ABSTRACT
Despite significant achievements in hematopoietic stem cell transplantation (HSCT), graft-versus-host disease (GVHD), especially intestinal GVHD, remains a major obstacle to this procedure. GVHD has long been regarded as a pathogenic immune response, and the intestine has been simply considered as a target of immune attack. In effect, multiple factors contribute to intestinal damage after transplantation. Impaired intestinal homeostasis including altered intestinal microbiome and epithelial damage results in delayed wound healing, amplified immune response and sustained tissue destruction, and it may not fully recover following immunosuppression. In this review, we summarize factors leading to intestinal damage and discuss the relationship between intestinal damage and GVHD. We also describe the great potential of remodeling intestinal homeostasis in GVHD management.
Introduction
Allogeneic hematopoietic stem cell transplantation (allo-HSCT) has been established as an effective therapy for hematological malignancies. The reconstituted immune system after transplantation is efficient to eliminate malignant cells, while it may lead to targeted host tissue injuries termed as graft-versus-host disease (GVHD).Citation1 The pathology of GVHD can be categorized into three steps: first, conditioning regimens cause tissue damage, in which the release of damage-associated molecular patterns (DAMPs), pathogen-associated molecular patterns (PAMPs), together with abundant pro-inflammatory cytokines activate the innate immune system; subsequently, host antigen-presented antigen-presenting cells (APCs) activate donor T cells, leading to proliferation, differentiation, migration and secretion of cytokines; finally, allogeneic T cells infiltrate and damage the target organs.Citation2,Citation3
The intestine not only is the major target of GVHD but also modulates the development of systemic GVHD.Citation2 The intestinal epithelium serves as the major site of nutrient digestion and absorption, and it is also crucial for preventing pathogen invasion and generating tolerance to intestinal microbes. In turn, microbes residing in the intestinal tract are tightly regulated, and their metabolic products benefit intestinal growth and shape mucosal immunity.Citation4,Citation5 While in the context of GVHD, intestinal barrier loss and dysbiosis break the balance of the intestinal homeostasis and activate the immune system ().Citation6–8
Figure 1. Intestinal epithelium in homeostasis and GVHD. a. The intestinal epithelium comprises crypts and villus. A mucous layer separates epithelial cells and intestinal bacteria, which is indispensable to intestinal homeostasis. ISCs give rise to all kinds of epithelial cells, and the activities of ISCs are tightly regulated by various cells and signals; b. Conditioning regimens induce epithelial damage and dysbiosis. Antibiotics and diet further affect the microbiome components and metabolites. Accumulated DAMPs and PAMPs activate the innate immune system and trigger donor lymphocyte infiltration, leading to impaired ISC niche and sustained tissue damage.(ISC, intestinal stem cell; EP, enterocyte progenitor; SP, secretory progenitor; EC, enterocyte; PC, Paneth cell; GC, goblet cell; EEC, enteroendocrine cell; TC, tuft cell; Mø, macrophage; Neu, neutrophil; Lym, lymphocyte; APC, antigen-presenting cell; SCFA, short-chain fatty acid; BA, bile acid; TMAO, trimethylamine N-oxide; DAMPs, damage-associated molecular patterns; PAMPs, pathogen-associated molecular patterns.).
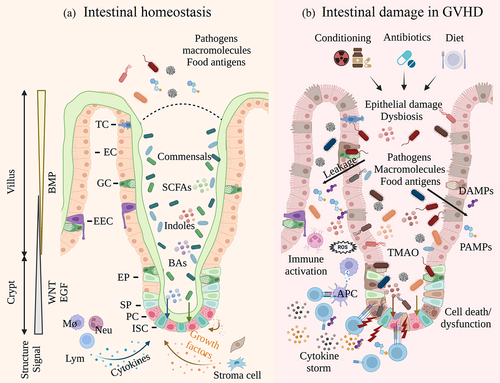
At present, immunosuppressive agents remain the preferred choice for GVHD treatment. With an evolving knowledge of T cell biology, therapeutics develop from broad immune suppression toward precision medicine, such as Ruxolitinib, a selective JAK1/2 inhibitor.Citation9 Notwithstanding these advances, treatment resistance still occurs in a large proportion of patients (especially in patients with intestinal GVHD) and emerges as a new challenge.Citation10 Indeed, the disturbance in intestinal ecosystem still exists after immunosuppression. Remodeling intestinal homeostasis, a field that we have long neglected, may become an important complementary strategy in GVHD management.Citation11
Here, we review the factors that leading to intestinal damage during HSCT. We also scrutinize the novel approaches to promoting tissue repair and intestinal flora intervention.
Intestinal damage in GVHD
Damage to the intestinal microbiome
The intestinal epithelium harbors large communities of microbes, including bacteria, archaea, fungi and viruses. Bacteria, the major of which belong to the Firmicutes and Bacteroidetes phyla and the minority of which belong to Proteobacteria, Actinobacteria, Fusobacteria and Verrucomicrobia phyla, represent the largest group within the intestinal microbes.Citation7,Citation8 However, several factors such as conditioning regimens, antibiotic exposure and dietary changes contribute to the flora disturbance and exacerbate intestinal GVHD.
Conditioning regimens
Based on 16S rRNA gene sequencing, several preclinical studies have investigated the impact of cancer therapy on fecal flora. Most of these studies observed a reduced diversity of intestinal bacteria after radiotherapy or chemotherapy.Citation12–18 The major components of intestinal bacteria, Firmicutes Citation12–14,Citation19,Citation20 or Bacteroidetes Citation14,Citation18,Citation19,Citation21–23, are more likely to decrease, while the proportions of Proteobacteria Citation13,Citation22,Citation23 and Verrucomicrobia Citation12,Citation13,Citation20 tend to increase. On the genus level, Clostridium Citation12,Citation13, Lactobacillus Citation15,Citation19,Citation22,Citation23 Ruminococcus Citation13 and Blautia Citation22 may account for the loss of Firmicute, and Bacteroides may account for the decreased abundance of Bacteroidetes. Citation19,Citation21,Citation23 Meanwhile, some opportunistic pathogens increase after cancer therapy, such as Akkermansia belonging to Verrucomicrobia,Citation13,Citation19,Citation20 and Enterococcus belonging to Firmicutes.Citation14,Citation22,Citation24 Moreover, the perturbation of intestinal microbiome increases inflammatory response and amplifies tissue damage. Using bacterial–epithelial coculture, radiation-altered microbes enhanced the secretion of IL-1β and TNF-α from HT29 epithelial cells. Mice inoculated with irradiated microbes also exhibited more severe intestinal damage compared to those which received naïve microbes.Citation13
In clinical studies, the stool specimens collected from patients with hematological malignancies had significantly lower microbial diversity than healthy controls.Citation25–28 Chemotherapy further disrupted the bacteria diversity and its componentsCitation26,Citation28–32, which was characterized by steep decrease in proportions of Blautia Citation31,Citation32 and Clostridium Citation30,Citation32,Citation33, and an expansion of Enterococcus Citation28,Citation30,Citation33,Citation34.
Altogether, conditioning regimens dramatically shift the structure of the intestinal microbes.
Antibiotics
The intestine is a strict anaerobic environment under normal circumstances. Therefore, most intestinal bacteria are anaerobic, and they are 100–1000 times numerous than aerobic bacteria or facultative anaerobes (e.g., Enterococcus).Citation7,Citation35 During HSCT, antibiotics are commonly prescribed to prevent bacterial infection. Nevertheless, antibiotics are a double-edged sword. Broad-spectrum antibiotics and heavy antibiotic exposure may shift the bacterial profiles by depleting commensal bacteria and inducing the expansion of pathogenic populations.
In murine transplant models, several studies have investigated the antibiotics’ effect on intestinal flora and GVHD. Ampicillin, imipenem-cilastatin, meropenem, etc., were used as broad-spectrum antibiotics. Aztreonam, cefepime, etc., were antibiotics with minor anaerobic activities. In these studies, broad-spectrum antibiotics increased epithelial damage and the GVHD-related mortality rate. Changes in bacterial compositions revealed that broad-spectrum antibiotics suppress the anaerobic commensals such as Lactobacillus, Clostridia, Blautia, and increase the abundance of pathogens like Enterococcus and mucus-degrading microbes such as Akkermansia muciniphila and Bacteroides thetaiotaomicron.Citation36–38 Enterococcus activates the mucosal immune system and secretes metalloprotease that impairs the epithelial barrier.Citation36 Mucus-degrading bacteria also compromise the mucus layer and increase intestinal permeability.Citation37,Citation38 Conversely, antibiotics with reduced activities against anaerobes better preserved the intestinal bacterial diversity and spared more commensal bacteria.
The results from patients were similar to those from mice. Metronidazole, a drug highly active against anaerobes, increased the risk of Enterococcus dominance by threefold.Citation39 Broad-spectrum antibiotics or antibiotics that inhibit anaerobes exacerbated the loss of Blautia Citation40, Lactobacillus Citation37, Bacteroidetes Citation37,Citation41 and Clostridiales Citation42,Citation43 (Clostridium cluster XIVa)Citation44. An increased proportion of Enterococcus was more prominent in patients developing GVHD,Citation45,Citation46 and the abundance of Blautia was negatively related to GVHD-related mortality.Citation41,Citation46 Apart from this, numerous clinical studies indicated that antibiotic exposure during HSCT aggravated the incidence or severity of GVHD.Citation37,Citation41–43,Citation47–51 Early and prolonged use of antibiotics even induced more severe dysbiosis.Citation42,Citation48,Citation52
In general, irrational use of antibiotics, especially broad-spectrum antibiotics, may contribute to dysbiosis and increase the risk of GVHD.
