan NA, Berkowitz DE, Pluznick JL. Microbial short chain fatty acid metabolites lower blood pressure via endothelial G protein-coupled receptor 41. Physiol Genomics. 2016;48:826–834. doi:10.1152/physiolgenomics.00089.2016.
- Li X, Watanabe K, Kimura I. Gut microbiota dysbiosis drives and implies novel therapeutic strategies for diabetes mellitus and related metabolic diseases. Front Immunol. 2017;8:1882. doi:10.3389/fimmu.2017.01882.
- Parada Venegas D, De la Fuente MK, Landskron G, González MJ, Quera R, Dijkstra G, Harmsen HJM, Faber KN, Hermoso MA. Short Chain Fatty Acids (SCFAs)-mediated gut epithelial and immune regulation and its relevance for inflammatory bowel diseases. Front Immunol. 2019;10:277. doi:10.3389/fimmu.2019.00277.
- Peredo-Lovillo A, Romero-Luna HE, Jiménez-Fernández M. Health promoting microbial metabolites produced by gut microbiota after prebiotics metabolism. Food Res Int. 2020;136:109473. doi:10.1016/j.foodres.2020.109473.
- Cani PD. Gut cell metabolism shapes the microbiome. Science. 2017;357:548–549. doi:10.1126/science.aao2202.
- Byndloss MX, Olsan EE, Rivera-Chávez F, Tiffany CR, Cevallos SA, Lokken KL, Torres TP, Byndloss AJ, Faber F, Gao Y, et al. Microbiota-activated PPAR-γ signaling inhibits dysbiotic Enterobacteriaceae expansion. Science. 2017;357:570–575. doi:10.1126/science.aam9949.
- Makki K, Deehan EC, Walter J, Bäckhed F. The impact of dietary fiber on gut microbiota in host health and disease. Cell Host Microbe. 2018;23:705–715. doi:10.1016/j.chom.2018.05.012.
- Zhong H, Ren H, Lu Y, Fang C, Hou G, Yang Z, Chen B, Yang F, Zhao Y, Shi Z, et al. Distinct gut metagenomics and metaproteomics signatures in prediabetics and treatment-naïve type 2 diabetics. EBioMedicine. 2019;47:373–383. doi:10.1016/j.ebiom.2019.08.048.
- Depommier C, Everard A, Druart C, Plovier H, Van Hul M, Vieira-Silva S, Falony G, Raes J, Maiter D, Delzenne NM, et al. Supplementation with Akkermansia muciniphila in overweight and obese human volunteers: a proof-of-concept exploratory study. Nat Med. 2019;25:1096–1103. doi:10.1038/s41591-019-0495-2.
- Brahe LK, Le Chatelier E, Prifti E, Pons N, Kennedy S, Hansen T, Pedersen O, Astrup A, Ehrlich SD, Larsen LH. Specific gut microbiota features and metabolic markers in postmenopausal women with obesity. Nutr Diabetes. 2015;5:e159. doi:10.1038/nutd.2015.9.
- Hug H, Mohajeri MH, La Fata G. Toll-like receptors: regulators of the immune response in the human Gut. Nutrients. 2018;10:203. doi:10.3390/nu10020203.
- Yang J, Yan H. TLR5: beyond the recognition of flagellin. Cell Mol Immunol. 2017;14:1017–1019. doi:10.1038/cmi.2017.122.
- Aw W, Fukuda S. Understanding the role of the gut ecosystem in diabetes mellitus. Journal Diabetes Investig. 2018;9:5–12. doi:10.1111/jdi.12673.
- Saad MJA, Santos A, Prada PO. Linking Gut microbiota and inflammation to obesity and insulin resistance. Physiol. 2016;31:283–293. doi:10.1152/physiol.00041.2015.
- Cani PD, Amar J, Iglesias MA, Poggi M, Knauf C, Bastelica D, Neyrinck AM, Fava F, Tuohy KM, Chabo C, et al. Metabolic Endotoxemia Initiates Obesity and Insulin Resistance. Diabetes. 2007;56:1761–1772. doi:10.2337/db06-1491.
- Tran HQ, Ley RE, Gewirtz AT, Chassaing B. Flagellin-elicited adaptive immunity suppresses flagellated microbiota and vaccinates against chronic inflammatory diseases. Nat Commun. 2019;10:5650. doi:10.1038/s41467-019-13538-y.
- Zugasti O, Tavignot R, Royet J. Gut bacteria-derived peptidoglycan induces a metabolic syndrome-like phenotype via NF-κB-dependent insulin/PI3K signaling reduction in Drosophila renal system. Sci Rep. 2020;10:14097. doi:10.1038/s41598-020-70455-7.
