ABSTRACT
The intestinal microbiota is recognized to play a role in the defense against infection, but conversely also acts as a reservoir for potentially pathogenic organisms. Disruption to the microbiome can increase the risk of invasive infection from these organisms; therefore, strategies to restore the composition of the gut microbiota are a potential strategy of key interest to mitigate this risk. Fecal (or Intestinal) Microbiota Transplantation (FMT/IMT), is the administration of minimally manipulated screened healthy donor stool to an affected recipient, and remains the major ‘whole microbiome’ therapeutic approach at present. Driven by the marked success of using FMT in the treatment of recurrent Clostridioides difficile infection, the potential use of FMT in treating other infectious diseases is an area of active research. In this review, we discuss key examples of this treatment based on recent findings relating to the interplay between microbiota and infection, and potential further exploitations of FMT/IMT.
Introduction
The intestinal microbiome has an important function in the defense against infectious diseases. This defensive system includes a consortium of phylogenetically diverse commensal microbes, including bacteria and other components. Colonization resistance is the term used to describe the way in which the microbiome operates both directly and indirectly to prevent colonization and invasive infection from pathogens, as well as to provide immune regulationCitation1.
One such direct route by which members of the intestinal microbiome may contribute to colonization resistance is through the production of bacteriocins/antimicrobial peptides (AMPs) and other proteins by commensal bacteria that may kill pathobionts and other competitors through different mechanisms, including attack on bacterial cell walls.Citation2 As an example of such an AMP, Type VI secretion system (T6SS) is a protein translocation complex secreted by members of the Bacteroidetes that has wide-spanning functions in killing and reducing the function and colonization ability of invading pathogens.Citation3 An alternative direct route is the ability of commensal bacteria to act in competition with pathogens for resources and niches, e.g. indigenous E. coli competing with pathogenic E. coli 0157 for the amino acid proline (which it can exploit to promote growth);Citation4 in addition, E. coli Nissle 1917 is able to compete with Shigella and limit its ability to cause invasive disease within the gut wall.Citation5 As an additional route, gut microbial metabolites may also directly impact upon the life cycle of pathogenic bacteria, including bile acids, tryptophan-based metabolites, and short chain fatty acids (SCFAs); SCFAs are by-products of bacterial fermentation from nondigestible carbohydrates, and can induce production of AMPsCitation6 and inhibit growth and fitness of pathogens, both directlyCitation7 and via routes including intracellular acidification.Citation8
Indirect mechanisms of colonization resistance include microbiome-mediated regulation of the integrity of the gut barrier function to prevent penetration/ translocation of potential pathogens.Citation9 Mucins are glycoproteins which act to protect the gut barrier against inflammation and colitis.Citation10 Pathogens such as Clostridioides difficile are recognized to decrease the level of the major intestinal mucin, muc2;Citation11 conversely, the commensal bacterial species, Bifidobacterium longum, restores growth of mucin.Citation12
Modulation of innate and adaptive immune cells to enhance mucosal immunity is also an important role of microbially-secreted metabolites and microbial-associated molecular patterns (MAMPs).Citation13–15 Toll-like receptors maintain intestinal homeostasis via their interaction with commensal bacteria.Citation16 SCFAs including butyrate have a role in providing an energy source for intestinal epithelial cells as well as influencing T helper cell responses.Citation17 In the presence of commensal bacteria, dendritic cells selectively induce immunoglobulin A (IgA), which also has an important immune function in prevention against invasive disease.Citation18
Perturbation of the intestinal microbiota can be driven by factors such as medications (including antibiotics, opioids, immunosuppressive agents, and chemotherapeutics), diet, surgery, host immune status, and comorbid conditions.Citation19 Such disruption of the microbiota not only alters its composition, but additionally may reduce the protective functions that it provides, including colonization resistance. Microbiome disruption and loss of colonization resistance is recognized to increase the risk of pathogens causing invasive disease and aberrant immune responses.
From a clinical viewpoint, manipulation of the gut microbiome to counter this perturbation and restore premorbid microbiome functionality is a relatively novel approach to reinstate colonization resistance, and may be a strategy that could be exploited for the treatment of particular infectious diseases; such new approaches are of particular pertinence and interest in an era of rising antimicrobial resistance. Gut microbiome manipulation strategies that have been explored encompass several modalities, such as prebiotics, probiotics, phage therapy, dietary manipulation, and fecal (or intestinal) microbiota transplantation (FMT).Citation20 There are two particular attractions about FMT as an approach as a ‘microbiome therapeutic.’ Firstly, from a theoretical perspective, this is a ‘whole microbiome’ approach, attempting to rest and restore both the entire composition and functionality of an ecological community. Secondly, from a clinical perspective, there is already sound evidence from the scenario of recurrent C. difficile infection (rCDI) that this approach may be highly effective and overall safe. In this review, we will discuss the rationale and utility of FMT in a range of infectious diseases and potential further applications.
Fecal (or intestinal) microbiota transplantation (FMT/IMT)
Overview
Fecal microbiota transplantation (FMT; also known as ‘intestinal microbiota transplantation (IMT)’;Citation21 see Supplementary Material) is the transfer of screened healthy donor stool to a recipient’s gastrointestinal tract via routes including nasogastric tube, enema, colonoscopy or capsules. The express aim of the procedure is manipulation of an affected intestinal microbiome to restore premorbid microbiome composition and function, as well aiding recovery of host-microbiome interactions.Citation22 Importantly, good tolerability of the procedure has been seen in immunocompromised patients.Citation23 FMT administration should strongly adhere to international guidelines to ensure donor blood and stool are screened for potentially transmissible pathogens.Citation24–26 A fatality from an ESBL–producing Escherichia coli bacteremia transmitted from FMT donor stool in the United States has been previously reported after transmission to two patients that was not screened for ESBL producing organisms.Citation27 Additionally, systemic infection from Shiga toxin–producing Escherichia coli (STEC) from a single donor to seven patients has also been reported.Citation28 These recent complications have prompted the Food and Drug Administration to issue additional warnings regarding donor testing and quarantine.Citation29 However, reassuringly, no significant delayed complications or adverse effects related to infections have been described in longitudinal studies looking at long term follow up of patients who have received FMT administration.Citation30–32
C. difficile infection (CDI) is a healthcare associated cause of diarrhea, precipitated by the use of antibiotics, and rCDI carries a significantly higher mortality than a single occurrence.Citation33 FMT has been seen to confer a high success rate in the treatment of rCDI.Citation34 The success in treatment of rCDI has led to a greater understanding of the wide interplay between the intestinal microbiome and defense against invading pathobionts and the role that FMT can play to restore and protect against invasive infection.Citation35 The exploration into the mechanisms that contribute to the success of FMT in this field has led to the potential role of FMT to be explored in the treatment or prevention of other diseases with a link to the intestinal microbiome, including other infections. There currently exists an urgent need to seek non-antimicrobial options to address infectious diseases due to the global epidemic of antimicrobial resistance. Although life-saving, antimicrobials impact on the function of the intestinal microbiome and the subsequent long-term health consequences of their use is also increasingly being recognized, therefore FMT as a modality to restore the body’s own protection against invasive infection is of great interest. provides an overview of potential targets of FMT in management of infections and summarizes the human intervention studies to date (animal studies related to the potential utility of fecal transplantation in infectious diseases have been recently comprehensively reviewed elsewhere in this journal,Citation90 and are therefore not reviewed further here).
Table 1. Outcomes of studies utilizing fecal (or intestinal) microbiota transplantation in the treatment of human infectious diseases
Abbreviations: allo-HSCT – allogeneic hematopoietic stem-cell transplantation, COVID-19 – coronavirus disease 2019, CPE – carbapenemase-producing Enterobacterales, CRE – carbapenem-resistant Enterobacterales, ESBL – extended-spectrum beta-lactamase, ESBL-E – extended-spectrum beta-lactamase Enterobacterales, FMT – Fecal (or Intestinal) Microbiota Transplantation, HBeAg – hepatitis B virus e-antigen, HBsAg – hepatitis B surface antigen, HIV – human immunodeficiency virus, HSCT – hematopoietic stem-cell transplantation, MDR – multidrug-resistant, MDRO – multidrug-resistant organism, MRSA – methicillin-resistant Staphylococcus aureus, n/a – not available, NDM – New Delhi metallo-beta-lactamase 1, No. – number, rCDI – recurrent Clostridioides difficile infection, UTI – urinary tract infection, VRE – vancomycin-resistant Enterococci.
Potential mechanisms of action of FMT/IMT
In the best-studied application of FMT to infectious disease – rCDI – it has been shown consistently that successful FMT is associated with the rapid and sustained restoration of a gut microbiome with high diversity and taxonomic profile similar to that of healthy donors.Citation91 A relatively consistent finding between both CDI and non-CDI FMT studies is that high donor microbiota diversity and/or enrichment in particular commensal bacteria appear to be associated with FMT success.Citation92 Studies in which either commensal bacteria cultured from healthy stool donorsCitation93,Citation94 or spores derived from alcohol-shocked donor stoolCitation95 have been given as alternatives to conventional FMT in rCDI patients support the concept that transfer of commensal bacteria from donor to recipient is a central component of the efficacy of FMT, at least in this setting. However, the further demonstration in a pilot study that sterile, filtered FMT may have comparable efficacy to conventional FMT in the treatment of rCDICitation96 suggests that soluble components within FMT – including metabolites, microbial proteins, and/or bacteriophages and other nonbacterial microbiome components – may also be key mediators to the efficacy of FMT.
A summary of both established and proposed mechanisms of FMT is presented in ; many studies of such potential mechanisms have focused upon whether FMT may restore aspects of colonization resistance. For instance, the impact of FMT upon gut microbial metabolites has been extensively investigated.Citation97 After FMT for rCDI, there is restoration of a range of SCFAs within the gut from very low levels up to levels similar to healthy donors;Citation98 this includes the five carbon SCFA, valerate, which directly limits the growth of C. difficile.Citation7 In addition, successful FMT is associated with the restoration of microbial bile salt hydrolases and an associated recovery of the premorbid gut bile acid milieu, removing bile acids which act as potential germination triggers (such as taurocholic acid) and restoring secondary bile acids which limit the growth of C. difficile.Citation99 Such changes in gut microbial metabolites may act beneficially beyond just a direct effect upon a specific gut pathogen itself, and impact upon host responses more generally; for instance, FMT-related changes in gut bile acid profiles have also been associated with altered farnesoid X receptor-pathway signaling,Citation100 and the secondary bile acids that are enriched post-FMT are associated with an impact upon regulatory T cells.Citation101
Figure 1. Uses of Fecal (or Intestinal) Microbiota Transplantation in the field of Infectious Diseases. Figure created with BioRender.com.