Diet and nutrient
Diet provides energy and nutrient, it also regulates the intestinal microbial ecology and the host-microbes interactions.Citation53,Citation54
Oral and gastrointestinal mucositis frequently prevent eating and drinking in tumor treatment. To meet nutrient requirements, patients often receive enteral nutrition (EN) or parenteral nutrition (PN) during HSCT. Compared with PN, EN was associated with a reduced risk of GVHD.Citation40,Citation55–58 Besides, patients in the EN group showed advantages in neutrophil engraftmentCitation56, infection controlCitation55,Citation59 and overall survivalCitation55–58 over those who received PN. Compared with patients who received EN, PN resulted in loss of Blautia, Dorea and Bacteroides (accompanied by decreased short-chain fatty acid (SCFA) production), and an increase in Streptococcus, which possibly aggravates the development of GVHD.Citation40,Citation60
More recently, experiments have demonstrated that some dietary components directly affect the intestinal microbial structure and influence the risk of GVHD. Serum level of high stearic acid is a reliable biomarker for predicting GVHD in patients receiving HSCT. In murine aGVHD models, a high stearic acid diet aggravated GVHD, resulting in loss of commensals and enrichment of Akkermansia. Mice fed with a diet including Akkermansia muciniphila exacerbated GVHD, while antibiotics against Akkermansia muciniphila attenuated GVHD.Citation61 Enterococcus dominance, another potential driver of GVHD, frequently occurred in mice and humans suffering from GVHD. As Enterococcus depends on lactose and galactose metabolism, dietary lactose depletion in mice suppressed Enterococcus and ameliorated the severity of GVHD.Citation62 Besides, a high-fat diet and obesity in mice led to loss of Clostridiaceae, and expansion of Akkermansia muciniphila and Enterococcus, which may explain recipients with high body mass index are more susceptible to GVHD.Citation63 Tyrosine supplement in diet restored the intestinal microbes (e.g., the recovery of Lachnospiraceae) and ameliorated GVHD in murine models. Tyrosine deprivation worsened the GVHD-related outcomes.Citation64 In another report, Lachnospiraceae was found to accumulate in leukemia patients post-radiation and support gastrointestinal repair.Citation65
Damage to the intestinal epithelial cells (IECs)
The epithelial cell components originate from Lgr5+ ISCs, which reside at the bottom of the crypt. ISCs proliferate and give rise to progenitor cells, the latter cells further differentiate into enterocytes (ECs) that take charge of nutrient absorption, Paneth cells (PCs) that secrete antimicrobial peptides and lysozymes, goblet cells (GCs) that secrete mucus, enteroendocrine cells (EECs) that release hormones, etc. The intestinal epithelial barrier, which consists of different IECs with intact tight junctions and a chemical barrier that includes mucus and antibacterial substances, is critical for maintaining intestinal homeostasis.Citation4
Conditioning regimens
Conditioning regimens are often used to deplete tumor cells and vacate bone marrow stem cell niches. The intestinal epithelium is extremely vulnerable to conditioning toxicity due to its high cellular turnover. Conditioning regimens prefer to kill crypt cells, leading to considerable cell death and reduced cell production, which is characterized by crypt hypoplasia, shortened villus and impaired tight junctions.Citation66–68 The loss of IECs results in impaired absorptive activity,Citation69 and also disturbs the intestinal integrity with elevated endotoxin translocation and macromolecule leakage.Citation69–71 Intestinal toxicity positively correlates with the conditioning intensity and predicts GVHD severity.Citation71,Citation72
To explore these further, transgenic mice with improved intestinal integrity were studied in intestinal damage and GVHD. The Tg222 mice have a strengthened mucus layer. After intensive chemotherapy, Tg222 mice had lower tissue damage and better-preserved intestinal microbes than wild-type mice, which was linked to reduced bacterial translocations.Citation24 Cytoskeletal contraction, a process regulated by long myosin light chain kinase (MLCK210), is one of the major mechanisms of tight junction disruption. MLCK210 expression and myosin light chain phosphorylation increase in intestinal epithelium suffering from GVHD. Genetic knockout of MLCK210 promoted intestinal barrier recovery from radiation, prevented T cell infiltration and limited GVHD propagation.Citation73
Dysregulated microbial metabolites
Previously, we describe the impact of diet on intestinal microbes. On the other hand, intestinal microbes metabolize dietary components and secrete metabolites, which have a profound impact on human health.Citation53 Diet, microbial signatures and metabolite profiles are complex and closely related. For example, most genera in the Firmicutes prefer dietary protein that provides branched-chain fatty acids and aromatic metabolites, while Clostridium avidly uses dietary fiber as a carbon source; Bacteroides prefer dietary fiber over dietary protein, in contrast to other genera in Bacteroidetes.Citation54 Among diverse microbial metabolites, SCFAs, bile acids (BAs) and indole derivatives play vital roles in maintaining intestinal homeostasis.
SCFAs are 1–6 carbon volatile fatty acids that are mostly derived from dietary fibers.Citation54 Butyrate, the best-studied SCFA by far, can be produced by families belonging to the Clostridiales order such as Lachnospiraceae, Ruminococcaceae, Erysipelotrichaceae, and genera like Clostridium, Dorea, Blautia and Roseburia. Butyrate has diverse impacts on intestinal cells and host functions. First, butyrate can be metabolized via fatty acid oxidation and becomes the major energy source for IECs. Besides, butyrate serves as a ligand for G protein-coupled receptors (GPCR) and aryl hydrocarbon receptors (AhR). Acetyl-CoA which is metabolized from butyrate also takes part in transcriptional regulations by enhancing histone acetylation.Citation74 Previously, accumulating evidence has identified a reduced level of butyrate or butyrogenic bacteria in patients suffering from GVHD.Citation40,Citation48,Citation52,Citation75,Citation76 In murine studies, researchers also found butyrate decreased in intestinal tissue and stools after allo-HSCT, together with the reduced level of histone acetylation in IECs. Intragastric gavage of butyrate in recipients restored the loss of butyrate and histone acetylation in IECs, accompanied by a protective effect in GVHD. Recipients treated with Clostridiales also showed an increased level of butyrate and attenuated GVHD. Mechanistically, histone acetylation directly acts on IECs to suppress the expression of apoptosis-related genes, and upregulate the production of junction proteins. In vitro, butyrate enhanced the survival and growth of intestinal organoids, and butyrate-pretreated organoids were more tolerant when cocultured with allogenic T cells.Citation77
As a downstream metabolite of cholesterol, BAs can be classified into two groups. Primary BAs (PBAs) that are conjugated with taurine or glycine are synthesized in the liver. When released in the intestine, 95% of PBAs recirculate to the liver by enterohepatic circulation, the rest are modified by bacteria secreting bile acid hydrolase (such as Bacteroides, Clostridium, Lactobacillus, Bifidobacterium and Enterococcus) to secondary BAs (SBAs). Except for facilitating the digestion and absorption of dietary lipids, BAs trigger inhibitory or active signals by binding farnesoid X receptor (FXR) and G protein-coupled bile acid receptor-1 (Gpbar-1 or TGR5).Citation78 For example, deoxycholic acid (DCA), also known as a strong FXR antagonist, induces intestinal inflammation and promotes stem cell expansion.Citation79,Citation80 Lithocholic acid (LCA), another SBA, activates the TGR5 and stimulates the proliferation of ISCs and intestinal regeneration.Citation81 BA-dysmetabolism has been linked with many diseases such as inflammatory bowel disease (IBD), which is characterized by reduced levels of SBAs in stool samples.Citation82,Citation83 In a murine model, GVHD disrupted TGR5 and reduced the levels of BAs. Using cytokine-organoid coculture, TNF and IFN-γ killed cells and prevented intestinal organoid growth. Adding BAs to this coculture system, especially an SBA named tauroursodeoxycholic acid (TUDCA), rescued the intestinal organoid from cytokine-mediated cytotoxicity. In vivo, pre-administration of TUCDA attenuated GVHD, probably due to decreased intestinal antigen presentation and reduced T cell activation. ISCs and PCs were also better preserved as TUCDA downregulated the apoptosis-related genes.Citation84 Ursodeoxycholic acid (UDCA), another SBA used in several clinical trials, reduced the incidence of GVHD.Citation85,Citation86
Tryptophan is an essential amino acid for humans. Tryptophan can be metabolized by both epithelial cells and immune cells via the kynurenine (Kyn) pathway, and it is also degraded by intestinal bacteria into indoles and indole derivatives. Many indole derivatives are ligands for AhR or pregnane X receptor (PXR), including indoleacrylic acid (IA), indole-3-aldehyde (IAld), indole-3-acid-acetic (IAA), indole-3-propionic acid (IPA), etc.Citation87 Activation of AhR or PXR promotes barrier integrity, partly by inducing GC differentiation and maintaining tight junctions.Citation88–90 In GVHD, a new protective mechanism of indole derivatives has been found, in which indole-3-carboxaldehyde (ICA) activates the type-I IFN signal, limits radiation damage and promotes epithelial regeneration.Citation91
In addition, mounting evidence suggests that metabolites can regulate immune cell activities.Citation92 For example, butyrate acts on T cells and increases H3 acetylation in the locus of Foxp3, thereby promoting the differentiation of regulatory T cells (Tregs).Citation93 LCA metabolites (3-oxoLCA and isoalloLCA) regulate host immune responses by modulating Th17 and Tregs differentiation.Citation94 Activated AhR promotes IL-22 production from lamina propria lymphocytes and accelerates intestinal epithelial proliferation.Citation95 Moreover, some metabolites even accelerate GVHD, such as trimethylamine N-oxide (TMAO) and retinoic acid.Citation96,Citation97
Immune cell infiltration
Immune cell activation and infiltration mediate sustained tissue damage. Here, we describe that immune responses are closely related to disturbed intestinal homeostasis, allowing immune cells to invade and further destroy ISCs and the ISC niche.
