ABSTRACT
Enterohemorrhagic Escherichia coli (EHEC) O157:H7 is a principally foodborne pathogen linked to serious diseases, including bloody diarrhea, hemorrhagic colitis, and hemolytic uremic syndrome. Comparative genomics analysis revealed that EHEC O157 contains 177 unique genomic islands, termed O islands, compared with the nonpathogenic E. coli K-12 laboratory strain. These O islands contribute largely to the pathogenicity of EHEC O157:H7 by providing numerous virulence factors, effectors, virulence regulatory proteins, and virulence regulatory sRNAs. The present review aimed to provide a comprehensive understanding of the research progress on the function of O islands, especially focusing on virulence-related O islands.
Introduction
Enterohemorrhagic Escherichia coli (EHEC) O157:H7 is an important human pathogen that specifically colonizes the large intestine, causing disease.Citation1, Citation2 Shiga toxins (Stxs), which are the major virulence factors of EHEC O157:H7, are known to cause damage to a variety of cell types and have often been associated with hemorrhagic colitis (HC) and the lethal hemolytic uremic syndrome (HUS) in humans.Citation3,Citation4 The pathogenesis of EHEC O157:H7 infections is characterized by the formation of an attaching and effacing (A/E) lesion that involves the intimate attachment of bacteria to the host enterocyte membrane, the subversion of actin and cytoskeletal components, and the formation of a pedestal structure beneath the adherent bacteria.Citation5,Citation6 The ability of EHEC O157:H7 to form A/E lesions is conferred by a large pathogenicity island, termed locus of enterocyte effacement (LEE), which consists of five polycistronic operons (LEE1 to LEE5).Citation7 In particular, LEE encodes a type III secretion system (T3SS) that exports effector molecules, including the intimin adhesin, the translocated intimin receptor (Tir), and several secreted proteins (Esp), which are important in the modification of the host cell signal transduction during the formation of A/E lesions.Citation1,Citation8
Comparative genomic analyses revealed that the EHEC O157:H7 strain EDL933 contains 177 genomic islands, termed O islands (OIs), which are absent from the genome of the nonpathogenic E. coli K-12 MG1655. These OIs encompass 1387 genes (26% of the total), with most of them encoding hypothetical albeit as-yet-uncharacterized proteins.Citation9,Citation10 Studies on the function of OIs have made substantial progress during the last 2 decades, and a growing number of OI-associated genes have been assigned a function. Among these 1387 OI genes, 69 genes (4.97%) are associated with EHEC O157:H7 virulence, including 31 genes (2.24%) encoding virulence factors, 26 genes (1.87%) encoding effectors, and 12 genes (0.87%) encoding virulence regulatory proteins; 47 genes (3.39%) are associated with other biological processes; the function of remaining 1271 genes (91.64%) has been predicted or is unknown (). In the present review, we aimed to provide a broad overview of the function of OIs, especially focusing on OIs associated with the virulence and pathogenicity of EHEC O157:H7. For convenience, we grouped these OIs into four main categories according to the function of genes contained in these OIs: (1) OIs encoding virulence factors, (2) OIs encoding effectors, (3) OIs encoding virulence regulatory proteins, and (4) OIs encoding virulence regulatory sRNAs.
OIs encoding virulence factors
Virulence factors, encoded by pathogenicity islands, plasmids, and prophages, are necessary for colonization and replication within the host, neutralization of host defenses, and spread into new hosts.Citation11 The main virulence determinants of EHEC O157:H7 include adhesins, T3SS that inject effector proteins into host cells, Stxs, and iron acquisition systems.Citation12 To date, eight OIs, namely OI-15, OI-43, OI-45, OI-48, OI-57, OI-93, OI-122, and OI-148, have been reported to encode virulence factors ( and ).
Table 1. Virulence-related O islands in EHEC O157:H7
Figure 2. Graphical representation of the gene content and organization of virulence-related OIs. Arabic numerals represent the starting and ending positions of OIs in the EHEC O157:H7 strain EDL933 genome (GenBank accession no. AE005174). Roman numerals represent the four categories of virulence-related OIs: I = OIs encoding virulence factors, II = OIs encoding effectors, III = OIs encoding virulence regulatory proteins, and IV = OIs encoding virulence regulatory sRNAs. Arrows represent genes, which are color-coded to indicate functions: blue = genes encoding virulence factors, red = genes encoding effectors, green = genes encoding virulence regulatory proteins, yellow = genes encoding virulence regulatory sRNAs, and gray = genes not related to EHEC O157:H7 virulence or are with unknown functions
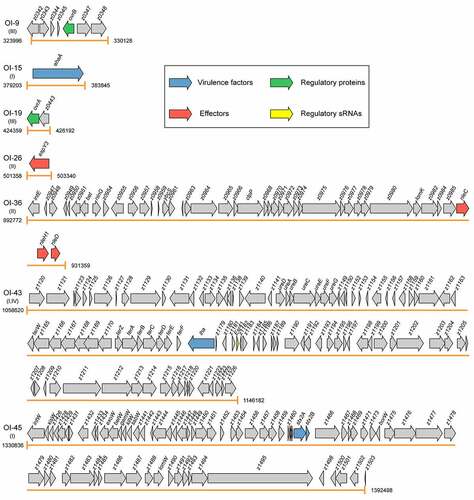
OI-15
OI-15 is a 4643 bp genomic region (ranging from 379203 bp to 383845 bp in the EHEC O157:H7 EDL933 genome). The phylogenetic analyses of complete genome sequences of 143 available EHEC O157:H7 strains revealed that these EHEC O157:H7 strains have been classified into 9 distinct clades (from clade 1 to clade 9, ). BLASTN searches showed that OI-15 is highly conserved and widely distributed in all 143 EHEC O157:H7 strains of 9 clades ( and Table S1). OI-15 contains only a single open reading frame (ORF), ehaA (z0402).Citation9 The ehaA gene encodes the AID15 adhesin, which contains a pertactin domain encompassing part of the repetitive beta-helical domain, followed by a C-terminal autotransporter domain encompassing the beta-barrel domain. Deletion of ehaA was reported to cause a significant reduction in the adherence of EHEC O157:H7 in pig ileal loops but not to HEp-2 and IPEC-J2 cells.Citation13 Timothy and his colleagues demonstrated that EhaA is located at the cell surface, and its overexpression in E. coli K-12 cells resulted in the formation of large cell aggregates, promoted significant biofilm formation, and mediated adhesion to primary epithelial cells of the bovine terminal rectum.Citation14
Figure 3. The prevalence of virulence-related OIs in EHEC O157:H7 strains. Maximum likelihood tree was constructed using PhyML based on 3440 single-copy core genes shared between E. coli K-12 MG1655 and 143 EHEC O157:H7 strains. Based on the phylogenetic analysis, these EHEC O157:H7 strains are classified into nine distinct clades (clade 1 to 9). The prevalence of different virulence-related OIs in EHEC O157:H7 strains was determined using BLASTN searches and are shown using heatmap. The search criteria are coverage ≥85% and identity ≥90%. Red and blue cells represent the presence and absence of OIs in a particular EHEC O157:H7 strain, respectively
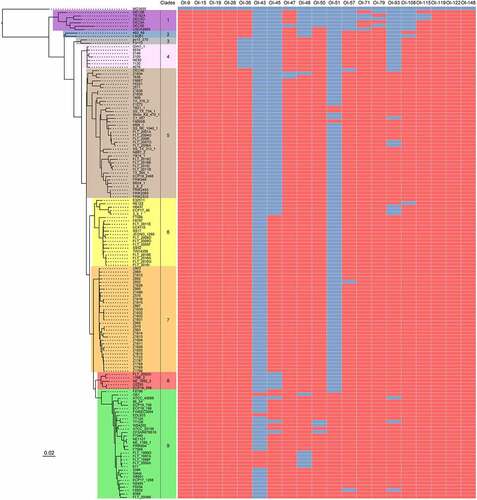
OI-43/48
Contain a Pfam: Pertactin domain encompassing part of the repetitive beta-helical (passenger) domain, followed by a C-terminal Pfam: Autotransporter domain encompassing the beta-barrel (translocation) domain. contain a Pfam: Pertactin domain encompassing part of the repetitive beta-helical (passenger) domain, followed by a C-terminal Pfam: Autotransporter domain encompassing the beta-barrel (translocation) domain.