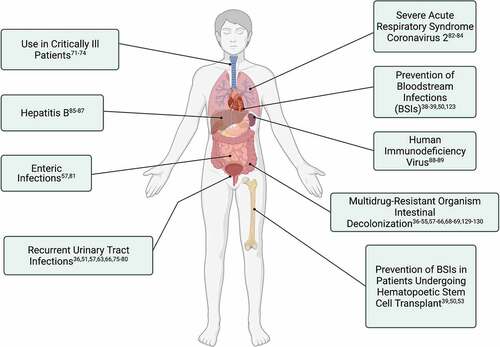
Figure 2. Proposed mechanisms of efficacy of fecal (or intestinal) microbiota transplantation in treating infectious diseases.Abbreviations: FMT: fecal microbiota transplant; FXR: farnesoid X receptor; IL: interleukin; IMT: intestinal microbiota transplant;miRNA: microRNA; SCFA: short chain fatty acids. Figure created with BioRender.com.
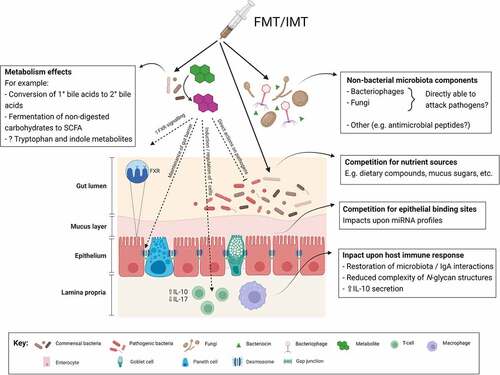
A number of studies have described FMT-related changes in gut bacteriophage or fungal profiles, or defined profiles that predict treatment success, although these specific profiles are heterogeneous between conditions. For instance, while low relative abundance of Caudovirales bacteriophages in the gut predicts response to FMT in both patients with CDI and those with ulcerative colitis (UC),Citation102–105 low gut levels of Candida albicans is associated with successful FMT in CDI patientsCitation106 but failure of FMT in UC.Citation107 Given the established role of the virome in colonization resistanceCitation108 – including a role for bacteriophages in lysing infected cells and reducing bacterial fitnessCitation109 – there is a clear rationale as to why these changes in bacteriophage profiles may contribute to the efficacy of FMT.
FMT-related changes in host immune responses have also been increasingly well-defined; for instance, FMT for rCDI has been associated with restoration of gut bacteria-IgA interactionsCitation110 and may even reverse a CDI-related immunosenescent phenotype through its impact upon T cell repertoires.Citation111 Both mouse and early human studies have associated successful FMT with increased interleukin-10 production by innate and adaptive immune cells, reduced interleukin-17 production, and reduced ability of macrophages, monocytes and dendritic cells to present MHCII-dependent bacterial antigens to colonic T cells.Citation112,Citation113 FMT-related changes in the gut microbiome have also been associated with changes in circulating and intestinal tissue microRNAsCitation114 and reduction in complexity of serum N-glycan profiles toward that found in healthy donors,Citation115 providing a potential link between the gut microbiome and epigenetic changes that may affect several aspects of host physiology, immune and otherwise.
There are grounds for expecting that FMT may also restore other aspects of colonization resistance, although there are limited data from human studies at present. For instance, FMT has been demonstrated to transfer bacteriocins in piglets,Citation116 and commensal bacteria in FMT outcompete C. difficile for proline as an energy source in the gut of a mouse CDI model;Citation117 however, comparable studies have not been published using human samples at present.
While stool derived from almost any donor who passes screening protocols appears to work effectively in FMT to treat recurrent CDI patients, experience of FMT in non-CDI conditions demonstrates much more heterogeneity in response overall.Citation92 In addition to exploring microbiota ‘signatures’ of donors or recipients that may predict response to FMT, a further focus of research is investigation of microbiota functions that may also be predictive. Other potentially relevant factors relating to donors and recipients within this scenario may include genetics, immune status, and clinical factors (e.g. coexisting medications). Factors including modality used to prepare FMT, use of any gut preparation prior to the procedure and/or diet of donor and recipient may also be relevant to consider.Citation92
Potential clinical applications of IMT/FMT in infectious diseases:
Multidrug-resistant organism (MDRO) decolonization
The intestinal microbiome is recognized to act as a reservoir for pathogens that carry antimicrobial resistant genes (ARGs), the collection of which is known as the resistome.Citation118 Selection pressure from antimicrobials increases the genetic size of the resistome.Citation119 Multidrug-resistant organisms (MDROs) are defined as bacteria resistant to more than three classes of antibiotics.Citation120 Resistant ESKAPE pathogens that all colonise the intestine (Enterococcus faecium, Staphylococcus aureus, Klebsiella pneumoniae, Acinetobacter baumannii, Pseudomonas aeruginosa, and Enterobacter species) have been given priority status for new therapy development by the World Health Organization.Citation121 The intestinal niche of MDROs has health implications to the host, as invasive infection can occur after translocation across the gut barrier or fecal contamination of other body sites. Infections from MDROs have poorer outcomes than infections sensitive to first line therapy, due to the poorer efficacy and worse toxicity of second line therapy, as well as the increased cost of these agentsCitation122 and this is of particular significance in immunocompromised patients where mortality with MDRO infections are significantly higher.Citation123 Attempts to stop the spread of MDROs includes use of antimicrobial stewardship programs and hospital infection control procedures; however, more targeted therapies such as probiotics and selective digestive decontamination have had variable outcomes.Citation124
Mouse models have provided proof of concept of the impact of FMT upon intestinal MDRO dynamics. For instance, administration to mice of FMT containing the commensal bacteria Barnesiella was associated with intestinal clearance of vancomycin-resistant enterococci (VRE);Citation125 in addition, a four-strained consortium of commensal bacteria containing Blautia producta reduced susceptibility to VRE in a rodent model, with this protection attributable to production of a lantibiotic.Citation126 Studies of FMT in the treatment of rCDI in humans have demonstrated a reduction in diversity and number of ARGs post-FMT;Citation55,Citation58,Citation60,Citation127 more recently, a similar finding has also been described in patients being treated with FMT for liver cirrhosis.Citation128
Collectively, this evidence has led to the exploration of the use of FMT as a tool to “decolonize” the intestine to eradicate carriage of MDROs, which has been reported in a range of case reports, case series, and a single randomised trial (see ).Citation36–46,Citation48–52,Citation54,Citation56,Citation61–66,Citation68,Citation69,Citation129,Citation130 One of the perceived benefits of using FMT for this purpose has been that patients pose a lower nosocomial risk to others in a healthcare setting. Results from studies looking at intestinal decolonization of MDROs following FMT have been highly variable, in part due to the heterogeneity of the study design, patient cohorts, and FMT administration protocols.Citation131 Biliński and colleagues reported decolonization rates or 75% in 20 patients,Citation41 and Saïdani and colleagues also reported similarly high decolonization rates of 80% in 10 patients at 14 days (with a decolonization rate of 10% in a comparator arm). However, conversely, Davido and coworkers reported decolonization rate in eight patients as low as 37.5% after three months;Citation43 in comparison, spontaneous decolonization rates for intestinal MDROs are reported as high as 48.2% after 90 days.Citation132 The only reported randomized control trial (RCT) to date in this area demonstrated a non-significant decrease in rates of ESBL-E and CPE carriage in FMT-treated patients compared to the control group; in part, this was attributed to the low number of patients recruited and early termination of the trial by participants due to diarrhea.Citation52 Although the reduction in the burden of ARG carriage in the gut has been described, the role of FMT as an infection control or an intestinal decolonization measure is still uncertain.
Prevention of bloodstream infections (BSIs) in specific populations
In vulnerable populations with a disrupted gut microbiota (e.g. perturbed in terms of taxonomic profile or diversity of commensal bacteria), the risk of bloodstream infections (BSIs) has been noted to be increased.Citation133 Studies reporting outcomes on the impact of FMT upon rCDI have reported a decrease in bloodstream infections (BSIs) post-FMT.Citation134 Additionally, in studies investigating the impact of FMT in MDRO-colonized patients, a reduction in both MDRO-related and all-cause BSIs post-FMT has also been observed.Citation38,Citation50 The impact of FMT on the reduction of MDRO infections is currently being studied in two clinical trials, one looking specifically at patients with renal transplants (NCT02312986, NCT02922816).
In hematopoietic stem cell transplant (HSCT) patients – where preceding chemotherapyCitation135 and the frequent need for broad-spectrum antibiotic therapyCitation136 impacts the intestinal microbiome – lower intestinal microbial diversity is seen to correlate with worse survival post-HSCT.Citation137 An associated increased risk of BSIs in HSCT patients with intestinal domination with Gram negative organisms is also seen, and increased mortality in those colonized with MDROs.Citation138–141 In terms of infection outcomes, studies looking at the use of FMT in HSCT patients have noted a reduction in days of fever,Citation142 and reduction in number of BSIs in HSCT post-FMT.Citation39,Citation50
Prevention of invasive disease in the Intensive Care Unit (ICU) setting using FMT has also been explored. Critical illness is recognized to dramatically impact the ecology of the microbial communities within the gut.Citation143 The causes for disruption within the intensive care setting include hypoxic injury, enteral feeding, use of medications (such as proton pump inhibitors, antibiotics, and vasopressors), and intestinal dysmotility, collectively resulting in a reduction in diversity and beneficial functional output of commensal bacteria.Citation144 These changes within the gut microbiome are associated with an increase in infectious complications and mortality in patients with severe systemic inflammatory response syndrome (SIRS).Citation145 Mouse models have reported improved survival in septic mice following FMT with an improvement in the gut barrier function.Citation146,Citation147 To date, several case reports have noted a decrease in SIRS response including fever in patients in the intensive care setting following FMT;Citation72–74 in addition, a case series of 18 ICU patients with antibiotic-associated diarrhea were treated with FMT, with full resolution of symptoms occurring in eight out of eighteen patients.Citation71
Future targets to use FMT as a safe and cost-effective method to prevent BSIs could be aimed patient cohorts who are recognized to be at particular risk from their colonizing MDRO (utilizing a scoring system such as the INCREMENT scoreCitation148), or cohorts recognized to be at increased risk of BSIs due to the risk of microbiome disruption related to preceding drug therapy (i.e. chemotherapy or prolonged antibiotics) or chronic disease.
Recurrent urinary tract infections (rUTIs):
An important subgroup explored in the prevention of invasive infection is that of rUTIs. Non-antimicrobial options to treat rUTIs have limited evidence,Citation149 and the risk of antimicrobial resistance increases with recurrent courses of anti-infectives.Citation150 Increased abundance of uropathogenic organisms in the gut has been seen to be a direct risk factor for occurrence of UTIs with the same organism;Citation151 therefore, re-establishment of the composition of the intestinal microbiome to restore colonization resistance and reduce the burden of invasive infection has been explored using FMT.
