ABSTRACT
Lysophospholipids (LPLs) are crucial for regulating epithelial integrity and homeostasis in eukaryotes, however the effects of LPLs produced by bacteria on host cells is largely unknown. The membrane of the human bacterial pathogen Campylobacter jejuni is rich in LPLs. Although C. jejuni possesses several virulence factors, it lacks traditional virulence factors like type III secretion systems, present in most enteropathogens. Here, we provide evidence that membrane lipids lysophosphatidylethanolamines (lysoPEs) of C. jejuni are able to lyse erythrocytes and are toxic for HeLa and Caco-2 cells. Lactate dehydrogenase (LDH) release assays and confocal microscopy revealed that lysoPE permeabilizes the cells. LysoPE toxicity was partially rescued by oxidative stress inhibitors, indicating that intracellular reactive oxygen species may contribute to the cell damage. Our results show that especially the short-chain lysoPEs (C:14) which is abundantly present in the C. jejuni membrane may be considered as a novel virulence factor.
Introduction
Lysophospholipids (LPLs) are bioactive signaling molecules containing a single fatty acid tail. In eukaryotic cells, LPLs exhibit diverse biological properties, such as promoting cell growth, acting as potent lipid mediators, or reducing bacterial infections.Citation1,Citation2 LPLs are generated as metabolic intermediates in phospholipid synthesis or during membrane degradation.Citation3 The formation of LPLs from phospholipids is due to activation of phospholipase A1 or A2. Phospholipase A1 (PldA1) and phospholipase A2 (PldA2), hydrolyzing the stereospecific numbering (Sn)-1 and −2 acyl chain, respectively.Citation4 (Sn)-1 LPLs possess more shorter, saturated acyl chains than (Sn)-2 LPLs while (Sn)-2 LPLs possess more unsaturated acyl chains.Citation5 (Sn)-1 LPLs and (Sn)-2 LPLs might have different biological functions as only (Sn)-1 LPLs can act as mediators of antimicrobial activity toward Gram-positive bacteria.Citation6 Lysophosphatidic acid (lysoPA) is important in controlling and signaling cancer;Citation7 lysophosphatidylcholine (lysoPC) evokes cellular injury by oxidative events that involve formation of low-density lipoprotein. Both lysoPA and lysoPC of the host trigger the release of the proinflammatory flagellin from Salmonella thereby enhancing the innate and inflammatory responses toward this bacterium.Citation8 The role of other LPLs like lysophosphatidylethanolamine (lysoPE) has not been elucidated to such a high degree.
Bacteria usually contain small amounts (<1%) of LPLs in their membrane,Citation3 mostly found in the form of lysoPE.Citation9 It has been mentioned that lysoPEs isolated from Bacteroidetes Chitinophaga spp. have antimicrobial activities against certain Gram-positive bacteria.Citation10 LysoPA and its precursor lysoPC derived from Lactobacillus plantarum has been considered being toxic for humans and could disturb the signaling networks in host cells.Citation11 The biological function of LPLs in bacteria is still poorly understood, but they may play a role in bacterial survival or invasion.Citation2 LPLs may be an underestimated factor in bacterial pathogenesis and inflammation response of the host.
We previously showed that the bacterial pathogen Campylobacter jejuni possesses a wide spectrum of LPLs that varies dependent on the environmental conditions.Citation12 C. jejuni is the leading cause of bacterial foodborne human gastroenteritis in developed countries.Citation13 Symptomatic infection typically involves intestinal inflammation, fever, and bloody diarrhea.Citation13 C. jejuni is supposed to penetrate the intestinal mucus layer, colonize the crypts, and disrupt the epithelial barrier.Citation14 Although C. jejuni possesses a number of virulence factors, such as flagella, proteases, adhesins, type VI secretion system, and cytolethal distending toxin, it lacks traditional virulence factors like type III secretion systems,Citation15 present in most enteropathogens and therefore the molecular basis of C. jejuni infection is still poorly understood.Citation16
In the present study, we investigated the biological role(s) of the LPLs of C. jejuni as potential virulence determinant. We demonstrate that C. jejuni PldA generates both (Sn)-1 and (Sn)-2 LPLs. The generated short-chain fatty acids lysoPE was found to exert hemolytic activity and effectively damage different types of eukaryotic cells, indicating that it may act as a virulence factor.