OI-43 is an 87563 bp island (ranging from 1058620 bp to 1146182 bp in the EHEC O157:H7 EDL933 genome) containing 97 ORFs (from z1129 to z1226). OI-48 comprises an 87548 bp island (ranging from 1454242 bp to 1541789 bp according to the EHEC O157:H7 EDL933 genome) including 106 ORFs (from z1559 to z1664). The EHEC O157:H7 strain EDL933 contains both the OI-43 and OI-48 islands, which are duplicates, whereas the other EHEC O157:H7 strains contain either OI-43 or OI-48 ( and Table S1). In addition to the strain EDL933, six EHEC O157:H7 strains of clade 9 contain OI-43, including OK1, 611, FLT_1999 G, FLT_1997A, FLT_1998 F, and FLT_2000A ( and Table S1). OI-48 is widely distributed in EHEC O157:H7 strains from clade 3 to clade 9, except for one strain in clade 5 (strain Z1834) and six strains that contain OI-43 in clade 9 ( and Table S1). The genes in OI-43/48 are classified into 3 groups: a 7-gene cluster ureDABCEFG, which encodes urease and accessory proteins hydrolyzing urea to ammonia and carbon dioxide; telluride resistance genes terZABCDEF; and 2 putative adhesin genes, iha (encoding an IrgA homolog adhesin), and aidA-1 (autotransporter adhesin involved in diffuse adherence).Citation10 The telluride resistance proteins, Iha adhesin, and urease were shown to contribute to EHEC O157:H7 pathogenesis by promoting the adherence of the pathogen to the intestinal epithelium of the host. Deletion of the telluride resistance gene cluster reduced the ability of EHEC O157:H7 to adhere to and form large clusters on IPEC-J2 and HEp-2 cells,Citation15 whereas, deletion of the iha and ureC genes had no effect on bacterial adherence in vitro but was found to significantly reduce the colonization of EHEC O157:H7 in ligated pig intestines. In contrast, deletion of the aidA gene had no effect on bacterial adherence neither in vitro nor in vivo.Citation15
OI-45/93
OI-45 and OI-93 are Stx-converting bacteriophages. Of note, Stxs are bacteriophage-encoded cytotoxins that damage a variety of cell types.Citation87 Moreover, Stxs are divided into two groups, i.e., Stx1 and Stx2, with a 56% homology in their amino acid sequences. OI-45 is a 61663 bp island (ranging from 1330836 bp to 1392498 bp according to the EHEC O157:H7 EDL933 genome) that contains 69 ORFs (from z1424 to z1504). OI-45 is present in all the EHEC O157:H7 strains of clade 7 and in the majority of strains in clade 9 (except for strains ATCC 43888, TT12B, and CFSAN076619) and clade 6 (except for strains E32511, NE122, H6437, ECP17-46, and 3-5-1) ( and Table S1). In particular, z1464 and z1465 encode the A (Stx2A) and B (Stx2B) subunit of Stx 2, respectively. OI-93 is a 48916 bp island (ranging from 2966157 bp to 3015072 bp according to the EHEC O157:H7 EDL933 genome) that contains 65 ORFs (from z3305 to z3375). OI-93 is widely distributed in EHEC O157:H7 strains from clade 5 to clade 9, except for four strains of clade 5 (strains DEC4E, C1_057, FLT_2007 G, and FLT_2006A), three strains of clade 6 (strains H6437, ECP17-46, and 3-5-1), and three strains of clade 9 (strains ATCC 43888, TT12B, and F8952) ( and Table S1). Similarly, z3344 and z3343 encode the A (Stx1A) and B (Stx1B) subunit of Stx 1, respectively. It is known that Stxs play essential roles in the initial step of the colonization of the intestinal mucosa. After crossing the intestinal barrier, the B subunit of Stxs interacts with the globotriaosylceramide (Gb3) or globotetraosylceramide (Gb4) host receptors, allowing the internalization of the A subunit to the cytoplasm.Citation16 The A subunit of Stxs inhibits the synthesis of host proteins through the specific removal of a single adenine residue from the 28S rRNA of the 60S ribosomal subunit.Citation17 Stxs have also been implicated in the interaction between EHEC O157:H7 and human macrophages. Global gene expression profiling revealed that Stx genes were significantly upregulated in EHEC O157:H7 infected macrophages.Citation18 Subsequent survival and cytotoxicity assays found that the initial uptake of Stx mutants was higher than that of wild-type; however, survival rates were significantly lower at 24 h postinfection.Citation18
OI-57
OI-57 is an 80502 bp island (ranging from 1849324 to 1929825 in the EHEC O157:H7 EDL933 genome) that contains 97 ORFs (from z2048 to z2151). OI-57 is widely distributed in EHEC O157:H7 strains from clade 2 to clade 9, except for one strain of clade 5 (strain DEC4E), one strain of clade 7 (strain Z852), and one strain of clade 9 (strain F8952) ( and Table S1). The adfO gene (z2053) encodes a virulence factor, which exhibits similarity to Paa, a virulence-associated protein of the porcine enteropathogenic E. coli (EPEC) that was implicated in the colonization of the pig ileum.Citation19 An EHEC O157:H7 adfO mutant was reported to exhibit marked reductions in its ability to adhere to HeLa cells as well as to produce and secrete several proteins (including z1931 and z3065) into the supernatant.Citation20 The adfO virulence gene has been found to always be present together with ckf (z2054), which encodes a putative phage-associated bacterial cell-killing factor.Citation88 When produced in excess, paralogues of Ckf have been reported to disrupt bacterial host membranes.Citation9
OI-122
OI-122 is a 23455 bp island (ranging from 3919348 bp to 3942802 bp in the EHEC O157:H7 EDL933 genome), that contains 26 ORFs (from z4313 to z4340). OI-122 is widely distributed in all the 143 EHEC O157:H7 strains of 9 clades ( and Table S1). In particular, pagC (z4321) encodes a virulence factor, which has significant homology to the Salmonella enterica serovar typhimurium PagC, that is important for bacterial survival and upregulated in macrophages.Citation21,Citation22 A pagC mutant in Citrobacter rodentium, which causes A/E lesions in mice, had a significantly lower competitive index, indicating its importance for the establishment of in vivo infection.Citation23 Of note, Efa1 (encoded by efa1) was first identified as a factor influencing the adhesion of a clinical EHEC O111:H- strain to cultured epithelial cells.Citation89 However, EHEC O157:H7 lacks the full-length efa1 gene, but carries a truncated version of efa1 (efa1ʹ) in the chromosome (z4332 and z4333). Accordingly, a EHEC O157:H7 efa1ʹ mutant was shown to exhibit reduced adherence to cultured HeLa cells and reduced expression and secretion of LEE-encoded proteins.Citation24 However, the efa1ʹ mutation did not significantly affect the course of fecal shedding of EHEC O157:H7 following experimental inoculation of 10- to 14-d-old calves or 6-week-old sheep.Citation24
OI-148
OI-148 is a 43418 bp island (ranging from 4649862 bp to 4693279 bp in the EHEC O157:H7 EDL933 genome) that contains 54 ORFs (from z5087 to z5143). OI-148 is highly conserved and widely distributed in all the 143 EHEC O157:H7 strains of 9 clades ( and Table S1). OI-148 harbors the LEE pathogenicity island, which is required for the intimate adherence of EHEC O157:H7 to host epithelial cells and the formation of A/E lesions. More specifically, LEE contains 41 genes (from z5087 to z5143) grouped into 5 polycistronic operons (LEE1–LEE5).Citation7 Among them, LEE1, LEE2, and LEE3 harbor the esc and sep genes, which encode for the components of the T3SS that allow the direct injection of bacterial effector proteins into host cells resulting in the subversion of host cell signaling pathways and formation of A/E lesions.Citation25 The LEE5 operon contains E. coli attaching and effacing (eae) and tir genes, encoding for the intimin adhesion protein and its Tir receptor.Citation29 The LEE4 contains the genes encoding translocator proteins, which form the tip of the T3SS (EspB and EspD), the EspA needle filament, the EscF needle protein, the CesD2 chaperone, the SepL gatekeeper protein, and the EspF effector protein.Citation26 Deletion of LEE has been demonstrated to completely abolish the ability of EHEC O157:H7 to adhere to host cells and form A/E lesions.Citation27 Intriguingly, the EPEC LEE expressed from a multicopy plasmid transformed into an E. coli K-12 laboratory strain was necessary and sufficient to give rise to the A/E phenotype on human epithelial cells in culture.Citation90 In contrast, the EHEC O157:H7 LEE alone was not sufficient to confer the A/E phenotype when expressed in an E. coli laboratory strain,Citation28 suggesting that certain factors or regulatory proteins outside of EHEC LEE are necessary for the A/E phenotype.
OIs encoding effectors
The effectors of EHEC O157:H7 are encoded on either the LEE, prophages, or insertion elements.Citation91 These effectors are known to be key modulators of the innate immune system of intestinal epithelial cells, and have been found to exert their function mainly through disturbing the nuclear factor kappa Β (NF-κB)-regulated signal transduction pathways.Citation92,Citation93 Once translocated, these effectors are targeted to various intracellular compartments and modulate diverse signaling pathways and physiological processes, including ion secretion, apoptosis, membrane insertion, and cytoskeleton changes.Citation94 To date, eight OIs, namely OI-26, OI-36, OI-50, OI-57, OI-71, OI-79, OI-122, and OI-148 have been reported to harbor genes encoding effector proteins ( and ).
OI-26
OI-26 is a 1983 bp region (ranging from 501358 bp to 503340 bp in the EHEC O157:H7 EDL933 genome), which is highly conserved and widely distributed in all the 143 EHEC O157:H7 strains of 9 clades ( and Table S1). OI-26 contains only a single ORF, i.e., espY3 (z0521). The EspY3 protein contains an N-terminal WEX5F domain with homology to SopD (Salmonella outer protein D), a well-characterized T3SS-1 (SPI-1) effector protein of Salmonella.Citation95 EspY3 has been shown to localize in the pedestal region.Citation30 The EspY3 effector was reported to induce the elongation of polymerized actin pedestals in infected host epithelial cells, thus inducing a significant increase in the size of pedestal areas.Citation30
OI-36
OI-36 is a 38588 bp island (ranging from 892772 bp to 931359 bp in the EHEC O157:H7 EDL933 genome) that contains 42 ORFs (from z0946 to z0990). Bioinformatics analysis showed that OI-36 existed in EHEC O157:H7 strains of seven clades (clades 1, 2, and 5 to 9) but not in the strains of the other two clades (clades 3 and 4) ( and Table S1). The nleC (z0986), nleH1 (z0989), and nleD (z0990) genes within OI-36 encode three translocated effectors.Citation96 Particularly, NleC, which acts as a zinc protease, was found to compromise the activation of NF-κB by directly cleaving the NF-κB subunit p65,Citation31 resulting in a decrease in the total nuclear entry of active p65. Consequently, NleC-mediated proteolysis suppressed the activation of NF-κB and in turn impaired the secretion of interleukin-1β (IL-1β), IL-8, and tumor necrosis factor-α (TNF-α).Citation31,Citation32 NleH1 has significant sequence similarity to Shigella flexneri OspG, a protein known to interfere with the activation of NF-kBCitation97 and has been shown to bind directly to a subunit of NF-kB, the ribosomal protein S3 (RPS3).Citation33 One of the functions of RPS3 is to guide the recruitment of the p65 NF-κB subunit to specific promoters in response to different stimuli. NleH1 functions by reducing the nuclear abundance of RPS3 to dampen host transcriptional outputs.Citation33 Furthermore, deleting nleH1 from EHEC O157:H7 produced a hypervirulent Stx-producing E. coli phenotype in a gnotobiotic piglet model.Citation33 Finally, NleD is a metalloprotease, which directly cleaves p38, a crucial part of the MAPK signaling pathway, and JNK within the activation loop in pathogen-infected epithelial cells, thus suppressing the host immune response.Citation34 In addition, NleD was also shown to specifically cleave and inactivate the p65 subunit of NF-κB.Citation35
OI-50
OI-50 is a 47315 bp island (ranging from 1626570 bp to 1673884 bp in the EHEC O157:H7 EDL933 genome) that contains 162 ORFs (from z1664 to z1829). OI-50 is widely distributed in EHEC O157:H7 strains from clade 2 to 9, except for three strains of clade 9 (strains TT12B, WS4202, and CFSAN076619) ( and Table S1). The espK (z1829), espN (z1824), and nleL (z1822) genes within OI-50 encode translocated effectors. Interestingly, EspK is homologous to the Gifsy phage-encoded Salmonella enterica serovar typhimurium type III secreted effector GogB.Citation98 When transiently expressed in COS-7, a cell line of immortalized kidney fibroblasts from the African green monkey, EspK localizes to the cytoplasm.Citation36 Inactivation of espK did not impair the adherence or actin nucleation during the infection of HeLa cells but significantly reduced the persistence of EHEC O157:H7 in the intestine of orally inoculated calves.Citation36 NleL (previously known as EspX7) is an HECT-type E3 ligase, which modulates the formation of the pedestal used for the adherence of EHEC O157:H7 to host epithelial cells.Citation37,Citation38 The c-Jun NH2-terminal kinases (JNKs) are known to constitute host substrates of NleL, and their NleL-mediated monoubiquitination has been shown to prevent their interaction with the upstream kinase MKK7, thus disrupting the phosphorylation and activation of the JNK signaling pathway.Citation38 A recent work revealed that NleL disrupted the host NF-κB signaling by targeting several components of the NF-κB pathway, including TRAF2, TRAF5, TRAF6, IKKα, and IKKβ.Citation39
OI-57
The NleG homologs constitute the largest family of T3SS-delivered effectors, with 14 members in EHEC O157:H7. In addition to encoding for two virulence factors (AdfO and Ckf), OI-57 also encodes three NleG effectors, i.e., NleG2-3 (z2149), NleG6-2 (z2150), and NleG5-2 (z2151).Citation88 The C-terminal domain of NleG2-3 (residues 90 to 191) is the most conserved region in NleG proteins, containing a RING finger/U-box motif.Citation40 Bacterial-encoded NleG effectors, including NleG2-3, NleG6-2, and NleG5-2 were demonstrated to function as E3 ubiquitin ligases analogous to RING finger and U-box enzymes in eukaryotes.Citation40 The NleG2-3 effector was found to reside in the host cytosol, triggering the ubiquitination-mediated degradation of hexokinase-2 and SNAP29 (synaptosomal-associated protein of 29 kDa).Citation41 However, the exact functions of NleG2-3, NleG6-2, and NleG 5–2 in EHEC O157:H7 virulence and infection remain unclear.