Patients who were treated with FMT for rCDI were also noted to have a reduction in their occurrence of rUTIs.Citation36,Citation77,Citation79,Citation80 Three case reports also describe use of FMT specifically to attempt to treat rUTIs, where no further UTIs were noted in patients after 8–12 weeks.Citation57,Citation63,Citation76 FMT has also been used specifically to attempt to prevent rUTIs in renal transplant patients. These patients are recognized to have a lower intestinal microbial diversity than healthy controlsCitation152 and rUTIs are recognized to impact on the kidney graft function in these patients;Citation153 as such, the restoration of the intestinal microbiota with FMT and prevention of invasive disease in this cohort could be of prognostic value. Two case reports and a case series have all reported a reduction in the occurrence of UTIs in renal transplant patients post-FMT despite no change in the risk factors predisposing the patients to recurrent infection.Citation50,Citation51,Citation75
Bacterial enteric infections
As seen in rCDI, the intestinal microbiota is the first line of defense against enteric infections, and a deeper understanding of the role of the gut microbiota has arisen from studying the relationship between these pathogens and commensal bacteria. Examples include the commensal Blautia obeum, which has been demonstrated to block infection from Vibrio cholera via hydrolysis of bile acids.Citation154 Another commensal bacterium, Clostridium scindens, has been recognized to possess antimicrobial features against infection from Entamoeba histolytica and C. difficile via the biotransformation of primary to secondary bile acids.Citation155,Citation156 Mouse studies have shown that FMT reduced intestinal bacterial load of Campylobacter jejuni, a common cause of foodborne gastrointestinal infection, and additionally lowered cell damage caused by the bacteria, as well as susceptibility to the disease.Citation157,Citation158 In humans, successful eradication of chronic Salmonella infection after FMT has been reported twice in the literature, firstly in two patients with Salmonella infection alongside prolonged carbapenem usageCitation81 and secondly as a modality to eradicate asymptomatic chronic Salmonella carriage in two patients where carriage had an impact on their occupation in the food industry.Citation57 The exploitation of FMT to treat enteric infections in humans has not been fully explored to date; however, the restoration of microbiota-derived metabolites seen in preliminary studies of these infections have also been seen in the mechanisms explored as contributors to the success of FMT for rCDI.Citation35 This modality therefore may have either a prophylactic role or use in chronic or relapsing infections where long term antibiotic use has a detrimental effect on the host.
Viral infections:
The microbial communities in the intestine and respiratory system have a shared mucosal immune system that may be referred to as the ‘gut-lung axis,’ suggesting that there may be a role for FMT as a preventative or supportive measure in respiratory disorders.Citation159 Infection with respiratory viruses such as respiratory syncytial virus and influenza have been noted in mouse models to result in transient changes in intestinal microbiota composition, with an increase in Bacteroidetes and a decrease in Firmicutes phyla abundance as well as increased levels of lipocalin-2 concentrations, suggesting inflammation, posing an increased potential risk of subsequent bacterial infection.Citation160 A recent study indicated that patients with a higher load of severe acute respiratory syndrome coronavirus 2 (SARS-CoV-2) RNA in the stool had lower levels of commensal bacteria that produce protective metabolites such as short-chain fatty acids and tryptophan and higher levels of pathobionts such as Collinsella aerofaciens and Morganella morganii.Citation161 A report on patients treated with FMT for rCDI during the coronavirus disease 2019 (COVID-19) pandemic noted full resolution from COVID-19 in two patients with concurrent CDI and COVID-19 infection.Citation83 A further case series of two patients treated with FMT for rCDI with coexisting SARS-CoV-2 infection suggested that FMT may have had a role in the shortened recovery time seen in these patients.Citation82 Another trial looked at administering FMT to patients one month following hospital discharge post-COVID-19, and noted improvement in both gastrointestinal symptoms and in microbial diversity.Citation84
A similar ‘gut-liver axis’ is also purported to exist. Hepatitis B-infected patients with chronic carriage or decompensated liver cirrhosis are recognized to have a lower diversity of commensal bacteria and lower levels of microbial metabolites than healthy controls or those with asymptomatic hepatitis B carriage, which suggests that the gut microbiota may be responsible for modulating the effects of the virus on the liver.Citation162,Citation163 Response to treatment for hepatitis B is measured serologically using hepatitis B e-antigen (HBeAg) and hepatitis B surface antigen (HBsAg), where seroconversion of HBsAg is the ideal endpoint. Changes in HBeAg post-FMT has been seen in two case series: one with five patients (where decline in HBeAg was seen without any cases of seroconversion) and another study of twelve patients (where two patients had loss of presence of HBeAg, but no loss of HBsAg).Citation85,Citation86 A further case series reported HBsAg decline following FMT in HBeAg negative patients with a shift in the microbiota composition.Citation87 Similarly, in human immunodeficiency virus (HIV) infection, disturbance of the microbiome is recognized to be related to virus response; the depletion of CD4+ cells is seen first in gut-associated lymphoid tissue, and this impacts on gut barrier function and Th17 cells function.Citation164–166 A study of FMT administered to six macaques infected with simian immunodeficiency virus showed some immune restoration with significant increases in the number of peripheral Th17 and Th22 cells and reduced CD4 + T cell activation in gastrointestinal tissues, indicating some potential to enhance the immune system via T cell integrity. In humans, an early study looking at the use of FMT for rCDI in a severely immunocompromised patient noted an improvement in CD4+ counts as well as full recovery from rCDI.Citation167 Sustained increase in microbial diversity similar to donor stool for up to 24 weeks was seen in another study of FMT administered to six HIV-infected patients – however, no changes were seen in inflammatory markers.Citation89 A more recent study administered weekly oral capsules of FMT for eight weeks to 14 HIV positive patients and followed them up for 48 weeks; researchers described a sustained increase in bacterial diversity and reduction in intestinal fatty acid binding protein, which is recognized as a marker of gut barrier dysfunction.Citation88 The clinical impact of this improvement in diversity is yet to be fully established in HIV patients. In terms of viral enteric infections, a case series of non-rCDI indications for FMT reported on a case attempting to trial FMT on a patient with chronic norovirus infection, without any change in their symptoms following FMT.Citation57
Conclusion:
As the understanding grows of the role of the intestinal microbiota in both the defense against infection but also as a potential reservoir of pathogens, so our knowledge also expands regarding postulated strategies for reversing a perturbed microbiome. The role for FMT in protecting against and treating infection appears to be most valuable in vulnerable cohorts and the scenario of chronic or relapsing infection; however, a limitation is that current evidence for a number of potential applications are at present derived from rodent studies only or small, early phase human studies. Although the administration of FMT is becoming more refined in terms of the availability and ease of capsulized administration, with further understanding of mechanisms of actions, refined bacterial consortiums that could potentially be personalized to target specific organisms would be of greater value, and would overcome the potential risks identified with FMT administration.Citation168 Manipulation of the microbiota is an attractive target for infections due to the global effort to reduce the use of antibiotics and the worldwide antimicrobial resistance crisis; however, large scale RCTs are needed to confirm the true utility in each of these different conditions.
Author contribution
All authors contributed to the idea of the commentary and writing the manuscript. All authors approved the final version for publication.
Supplemental Material
Download MS Word (29.5 KB)Disclosure statement
Benjamin H. Mullish reports personal fees from Finch Therapeutics Group and Ferring Pharmaceuticals, outside the submitted work. Julian R. Marchesi reports consultancy fees from Cultech Ltd., Flerie Invest and Enterobiotix Ltd., outside the submitted work. No other potential competing interests were reported.).
Data availability statement
Data sharing is not applicable to this article as no new data were created or analyzed in this study.
Supplementary material
Supplemental data for this article can be accessed on the publisher’s website.
Additional information
Funding
References
- Keith JW, Pamer EG. Enlisting commensal microbes to resist antibiotic-resistant pathogens. J Exp Med. 2019;216(1):10–21. doi:10.1084/jem.20180399.
- Mukherjee S, Hooper LV. Antimicrobial Defense of the Intestine. Immunity. 2015;42(1):28–39. doi:10.1016/j.immuni.2014.12.028.
- Russell AB, Wexler AG, Harding BN, Whitney JC, Bohn AJ, Goo YA, Tran BQ, Barry NA, Zheng H, Peterson SB, et al. A type VI secretion-related pathway in bacteroidetes mediates interbacterial antagonism. Cell Host Microbe. 2014;16(2):227–236. doi:10.1016/j.chom.2014.07.007.
- Momose Y, Hirayama K, Itoh K. Competition for proline between indigenous Escherichia coli and E. coli O157:H7 in gnotobiotic mice associated with infant intestinal microbiota and its contribution to the colonization resistance against E. coli O157:H7. Antonie Van Leeuwenhoek. 2008;94(2):165–171. doi:10.1007/s10482-008-9222-6.
- Altenhoefer A, Oswald S, Sonnenborn U, Enders C, Schulze J, Hacker J, Oelschlaeger TA. Recognition of commensal microflora by toll-like receptors is required for intestinal homeostasis. FEMS Immunol Med Microbiol. 2004;40(3):223–229. doi:10.1016/S0928-8244(03)00368-7.
- Zhao Y, Chen F, Wu W, Sun M, Bilotta AJ, Yao S, Xiao Y, Huang X, Eaves-Pyles TD, Golovko G, et al. GPR43 mediates microbiota metabolite SCFA regulation of antimicrobial peptide expression in intestinal epithelial cells via activation of mTOR and STAT3. Mucosal Immunol. 2018;11(3):752–762. doi:10.1038/mi.2017.118.
- McDonald JAK, Mullish BH, Pechlivanis A, Liu Z, Brignardello J, Kao D, Holmes E, Li JV, Clarke TB, Thursz MR, et al. Inhibiting growth of clostridioides difficile by restoring valerate, produced by the intestinal microbiota. Gastroenterology 2018; accessed 2018 Jul 27]. 155(5):1495–1507.e15. https://linkinghub.elsevier.com/retrieve/pii/S0016508518347711
- Sorbara MT, Dubin K, Littmann ER, Moody TU, Fontana E, Seok R, Leiner IM, Taur Y, Peled JU, Van Den Brink MRM, et al. Inhibiting antibiotic-resistant Enterobacteriaceae by microbiota-mediated intracellular acidification. J Exp Med. 2019;216(1):84–98. doi:10.1084/jem.20181639.
- Kim S, Covington A, Pamer EG. The intestinal microbiota: antibiotics, colonization resistance, and enteric pathogens. Immunol Rev. 2017;279(1):90–105. doi:10.1111/imr.12563.
- Petersson J, Schreiber O, Hansson GC, Gendler SJ, Velcich A, Lundberg JO, Roos S, Holm L, Phillipson M. Importance and regulation of the colonic mucus barrier in a mouse model of colitis. Am J Physiol Gastrointest Liver Physiol. 2011;300(2):G327–33. doi:10.1152/ajpgi.00422.2010.
- Engevik MA, Yacyshyn MB, Engevik KA, Wang J, Darien B, Hassett DJ, Yacyshyn BR, Worrell RT. Human Clostridium difficile infection: altered mucus production and composition. Am J Physiol Gastrointest Liver Physiol. 2015;308(6):G510–24. doi:10.1152/ajpgi.00091.2014.
- Schroeder BO, Birchenough GMH, Ståhlman M, Arike L, Johansson ME, Hansson ,GC, Bäckhed F. Bifidobacteria or fiber protects against diet-induced microbiota-mediated colonic mucus deterioration. Cell Host Microbe. 2018;23(1):27–40.e7. doi:10.1016/j.chom.2017.11.004.
