ABSTRACT
An imbalance in the crosstalk between the host and gut microbiota affects the intestinal barrier function, which results in inflammatory diseases and colorectal cancer. The colon epithelium protects itself from a harsh environment and various pathogenic organisms by forming a double mucus layer, primarily comprising mucins. Recent studies are focusing on how dietary patterns alter the gut microbiota composition, which in turn regulates mucin expression and maintains the intestinal layers. In addition, modulation of gut microbiota by microbiotic therapy (involving fecal microbiota transplantation) has emerged as a significant factor in the pathologies associated with dysbiosis. Therefore, proper communication between host and gut microbiota via different dietary patterns (prebiotics and probiotics) is needed to maintain mucus composition, mucin synthesis, and regulation. Here, we review how the interactions between diet and gut microbiota and bacterial metabolites (postbiotics) regulate mucus layer functionalities and mucin expression in human health and disease.
Introduction
Various bacteria, archaea, viruses, phages, yeast, protozoa, and fungi are present in the human body and are known as microbiota. These organisms are located in the skin, lungs, gut, vaginal, and urinal tracts of the body and form a symbiotic relationship because they associate with almost all human cells.Citation1,Citation2 Microbiota within a specific microenvironment such as the gut is named “gut microbiota”.Citation3 Around 1011 bacterial cells and 10 million bacterial genes (gut microbiome) in the large intestine contribute to a healthy metabolic status in the host. In the large intestine, the microbial population is very dense in the lumen and sparse near epithelial cells, though some bacterial species adhere and reside in the mucus layer.Citation4 In germ-free mice, mucus layer formation and mucin glycosylation pattern were distinct from conventionally raised mice due to the absence of microbiota.Citation5 Additionally, microbial products such as lipopolysaccharides and peptidoglycans in conventional mice stimulate mucus secretion.Citation6 Thus, the dynamic interplay between the gut microbiota and the host is important to maintain the intestinal mucus layer.
Diet, genetics, drugs, environmental factors, and the state of disease mediate gut dysbiosis, which results in the infiltration of bacterial components from the lumen to the mucus layer (). Individuals with low bacterial richness are more prone to metabolic disorders as compared to those with high bacterial richness.Citation7 In healthy individuals, the gut microbiota is linked with higher mucus layer thickness, which results in improved glucose metabolism by the short-chain fatty acids (SCFAs). In contrast, thinner mucus is linked with alterations in gut microbiota in disease,Citation8,Citation9 though the underlying mechanism and which microbiota are involved in forming the mucus layer are not known. Research on the role of microbiota in metabolic diseases waned in the early 2000s; however, more recent studies on the interdependence of gut microbiota in inflammatory bowel diseases (Ulcerative colitis, UC and Crohn’s disease, CD) and colorectal cancer (CRC),Citation10 revived interest in the field. For instance, fecal metagenomics analysis revealed several bacterial species (Bacteroides fragilis, Fusobacterium nucleatum, Porphyromonas asaccharolytica, Parvimonas micra, Prevotella intermedia, Alistipes finegoldii and Thermanaerovibrio acidaminovorans) enriched in CRC patients, which could serve as potential bacterial markers for the identification of CRC.Citation11,Citation12
Figure 1. Amelioration of gut microbiota and mucins. (1). Consumption of a Western-style diet (rich in high-fat calories and low in fiber) and other factors mediates microbiota alterations (dysbiosis) in the colon. (2). This altered microbiota, along with their metabolites, are responsible for increasing the intestinal permeability and reducing the mucus layer thickness by decreasing MUC2 expression; the result is the invasion of pathogens into the epithelium. (3). Administration of pre- (GOS and FOS) and probiotics (majorly bifidobacteria and lactobacilli) and prudent diet improves the intestinal layer functionalities and maintains proper gut health (eubiosis)
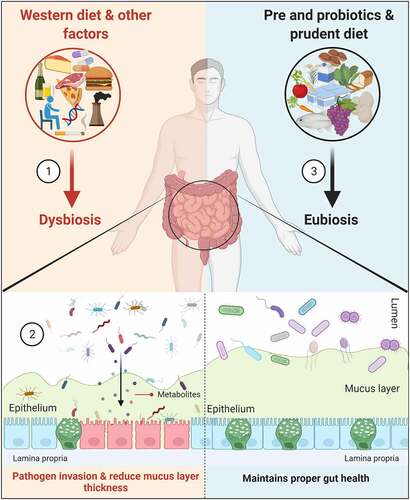
Bacteria in the colon convert dietary fiber into SCFAs, which act as an energy source to the host, particularly in the case of butyric acid.Citation13,Citation14 In the absence of dietary polysaccharides, Bacteroides thetaiotaomicron rely on mucin glycans as an energy source, which results in a reduced colonic mucus layer.Citation15 As compared to the small intestine (made up of a single mucus layer), the colon has two distinct mucus layers, i.e., outer (loose) and inner (tight) layers tethered to the epithelium.Citation16 The inner tight mucus layer is rich in mucin-2 (MUC2), secreted from the goblet cells as a disulfide cross-linked network and less colonized by commensal microorganisms.Citation17 The polysaccharide content, majorly O-linked glycans (80% of the mucin biomass) in the mucus layer, acts as an energy source for the gut bacteria.Citation18 One study found genetic deletion of Muc2 (Muc2−/-) is associated with the development of colitis-associated CRC in mice. Further, Muc2−/- mouse epithelium showed changes in mucosal thickening, increased proliferation, and superficial erosions in the distal colon due to reduced mucus layer thickness with altered microbiota.Citation19 Also, both proximal and distal colons in Muc2−/- mice are negative for the expression of mucins Muc5ac and Muc5b, while transient de novo expression of Muc6 was observed in the distal colon. The authors suggest that a small subset of goblet-like cells in the crypt base might be expressing Muc6 as a compensatory mechanism in Muc2−/- mice.Citation19
The symbiotic relationship between host and gut microbiota is important to maintain intestinal mucus layer homeostasis and prevents CRC. Hence, secretion of mucins by the goblet cells and degradation by the gut microbiota needs to be balanced.Citation20 The present review summarizes the evidence in animal and human studies elucidating the different strategies including prebiotics, probiotics, and postbiotics (metabolites secreted by the gut microorganisms) on gut microbiota modulation, mucus composition, and mucins regulation.
Interaction of diet and gut microbiota: CRC risk
CRC is the 3rd leading cause of cancer-related deaths in the USA, with an estimated 1,49,500 new cases and 52,980 deaths in 2021.Citation21 It is a multifactorial disease in which several genetic, environmental, and dietary factors are involved. Further, recent studies highlighted the central role of gut microbiota during the development of CRC. In the United States, around 50–60% of CRC incidents were estimated to be due to a change in lifestyle, especially diet;Citation22–24 however, the exact mechanism by which dietary factors cause CRC remains unknown. Several studies signify that these factors are strongly related to gut microbiota during the development of CRC.Citation25 Here, we are focusing only on dietary patterns that alter gut microbiota and their postbiotics role in CRC development, though the role of other factors has been extensively reviewed by others.Citation26–28
According to epidemiologic studies, sporadic CRC can be associated with the diet.Citation29 As shown in , a Western-style diet (rich in fat or sucrose, red and processed meat, and low in fiber) alters gut microbiota, and can influence the integrity of the intestinal mucus layer; however, the association between diet, gut microbiota, and mucus layer remain unclear.Citation30 Some of the studies delineating the role of dietary fibers in CRC have reported conflicting results.Citation31 This is due to the source of fiber (cereals vs. fruits) or individuals having a different composition of gut microbiota, variation in the treatment duration, and heterogeneity in tumor subtypes.Citation32 Recently, experts from World Cancer Research Fund and American Institute for Cancer Research in 2017 (https://www.wcrf.org/dietandcancer/colorectal-cancer) reported that consumption of red and processed meat (100 g/day, each) increases the risk of CRC, while intake of whole grains and dairy products (90 and 400 g/day) decreased the risk for CRC.Citation33 Another study, switched mice from chow to a western diet for 28 days and showed an increase in the permeability of the intestine and a decrease in the thickness of the inner mucus layer (especially reducedMuc2 polymeric network). By contrast, supplementing mice with inulin fiber along with Bifidobacterium longum sufficiently restored mucus growth.Citation30 The absence of dietary fiber in gnotobiotic mice (populated with known organisms) increases access of Citrobacter rodentium to the colonic mucus layer, damaging the mucosa and resulting in colitis and eventually CRC. The increase in C. rodentium results in higher expression of carbohydrate-active enzymes that in turn degrade mucins for their energy.Citation17 In contrast to this, colonization of butyrate-producing bacterium Butyrivibrio fibrisolvens in the presence of a high fiber diet (2% cellulose and 6% fructo-oligosaccharide/inulin) protected gnotobiotic mice from CRC development.Citation34
Interestingly, consumption of a western diet by Japanese migrants in Hawaii increased their risk of developing CRC, similar to the level observed in white individuals in the United States.Citation35 This is due to the high intake of red meat associated with altered activities of type I and II carcinogen-metabolizing enzymes, resulting in the formation of heterocyclic aromatic amines (HAAs).Citation35 These HAAs require activation by CYP1A2 and N-acetyltransferase before they can bind to the DNA.Citation36 Another study examined the association between diet and gut microbiota in African Americans (AAs), prone to CRC risk, and native Africans (NAs), with low CRC risk.Citation37 The study found that AAs consume more animal products such as meat, protein, saturated fat, and cholesterol compared with NAs, which was associated with higher colonic mucosal crypt proliferation and lower fecal Lactobacilli species.Citation37
Similarly, the same group also investigated how diet (fat and fiber) and gut microbiota mediate CRC risk in AAs and NAs.Citation38 When AAs switched to a high-fiber and low-fat diet for 2 weeks, they exhibited decreases in mucosal inflammation (CD3+ lymphocytes and CD68+ lamina propria macrophages) and secondary bile acid synthesis and increases in saccharolytic fermentation and butyrogenesis. These findings suggest consumption of less meat, fat and more carbohydrates and fiber might increase butyrogenesis, thereby mitigating CRC risk.Citation29,Citation37,Citation38 Recently, Fusobacterium nucleatum-infected patients showed a lower risk of CRC upon intake of a prudent diet, which was rich in whole grains and dietary fiber.Citation39 The increased amount of F. nucleatum in CRC tumor tissues was associated with poor survival,Citation40 though the exact mechanism by which dietary fiber decreased the risk of CRC is not known. The proposed mechanism is that greater fermentation of carbohydrates present in the prudent diet alters the SCFAs composition, which results in a change in pH, longer transit time in the gut, and greater immune surveillance to inhibit the colonization of F. nucleatum.Citation38,Citation39 Overall, the presence of distinct bacteria populations that prevent mucus layer damage, inflammation, and associated CRC depends on the consumption of specific dietary fibers and phytochemicals, also.