OI-71
OI-71 is a 57984 bp island (ranging from 2271618 bp to 2329601 bp in the EHEC O157:H7 EDL933 genome) that contains 77 ORFs (including z2558, z2560, z2561, z2562, z2563, z2565, and z6010 to z6081). OI-71 is widely distributed in EHEC O157:H7 strains from clade 1 to 9, except for three strains of clade 1 (strains TB182A, DEC5D, and USDA5905) ( and Table S1). OI-71 contains four effector genes, including nleA (z6024, also called espI), nleF (z6020), nleH2 (z6021), and espM1 (z2565).Citation9 The NleA effector localizes to the Golgi apparatus.Citation42 NleA was first shown to compromise the Sec23/24 complex, which is a component of the mammalian COPII protein coat that shapes intracellular protein transport vesicles by directly binding to Sec24.Citation43 Moreover, NleA is known to directly target the Nod-like receptor 3 (NLRP3), one of the three basic components of the inflammasome; in particular, NleA interrupts the deubiquitination of NLRP3, which is a prerequisite for the assembly of the inflammasome.Citation44 Consequently, NleA reduces the formation of the NLRP3 inflammasome and prevents the activation of caspase-1 in host cells.Citation44 Similarly, NleF localizes to the cytoplasm.Citation99 Challenge of gnotobiotic piglets with wild-type and nleF mutant EHEC O157:H7 revealed a role of NleF in the colonization of the colon and rectoanal junction of piglets.Citation99 EHEC O157:H7 was also reported to use NleF to counteract the host inflammatory response by dampening the caspase-4-mediated inflammatory epithelial cell death and preventing the production of IL-1β.Citation45 In addition, NleF not only interrupted the heterodimerization of caspase-4-p19 and caspase-4-p10, but also inhibited the interaction of caspase-1 and caspase-4.Citation45 NleH2 (303 amino acids) shares an 84% amino acid sequence identity with NleH1 (293 amino acids). Similar to NleH1, NleH2 also binds directly to RPS3, and colocalized with RPS3 in the cytoplasm, but not in cell nuclei.Citation33 In contrast to NleH1, NleH2 stimulated the RPS3-dependent transcription, as well as an AP-1-dependent reporter.Citation33 EHEC O157:H7 encodes two espM alleles, espM1 and espM2, which reside in OI-71 and OI-108, respectively. Both EspM1 and EspM2 effectors were found to activate the RhoA pathway and induce the formation of stress fibers upon infection of host cells.Citation46 In addition to the induction of the formation of stress fibers, both EspM1 and EspM2 also repressed the formation of actin pedestals during an EHEC O157:H7 infection.Citation47
OI-79
OI-79 is a 45179 bp island (ranging from 2743224 bp to 2788402 bp in the EHEC O157:H7 EDL933 genome) that contains 54 ORFs (from z3069 to z3130). OI-79 is widely distributed in EHEC O157:H7 strains from clade 1 to 9, except for three strains of clade 1 (strain DEC5B, DEC5D, and DEC5E) ( and Table S1). The espJ (z3071) and espFu (z3072) genes encode translocated effectors. EspJ has an 22% sequence identity to HopF, an effector protein of Pseudomonas syringae.Citation100 In EPEC, EspJ has been identified as a unique ADP ribosyltransferase that directly inhibits Src kinase activity by simultaneous amidation and ADP ribosylation of the conserved kinase-domain residue, Src E310, resulting in glutamine-ADP ribose.Citation48 Although EspJ is not required for the formation of A/E lesions in HEp-2 cells or human intestinal explants, in-vivo studies performed using C57BL/6 J mice have shown that EspJ affects the dynamics of the clearance of C. rodentium from the intestinal tract of the host, suggesting the role for EspJ in host survival and pathogen transmission.Citation49 EspFU bridges the interaction of Tir with the host IRSp53 or IRTKS proteins and N-WASP.Citation50 The C terminus of EspFU then interacts with the GTPase-binding domain (GBD) to activate N-WASP, recruiting the Arp2/3 complex and leading to actin polymerization.Citation50 After initial EHEC O157:H7 colonization of the intestinal surface, EspFU was also demonstrated to stabilize the bacterial association with the epithelial cytoskeleton and promote the expansion of infection beyond its initial sites.Citation51
OI-122
OI-122, in addition to encoding virulence factors (pagC and efa1), encodes three effectors, i.e., EspL2 (z4326), NleB1 (z4328), and NleE (z4329).Citation101 EspL2 localizes to the cytosolic side of the plasma membrane.Citation52 Annexin 2 (also known as annexin II) is the target of EspL2 in host cells.Citation52 The interaction of EspL2 with annexin 2 was shown to increase the bundling activity of F-actin and strengthen the membrane–cytoskeleton linkage, resulting in the condensation of actin fibers and the induction of a pseudopod-like structure.Citation52 EHEC NleB1 has an 89% sequence identity to NleB from C. rodentium, with only a difference of 7 amino acids between the EHEC and EPEC polypeptides.Citation102 NleB1 was reported to directly inactivate the death domains in several proteins (including TRADD, FADD, RIPK1, and TNFR1) dependent on its N-GlcNAc transferase activity.Citation53 These modifications blocked the interactions between the death domains, thereby disrupting the inflammatory NF-κB signaling, caspase 8-dependent apoptosis, and necroptosis.Citation54 NleE is a highly conserved T3SS effector protein of A/E pathogens that is encoded in the same operon containing NleB1. NleE inhibits the activation of NF-κB by preventing the activation of IKKβ and consequently the degradation of the IκB NF-kB inhibitor.Citation55 This activity of NleE was shown to be enhanced by NleB1.Citation55 In addition, NleE is also known to inhibit the nuclear translocation of the NF-κB subunit p65, thereby reducing the IL-8 response during bacterial infection.Citation56
OI-148
In addition to genes encoding virulence factors, seven genes (including map, espF, espG, espH, espB, tir, and espZ) encoding effectors are also present in OI-148.Citation103 In initial stages of EHEC infection, the mitochondrial-associated Map protein was found to be responsible for the transient formation of filopodium-like structures at the sites of bacterial infection, with the process being dependent on the Cdc42 small G protein.Citation57 Map is also essential for the disruption of the intestinal barrier function and alteration of tight junctions, and this activity was reported to be independent of mitochondrial targeting.Citation58 EspF is a proline-rich effector protein in EHEC O157:H7, containing four proline-rich repeats.Citation104 It has been shown to play a role in the disruption of the intestinal barrier function, being required for the loss of transepithelial resistance for increased monolayer permeability, and for the redistribution of the tight junction-associated protein occluding.Citation59 EspG was also reported to trigger the formation of actin stress fibers and the destruction of microtubule networks underneath adherent bacteria in fibroblasts.Citation60 It has also been shown to interact with tubulins, stimulating the destabilization of microtubules in vitro, with this destabilization triggering the activation of the RhoA-ROCK signaling pathway through the activation of the guanine nucleotide exchange factor (GEF-H1).Citation60 EspH localizes to the host cell membrane and modulates the host actin cytoskeleton structure, affecting the formation of filopodium and pedestal structures.Citation61 In addition to its role in translocation, EspB was reported to exert an effector activity. Cytosolic EspB was shown to localize to the region of the bacterial attachment,Citation62 and cells transfected with EspB displayed altered morphology associated with a reduced number of stress fibers.Citation63 Additionally, EHEC EspB has been shown to bind α-catenin, a cytoskeleton-associated molecule, consistent with its role in modulating the host cell cytoskeleton.Citation64 Tir (also called EspE) serves as a receptor for the intimin adhesion protein on the bacterial surface, triggering a number of signaling cascades and leading to the formation of pedestal structures and A/E lesions.Citation26 EspZ (also known as SepZ) has been shown to be localized in pedestals alongside phosphorylated Tir.Citation65 The translocation of EspZ was demonstrated to be restricted to the formation of pedestals and not involved in the disruption of the integrity of cellular tight junctions or in mediating cytoskeletal rearrangements.Citation65 EspZ has also been identified as a gatekeeper that regulates the translocation of Tir, as well as other effectors including Map and EspF.Citation66
OIs encoding virulence regulatory proteins
To ensure the successful EHEC O157:H7 colonization, virulence-related genes are subjected to strict regulation that ensures their expression only under optimal environmental conditions, while also avoiding intense metabolic cost or alerting the host immune system.Citation76 The coordinated expression of virulence genes is controlled by regulatory proteins, which precisely activate or repress particular genes depending on environmental factors and the stage of infection.Citation105 Several OI-encoded virulence regulatory proteins have been demonstrated to affect the expression of EHEC O157:H7 virulence genes, and the precise molecular mechanisms underlying this regulation have also been revealed in recent years. These OIs encoding virulence regulatory proteins include OI-9, OI-19, OI-47, OI-50, OI-51, OI-115, OI-119, and OI-148 (, and ).
Figure 4. Regulation of LEE by regulatory proteins and sRNAs encoded in O islands. The master LEE regulator, Ler recognizes AT rich sequences and activates the transcription of LEE operons LEE2, LEE3, LEE4, and LEE5. The OvrA, OvrB, LmiA, RgdR, GrlA, and EtrB regulatory proteins encoded in O islands, directly activate the transcription of LEE genes through directly binding to the promoter region of LEE1. GrvA indirectly activates the expression of LEE genes through GadW and GadE. In contrast, GadE directly represses the expression of LEE genes, while EtrA, EivF, and YhiF indirectly repress the expression of LEE genes. PsrA and PsrB indirectly regulate LEE genes through the GAD acid stress response regulators, GadE and YhiF. The cis-encoded sRNA Arl regulates ler mRNA post-transcriptionally by specifically targeting its 3′ region, preventing its translation, and thus the expression of LEE genes. The Esr41 sRNA regulates the expression of ler at a post-transcriptional level in an Hfq-dependent manner; however, the mechanism of this regulation remains unclear. sRNA350 positively regulates the expression of LEE genes through a still unknown mechanism. The figure has been inspired by the previous work of Furniss et al. (2017), Bhatt et al. (2016), and Platenkamp et al. (2018)
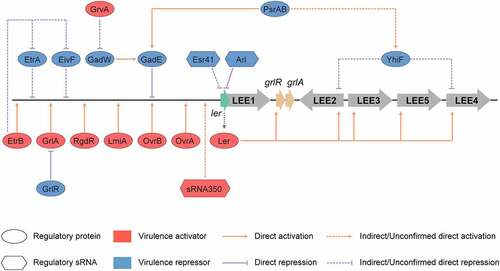
OI-9
OI-9 is a 6133 bp island (ranging from 323996 bp to 330128 bp in the EHEC O157:H7 EDL933 genome), which is highly conserved and widely distributed in all 143 EHEC O157:H7 strains of 9 clades ( and Table S1). OI-9 comprises seven ORFs (from z0342 to z0348). In particular, z0342, and z0346 (also termed OI-encoded virulence regulator B, ovrB) are predicted to encode two putative regulators; z0343 encodes an oxidoreductase, and z0347 encodes a hydrolase; z0348 encodes a major facilitator superfamily (MFS) transporter, whereas z0344 and z0345 encode hypothetical proteins of unknown function. We recently found that ovrB encodes a novel transcriptional activator, which is required for bacterial adherence to host cells and the expression of LEE genes in EHEC O157:H7.Citation67 Deletion of ovrB significantly reduced bacterial adherence to HeLa and Caco-2 cells, as well as the expression of LEE genes in EHEC O157:H7. OvrB was demonstrated to directly bind to the promoter region of LEE1, activating the transcription of ler (encoding a master regulator of LEE genes), which in turn activates LEE1-5 genes to promote EHEC O157:H7 adherence. Electrophoretic mobility shift assay (EMSA) and chromatin immunoprecipitation quantitative PCR (ChIP-qPCR) analysis showed that OvrB directly bound to the LEE1 promoter, regulating the expression of LEE1-LEE5 via the master LEE regulator, Ler. Furthermore, mouse oral infection assays revealed that OvrB promoted EHEC O157:H7 colonization in the mouse intestine. We also showed that OvrB is a widespread transcriptional activator of virulence genes in different E. coli pathotypes among various pathogens. In contrast, deletion of other OI-9 genes (z0342, z0343, z0344, z0345, z0347, and z0348) had no visible effect on EHEC O157:H7 virulence, as both the adherence and levels of expression of LEE genes in all mutants were comparable to those of the wild-type EHEC O157:H7.Citation67 We thus proposed that these genes might be redundant or involved in other bacterial processes. This hypothesis needs to be further investigated.