As mentioned before, conditioning regimens cause tissue damage and bacterial translocation. DAMPs, PAMPs, as well as a bunch of cytokines rapidly accumulate and recruit neutrophils to the damaged intestine.Citation3,Citation98,Citation99 In contrast, germ-free condition reduces the level of neutrophil migration.Citation98 Neutrophils amplify tissue damage by releasing ROS, and they also express high levels of MHC-II and contribute to T cell activation. Defect in ROS production or lacking TLR compromises neutrophil function and reduces GVHD severity.Citation98,Citation99 Besides, microbiome regulates MHC-II expression on IECs. Upon radiation or dysbiosis, the level of MHC-II on IEC elevates, and these MHC-II expressing IECs activate T cells and initiate GVHD. Meanwhile, MHC-II is absent in IECs of germ-free mice, even after radiation. Specific deletion of MHC-II blocks T cell activation and the subsequent GVHD.Citation100
Once activated, T cells migrate to the target organs and mediate killing effects. Early after HSCT, T cell density increases throughout the intestinal epithelium. Notably, the crypt region where MAdCAM-1+ vessels located are is the first and primary target of immune attack, resulting in rapid loss of ISCs before tissue destruction.Citation101 PCs and GCs are also targets of GVHD, leading to flora disturbance, increased bacterial translocation and amplified GVHD severity.Citation102–104 Besides, innate lymphoid cells (ILCs) promote ISC regeneration via secretion of IL-22. Depletion of host ILCs and IL-22 by donor T cells dampens tissue healing and boosts the development of GVHD.Citation105–107
Additionally, T cells regulate ISC function through variant cytokines. In a murine T cell/cytokine-organoid coculture system, Tregs and IL-10 maintain ISC self-renew, while T helper (Th) cells and their cytokines (IFN-γ, IL-13 and IL-17A) promote ISC differentiation.Citation108 Interferon regulatory factor 2 (IRF2) is a negative regulator of the IFN signal. Mice completely lacking IRF2 or with a selective IRF2 deletion in IECs have significantly fewer ISCs and accumulated immature PCs.Citation109 Excessive IFN also activates the JAK/STAT pathway, promoting proapoptotic gene expression and depleting ISCs and PCs.Citation110 Ruxolitinib, a JAK1/2 inhibitor, prevents T cell-mediated damage and restores the loss of ISCs and PCs.Citation110,Citation111 Enrichment of another cytokine TNF-α enhances ISC expansion but induces differentiated cell necroptosis.Citation112
Interplay between intestinal damage and GVHD
Peri-transplantation treatments break tissue homeostasis and immune tolerance. Epithelial damage and dysbiosis lead to massive leakage of macromolecules, bacterial products and food antigens, which further accelerates antigen presentation, T cell accumulation and GVHD propagation. Moreover, disturbances in metabolite profiles and cytokines result in cellular dysfunction, both in epithelial cells and immune cells. Altogether, the above results suggest that intestinal damage and GVHD are tightly linked and mutually facilitated.
Novel approaches targeting the intestinal ecosystem
The self-renew and differentiation of ISCs are precisely regulated by signals from the basal side including Wnt (stem cell maintenance and proliferation), Notch (lineage decision) and BMP (negative regulation of crypt proliferation), and signals from the apical side such as microbes and dietary components.Citation113 ISCs are also affected by immune cells and metabolism.Citation108,Citation114 The development of GVHD not only destroys the cellular components but also disrupts the complex regulatory network. Given that immunosuppression has not fully addressed GVHD, strategies that preserve the intestinal ecosystem should catch enough attention ().Citation9,Citation11,Citation115
Promoting tissue repair
Pretransplant conditioning and GVHD lead to a sharp reduction in the ISC pool. Restoring ISCs may promote tissue repair and limit GVHD development.
R-spondin1 has been identified as a potent Wnt agonist, which stabilizes β-catenin and activates the downstream genes.Citation116 In murine transplant models, injection of R-spondin1 significantly ameliorated GVHD. Mechanistically, R-spondin1 boosts crypt cell expansion and increases the number of ISCs after radiation.Citation117 Moreover, R-spondin1 promotes PC differentiation. The increased production of α-defensins from PCs prevents dysbiosis and restores bacterial diversity.Citation118 Consistently, Reg3γ is an antibacterial protein that is secreted by PCs and is negatively related to GVHD severity. IL-22 administration restores the loss of Reg3γ and prevents the apoptosis of ISCs and PCs.Citation119 IL-22 also enhances ISC recovery via STAT3 phosphorylation.Citation120 Combination of IL-22 and systemic corticosteroids showed good clinical efficacy as an initial treatment for lower intestinal GVHD, as 70% (19/27) of patients achieved a day-28 treatment response with an increased fecal microbial diversity.Citation121 Additionally, lithium stimulates Wnt signal via GSK3 inhibition and drives epithelial regeneration.Citation122 Teduglutide, a GLP-2 agonist, promotes the expansion of PCs and ISCs and improves the microbiome diversity.Citation123 IL25, a growth factor of GCs, inhibits bacterial translocation and attenuates GVHD.Citation104 IFN-I and RIG-I signals have also been reported to fuel ISC regeneration and benefit GVHD control.Citation124,Citation125 It is worth noting that most of these drugs above do not impair T cell reconstitution and the graft versus leukemia (GVL) effect, indicating a non-immunosuppressive direction for GVHD management.
Intestinal flora intervention
Intestinal Flora disturbance in HSCT leads to immune activation and epithelial cell dysfunction, which finally fuel the development of GVHD. Interventions on microbes are widely investigated, including oral prebiotics/probiotics and fecal microbiota transplantation (FMT).
Probiotics are beneficial bacteria or yeast for the host, and prebiotics are digestive fibers that support probiotic colonization. Compared with historical control, clinical application of the prebiotic mixture GFO (glutamine, fiber and oligosaccharides) preserved the butyrate-producing bacteria, shortened the duration of diarrhea and reduced the risk of GVHD.Citation126 In another report, the microbial structure altered by FOS (fructo-oligosaccharides) could not be maintained after HSCT. No advantages in survival or GVHD were found in patients receiving FOS.Citation127 Besides, administration of Bacteroides fragilis and Lactobacillus rhamnosus GG through oral gavage reduced GVHD and improved survival in experimental models.Citation128,Citation129 However, Lactobacillus rhamnosus GG failed to reduce the incidence of GVHD in clinical trials.Citation130,Citation131
Although prebiotics/probiotics have clear constituents and controlled quality, FMT rapidly restores the composition and function of intestinal microbes in GVHD. In murine models, mice given FMT from healthy donors were characterized by reduced immune cell attack and GVHD severity.Citation129 Moreover, growing evidences suggest that either donor or third-party FMT is safe and effective for patients developing GVHD.Citation132–138 Most patients achieved clinical symptom improvement, and nearly 50–70% of the patients acquired clinical remission. Fecal samples after FMT displayed increased diversity and higher levels of beneficial bacteria. Microbiome functionality related to lipids, carbohydrates, amino acids, nucleotides and energy metabolism was also restored by FMT.Citation139 Additionally, only a small population of patients developed infection events that might have resulted from FMT. DeFilipp et al. reported that 1 out of 13 patients developed C. difficile colitis and 1 developed bacteremia.Citation133 In another study, the infection rate within the first month after FMT was 5/17, whereas none of the pathogens was detectable in the donor fecal sample.Citation134 Patients given FMT did not experience a higher infection rate compared to those without FMT (5/23 vs 7/18).Citation135
Moreover, patients suffering from tumors have massively different microbial structures from healthy people,Citation25–28 some commensals (such as Bifidobacterium, Ruminococcaceae and Bacteroides) promote the anti-tumor effect of immune checkpoint inhibitorsCitation140–142 or correlate with immune responses in chimeric antigen receptor T cell (CAR-T) therapies,Citation143,Citation144 suggesting that some species may fuel tumor growth or become powerful weapons against malignant cells. However, little research has explored the roles of these microbes in disease recurrence following HSCT. Only Peled, et al. reported a higher ambulance of Eubacterium limosum between day 0 and day 21 negatively correlated with relapse.Citation145 What’s the difference in microbial signature between relapsed patients and non-relapsed patients? Whether modifications of intestinal microbiome after HSCT improve the GVL effect require further research.
Outstanding issues and future perspective in intestinal GVHD
Despite progress with remodeling intestinal ecosystem in the treatment of GVHD, there are still many unknowns about the damaged ISC niche and its regulatory network. Except for the sharp decreased ISC pool in GVHD, the functional impairment of ISC may vary widely from mild cases to fatal outcomes, leading to distinct quantity, composition even function of IECs. The altered extracellular signal molecules, the disturbance of intracellular signal pathways, and the imbalance between cell proliferation and differentiation of ISC need to be answered in future research.
Additionally, 16S rRNA sequencing and metabolomics reveal that dysbiosis and dysregulated metabolites are closely related to the development of GVHD. However, intestinal flora intervention, especially prebiotics and probiotics, sometimes fails in GVHD treatment. One possible reason is that dysbiosis is not only affected by external factors but also by the internal interactions between different microbes. Therefore, not all aberrations in intestinal microbes are necessarily pathogenic. Selective removal or supplement of certain bacteria might not have a strong and long-lasting effect, as it could be interfered by other species or environmental factors. Uncovering interactions within microbiota community and remodeling microbial structure may ultimately improve microbial function and attenuate intestinal GVHD.
Conclusions
The intestine should not be merely considered as a target of immune attack. Many factors, especially epithelial damage and dysbiosis, are upstream regulators that influence the incidence and severity of GVHD. Since immunosuppression only partly resolves this disease, remodeling the intestinal ecosystem may overcome drug resistance and improve the therapeutic effect.
Author contributions
He Huang and Yanmin Zhao designed; Fei Gao performed the literature search and wrote this paper. All authors revised and approved the final manuscript.
Acknowledgments
Figures were created using BioRender (http://www.biorender.com). We really appreciate Runnan Chen for polishing the English of the final version.
Disclosure statement
No potential conflict of interest was reported by the authors.
Additional information
Funding
References
- Ferrara JL, Levine JE, Reddy P, Holler E. Graft-versus-host disease. Lancet. 2009;373(9674):1550–16. doi:10.1016/S0140-6736(09)60237-3.