Results
C. jejuni PldA produces (Sn)-1 as well as (Sn)-2 LPLs
To ensure that C. jejuni produced lysolipids under the conditions employed, we extracted LPLs from 16 h old cultures of C. jejuni wildtype 81116 and its isogenic ΔpldA mutant strain growth under microaerophilic conditions at 42°C. Liquid chromatography tandem mass spectrometry (LC-MS/MS) of extracted lipids clearly demonstrated the presence of both (Sn)-1 and −2 acyl chain LPLs in wildtype C. jejuni. For example, the majority of lysoPG 18:0 was present as (Sn)-1 lysoPG (0/18:0), while (Sn)-2 lysoPG (18:0/0) was roughly 4 times less detected (). Both lysoPG species were virtually absent in a mutant strain lacking a functional PldA (). Similar results were observed for other LPLs. This indicates that PldA is the primary enzyme involved in LPL formation in C. jejuni and that this enzyme is able to cleave both (Sn)-1 and −2 acyl chains but prefers the (Sn)-1 site.
C. jejuni LPLs cause erythrocytes to lyse
To test the effect of bacterial LPLs on eukaryotic cells, we first determined the hemolytic activity of wildtype C. jejuni and the ΔpldA mutant. Wildtype C. jejuni caused strong hemolysis of horse erythrocytes in contrast to C. jejuni ΔpldA. Complementation of the mutant (C. jejuni ΔpldA +pldA) restored the strong hemolytic activity (). No hemolysis was observed by using C. jejuni cell-free culture supernatant () nor when the pellet fraction of ultracentrifugated cell-free culture supernatant was used, suggesting that the hemolysis required bacteria-host cell contact. In other bacterial species phospholipase A itself has been shown to induce hemolysis. These enzymes generally prefer phosphatidylcholine (PC) as substrates.Citation17 C. jejuni-mediated hemolysis was observed for both PC-rich (horse, chicken and human) and PC-deficient (sheep) cells indicating that the hemolysis was PC independent (). Furthermore, the C. jejuni-induced hemolysis was maintained after heating of the bacteria (75°C, 30 min) (), indicating that the activity was insensitive to denaturation. Hemolysis was also still present when horse erythrocytes were incubated with isolated membranes of C. jejuni, even after proteinase K treatment followed by heat inactivation (). These results together strongly suggest that C. jejuni LPLs are causing hemolysis.
Figure 2. C. jejuni lysoPE induced hemolysis. (a) C. jejuni strains were incubated with erythrocytes from different species; the hemolysis results depicted in the left panel were quantified by measuring absorbance at 420 nm. (b-e) Hemolysis of horse erythrocytes after incubation with: (b) live, heat-treated or sonicated C. jejuni, or with the cell-free supernatant of C. jejuni; (c) live bacteria, whole membrane or protease K-treated membranes of C. jejuni; (d) purified major phospholipid classes; (e) commercially available LPLs. MilliQ water and DPBS were used as positive (100% value) and negative (0% value) control in the hemolysis assay, respectively. Data of three independent experiments with three independent preparations of bacterial samples are presented as mean values ± standard deviation, *P < .1, **P < .01, ***P < .001, ****P < .0001, ns P > .1.