Phytochemicals are secondary plant metabolites that constitute dietary fiber which are poorly metabolized in the upper gastro-intestinal tract and modulate the intestinal microbiota in colon such as Akkermansia muciniphila, resulting in prevention of intestinal inflammation in mice.Citation41,Citation42 Supplementation of Akkermansia in the high-fat diet-fed mice restores the lipopolysaccharide mediated gut permeability and leakage and preserves the intestinal mucus layer thickness.Citation43 In addition to promoting anti-inflammation, treatment of polyphenols extracted from blueberries and olive oil also showed significant inhibition of growth in CRC cell lines (HCT-116 and HT-29) along with induction of cell cycle arrest, and apoptosis.Citation44,Citation45 Administration of phytochemical pterostilbene (structurally similar to resveratrol) decreased tumors in colon along with reduction of β-catenin and cyclin-D1 markers in chemically induced (Azoxymethane, AOM) CRC mice model.Citation46 Further, pterostilbene also led to decrease in pro-inflammatory cytokines, tumor necrosis factor-alpha, interleukin (IL)-1beta and IL-4 in mucosa suggesting its potential role in CRC prevention.Citation46 Taken together, phytochemicals and their microbial metabolites could be used as a complementary therapy against CRC while, its importance toward clinical trials depends on individual gut microbial composition.
Amelioration of gut microbiota and mucins: pre and probiotics
Modulation of gut microbiota by different interventions is needed to protect individuals at high risk of colitis and CRC. Various studies have shown that the inclusion of non-digestible carbohydrates in the diet called prebiotics improves gut microbiota. Prebiotics are “substrates that are selectively utilized by host microorganisms conferring health benefits to the host”.Citation47 The concept of using prebiotics was first defined by Glenn Gibson and Marcel Roberfroid in 1995.Citation48 Prebiotics are selectively fermented by probiotic microorganisms, leading to the production of SCFAs (acetate, propionate, and butyrate).Citation49 Further, SCFAs have different modes of action, such as acting as an energy source to the colonocytes, regulating MUC2 expression for the intestinal barrier function, and activating G-protein-coupled receptor signaling to modulate immune function ().Citation50 In addition, SCFAs also participate in the activation of AIM2 and NLRP3 inflammasomes, stimulating interleukin-18 production that results in improved epithelial barrier function.Citation50–52 Indeed, prebiotic supplementation decreased colonization of pathogens in human studies, potentially through SCFA action. The most common prebiotics are galacto-oligosaccharides (GOS) and fructose-oligosaccharides (FOS).Citation53 GOS is composed of oligo-galactose and produced commercially from lactose by the enzyme β-galactosidase; however, FOS is naturally present in chicory root, onion, garlic, asparagus, and banana, as well as synthesized commercially.Citation54 Here, we discuss the effect of GOS and FOS on the mucus layer functionalities, gut microbiota modulation, and CRC prevention.
Figure 2. Mechanism(s) of action of prebiotics and probiotics. The health benefits of prebiotics on the host are involved with various mechanisms, viz., 1) Selectively stimulate the growth of beneficial probiotic organisms. 2) Modulate various cytokines to inhibit the secretion of pro-inflammatory markers. 3) & 4) Release SCFAs and increase the absorption of minerals. In case of probiotics: A) Mediate the secretion of metabolites from the prebiotics (e.g., fiber) or directly involved in the inhibition of CRC tumor growth by increasing apoptosis. B) Suppress the growth of harmful bacteria by reducing intestinal luminal pH. C) Secrete the anti-microbial peptides (bacteriocins and β-defensins) to inhibit the growth of pathobionts. D) Decrease colonic inflammation by reducing Th17 and increasing Treg cells
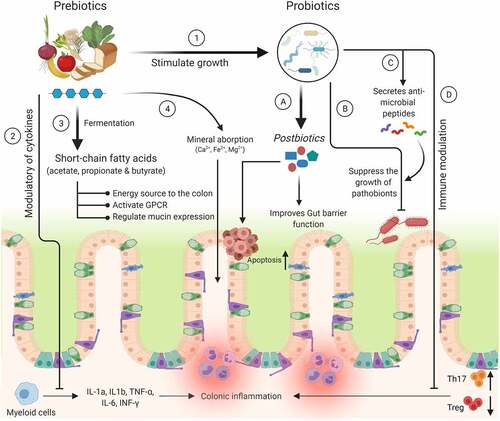
Due to its β (1–4) glycoside linkage, GOS is not metabolized in the small intestine and reaches the colon, where it is degraded by the gut microbiota to release SCFAs, lactate, and several gases.Citation55 It has been reported that GOS is involved in the modulation of the intestinal goblet cell and mucus barrier functions.Citation56 In addition, incubation of GOS with LS174T cells stimulates goblet cells to produce mucus and also decreases the expression of genes involved in mucus layer permeability.Citation56 Supplementation of GOS to germ-free rats resulted in reduced mucus distribution (neutral, acid, and sulfonated mucins) in the proximal colon compared with conventional rats. The reason might be due to more production of SCFAs by the microorganisms present in conventional rats.Citation57 In another study, feeding of GOS (5%) to BALB/c mice for 4 weeks led to higher expression of intestinal mucosa-associated mucins (Muc2 and Muc4) at the transcript level as compared to control animals.Citation58 Administration of GOS (derived from lactulose) for 20 weeks decreased colon tumor formation in a chemically (azoxymethane and dextran sodium sulfate) induced CRC rat model.Citation59 Furthermore, metagenomic sequencing of colon gut microbiota revealed that GOS-treated animals had significantly reduced pro-inflammatory microorganisms and increased beneficial Bifidobacterium levels compared with control animals.Citation59 Next, treatment of CRC line (LS174T) with GOS containing lactose (GOS-lac) resulted in a significant increase in the expression of MUC2 and its co-secreted molecule trefoil factor-3 (maintains mucus layer integrity) along with RELMβ (anti-parasitic activity); thus, GOS formulations may be effective in treating gut-associated disorders.Citation56 Studies have shown that intestinal alkaline phosphatase (ALP) has a protective role in inflammatory disease and also prevents bacterial invasion by detoxifying lipopolysaccharide.Citation60 Rats on a high-fat diet containing GOS showed elevated colonic ALP activity, improved intestinal barrier function, and greater microbial fermentation compared with rats without GOS, suggesting its importance in gut epithelial homeostasis.Citation61
Another non-digestible carbohydrate is FOS and beneficial to the host health because of its protective role in intestinal microbiota.Citation48 FOS have 2 to 7 fructose units with a β (2–1) linkage. Similar to GOS, FOS are not metabolized in the small intestine and reach the colon, where they stimulate endogenous bifidobacteria and lactobacilli species.Citation62 FOS supplementation (60 g/kg body weight) for 35 days resulted in a decrease in the number of aberrant crypt foci in the colon of rats with chemically (1, 2-dimethylhydrazine) induced CRC.Citation63 Similarly, in a genetically engineered ApcMin/+ mouse model, feeding with short-chain FOS (scFOS) delayed or reduced the development of adenoma tumors in the colon due to activation of anti-tumor immunity.Citation64 Moreover, feeding of FOS (50% in the diet) to growing rats led to an increase in Bifidobacterium and Lactobacillus populations and Muc4 (involved in intestinal epithelial cell differentiation, renewal and lubrication) expression compared with untreated and aging rats, suggesting that consumption of FOS could be effective in modulating the microbiota in younger rats.Citation65 Further, healthy human subjects ingesting FOS (12.5 g/day) showed a promising increase in fecal bifidobacteria and no effect on fecal total anaerobes, change in pH, nitroreductase, azoreductase, and β-glucuronidase activities, along with unchanged concentrations of bile acids and neutral sterols, which are involved in CRC progression.Citation66 On the contrary, consumption of scFOS (10 g/day for 3 months) resulted in an increase in butyrate (acts as the principal energy source for colonocytes and increases mucin production) levels in feces of patients with different sized adenomas (<10 mm or larger in diameter).Citation67 Overall, studies are focusing on the role of GOS and FOS in mucus production and prevention of intestinal metabolic disorders and CRC. However, the exact mode of action is not known. Therefore, supplementation of GOS/FOS in food could be a valuable strategy to treat these intestinal disorders and requires further investigation.