- Zeiser R, Blazar BR, Longo DL. Acute graft-versus-host disease — biologic process, prevention, and therapy. N Engl J Med. 2017;377(22):2167–2179. doi:10.1056/NEJMra1609337.
- Martinez-Cibrian N, Zeiser R, Perez-Simon JA. Graft-versus-host disease prophylaxis: pathophysiology-based review on current approaches and future directions. Blood Rev. 2021;48:100792. doi:10.1016/j.blre.2020.100792.
- Allaire JM, Crowley SM, Law HT, Chang SY, Ko HJ, Vallance BA. The intestinal epithelium: central coordinator of mucosal immunity. Trends Immunol. 2018;39(9):677–696. doi:10.1016/j.it.2018.04.002.
- Kayama H, Okumura R, Takeda K. Interaction between the microbiota, epithelia, and immune cells in the intestine. Annu Rev Immunol. 2020;38(1):23–48. doi:10.1146/annurev-immunol-070119-115104.
- Nalle SC, Turner JR. Intestinal barrier loss as a critical pathogenic link between inflammatory bowel disease and graft-versus-host disease. Mucosal Immunol. 2015;8(4):720–730. doi:10.1038/mi.2015.40.
- Staffas A, Burgos da Silva M, van den Brink MR. The intestinal microbiota in allogeneic hematopoietic cell transplant and graft-versus-host disease. Blood. 2017;129(8):927–933. doi:10.1182/blood-2016-09-691394.
- Van Lier YF, Van den Brink MRM, Hazenberg MD, Markey KA. The post-hematopoietic cell transplantation microbiome: relationships with transplant outcome and potential therapeutic targets. Haematologica. 2021;106(8):2042–2053. doi:10.3324/haematol.2020.270835.
- Toubai T, Magenau J. Immunopathology and biology-based treatment of steroid-refractory graft-versus-host disease. Blood. 2020;136(4):429–440. doi:10.1182/blood.2019000953.
- Zeiser R, von Bubnoff N, Butler J, Mohty M, Niederwieser D, Or R, Szer J, Wagner EM, Zuckerman T, Mahuzier B, et al. Ruxolitinib for glucocorticoid-refractory acute graft-versus-host disease. N Engl J Med. 2020;382(19):1800–1810. doi:10.1056/NEJMoa1917635.
- Chakraverty R, Teshima T. Graft-versus-host disease: a disorder of tissue regeneration and repair. Blood. 2021;138(18):1657–1665. doi:10.1182/blood.2021011867.
- Goudarzi M, Mak TD, Jacobs JP, Moon BH, Strawn SJ, Braun J, Brenner DJ, Fornace AJ, Li H-H. An integrated multi-omic approach to assess radiation injury on the host-microbiome axis. Radiat Res. 2016;186(3):219–234. doi:10.1667/RR14306.1.
- Gerassy-Vainberg S, Blatt A, Danin-Poleg Y, Gershovich K, Sabo E, Nevelsky A, Daniel S, Dahan A, Ziv O, Dheer R, et al. Radiation induces proinflammatory dysbiosis: transmission of inflammatory susceptibility by host cytokine induction. Gut. 2018;67(1):97–107. doi:10.1136/gutjnl-2017-313789.
- Li Y, Yan H, Zhang Y, Li Q, Yu L, Li Q, Liu C, Xie Y, Chen K, Ye F, et al. Alterations of the gut microbiome composition and lipid metabolic profile in radiation enteritis. Front Cell Infect Microbiol. 2020;10:541178. doi:10.3389/fcimb.2020.541178.
- Zhao TS, Xie LW, Cai S, Xu JY, Zhou H, Tang LF, Yang C, Fang S, Li M, Tian Y, et al. Dysbiosis of gut microbiota is associated with the progression of radiation-induced intestinal injury and is alleviated by oral compound probiotics in mouse model. Front Cell Infect Microbiol. 2021;11:717636. doi:10.3389/fcimb.2021.717636.
- Zhou B, Xia X, Wang P, Chen S, Yu C, Huang R, Zhang R, Wang Y, Lu L, Yuan F, et al. Induction and amelioration of methotrexate-induced gastrointestinal toxicity are related to immune response and gut microbiota. EBioMedicine. 2018;33:122–133. doi:10.1016/j.ebiom.2018.06.029.
- Huang X, Fang Q, Rao T, Zhou L, Zeng X, Tan Z. Leucovorin ameliorated methotrexate induced intestinal toxicity via modulation of the gut microbiota. Toxicol Appl Pharmacol. 2020;391:114900. doi:10.1016/j.taap.2020.114900.
- Xu X, Zhang X. Effects of cyclophosphamide on immune system and gut microbiota in mice. Microbiol Res. 2015;171:97–106. doi:10.1016/j.micres.2014.11.002.
- Cui M, Xiao H, Li Y, Zhou L, Zhao S, Luo D, Zheng Q, Dong J, Zhao Y, Zhang X, et al. Faecal microbiota transplantation protects against radiation-induced toxicity. EMBO Mol Med. 2017;9(4):448–461. doi:10.15252/emmm.201606932.
- Wang W, Hu L, Chang S, Ma L, Li X, Yang Z, Du C, Qu X, Zhang C, Wang S, et al. Total body irradiation-induced colon damage is prevented by nitrate-mediated suppression of oxidative stress and homeostasis of the gut microbiome. Nitric Oxide. 2020;102:1–11. doi:10.1016/j.niox.2020.05.002.
- Li M, Gu MM, Lang Y, Shi J, Chen BPC, Guan H. The vanillin derivative VND3207 protects intestine against radiation injury by modulating p53/NOXA signaling pathway and restoring the balance of gut microbiota. Free Radic Biol Med. 2019;145:223–236. doi:10.1016/j.freeradbiomed.2019.09.035.
- Lu L, Jiang M, Zhu C, He J, Fan S. Amelioration of whole abdominal irradiation-induced intestinal injury in mice with 3,3’-Diindolylmethane (DIM). Free Radic Biol Med. 2019;130:244–255. doi:10.1016/j.freeradbiomed.2018.10.410.
- Ying M, Yu Q, Zheng B, Wang H, Wang J, Chen S. Cultured Cordyceps sinensis polysaccharides modulate intestinal mucosal immunity and gut microbiota in cyclophosphamide-treated mice. Carbohydr Polym. 2020;235:115957. doi:10.1016/j.carbpol.2020.115957.
- Hueso T, Ekpe K, Mayeur C, Gatse A, Joncquel-Chevallier Curt M, Gricourt G, Rodriguez C, Burdet C, Ulmann G, Neut C, et al. Impact and consequences of intensive chemotherapy on intestinal barrier and microbiota in acute myeloid leukemia: the role of mucosal strengthening. Gut Microbes. 2020;12(1):1800897. doi:10.1080/19490976.2020.1800897.
- Rajagopala SV, Yooseph S, Harkins DM, Moncera KJ, Zabokrtsky KB, Torralba MG, Tovchigrechko A, Highlander SK, Pieper R, Sender L, et al. Gastrointestinal microbial populations can distinguish pediatric and adolescent Acute Lymphoblastic Leukemia (ALL) at the time of disease diagnosis. Bmc Genomics. 2016;17(1):635. doi:10.1186/s12864-016-2965-y.
- Rajagopala SV, Singh H, Yu Y, Zabokrtsky KB, Torralba MG, Moncera KJ, Frank B, Pieper R, Sender L, Nelson KE, et al. Persistent gut microbial dysbiosis in children with Acute Lymphoblastic Leukemia (ALL) During Chemotherapy. Microb Ecol. 2020;79(4):1034–1043. doi:10.1007/s00248-019-01448-x.
- Chua LL, Rajasuriar R, Lim YAL, Woo YL, Loke P, Ariffin H. Temporal changes in gut microbiota profile in children with acute lymphoblastic leukemia prior to commencement-, during-, and post-cessation of chemotherapy. Bmc Cancer. 2020;20(1):151. doi:10.1186/s12885-020-6654-5.
- De Pietri S, Ingham AC, Frandsen TL, Rathe M, Krych L, Castro-Mejia JL, Nielsen DS, Nersting J, Wehner PS, Schmiegelow K, et al. Gastrointestinal toxicity during induction treatment for childhood acute lymphoblastic leukemia: the impact of the gut microbiota. Int J Cancer. 2020;147(7):1953–1962. doi:10.1002/ijc.32942.
- Hakim H, Dallas R, Wolf J, Tang L, Schultz-Cherry S, Darling V, Johnson C, Karlsson EA, Chang T-C, Jeha S, et al. Gut microbiome composition predicts infection risk during chemotherapy in children with Acute Lymphoblastic Leukemia. Clin Infect Dis. 2018;67(4):541–548. doi:10.1093/cid/ciy153.
- Zwielehner J, Lassl C, Hippe B, Pointner A, Switzeny OJ, Remely M, Kitzweger E, Ruckser R, Haslberger AG. Changes in human fecal microbiota due to chemotherapy analyzed by TaqMan-PCR, 454 sequencing and PCR-DGGE fingerprinting. PLos One. 2011;6(12):e28654. doi:10.1371/journal.pone.0028654.
- Montassier E, Batard E, Massart S, Gastinne T, Carton T, Caillon J, Le Fresne S, Caroff N, Hardouin JB, Moreau P, et al. 16S rRNA gene pyrosequencing reveals shift in patient faecal microbiota during high-dose chemotherapy as conditioning regimen for bone marrow transplantation. Microb Ecol. 2014;67(3):690–699. doi:10.1007/s00248-013-0355-4.
- Montassier E, Gastinne T, Vangay P, Al-Ghalith GA, Bruley des Varannes S, Massart S, Moreau P, Potel G, de La Cochetière MF, Batard E, et al. Chemotherapy-driven dysbiosis in the intestinal microbiome. Aliment Pharmacol Ther. 2015;42(5):515–528. doi:10.1111/apt.13302.