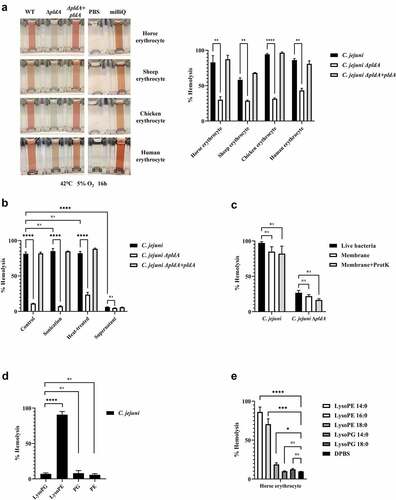
Short lysoPEs are responsible for hemolysis
In order to determine the phospholipid species responsible for the red blood cell lysis, we separated the major phospholipid classes PG, PE, lysoPG, and lysoPE from C. jejuni wildtype. The purity of the phospholipid classes samples are shown figure S1. Minimal lysis was observed when PG, PE, or lysoPG were incubated with horse erythrocytes, whereas the lysoPE fraction lysed more than 90% of the cells (). This indicates that C. jejuni lysoPE is the primary cause for hemolysis. Next, we investigated the effect of the length of the fatty acid tail using commercial lysoPE species. All lysoPE species induced hemolysis but the shortest fatty acid tail containing lysoPE were most effective (). Of note, lysoPE14 and lysoPE16 make up 50% of the C. jejuni lysoPE molecules.Citation12 Together, these results indicate that short lysoPE species disrupt the integrity of the cell membrane of horse erythrocytes.
Short lysoPEs are also toxic for epithelial cells
During the natural infection, C. jejuni is in close contact with mucosal epithelial cells. To determine whether lysoPE may also damage epithelial cells, we measured the LDH release. Wildtype C. jejuni caused considerable LDH release from the human HeLa and Caco-2 cells after 5 h of incubation. This effect was much less for ΔpldA mutant, while the complemented C. jejuni ΔpldA +pldA mutant regained the harmful wildtype behavior (). Strong LDH release was also observed after exposure to purified C. jejuni-derived lysoPE (). In agreement with the hemolysis, the short fatty acid tail containing lysoPE 14:0 caused the highest LDH release (). Together, the results indicate that short-chain lysoPE as present in C. jejuni not only displays hemolytic activity but also causes damage to epithelial cells.
Figure 3. C. jejuni lysoPE-induced toxicity for host cells rescued by vitamin E and DPPD. (a) LDH release of HeLa and Caco-2 cells treated with C. jejuni strains . (b) HeLa cells treated with purified phospholipid fractions. (c) HeLa cells treated with commercially available LPLs. (d-e) Horse erythrocytes and (f- g) HeLa cells were treated without or with vitamin E or DPPD, washed and then exposed to lysoPE 14:0. Data are from three independent experiments with three independent preparations of bacterial samples and presented as mean values ± standard deviation, *P < .1, **P < .01, ***P < .001, ****P < .0001, ns P > .1.
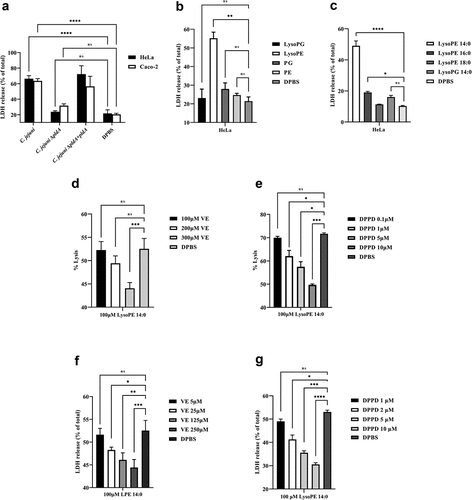
LysoPE induces cell damage by oxidative stress
To investigate whether the lysoPE-induced cell damage is due to oxidative stress as seen for lysoPC,Citation18 the effect of two antioxidants, vitamin E, and DPPD, was examined. Horse erythrocytes were pre-exposed to antioxidant, and then incubated with lysoPE 14:0. For both antioxidants, a clear concentration-dependent inhibition of lysoPE-induced hemolysis was observed (). Experiments with epithelial cells yielded similar results with significantly less damage after pre-treatment of cells with vitamin E and DPPD (). To corroborate these findings we applied confocal microscopy. In the absence of LPLs, epithelial cell membranes were impermeable to the green fluorescent lectin WGA ( & S2A). However, after incubation with lysoPE 14:0 also nuclear membranes became WGA-positive (red arrows in & S2B), but not for lysoPG 14:0 treatment ( & S2C), indicating that lysoPE enabled the lectin to pass the plasma membrane and enter the cells. Pretreatment of the cells with antioxidant prior to lysoPE 14:0 treatment prevented nuclear membrane staining ( & S2D) consistent with the LDH release results. Together, these results point to oxidative stress as a major factor in the short chain lysoPE-induced cell damage.