Probiotics are live microorganisms that, when administered in adequate amounts, confer a health benefit to the host.Citation68 The mechanisms of probiotics are briefly summarized in . Studies have shown that probiotic supplementation diminished symptoms in patients with inflammatory bowel diseases (IBDs) by enhancing the intestinal barrier at the mucosal surface.Citation69 Oral administration of clinically tested VSL#3 probiotic cocktail (having Lactobacilli, Bifidobacteria, and Streptococci, 3 × 109 cells) to rats for 7 days resulted in an increase in the mucin secretion by 60% in the colonic lumen and stimulated Muc2 and slight elevation of Muc1 and Muc3 gene expression upon exposed isolated rat colonic loops to the VSL#3 formula.Citation69 Further, incubation of colonic epithelial cell line (LS174T) with conditioned media from live VSL#3 bacteria did not stimulate mucin secretion. However, lactobacilli secreted products in the secretome showed promising mucin secretion. The upregulation of mucins is likely due to an increase in the activity of already differentiated goblet cells as a strategy for allowing colonization of the microbiota.Citation69 Similarly, potential probiotic Lactobacillus rhamnosus CNCM I-3690 (5 × 109 cfu) promotes the expression of endogenous protease inhibitor Kazal-type 4 (Spink4) and amino acid transporter SLC7A7 in specific pathogen-free C57BL/6 mice.Citation70 The upregulation of Spink4 inhibits the proteolytic degradation of epithelial and mucosal tissue in IBDs, while SLC7A7 increases the availability of the amino acids for the growth of intestinal epithelial cells.Citation71 Additionally, supplementation of CNCM I-3690 increased the production of IL-10 in the colon tissue to protect the mucus barrier by suppressing protein misfolding and ER stress in goblet cells. These results suggest that the administration of CNCM I-3690 could be a promising strategy to prevent gut barrier dysfunction.Citation70
Mucin regulation and intestinal barrier function by gut microbiota metabolites: postbiotics
Interaction of gut microbiota and the host is crucial for maintaining gut homeostasis. The crosstalk between the microbiota and host occurs via metabolites (postbiotics), which are released via fermentation from non-digestible compounds in the colon.Citation72 The metabolites or products derived from the microorganisms are effective in treating many diseases,Citation73 and these postbiotics are currently being used in clinical studies (). Below we describe the bacterial metabolites and their role in mucin regulation and intestinal mucus layer formation ().
Table 1. Clinical Studies reporting interventions with postbiotics products in infants, toddlers, and adults
Figure 3. Mucin regulation and intestinal barrier function by gut microbiota metabolites
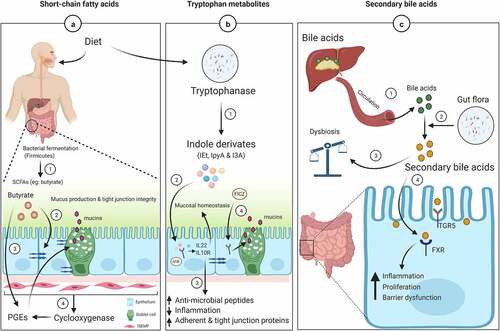
Short-chain fatty acids
SCFAs (acetate, propionate, and butyrate) are produced via bacterial fermentationCitation13,Citation14 and help to create a barrier between the gut epithelium and the external environment. Butyrate is mainly produced by Firmicutes bacteria and is the preferred metabolic substrate for colon epithelial cells, whereas acetate and propionate are predominantly produced by Bacteroidetes.Citation72 The role of butyrate in regulating mucin expression was evaluated in the HT29-Cl.16E CRC cell line (derived from HT-29) cultured in glucose-enriched media. Treatment with butyrate (2 or 5 mM) for 24 hours significantly increased the expression of MUC3 and MUC5B but decreased MUC5AC levels and had no effect on MUC2 expression in the presence of a glucose-high medium. Similar results were observed in the absence of glucose and the presence of butyrate, except in this case, MUC5AC and MUC2 levels were higher. These effects were specific to butyrate and not observed with acetate and propionate treatments, suggesting butyrate regulates mucin expression because it is a major energy source for the colonocytes.Citation74 Contrarily, both butyrate (1 mM) and propionate (1–15 mM) induced increased expression of MUC2 at the transcript levels in LS174T cells. MUC2 promoter analysis suggested that an active butyrate-responsive region containing an AP1 (c-Fos/c-Jun) cis-element is required for the activation of MUC2 via acetylation and methylation of histones.Citation75 Further, an investigation of the effect of butyrate on mucin secretion in LS174T CRC cells revealed the influence of beneficial probiotics (Lactobacillus and Bifidobacterium) species.Citation76 Treatment of LS174T cells with butyrate (6 or 9 mM) led to increased mucin protein content, which enhanced adherence of probiotic strains, thereby inhibiting pathogenic Escherichia coli (E.coli) attachment. Also, butyrate increased the expression MUC3, MUC4, and MUC12, while having no effect on MUC2 levels. The up-regulation of specific mucin genes was accompanied by upregulation of genes involved in mitogen-activated protein kinase signaling, which are associated with increased cell mass and cellular growth.Citation76 Rectal administration of butyrate (140 mM) resulted in decreased inflammation in mice challenged with Citrobacter rodentium. In addition, the expression of IL-10, Tgf-β, and Muc2 was elevated along with pathogen clearance genes (IL17A and IL1β) and genes involved in intestinal barrier repair and restoration (Relm, Tff3 and Myd88).Citation77
The intestinal mucus layer protects gut epithelial cells from various foreign objects by secreting mucus. This secretion can be influenced by physiological and immune modulators like prostaglandins (PGEs). Studies suggest that intestinal sub-epithelial myofibroblasts (ISEMF, present below the epithelium) regulate epithelial cell functions such as proliferation, differentiation, secretion, and motility.Citation78 During tissue injury or inflammation, ISEMF express cyclooxygenases (COX), responsible for the synthesis of PGEs (orchestrates inflammatory response) ()). Inhibition of COX through non-steroidal anti-inflammatory drugs results in intestinal lesions such as intestinal bleeding, perforation, ulcers, inflammation, and strictures requiring surgery.Citation79 Therefore, analogs of PGEs play an important role in protecting the intestinal mucus barrier. Co-culturing CRC cell lines (LS174T and T84) directly with intestinal myofibroblasts (CCD-18Co) in the presence of SCFAs (0.025–4.0 mM) as well PGE1or PGE2 (0.01–100 ng/ml) for 24 hours led to increased expression of MUC2 as compared to a monolayer cell culture system.Citation78
As stated above, dysbiosis is associated with several diseases, including IBDs. The levels of butyrate were significantly reduced in the stools of Japanese patients with UC and CD as compared to healthy individuals. In contrast, UC patients had higher levels of mucin O-glycans in their stools, likely due to the altered gut microbiota, which utilize O-glycans less efficiently. These findings suggest that O-glucans serve as an endogenous fermentation source for butyrate-producing bacteria.Citation80 Therefore, understanding the role of SCFAs, especially butyrate, in modulating mucus layer synthesis is crucial in the management of gastrointestinal diseases.