OI-19
OI-19 is a 1834 bp island (ranging from 424359 bp to 426192 bp in the EHEC O157:H7 EDL933 genome), which is highly conserved and widely distributed in all the 143 EHEC O157:H7 strains of 9 clades ( and Table S1). OI-19 includes two ORFs, z0442 (also termed OI-encoded virulence regulator A, ovrA) and z0443. The ovrA gene has been predicted to encode a putative transcriptional regulator containing an AraC-like DNA-binding helix-turn-helix (HTH) domain (pfam 12833) in the C-terminal region, whereas z0443 encodes a hypothetical protein of unknown function. We found that OvrA constitutes a novel transcriptional regulator that promotes bacterial adherence by activating the expression of LEE genes in EHEC O157:H7.Citation68 Deletion of ovrA resulted in the reduced adherence of EHEC O157:H7 to cultured host cells (HeLa and Caco-2), and reductions in the formation of A/E lesions and expression of LEE genes. Conversely, z0443 deletion did not obviously affect EHEC O157:H7 virulence. EMSA, ChIP-qPCR, and DNase I footprinting analyses revealed that OvrA directly bound to a 17-base pair motif (5′-GACATTTAATGATAATG-3′; −183 to −167 from the ler translational start site) within the LEE1 promoter region to activate the expression of ler, and in turn activate the expression of LEE1–LEE5 via the master LEE regulator Ler. Mouse colonization experiments revealed that OvrA promoted EHEC O157:H7 adherence in the mouse intestine, preferentially the colon. Moreover, OvrA was also shown to be involved in the regulation of virulence in other non-O157 pathogenic E. coli, including EHEC strains O145:H28 and O157:H16 and EPEC strain O55:H7.Citation68
OI-47
OI-47 is a 31727 bp island (ranging from 1420969 bp to 1452695 bp in the EHEC O157:H7 EDL933 genome) that encodes 27 ORFs (from z1528 to z1556). OI-47 is widely distributed in nearly all EHEC O157:H7 strains of nine clades, except for one strain of clade 1 (strain DEC5E) and two strains of clade 5 (strains Z1834 and 7636) ( and Table S1). GrvA (for global regulator of virulence A, z1531) encodes a Tox-R family transcriptional regulator, which has been found to be also involved in the regulation of LEE.Citation69 Moreover, GrvA works in conjunction with RcsB, a regulator involved in the activation and repression of LEE; however, the underlying mechanism was not understood until recently.Citation69,Citation70 RcsB positively regulates the expression of grvA by directly binding to the grvA promoter region; this binding however is temperature sensitive.Citation70 In addition, GrvA positively regulates LEE by indirectly downregulating GadE, a regulator of acid tolerance and known repressor of ler.Citation70,Citation106 Moreover, the repression of gadE by GrvA was reported to be dependent on an intact gadW.Citation70
OI-50
OI-50 also encodes a putative AraC/XylS family regulator, PsrA (prophage-encoded secretion regulator A, z1789). Deletion of psrA was shown to significantly increase T3SS secretion in a manner analogous to the deletion of the entire OI-50 region.Citation71 Interestingly, PsrB (z2104), present on OI-57, is the closest homolog of PsrA (90% identity). Deletion of psrB did not have a significant effect on T3SS secretion but repressed secretion when provided in trans in the psrA deletion mutant. Both PsrA and PsrB were shown to indirectly regulate T3SS through the GAD acid stress response regulators GadE and YhiF. Furthermore, the same study also demonstrated that OI-50 was required for the persistence of infection in a ruminant model of colonization and that the effector encoding loci and PsrA regulator both contributed to this persistence phenotype.Citation71
OI-51
OI-51 is a 15582 bp island (ranging from 1678561 bp to 1694142 bp in the EHEC O157:H7 EDL933 genome) that contains 22 ORFs (from z1835 to z1857). OI-51 existed in all the EHEC O157:H7 strains of clade 9 and three strains of clade 5 (strains TB21-1, SS TX 754–1, and F8092B) ( and Table S1). Deletion of OI-51 resulted in a reduction in the expression of LEE and T3SS. In addition, the deletion led to a reduced capacity for attachment to epithelial cells, also significantly reducing the levels of EHEC O157:H7 excretion in sheep.Citation72 Through a combination of deletion and complementation analyses, RgdR was identified as a novel regulator of OI-51, thereby able to stimulate T3SS and bacterial adherence. Mechanistically, the stimulation of T3SS by RgdR was reported to occur through the initial activation of transcription from the LEE1 promoter, leading to the induction of the Ler autoregulatory cascade that in turn promoted the expression of the remaining LEE operons and T3SS.Citation72
OI-115
OI-115 is a 16948 bp island (ranging from 3786306 bp to 3803253 bp in the EHEC O157:H7 EDL933 genome) that contains 21 ORFs (from z4180 to z4200). OI-115 is widely distributed in nearly all EHEC O157:H7 strains of nine clades, except for two strains of clade 1 (strains TB182A and DEC5D) ( and Table S1). OI-115 harbors a gene cluster encoding components of a second cryptic T3SS, E. coli type III secretion system 2 (ETT2). The functionality and role of the ETT2 system have been controversial, with the locus being subject to widespread mutational attrition.Citation107 However, two system-encoded regulators, EtrA (z4184) and EivF (z4198), were found to be functional repressors of LEE in EHEC O157:H7.Citation73 Mutational inhibition of etrA and eivF from the ETT2 cluster was demonstrated to lead to the significantly increased secretion of LEE-encoded proteins (including EspA, EspB, Tir, and EspP) and to increased adhesion to human intestinal cells (Int407). Transcriptional fusions and microarrays analyses indicated that EtrA and EivF exert profound negative effects on gene transcription within LEE. Consistent with these observations, the expression of these regulators in an EHEC O26:H- strain led to the suppression of protein secretion under LEE-inducing conditions.Citation73 In addition to EtrA and EivF, ETT2 also encodes a third virulence regulator, EtrB (z4167), which was reported to play an important role in EHEC O157:H7 pathogenesis.Citation74 The etrB gene is expressed as a monocistronic transcript, and EtrB positively autoregulates its expression. EtrB directly interacts with the ler regulatory region to activate the expression of LEE and promote the formation of A/E lesions. In particular, EtrB activates the expression of LEE, not only through direct regulation but also by repressing the expression of eivF and etrA. Moreover, EtrB was also found to modulate the expression of genes encoding products with distinct functions, including the non-LEE-encoded effector NleA, a fimbrial adhesin (Loc11), a small RNA (RyeA/SraC), and a gene involved in maltose and tryptophan metabolism.Citation74
OI-119
OI-119 is a 3267 bp island (ranging from 3878697 bp to 3881963 bp in the EHEC O157:H7 EDL933 genome), which is highly conserved and widely distributed in all the 143 EHEC O157:H7 strains of 9 clades ( and Table S1). OI-119 contains five ORFs (from z4267 to z4271). The z4267 gene (also termed lmiA for low-magnesium-induced regulator A) encodes a putative DNA-binding protein, z4268 and z4269 encode hypothetical proteins of unknown function, whereas z4270 and z4271 encode putative ATP-binding proteins of the ABC transport system. We recently discovered that LmiA, as a novel virulence regulator within OI-119, promoted the bacterial adherence to epithelial cells and expression of LEE genes, facilitating EHEC O157:H7 colonization.Citation75 In contrast, a ΔlmiA mutant exhibited significantly reduced bacterial adherence, as evidenced by bacterial adherence and FAS assays, as well as suppressed the transcriptional and translational expression of LEE genes compared with that of the wild-type. EMSA, ChIP-qPCR, and DNase I footprinting analyses revealed that LmiA directly bound to a 17-base pair motif (5′- TTAAAGTCGTTTGTTAA −3′; −247 to −231 from the ler proximal transcriptional start site) within the LEE1 promoter region to activate the expression of ler, and in turn promote the expression of LEE1-5 genes through Ler. Furthermore, LmiA was reported to be an essential element that integrates the low-magnesium signals from the large intestine into this LEE regulatory network via the PhoP/PhoQ two-component regulatory system.Citation75 This LmiA-mediated virulence regulatory pathway is widely present in a range of EHEC and EPEC serotypes. Disruption of this pathway significantly decreased EHEC O157:H7 adherence in the mouse intestinal tract. Moreover, feeding mice a magnesium-rich diet significantly reduced EHEC O157:H7 adherence in vivo. Therefore, our findings supported the use of magnesium as a dietary supplement and provided greater insights into the dietary cues that can prevent EHEC and EPEC infections in humans.Citation75
OI-148
OI-148 encodes three virulence regulatory proteins: Ler (locus of enterocyte effacement regulator), GrlA (global regulator of LEE activator), and GlrR (global regulator of LEE repressor). Ler, encoded by the first gene in the LEE1 operon, is an HNS-like regulatory proteinCitation108 that recognizes AT rich sequences and acts as a derepressor for the transcriptional silencing exerted by the histone-like nucleoid-structuring protein (H-NS). Ler is required for the increased transcription of LEE operons, LEE2, LEE3, LEE4, LEE5, as well as that of grlRA and espF, espG, and map.Citation77 Additionally, Ler is also required for the expression of non-LEE located virulence genes, including espC, stcE, and lpf.Citation76,Citation78 Consistently, EHEC O157:H7 nonpolar ler mutants were unable to form A/E lesions on HEp-2 cells.Citation109 In addition, these ler mutants failed to express type III secreted effectors and showed a decreased expression of non-LEE-encoded virulence factors.Citation109 Both GrlA and GrlR are encoded by the grlRA operon located between the rorf3 gene and the LEE2 operon in LEE. GrlA drives the expression of virulence genes, probably through its direct binding to the suboptimal 18-base-pair spacer between the −10 and −35 elements of the distal LEE1 promoter, P1, via a HTH DNA-binding motif.Citation79,Citation80 This binding has been suggested to drive the expression of ler and subsequently all the LEE genes. Conversely GrlR, the cellular levels of which are regulated in a growth phase-dependent manner,Citation81 was shown to antagonize this system by directly binding to GrlA, preventing its interaction with the LEE1 promoter to repress the transcription of LEE genes.Citation82
OIs encoding virulence-sRNA
Small regulatory RNAs (sRNAs) are typically noncoding RNAs that are 50 to 300 nucleotides in length.Citation110 The majority of sRNAs are known to target mRNAs, affecting the transcriptional elongation, stability, or translation of mRNAs.Citation110 Moreover, sRNAs have been demonstrated to coregulate numerous biological processes, including oxidative stress, acid stress, motility, quorum sensing, antibiotic resistance, and virulence.Citation110 A previous study reported that the density of sRNAs encoded in OIs was 39 sRNAs per Mb of DNA, compared with 23 sRNAs per Mb of DNA in the core genome.Citation111 To date, four sRNAs encoded in OIs have been identified to be involved in the regulation of EHEC O157:H7 virulence, including Arl (OI-148), sRNA350 (OI-148), Esr41 (OI-43), and Esr055 (OI-93) (, and ).