Figure 4. LysoPE permeabilizes the epithelial cells membrane. HeLa cells incubated with (a) DPBS, (b) lysoPE 14:0, (c) lysoPG 14:0, or (d) pre-treated with vitamin E, washed and incubated with lysoPE 14:0 were stained with the membrane stain fluorescent WGA (green) and/or nuclear DAPI stain (blue) and visualized by confocal microscopy. Red arrows point to nuclear membrane stained with WGA. White scale bars represent 5 μm.
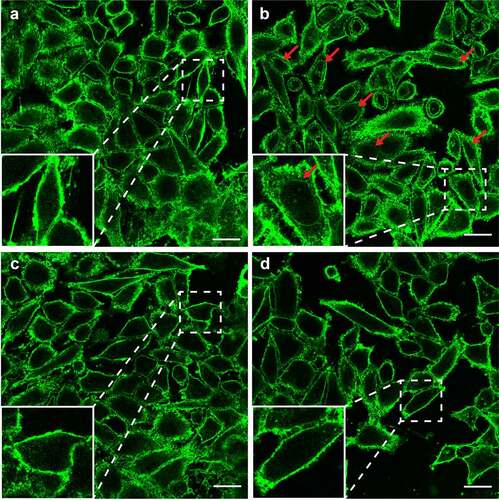
Discussion
In eukaryotic cells, LPLs play an essential role in a broad variety of biological processes.Citation1 Recently, the human gut microbiota has been shown to contribute to the production of lysoPC, which causes damage of the epithelial barrier.Citation19 Some bacterial pathogens, including C. jejuni, can produce large amounts of LPLs but their effect on host cell biology is largely unknown. Here, we show that the PldA of C. jejuni possesses phospholipase activity that generates (Sn)-1 and (Sn)-2 LPLs which is more typical for phospholipase class B proteins.Citation20 We also for the first time provide evidence that the produced lysoPE phospholipids can lyse erythrocytes and damage epithelial cells. This effect is especially evident for short-chain lysoPE species and can be rescued by oxidative stress inhibitors. These results indicate that C. jejuni lysoPE may be an important unforeseen bacterial virulence factor that causes cell damage (at least partially) via an oxidative stress-sensitive mechanism.
The finding that C. jejuni PldA generates both (Sn)-1 and (Sn)-2 LPLs was unexpected as the amino acid sequence characteristics suggest that the enzyme belongs to the phospholipid class A family of proteins.Citation21 The observed virtual absence of LPLs in C. jejuni ΔpldA indicates that no other phospholipases are active. However, our LC-MS/MS results clearly indicate that the C. jejuni PldA enzyme prefers to cleave at the (Sn)-1 site. The position of (Sn) cleavage is relevant as PldA1 generates mostly saturated LPL, while PldA2 generates mostly unsaturated or cyclo phospholipids,Citation5 with different biological effects on membrane function.Citation22 The finding that the C. jejuni PldA enzyme prefers to cleave at the (Sn)-1 site implies the formation of a large amount of membrane integrity reducing LPLs.Citation22
The first evidence of a cytotoxic effect of C. jejuni LPLs was the observed hemolysis caused by C. jejuni wild type but not C. jejuni ΔpldA. Complementation of the pldA defect confirmed the crucial role of LPL formation in the toxicity. C. jejuni-induced hemolytic activity has previously been reported for both type VI secretion system-positive and negative C. jejuni strains, but the causing factor is still unclear.Citation23,Citation24 It has been speculated that the hemolysis was due to an intracellular component released after cell death or lysis,Citation25 or by the PldA directly targeting host cells membranes. Here we provide evidence that the PldA products, the LPLs, exert strong hemolytic activity.