- van Vliet MJ, Tissing WJ, Dun CA, Meessen NE, Kamps WA, de Bont ES, van Vliet M, de Bont E, Harmsen H. Chemotherapy treatment in pediatric patients with acute myeloid leukemia receiving antimicrobial prophylaxis leads to a relative increase of colonization with potentially pathogenic bacteria in the gut. Clin Infect Dis. 2009;49(2):262–270. doi:10.1086/599346.
- Rashidi A, Kaiser T, Shields-Cutler R, Graiziger C, Holtan SG, Rehman TU, Wasko J, Weisdorf DJ, Dunny G, Khoruts A, et al. Dysbiosis patterns during re-induction/salvage versus induction chemotherapy for acute leukemia. Sci Rep. 2019;9(1):6083. doi:10.1038/s41598-019-42652-6.
- Guarner F, Malagelada JR. Gut flora in health and disease. Lancet. 2003;361(9356):512–519. doi:10.1016/S0140-6736(03)12489-0.
- Jenq RR, Ubeda C, Taur Y, Menezes CC, Khanin R, Dudakov JA, Liu C, West ML, Singer NV, Equinda MJ, et al. Regulation of intestinal inflammation by microbiota following allogeneic bone marrow transplantation. J Exp Med. 2012;209(5):903–911. doi:10.1084/jem.20112408.
- Shono Y, Docampo MD, Peled JU, Perobelli SM, Velardi E, Tsai JJ, Slingerland AE, Smith OM, Young LF, Gupta J, et al. Increased GVHD-related mortality with broad-spectrum antibiotic use after allogeneic hematopoietic stem cell transplantation in human patients and mice. Sci Transl Med. 2016;8(339). doi:10.1126/scitranslmed.aaf2311.
- Hayase E, Hayase T, Jamal MA, Miyama T, Chang CC, Ortega MR, Ahmed SS, Karmouch JL, Sanchez CA, Brown AN, et al. Mucus-degrading Bacteroides link carbapenems to aggravated graft-versus-host disease. Cell. 2022;185(20):3705–3719.e14. doi:10.1016/j.cell.2022.09.007.
- Taur Y, Xavier JB, Lipuma L, Ubeda C, Goldberg J, Gobourne A, Lee YJ, Dubin KA, Socci ND, Viale A, et al. Intestinal domination and the risk of bacteremia in patients undergoing allogeneic hematopoietic stem cell transplantation. Clin Infect Dis. 2012;55(7):905–914. doi:10.1093/cid/cis580.
- Jenq RR, Taur Y, Devlin SM, Ponce DM, Goldberg JD, Ahr KF, Littmann ER, Ling L, Gobourne AC, Miller LC, et al. Intestinal Blautia is associated with reduced death from graft-versus-host disease. Biol Blood Marrow Tr. 2015;21(8):1373–1383. doi:10.1016/j.bbmt.2015.04.016.
- Lee SE, Lim JY, Ryu DB, Kim TW, Park SS, Jeon YW, Yoon J-H, Cho B-S, Eom K-S, Kim Y-J, et al. Alteration of the intestinal microbiota by broad-spectrum antibiotic use correlates with the occurrence of intestinal graft-versus-host disease. Biol Blood Marrow Tr. 2019;25(10):1933–1943. doi:10.1016/j.bbmt.2019.06.001.
- Weber D, Jenq RR, Peled JU, Taur Y, Hiergeist A, Koestler J, Dettmer K, Weber M, Wolff D, Hahn J, et al. Microbiota disruption induced by early use of broad-spectrum antibiotics is an independent risk factor of outcome after allogeneic stem cell transplantation. Biol Blood Marrow Tr. 2017;23(5):845–852. doi:10.1016/j.bbmt.2017.02.006.
- Tanaka JS, Young RR, Heston SM, Jenkins K, Spees LP, Sung AD, Corbet K, Thompson JC, Bohannon L, Martin PL, et al. Anaerobic antibiotics and the risk of graft-versus-host disease after allogeneic hematopoietic stem cell transplantation. Biol Blood Marrow Tr. 2020;26(11):2053–2060. doi:10.1016/j.bbmt.2020.07.011.
- Weber D, Hiergeist A, Weber M, Dettmer K, Wolff D, Hahn J, Herr W, Gessner A, Holler E. Detrimental effect of broad-spectrum antibiotics on intestinal microbiome diversity in patients after allogeneic stem cell transplantation: lack of commensal sparing antibiotics. Clin Infect Dis. 2019;68(8):1303–1310. doi:10.1093/cid/ciy711.
- Holler E, Butzhammer P, Schmid K, Hundsrucker C, Koestler J, Peter K, Zhu W, Sporrer D, Hehlgans T, Kreutz M, et al. Metagenomic analysis of the stool microbiome in patients receiving allogeneic stem cell transplantation: loss of diversity is associated with use of systemic antibiotics and more pronounced in gastrointestinal graft-versus-host disease. Biol Blood Marrow Transplant. 2014;20(5):640–645. doi:10.1016/j.bbmt.2014.01.030.
- Golob JL, Pergam SA, Srinivasan S, Fiedler TL, Liu C, Garcia K, Mielcarek M, Ko D, Aker S, Marquis S, et al. Stool microbiota at neutrophil recovery is predictive for severe acute graft vs host disease after hematopoietic cell transplantation. Clin Infect Dis. 2017;65(12):1984–1991. doi:10.1093/cid/cix699.
- Routy B, Letendre C, Enot D, Chenard-Poirier M, Mehraj V, Seguin NC, Guenda K, Gagnon K, Woerther P-L, Ghez D, et al. The influence of gut-decontamination prophylactic antibiotics on acute graft-versus-host disease and survival following allogeneic hematopoietic stem cell transplantation. Oncoimmunology. 2017;6(1):6. doi:10.1080/2162402X.2016.1258506.
- Simms-Waldrip TR, Sunkersett G, Coughlin LA, Savani MR, Arana C, Kim J, Kim M, Zhan X, Greenberg DE, Xie Y, et al. Antibiotic-induced depletion of anti-inflammatory clostridia is associated with the development of graft-versus-host disease in pediatric stem cell transplantation patients. Biol Blood Marrow Tr. 2017;23(5):820–829. doi:10.1016/j.bbmt.2017.02.004.
- Hidaka D, Hayase E, Shiratori S, Hasegawa Y, Ishio T, Tateno T, Okada K, Goto H, Sugita J, Onozawa M, et al. The association between the incidence of intestinal graft-vs-host disease and antibiotic use after allogeneic hematopoietic stem cell transplantation. Clin Transplant. 2018;32(9):32. doi:10.1111/ctr.13361.
- Nishi K, Kanda J, Hishizawa M, Kitano T, Kondo T, Yamashita K, Takaori-Kondo A. Impact of the use and type of antibiotics on acute graft-versus-host disease. Biol Blood Marrow Tr. 2018;24(11):2178–2183. doi:10.1016/j.bbmt.2018.06.031.
- Elgarten CW, Li YM, Getz KD, Hemmer M, Huang YSV, Hall M, Wang T, Kitko CL, Jagasia MH, Nishihori T, et al. Broad-spectrum antibiotics and risk of graft-versus-host disease in pediatric patients undergoing transplantation for acute leukemia: association of carbapenem use with the risk of acute graft-versus-host disease. Transpl Cell Ther. 2021;27(2):.e177.1–.e177.8. doi:10.1016/j.jtct.2020.10.012.
- Meedt E, Hiergeist A, Gessner A, Dettmer K, Liebisch G, Ghimire S, Poeck H, Edinger M, Wolff D, Herr W, et al. Prolonged suppression of butyrate-producing bacteria is associated with acute gastrointestinal graft-vs-host disease and transplantation-related mortality after allogeneic stem cell transplantation. Clin Infect Dis. 2022;74(4):614–621. doi:10.1093/cid/ciab500.
- Kolodziejczyk AA, Zheng D, Elinav E. Diet–microbiota interactions and personalized nutrition. Nat Rev Microbiol. 2019;17(12):742–753. doi:10.1038/s41579-019-0256-8.
- Zeng X, Xing X, Gupta M, Keber FC, Lopez JG, Lee YJ, Roichman A, Wang L, Neinast MD, Donia MS, et al. Gut bacterial nutrient preferences quantified in vivo. Cell. 2022;185(18):3441–3456.e19. doi:10.1016/j.cell.2022.07.020.
- Seguy D, Berthon C, Micol JB, Darre S, Dalle JH, Neuville S, Bauters F, Jouet J-P, Yakoub-Agha I. Enteral feeding and early outcomes of patients undergoing allogeneic stem cell transplantation following myeloablative conditioning. Transplantation. 2006;82(6):835–839. doi:10.1097/01.tp.0000229419.73428.ff.
- Seguy D, Duhamel A, Rejeb MB, Gomez E, Buhl ND, Bruno B, Cortot A, Yakoub-Agha I. Better outcome of patients undergoing enteral tube feeding after myeloablative conditioning for allogeneic stem cell transplantation. Transplantation. 2012;94(3):287–294. doi:10.1097/TP.0b013e3182558f60.
- Gonzales F, Bruno B, Fuentes MA, De Berranger E, Guimber D, Behal H, Gandemer V, Spiegel A, Sirvent A, Yakoub-Agha I, et al. Better early outcome with enteral rather than parenteral nutrition in children undergoing MAC allo-SCT. Clin Nutr. 2018;37(6):2113–2121. doi:10.1016/j.clnu.2017.10.005.
- Beckerson J, Szydlo RM, Hickson M, Mactier CE, Innes AJ, Gabriel IH, Palanicawandar R, Kanfer EJ, Macdonald DH, Milojkovic D, et al. Impact of route and adequacy of nutritional intake on outcomes of allogeneic haematopoietic cell transplantation for haematologic malignancies. Clin Nutr. 2019;38(2):738–744. doi:10.1016/j.clnu.2018.03.008.