OI-148
ggsOI-148 encodes two virulence regulatory sRNAs: Arl (antisense regulator of ler RNA) and sRNA350. The cis-encoded sRNA Arl was shown to influence the expression of LEE in EHEC O157:H7.Citation85 Arl is located downstream of ler but transcribed from the antisense strand. Consequently, Arl exhibits extensive complementarity to the LEE1-encoded ler mRNA. The transcription of arl is stimulated by elevated cytoplasmic levels of iron or hydroxyl radical but does not require the iron-responsive transcriptional factor Fur.Citation85 In addition, Arl regulates the ler-encoded LEE1 mRNA post-transcriptionally by specifically targeting the 3′ region of ler, preventing its translation, and thus the expression of LEE genes.Citation85 sRNA350 is located downstream of the cesF gene, and acts as a global regulator of the LEE island.Citation86 Overexpression of sRNA350 in wild-type EHEC O157:H7 resulted in the upregulation of various genes within LEE, including ler, sepL, espA, tir, eae, and escV, as measured by qRT-PCR.Citation86 However, the transcript levels of nleA were unaffected.Citation86
OI-43
The OI-43 encoded sRNA Esr41 (EHEC O157 small RNA #41) is located in an intergenic region between z1181 and z1182. Esr41 is approximately 70 nucleotides long and harbors a 3ʹ GC-rich palindrome sequence followed by a long poly(U), which is characteristic of rho-independent terminators and also a structural feature required for the action of Hfq.Citation112 Esr41 was reported to repress the expression of LEE and reduce EHEC O157:H7 adhesion to host cells.Citation83 EHEC O157:H7 harboring an esr41-expressing multicopy plasmid abolished the expression of LEE by downregulating the expression of ler and pch that are known positive regulators of LEE.Citation83 Esr41 regulates the expression of ler at a post-transcriptional level in an Hfq-dependent manner.Citation83 However, while the repression of LEE appears to be attributed to an interaction between the leader region of the ler mRNA and Esr41, no sites of complementarity have been identified between Esr41 and ler, and hence the mechanism of regulation remains unclear.
OI-93
The OI-93 encoded sRNA Esr055 was identified in a screen for upregulated genes/sRNAs from EHEC attached to HeLa cells compared with EHEC grown in Dulbecco modified Eagle medium (DMEM).Citation105 Esr055 is located in an intergenic region between z3342 and stx1B, which encode a 9-O-acetyl-N-acetylneuraminic acid deacetylase, and the B subunit of Stx 1, respectively.Citation84 Further characterization revealed that an esr055 deletion increased the adherence of EHEC O157:H7 to HeLa cells. Additionally, greater numbers of the esr055 deletion strain were recovered from the colons than from the ceca of infected mice.Citation84 The expression of Esr055 is directly activated by the DeoR regulator, the expression of which is positively affected by DNA, which is significantly more abundant in the ileum than in the colon of mice.Citation84 Finally, RNA-seq experiments comparing the expression of wild-type and Δesr055 EHEC O157:H7 strains grown in vitro revealed the differential expression of over 400 genes, and led to the identification of 5 candidate genes (z0568, z0974, z1356, z1926, and z5187) as direct targets of Esr055 using informatics predictions.Citation84
Conclusions and future perspectives
Genomic comparison between EHEC O157:H7 and E. coli K-12 provided a broad array of whole genome-level information with biological and medical importance.Citation9,Citation10 The presence of a well-conserved 4.1-Mb sequence can be considered a chromosomal backbone in E. coli and numerous strain-specific DNA segments of foreign origins indicate how the two strains have been diversified from a common ancestral lineage. There is no doubt that the acquisition of OIs is critical for EHEC O157:H7 to evolve into successful human intestinal pathogens. Several comparative and epidemiological studies have indicated that EHEC O157:H7 descended from a nontoxigenic and less virulent EPEC O55:H7 ancestor via the following four sequential events: (1) acquisition of Stx2-containing bacteriophage (OI-45), (2) acquisition of the virulence plasmid pO157 and the genes encoding O antigens (OI-84), (3) acquisition of the Stx1-containing bacteriophage (OI-93), and (4) loss of the ability to ferment D-sorbitol and loss of beta-glucuronidase activity.Citation113,Citation114 Therefore, acquisition of these key OIs may promote the evolution of EHEC O157:H7 into the most virulent EHEC serotype, responsible for severer diseases and having low infection dose.Citation115
Horizontally acquired OIs contribute to EHEC O157:H7 pathogenesis by providing various virulence elements, including virulence factors, effectors, as well as virulence regulatory proteins and sRNAs. The functions of several OIs were only established in recent years, revealing the association of more virulence elements with the virulence and pathogenesis of EHEC O157:H7. Nevertheless, the majority of OIs are comprised of genes of unknown function. For example, only the OI-9 encoded OvrB is required for bacterial adherence to host cells and the expression of LEE genes in EHEC O157:H7, as deletion of other OI-9 genes (z0342, z0343, z0344, z0345, z0347, and z0348) did not have a visible effect on EHEC O157:H7 virulence.Citation67 Whether these OI-associated genes are redundant or involved in other bacterial processes needs to be further investigated. Moreover, the majority of current studies has mainly focused on investigating the relationship between OIs and the virulence of EHEC O157:H7. In contrast, studies on the functions of OIs associated with other physiological processes have been relatively limited. We previously reported that the OI-29 encoded z0639 (also termed GmrA) was required for motility and flagellar synthesis in EHEC O157:H7.Citation116 GmrA was shown to directly bind to the promoter of fliA, an RNA polymerase sigma factor, thereby regulating the FliA-controlled flagellar genes.Citation116 Therefore, future studies should focus on the effect of OIs on other physiological functions, such as bacterial motility, acid resistance, and survival in adversity, which might facilitate an improved understanding of the EHEC pathogenicity and adaptation mechanisms to the host.
Diarrheic diseases caused by EHEC are an important public health problem worldwide. Outbreak surveillance data from the CDC have reported that EHEC O157:H7 alone results in more than 73,000 cases of illness, 2200 hospitalizations, and 60 deaths annually in the United States.Citation117 Although several therapeutic strategies have been developed, including the use of antibiotics and vaccinations, there is no effective treatment for EHEC infections.Citation118 Furthermore, the use of antibiotics has also been contraindicated, as antibiotic-induced bacterial cell lysis would lead to an increased release of Stxs.Citation119 Therefore, highly effective measures for the prevention and control of EHEC O157:H7 infections are essential. Considering that the deletion of many OIs genes would significantly decrease EHEC O157:H7 adherence and expression of virulence genes both in vitro and in vivo, these virulence-related OIs genes might hence be used as potential targets for the development of novel therapeutics for the treatment of EHEC O157:H7 infections.
Supplemental Material
Download MS Excel (21.6 KB)Disclosure statement
No potential conflict of interest was reported by the author(s).
Supplementary material
Supplemental data for this article can be accessed on the publisher’s website.
Additional information
Funding
References
- Michino H, Araki K, Minami S, Takaya S, Sakai N, Miyazaki M, Ono A, Yanagawa H. Massive outbreak of Escherichia coli O157: H7 infection in schoolchildren in Sakai city, Japan, associated with consumption of white radish sprouts. Am J Epidemiol. 1999;150(8):787–24. doi:10.1093/oxfordjournals.aje.a010082.
- Bavaro MF. E. coli O157:H7 and other toxigenic strains: the curse of global food distribution. Curr Gastroenterol Rep. 2012;14(4):317–323. doi:10.1007/s11894-012-0264-6.
- Riley LW, Remis RS, Helgerson SD, McGee HB, Wells JG, Davis BR, Hebert RJ, Olcott ES, Johnson LM, Hargrett NT, et al. Hemorrhagic colitis associated with a rare Escherichia coli serotype. N Engl J Med. 1983;308(12):681–685. doi:10.1056/NEJM198303243081203.
- Karmali MA, Steele BT, Petric M, Lim C. Sporadic cases of haemolytic-uraemic syndrome associated with faecal cytotoxin and cytotoxin-producing Escherichia coli in stools. Lancet. 1983;1:619–620. doi:10.1016/S0140-6736(83)91795-6.
- Vallance BA, Chan C, Robertson ML, Finlay BB. Enteropathogenic and enterohemorrhagic Escherichia coli infections: emerging themes in pathogenesis and prevention. Can J Gastroenterol. 2002;16(11):771–778. doi:10.1155/2002/410980.
- Garmendia J, Frankel G, Crepin VF. Enteropathogenic and enterohemorrhagic Escherichia coli infections: translocation, translocation, translocation. Infect Immun. 2005;73(5):2573–2585. doi:10.1128/IAI.73.5.2573-2585.2005.
- Franzin FM, Sircili MP. Locus of enterocyte effacement: a pathogenicity island involved in the virulence of enteropathogenic and enterohemorrhagic Escherichia coli subjected to a complex network of gene regulation. Biomed Res Int. 2015;2015:534738. doi:10.1155/2015/534738.
- Paton AW, Paton JC. Direct detection of Shiga toxigenic Escherichia coli strains belonging to serogroups O111, O157, and O113 by multiplex PCR. J Clin Microbiol. 1999;37(10):3362–3365. doi:10.1128/JCM.37.10.3362-3365.1999.
- Perna NT, Plunkett G, Burland V, Mau B, Glasner JD, Rose DJ, Mayhew GF, Evans PS, Gregor J, Kirkpatrick HA, et al. Genome sequence of enterohaemorrhagic Escherichia coli O157:H7. Nature. 2001;409(6819):529–533. doi:10.1038/35054089.
- Hayashi T, Makino K, Ohnishi M, Kurokawa K, Ishii K, Yokoyama K, Han CG, Ohtsubo E, Nakayama K, Murata T, et al. Complete genome sequence of enterohemorrhagic Escherichia coli O157: H7 and genomic comparison with a laboratory strain K-12. DNA Res. 2001;8:11–22. doi:10.1093/dnares/8.1.11.
- Boyd EF. Bacteriophage-encoded bacterial virulence factors and phage-pathogenicity island interactions. Adv Vir Res. 2012;82:91–118.
- Hacker J, Kaper JB. Pathogenicity islands and the evolution of microbes. Annu Rev Microbiol. 2000;54(1):641–679. doi:10.1146/annurev.micro.54.1.641.
- Yin X, Chambers JR, Wheatcroft R, Johnson RP, Zhu J, Liu B, Gyles CL. Adherence of Escherichia coli O157:H7 mutants in vitro and in ligated pig intestines. Appl Environ Microbiol. 2009;75(15):4975–4983. doi:10.1128/AEM.00297-09.