Fractionation of the major phospholipid classes of C. jejuni identified lysoPE as prime hemolysis inducing factor (). So far only lysoPA and lysoPC have been reported to affect erythrocytes.Citation26 We found that besides the head group, also the length of the tail of the lysoPE is important for hemolysis as especially short lysoPEs were toxic (). This resembles observations with lysoPC where increasing the chain length of the hydrophobic tail decreases the rate of the hemolytic reaction.Citation27 According to our previous results the phospholipidome of C. jejuni can consists of more than 33% lysoPE of which almost 50% is present as lysoPE 14 and 16.Citation12 This likely explains why the membranes of live or dead C. jejuni bacteria are toxic for erythrocytes.
Interestingly, the cytotoxicity of C. jejuni membranes and purified lysoPE was also observed for epithelial cells as evident from the strong PldA dependent increase of LDH release and the staining of intracellular membranes with WGA in lysoPE-treated cells only ( & S2B). Maximum LDH release and intracellular staining were observed after exposure to short chain fatty acid containing lysoPE (). In humans, short-chain fatty acids have been identified as signaling molecules between the gut microbiota and the host, and are regarded as toxic at high concentration.Citation28 C. jejuni has been shown to induce LDH release in human neutrophils and dendritic cells (less than 10%), but in epithelial cells the LDH release is relatively low.Citation15,Citation29 We were able to strongly increase the LDH release from the epithelial cells by replacing the tissue culture medium with DPBS during the incubation with C. jejuni (Fig. S3). We noticed that calcium excess in the culture medium reduces the C. jejuni cytotoxicity as has been noted for C. coli PldA.Citation30
What is causing lysoPE-induced cell damage? It has been shown that the incorporation of even a small amount (1 mol.%) of fatty acids or lysolipids in lipid membranes creates instabilities in the lipid bilayer.Citation31 One theory for LPL-induced cell damage is that LPLs, such as lysoPC, can evoke an oxidant stress-dependent transient membrane permeabilization in cells.Citation32 Our results support this hypothesis as two antioxidants, vitamin E and DPPD, protect the cells from the LPLs damage. Both inhibitors reduced the lysoPE 14:0 induced cytotoxicity and also inhibited the intracellular membrane staining (). The mechanism of toxicity of lysoPE 14:0 may thus resemble the effect of as lysoPC leading to a stress-dependent transient membrane permeabilization.Citation32
In conclusion, we for the first time identified C. jejuni lysolipids, especially lysoPE, as cytotoxic factor. The toxic short-tailed lysoPE induces hemolysis and induces oxidant stress-dependent membrane leakage in epithelial cells. Bacterial lysoPE can thus be considered as a novel virulence factor of C. jejuni and possibly other bacterial pathogens that generate large amounts of toxic lysoPE.
Materials and methods
Bacteria and mammalian cell culture
C. jejuni wildtype strain 81116, originally isolated from a human waterborne outbreak,Citation33 its isogenic pldA mutant (C. jejuni ΔpldA), and the complemented pldA mutant (C. jejuni ΔpldA +pldA)Citation12 were routinely grown on saponin agar as described.Citation12 HeLa cellsCitation34 and Caco-2 cells (ATCC-HTB-37) were grown in 25 cm2 flasks in Dulbecco’s modified Eagle’s medium (DMEM) containing 10% fetal calf serum (FCS) at 37°C and 10% CO2.
Membrane isolation, lipid LC-MS/MS analysis, and extraction
C. jejuni membranes were collected by N-lauroylsarcosine assay,Citation35 using sonication and Tris (PH 8.0) buffer instead of French pressure cell press and HEPES buffer, respectively. Lipid extraction and analysis were done as described before.Citation12 For more information on membrane isolation and lipid extraction and analysis see supplementary materials (Supplementary Material 1). Commercial (lyso)phospholipids, lysoPE 14:0, lysoPE 16:0, lysoPE 18:0, lysoPG 14:0, lysoPG 18:0 and PE 16:0 were purchased from Avanti Polar Lipids Inc. (Alabama, USA) to investigate the effect of the length of LPL fatty acid tail on biological functions.