Tryptophan metabolite
Among the bacterial metabolites, tryptophan (Trp) is one of the essential amino acids supplied through the diet and plays an important role in intestinal permeability.Citation81 Most of the Trp (95%) is catabolized by the host enzyme indoleamin 2,3-dioxygenase 1 (IDO1) to produce kynurenine. In addition, some of the Trp (4–6%) is also degraded by the gut microorganisms (via tryptophanase) to produce indole derivatives (indole-3-ethanol-IEt, indole-3-pyruvate-IPyA, and indole-3-aldehyde-I3A and 3-indole-propionic acid, IPA), serotonin and tryptamine. These metabolites regulate intestinal barrier function by upregulating aryl hydrocarbon receptor (AhR, transcription factor) in mice and humans.Citation82,Citation83 Trp metabolites are thought to be produced by Lactobacillus reuteri and Clostridium sporogenes in mice and the human gut. The activation of AhR by indole derivatives results in IL-22 as well as IL-10 R secretion to stimulate anti-microbial peptide expression, epithelial cell proliferation, tight junction protein expression, and mucin production while inhibiting lipopolysaccharide-induced inflammation.Citation81,Citation84 The tryptophan metabolite signal also occurs through pregnane X receptor (PXR) to regulate intestinal integrity.Citation85 Mice deficient in PXR showed a leaky gut pathology that resulted in decreased Muc2 expression, suggesting Ahr and PXR pathways are necessary for the expression of Muc2.Citation85 Feeding rats a high-fat diet damages the epithelial barrier, which is reversed by IPA treatment via increased Muc2 and Muc4 expression, which strengthen the mucosal layer.Citation86,Citation87 In addition, IPA also up-regulates MUC2 expression in a colonic spheroid culture system.Citation88 A combination of Trp and Lactobacillus plantarum KLDS 1.0386 (high Trp-metabolic activity) treatment improved the epithelial and mucus barrier by decreasing the expression of pro-inflammatory cytokines (TNF-α, IL-1β, and IL-6) in adextran sodium sulfate (DSS)-induced colitis mouse model.Citation89 The combination also increased the expression of tight-junction proteins (ZO-1, claudin, and occluding), mucins (Muc1 and Muc2), and Trp metabolite (IAA) in the colon. IAA upregulates AHR transcript levels, resulting in activation of the IL-22/STAT3 signaling pathway.Citation89
Indole derivatives (IEt, IPyA, and I3A) maintain the apical junctional complex (AJC), which comprises tight junction (ZO1 and occludin) and adherent junction (E-cadherin and β-catenin) proteins.Citation90 A recent study demonstrated that diets rich in Trp ameliorates DSS-induced colitis in mice by supplying indole derivatives, which activate the AhR receptor. The mechanism by which these derivatives modulate AJC is by inhibiting activation of actin regulatory (ezrin and non-muscle myosin II) proteins, thereby decreasing intestinal permeabilityCitation83 ()). Similarly, a photoproduct of Trp (FICZ) activates AhR, resulting in enhanced MUC2 expression and goblet cell proliferation, and inhibition of bacterial infiltration in DSS-induced colitis mice. This finding suggests the upregulation of MUC2 by FICZ might be due to AhR-ERK signaling.Citation91 Therefore, a protein diet rich in Trp could be beneficial to the host by improving intestinal defenses.
Secondary bile acids
Metabolites from the gut microbiota as well as host-derived molecules play an important role in human metabolism. For example, bile acids (BAs) are synthesized from cholesterol in the host liver by the enzyme cholesterol 7 alpha-hydroxylase (CYP7A1) and subsequently conjugated to glycine or taurine to form bile salts.Citation92 The conjugated BAs are reabsorbed in the gut via apical bile salt transporters, whereas 5–10% of BAs are not reabsorbed and instead are metabolized by the gut microbiota to produce secondary BAs (deoxycholic and lithocholic acids).Citation92 These secondary BAs act as signaling molecules via nuclear farnesoid X receptor (FXR) and G-protein-coupled receptor (TGR5) to regulate many biological functionsCitation93 ()). A diet containing high-fat increases the secondary BAs, mostly deoxycholic acid (DCA), to induce dysbiosis by inhibiting the growth of Clostridium perfringens, Bacteroides fragilis, Lactobacilli, and Bifidobacteria, which are associated with intestinal tumors.Citation94 Supplementation with DCA (0–100 μM) induced higher expression of MUC2 and E-cadherin, while markedly decreasing the expression of tumor invasion and migratory molecules, i.e., Snail and MMP9, in gastric cancer cells.Citation95 The upregulation of MUC2 is useful for the prediction of gastric cancer and its prognosis.Citation95 Similar results were also observed with CRC cell line (H3) where DCA regulates MUC2 expression via multiple pathways (EGFR/PKC/Ras/Raf-1/MEK1/ERK/CREB, PI3/Akt/IkappaB/NF-kappaB).Citation96
Transfer of fecal microbiota from DCA-fed animals to Apcmin/+ mice along with the antibiotic streptomycin resulted in low-grade inflammation and increased tumor burden due to gut microbiota alteration.Citation97 In addition, DCA-fed animals also showed a reduction in the expression of E-cadherin and up-regulation of β‐catenin levels. The reduction of E-cadherin might be due to alteration of Fusobacterium nucleatum adhesin FadA, which is required for attaching E-cadherin on epithelial cells to mediate Wnt/β‐catenin signaling.Citation97,Citation98 In a later study, cholic acid-fed Apcmin/+ mice showed an increase in the mucin-degrading bacteria (Akkermansia and Bacteroides) and a decrease in SCFAs and MUC2 expression, resulting in cancer progression via STAT3 signaling.Citation99 Therefore, understanding the association between BAs and microbiota on intestinal epithelial cells is important in mucosal physiology.
Fecal microbiota transplantation
Recently, fecal microbiota transplantation (FMT) has gained importance for treating gut dysbiosis.Citation100 FMT involves transferring stools (in capsule form) from healthy individuals to patients with disease via an endoscope and nasoenteric tube.Citation101 The overall goal of FMT is to establish a new microbiota community in the gut to treat IBD, autoimmune disorders and metabolic diseases.Citation102 In the case of Clostridium difficile infection, which causes inflammation in the colon, FMT is used as a second-line treatment with a 92% success rate in clinical studies.Citation103,Citation104 FMT donors are mainly from two sources: patient-directed donors and universal donors via stool banks. Patient-directed donors are usually family members of the patient and are less frequently used because of cost.Citation105 However, universal donors (healthy volunteers) from stool banks have been widely used for FMT due to extensive screening procedures (https://www.openbiome.org/safety). Feces from CRC patients fed to conventional (treated with AOM) and germ-free mice showed high-grade dysplasia with increase in macroscopic polyps, tumor cell proliferation, and inflammation compared to healthy stool-fed mice.Citation106 Transfer of microbiota from wild mice to laboratory mice also showed resistance to chemically (AOM/DSS) induced CRC and improvement in the inflammation.Citation107 In addition to mouse models, application of FMT to piglets infected with E. coli K88 (to cause epithelial injury) enhanced beneficial bacteria, Lactobacillus and Succinivibrio, along with an increase in metabolites and metabolic pathways (branched-chain amino acids and linoleic acid metabolism).Citation108 Further, FMT modulated gut barrier function by decreasing intestinal permeability and increasing mucin (Muc2) and tight junction proteins (ZO-1 and occludin) in the piglets.Citation108 In a recent study, administration of feces from CRC patients in ApcMin/+ mice led to an increase in proliferation, pro-inflammatory cytokines, and decreased apoptosis in tumors cells.Citation109 Additionally, these mice also exhibited diminished gut barrier function tight junction proteins, ZO-1, occludin, and claudin3 and activation of Wnt signaling pathway.Citation109 In the future, FMT could be used as microbiotic therapy to treat various colonic diseases, and further clinical evidence is needed to establish its safety.
Conclusion and perspective
The gut microbiota and the host epithelial mucus layer are key players in maintaining and protecting the large intestine. Mucins, which are major components of mucus, not only act as a defense against pathogens but also serve as an energy source for the microorganisms. In disease states such as IBDs, CRC, and metabolic diseases, metabolites secreted from pathogenic bacteria reduce the thickness of the intestinal mucus layer. Therefore, researchers are focusing on ways to improve the gut barrier function and host health by various treatment strategies. Mostly, nutritional interventions (prebiotics, probiotics, and both) seems to improve the mucus layer by modulating the gut microbiota, and more studies are needed to establish their effective dose and safety concerns. Additional studies on the degradation of prebiotic fibers by specific probiotic microorganisms are needed to understand their mechanism of action in intestinal homeostasis. Similarly, metabolites produced during bacterial fermentation and their secondary metabolites facilitate the synthesis and regulation of mucins. Further research is needed to understand how dietary patterns regulate the mucins for mucus layer formation and modulation of gut microbiota, along with how FMT can be utilized in human health and disease. Below some of the fundamental questions that remain to be addressed are briefly listed below:
Can we modulation the gut-microbiota by prebiotics, probiotics, or both to treat inflammatory bowel diseases and colorectal cancer (CRC) patients?
