ABSTRACT
Synbiotics are increasingly used by the general population to boost immunity. However, there is limited evidence concerning the immunomodulatory effects of synbiotics in healthy individuals. Therefore, we conducted a double-blind, randomized, placebo-controlled study in 106 healthy adults. Participants were randomly assigned to receive either synbiotics (containing Bifidobacterium lactis HN019 1.5 × 108 CFU/d, Lactobacillus rhamnosus HN001 7.5 × 107 CFU/d, and fructooligosaccharide 500 mg/d) or placebo for 8 weeks. Immune parameters and gut microbiota composition were measured at baseline, mid, and end of the study. Compared to the placebo group, participants receiving synbiotic supplementation exhibited greater reductions in plasma C-reactive protein (P = 0.088) and interferon-gamma (P = 0.008), along with larger increases in plasma interleukin (IL)-10 (P = 0.008) and stool secretory IgA (sIgA) (P = 0.014). Additionally, synbiotic supplementation led to an enrichment of beneficial bacteria (Clostridium_sensu_stricto_1, Lactobacillus, Bifidobacterium, and Collinsella) and several functional pathways related to amino acids and short-chain fatty acids biosynthesis, whereas reduced potential pro-inflammatory Parabacteroides compared to baseline. Importantly, alternations in anti-inflammatory markers (IL-10 and sIgA) were significantly correlated with microbial variations triggered by synbiotic supplementation. Stratification of participants into two enterotypes based on pre-treatment Prevotella-to-Bacteroides (P/B) ratio revealed a more favorable effect of synbiotic supplements in individuals with a higher P/B ratio. In conclusion, this study suggested the beneficial effects of synbiotic supplementation on immune parameters, which were correlated with synbiotics-induced microbial changes and modified by microbial enterotypes. These findings provided direct evidence supporting the personalized supplementation of synbiotics for immunomodulation.
Introduction
Gut microbiota constantly interacts with the host immune system, maintaining the homeostasis of normal physiology and function.Citation1 Due to this dynamic bidirectional relationship, modifying the gut microbiota may regulate immune function and host susceptibility to infection.Citation2 Therefore, gut microbiota-targeted measures for enhancing human immunity capacity gain growing attention.
Synbiotics, as a mixture of live microorganisms (e.g., probiotics) and substrates (e.g., prebiotics) that are selectively utilized by host microorganisms, have emerged as potential modulators of gut microbiota and immunity.Citation3 The synbiotic and its main ingredients, probiotics as well as prebiotics, have been demonstrated to improve inflammation biomarkers in individuals under pathological conditions.Citation4–7 In contrast, evidence regarding the immunity-regulating effects of synbiotic products in healthy individuals is limited and yields inconsistent results.Citation5,Citation8 However, in recent years, the use of synbiotic products has increased in the general population, particularly among individuals with self-rereported favorable health quality, as a means to boost their immunity.Citation9 Therefore, investigating the effects of synbiotics on immune parameters in healthy individuals has significant implications. Moreover, whether microbial alternation induced by synbiotic supplementation contributes to its immunomodulation properties is poorly understood. Recent studies have revealed that individuals with different enterotypes, identified based on the ratio of Prevotella-to-Bacteroides (P/B), respond differently to a specific diet for weight control.Citation10–12 Nevertheless, the potential influence of microbial enterotypes on the immunomodulatory effects of synbiotics remains unclear.
Species or strains from Lactobacillus and Bifidobacterium are prominent probiotics possessing immunomodulation properties.Citation13 Notably, Bifidobacterium lactis (B. lactis HN019) and Lactobacillus rhamnosus HN001 (L. rhamnosus HN001) have demonstrated immunity-enhancing properties, particularly when combined with oligosaccharide-rich substrates, in both animal and human models.Citation14–16 Based on this knowledge, our study aimed to conduct a double-blind, randomized, placebo-controlled trial to investigate the immunomodulatory and microbial effects of a synbiotic supplement (comprising B. lactis HN019, L. rhamnosus HN001, and fructooligosaccharide). Specifically, we intended to address the following three questions: (1) Could synbiotic supplementation induce alternations in immune parameters among healthy adults? (2) Were these alternations associated with gut microbiota variations triggered by synbiotic supplementation? (3) Were synbiotic effects affected by microbial enterotypes, as inferred from the pre-treatment P/B ratio?