Hemolysis and cytotoxicity assays
Hemolysis and cytotoxicity were determined as describedCitation24 using heat-treated (75°C, 30 min) bacteria,Citation36 sonicated (3 × 60s) bacteria, isolated membranes, 10 μmol C. jejuni purified LPLs or 50 μmol commercial LPLs. Hemolysis was expressed as percentage of cell lysis (absorbance OD420) compared to the positive control (cells lysed with milliQ water). Host cell cytotoxicity was determined by measurement of the lactate dehydrogenase (LDH) release from 106 tissue culture cells at 5 h after addition of C. jejuni at a bacteria to host cell ratio of 100:1, or of the indicated amount of LPL. When appropriate, host cells were pre-treated (16 h) with one of the antioxidants, vitamin E and N,N’-diphenyl-1,4-phenylenediamine (DPPD) (Sigma-Aldrich).Citation32 For more detailed information on hemolysis and cytotoxicity assays see Supplementary Material 1. Data are expressed as the mean ±SEM of at least three independent experiments. Statistical significance was determined using two-way ANOVA analysis with Geisser-Greenhouse correction using Prism software (GraphPad, San Diego, CA).
Confocal microscopy
Confocal microscopyCitation37 was performed on cells (106) incubated (5 h) with commercial lysoPE 14:0 or lysoPG 14:0 to visualize the lysoPE-induced cell damage. When appropriate antioxidants were added 16 h before LPL treatment and washed away before lysoPE exposure. Cells were fixed and membranes were stained with plasma membrane counterstain Wheat Germ Agglutinin (WGA) Alexa Fluor™ 488 Conjugate (W11261, Invitrogen). Nucleic acids were stained with DAPI (D21490, Invitrogen) without permeabilization. Images were collected on a Leica SPE-II confocal microscope.
Supplemental Material
Download Zip (17.8 MB)Disclosure statement
No potential conflict of interest was reported by the author(s).
Data availability statement
The authors confirm that the data supporting the findings of this study are available within the article and its supplementary materials.
Supplementary material
Supplemental data for this article can be accessed online at https://doi.org/10.1080/19490976.2022.2091371
Additional information
Funding
References
- Li YF, Li RS, Samuel SB, Cueto R, Li XY, Wang H, Yang XF. Lysophospholipids and their G protein-coupled receptors in atherosclerosis. Front Biosci. 2016 Landmark Ed;21(1):70–10. doi:10.2741/4377.
- Zou D, Pei J, Lan J, Sang H, Chen H, Yuan H, Wu D, Zhang Y, Wang Y, Wang D, et al. A SNP of bacterial blc disturbs gut lysophospholipid homeostasis and induces inflammation through epithelial barrier disruption. EBioMedicine. 2020;52:102652. doi:10.1016/j.ebiom.2020.102652.
- Zheng L, Lin Y, Lu S, Zhang J, Bogdanov M. Biogenesis, transport and remodeling of lysophospholipids in Gram-negative bacteria. Biochim Biophys Acta Mol Cell Biol Lipids. 2017;1862(11):1404–1413. doi:10.1016/j.bbalip.2016.11.015.
- Schmiel DH, Miller VL. Bacterial phospholipases and pathogenesis. Microbes Infect. 1999;1(13):1103–1112. doi:10.1016/S1286-4579(99)00205-1.
- Zarringhalam K, Zhang L, Kiebish MA, Yang K, Han X, Gross RW, Chuang J. Statistical analysis of the processes controlling choline and ethanolamine glycerophospholipid molecular species composition. PLoS One. 2012;7(5):e37293. doi:10.1371/journal.pone.0037293.
- Meylaers K, Clynen E, Daloze D, DeLoof A, Schoofs L. Identification of 1-lysophosphatidylethanolamine (C16:1) as an antimicrobial compound in the housefly, Musca domestica. Insect Biochem Mol Biol. 2004;34(1):43–49. doi:10.1016/j.ibmb.2003.09.001.
- Gotoh M, Fujiwara Y, Yue J, Liu J, Lee S, Fells J, Uchiyama A, Murakami-Murofushi K, Kennel S, Wall J, et al. Controlling cancer through the autotaxin-lysophosphatidic acid receptor axis. Biochem Soc Trans. 2012;40(1):31–36. doi:10.1042/BST20110608.
- Subramanian N, Qadri A. Lysophospholipid sensing triggers secretion of flagellin from pathogenic Salmonella. Nat Immunol. 2006;7(6):583–589. doi:10.1038/ni1336.