- Pickard JM, Zeng MY, Caruso R, Núñez G. Gut microbiota: role in pathogen colonization, immune responses, and inflammatory disease. Immunol Rev. 2017;279(1):70–89. doi:10.1111/imr.12567.
- Wuethrich I, Pelzer BW, Khodamoradi Y, Vehreschild MJGT. The role of the human gut microbiota in colonization and infection with multidrug-resistant bacteria. Gut Microbes. 2021;13(1):1–13. doi:10.1080/19490976.2021.1911279.
- Le Guern R, Stabler S, Gosset P, Pichavant M, Grandjean T, Faure E, Karaca Y, Faure K, Kipnis E, Dessein R. Colonization resistance against multi-drug-resistant bacteria: a narrative review. J Hosp Infect. 2021;118:48–58. doi:10.1016/j.jhin.2021.09.001.
- Rakoff-Nahoum S, Paglino J, Eslami-Varzaneh F, Edberg S, Medzhitov R. Recognition of commensal microflora by toll-like receptors is required for intestinal homeostasis. Cell. 2004;118(2):229–241. doi:10.1016/j.cell.2004.07.002.
- Donohoe DR, Garge N, Zhang X, Sun W, O’Connell TM, Bunger MK, Bultman SJ. The microbiome and butyrate regulate energy metabolism and autophagy in the mammalian colon. Cell Metab. 2011;13(5):517–526. doi:10.1016/j.cmet.2011.02.018.
- Macpherson AJ, Uhr T. Induction of protective IgA by intestinal dendritic cells carrying commensal bacteria. Science. 2004;303(5664):1662–1665. doi:10.1126/science.1091334.
- Sommer F, Bäckhed F. The gut microbiota-masters of host development and physiology. Nat Rev Microbiol. 2013;11(4):227–238. doi:10.1038/nrmicro2974.
- Libertucci J, Young VB. The role of the microbiota in infectious diseases. Nat Microbiol. 2019;4(1):35–45. doi:10.1038/s41564-018-0278-4.
- Craven LJ, McIlroy JR, Mullish BH, Marchesi JR. Letter: intestinal microbiota transfer—updating the nomenclature to increase acceptability. Aliment Pharmacol Ther. 2020;52(10):1622–1623. doi:10.1111/apt.16109.
- Baunwall SMD, Lee MM, Eriksen MK, Mullish BH, Marchesi JR, Dahlerup JF, Hvas CL. Faecal microbiota transplantation for recurrent Clostridioides difficile infection: an updated systematic review and meta-analysis. EClinicalMedicine [Internet]. 2020;[[accessed 2021 Feb 9]]. 29–30. https://pubmed.ncbi.nlm.nih.gov/33437951/
- Shogbesan O, Poudel DR, Victor S, Jehangir A, Fadahunsi O, Shogbesan G, Donato A. A systematic review of the efficacy and safety of fecal microbiota transplant for clostridium difficile infection in immunocompromised patients [Internet]. Can J Gastroenterol Hepatol. 2018; accessed 2019 Jul 18. 2018:1–10. https://www.hindawi.com/journals/cjgh/2018/1394379/
- Keller JJ, Ooijevaar RE, Hvas CL, Terveer EM, Lieberknecht SC, Högenauer C, Arkkila P, Sokol H, Gridnyev O, Mégraud F, et al. A standardised model for stool banking for faecal microbiota transplantation: a consensus report from a multidisciplinary UEG working group. United Eur Gastroenterol J [Internet]. 2021; [accessed 2021 May 5]. 9. https://pubmed.ncbi.nlm.nih.gov/33151137/
- Baunwall SMD, Terveer EM, Dahlerup JF, Erikstrup C, Arkkila P, Vehreschild MJ, Ianiro G, Gasbarrini A, Sokol H, Kump PK, et al. The use of faecal microbiota transplantation (FMT) in Europe: a Europe-wide survey. Lancet Reg Heal - Eur. 2021;9:100181. doi:10.1016/j.lanepe.2021.100181.
- Mullish BH, Quraishi MN, Segal JP, McCune VL, Baxter M, Marsden GL, Moore DJ, Colville A, Bhala N, Iqbal TH, et al. The use of faecal microbiota transplant as treatment for recurrent or refractory Clostridium difficile infection and other potential indications: Joint British Society of Gastroenterology (BSG) and Healthcare Infection Society (HIS) guidelines. Gut [Internet]. 2018;67(11):1920–1941. doi:10.1136/gutjnl-2018-316818.
- DeFilipp Z, Bloom PP, Soto MT, Mansour MK, Sater MRA, Huntley MH, Turbett S, Chung RT, Chen YB, Hohmann EL. Drug-resistant e. coli bacteremia transmitted by fecal microbiota transplant. N Engl J Med. 2019; [accessed 2019 Nov 16]. 381(21):2043–2050. http://www.ncbi.nlm.nih.gov/pubmed/31665575
- Zellmer C, Sater MRA, Huntley MH, Osman M, Olesen SW, Ramakrishna B. Shiga toxin-producing Escherichia coli transmission via fecal microbiota transplant. Clin Infect Dis. 2021;72(11):E876–80. doi:10.1093/cid/ciaa1486.
- Administration UF and D. Safety alert regarding use of fecal microbiota for transplantation and risk of serious adverse events likely due to transmission of pathogenic organisms | FDA [Internet]. [accessed 2020 Apr 25]. https://www.fda.gov/vaccines-blood-biologics/safety-availability-biologics/safety-alert-regarding-use-fecal-microbiota-transplantation-and-risk-serious-adverse-events-likely
- Ooijevaar RE, van Nood E, Goorhuis A, Terveer EM, van Prehn J, Verspaget HW, van Beurden YH, Dijkgraaf MGW, and Keller JJ. Ten-year follow-up of patients treated with fecal microbiota transplantation for recurrent clostridioides difficile infection from a randomized controlled trial and review of the literature. Microorganisms. 2021 Mar 6;9(3):548. doi: 10.3390/microorganisms9030548.
- Urbonas T, Ianiro G, Gedgaudas R, Sabanas P, Urba M, Kiudelis V, Kiudelis G, Petkevicius V, Vitkauskiene A, Cammarota G, et al. Fecal microbiome transplantation for recurrent clostridioides difficile infection: treatment efficacy, short and long-term follow-up results from consecutive case series. J Gastrointestin Liver Dis. 2021. doi:10.15403/jgld-3800.
- Saha S, Mara K, Pardi DS, Khanna S. Long-term safety of fecal microbiota transplantation for recurrent clostridioides difficile infection. Gastroenterology. 2021;160(6):1961–1969.e3. doi:10.1053/j.gastro.2021.01.010.
- Olsen MA, Yan Y, Reske KA, Zilberberg MD, Dubberke ER. Recurrent clostridium difficile infection is associated with increased mortality. Clin Microbiol Infect. 2015;21(2):164–170. doi:10.1016/j.cmi.2014.08.017.
- van Nood E, Vrieze A, Nieuwdorp M, Fuentes S, Zoetendal EG, de Vos WM, Visser CE, Kuijper EJ, Bartelsman JFWMWM, Tijssen JGPP, et al. Duodenal infusion of donor feces for recurrent clostridium difficile. N Engl J Med [Internet]. 2013;368(5):407–415. doi:10.1056/NEJMoa1205037.
- Khoruts A, Sadowsky MJ. Understanding the mechanisms of faecal microbiota transplantation. Nat Rev Gastroenterol Hepatol [Internet]. 2016; [[accessed 2017 Oct 3]]. 13(9):508–516. http://www.ncbi.nlm.nih.gov/pubmed/27329806
- Aira A, Rubio E, Vergara Gómez A, Fehér C, Casals-Pascual C, González B, Morata L, Rico V, Soriano A. rUTI resolution after FMT for clostridioides difficile infection: a case report. Infect Dis Ther. 2021;10(2):1065–1071. doi:10.1007/s40121-020-00365-8.
- Baron SA, Cassir N, Mékidèche T, Mlaga KD, Brouqui P, Rolain JM. Successful treatment and digestive decolonisation of a patient with osteitis caused by a carbapenemase-producing Klebsiella pneumoniae isolate harbouring both NDM-1 and OXA-48 enzymes. J Glob Antimicrob Resist. 2019;18:225–229. doi:10.1016/j.jgar.2019.06.001.
- Bar-Yoseph H, Carasso S, Shklar S, Korytny A, Even Dar R, Daoud H, Nassar R, Maharshak N, Hussein K, Geffen Y, et al. Oral capsulized fecal microbiota transplantation for eradication of carbapenemase-producing Enterobacteriaceae colonization with a metagenomic perspective. Clin Infect Dis [Internet]. 2020; [[accessed 2021 Feb 12]. https://pubmed.ncbi.nlm.nih.gov/32511695/
- Battipaglia G, Malard F, Rubio MT, Ruggeri A, Mamez AC, Brissot E, Giannotti F, Dulery R, Joly AC, Baylatry MT, et al. Fecal microbiota transplantation before or after allogeneic hematopoietic transplantation in patients with hematologic malignancies carrying multidrug-resistance bacteria. Haematologica. 2019;104(8):1682–1688. doi:10.3324/haematol.2018.198549.
- Biliński J, Grzesiowski P, Muszyński J, Wróblewska M, Mądry K, Robak K, Dzieciątkowski T, Wiktor-Jedrzejczak W, Basak GW. Fecal microbiota transplantation inhibits multidrug-resistant gut pathogens: preliminary report performed in an immunocompromised host. Arch Immunol Ther Exp (Warsz). 2016;64(3):255–258. doi:10.1007/s00005-016-0387-9.
- Bilinski J, Grzesiowski P, Sorensen N, Madry K, Muszynski J, Robak K, Wroblewska M, Dzieciatkowski T, Dulny G, Dwilewicz-Trojaczek J, et al. Fecal microbiota transplantation in patients with blood disorders inhibits gut colonization with antibiotic-resistant bacteria: results of a prospective, single-center study. Clin Infect Dis [Internet] 2017; accessed 2020 Jan 19]. 65(3):364–370. http://academic.oup.com/cid/article/65/3/364/3088486/Fecal-Microbiota-Transplantation-in-Patients-With
- Crum-Cianflone NF, Sullivan E, Ballon-Landa G, Onderdonk AB. Fecal microbiota transplantation and successful resolution of multidrug-resistant-organism colonization. J Clin Microbiol. 2015;53(6):1986–1989. doi:10.1128/JCM.00820-15.
- Davido B, Batista R, Michelon H, Lepainteur M, Bouchand F, Lepeule R, Salomon J, Vittecoq D, Duran C, Escaut L, et al. Is faecal microbiota transplantation an option to eradicate highly drug-resistant enteric bacteria carriage? J Hosp Infect. 2017;95(4):433–437. doi:10.1016/j.jhin.2017.02.001.
- Davido B, Batista R, Fessi H, Michelon H, Escaut L, Lawrence C, Denis M, Perronne C, Salomon J, Dinh A. Fecal microbiota transplantation to eradicate vancomycin-resistant enterococci colonization in case of an outbreak. Med Mal Infect. 2019;49(3):214–218. doi:10.1016/j.medmal.2018.11.002.