- Wells TJ, Sherlock O, Rivas L, Mahajan A, Beatson SA, Torpdahl M, Webb RI, Allsopp LP, Gobius KS, Gally DL, et al. EhaA is a novel autotransporter protein of enterohemorrhagic Escherichia coli O157:H7 that contributes to adhesion and biofilm formation. Environ Microbiol. 2008;10(3):589–604. doi:10.1111/j.1462-2920.2007.01479.x.
- Yin X, Wheatcroft R, Chambers JR, Liu B, Zhu J, Gyles CL. Contributions of O Island 48 to adherence of enterohemorrhagic Escherichia coli O157:H7 to epithelial cells in vitro and in ligated pig ileal loops. Appl Environ Microbiol. 2009;75(18):5779–5786. doi:10.1128/AEM.00507-09.
- Nataro JP, Kaper JB. Diarrheagenic Escherichia coli. Clin Microbiol Rev. 1998;11:142–201.
- Saxena SK, O’Brien AD, Ackerman EJ. Shiga toxin, Shiga-like toxin II variant, and ricin are all single-site RNA N-glycosidases of 28 S RNA when microinjected into Xenopus oocytes. J Biol Chem. 1989;264(1):596–601. doi:10.1016/S0021-9258(17)31302-9.
- Poirier K, Faucher SP, Beland M, Brousseau R, Gannon V, Martin C, Harel J, Daigle F. Escherichia coli O157:H7 survives within human macrophages: global gene expression profile and involvement of the Shiga toxins. Infect Immun. 2008;76(11):4814–4822. doi:10.1128/IAI.00446-08.
- Batisson I, Guimond MP, Girard F, An H, Zhu C, Oswald E, Fairbrother JM, Jacques M, Harel J. Characterization of the novel factor paa involved in the early steps of the Adhesion mechanism of attaching and effacing Escherichia coli. Infect Immun. 2003;71(8):4516–4525. doi:10.1128/IAI.71.8.4516-4525.2003.
- Ho TD, Davis BM, Ritchie JM, Waldor MK. Type 2 secretion promotes enterohemorrhagic Escherichia coli adherence and intestinal colonization. Infect Immun. 2008;76(5):1858–1865. doi:10.1128/IAI.01688-07.
- Miller VL, Beer KB, Loomis WP, Olson JA, Miller SI. An unusual pagC::TnphoA mutation leads to an invasion- and virulence-defective phenotype in Salmonellae. Infect Immun. 1992;60(9):3763–3770. doi:10.1128/iai.60.9.3763-3770.1992.
- Faucher SP, Porwollik S, Dozois CM, McClelland M, Daigle F. Transcriptome of Salmonella enterica serovar Typhi within macrophages revealed through the selective capture of transcribed sequences. Proc Natl Acad Sci U S A. 2006;103(6):1906–1911. doi:10.1073/pnas.0509183103.
- Wickham ME, Lupp C, Mascarenhas M, Vazquez A, Coombes BK, Brown NF, Coburn BA, Deng W, Puente JL, Karmali MA, et al. Bacterial genetic determinants of non-O157 STEC outbreaks and hemolytic-uremic syndrome after infection. J Infect Dis. 2006;194(6):819–827. doi:10.1086/506620.
- Stevens MP, Roe AJ, Vlisidou I, van Diemen PM, La Ragione RM, Best A, Woodward MJ, Gally DL, Wallis TS. Mutation of toxB and a truncated version of the efa-1 gene in Escherichia coli O157:H7 influences the expression and secretion of locus of enterocyte effacement-encoded proteins but not intestinal colonization in calves or sheep. Infect Immun. 2004;72(9):5402–5411. doi:10.1128/IAI.72.9.5402-5411.2004.
- Jarvis KG, Girón JA, Jerse AE, McDaniel TK, Donnenberg MS, Kaper JB. Enteropathogenic Escherichia coli contains a putative type III secretion system necessary for the export of proteins involved in attaching and effacing lesion formation. Proc Natl Acad Sci U S A. 1995;92(17):7996–8000. doi:10.1073/pnas.92.17.7996.
- Lodato PB, Kaper JB. Post-transcriptional processing of the LEE4 operon in enterohaemorrhagic Escherichia coli. Mol Microbiol. 2009;71(2):273–290. doi:10.1111/j.1365-2958.2008.06530.x.
- Mcdaniel TK, Jarvis KG, Donnenberg MS, Kaper JB. A genetic locus of enterocyte effacement conserved among diverse enterobacterial pathogens. Proc Natl Acad Sci U S A. 1995;92(5):1664–1668. doi:10.1073/pnas.92.5.1664.
- Elliott SJ, Yu J, Kaper JB. The cloned locus of enterocyte effacement from enterohemorrhagic Escherichia coli O157:H7 is unable to confer the attaching and effacing phenotype upon E. coli K-12. Infect Immun. 1999;67(8):4260–4263. doi:10.1128/IAI.67.8.4260-4263.1999.
- Elliott SJ, Wainwright LA, McDaniel TK, Jarvis KG, Deng YK, Lai LC, McNamara BP, Donnenberg MS, Kaper JB. The complete sequence of the locus of enterocyte effacement (LEE) from enteropathogenic Escherichia coli E2348/69. Mol Microbiol. 1998;28:1–4. doi:10.1046/j.1365-2958.1998.00783.x.
- Larzabal M, Silva WM, Riviere NA, Cataldi AA. Novel effector protein EspY3 of type III secretion system from enterohemorrhagic Escherichia coli is localized in actin pedestals. Microorganisms. 2018;6(4):112. doi:10.3390/microorganisms6040112.
- Yen H, Ooka T, Iguchi A, Hayashi T, Sugimoto N, Tobe T, Van Nhieu GT. NleC, a type III secretion protease, compromises NF-κB activation by targeting p65/RelA. PLoS Pathog. 2010;6(12):e1001231. doi:10.1371/journal.ppat.1001231.
- Pearson JS, Riedmaier P, Marches O, Frankel G, Hartland EL. A type III effector protease NleC from enteropathogenic Escherichia coli targets NF-κB for degradation. Mol Microbiol. 2011;80(1):219–230. doi:10.1111/j.1365-2958.2011.07568.x.
- Gao X, Wan F, Mateo K, Callegari E, Wang D, Deng W, Puente J, Li F, Chaussee MS, Finlay BB, et al. Bacterial effector binding to ribosomal protein S3 subverts NF-κB function. PLoS Pathog. 2009;5(12):e1000708. doi:10.1371/journal.ppat.1000708.
- Gur-Arie L, Eitan-Wexler M, Weinberger N, Rosenshine I, Livnah O. The bacterial metalloprotease NleD selectively cleaves mitogen-activated protein kinases that have high flexibility in their activation loop. J Biol Chem. 2020;295(28):9409–9420. doi:10.1074/jbc.RA120.013590.
- Baruch K, Gur-Arie L, Nadler C, Koby S, Yerushalmi G, Ben-Neriah Y, Yogev O, Shaulian E, Guttman C, Zarivach R, et al. Metalloprotease type III effectors that specifically cleave JNK and NF-κB. EMBO J. 2011;30(1):221–231. doi:10.1038/emboj.2010.297.
- Vlisidou I, Marches O, Dziva F, Mundy R, Frankel G, Stevens MP. Identification and characterization of EspK, a type III secreted effector protein of enterohaemorrhagic Escherichia coli O157:H7. FEMS Microbiol Lett. 2006;263(1):32–40. doi:10.1111/j.1574-6968.2006.00410.x.
- Piscatelli H, Kotkar SA, McBee ME, Muthupalani S, Schauer DB, Mandrell RE, Leong JM, Zhou D, van der Goot FG. The EHEC type III effector NleL is an E3 ubiquitin ligase that modulates pedestal formation. PLoS One. 2011;6(4):e19331. doi:10.1371/journal.pone.0019331.
- Sheng X, You Q, Zhu H, Chang Z, Li Q, Wang H, Wang C, Wang H, Hui L, Du C, et al. Bacterial effector NleL promotes enterohemorrhagic E. coli-induced attaching and effacing lesions by ubiquitylating and inactivating JNK. PLoS Pathog. 2017;13(7):e1006534. doi:10.1371/journal.ppat.1006534.
- Sheng X, You Q, Zhu H, Li Q, Gao H, Wang H, You C, Meng Q, Nie Y, Zhang X, et al. Enterohemorrhagic E. coli effector NleL disrupts host NF-κB signaling by targeting multiple host proteins. J Mol Cell Biol. 2020;12(4):318–321. doi:10.1093/jmcb/mjaa003.
- Wu B, Skarina T, Yee A, Jobin MC, Dileo R, Semesi A, Fares C, Lemak A, Coombes BK, Arrowsmith CH, et al. NleG Type 3 effectors from enterohaemorrhagic Escherichia coli are U-Box E3 ubiquitin ligases. PLoS Pathog. 2010;6(6):e1000960. doi:10.1371/journal.ppat.1000960.
- Valleau D, Little DJ, Borek D, Skarin T, Quaile AT, Leo RD, Houliston S, Lemak A, Arrowsmith CH, Coombes BK, et al. Functional diversification of the NleG effector family in enterohemorrhagic Escherichia coli. Proc Natl Acad Sci U S A. 2018;115(40):10004–10009. doi:10.1073/pnas.1718350115.
- Gruenheid S, Sekirov I, Thomas NA, Deng W, O’Donnell P, Goode D, Li Y, Frey EA, Brown NF, Metalnikov P, et al. Identification and characterization of NleA, a non-LEE-encoded type III translocated virulence factor of enterohaemorrhagic Escherichia coli O157:H7. Mol Microbiol. 2004;51(5):1233–1249. doi:10.1046/j.1365-2958.2003.03911.x.
- Kim J, Thanabalasuriar A, Chaworth-Musters T, Fromme JC, Frey EA, Lario PI, Metalnikov P, Rizg K, Thomas NA, Lee SF, et al. The bacterial virulence factor NleA inhibits cellular protein secretion by disrupting mammalian COPII function. Cell Host Microbe. 2007;2(3):160–171. doi:10.1016/j.chom.2007.07.010.
- Yen H, Sugimoto N, Tobe T, Philpott DJ. Enteropathogenic Escherichia coli uses NleA to inhibit NLRP3 inflammasome activation. PLoS Pathog. 2015;11(9):e1005121. doi:10.1371/journal.ppat.1005121.
- Song T, Li K, Zhou W, Zhou J, Jin Y, Dai H, Xu T, Hu M, Ren H, Yue J, et al. A type III effector NleF from EHEC inhibits epithelial inflammatory cell death by targeting Caspase-4. Biomed Res Int. 2017;2017:4101745. doi:10.1155/2017/4101745.
- Arbeloa A, Bulgin RR, MacKenzie G, Shaw RK, Pallen MJ, Crepin VF, Berger CN, Frankel G. Subversion of actin dynamics by EspM effectors of attaching and effacing bacterial pathogens. Cell Microbiol. 2008;10(7):1429–1441. doi:10.1111/j.1462-5822.2008.01136.x.
- Simovitch M, Sason H, Cohen S, Zahavi EE, Melamed-Book N, Weiss A, Aroeti B, Rosenshine I. EspM inhibits pedestal formation by enterohaemorrhagic Escherichia coli and enteropathogenic E. coli and disrupts the architecture of a polarized epithelial monolayer. Cell Microbiol. 2010;12(4):489–505. doi:10.1111/j.1462-5822.2009.01410.x.