- Giles DK, Hankins JV, Guan Z, Trent MS. Remodelling of the Vibrio cholerae membrane by incorporation of exogenous fatty acids from host and aquatic environments. Mol Microbiol. 2011;79(3):716–728. doi:10.1111/j.1365-2958.2010.07476.x.
- Bill M, Brinkmann S, Oberpaul M, Patras MA, Leis B, Marner M, Maitre M, Hammann PE, Vilcinskas A, Schuler SMM, et al. Novel glycerophospholipid, lipo- and N-acyl amino acids from Bacteroidetes: isolation, structure elucidation and bioactivity. Molecules. 2021;26(17):5195. doi:10.3390/molecules26175195.
- Kim H, Kim M, Myoung K, Kim W, Ko J, Kim KP, Cho E. Comparative lipidomic analysis of extracellular vesicles derived from Lactobacillus plantarum APsulloc 331261 living in green tea leaves using liquid chromatography-mass spectrometry. Int J Mol Sci. 2020;21:8076.
- Cao X, Brouwers J, van Dijk L, van de Lest C, Parker C, Huynh S, van Putten J, Kelly DJ, Wosten MM. The unique phospholipidome of the enteric pathogen Campylobacter jejuni: lysophosholipids are required for motility at low oxygen availability. J Mol Biol. 2020;432(19):5244–5258. doi:10.1016/j.jmb.2020.07.012.
- Burnham PM, Hendrixson DR. Campylobacter jejuni: collective components promoting a successful enteric lifestyle. Nat Rev Microbiol. 2018;16(9):551–565. doi:10.1038/s41579-018-0037-9.
- Lobo de Sa FD, Schulzke JD, Bucker R. Diarrheal mechanisms and the role of intestinal barrier dysfunction in Campylobacter infections. Curr Top Microbiol Immunol. 2021;431:203–231. doi:10.1007/978-3-030-65481-8_8.
- Bouwman LI, de Zoete MR, Bleumink-Pluym NM, Flavell RA, van Putten JP, de Zoete MR. Inflammasome activation by campylobacter jejuni. J Immunol. 2014;193(9):4548–4557. doi:10.4049/jimmunol.1400648.
- Elmi A, Nasher F, Dorrell N, Wren B, Gundogdu O. Revisiting Campylobacter jejuni virulence and fitness factors: role in sensing, adapting, and competing. Front Cell Infect Microbiol. 2021;10:607704. doi:10.3389/fcimb.2020.607704.
- Istivan TS, Coloe PJ, Fry BN, Ward P, Smith SC. Characterization of a haemolytic phospholipase A2 activity in clinical isolates of Campylobacter concisus. J Med Microbiol. 2004;53(6):483–493. doi:10.1099/jmm.0.45554-0.
- Kim EA, Kim JA, Park MH, Jung SC, Suh SH, Pang MG, Kim YJ. Lysophosphatidylcholine induces endothelial cell injury by nitric oxide production through oxidative stress. J Matern Fetal Neonatal Med. 2009;22(4):325–331. doi:10.1080/14767050802556075.
- Tang X, Wang W, Hong G, Duan C, Zhu S, Tian Y, Han C, Qian W, Lin R, Hou X. Gut microbiota-mediated lysophosphatidylcholine generation promotes colitis in intestinal epithelium-specific Fut2 deficiency. J Biomed Sci. 2021;28:20–z.
- Weiler AJ, Spitz O, Gudzuhn M, Schott-Verdugo SN, Kamel M, Thiele B, Streit WR, Kedrov A, Schmitt L, Gohlke H, et al. A phospholipase B from Pseudomonas aeruginosa with activity towards endogenous phospholipids affects biofilm assembly. Biochim Biophys Acta Mol Cell Biol Lipids. 2022;1867(4):159101. doi:10.1016/j.bbalip.2021.159101.
- Brok RG, Boots AP, Dekker N, Verheij HM, Tommassen J. Sequence comparison of outer membrane phospholipases A: implications for structure and for the catalytic mechanism. Res Microbiol. 1998;149(10):703–710. doi:10.1016/S0923-2508(99)80017-5.