- Guieze R, Lemal R, Cabrespine A, Hermet E, Tournilhac O, Combal C, Bay J-O, Bouteloup C. Enteral versus parenteral nutritional support in allogeneic haematopoietic stem-cell transplantation. Clin Nutr. 2014;33(3):533–538. doi:10.1016/j.clnu.2013.07.012.
- D’Amico F, Biagi E, Rampelli S, Fiori J, Zama D, Soverini M, Barone M, Leardini D, Muratore E, Prete A, et al. Enteral nutrition in pediatric patients undergoing hematopoietic SCT promotes the recovery of gut microbiome homeostasis. Nutrients. 2019;11(12):11. doi:10.3390/nu11122958.
- Yang B, Zhang X, Gong H, Huang Y, Wang C, Liu H, Dong C, Ma S, Wu X, Wu D, et al. High stearic acid diet modulates gut microbiota and aggravates acute graft-versus-host disease. Signal Transduct Target Ther. 2021;6(1):277. doi:10.1038/s41392-021-00600-9.
- Stein-Thoeringer CK, Nichols KB, Lazrak A, Docampo MD, Slingerland AE, Slingerland JB, Clurman AG, Armijo G, Gomes ALC, Shono Y, et al. Lactose drives Enterococcus expansion to promote graft-versus-host disease. Science. 2019;366(6469):1143–1149. doi:10.1126/science.aax3760.
- Khuat LT, Le CT, Pai CS, Shields-Cutler RR, Holtan SG, Rashidi A, Parker SL, Knights D, Luna JI, Dunai C, et al. Obesity induces gut microbiota alterations and augments acute graft-versus-host disease after allogeneic stem cell transplantation. Sci Transl Med. 2020;12(571):12. doi:10.1126/scitranslmed.aay7713.
- Li X, Lin Y, Li X, Xu X, Zhao Y, Xu L, Gao Y, Li Y, Tan Y, Qian P, et al. Tyrosine supplement ameliorates murine aGVHD by modulation of gut microbiome and metabolome. EBioMedicine. 2020;61:103048. doi:10.1016/j.ebiom.2020.103048.
- Guo H, Chou WC, Lai Y, Liang K, Tam JW, Brickey WJ, Chen L, Montgomery ND, Li X, Bohannon LM, et al. Multi-omics analyses of radiation survivors identify radioprotective microbes and metabolites. Science. 2020;370(6516):370. doi:10.1126/science.aay9097.
- Ijiri K, Potten CS. Response of intestinal cells of differing topographical and hierarchical status to ten cytotoxic drugs and five sources of radiation. Br J Cancer. 1983;47(2):175–185. doi:10.1038/bjc.1983.25.
- Ijiri K, Potten CS. Further studies on the response of intestinal crypt cells of different hierarchical status to eighteen different cytotoxic agents. Br J Cancer. 1987;55(2):113–123. doi:10.1038/bjc.1987.25.
- Keefe DM, Brealey J, Goland GJ, Cummins AG. Chemotherapy for cancer causes apoptosis that precedes hypoplasia in crypts of the small intestine in humans. Gut. 2000;47(5):632–637. doi:10.1136/gut.47.5.632.
- Blijlevens NM, Donnelly JP, de Pauw BE. Prospective evaluation of gut mucosal barrier injury following various myeloablative regimens for haematopoietic stem cell transplant. Bone Marrow Transplant. 2005;35(7):707–711. doi:10.1038/sj.bmt.1704863.
- Hill GR, Crawford JM, Cooke KR, Brinson YS, Pan L, Ferrara JL. Total body irradiation and acute graft-versus-host disease: the role of gastrointestinal damage and inflammatory cytokines. Blood. 1997;90(8):3204–3213. doi:10.1182/blood.V90.8.3204.
- Johansson JE, Brune M, Ekman T. The gut mucosa barrier is preserved during allogeneic, haemopoietic stem cell transplantation with reduced intensity conditioning. Bone Marrow Transplant. 2001;28(8):737–742. doi:10.1038/sj.bmt.1703230.
- Johansson JE, Ekman T. Gut toxicity during hemopoietic stem cell transplantation may predict acute graft-versus-host disease severity in patients. Dig Dis Sci. 2007;52(9):2340–2345. doi:10.1007/s10620-006-9404-x.
- Nalle SC, Zuo L, Ong M, Singh G, Worthylake AM, Choi W, Manresa MC, Southworth AP, Edelblum KL, Baker GJ, et al. Graft-versus-host disease propagation depends on increased intestinal epithelial tight junction permeability. J Clin Invest. 2019;129(2):902–914. doi:10.1172/JCI98554.
- Martin-Gallausiaux C, Marinelli L, Blottiere HM, Larraufie P, Lapaque N. SCFA: mechanisms and functional importance in the gut. Proc Nutr Soc. 2021;80(1):37–49. doi:10.1017/S0029665120006916.
- Romick-Rosendale LE, Haslam DB, Lane A, Denson L, Lake K, Wilkey A, Watanabe M, Bauer S, Litts B, Luebbering N, et al. Antibiotic exposure and reduced short chain fatty acid production after hematopoietic stem cell transplant. Biol Blood Marrow Transplant. 2018;24(12):2418–2424. doi:10.1016/j.bbmt.2018.07.030.
- Payen M, Nicolis I, Robin M, Michonneau D, Delannoye J, Mayeur C, Kapel N, Berçot B, Butel M-J, Le Goff J, et al. Functional and phylogenetic alterations in gut microbiome are linked to graft-versus-host disease severity. Blood Adv. 2020;4(9):1824–1832. doi:10.1182/bloodadvances.2020001531.
- Mathewson ND, Jenq R, Mathew AV, Koenigsknecht M, Hanash A, Toubai T, Oravecz-Wilson K, Wu S-R, Sun Y, Rossi C, et al. Gut microbiome–derived metabolites modulate intestinal epithelial cell damage and mitigate graft-versus-host disease. Nat Immunol. 2016;17(5):505–513. doi:10.1038/ni.3400.
- Jia W, Xie G, Jia W. Bile acid–microbiota crosstalk in gastrointestinal inflammation and carcinogenesis. Nat Rev Gastroenterol Hepatol. 2018;15(2):111–128. doi:10.1038/nrgastro.2017.119.
- Fu T, Coulter S, Yoshihara E, Oh TG, Fang S, Cayabyab F, Zhu Q, Zhang T, Leblanc M, Liu S, et al. FXR regulates intestinal cancer stem cell proliferation. Cell. 2019;176(5):1098–1112.e18. doi:10.1016/j.cell.2019.01.036.
- Xu M, Shen Y, Cen M, Zhu Y, Cheng F, Tang L, Zheng X, Kim JJ, Dai N, Hu W, et al. Modulation of the gut microbiota-farnesoid X receptor axis improves deoxycholic acid-induced intestinal inflammation in mice. J Crohns Colitis. 2021;15(7):1197–1210. doi:10.1093/ecco-jcc/jjab003.
- Sorrentino G, Perino A, Yildiz E, El Alam G, Bou Sleiman M, Gioiello A, Pellicciari R, Schoonjans K. Bile acids signal via TGR5 to activate intestinal stem cells and epithelial regeneration. Gastroenterology. 2020;159(3):956–968.e8. doi:10.1053/j.gastro.2020.05.067.
- Duboc H, Rajca S, Rainteau D, Benarous D, Maubert MA, Quervain E, Thomas G, Barbu V, Humbert L, Despras G, et al. Connecting dysbiosis, bile-acid dysmetabolism and gut inflammation in inflammatory bowel diseases. Gut. 2013;62(4):531–539. doi:10.1136/gutjnl-2012-302578.
- Sinha SR, Haileselassie Y, Nguyen LP, Tropini C, Wang M, Becker LS, Sim D, Jarr K, Spear ET, Singh G, et al. Dysbiosis-induced secondary bile acid deficiency promotes intestinal inflammation. Cell Host & Microbe. 2020;27(4):659–670.e5. doi:10.1016/j.chom.2020.01.021.
- Haring E, FM U, Andrieux G, Proietti M, Bulashevska A, Sauer B, Braun M, de Vega Gomez L, Esser E,R, Martin P,F, et al. Bile acids regulate intestinal antigen presentation and reduce graft-versus-host disease without impairing the graft-versus-leukemia effect. Haematologica. 2021;106(8):2131–2146. doi:10.3324/haematol.2019.242990.
- Ruutu T, Eriksson B, Remes K, Juvonen E, Volin L, Remberger M, Parkkali T, Hägglund H, Ringdén O. Ursodeoxycholic acid for the prevention of hepatic complications in allogeneic stem cell transplantation. Blood. 2002;100(6):1977–1983. doi:10.1182/blood-2001-12-0159.
- Ruutu T, Juvonen E, Remberger M, Remes K, Volin L, Mattsson J, Nihtinen A, Hägglund H, Ringdén O. Improved survival with ursodeoxycholic acid prophylaxis in allogeneic stem cell transplantation: long-term follow-up of a randomized study. Biol Blood Marrow Transplant. 2014;20(1):135–138. doi:10.1016/j.bbmt.2013.10.014.
- Agus A, Planchais J, Sokol H. Gut microbiota regulation of tryptophan metabolism in health and disease. Cell Host & Microbe. 2018;23(6):716–724. doi:10.1016/j.chom.2018.05.003.
- Wlodarska M, Luo C, Kolde R, d’Hennezel E, Annand JW, Heim CE, Krastel P, Schmitt EK, Omar AS, Creasey EA, et al. Indoleacrylic acid produced by commensal peptostreptococcus species suppresses inflammation. Cell Host & Microbe. 2017;22(1):25–37.e6. doi:10.1016/j.chom.2017.06.007.
- Scott SA, Fu J, Chang PV. Microbial tryptophan metabolites regulate gut barrier function via the aryl hydrocarbon receptor. Proc Natl Acad Sci USA. 2020;117(32):19376–19387. doi:10.1073/pnas.2000047117.