- Young JC, Clements A, Lang AE, Garnett JA, Munera D, Arbeloa A, Pearson J, Hartland EL, Matthews SJ, Mousnier A, et al. The Escherichia coli effector EspJ blocks Src kinase activity via amidation and ADP ribosylation. Nat Commun. 2014;5(1):5887. doi:10.1038/ncomms6887.
- Dahan S, Wiles S, La Ragione RM, Best A, Woodward MJ, Stevens MP, Shaw RK, Chong Y, Knutton S, Phillips A, et al. EspJ is a prophage-carried type III effector protein of attaching and effacing pathogens that modulates infection dynamics. Infect Immun. 2005;73(2):679–686. doi:10.1128/IAI.73.2.679-686.2005.
- Campellone KG, Robbins D, Leong JM. EspFU is a translocated EHEC effector that interacts with Tir and N-WASP and promotes Nck-independent actin assembly. Dev Cell. 2004;7(2):217–228. doi:10.1016/j.devcel.2004.07.004.
- Ritchie JM, Brady MJ, Riley KN, Ho TD, Campellone KG, Herman IM, Donohue-Rolfe A, Tzipori S, Waldor MK, Leong JM. EspF U, a type III-translocated effector of actin assembly, fosters epithelial association and late-stage intestinal colonization by E. coli O157:H7. Cell Microbiol. 2008;10(4):836–847. doi:10.1111/j.1462-5822.2007.01087.x.
- Miyahara A, Nakanishi N, Ooka T, Hayashi T, Sugimoto N, Tobe T. Enterohemorrhagic Escherichia coli effector EspL2 induces actin microfilament aggregation through annexin 2 activation. Cell Microbiol. 2009;11(2):337–350. doi:10.1111/j.1462-5822.2008.01256.x.
- Li S, Zhang L, Yao Q, Li L, Dong N, Rong J, Gao W, Ding X, Sun L, Chen X, et al. Pathogen blocks host death receptor signaling by arginine GlcNAcylation of death domains. Nature. 2013;501(7466):242–246. doi:10.1038/nature12436.
- Pearson JS, Hartland EL. A surprising sweetener from enteropathogenic Escherichia coli. Gut Microbes. 2014;5(6):766–769. doi:10.4161/19490976.2014.983762.
- Nadler C, Baruch K, Kobi S, Mills E, Haviv G, Farago M, Alkalay I, Bartfeld S, Meyer TF, Ben-Neriah Y, et al. The type III secretion effector NleE inhibits NF-κB activation. PLoS Pathog. 2010;6(1):e1000743. doi:10.1371/journal.ppat.1000743.
- Newton HJ, Pearson JS, Badea L, Kelly M, Lucas M, Holloway G, Wagstaff KM, Dunstone MA, Sloan J, Whisstock JC, et al. The type III effectors NleE and NleB from enteropathogenic E. coli and OspZ from Shigella block nuclear translocation of NF-κB p65. PLoS Pathog. 2010;6(5):e1000898. doi:10.1371/journal.ppat.1000898.
- Kenny B, Ellis S, Leard AD, Warawa J, Mellor H, Jepson MA. Co-ordinate regulation of distinct host cell signalling pathways by multifunctional enteropathogenic Escherichia coli effector molecules. Mol Microbiol. 2002;44(4):1095–1107. doi:10.1046/j.1365-2958.2002.02952.x.
- Dean P, Kenny B. Intestinal barrier dysfunction by enteropathogenic Escherichia coli is mediated by two effector molecules and a bacterial surface protein. Mol Microbiol. 2004;54(3):665–675. doi:10.1111/j.1365-2958.2004.04308.x.
- McNamara BP, Koutsouris A, O’Connell CB, Nougayrede JP, Donnenberg MS, Hecht G. Translocated EspF protein from enteropathogenic Escherichia coli disrupts host intestinal barrier function. J Clin Invest. 2001;107(5):621–629. doi:10.1172/JCI11138.
- Matsuzawa T, Kuwae A, Yoshida S, Sasakawa C, Abe A. Enteropathogenic Escherichia coli activates the RhoA signaling pathway via the stimulation of GEF-H1. EMBO J. 2004;23(17):3570–3582. doi:10.1038/sj.emboj.7600359.
- Tu X, Nisan I, Yona C, Hanski E, Rosenshine I. EspH, a new cytoskeleton-modulating effector of enterohaemorrhagic and enteropathogenic Escherichia coli. Mol Microbiol. 2003;47(3):595–606. doi:10.1046/j.1365-2958.2003.03329.x.
- Taylor KA, O’Connell CB, Luther PW, Donnenberg MS. The EspB protein of enteropathogenic Escherichia coli is targeted to the cytoplasm of infected HeLa cells. Infect Immun. 1998;66(11):5501–5507. doi:10.1128/IAI.66.11.5501-5507.1998.
- Taylor KA, PaW L, Donnenberg MS. Expression of the EspB protein of enteropathogenic Escherichia coli within HeLa cells affects stress fibers and cellular morphology. Infect Immun. 1999;67(1):120–125. doi:10.1128/IAI.67.1.120-125.1999.
- Kodama T, Akeda Y, Kono G, Takahashi A, Imura K, Iida T, Honda T. The EspB protein of enterohaemorrhagic Escherichia coli interacts directly with alpha-catenin. Cell Microbiol. 2002;4(4):213–222. doi:10.1046/j.1462-5822.2002.00176.x.
- Kanack KJ, Crawford JA, Tatsuno I, Karmali MA, Kaper JB. SepZ/EspZ is secreted and translocated into HeLa cells by the enteropathogenic Escherichia coli type III secretion system. Infect Immun. 2005;73(7):4327–4337. doi:10.1128/IAI.73.7.4327-4337.2005.
- Berger CN, Crepin VF, Baruch K, Mousnier A, Rosenshine I, Frankel G, Sansonetti PJ. EspZ of enteropathogenic and enterohemorrhagic Escherichia coli regulates type III secretion system protein translocation. mBio. 2012;3(5):e00317–12. doi:10.1128/mBio.00317-12.
- Liu Y, Liu B, Yang P, Wang T, Chang Z, Wang J, Wang Q, Li W, Wu J, Huang D, et al. LysR-type transcriptional regulator OvrB encoded in O island 9 drives enterohemorrhagicEscherichia coli O157:H7 virulence. Virulence. 2019;10(1):783–792. doi:10.1080/21505594.2019.1661721.
- Liu B, Wang J, Wang L, Ding P, Yang P, Yang B. Transcriptional activator OvrA encoded in O Island 19 modulates virulence gene expression in enterohemorrhagic Escherichia coli O157:H7. J Infect Dis. 2020;221:820–829.
- Tobe T, Ando H, Ishikawa H, Abe H, Tashiro K, Hayashi T, Kuhara S, Sugimoto N. Dual regulatory pathways integrating the RcsC-RcsD-RcsB signaling system control enterohaemorrhagic Escherichia coli pathogenicity. Mol Microbiol. 2005;58:320–333.
- Morgan JK, Carroll RK, Harro CM, Vendura KW, Shaw LN, Riordan JT. Global regulator of virulence A (GrvA) coordinates expression of discrete pathogenic mechanisms in enterohemorrhagic Escherichia coli through interactions with GadW-GadE. J Bacteriol. 2016;198(3):394–409. doi:10.1128/JB.00556-15.
- Tree JJ, Roe AJ, Flockhart A, McAteer SP, Xu X, Shaw D, Mahajan A, Beatson SA, Best A, Lotz S, et al. Transcriptional regulators of the GAD acid stress island are carried by effector protein-encoding prophages and indirectly control type III secretion in enterohemorrhagic Escherichia coli O157:H7. Mol Microbiol. 2011;80(5):1349–1365. doi:10.1111/j.1365-2958.2011.07650.x.
- Flockhart AF, Tree JJ, Xu X, Karpiyevich M, McAteer SP, Rosenblum R, Shaw DJ, Low CJ, Best A, Gannon V, et al. Identification of a novel prophage regulator in Escherichia coli controlling the expression of type III secretion. Mol Microbiol. 2012;83(1):208–223. doi:10.1111/j.1365-2958.2011.07927.x.
- Zhang L, Chaudhuri RR, Constantinidou C, Hobman JL, Patel MD, Jones AC, Sarti D, Roe AJ, Vlisidou I, Shaw RK, et al. Regulators encoded in the Escherichia coli type III secretion system 2 gene cluster influence expression of genes within the locus for enterocyte effacement in enterohemorrhagic E. coli O157:H7. Infect Immun. 2004;72(12):7282–7293. doi:10.1128/IAI.72.12.7282-7293.2004.
- Luzader DH, Willsey GG, Wargo MJ, Kendall MM. The type three secretion system 2-encoded regulator EtrB modulates enterohemorrhagic Escherichia coli virulence gene expression. Infect Immun. 2016;84(9):2555–2565. doi:10.1128/IAI.00407-16.
- Liu Y, Han R, Wang J, Yang P, Wang F, Yang B, Boyle JP. Magnesium sensing regulates intestinal colonization of enterohemorrhagic Escherichia coli O157:H7. mBio. 2020;11(6):e02470–20. doi:10.1128/mBio.02470-20.
- Mellies JL, Barron AM, Carmona AM. Enteropathogenic and enterohemorrhagic Escherichia coli virulence gene regulation. Infect Immun. 2007;75(9):4199–4210. doi:10.1128/IAI.01927-06.
- Sharma VK, Zuerner RL. Role of hha and ler in transcriptional regulation of the esp operon of enterohemorrhagic Escherichia coli O157:H7. J Bacteriol. 2004;186(21):7290–7301. doi:10.1128/JB.186.21.7290-7301.2004.
- Rojas-Lopez M, Arenas-Hernandez MM, Medrano-Lopez A, Martinez De La Pena CF, Puente JL, Martinez-Laguna Y, Torres AG. Regulatory control of the Escherichia coli O157:H7 lpf1 operon by H-NS and Ler. J Bacteriol. 2011;193(7):1622–1632. doi:10.1128/JB.01082-10.
- Islam MS, Bingle LE, Pallen MJ, Busby SJ. Organization of the LEE1 operon regulatory region of enterohaemorrhagic Escherichia coli O157:H7 and activation by GrlA. Mol Microbiol. 2011;79(2):468–483. doi:10.1111/j.1365-2958.2010.07460.x.
- Jimenez R, Cruz-Migoni SB, Huerta-Saquero A, Bustamante VH, Puente JL. Molecular characterization of GrlA, a specific positive regulator of ler expression in enteropathogenic Escherichia coli. J Bacteriol. 2010;192(18):4627–4642. doi:10.1128/JB.00307-10.
- Iyoda S, Watanabe H. ClpXP protease controls expression of the type III protein secretion system through regulation of RpoS and GrlR levels in enterohemorrhagic Escherichia coli. J Bacteriol. 2005;187(12):4086–4094. doi:10.1128/JB.187.12.4086-4094.2005.
- Padavannil A, Jobichen C, Mills E, Velazquez-Campoy A, Li M, Leung KY, Mok YK, Rosenshine I, Sivaraman J. Structure of GrlR-GrlA complex that prevents GrlA activation of virulence genes. Nat Commun. 2013;4(1):2546. doi:10.1038/ncomms3546.