How these dietary factors were effective in intestinal layer functionalities and improving gut-microbiota?
What the molecular mechanism(s) are involved in the regulation of mucins by postbiotics?
Is fecal microbiota transplantation being an effective strategy to restore the dysbiosis associated with CRC patients?
Can we use tumor (CRC) associated bacteria for pre-clinical and clinical studies as diagnostic or prognostic markers?
Acknowledgments
We thank Dr. Jessica Mercer for editing this manuscript. Figures are created with BioRender.com.
Disclosure statement
SKB is one of the co-founders of Sanguine Diagnostics and Therapeutics, Inc. Other authors declare no competing interests.
Additional information
Funding
References
- Cani PD. Human gut microbiome: hopes, threats and promises. Gut. 2018;67(9):1716–17. doi:10.1136/gutjnl-2018-316723.
- Cani PD, Van Hul M, Lefort C, Depommier C, Rastelli M, Everard A. Microbial regulation of organismal energy homeostasis. Nat Metab. 2019;1(1):34–46. doi:10.1038/s42255-018-0017-4.
- Knight R, Callewaert C, Marotz C, Hyde ER, Debelius JW, McDonald D, Sogin ML. The microbiome and human biology. Annu Rev Genomics Hum Genet. 2017;18(1):65–86. doi:10.1146/annurev-genom-083115-022438.
- Sender R, Fuchs S, Milo R. Are we really vastly outnumbered? Revisiting the ratio of bacterial to host cells in humans. Cell. 2016;164:337–340. doi:10.1016/j.cell.2016.01.013.
- Johansson ME, Jakobsson HE, Holmen-Larsson J, Schutte A, Ermund A, Rodriguez-Pineiro AM, Arike L, Wising C, Svensson F, Bäckhed F, et al. Normalization of host intestinal mucus layers requires long-term microbial colonization. Cell Host Microbe. 2015;18:582–592. doi:10.1016/j.chom.2015.10.007.
- Petersson J, Schreiber O, Hansson GC, Gendler SJ, Velcich A, Lundberg JO, Roos S, Holm L, Phillipson M. Importance and regulation of the colonic mucus barrier in a mouse model of colitis. Am J Physiol Gastrointest Liver Physiol. 2011;300:G327–33.
- Le Chatelier E, Nielsen T, Qin J, Prifti E, Hildebrand F, Falony G, Almeida M, Arumugam M, Batto J-M, Kennedy S, et al. Richness of human gut microbiome correlates with metabolic markers. Nature. 2013;500(7464):541–546. doi:10.1038/nature12506.
- Konig J, Wells J, Cani PD, Garcia-Rodenas CL, MacDonald T, Mercenier A, Whyte J, Troost F, Brummer R-J. Human intestinal barrier function in health and disease. Clin Transl Gastroenterol. 2016;7(10):e196. doi:10.1038/ctg.2016.54.
- Wells JM, Brummer RJ, Derrien M, MacDonald TT, Troost F, Cani PD, Theodorou V, Dekker J, Méheust A, de Vos WM, et al. Homeostasis of the gut barrier and potential biomarkers. Am J Physiol Gastrointest Liver Physiol. 2017;312(3):G171–G93. doi:10.1152/ajpgi.00048.2015.
- Tilg H, Adolph TE, Gerner RR, Moschen AR. The intestinal microbiota in colorectal cancer. Cancer Cell. 2018;33(6):954–964. doi:10.1016/j.ccell.2018.03.004.
- Dai Z, Coker OO, Nakatsu G, Wu WKK, Zhao L, Chen Z, Chan FKL, Kristiansen K, Sung JJY, Wong SH, et al. Multi-cohort analysis of colorectal cancer metagenome identified altered bacteria across populations and universal bacterial markers. Microbiome. 2018;6(1):70. doi:10.1186/s40168-018-0451-2.
- Wirbel J, Pyl PT, Kartal E, Zych K, Kashani A, Milanese A, Fleck JS, Voigt AY, Palleja A, Ponnudurai R, et al. Meta-analysis of fecal metagenomes reveals global microbial signatures that are specific for colorectal cancer. Nat Med. 2019;25:679–689. doi:10.1038/s41591-019-0406-6.
- Pothuraju R, Sharma RK, Onteru SK, Singh S, Hussain SA. Hypoglycemic and hypolipidemic effects of aloe vera extract preparations: a review. Phytother Res. 2016;30(2):200–207. doi:10.1002/ptr.5532.
- Morrison DJ, Preston T. Formation of short chain fatty acids by the gut microbiota and their impact on human metabolism. Gut Microbes. 2016;7(3):189–200. doi:10.1080/19490976.2015.1134082.
- Earle KA, Billings G, Sigal M, Lichtman JS, Hansson GC, Elias JE, Amieva M, Huang K, Sonnenburg J. Quantitative imaging of gut microbiota spatial organization. Cell Host Microbe. 2015;18(4):478–488. doi:10.1016/j.chom.2015.09.002.
- Pothuraju R, Krishn SR, Gautam SK, Pai P, Ganguly K, Chaudhary S, Rachagani S, Kaur S, Batra SK. Mechanistic and functional shades of mucins and associated glycans in colon cancer. Cancers (Basel). 2020;12(3):649.
- Desai MS, Seekatz AM, Koropatkin NM, Kamada N, Hickey Ca, Wolter M, Pudlo NA, Kitamoto S, Terrapon N, Muller A, et al. A dietary fiber-deprived gut microbiota degrades the colonic mucus barrier and enhances pathogen susceptibility. Cell. 2016;167(5):1339–53 e21. doi:10.1016/j.cell.2016.10.043.
- Johansson ME, Sjovall H, Hansson GC. The gastrointestinal mucus system in health and disease. Nat Rev Gastroenterol Hepatol. 2013;10(6):352–361. doi:10.1038/nrgastro.2013.35.
- Van der Sluis M, De Koning BA, De Bruijn AC, Velcich A, Meijerink JP, Van Goudoever JB, Büller HA, Dekker J, Van Seuningen I, Renes IB, et al. Muc2-deficient mice spontaneously develop colitis, indicating that MUC2 is critical for colonic protection. Gastroenterology. 2006;131(1):117–129. doi:10.1053/j.gastro.2006.04.020.
- Birchenough G, Schroeder BO, Backhed F, Hansson GC. Dietary destabilisation of the balance between the microbiota and the colonic mucus barrier. Gut Microbes. 2019;10(2):246–250. doi:10.1080/19490976.2018.1513765.
- Siegel RL, Miller KD, Fuchs HE, Jemal A. Cancer statistics, 2021. CA Cancer J Clin. 2021;71(1):7–33. doi:10.3322/caac.21654.
- Islami F, Goding Sauer A, Miller KD, Siegel RL, Fedewa SA, Jacobs EJ, McCullough ML, Patel AV, Ma J, Soerjomataram I, et al. Proportion and number of cancer cases and deaths attributable to potentially modifiable risk factors in the United States. CA Cancer J Clin. 2018;68(1):31–54. doi:10.3322/caac.21440.
- Rothschild D, Weissbrod O, Barkan E, Kurilshikov A, Korem T, Zeevi D, Costea PI, Godneva A, Kalka IN, Bar N, et al. Environment dominates over host genetics in shaping human gut microbiota. Nature. 2018;555(7695):210–215. doi:10.1038/nature25973.
- Song M, Giovannucci E. Preventable incidence and mortality of carcinoma associated with lifestyle factors among white adults in the United States. JAMA Oncol. 2016;2(9):1154–1161. doi:10.1001/jamaoncol.2016.0843.
- Song M, Chan AT. Environmental factors, gut microbiota, and colorectal cancer prevention. Clin Gastroenterol Hepatol. 2019;17(2):275–289. doi:10.1016/j.cgh.2018.07.012.
- Cani PD, Jordan BF. Gut microbiota-mediated inflammation in obesity: a link with gastrointestinal cancer. Nat Rev Gastroenterol Hepatol. 2018;15(11):671–682. doi:10.1038/s41575-018-0025-6.
- Kyriachenko Y, Falalyeyeva T, Korotkyi O, Molochek N, Kobyliak N. Crosstalk between gut microbiota and antidiabetic drug action. World J Diabetes. 2019;10(3):154–168. doi:10.4239/wjd.v10.i3.154.