Results
A total of 170 individuals were initially screened for eligibility, of whom 106 subjects were eventually enrolled. No one dropped out or was excluded for noncompliance throughout the study (Figure S1 in Supplementary material). There was no adverse event reported during the intervention. Baseline characteristics were well-balanced between the two groups (). Physical activity, dietary intake, and healthy food diversity indexCitation17 remained unchanged in both treatment groups (Table S1 in Supplementary material).
Table 1. Baseline Characteristics1..
Effects on immune parameters
As shown in , an 8-week supplementation with synbiotic significantly reduced plasma C-reactive protein (CRP) by 21% (P = .005) and interferon-gamma (IFN-γ) by 12% (P = .008) compared to baseline. These reductions were greater than those observed in the placebo group (P = .088 for CRP, P = .008 for IFN-γ). Plasma interleukin (IL)-10 remained unchanged in the synbiotic group but decreased by 16% at week 8 in the placebo group (P < .001). Consequently, there was a statistically significant increase in IL-10 levels in the synbiotic group compared to the placebo (P = .008). Furthermore, the level of fecal secretory IgA (sIgA) increased by 24% following the 8-week synbiotic intervention (P = .043), resulting in higher growth in the synbiotic group relative to placebo (P = .014). The plasma concentrations of IL-6, IL-8, and tumor necrosis factor-alpha (TNF-α) as well as the saliva level of sIgA varied similarly in both treatment groups. Moreover, we observed that synbiotic supplementation consistently led to greater reductions in plasma CRP in men compared to women (week 4: P = .040; week 8: P = .016). Similarly, only male individuals exhibited a significant down-regulation trend in plasma IFN-γ and an up-regulation trend in IL-10 and fecal sIgA compared to placebo (Figure S2 in Supplement material).
Figure 1. Alternations in systemic inflammation and mucosal immunity markers after synbiotic supplementation. The point indicated adjusted mean fold changes of CRP (a), IFN-gamma (b), IL-6 (c), IL-8 (d), IL-10 (e), TNF-alpha (f), saliva sIga (g) and stool sIga (h) at week 4 or week 8 from baseline, which were the least square means estimated using generalized linear mixed models, with time (week 0, 4, 8), group (synbiotic, placebo), and group-by-time as fixed effects, subjects as a random effect, and baseline value as a covariate. Error bars indicated 95% confidence intervals. Abbreviations: CRP, C-reactive protein; IFN, interferon; IL, interleukin; TNF, tumor necrosis factor; sIga, secretory IgA.
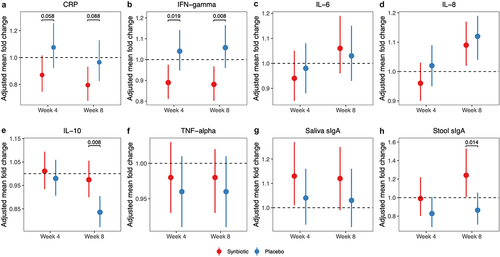
Minor within-group changes were observed in the absolute counts of T lymphocytes, B lymphocytes, and natural killer cells, but no significant between-group difference was found (). Physiological and biochemical markers, including body weight, body mass index (BMI), blood pressure, body fat percentage, and indicators regarding glucose and lipid metabolism, did not differ between the synbiotic group and placebo group (Table S2 in Supplementary material). Additionally, synbiotic supplementation did not reduce the occurrence of upper respiratory tract infection (URTI) episodes or affect the average duration and severity of each URTI episode (Table S3 in Supplementary material).