- Dias C, Pipa S, Duarte-Ribeiro F, Mota M. Fecal microbiota transplantation as a potential way to eradicate multiresistant microorganisms. IDCases. 2018;12:13. doi:10.1016/j.idcr.2018.02.010.
- Dinh A, Fessi H, Duran C, Batista R, Michelon H, Bouchand F, Lepeule R, Vittecoq D, Escaut L, Sobhani I, et al. Clearance of carbapenem-resistant Enterobacteriaceae vs vancomycin-resistant enterococci carriage after faecal microbiota transplant: a prospective comparative study. J Hosp Infect. 2018;99(4):481–486. doi:10.1016/j.jhin.2018.02.018.
- Eysenbach L, Allegretti JR, Aroniadis O, Brandt L, Donovan D, Fischer M, Grinspan A, Kassam Z, Kelly CR, and Kim C, et al. Clearance of Vancomycin-resistant Enterococcus colonization with fecal microbiota transplantation among patients with recurrent clostridium difficile infection. Open Forum Infectious Diseases. 2016 December ;3(suppl_1):2119. https://doi.org/10.1093/ofid/ofw172.1667
- Freedman A, Eppes S. 1805 use of stool transplant to clear fecal colonization with Carbapenem-Resistant Enterobacteriaceae (CRE): proof of Concept. Open Forum Infect Dis. 2014;1(suppl_1):S65–S65. doi:10.1093/ofid/ofu051.177.
- García-Fernández S, Morosini MI, Cobo M, Foruny JR, López-Sanromán A, Cobo J, Romero J, Cantón R, Del Campo R. Gut eradication of VIM-1 producing ST9 Klebsiella oxytoca after fecal microbiota transplantation for diarrhea caused by a Clostridium difficile hypervirulent R027 strain. Diagn Microbiol Infect Dis. 2016;86(4):470–471. doi:10.1016/j.diagmicrobio.2016.09.004.
- Ghani R, Mullish BH, McDonald JAK, Ghazy A, Williams HRT, Brannigan ET, Mookerjee S, Satta G, Gilchrist M, Duncan N, et al. Disease prevention not decolonization: a model for fecal microbiota transplantation in patients colonized with multidrug-resistant organisms. Clin Infect Dis [Internet]. 2021; accessed [2020 Sep 23].;72(8):1444–1447. doi: 10.1093/cid/ciaa948/5873448.
- Grosen AK, Povlsen JV, Lemming LE, Jørgensen SMD, Dahlerup JF, Hvas CL. Faecal microbiota transplantation eradicated extended-spectrum beta-lactamase-producing Klebsiella pneumoniae from a renal transplant recipient with recurrent urinary tract infections. Case Rep Nephrol Dial. 2019;9(2):102–107. doi:10.1159/000502336.
- Huttner BD, de Lastours V, Wassenberg M, Maharshak N, Mauris A, Galperine T, Zanichelli V, Kapel N, Bellanger A, Olearo F, et al. A 5-day course of oral antibiotics followed by faecal transplantation to eradicate carriage of multidrug-resistant Enterobacteriaceae: a randomized clinical trial. Clin Microbiol Infect. 2019;25(7):830–838. doi:10.1016/j.cmi.2018.12.009.
- Innes AJ, Mullish BH, Fernando F, Adams G, Marchesi JR, Apperley JF, Brannigan E, Davies F, and Pavlů J, et al. Faecal microbiota transplant: a novel biological approach to extensively drug-resistant organism-related non-relapse mortality. Bone Marrow Transplant. 2017;52(10):1452–1454. doi: 10.1038/bmt.2017.151.[accessed 2017 July 17].
- Jang M-O, An JH, Jung S-I, Park K-H. Refractory clostridium difficile infection cured with fecal microbiota transplantation in Vancomycin-resistant Enterococcus colonized patient. Intest Res. 2015;13(1):80. doi:10.5217/ir.2015.13.1.80.
- Jouhten H, Mattila E, Arkkila P, Satokari R. Reduction of antibiotic resistance genes in intestinal microbiota of patients with recurrent clostridium difficile infection after fecal microbiota transplantation. Clin Infect Dis. 2016;63(5):710–711. doi:10.1093/cid/ciw390.
- Lagier JC, Million M, Fournier PE, Brouqui P, Raoult D. Faecal microbiota transplantation for stool decolonization of OXA-48 carbapenemase-producing Klebsiella pneumoniae. J Hosp Infect. 2015;90(2):173–174. doi:10.1016/j.jhin.2015.02.013.
- Lahtinen P, Mattila E, Anttila VJ, Tillonen J, Teittinen M, Nevalainen P, Salminen S, Satokari R, Arkkila P. Faecal microbiota transplantation in patients with Clostridium difficile and significant comorbidities as well as in patients with new indications: a case series. World J Gastroenterol. 2017;23(39):7174–7184. doi:10.3748/wjg.v23.i39.7174.
- Leung V, Vincent C, Edens TJ, Miller M, Manges AR. Antimicrobial resistance gene acquisition and depletion following fecal microbiota transplantation for recurrent clostridium difficile infection. Clin Infect Dis. 2018;66(3):456–459. doi:10.1093/cid/cix821.
- Merli P, Putignani L, Ruggeri A, Del Chierico F, Gargiullo L, Galaverna F, Gaspari S, Pagliara D, Russo A, Pane S, et al. Decolonization of multi-drug resistant bacteria by fecal microbiota transplantation in five pediatric patients before allogeneic hematopoietic stem cell transplantation: gut microbiota profiling, infectious and clinical outcomes. Haematologica. 2020;105(11):2686–2690. doi:10.3324/haematol.2019.244210.
- Millan B, Park H, Hotte N, Mathieu O, Burguiere P, Tompkins TA, Kao D, Madsen KL. Fecal microbial transplants reduce antibiotic-resistant genes in patients with recurrent clostridium difficile infection. Clin Infect Dis. 2016; [accessed 2019 Oct 5]. 62(12):1479–1486. http://www.ncbi.nlm.nih.gov/pubmed/27025836
- Ponte A, Pinho R, Mota M. Fecal microbiota transplantation: is there a role in the eradication of carbapenem-resistant Klebsiella pneumoniae intestinal carriage? Rev Esp Enfermedades Dig. 2017;109:392–393.
- Saïdani N, Lagier JC, Cassir N, Million M, Baron S, Dubourg G, Eldin C, Kerbaj J, Valles C, Raoult D, et al. Faecal microbiota transplantation shortens the colonisation period and allows re-entry of patients carrying carbapenemase-producing bacteria into medical care facilities. Int J Antimicrob Agents. 2019;53(4):355–361. doi:10.1016/j.ijantimicag.2018.11.014.
- Singh R, van Nood E, Nieuwdorp M, van Dam B, ten Berge IJM, Geerlings SE, Bemelman FJ. Donor feces infusion for eradication of extended spectrum beta-Lactamase producing Escherichia coli in a patient with end stage renal disease. Clin Microbiol Infect. 2014;20(11):O977–8. doi:10.1111/1469-0691.12683.
- Christen R, Edmond E, Drancourt M. Fecal microbiota transplantation against intestinal colonization by extended spectrum beta-lactamase producing Enterobacteriaceae: a proof of principle study ISRCTN48328635 ISRCTN. BMC Res Notes. 2018;11(1):11. doi:10.1186/s13104-017-3119-2.
- Sohn KM, Cheon S, Kim YS. Can Fecal Microbiota Transplantation (FMT) eradicate fecal colonization with Vancomycin-resistant Enterococci (VRE)? Infect. Control Hosp Epidemiol. 2016;37(12):1519–1521. doi:10.1017/ice.2016.229.
- Stalenhoef JE, Terveer EM, Knetsch CW, Van’t Hof PJ, Vlasveld IN, Keller JJ, Visser LG, Kuijper EJ. Fecal microbiota transfer for multidrug-resistant gram-negatives: a clinical success combined with microbiological failure. Open Forum Infect Dis. 2017;4(2):ofx047. doi:10.1093/ofid/ofx047.
- Stripling J, Kumar R, Baddley JW, Nellore A, Dixon P, Howard D, Ptacek T, Lefkowitz EJ, Tallaj JA, Benjamin William HJ, et al. Loss of Vancomycin-resistant Enterococcus fecal dominance in an organ transplant patient with clostridium difficile colitis after fecal microbiota transplant. Open Forum Infect Dis. 2015;2(2):ofv078–ofv078. doi:10.1093/ofid/ofv078.
- Su F, Luo Y, Yu J, Shi J, Zhao Y, Yan M, Huang H, Tan Y. Tandem fecal microbiota transplantation cycles in an allogeneic hematopoietic stem cell transplant recipient targeting carbapenem-resistant Enterobacteriaceae colonization: a case report and literature review. Eur J Med Res. 2021;26(1):37. doi:10.1186/s40001-021-00508-8.
- Wei Y, Gong J, Zhu W, Guo D, Gu L, Li N, Li J. Fecal microbiota transplantation restores dysbiosis in patients with methicillin resistant Staphylococcus aureus enterocolitis. BMC Infect Dis. 2015;15(1). doi:10.1186/s12879-015-0973-1.
- Gouveia C, Palos C, Pereira P, Roque Ramos L, Cravo M. Fecal microbiota transplant in a patient infected with multidrug-resistant bacteria: a case report. GE Port J Gastroenterol. 2020;28(1):56–61. doi:10.1159/000507263.
- Dai M, Liu Y, Chen W, Buch H, Shan Y, Chang L, Bai Y, Shen C, Zhang X, Huo Y, et al. Rescue fecal microbiota transplantation for antibiotic-associated diarrhea in critically ill patients. Crit Care. 2019;23(1):324. doi:10.1186/s13054-019-2604-5.
- Li Q, Wang C, Tang C, He Q, Zhao X, Li N, Li J. Successful treatment of severe sepsis and diarrhea after vagotomy utilizing fecal microbiota transplantation: a case report. Crit Care. 2015;19(1):37. doi:10.1186/s13054-015-0738-7.
- Li Q, Wang C, Tang C, He Q, Zhao X, Li N, Li J. Therapeutic modulation and reestablishment of the intestinal Microbiota with fecal Microbiota transplantation resolves sepsis and diarrhea in a patient. Am J Gastroenterol. 2014;109(11):1832–1834. doi:10.1038/ajg.2014.299.
- Wei Y, Yang J, Wang J, Yang Y, Huang J, Gong H, Cui H, Chen D. Successful treatment with fecal microbiota transplantation in patients with multiple organ dysfunction syndrome and diarrhea following severe sepsis. Crit Care. 2016;20(1):332. doi:10.1186/s13054-016-1491-2.
- Biehl LM, Cruz Aguilar R, Farowski F, Hahn W, Nowag A, Wisplinghoff H, Vehreschild MJGT. Fecal microbiota transplantation in a kidney transplant recipient with recurrent urinary tract infection. Infection. 2018;46(6):871–874. doi:10.1007/s15010-018-1190-9.