- Song M, Chan AT, Sun J. Influence of the gut microbiome, diet, and environment on risk of colorectal cancer. Gastroenterology. 2020;158(2):322–340. doi:10.1053/j.gastro.2019.06.048.
- Ou J, Carbonero F, Zoetendal EG, DeLany JP, Wang M, Newton K, Gaskins HR, O’Keefe SJ. Diet, microbiota, and microbial metabolites in colon cancer risk in rural Africans and African Americans. Am J Clin Nutr. 2013;98(1):111–120. doi:10.3945/ajcn.112.056689.
- Schroeder BO, Birchenough GMH, Stahlman M, Arike L, Johansson MEV, Hansson GC, Bäckhed F. Bifidobacteria or fiber protects against diet-induced microbiota-mediated colonic mucus deterioration. Cell Host Microbe. 2018;23(1):27–40 e7. doi:10.1016/j.chom.2017.11.004.
- Tan J, Chen YX. Dietary and Lifestyle factors associated with colorectal cancer risk and interactions with microbiota: fiber, red or processed meat and alcoholic drinks. Gastrointest Tumors. 2016;3:17–24. doi:10.1159/000442831.
- Song M, Garrett WS, Chan AT. Nutrients, foods, and colorectal cancer prevention. Gastroenterology. 2015;148:1244–60 e16.
- Vieira AR, Abar L, Chan DSM, Vingeliene S, Polemiti E, Stevens C, Greenwood D, Norat T. Foods and beverages and colorectal cancer risk: a systematic review and meta-analysis of cohort studies, an update of the evidence of the WCRF-AICR Continuous Update Project. Ann Oncol. 2017;28:1788–1802. doi:10.1093/annonc/mdx171.
- Donohoe DR, Holley D, Collins LB, Montgomery SA, Whitmore AC, Hillhouse A, Curry KP, Renner SW, Greenwalt A, Ryan EP. A gnotobiotic mouse model demonstrates that dietary fiber protects against colorectal tumorigenesis in a microbiota- and butyrate-dependent manner. Cancer Discov. 2014;4:1387–1397. doi:10.1158/2159-8290.CD-14-0501.
- Marchand LL. Combined influence of genetic and dietary factors on colorectal cancer incidence in Japanese Americans. J Natl Cancer Inst Monogr. 1999;101–105. doi:10.1093/oxfordjournals.jncimonographs.a024220.
- Minchin RF, Reeves PT, Teitel CH, McManus ME, Mojarrabi B, Ilett KF, Kadlubar FF. N-and O-acetylation of aromatic and heterocyclic amine carcinogens by human monomorphic and polymorphic acetyltransferases expressed in COS-1 cells. Biochem Biophys Res Commun. 1992;185:839–844. doi:10.1016/0006-291X(92)91703-S.
- O’Keefe SJ, Chung D, Mahmoud N, Sepulveda AR, Manafe M, Arch J, Adada H, van der Merwe T. Why do African Americans get more colon cancer than Native Africans? J Nutr. 2007;137:175S–82S. doi:10.1093/jn/137.1.175S.
- O’Keefe SJ, Li JV, Lahti L, Ou J, Carbonero F, Mohammed K, Posma JM, Kinross J, Wahl E, Ruder E, et al. Fat, fibre and cancer risk in African Americans and rural Africans. Nat Commun. 2015;6(1):6342. doi:10.1038/ncomms7342.
- Mehta RS, Nishihara R, Cao Y, Song M, Mima K, Qian ZR, Nowak JA, Kosumi K, Hamada T, Masugi Y, et al. Association of dietary patterns with risk of colorectal cancer subtypes classified by fusobacterium nucleatum in tumor tissue. JAMA Oncol. 2017;3(7):921–927. doi:10.1001/jamaoncol.2016.6374.
- Mima K, Nishihara R, Qian ZR, Cao Y, Sukawa Y, Nowak JA, Yang J, Dou R, Masugi Y, Song M, et al. Fusobacterium nucleatum in colorectal carcinoma tissue and patient prognosis. Gut. 2016;65(12):1973–1980. doi:10.1136/gutjnl-2015-310101.
- Dingeo G, Brito A, Samouda H, Iddir M, La Frano MR, Bohn T. Phytochemicals as modifiers of gut microbial communities. Food Funct. 2020;11:8444–8471. doi:10.1039/D0FO01483D.
- Anhe FF, Roy D, Pilon G, Dudonne S, Matamoros S, Varin TV, Garofalo C, Moine Q, Desjardins Y, Levy E, et al. A polyphenol-rich cranberry extract protects from diet-induced obesity, insulin resistance and intestinal inflammation in association with increased Akkermansia spp. population in the gut microbiota of mice. Gut. 2015;64(6):872–883. doi:10.1136/gutjnl-2014-307142.
- Everard A, Belzer C, Geurts L, Ouwerkerk JP, Druart C, Bindels LB, Guiot Y, Derrien M, Muccioli GG, Delzenne NM, et al. Cross-talk between Akkermansia muciniphila and intestinal epithelium controls diet-induced obesity. Proc Natl Acad Sci U S A. 2013;110(22):9066–9071. doi:10.1073/pnas.1219451110.
- Bassani B, Rossi T, De Stefano D, Pizzichini D, Corradino P, Macrì N, Noonan DM, Albini A, Bruno A. Potential chemopreventive activities of a polyphenol rich purified extract from olive mill wastewater on colon cancer cells. J Funct Foods. 2016;27:236–248.
- Sun Y, Wu X, Cai X, Song M, Zheng J, Pan C, Qiu P, Zhang L, Zhou S, Tang Z, et al. Identification of pinostilbene as a major colonic metabolite of pterostilbene and its inhibitory effects on colon cancer cells. Mol Nutr Food Res. 2016;60(9):1924–1932. doi:10.1002/mnfr.201500989.
- Paul S, DeCastro AJ, Lee HJ, Smolarek AK, So JY, Simi B, Wang CX, Zhou R, Rimando AM, Suh N, et al. Dietary intake of pterostilbene, a constituent of blueberries, inhibits the beta-catenin/p65 downstream signaling pathway and colon carcinogenesis in rats. Carcinogenesis. 2010;31:1272–1278. doi:10.1093/carcin/bgq004.
- Gibson GR, Hutkins R, Sanders ME, Prescott SL, Reimer RA, Salminen SJ, Scott K, Stanton C, Swanson KS, Cani PD, et al. Expert consensus document: the International Scientific Association for Probiotics and Prebiotics (ISAPP) consensus statement on the definition and scope of prebiotics. Nat Rev Gastroenterol Hepatol. 2017;14(8):491–502. doi:10.1038/nrgastro.2017.75.
- Gibson GR, Roberfroid MB. Dietary modulation of the human colonic microbiota: introducing the concept of prebiotics. J Nutr. 1995;125:1401–1412. doi:10.1093/jn/125.6.1401.
- Fong W, Li Q, Yu J. Gut microbiota modulation: a novel strategy for prevention and treatment of colorectal cancer. Oncogene. 2020;39:4925–4943. doi:10.1038/s41388-020-1341-1.
- Markowiak-Kopec P, Slizewska K. The effect of probiotics on the production of short-chain fatty acids by human intestinal microbiome. Nutrients. 2020;12(4):1107.
- Ratajczak W, Ryl A, Mizerski A, Walczakiewicz K, Sipak O, Laszczynska M. Immunomodulatory potential of gut microbiome-derived short-chain fatty acids (SCFAs). Acta Biochim Pol. 2019;66:1–12.
- Keshteli AH, Madsen KL, Dieleman LA. Diet in the pathogenesis and management of ulcerative colitis; a review of randomized controlled dietary interventions. Nutrients. 2019;11(7):1498.
- Davani-Davari D, Negahdaripour M, Karimzadeh I, Seifan M, Mohkam M, Masoumi SJ, Berenjian A, Ghasemi Y. Prebiotics: definition, types, sources, mechanisms, and clinical applications. Foods. 2019;8(3):92.
- Lomax AR, Calder PC. Prebiotics, immune function, infection and inflammation: a review of the evidence. Br J Nutr. 2009;101:633–658. doi:10.1017/S0007114508055608.
- Ohtsuka K, Tsuji K, Nakagawa Y, Ueda H, Ozawa O, Uchida T, Ichikawa T. Utilization and metabolism of [U-14C]4ʹ galactosyllactose (O-beta-D-galactopyranosyl-(1—-4)-O-beta-D-galactopyranosyl-(1—-4)- D-glucopyranose) in rats. J Nutr Sci Vitaminol (Tokyo). 1991;37:173–184. doi:10.3177/jnsv.37.173.