- Venkatesh M, Mukherjee S, Wang H, Li H, Sun K, Benechet AP, Qiu Z, Maher L, Redinbo M, Phillips R, et al. Symbiotic bacterial metabolites regulate gastrointestinal barrier function via the xenobiotic sensor PXR and Toll-like receptor 4. Immunity. 2014;41(2):296–310. doi:10.1016/j.immuni.2014.06.014.
- Swimm A, Giver CR, DeFilipp Z, Rangaraju S, Sharma A, Ulezko Antonova A, Sonowal R, Capaldo C, Powell D, Qayed M, et al. Indoles derived from intestinal microbiota act via type I interferon signaling to limit graft-versus-host disease. Blood. 2018;132(23):2506–2519. doi:10.1182/blood-2018-03-838193.
- Rooks MG, Garrett WS. Gut microbiota, metabolites and host immunity. Nat Rev Immunol. 2016;16(6):341–352. doi:10.1038/nri.2016.42.
- Arpaia N, Campbell C, Fan X, Dikiy S, van der Veeken J, deRoos P, deRoos P, Liu H, Cross JR, Pfeffer K, et al. Metabolites produced by commensal bacteria promote peripheral regulatory T-cell generation. Nature. 2013;504(7480):451–455. doi:10.1038/nature12726.
- Hang S, Paik D, Yao L, Kim E, Trinath J, Lu J, Ha S, Nelson BN, Kelly SP, Wu L, et al. Bile acid metabolites control TH17 and Treg cell differentiation. Nature. 2019;576(7785):143–148. doi:10.1038/s41586-019-1785-z.
- Hou Q, Ye L, Liu H, Huang L, Yang Q, Turner JR, Yu Q. Lactobacillus accelerates ISCs regeneration to protect the integrity of intestinal mucosa through activation of STAT3 signaling pathway induced by LPLs secretion of IL-22. Cell Death Differ. 2018;25(9):1657–1670. doi:10.1038/s41418-018-0070-2.
- Wu K, Yuan Y, Yu H, Dai X, Wang S, Sun Z, Wang F, Fei H, Lin Q, Jiang H, et al. The gut microbial metabolite trimethylamine N-oxide aggravates GVHD by inducing M1 macrophage polarization in mice. Blood. 2020;136(4):501–515. doi:10.1182/blood.2019003990.
- Aoyama K, Saha A, Tolar J, Riddle MJ, Veenstra RG, Taylor PA, Blomhoff R, Panoskaltsis-Mortari A, Klebanoff CA, Socié G, et al. Inhibiting retinoic acid signaling ameliorates graft-versus-host disease by modifying T-cell differentiation and intestinal migration. Blood. 2013;122(12):2125–2134. doi:10.1182/blood-2012-11-470252.
- Schwab L, Goroncy L, Palaniyandi S, Gautam S, Triantafyllopoulou A, Mocsai A, Reichardt W, Karlsson FJ, Radhakrishnan SV, Hanke K, et al. Neutrophil granulocytes recruited upon translocation of intestinal bacteria enhance graft-versus-host disease via tissue damage. Nat Med. 2014;20(6):648–654. doi:10.1038/nm.3517.
- Hulsdunker J, Ottmuller KJ, Neeff HP, Koyama M, Gao Z, Thomas OS, Follo M, Al-Ahmad A, Prinz G, Duquesne S, et al. Neutrophils provide cellular communication between ileum and mesenteric lymph nodes at graft-versus-host disease onset. Blood. 2018;131(16):1858–1869. doi:10.1182/blood-2017-10-812891.
- Koyama M, Mukhopadhyay P, Schuster IS, Henden AS, Hulsdunker J, Varelias A, Vetizou M, Kuns RD, Robb RJ, Zhang P, et al. MHC class II antigen presentation by the intestinal epithelium initiates graft-versus-host disease and is influenced by the microbiota. Immunity. 2019;51(5):885–898.e7. doi:10.1016/j.immuni.2019.08.011.
- Fu YY, Egorova A, Sobieski C, Kuttiyara J, Calafiore M, Takashima S, Clevers H, Hanash AM. T cell recruitment to the intestinal stem cell compartment drives immune-mediated intestinal damage after allogeneic transplantation. Immunity. 2019;51(1):90–103.e3. doi:10.1016/j.immuni.2019.06.003.
- Eriguchi Y, Takashima S, Oka H, Shimoji S, Nakamura K, Uryu H, Shimoda S, Iwasaki H, Shimono N, Ayabe T, et al. Graft-versus-host disease disrupts intestinal microbial ecology by inhibiting Paneth cell production of α-defensins. Blood. 2012;120(1):223–231. doi:10.1182/blood-2011-12-401166.
- Levine JE, Huber E, Hammer ST, Harris AC, Greenson JK, Braun TM, Ferrara JLM, Holler E. Low Paneth cell numbers at onset of gastrointestinal graft-versus-host disease identify patients at high risk for nonrelapse mortality. Blood. 2013;122(8):1505–1509. doi:10.1182/blood-2013-02-485813.
- Ara T, Hashimoto D, Hayase E, Noizat C, Kikuchi R, Hasegawa Y, Matsuda K, Ono S, Matsuno Y, Ebata K, et al. Intestinal goblet cells protect against GVHD after allogeneic stem cell transplantation via Lypd8. Sci Transl Med. 2020;12(550):12. doi:10.1126/scitranslmed.aaw0720.
- Hanash AM, Dudakov JA, Hua G, O’Connor MH, Young LF, Singer NV, West M, Jenq R, Holland A, Kappel L, et al. Interleukin-22 protects intestinal stem cells from immune-mediated tissue damage and regulates sensitivity to graft versus host disease. Immunity. 2012;37(2):339–350. doi:10.1016/j.immuni.2012.05.028.
- Munneke JM, Bjorklund AT, Mjosberg JM, Garming-Legert K, Bernink JH, Blom B, Huisman C, van Oers MHJ, Spits H, Malmberg K-J, et al. Activated innate lymphoid cells are associated with a reduced susceptibility to graft-versus-host disease. Blood. 2014;124(5):812–821. doi:10.1182/blood-2013-11-536888.
- Hazenberg MD, Haverkate NJE, van Lier YF, Spits H, Krabbendam L, Bemelman WA, Buskens CJ, Blom B, Shikhagaie MM. Human ectoenzyme-expressing ILC3: immunosuppressive innate cells that are depleted in graft-versus-host disease. Blood Adv. 2019;3(22):3650–3660. doi:10.1182/bloodadvances.2019000176.
- Biton M, Haber AL, Rogel N, Burgin G, Beyaz S, Schnell A, Ashenberg O, Su C-W, Smillie C, Shekhar K, et al. T helper cell cytokines modulate intestinal stem cell renewal and differentiation. Cell. 2018;175(5):1307–1320.e22. doi:10.1016/j.cell.2018.10.008.
- Sato T, Ishikawa S, Asano J, Yamamoto H, Fujii M, Sato T, Yamamoto K, Kitagaki K, Akashi T, Okamoto R, et al. Regulated IFN signalling preserves the stemness of intestinal stem cells by restricting differentiation into secretory-cell lineages. Nat Cell Biol. 2020;22(8):919–926. doi:10.1038/s41556-020-0545-5.
- Takashima S, Martin ML, Jansen SA, Fu Y, Bos J, Chandra D, O’Connor MH, Mertelsmann AM, Vinci P, Kuttiyara J, et al. T cell–derived interferon-γ programs stem cell death in immune-mediated intestinal damage. Sci Immunol. 2019;4(42):4. doi:10.1126/sciimmunol.aay8556.
- Eriguchi Y, Nakamura K, Yokoi Y, Sugimoto R, Takahashi S, Hashimoto D, Teshima T, Ayabe T, Selsted ME, Ouellette AJ, et al. Essential role of IFN-γ in T cell–associated intestinal inflammation. JCI Insight. 2018;3(18):3. doi:10.1172/jci.insight.121886.
- Lee C, An M, Joung JG, Park WY, Chang DK, Kim YH, Hong SN. TNFα Induces LGR5+ Stem Cell Dysfunction in Patients with Crohn’s Disease. Cell Mol Gastroenterol Hepatol. 2022;13(3):789–808. doi:10.1016/j.jcmgh.2021.10.010.
- Santos AJM, Lo YH, Mah AT, Kuo CJ. The intestinal stem cell niche: homeostasis and adaptations. Trends Cell Biol. 2018;28(12):1062–1078. doi:10.1016/j.tcb.2018.08.001.
- Funk MC, Zhou J, Boutros M. Ageing, metabolism and the intestine. EMBO Rep. 2020;21(7):e50047. doi:10.15252/embr.202050047.
- Socie G, Kean LS, Zeiser R, Blazar BR. Insights from integrating clinical and preclinical studies advance understanding of graft-versus-host disease. J Clin Invest. 2021;131(12):131. doi:10.1172/JCI149296.
- Kim KA, Kakitani M, Zhao J, Oshima T, Tang T, Binnerts M, Liu Y, Boyle B, Park E, Emtage P, et al. Mitogenic influence of human R-spondin1 on the intestinal epithelium. Science. 2005;309(5738):1256–1259. doi:10.1126/science.1112521.
- Takashima S, Kadowaki M, Aoyama K, Koyama M, Oshima T, Tomizuka K, Akashi K, Teshima T. The Wnt agonist R-spondin1 regulates systemic graft-versus-host disease by protecting intestinal stem cells. J Exp Med. 2011;208(2):285–294. doi:10.1084/jem.20101559.
- Hayase E, Hashimoto D, Nakamura K, Noizat C, Ogasawara R, Takahashi S, Ohigashi H, Yokoi Y, Sugimoto R, Matsuoka S, et al. R-Spondin1 expands Paneth cells and prevents dysbiosis induced by graft-versus-host disease. J Exp Med. 2017;214(12):3507–3518. doi:10.1084/jem.20170418.