- Hocquart M, Pham T, Kuete E, Tomei E, Lagier JC, and Raoult D. Successful fecal microbiota transplantation in a patient suffering from irritable bowel syndrome and recurrent urinary tract infections. Open Forum Infect Dis. 2019 Oct;6(10):ofz398. doi: 10.1093/ofid/ofz398. eCollection 2019 Oct.
- Ramos-Martínez A, Martínez-Ruiz R, Múñez-Rubio E, Valencia-Alijo A, Ferre-Aracil C, Vera-Mendoza MI. Effect of faecal microbiota transplantation on recurrent urinary tract infection in a patient with long-term suprapubic urinary catheter. J Hosp Infect. 2020;105(2):332–333. doi:10.1016/j.jhin.2020.01.016.
- Steed DB, Wang T, Raheja D, Waldman AD, Babiker A, Dhere T, Kraft CS, and Woodworth MH. Gram-negative Taxa and antimicrobial susceptibility after fecal microbiota transplantation for recurrent clostridioides difficile infection. mSphere. 2020 Oct 14;5(5):e00853-20. doi: 10.1128/mSphere.00853-20.
- Tariq R, Pardi DS, Tosh PK, Walker RC, Razonable RR, Khanna S. Fecal microbiota transplantation for recurrent clostridium difficile infection reduces recurrent urinary tract infection frequency. Clin Infect Dis [Internet]. 2017; [accessed 2020 Jan 19]. 65(10):1745–1747. http://academic.oup.com/cid/article/65/10/1745/3978076
- Wang T, Kraft CS, Woodworth MH, Dhere T, and Eaton ME. Fecal microbiota transplant for refractory clostridium difficile infection interrupts 25-year history of recurrent urinary tract infections. Open Forum Infect Dis. 2018 Jan 15;5(2):ofy016. doi: 10.1093/ofid/ofy016. eCollection 2018 Feb.
- Torres Soto M, Hammond S, Elshaboury RH, Johnson J, and Hohmann EL. Recurrent relatively resistant Salmonella infantis infection in 2 immunocompromised hosts cleared with prolonged antibiotics and fecal microbiota transplantation. Open Forum Infect Dis. 2018 Dec 14;6(1):ofy334. doi: 10.1093/ofid/ofy334. eCollection 2019 Jan.
- Biliński J, Winter K, Jasiński M, Szczȩś A, Bilinska N, Mullish BH, Małecka-Panas E, Basak GW. Rapid resolution of COVID-19 after faecal microbiota transplantation. Gut. 2022 Gut2022;71(1):230–232. doi:10.1136/gutjnl-2021-325010.
- Ianiro G, Bibbò S, Masucci L, Quaranta G, Porcari S, Settanni CR, Lopetuso LR, Fantoni M, Sanguinetti M, Gasbarrini A, et al. Maintaining standard volumes, efficacy and safety, of fecal microbiota transplantation for C. difficile infection during the COVID-19 pandemic: a prospective cohort study. Dig Liver Dis. 2020;52(12):1390–1395. doi:10.1016/j.dld.2020.09.004.
- Liu F, Ye S, Zhu X, He X, Wang S, Li Y, Lin J, Wang J, Lin Y, Ren X, et al. Gastrointestinal disturbance and effect of fecal microbiota transplantation in discharged COVID-19 patients. J Med Case Rep. 2021;15(1):60. doi:10.1186/s13256-020-02583-7.
- Chauhan A, Kumar R, Sharma S, Mahanta M, Vayuuru SK, Nayak B, Kumar S, Shalimar S. Fecal microbiota transplantation in Hepatitis B e antigen-positive chronic Hepatitis B patients: a pilot study. Dig Dis Sci. 2021;66(3):873–880. doi:10.1007/s10620-020-06246-x.
- Ren YD, Ye ZS, Yang LZ, Jin LX, Wei WJ, Deng YY, Chen XX, Xiao CX, Yu XF, Xu HZ, et al. Fecal microbiota transplantation induces hepatitis B virus e-antigen (HBeAg) clearance in patients with positive HBeAg after long-term antiviral therapy. Hepatology. 2017;65(5):1765–1768. doi:10.1002/hep.29008.
- Xie Y, Chen Z, Zhou F, Chen L, He J, Xiao C, Xu H, Ren J, Zhang X. IDDF2018-ABS-0201 Faecal microbiota transplantation induced HBSAG decline in HBEAG negative chronic hepatitis B patients after long-term antiviral therapy. Gut. 2018;67:A110.2–A111.
- Serrano-Villar S, Talavera-Rodríguez A, Gosalbes MJ, Madrid N, Pérez-Molina JA, Elliott RJ, Navia B, Lanza VF, Vallejo A, Osman M, et al. Fecal microbiota transplantation in HIV: a pilot placebo-controlled study. Nat Commun [Internet] 2021; accessed 2021 Mar 19]. 12(1): 1139. http://www.nature.com/articles/s41467-021-21472-1
- Vujkovic-Cvijin I, Rutishauser RL, Pao M, Hunt PW, Lynch SV, McCune JM, Somsouk M. Limited engraftment of donor microbiome via one-time fecal microbial transplantation in treated HIV-infected individuals. Gut Microbes. 2017;8:440–450.
- Secombe KR, Al-Qadami GH, Subramaniam CB, Bowen JM, Scott J, Van Sebille YZA, Snelson M, Cowan C, Clarke G, Gheorghe CE, et al. Guidelines for reporting on animal fecal transplantation (GRAFT) studies: recommendations from a systematic review of murine transplantation protocols. Gut Microbes. 2021;13(1):1979878. doi:10.1080/19490976.2021.1979878.
- Khoruts A, Sadowsky MJ. Understanding the mechanisms of faecal microbiota transplantation. Nat Rev Gastroenterol Hepatol [Internet]. 2016; [accessed 2018 Jan 3]. 13(9):508–516. http://www.ncbi.nlm.nih.gov/pubmed/27329806
- Danne C, Rolhion N, Sokol H. Recipient factors in faecal microbiota transplantation: one stool does not fit all. Nat Rev Gastroenterol Hepatol [Internet] 2021; accessed [2021 May 5]. 1–11. http://www.nature.com/articles/s41575-021-00441-5
- Petrof EO, Gloor GB, Vanner SJ, Weese SJ, Carter D, Daigneault MC, Brown EM, Schroeter K, Allen-Vercoe E. Stool substitute transplant therapy for the eradication of clostridium difficile infection: “RePOOPulating” the gut. Microbiome [Internet]. 2013 [accessed 2017 Oct 3]. 1(1):3. http://www.ncbi.nlm.nih.gov/pubmed/24467987
- Kao D, Wong K, Franz R, Cochrane K, Sherriff K, Chui L, Lloyd C, Roach B, Bai AD, Petrof EO, et al. The effect of a microbial ecosystem therapeutic (MET-2) on recurrent clostridioides difficile infection: a phase 1, open-label, single-group trial. Lancet Gastroenterol Hepatol [Internet]. 2021; [accessed 2021 Mar 3]. 0. https://linkinghub.elsevier.com/retrieve/pii/S2468125321000078
- Khanna S, Pardi DS, Kelly CR, Kraft CS, Dhere T, Henn MR, Lombardo M-J, Vulic M, Ohsumi T, Winkler J, et al. A novel microbiome therapeutic increases gut microbial diversity and prevents recurrent Clostridium difficile infection. J Infect Dis 2016; accessed 2017 Oct 3]. 214(2):173–181. http://www.ncbi.nlm.nih.gov/pubmed/26908752
- Ott SJ, Waetzig GH, Rehman A, Moltzau-Anderson J, Bharti R, Grasis JA, Cassidy L, Tholey A, Fickenscher H, Seegert D, et al. Efficacy of sterile fecal filtrate transfer for treating patients with Clostridium difficile infection. Gastroenterology [Internet] accessed 2017 Jun 9]. 152:799–811.e7. Available from. 2017;(4): http://linkinghub.elsevier.com/retrieve/pii/S0016508516353549
- Martinez-Gili L, McDonald JAK, Liu Z, Kao D, Allegretti JR, Monaghan TM, Barker GF, Miguéns Blanco J, Williams HRT, Holmes E, et al. Understanding the mechanisms of efficacy of fecal microbiota transplant in treating recurrent clostridioides difficile infection and beyond: the contribution of gut microbial-derived metabolites. Gut Microbes [Internet] 2020; accessed [2021 Jan 28]. 12(1):1810531. https://pubmed.ncbi.nlm.nih.gov/32893721/
- Brown JR-M, Flemer B, Joyce SA, Zulquernain A, Sheehan D, Shanahan F, O’Toole PW. Changes in microbiota composition, bile and fatty acid metabolism, in successful faecal microbiota transplantation for Clostridioides difficile infection. BMC Gastroenterol. 2018 [[accessed 2018 Aug 31]]. 18(1):131. http://www.ncbi.nlm.nih.gov/pubmed/30153805
- Mullish BH, McDonald JAK, Pechlivanis A, Allegretti JR, Kao D, Barker GF, Kapila D, Petrof EO, Joyce SA, Gahan CGM, et al. Microbial bile salt hydrolases mediate the efficacy of faecal microbiota transplant in the treatment of recurrent Clostridioides difficile infection. Gut [Internet] 2019; [ accessed 2019 Feb 11]. 68(10):1791–1800. https://gut.bmj.com/content/early/2019/02/11/gutjnl-2018-317842
- Monaghan T, Mullish BH, Patterson J, Wong GKSK, Marchesi JR, Xu H, Jilani T, Kao D. Effective fecal microbiota transplantation for recurrent Clostridioides difficile infection in humans is associated with increased signalling in the bile acid-farnesoid X receptor-fibroblast growth factor pathway. Gut Microbes. 2019; [accessed 2018 Sep 9]. 10:1–7. http://www.ncbi.nlm.nih.gov/pubmed/30183484
- Campbell C, McKenney PT, Konstantinovsky D, Isaeva OI, Schizas M, Verter J, Mai C, Jin WB, Guo CJ, Violante S, et al. Bacterial metabolism of bile acids promotes generation of peripheral regulatory T cells. Nature. 2020;581(7809):475–479. doi:10.1038/s41586-020-2193-0.
- Zuo T, Wong SH, Lam K, Lui R, Cheung K, Tang W, Ching JYL, Chan PKS, Chan MCW, Wu JCY, et al. Bacteriophage transfer during faecal microbiota transplantation in Clostridium difficile infection is associated with treatment outcome. Gut [Internet] 2018; [[accessed 2017 Oct 7]. 67:634–643. http://www.ncbi.nlm.nih.gov/pubmed/28539351
- Draper LA, Ryan FJ, Smith MK, Jalanka J, Mattila E, Arkkila PA, Ross RP, Satokari R, Hill C. Long-term colonisation with donor bacteriophages following successful faecal microbial transplantation. Microbiome [Internet]. 2018 [accessed 2018 Dec 21]. 6(1):220. https://microbiomejournal.biomedcentral.com/articles/10.1186/s40168-018-0598-x
- Fujimoto K, Kimura Y, Allegretti JR, Yamamoto M, Zhang Y-Z, Katayama K, Tremmel G, Kawaguchi Y, Shimohigoshi M, Hayashi T, et al. Functional restoration of bacteriomes and viromes by fecal microbiota transplantation. Gastroenterology. 2021;160(6):2089–2102.e12. doi:10.1053/j.gastro.2021.02.013.