- Figueroa-Lozano S, Ren C, Yin H, Pham H, van Leeuwen S, Dijkhuizen L, de Vos P. The impact of oligosaccharide content, glycosidic linkages and lactose content of galacto-oligosaccharides (GOS) on the expression of mucus-related genes in goblet cells. Food Funct. 2020;11:3506–3515. doi:10.1039/D0FO00064G.
- Meslin JC, Andrieux C, Sakata T, Beaumatin P, Bensaada M, Popot F, O Szylit, Durand M. Effects of galacto-oligosaccharide and bacterial status on mucin distribution in mucosa and on large intestine fermentation in rats. Br J Nutr. 1993;69:903–912. doi:10.1079/BJN19930090.
- Leforestier G, Blais A, Blachier F, Marsset-Baglieri A, Davila-Gay AM, Perrin E, Tomé D. Effects of galacto-oligosaccharide ingestion on the mucosa-associated mucins and sucrase activity in the small intestine of mice. Eur J Nutr. 2009;48:457–464. doi:10.1007/s00394-009-0036-8.
- Fernandez J, Moreno FJ, Olano A, Clemente A, Villar CJ, Lombo F. A galacto-oligosaccharides preparation derived from lactulose protects against colorectal cancer development in an animal model. Front Microbiol. 2018;9:2004. doi:10.3389/fmicb.2018.02004.
- Kaliannan K, Hamarneh SR, Economopoulos KP, Nasrin Alam S, Moaven O, Patel P, Malo NS, Ray M, Abtahi SM, Muhammad N, et al. Intestinal alkaline phosphatase prevents metabolic syndrome in mice. Proc Natl Acad Sci U S A. 2013;110(17):7003–7008. doi:10.1073/pnas.1220180110.
- Okazaki Y, Katayama T. Consumption of non-digestible oligosaccharides elevates colonic alkaline phosphatase activity by up-regulating the expression of IAP-I, with increased mucins and microbial fermentation in rats fed a high-fat diet. Br J Nutr. 2019;121:146–154. doi:10.1017/S0007114518003082.
- Tuohy KM, Kolida S, Lustenberger AM, Gibson GR. The prebiotic effects of biscuits containing partially hydrolysed guar gum and fructo-oligosaccharides–a human volunteer study. Br J Nutr. 2001;86:341–348. doi:10.1079/BJN2001394.
- Hsu CK, Liao JW, Chung YC, Hsieh CP, Chan YC. Xylooligosaccharides and fructooligosaccharides affect the intestinal microbiota and precancerous colonic lesion development in rats. J Nutr. 2004;134:1523–1528. doi:10.1093/jn/134.6.1523.
- Pierre F, Perrin P, Champ M, Bornet F, Meflah K, Menanteau J. Short-chain fructo-oligosaccharides reduce the occurrence of colon tumors and develop gut-associated lymphoid tissue in min mice. Cancer Res. 1997;57:225–228.
- Lima GC, Vieira VCC, Cazarin CBB, Ribeiro RDR, Junior SB, De Albuquerque CL, Vidal RO, Netto CC, Yamada ÁT, Augusto F, et al. Fructooligosaccharide intake promotes epigenetic changes in the intestinal mucosa in growing and ageing rats. Eur J Nutr. 2018;57(4):1499–1510. doi:10.1007/s00394-017-1435-x.
- Bouhnik Y, Flourie B, Riottot M, Bisetti N, Gailing MF, Guibert A, Bornet F, Rambaud JC. Effects of fructo-oligosaccharides ingestion on fecal bifidobacteria and selected metabolic indexes of colon carcinogenesis in healthy humans. Nutr Cancer. 1996;26:21–29. doi:10.1080/01635589609514459.
- Boutron-Ruault MC, Marteau P, Lavergne-Slove A, Myara A, Gerhardt MF, Franchisseur C, Bornet F. Effects of a 3-mo consumption of short-chain fructo-oligosaccharides on parameters of colorectal carcinogenesis in patients with or without small or large colorectal adenomas. Nutr Cancer. 2005;53:160–168. doi:10.1207/s15327914nc5302_5.
- Hill C, Guarner F, Reid G, Gibson GR, Merenstein DJ, Pot B, Morelli L, Canani RB, Flint HJ, Salminen S, et al. Expert consensus document. The international scientific association for probiotics and prebiotics consensus statement on the scope and appropriate use of the term probiotic. Nat Rev Gastroenterol Hepatol. 2014;11(8):506–514. doi:10.1038/nrgastro.2014.66.
- Caballero-Franco C, Keller K, De Simone C, Chadee K. The VSL#3 probiotic formula induces mucin gene expression and secretion in colonic epithelial cells. Am J Physiol Gastrointest Liver Physiol. 2007;292(1):G315–22. doi:10.1152/ajpgi.00265.2006.
- Martin R, Chamignon C, Mhedbi-Hajri N, Chain F, Derrien M, Escribano-Vazquez U, Garault P, Cotillard A, Pham HP, Chervaux C, et al. The potential probiotic Lactobacillus rhamnosus CNCM I-3690 strain protects the intestinal barrier by stimulating both mucus production and cytoprotective response. Sci Rep. 2019;9(1):5398. doi:10.1038/s41598-019-41738-5.
- Yang HS, Fu DZ, Kong XF, Wang WC, Yang XJ, Nyachoti CM, Yin YL. Dietary supplementation with N-carbamylglutamate increases the expression of intestinal amino acid transporters in weaned Huanjiang mini-pig piglets. J Anim Sci. 2013;91(6):2740–2748. doi:10.2527/jas.2012-5795.
- Wang G, Huang S, Wang Y, Cai S, Yu H, Liu H, Zeng X, Zhang G, Qiao S. Bridging intestinal immunity and gut microbiota by metabolites. Cell Mol Life Sci. 2019;76(20):3917–3937. doi:10.1007/s00018-019-03190-6.
- Zolkiewicz J, Marzec A, Ruszczynski M, Feleszko W. Postbiotics-a step beyond pre- and probiotics. Nutrients. 2020;12(8):2189.
- Gaudier E, Jarry A, Blottiere HM, De Coppet P, Buisine MP, Aubert JP, Laboisse C, Cherbut C, Hoebler C. Butyrate specifically modulates MUC gene expression in intestinal epithelial goblet cells deprived of glucose. Am J Physiol Gastrointest Liver Physiol. 2004;287(6):G1168–74. doi:10.1152/ajpgi.00219.2004.
- Burger-van Paassen N, Vincent A, Puiman PJ, van der Sluis M, Bouma J, Boehm G, van Goudoever J, Van seuningen I, Renes I. The regulation of intestinal mucin MUC2 expression by short-chain fatty acids: implications for epithelial protection. Biochem J. 2009;420(2):211–219. doi:10.1042/BJ20082222.
- Jung TH, Park JH, Jeon WM, Han KS. Butyrate modulates bacterial adherence on LS174T human colorectal cells by stimulating mucin secretion and MAPK signaling pathway. Nutr Res Pract. 2015;9:343–349. doi:10.4162/nrp.2015.9.4.343.
- Jiminez JA, Uwiera TC, Abbott DW, Uwiera RRE, Inglis GD. Butyrate supplementation at high concentrations alters enteric bacterial communities and reduces intestinal inflammation in mice infected with citrobacter rodentium. mSphere. 2017;2(4):e00243-17.
- Willemsen LE, Koetsier MA, Van Deventer SJ, van Tol EA. Short chain fatty acids stimulate epithelial mucin 2 expression through differential effects on prostaglandin E(1) and E(2) production by intestinal myofibroblasts. Gut. 2003;52:1442–1447. doi:10.1136/gut.52.10.1442.
- Bjarnason I, Hayllar J, MacPherson AJ, Russell AS. Side effects of nonsteroidal anti-inflammatory drugs on the small and large intestine in humans. Gastroenterology. 1993;104:1832–1847. doi:10.1016/0016-5085(93)90667-2.
- Yamada T, Hino S, Iijima H, Genda T, Aoki R, Nagata R, Han K-H, Hirota M, Kinashi Y, Oguchi H, et al. Mucin O-glycans facilitate symbiosynthesis to maintain gut immune homeostasis. EBioMedicine. 2019;48:513–525. doi:10.1016/j.ebiom.2019.09.008.
- Taleb S. Tryptophan dietary impacts gut barrier and metabolic diseases. Front Immunol. 2019;10:2113. doi:10.3389/fimmu.2019.02113.
- Gheorghe CE, Martin JA, Manriquez FV, Dinan TG, Cryan JF, Clarke G. Focus on the essentials: tryptophan metabolism and the microbiome-gut-brain axis. Curr Opin Pharmacol. 2019;48:137–145. doi:10.1016/j.coph.2019.08.004.