- Platnich JM, Muruve DA. NOD-like receptors and inflammasomes: a review of their canonical and non-canonical signaling pathways. Arch Biochem and Biophys. 2019;670:4–14. doi:10.1016/j.abb.2019.02.008.
- Ðanić M, Stanimirov B, Pavlović N, Goločorbin-Kon S, Al-Salami H, Stankov K, Mikov M. Pharmacological applications of bile acids and their derivatives in the treatment of metabolic syndrome. Front Pharmacol. 2018;9:1382. doi:10.3389/fphar.2018.01382.
- Kim H, Fang S. Crosstalk between FXR and TGR5 controls glucagon-like peptide 1 secretion to maintain glycemic homeostasis. Laboratory Animal Research. 2018;34(4):140–146. doi:10.5625/lar.2018.34.4.140.
- Chen ML, Takeda K, Sundrud MS. Emerging roles of bile acids in mucosal immunity and inflammation. Mucosal Immunol. 2019;12:851–861. doi:10.1038/s41385-019-0162-4.
- Pathak P, Xie C, Nichols RG, Ferrell JM, Boehme S, Krausz KW, Patterson AD, Gonzalez FJ, Chiang JYL. Intestine farnesoid X receptor agonist and the gut microbiota activate G-protein bile acid receptor-1 signaling to improve metabolism. Hepatology. 2018;68:1574–1588. doi:10.1002/hep.29857.
- Rodríguez-Morató J, Matthan NR. Nutrition and gastrointestinal microbiota, microbial-derived secondary bile acids, and cardiovascular disease. Curr Atheroscler Rep. 2020;22:47. doi:10.1007/s11883-020-00863-7.
- Rowland I, Gibson G, Heinken A, Scott K, Swann J, Thiele I, Tuohy K. Gut microbiota functions: metabolism of nutrients and other food components. Eur J Nutr. 2018;57:1–24. doi:10.1007/s00394-017-1445-8.
- Murakami M, Iwamoto J, Honda A, Tsuji T, Tamamushi M, Ueda H, Monma T, Konishi N, Yara S, Hirayama T, et al. Detection of Gut dysbiosis due to reduced clostridium subcluster xiva using the fecal or serum bile acid profile. Inflamm Bowel Dis. 2018;24:1035–1044. doi:10.1093/ibd/izy022.
- Hylemon PB, Harris SC, Ridlon JM. Metabolism of hydrogen gases and bile acids in the gut microbiome. FEBS Lett. 2018;592:2070–2082. doi:10.1002/1873-3468.13064.
- Smith NW, Shorten PR, Altermann EH, Roy NC, McNabb WC. Hydrogen cross-feeders of the human gastrointestinal tract. Gut Microbes. 2019;10(3):270–288. doi:10.1080/19490976.2018.1546522.
- Singer-Englar T, Barlow G, Mathur R. Obesity, diabetes, and the gut microbiome: an updated review. Expert Review of Gastroenterology & Hepatology. 2019;13(1):3–15. doi:10.1080/17474124.2019.1543023.
- van de Pol JAA, van Best N, Mbakwa CA, Thijs C, Savelkoul PH, Arts ICW, Hornef MW, Mommers M, Penders J. Gut colonization by methanogenic archaea is associated with organic dairy consumption in children. Front Microbiol. 2017:8. doi:10.3389/fmicb.2017.00355.
- Crovesy L, Masterson D, Rosado EL. Profile of the gut microbiota of adults with obesity: a systematic review. Eur J Clin Nutr. 2020;74(9):1251–1262. doi:10.1038/s41430-020-0607-6.
- Tomasova L, Konopelski P, Ufnal UM. Gut bacteria and hydrogen sulfide: the new old players in circulatory system homeostasis. Molecules. 2016;21(11):1558. doi:10.3390/molecules21111558.
- Bag S, Ghosh TS, Das B. Complete Genome Sequence of Collinsella aerofaciens Isolated from the Gut of a Healthy Indian Subject. Genome Announc. 2017;5:e01361–17. doi:10.1128/genomeA.01361-17.
- Bełtowski J, Wójcicka G, Jamroz-Wiśniewska A. Hydrogen sulfide in the regulation of insulin secretion and insulin sensitivity: implications for the pathogenesis and treatment of diabetes mellitus. Biochem Pharmacol. 2018;149:60–76. doi:10.1016/j.bcp.2018.01.004.
- Gomez JAL, Mukhopadhya I, Duncan SH, Louis P, Shaw S, Collie-Duguid E, Crost E, Juge N, Flint HJ. Formate cross-feeding and cooperative metabolic interactions revealed by transcriptomics in co-cultures of acetogenic and amylolytic human colonic bacteria. Environ Microbiol. 2019;21(1):259–271. doi:10.1111/1462-2920.14454.