- Gogokhia L, Buhrke K, Bell R, Hoffman B, Brown DG, Hanke-Gogokhia C, Ajami NJ, Wong MC, Ghazaryan A, Valentine JF, et al. Expansion of bacteriophages is linked to aggravated intestinal inflammation and colitis. Cell Host Microbe [Internet]. 2019;25(2):285–299.e8. Available from. doi:10.1016/j.chom.2019.01.008.
- Zuo T, Wong SH, Cheung CP, Lam K, Lui R, Cheung K, Zhang F, Tang W, Ching JYL, Wu JCY, et al. Gut fungal dysbiosis correlates with reduced efficacy of fecal microbiota transplantation in Clostridium difficile infection. Nat Commun 2018; [ accessed 2019 Feb 22]. 9(1):3663. http://www.nature.com/articles/s41467-018-06103-6
- Leonardi I, Paramsothy S, Doron I, Semon A, Kaakoush NO, Clemente JC, Faith JJ, Borody TJ, Mitchell HM, Colombel JF, et al. Fungal Trans-kingdom dynamics linked to responsiveness to Fecal Microbiota Transplantation (FMT) therapy in ulcerative colitis. Cell Host Microbe. 2020;27(5):823–829.e3. doi:10.1016/j.chom.2020.03.006.
- Liang G, Bushman FD. The human virome: assembly, composition and host interactions. Nat Rev Microbiol. 2021;19(8):514–527. doi:10.1038/s41579-021-00536-5.
- Ducarmon QR, Zwittink RD, Hornung BVH, van Schaik W, Young VB, Kuijper EJ. Gut microbiota and colonization resistance against bacterial enteric infection. Microbiol Mol Biol Rev. 2019;83(3):e00007–19. doi:10.1128/MMBR.00007-19.
- Huus KE, Frankowski M, Pučić-Baković M, Vučković F, Lauc G, Mullish BH, Marchesi JR, Monaghan TM, Kao D, Finlay BB. Changes in IgA-targeted microbiota following fecal transplantation for recurrent Clostridioides difficile infection. Gut Microbes. 2021; [accessed 2021 Jan 28]. 13:1–12. Available from https://pubmed.ncbi.nlm.nih.gov/33382360/
- Huang J, Liu J, Tian R, Liu K, Zhuang P, Sherman HT, Budjan C, Fong M, Jeong M-S, Kong X-J, et al. A multi-factorial observational study on sequential fecal microbiota transplant in patients with medically refractory clostridioides difficile infection. Cells. 2021;11(1):10. doi:10.3390/cells11010010.
- Segal JP, Mullish BH, Quraishi MN, Iqbal T, Marchesi JR, Sokol H. Mechanisms underpinning the efficacy of faecal microbiota transplantation in treating gastrointestinal disease [Internet]. Therap Adv Gastroenterol. 2020; [accessed 2021 Jan 28]. 13:175628482094690. doi:10.1177/1756284820946904.
- Burrello C, Garavaglia F, Cribiù FM, Ercoli G, Lopez G, Troisi J, Colucci A, Guglietta S, Carloni S, Guglielmetti S, et al. Therapeutic faecal microbiota transplantation controls intestinal inflammation through IL10 secretion by immune cells. Nat Commun 2018; [ accessed 2019 Feb 23]. 9(1):5184. http://www.nature.com/articles/s41467-018-07359-8
- Monaghan TM, Seekatz AM, Markham NO, Yau TO, Hatziapostolou M, Jilani T, Christodoulou N, Roach B, Birli E, Pomenya O, et al. Fecal microbiota transplantation for recurrent Clostridioides difficile infection associates with functional alterations in circulating microRNAs. Gastroenterology. 2021;161(1):255–270.e4. doi:10.1053/j.gastro.2021.03.050.
- Monaghan TM, Pučić-Baković M, Vučković F, Lee C, Kao D, Wójcik I, Kliček F, Polytarchou C, Roach B, Louie T, et al. Decreased complexity of serum N-glycan structures associates with successful fecal microbiota transplantation for recurrent Clostridioides difficile infection. Gastroenterology. 2019;157(6):1676–1678.e3. doi:10.1053/j.gastro.2019.08.034.
- Hu J, Ma L, Nie Y, Chen J, Zheng W, Wang X, Xie C, Zheng Z, Wang Z, Yang T, et al. A microbiota-derived bacteriocin targets the host to confer diarrhea resistance in early-weaned piglets. Cell Host Microbe. 2018;24(6):817–832.e8. doi:10.1016/j.chom.2018.11.006.
- Battaglioli EJ, Hale VL, Chen J, Jeraldo P, Ruiz-Mojica C, Schmidt BA, Rekdal VM, Till LM, Huq L, Smits SA, et al. Clostridioides difficile uses amino acids associated with gut microbial dysbiosis in a subset of patients with diarrhea. Sci Transl Med Internet] 2018; [accessed 2019 Mar 10]; 10(464):eaam7019. http://www.ncbi.nlm.nih.gov/pubmed/30355801
- van Schaik W. The human gut resistome. Philos Trans R Soc B Biol Sci. 2015;370(1670):20140087. doi:10.1098/rstb.2014.0087.
- Wright GD. The antibiotic resistome: the nexus of chemical and genetic diversity. Nat Rev Microbiol. 2007;5(3):175–186. doi:10.1038/nrmicro1614.
- Magiorakos AP, Srinivasan A, Carey RB, Carmeli Y, Falagas ME, Giske CG, Harbarth S, Hindler JF, Kahlmeter G, Olsson-Liljequist B, et al. Multidrug-resistant, extensively drug-resistant and pandrug-resistant bacteria: an international expert proposal for interim standard definitions for acquired resistance. Clin Microbiol Infect. 2012;18(3):268–281. doi:10.1111/j.1469-0691.2011.03570.x.
- Tacconelli E, Magrini N. WHO (2017) Global priority list of antibiotic-resistant bacteria to guide research, discovery, and development of new antibiotics. Cad Pesqui. 2017;43:348–365.
- De Oliveira DMP, Forde BM, Kidd TJ, Harris PNA, Schembri MA, Beatson SA, Paterson DL, Walker MJ. Antimicrobial resistance in ESKAPE pathogens. Clin Microbiol Rev. 2020;33(3):e00181–19. doi:10.1128/CMR.00181-19.
- Cattaneo C, Di Blasi R, Skert C, Candoni A, Martino B, Di Renzo N, Delia M, Ballanti S, Marchesi F, Mancini V, et al. Bloodstream infections in haematological cancer patients colonized by multidrug-resistant bacteria. Ann Hematol. 2018;97(9):1717–1726. doi:10.1007/s00277-018-3341-6.
- Tacconelli E, Mazzaferri F, de Smet AM, Bragantini D, Eggimann P, Huttner BD, Kuijper EJ, Lucet JC, Mutters NT, Sanguinetti M, et al. ESCMID-EUCIC clinical guidelines on decolonization of multidrug-resistant Gram-negative bacteria carriers. Clin Microbiol Infect. 2019;25(7):807–817. doi:10.1016/j.cmi.2019.01.005.
- Ubeda C, Bucci V, Caballero S, Djukovic A, Toussaint NC, Equinda M, Lipuma L, Ling L, Gobourne A, No D, et al. Intestinal microbiota containing Barnesiella species cures vancomycin-resistant Enterococcus faecium colonization. Infect Immun. 2013;81(3):965–973. doi:10.1128/IAI.01197-12.
- Kim SG, Becattini S, Moody TU, Shliaha PV, Littmann ER, Seok R, Gjonbalaj M, Eaton V, Fontana E, Amoretti L, et al. Microbiota-derived lantibiotic restores resistance against vancomycin-resistant Enterococcus. Nature. 2019;572(7771):665–669. doi:10.1038/s41586-019-1501-z.
- Moss EL, Falconer SB, Tkachenko E, Wang M, Systrom H, Mahabamunuge J, Relman DA, Hohmann EL, Bhatt AS, Isaacson RE. Long-term taxonomic and functional divergence from donor bacterial strains following fecal microbiota transplantation in immunocompromised patients. PLoS One. 2017;12(8):e0182585. doi:10.1371/journal.pone.0182585.
- Bajaj JS, Shamsaddini A, Fagan A, Sterling RK, Gavis E, Khoruts A, Fuchs M, Lee H, Sikaroodi M, Gillevet PM. Fecal microbiota transplant in cirrhosis reduces gut microbial antibiotic resistance genes: analysis of two trials. Hepatol Commun. 2021;5(2):258–271. doi:10.1002/hep4.1639.
- Bahl MI, Jørgensen SMD, Skriver AH, Larsen NA, Wang M, Licht TR, Dahlerup JF, Hvas CL. Faecal microbiota transplantation for eradication of co-infection with Clostridioides difficile and extensively drug-resistant KPC-producing Klebsiella pneumoniae. Scand J Gastroenterol. 2020;55(5):626–630. doi:10.1080/00365521.2020.1753806.
- Dubberke ER, Mullane KM, Gerding DN, Lee CH, Louie TJ, Guthertz H, Jones C. Clearance of vancomycin-resistant Enterococcus concomitant with administration of a microbiota-based drug targeted at recurrent Clostridium difficile infection. Open Forum Infect Dis. 2016;3(3). doi:10.1093/ofid/ofw133.
- Ghani R, Mullish BH, Davies FJ, Marchesi JR. How to adapt an intestinal microbiota transplantation program to reduce the risk of invasive multidrug-resistant infection. Clin Microbiol Infect. 2021. doi:10.1016/j.cmi.2021.11.006.
- Davido B, Moussiegt A, Dinh A, Bouchand F, Matt M, Senard O, Deconinck L, Espinasse F, Lawrence C, Fortineau N, et al. Germs of thrones - spontaneous decolonization of Carbapenem-Resistant Enterobacteriaceae (CRE) and Vancomycin-Resistant Enterococci (VRE) in Western Europe: is this myth or reality? Antimicrob Resist Infect Control. 2018;7(1). doi:10.1186/s13756-018-0390-5.
- Montassier E, Al-Ghalith GA, Ward T, Corvec S, Gastinne T, Potel G, Moreau P, de la Cochetiere MF, Batard E, Knights D. Pretreatment gut microbiome predicts chemotherapy-related bloodstream infection. Genome Med. 2016;8(1):49. doi:10.1186/s13073-016-0301-4.