- Scott SA, Fu J, Chang PV. Microbial tryptophan metabolites regulate gut barrier function via the aryl hydrocarbon receptor. Proc Natl Acad Sci U S A. 2020;117(32):19376–19387. doi:10.1073/pnas.2000047117.
- Lanis JM, Alexeev EE, Curtis VF, Kitzenberg DA, Kao DJ, Battista KD, Gerich ME, Glover LE, Kominsky DJ, Colgan SP, et al. Tryptophan metabolite activation of the aryl hydrocarbon receptor regulates IL-10 receptor expression on intestinal epithelia. Mucosal Immunol. 2017;10(5):1133–1144. doi:10.1038/mi.2016.133.
- Venkatesh M, Mukherjee S, Wang H, Li H, Sun K, Benechet AP, Qiu Z, Maher L, Redinbo M, Phillips R, et al. Symbiotic bacterial metabolites regulate gastrointestinal barrier function via the xenobiotic sensor PXR and toll-like receptor 4. Immunity. 2014;41(2):296–310. doi:10.1016/j.immuni.2014.06.014.
- Li J, Zhang L, Wu T, Li Y, Zhou X, Ruan Z. Indole-3-propionic acid improved the intestinal barrier by enhancing epithelial barrier and mucus barrier. J Agric Food Chem. 2021;69(5):1487–1495. doi:10.1021/acs.jafc.0c05205.
- Zhao ZH, Xin FZ, Xue Y, Hu Z, Han Y, Ma F, Zhou D, Liu XL, Cui A, Liu Z, et al. Indole-3-propionic acid inhibits gut dysbiosis and endotoxin leakage to attenuate steatohepatitis in rats. Exp Mol Med. 2019;51:1–14.
- Wlodarska M, Luo C, Kolde R, d’Hennezel E, Annand JW, Heim CE, Krastel P, Schmitt EK, Omar AS, Creasey EA, et al. Indoleacrylic acid produced by commensal peptostreptococcus species suppresses inflammation. Cell Host Microbe. 2017;22(1):25–37 e6. doi:10.1016/j.chom.2017.06.007.
- Shi J, Du P, Xie Q, Wang N, Li H, Smith EE, Li C, Liu F, Huo G, Li B, et al. Protective effects of tryptophan-catabolizing Lactobacillus plantarum KLDS 1.0386 against dextran sodium sulfate-induced colitis in mice. Food Funct. 2020;11(12):10736–10747. doi:10.1039/D0FO02622K.
- Zihni C, Mills C, Matter K, Balda MS. Tight junctions: from simple barriers to multifunctional molecular gates. Nat Rev Mol Cell Biol. 2016;17:564–580. doi:10.1038/nrm.2016.80.
- Yin J, Yang K, Zhou C, Xu P, Xiao W, Yang H. Aryl hydrocarbon receptor activation alleviates dextran sodium sulfate-induced colitis through enhancing the differentiation of goblet cells. Biochem Biophys Res Commun. 2019;514:180–186. doi:10.1016/j.bbrc.2019.04.136.
- Sayin SI, Wahlstrom A, Felin J, Jantti S, Marschall HU, Bamberg K, Angelin B, Hyötyläinen T, Orešič M, Bäckhed F, et al. Gut microbiota regulates bile acid metabolism by reducing the levels of tauro-beta-muricholic acid, a naturally occurring FXR antagonist. Cell Metab. 2013;17:225–235. doi:10.1016/j.cmet.2013.01.003.
- Parseus A, Sommer N, Sommer F, Caesar R, Molinaro A, Stahlman M, Greiner TU, Perkins R, Bäckhed F. Microbiota-induced obesity requires farnesoid X receptor. Gut. 2017;66(3):429–437. doi:10.1136/gutjnl-2015-310283.
- Floch MH, Binder HJ, Filburn B, Gershengoren W. The effect of bile acids on intestinal microflora. Am J Clin Nutr. 1972;25(12):1418–1426. doi:10.1093/ajcn/25.12.1418.
- Pyo JS, Ko YS, Kang G, Kim DH, Kim WH, Lee BL, ohn JH. Bile acid induces MUC2 expression and inhibits tumor invasion in gastric carcinomas. J Cancer Res Clin Oncol. 2015;141:1181–1188. doi:10.1007/s00432-014-1890-1.
- Lee HY, Crawley S, Hokari R, Kwon S, Kim YS. Bile acid regulates MUC2 transcription in colon cancer cells via positive EGFR/PKC/Ras/ERK/CREB, PI3K/Akt/IkappaB/NF-kappaB and p38/MSK1/CREB pathways and negative JNK/c-Jun/AP-1 pathway. Int J Oncol. 2010;36:941–953.
- Cao H, Xu M, Dong W, Deng B, Wang S, Zhang Y, Wang S, Luo S, Wang W, Qi Y, et al. Secondary bile acid-induced dysbiosis promotes intestinal carcinogenesis. Int J Cancer. 2017;140(11):2545–2556. doi:10.1002/ijc.30643.
- Rubinstein MR, Wang X, Liu W, Hao Y, Cai G, Han YW. Fusobacterium nucleatum promotes colorectal carcinogenesis by modulating E-cadherin/beta-catenin signaling via its FadA adhesin. Cell Host Microbe. 2013;14:195–206. doi:10.1016/j.chom.2013.07.012.
- Wang S, Dong W, Liu L, Xu M, Wang Y, Liu T, Zhang Y, Wang B, Cao H. Interplay between bile acids and the gut microbiota promotes intestinal carcinogenesis. Mol Carcinog. 2019;58(7):1155–1167. doi:10.1002/mc.22999.
- Bakken JS, Borody T, Brandt LJ, Brill JV, Demarco DC, Franzos MA, Kelly C, Khoruts A, Louie T, Martinelli LP, et al. Treating clostridium difficile infection with fecal microbiota transplantation. Clin Gastroenterol Hepatol. 2011;9(12):1044–1049. doi:10.1016/j.cgh.2011.08.014.
- Brandt LJ, Aroniadis OC. An overview of fecal microbiota transplantation: techniques, indications, and outcomes. Gastrointest Endosc. 2013;78(2):240–249. doi:10.1016/j.gie.2013.03.1329.
- Choi HH, Cho YS. Fecal microbiota transplantation: current applications, effectiveness, and future perspectives. Clin Endosc. 2016;49:257–265. doi:10.5946/ce.2015.117.
- Kelly CR, Khoruts A, Staley C, Sadowsky MJ, Abd M, Alani M, Bakow B, Curran P, McKenney J, Tisch A, et al. Effect of fecal microbiota transplantation on recurrence in multiply recurrent clostridium difficile infection: a randomized trial. Ann Intern Med. 2016;165:609–616. doi:10.7326/M16-0271.
- Konturek PC, Koziel J, Dieterich W, Haziri D, Wirtz S, Glowczyk I, Konturek K, Neurath MF, Zopf Y. Successful therapy of clostridium difficile infection with fecal microbiota transplantation. J Physiol Pharmacol. 2016;67:859–866.
- Kim KO, Schwartz MA, Lin OST, Chiorean MV, Gluck M. Reducing cost and complexity of fecal microbiota transplantation using universal donors for recurrent clostridium difficile Infection. Adv Ther. 2019;36(8):2052–2061. doi:10.1007/s12325-019-00974-x.
- Wong SH, Zhao L, Zhang X, Nakatsu G, Han J, Xu W, Xiao X, Kwong TNY, Tsoi H, Wu WKK, et al. Gavage of fecal samples from patients with colorectal cancer promotes intestinal carcinogenesis in germ-free and conventional mice. Gastroenterology. 2017;153(6):1621–33 e6. doi:10.1053/j.gastro.2017.08.022.
- Rosshart SP, Vassallo BG, Angeletti D, Hutchinson DS, Morgan AP, Takeda K, Hickman HD, McCulloch JA, Badger JH, Ajami NJ, et al. Wild mouse gut microbiota promotes host fitness and improves disease resistance. Cell. 2017;171(5):1015–28 e13. doi:10.1016/j.cell.2017.09.016.
- Cheng S, Ma X, Geng S, Jiang X, Li Y, Hu L, Li J, Wang Y, Han X. Fecal microbiota transplantation beneficially regulates intestinal mucosal autophagy and alleviates gut barrier injury. mSystems. 2018;3(5):e00137-18.
- Li L, Li X, Zhong W, Yang M, Xu M, Sun Y, Ma J, Liu T, Song X, Dong W, et al. Corrigendum to ‘Gut microbiota from colorectal cancer patients enhances the progression of intestinal adenoma in Apc(min)(/+) mice’ [EBioMedicine 48 (2019) 301-315]. EBioMedicine. 2020;53:102680. doi:10.1016/j.ebiom.2020.102680.