- Moreno-Navarrete JM, Serino M, Blasco-Baque V, Azalbert V, Barton RH, Cardellini M, Latorre J, Ortega F, Sabater-Masdeu M, Burcelin R, et al. Gut microbiota interacts with markers of adipose tissue browning, insulin action and plasma acetate in morbid obesity. Mol Nutr Food Res. 2018;62(3):1700721. doi:10.1002/mnfr.201700721.
- Vazquez‐Moreno M, Perez-Herrera A, Locia‐Morales D, Dizzel S, Meyre D, Stearns JC, Cruz M. Association of gut microbiome with fasting triglycerides, fasting insulin and obesity status in Mexican children. Pediatr Obes. 2020:e12748. doi:10.1111/ijpo.12748.
- Rousseau M, Guénard F, Garneau V, Allam-Ndoul B, Lemieux S, Pérusse L, Vohl M-C. Associations Between Dietary Protein Sources, Plasma BCAA and Short-Chain Acylcarnitine Levels in Adults. Nutrients. 2019;11(1):173. doi:10.3390/nu11010173.
- Chen T, Ni Y, Ma X, Bao Y, Liu J, Huang F, Hu C, Xie G, Zhao A, Jia W, et al. Branched-chain and aromatic amino acid profiles and diabetes risk in Chinese populations. Sci Rep. 2016;6(1):20594. doi:10.1038/srep20594.
- Pedersen HK, Gudmundsdottir V, Nielsen HB, Hyotylainen T, Nielsen T, Jensen BAH, Forslund K, Hildebrand F, Prifti E, Falony G, et al. Human gut microbes impact host serum metabolome and insulin sensitivity. Nature. 2016;535(7612):376–381. doi:10.1038/nature18646.
- Lischka J, Schanzer A, Hojreh A, Ssalamah AB, Item CB, Gier C, NK DW, TF M, Jakober I, Greber‐Platzer S, et al. A branched-chain amino acid-based metabolic score can predict liver fat in children and adolescents with severe obesity. Pediatr Obes. 2020;e12739. doi:10.1111/ijpo.12739.
- Liu R, Hong J, Xu X, Feng Q, Zhang D, Gu Y, Shi J, Zhao S, Liu W, Wang X, et al. Gut microbiome and serum metabolome alterations in obesity and after weight-loss intervention. Nat Med. 2017;23(7):859–868. doi:10.1038/nm.4358.
- Gryp T, Vanholder R, Vaneechoutte M, Glorieux G. P-Cresyl Sulfate Toxins (Basel). 2017;9:52. doi:10.3390/toxins9020052.
- Saito Y, Sato T, Nomoto K, Tsuji H. Identification of phenol- and p-cresol-producing intestinal bacteria by using media supplemented with tyrosine and its metabolites. FEMS Microbiol Ecol. 2018;94(9):fiy125. doi:10.5625/lar.2018.34.4.140.
- Zhang X, Ojanen X, Zhuang H, Wu N, Cheng S, Wiklund WP. Branched-chain and aromatic amino acids are associated with insulin resistance during pubertal development in girls. J Adolesc Health. 2019;65(3):337–343. doi:10.1016/j.jadohealth.2019.01.030.
- Nicolucci AC, Hume MP, Martínez I, Mayengbam S, Walter J, Reimer RA. Prebiotics reduce body fat and alter intestinal microbiota in children who are overweight or with obesity. Gastroenterology. 2017;153(3):711–722. doi:10.1053/j.gastro.2017.05.055.
- Joseph N, Vasodavan K, Saipudin NA, Yusof BNM, Kumar S, Nordin SA. Gut microbiota and short-chain fatty acids (SCFAs) profiles of normal and overweight school children in Selangor after probiotics administration. J Func Foods. 2019;57:103–111. doi:10.1016/j.jff.2019.03.042.
- Wang C, Nagata S, Asahara T, Yuki N, Matsuda K, Tsuji H, Takahashi T, Nomoto K, Yamashiro Y. Intestinal microbiota profiles of healthy pre-school and school-age children and effects of probiotic supplementation. Ann Nutr Metab. 2015;67(4):257–266. doi:10.1159/000441066.
- Golloso-Gubat MJ, Ducarmon QR, Tan RCA, Zwittink RD, Kuijper EJ, Nacis JS, Santos NLC. Gut microbiota and dietary intake of normal-weight and overweight filipino children. Microorganisms. 2020;8(7):1015. doi:10.3390/microorganisms8071015.