- Ianiro G, Murri R, Sciumè GD, Impagnatiello M, Masucci L, Ford AC, Law GR, Tilg H, Sanguinetti M, Cauda R, et al. Incidence of bloodstream infections, length of hospital stay, and survival in patients with recurrent clostridioides difficile infection treated with fecal microbiota transplantation or antibiotics a prospective cohort study. Ann Intern Med [Internet] 2019; accessed 2019 Dec 11]. 171(10):695–702. http://www.ncbi.nlm.nih.gov/pubmed/31683278
- van Vliet MJ, Harmsen HJM, de Bont ESJM, Tissing WJE, Manchester M. The role of intestinal microbiota in the development and severity of chemotherapy-induced mucositis. PLoS Pathog. 2010;6(5):1–7. doi:10.1371/journal.ppat.1000879.
- Bhalodi AA, Van Engelen TSR, Virk HS, Wiersinga WJ. Impact of antimicrobial therapy on the gut microbiome. J Antimicrob Chemother. 2019;74(Supplement_1):I6–15. doi:10.1093/jac/dky530.
- Peled JU, Gomes ALC, Devlin SM, Littmann ER, Taur Y, Sung AD, Weber D, Hashimoto D, Slingerland AE, Slingerland JB, et al. Microbiota as predictor of mortality in allogeneic hematopoietic-cell transplantation. N Engl J Med. 2020;382(9):822–834. doi:10.1056/NEJMoa1900623.
- Taur Y, Xavier JB, Lipuma L, Ubeda C, Goldberg J, Gobourne A, Lee YJ, Dubin KA, Socci ND, Viale A, et al. Intestinal domination and the risk of bacteremia in patients undergoing allogeneic hematopoietic stem cell transplantation. Clin Infect Dis. 2012;55(7):905–914. doi:10.1093/cid/cis580.
- Stoma I, Littmann ER, Peled JU, Giralt S, van den Brink MRM, Pamer EG, Taur Y. Compositional flux within the intestinal microbiota and risk for bloodstream infection with Gram-negative bacteria. Clin Infect Dis. 2021;73(11):e4627–35. doi:10.1093/cid/ciaa068.
- Tamburini FB, Andermann TM, Tkachenko E, Senchyna F, Banaei N, Bhatt AS. Precision identification of diverse bloodstream pathogens in the gut microbiome. Nat Med. 2018;24(12):1809–1814. doi:10.1038/s41591-018-0202-8.
- Cattaneo C, Zappasodi P, Mancini V, Annaloro C, Pavesi F, Skert C, Ferrario A, Todisco E, Saccà V, Verga L, et al. Emerging resistant bacteria strains in bloodstream infections of acute leukaemia patients: results of a prospective study by the Rete Ematologica Lombarda (Rel). Ann Hematol. 2016;95(12):1955–1963. doi:10.1007/s00277-016-2815-7.
- Innes AJ, Mullish BH, Ghani R, Szydlo RM, Apperley JF, Olavarria E, Palanicawandar R, Kanfer EJ, Milojkovic D, McDonald JAK, et al. Fecal microbiota transplant mitigates adverse outcomes seen in patients colonized with multidrug-resistant organisms undergoing allogeneic hematopoietic cell transplantation. Front Cell Infect Microbiol. 2021;11:814. doi:10.3389/fcimb.2021.684659.
- Ojima M, Motooka D, Shimizu K, Gotoh K, Shintani A, Yoshiya K, Nakamura S, Ogura H, Iida T, Shimazu T. Metagenomic analysis reveals dynamic changes of whole gut microbiota in the acute phase of intensive care unit patients. Dig Dis Sci. 2016;61(6):1628–1634. doi:10.1007/s10620-015-4011-3.
- Dickson RP. The microbiome and critical illness. Lancet Respir Med. 2016;4(1):59–72. doi:10.1016/S2213-2600(15)00427-0.
- Shimizu K, Ogura H, Hamasaki T, Goto M, Tasaki O, Asahara T, Nomoto K, Morotomi M, Matsushima A, Kuwagata Y, et al. Altered gut flora are associated with septic complications and death in critically ill patients with systemic inflammatory response syndrome. Dig Dis Sci. 2011;56(4):1171–1177. doi:10.1007/s10620-010-1418-8.
- Gai X, Wang H, Li Y, Zhao H, He C, Wang Z, Zhao H. Fecal microbiota transplantation protects the intestinal mucosal barrier by reconstructing the gut microbiota in a murine model of Sepsis. Front Cell Infect Microbiol. 2021;11:912. doi:10.3389/fcimb.2021.736204.
- Kim SM, DeFazio JR, Hyoju SK, Sangani K, Keskey R, Krezalek MA, Khodarev NN, Sangwan N, Christley S, Harris KG, et al. Fecal microbiota transplant rescues mice from human pathogen mediated sepsis by restoring systemic immunity. Nat Commun. 2020;11(1):2354. doi:10.1038/s41467-020-15545-w.
- Gutiérrez-Gutiérrez B, Salamanca E, de Cueto M, Pascual A, Rodríguez-Baño J, Hsueh PR, Viale P, Paño-Pardo JR, Venditti M, Tumbarello M, et al. A predictive model of mortality in patients with bloodstream infections due to Carbapenemase-producing Enterobacteriaceae. Mayo Clin Proc. 2016;91(10):1362–1371. doi:10.1016/j.mayocp.2016.06.024.
- Loubet P, Ranfaing J, Dinh A, Dunyach-Remy C, Bernard L, Bruyère F, Lavigne JP, Sotto A. Alternative therapeutic options to antibiotics for the treatment of urinary tract infections. Front Microbiol. 2020;11:1509. doi:10.3389/fmicb.2020.01509.
- Bader MS, Hawboldt J, Brooks A. Management of complicated urinary tract infections in the era of antimicrobial resistance. Postgrad Med. 2010;122(6):7–15. doi:10.3810/pgm.2010.11.2217.
- Magruder M, Sholi AN, Gong C, Zhang L, Edusei E, Huang J, Albakry S, Satlin MJ, Westblade LF, Crawford C, et al. Gut uropathogen abundance is a risk factor for development of bacteriuria and urinary tract infection. Nat Commun. 2019;10(1):5521. doi:10.1038/s41467-019-13467-w.
- Swarte JC, Douwes RM, Hu S, Vila AV, Eisenga MF, van Londen M, Gomes-Neto AW, Weersma RK, Harmsen HJM, Bakker SJL. Characteristics and dysbiosis of the gut microbiome in renal transplant recipients. J Clin Med. 2020;9(2):386. doi:10.3390/jcm9020386.
- Britt NS, Hagopian JC, Brennan DC, Pottebaum AA, Santos CAQ, Gharabagi A, Horwedel TA. Effects of recurrent urinary tract infections on graft and patient outcomes after kidney transplantation. Nephrol Dial Transplant. 2017;32(10):1758–1766. doi:10.1093/ndt/gfx237.
- Alavi S, Mitchell JD, Cho JY, Liu R, Macbeth JC, Hsiao A. Interpersonal Gut microbiome variation drives susceptibility and resistance to cholera infection. Cell. 2020;181(7):1533–1546.e13. doi:10.1016/j.cell.2020.05.036.
- Burgess SL, Leslie JL, Uddin J, Oakland DN, Gilchrist C, Moreau GB, Watanabe K, Saleh M, Simpson M, Thompson BA, et al. Gut microbiome communication with bone marrow regulates susceptibility to amebiasis. J Clin Invest. 2020;140:4019–4024.
- Buffie CG, Bucci V, Stein RR, McKenney PT, Ling L, Gobourne A, No D, Liu H, Kinnebrew M, Viale A, et al. Precision microbiome reconstitution restores bile acid mediated resistance to Clostridium difficile. Nature [Internet] 2014; accessed [2017 Oct 7]. 517(7533)::205–208. http://www.ncbi.nlm.nih.gov/pubmed/25337874
- Heimesaat MM, Mrazek K, Bereswill S. Murine fecal microbiota transplantation lowers gastrointestinal pathogen loads and dampens pro-inflammatory immune responses in Campylobacter jejuni infected secondary abiotic mice. Sci Rep. 2019;9(1):19797. doi:10.1038/s41598-019-56442-7.
- Sun X, Winglee K, Gharaibeh RZ, Gauthier J, He Z, Tripathi P, Avram D, Bruner S, Fodor A, Jobin C. Microbiota-derived metabolic factors reduce Campylobacteriosis in mice. Gastroenterology. 2018;154(6):1751–1763.e2. doi:10.1053/j.gastro.2018.01.042.
- Budden KF, Gellatly SL, Wood DLA, Cooper MA, Morrison M, Hugenholtz P, Hansbro PM. Emerging pathogenic links between microbiota and the gut-lung axis. Nat Rev Microbiol. 2017;15(1):55–63. doi:10.1038/nrmicro.2016.142.
- Groves HT, Higham SL, Moffatt MF, Cox MJ, Tregoning JS. Respiratory viral infection alters the gut microbiota by inducing inappetence. MBio. 2020;11(1):e03236–19. doi:10.1128/mBio.03236-19.
- Zuo T, Liu Q, Zhang F, Lui GCY, Tso EYK, Yeoh YK, Chen Z, Boon SS, Chan FKL, Chan PKS, et al. Depicting SARS-CoV-2 faecal viral activity in association with gut microbiota composition in patients with COVID-19. Gut. 2021;70(2):276–284. Gut 2021. doi:10.1136/gutjnl-2020-322294.
- Lu H, Wu Z, Xu W, Yang J, Chen Y, Li L. Intestinal microbiota was assessed in cirrhotic patients with Hepatitis B virus infection. Microb Ecol. 2011;61(3):693–703. doi:10.1007/s00248-010-9801-8.
- Wei X, Yan X, Zou D, Yang Z, Wang X, Liu W, Wang S, Li X, Han J, Huang L, et al. Abnormal fecal microbiota community and functions in patients with hepatitis B liver cirrhosis as revealed by a metagenomic approach. BMC Gastroenterol. 2013;13(1):175. doi:10.1186/1471-230X-13-175.
- Koay WLA, Siems LV, Persaud D. The microbiome and HIV persistence: implications for viral remission and cure. Curr Opin HIV AIDS. 2018;13(1):61–68. doi:10.1097/COH.0000000000000434.
- Pandiyan P, Younes SA, Ribeiro SP, Talla A, McDonald D, Bhaskaran N, Levine AD, Weinberg A, Sekaly RP. Mucosal regulatory T cells and T helper 17 cells in HIV-associated immune activation. Front Immunol. 2016;7:228. doi:10.3389/fimmu.2016.00228.
- Nowak P, Troseid M, Avershina E, Barqasho B, Neogi U, Holm K, Hov JR, Noyan K, Vesterbacka J, Svärd J, et al. Gut microbiota diversity predicts immune status in HIV-1 infection. Aids. 2015;29(18):2409–2418. doi:10.1097/QAD.0000000000000869.
- Schünemann M, Oette M. Fecal microbiota transplantation for Clostridium difficile-associated colitis in a severely immunocompromised critically ill AIDS patient: a case report. AIDS (London, England). 2014;28(5):798–799. doi:10.1097/QAD.0000000000000148.
- Yadav D, Khanna S. Safety of fecal microbiota transplantation for Clostridioides difficile infection focusing on pathobionts and SARS-CoV-2. Therap Adv Gastroenterol. 2021;14:17562848211009694. doi:10.1177/17562848211009694.