- Zhao D, Kim YH, Jeong S, Greenson JK, Chaudhry MS, Hoepting M, Anderson ER, van den Brink MRM, Peled JU, Gomes ALC, et al. Survival signal REG3α prevents crypt apoptosis to control acute gastrointestinal graft-versus-host disease. J Clin Invest. 2018;128(11):4970–4979. doi:10.1172/JCI99261.
- Lindemans CA, Calafiore M, Mertelsmann AM, O’Connor MH, Dudakov JA, Jenq RR, Velardi E, Young LF, Smith OM, Lawrence G, et al. Interleukin-22 promotes intestinal-stem-cell-mediated epithelial regeneration. Nature. 2015;528(7583):560–564. doi:10.1038/nature16460.
- Ponce DM, Alousi AM, Nakamura R, Slingerland J, Calafiore M, Sandhu KS, Barker JN, Devlin S, Shia J, Giralt S, et al. A phase 2 study of Interleukin-22 and systemic corticosteroids as initial treatment for acute GVHD of the lower GI tract. Blood. 2022;141(12):1389–1401. doi:10.1182/blood.2021015111.
- Koyama M, Samson L, Ensbey KS, Takahashi S, Clouston AD, Martin PJ, Hill GR. Lithium attenuates graft-versus-host disease via effects on the intestinal stem cell niche. Blood. 2023;141(3):315–319. doi:10.1182/blood.2022015808.
- Norona J, Apostolova P, Schmidt D, Ihlemann R, Reischmann N, Taylor G, Köhler N, de Heer J, Heeg S, Andrieux G, et al. Glucagon-like peptide 2 for intestinal stem cell and Paneth cell repair during graft-versus-host disease in mice and humans. Blood. 2020;136(12):1442–1455. doi:10.1182/blood.2020005957.
- Fischer JC, Bscheider M, Eisenkolb G, Lin CC, Wintges A, Otten V, Lindemans CA, Heidegger S, Rudelius M, Monette S, et al. RIG-I/MAVS and STING signaling promote gut integrity during irradiation- and immune-mediated tissue injury. Sci Transl Med. 2017;9(386). doi:10.1126/scitranslmed.aag2513.
- Henden AS, Koyama M, Robb RJ, Forero A, Kuns RD, Chang K, Ensbey KS, Varelias A, Kazakoff SH, Waddell N, et al. IFN-λ therapy prevents severe gastrointestinal graft-versus-host disease. Blood. 2021;138(8):722–737. doi:10.1182/blood.2020006375.
- Yoshifuji K, Inamoto K, Kiridoshi Y, Takeshita K, Sasajima S, Shiraishi Y, Yamashita Y, Nisaka Y, Ogura Y, Takeuchi R, et al. Prebiotics protect against acute graft-versus-host disease and preserve the gut microbiota in stem cell transplantation. Blood Adv. 2020;4(19):4607–4617. doi:10.1182/bloodadvances.2020002604.
- Andermann TM, Fouladi F, Tamburini FB, Sahaf B, Tkachenko E, Greene C, Buckley MT, Brooks EF, Hedlin H, Arai S, et al. A fructo-oligosaccharide prebiotic is well tolerated in adults undergoing allogeneic hematopoietic stem cell transplantation: a phase i dose-escalation trial. Transplant Cell Ther. 2021;27(11):.e932.1–.e932.11. doi:10.1016/j.jtct.2021.07.009.
- Gerbitz A, Schultz M, Wilke A, Linde HJ, Scholmerich J, Andreesen R, Holler E. Probiotic effects on experimental graft-versus-host disease: let them eat yogurt. Blood. 2004;103(11):4365–4367. doi:10.1182/blood-2003-11-3769.
- Sofi MH, Wu Y, Ticer T, Schutt S, Bastian D, Choi HJ, Tian L, Mealer C, Liu C, Westwater C, et al. A single strain of Bacteroides fragilis protects gut integrity and reduces GVHD. JCI Insight. 2021;6(3):6. doi:10.1172/jci.insight.136841.
- Gorshein E, Wei C, Ambrosy S, Budney S, Vivas J, Shenkerman A, Manago J, McGrath MK, Tyno A, Lin Y, et al. Lactobacillus rhamnosus GG probiotic enteric regimen does not appreciably alter the gut microbiome or provide protection against GVHD after allogeneic hematopoietic stem cell transplantation. Clin Transplant. 2017;31(5):31. doi:10.1111/ctr.12947.
- Ladas EJ, Bhatia M, Chen L, Sandler E, Petrovic A, Berman DM, Hamblin F, Gates M, Hawks R, Sung L, et al. The safety and feasibility of probiotics in children and adolescents undergoing hematopoietic cell transplantation. Bone Marrow Transplant. 2016;51(2):262–266. doi:10.1038/bmt.2015.275.
- Qi X, Li X, Zhao Y, Wu X, Chen F, Ma X. Treating Steroid Refractory Intestinal Acute Graft-vs.-Host Disease with Fecal Microbiota Transplantation: a Pilot Study. Front Immunol. 2018;9:2195. doi:10.3389/fimmu.2018.02195.
- DeFilipp Z, Peled JU, Li S, Mahabamunuge J, Dagher Z, Slingerland AE, Del Rio C, Valles B, Kempner ME, Smith M, et al. Third-party fecal microbiota transplantation following allo-HCT reconstitutes microbiome diversity. Blood Adv. 2018;2(7):745–753. doi:10.1182/bloodadvances.2018017731.
- van Lier YF, Davids M, Haverkate NJE, de Groot PF, Donker ML, Meijer E, van Lier YF, de Groot PF, Heubel-Moenen FCJI, Nur E, et al. Donor fecal microbiota transplantation ameliorates intestinal graft-versus-host disease in allogeneic hematopoietic cell transplant recipients. Sci Transl Med. 2020;12(556):12. doi:10.1126/scitranslmed.aaz8926.
- Zhao Y, Li X, Zhou Y, Gao J, Jiao Y, Zhu B. Safety and Efficacy of Fecal Microbiota Transplantation for Grade IV Steroid Refractory GI-GvHD Patients: interim Results from FMT2017002 Trial. Front Immunol. 2021;12:678476. doi:10.3389/fimmu.2021.678476.
- Spindelboeck W, Halwachs B, Bayer N, Huber-Krassnitzer B, Schulz E, Uhl B, Gaksch L, Hatzl S, Bachmayr V, Kleissl L, et al. Antibiotic use and ileocolonic immune cells in patients receiving fecal microbiota transplantation for refractory intestinal GvHD: a prospective cohort study. Ther Adv Hematol. 2021;12:20406207211058333. doi:10.1177/20406207211058333.
- Bilinski J, Lis K, Tomaszewska A, Grzesiowski P, Dzieciatkowski T, Tyszka M, Karakulska‐Prystupiuk E, Boguradzki P, Tormanowska M, Halaburda K, et al. Fecal microbiota transplantation in patients with acute and chronic graft-versus-host disease—spectrum of responses and safety profile. Results from a prospective, multicenter study. Am J Hematol. 2021;96(3):E88–E91. doi:10.1002/ajh.26077.
- Goeser F, Sifft B, Stein-Thoeringer C, Farowski F, Strassburg CP, Brossart P, Higgins PG, Scheid C, Wolf D, Holderried TAW, et al. Fecal microbiota transfer for refractory intestinal graft-versus-host disease — Experience from two German tertiary centers. Eur J Haematol. 2021;107(2):229–245. doi:10.1111/ejh.13642.
- Zhang F, Zuo T, Yeoh YK, Cheng FWT, Liu Q, Tang W, Cheung KCY, Yang K, Cheung CP, Mo CC, et al. Longitudinal dynamics of gut bacteriome, mycobiome and virome after fecal microbiota transplantation in graft-versus-host disease. Nat Commun. 2021;12(1):65. doi:10.1038/s41467-020-20240-x.
- Vetizou M, Pitt JM, Daillere R, Lepage P, Waldschmitt N, Flament C, Rusakiewicz S, Routy B, Roberti MP, Duong CPM, et al. Anticancer immunotherapy by CTLA-4 blockade relies on the gut microbiota. Science. 2015;350(6264):1079–1084. doi:10.1126/science.aad1329.
- Sivan A, Corrales L, Hubert N, Williams JB, Aquino-Michaels K, Earley ZM, Benyamin FW, Man Lei Y, Jabri B, Alegre M-L, et al. Commensal Bifidobacterium promotes antitumor immunity and facilitates anti–PD-L1 efficacy. Science. 2015;350(6264):1084–1089. doi:10.1126/science.aac4255.
- Spencer CN, McQuade JL, Gopalakrishnan V, McCulloch JA, Vetizou M, Cogdill AP, Khan MAW, Zhang X, White MG, Peterson CB, et al. Dietary fiber and probiotics influence the gut microbiome and melanoma immunotherapy response. Science. 2021;374(6575):1632–1640. doi:10.1126/science.aaz7015.
- Hu Y, Li J, Ni F, Yang Z, Gui X, Bao Z, Zhao H, Wei G, Wang Y, Zhang M, et al. CAR-T cell therapy-related cytokine release syndrome and therapeutic response is modulated by the gut microbiome in hematologic malignancies. Nat Commun. 2022;13(1):5313. doi:10.1038/s41467-022-32960-3.
- Stein-Thoeringer CK, Saini NY, Zamir E, Blumenberg V, Schubert ML, Mor U, Fante MA, Schmidt S, Hayase E, Hayase T, et al. A non-antibiotic-disrupted gut microbiome is associated with clinical responses to CD19-CAR-T cell cancer immunotherapy. Nat Med. 2023;29(4):906–916. doi:10.1038/s41591-023-02234-6.
- Peled JU, Devlin SM, Staffas A, Lumish M, Khanin R, Littmann ER, Ling L, Kosuri S, Maloy M, Slingerland JB, et al. Intestinal microbiota and relapse after hematopoietic-cell transplantation. J Clin Oncol. 2017;35(15):1650–1659. doi:10.1200/JCO.2016.70.3348.