- Fuller N, Rand RP. The influence of lysolipids on the spontaneous curvature and bending elasticity of phospholipid membranes. Biophys J. 2001;81(1):243–254. doi:10.1016/S0006-3495(01)75695-0.
- Pickett CL, Auffenberg T, Pesci EC, Sheen VL, Jusuf SS. Iron acquisition and hemolysin production by Campylobacter jejuni. Infect Immun. 1992;60(9):3872–3877. doi:10.1128/iai.60.9.3872-3877.1992.
- Bleumink-Pluym NM, van Alphen LB, Bouwman LI, Wosten MM, van Putten JP. Identification of a functional type VI secretion system in Campylobacter jejuni conferring capsule polysaccharide sensitive cytotoxicity. PLoS Pathog. 2013;9:e1003393. doi:10.1371/journal.ppat.1003393.
- Wassenaar TM. Toxin production by Campylobacter spp. Clin Microbiol Rev. 1997;10(3):466–476. doi:10.1128/CMR.10.3.466.
- Golan DE, Brown CS, Cianci CM, Furlong ST, Caulfield JP. Schistosomula of Schistosoma mansoni use lysophosphatidylcholine to lyse adherent human red blood cells and immobilize red cell membrane components. J Cell Biol. 1986;103(3):819–828. doi:10.1083/jcb.103.3.819.
- Weltzien HU. Cytolytic and membrane-perturbing properties of lysophosphatidylcholine. Biochim Biophys Acta. 1979;559(2–3):259–287. doi:10.1016/0304-4157(79)90004-2.
- Morrison DJ, Preston T. Formation of short chain fatty acids by the gut microbiota and their impact on human metabolism. Gut Microbes. 2016;7(3):189–200. doi:10.1080/19490976.2015.1134082.
- Callahan S, Doster RS, Jackson JW, Kelley BR, Gaddy JA, Johnson JG. Induction of neutrophil extracellular traps by Campylobacter jejuni. Cell Microbiol. 2020;22(8):e13210. doi:10.1111/cmi.13210.
- Grant KA, Belandia IU, Dekker N, Richardson PT, Park SF. Molecular characterization of pldA, the structural gene for a phospholipase A from Campylobacter coli, and its contribution to cell-associated hemolysis. Infect Immun. 1997;65(4):1172–1180. doi:10.1128/iai.65.4.1172-1180.1997.
- Arouri A, Mouritsen OG. Membrane-perturbing effect of fatty acids and lysolipids. Prog Lipid Res. 2013;52(1):130–140. doi:10.1016/j.plipres.2012.09.002.
- Colles SM, Chisolm GM. Lysophosphatidylcholine-induced cellular injury in cultured fibroblasts involves oxidative events. J Lipid Res. 2000;41(8):1188–1198. doi:10.1016/S0022-2275(20)33425-8.
- Palmer SR, Gully PR, White JM, Pearson AD, Suckling WG, Jones DM, Rawes JC, Penner JL. Water-borne outbreak of campylobacter gastroenteritis. Lancet. 1983;1(8319):287–290. doi:10.1016/S0140-6736(83)91698-7.
- Rodriguez MS, Thompson J, Hay RT, Dargemont C. Nuclear retention of IκBα protects it from signal-induced degradation and inhibits nuclear factor κB transcriptional activation. J Biol Chem. 1999;274(13):9108–9115. doi:10.1074/jbc.274.13.9108.
- Hobb RI, Fields JA, Burns CM, Thompson SA. Evaluation of procedures for outer membrane isolation from Campylobacter jejuni. Microbiology (Reading). 2009;155(3):979–988. doi:10.1099/mic.0.024539-0.
- Groh CD, MacPherson DW, Groves DJ. Effect of heat on the sterilization of artificially contaminated water. J Travel Med. 1996;3(1):11–13. doi:10.1111/j.1708-8305.1996.tb00689.x.
- Li X, Wubbolts RW, Bleumink-Pluym NMC, van Putten JPM, Strijbis K. The transmembrane mucin MUC1 facilitates beta1-integrin-mediated bacterial invasion. mBio. 2021;12(2). doi:10.1128/mBio.03491-20.