- Sudo N, Soma A, Iyoda S, Oshima T, Ohto Y, Saito K, Sekine Y. Small RNA Esr41 inversely regulates expression of LEE and flagellar genes in enterohaemorrhagic Escherichia coli. Microbiol (Reading). 2018;164(5):821–834. doi:10.1099/mic.0.000652.
- Han R, Xu L, Wang T, Liu B, Wang L. A small regulatory RNA contributes to the preferential colonization of Escherichia coli O157: H7 in the large intestine in response to a low DNA concentration. Front Microbiol. 2017;8:274. doi:10.3389/fmicb.2017.00274.
- Tobe T, Yen H, Takahashi H, Kagayama Y, Ogasawara N, Oshima T, Marinus MG. Antisense transcription regulates the expression of the enterohemorrhagic Escherichia coli virulence regulatory gene ler in response to the intracellular iron concentration. PLoS One. 2014;9(7):e101582. doi:10.1371/journal.pone.0101582.
- Gruber CC, Sperandio V, Bäumler AJ. Global analysis of posttranscriptional regulation by GlmY and GlmZ in enterohemorrhagic Escherichia coli O157:H7. Infect Immun. 2015;83(4):1286–1295. doi:10.1128/IAI.02918-14.
- Jacewicz MS, Acheson DW, Binion DG, West GA, Lincicome LL, Fiocchi C, Keusch GT. Responses of human intestinal microvascular endothelial cells to Shiga toxins 1 and 2 and pathogenesis of hemorrhagic colitis. Infect Immun. 1999;67(3):1439–1444. doi:10.1128/IAI.67.3.1439-1444.1999.
- Imamovic L, Tozzoli R, Michelacci V, Minelli F, Marziano ML, Caprioli A, Morabito S. OI-57, a genomic Island of Escherichia coli O157, is present in other seropathotypes of Shiga toxin-producing E. coli associated with severe human disease. Infect Immun. 2010;78(11):4697–4704. doi:10.1128/IAI.00512-10.
- Nicholls L, Grant TH, Robins-Browne RM. Identification of a novel genetic locus that is required for in vitro adhesion of a clinical isolate of enterohaemorrhagic Escherichia coli to epithelial cells. Mol Microbiol. 2000;35(2):275–288. doi:10.1046/j.1365-2958.2000.01690.x.
- McDaniel TK, Kaper JB. A cloned pathogenicity island from enteropathogenic Escherichia coli confers the attaching and effacing phenotype on E. coli K-12. Mol Microbiol. 1997;23(2):399–407. doi:10.1046/j.1365-2958.1997.2311591.x.
- Tobe T, Beatson SA, Taniguchi H, Abe H, Bailey CM, Fivian A, Younis R, Matthews S, Marches O, Frankel G, et al. An extensive repertoire of type III secretion effectors in Escherichia coli O157 and the role of lambdoid phages in their dissemination. Proc Natl Acad Sci U S A. 2006;103(40):14941–14946. doi:10.1073/pnas.0604891103.
- Pearson JS, Hartland EL, Sperandio V, Hovde CJ. The inflammatory response during enterohemorrhagic Escherichia coli infection. Microbiol Spectr. 2014;2(4):EHEC-0012–2013. doi:10.1128/microbiolspec.EHEC-0012-2013.
- Gao X, Wang X, Pham TH, Feuerbacher LA, Lubos ML, Huang M, Olsen R, Mushegian A, Slawson C, Hardwidge PR. NleB, a bacterial effector with glycosyltransferase activity, targets GAPDH function to inhibit NF-κB activation. Cell Host Microbe. 2013;13(1):87–99. doi:10.1016/j.chom.2012.11.010.
- Kaper JB, Nataro JP, Mobley HL. Pathogenic Escherichia coli. Nat Rev Microbiol. 2004;2(2):123–140. doi:10.1038/nrmicro818.
- Miao EA, Miller SI. A conserved amino acid sequence directing intracellular type III secretion by Salmonella typhimurium. Proc Natl Acad Sci U S A. 2000;97(13):7539–7544. doi:10.1073/pnas.97.13.7539.
- Marches O, Wiles S, Dziva F, La Ragione RM, Schuller S, Best A, Phillips AD, Hartland EL, Woodward MJ, Stevens MP, et al. Characterization of two non-locus of enterocyte effacement-encoded type III-translocated effectors, NleC and NleD, in attaching and effacing pathogens. Infect Immun. 2005;73(12):8411–8417. doi:10.1128/IAI.73.12.8411-8417.2005.
- Kim DW, Lenzen G, Page AL, Legrain P, Sansonetti PJ, Parsot C. The Shigella flexneri effector OspG interferes with innate immune responses by targeting ubiquitin-conjugating enzymes. Proc Natl Acad Sci U S A. 2005;102(39):14046–14051. doi:10.1073/pnas.0504466102.
- Coombes BK, Wickham ME, Brown NF, Lemire S, Bossi L, Hsiao WW, Brinkman FS, Finlay BB. Genetic and molecular analysis of GogB, a phage-encoded type III-secreted substrate in Salmonella enterica serovar typhimurium with autonomous expression from its associated phage. J Mol Biol. 2005;348(4):817–830. doi:10.1016/j.jmb.2005.03.024.
- Echtenkamp F, Deng W, Wickham ME, Vazquez A, Puente JL, Thanabalasuriar A, Gruenheid S, Finlay BB, Hardwidge PR. Characterization of the NleF effector protein from attaching and effacing bacterial pathogens. FEMS Microbiol Lett. 2008;281(1):98–107. doi:10.1111/j.1574-6968.2008.01088.x.
- Shan L, Oh H, Chen J, Guo M, Zhou J, Alfano JR, Collmer A, Jia X, Tang X. The HopPtoF locus of Pseudomonas syringae pv. tomato DC3000 encodes a type III chaperone and a cognate effector. Mol Plant Microbe Interact. 2004;17(5):447–455. doi:10.1094/MPMI.2004.17.5.447.
- Konczy P, Ziebell K, Mascarenhas M, Choi A, Michaud C, Kropinski AM, Whittam TS, Wickham M, Finlay B, Karmali MA. Genomic O Island 122, locus for enterocyte effacement, and the evolution of virulent verocytotoxin-producing Escherichia coli. J Bacteriol. 2008;190(17):5832–5840. doi:10.1128/JB.00480-08.
- Araujo-Garrido JL, Bernal-Bayard J, Ramos-Morales F. Type III secretion effectors with arginine N-Glycosyltransferase activity. Microorganisms. 2020;8(3):357. doi:10.3390/microorganisms8030357.
- Ritchie JM, Waldor MK. The locus of enterocyte effacement-encoded effector proteins all promote enterohemorrhagic Escherichia coli pathogenicity in infant rabbits. Infect Immun. 2005;73(3):1466–1474. doi:10.1128/IAI.73.3.1466-1474.2005.
- Viswanathan VK, Koutsouris A, Lukic S, Pilkinton M, Simonovic I, Simonovic M, Hecht G. Comparative analysis of EspF from enteropathogenic and enterohemorrhagic Escherichia coli in alteration of epithelial barrier function. Infect Immun. 2004;72(6):3218–3227. doi:10.1128/IAI.72.6.3218-3227.2004.
- Yang B, Feng L, Wang F, Wang L. Enterohemorrhagic Escherichia coli senses low biotin status in the large intestine for colonization and infection. Nat Commun. 2015;6:6592.
- Vanaja SK, Bergholz TM, Whittam TS. Characterization of the Escherichia coli O157:H7 Sakai GadE regulon. J Bacteriol. 2009;191(6):1868–1877. doi:10.1128/JB.01481-08.
- Ren CP, Chaudhuri RR, Fivian A, Bailey CM, Antonio M, Barnes WM, Pallen MJ. The ETT2 gene cluster, encoding a second type III secretion system from Escherichia coli, is present in the majority of strains but has undergone widespread mutational attrition. J Bacteriol. 2004;186(11):3547–3560. doi:10.1128/JB.186.11.3547-3560.2004.
- Mellies JL, Elliott SJ, Sperandio V, Donnenberg MS, Kaper JB. The Per regulon of enteropathogenic Escherichia coli : identification of a regulatory cascade and a novel transcriptional activator, the locus of enterocyte effacement (LEE)-encoded regulator (Ler). Mol Microbiol. 1999;33(2):296–306. doi:10.1046/j.1365-2958.1999.01473.x.
- Elliott SJ, Sperandio V, Girón JA, Shin S, Mellies JL, Wainwright L, Hutcheson SW, McDaniel TK, Kaper JB. The Locus of Enterocyte Effacement (LEE)-encoded regulator controls expression of both LEE- and non-LEE-encoded virulence factors in enteropathogenic and enterohemorrhagic Escherichia coli. Infect Immun. 2000;68(11):6115–6126. doi:10.1128/IAI.68.11.6115-6126.2000.
- Waters LS, Storz G. Regulatory RNAs in bacteria. Cell. 2009;136(4):615–628. doi:10.1016/j.cell.2009.01.043.
- Tree JJ, Granneman S, McAteer SP, Tollervey D, Gally DL. Identification of bacteriophage-encoded anti-sRNAs in pathogenic Escherichia coli. Mol Cell. 2014;55(2):199–213. doi:10.1016/j.molcel.2014.05.006.
- Sudo N, Soma A, Muto A, Iyoda S, Suh M, Kurihara N, Abe H, Tobe T, Ogura Y, Hayashi T, et al. A novel small regulatory RNA enhances cell motility in enterohemorrhagic Escherichia coli. J Gen Appl Microbiol. 2014;60(1):44–50. doi:10.2323/jgam.60.44.
- Feng P, Lampel KA, Karch H, Whittam TS. Genotypic and phenotypic changes in the emergence of Escherichia coli O157:H7. J Infect Dis. 1998;177(6):1750–1753. doi:10.1086/517438.
- Wick LM, Qi W, Lacher DW, Whittam TS. Evolution of genomic content in the stepwise emergence of Escherichia coli O157:H7. J Bacteriol. 2005;187(5):1783–1791. doi:10.1128/JB.187.5.1783-1791.2005.
- Ho NK, Henry AC, Johnson-Henry K, Sherman PM. Pathogenicity, host responses and implications for management of enterohemorrhagic Escherichia coli O157:H7 infection. Can J Gastroenterol. 2013;27(5):281–285. doi:10.1155/2013/138673.
- Yang B, Wang S, Huang J, Yin Z, Jiang L, Hou W, Li X, Feng L. Transcriptional activator GmrA, encoded in genomic island OI-29, controls the motility of enterohemorrhagic Escherichia coli O157:H7. Front Microbiol. 2018;9:338. doi:10.3389/fmicb.2018.00338.
- Labarca JA, McDonald LC, Pinto ME, Palavecino E, González P, Cona E. Food-related illness and death in the United States. Emerg Infect Dis. 1999;5:607–625. doi:10.3201/eid0505.990502.
- Lim JY, Yoon J, Hovde CJ. A brief overview of Escherichia coli O157:H7 and its plasmid O157. J Microbiol Biotechnol. 2010;20(1):5–14. doi:10.4014/jmb.0908.08007.
- Zhang X, McDaniel AD, Keusch GT, Waldor MK, Wolf LE, Acheson DWK. Quinolone antibiotics induce Shiga toxin-encoding bacteriophages, toxin production, and death in mice. J Infect Dis. 2000;181(2):664–670. doi:10.1086/315239.