Table 2. Alternations in the absolute count of immune population after synbiotic supplementation.
Effects on gut microbiota and their association with immunological improvements
Amplicon sequencing was performed on 318 stool samples, resulting in a total of 21,879,501 sequences. The most abundant phylum was Firmicutes (72.2%), followed by Bacteroidota (14.4%), Actinobacteriota (9.2%), and Proteobacteria (3.8%). These four phyla comprised approximately 99.6% of the gut microbiota.
During the intervention, alpha indices and beta diversity based on Euclidean and weighted UniFrac distances did not differ between the synbiotic and placebo group (Figure S3 in Supplementary material). The composition characteristics of gut microbiota in participants before and after the synbiotic supplementation were described in . At the genus level, synbiotic supplementation increased the relative abundance of Collinsella (median fold change (FC) = 1.76, P = .004, false discovery rate (FDR)-P = .034), Bifidobacterium (median FC = 1.80, P < .001, FDR-P = .004), Lactobacillus (median FC = 2.67, P < .001, FDR-P = .007), and Clostridium_sensu_stricto_1 (median FC = 3.08, P = .019, FDR-P = .095), whereas decreased the relative abundance of Parabacteroides by 49% (P = .009, FDR-P = .063) compared to baseline (). None of these microbial alternations were found in the placebo group (Figure S4 in Supplementary material). Compared to placebo, synbiotic supplementation remarkably enriched Lactobacillus (P for interaction = .001, ). Notably, our subgroup analysis demonstrated that the administration of synbiotic supplements induced more variations (including Collinsella, Bifidobacterium, Lactobacillus, and so on) in female individuals, particularly at week 4 of the intervention (Figure S5 in Supplementary material). At the zero-radius operational taxonomy unit (zOTU) level, zOUT103 within L. rhamnosus, zOTU1158 as well as zOTU2973 within B. lactis substantially increased in the synbiotic group relative to the placebo (all P for interaction < 0.001, ). Moreover, our analysis of predicted functional pathways identified eight pathways related to the biosynthesis of several amino acids and short-chain fatty acids (SCFAs) enriched in the synbiotic group compared to the baseline ().
Figure 2. Composition characteristics of gut microbiota in participants before and after the synbiotic supplementation. (a) Phylum-level and (b) genus-level gut microbiota composition in participants at baseline, week 4, and week 8.
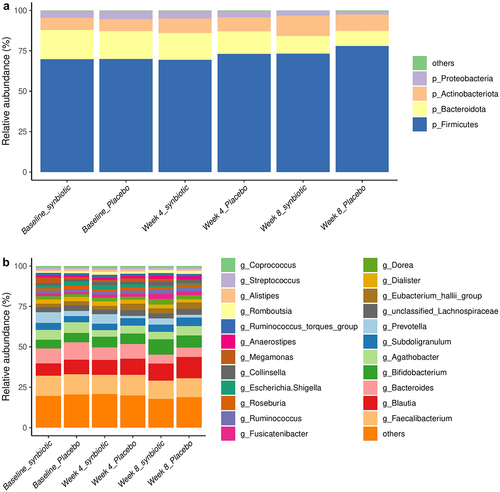
Figure 3. Alternations in gut microbiota composition and function profile during the synbiotic intervention. (a) Heatmap of log2 relative abundance of individual genera in the synbiotic group before and after the intervention. (b-e) Boxplot of Lactobacillus (b), zOTU103 within Lactobacillus rhamnosus (c), and zOTU1158 (d) as well as zOTU2973 (e) within Bifidobacterium lactis. dark lines in the boxes indicated medians, the width of the notches was the IQR, and error bars extended to most of the extreme values within 1.5 IQR. (f) significantly altered MetaCyc pathways at week 8 in the synbiotic group compared to baseline. FC were defined as the ratio of the abundance of pathways at week 8 and that at baseline. Significances of within- or between-group differences were identified by Wilcoxon signed rank test or Mann-Whitney U tests. Red dots indicated FDR-P was less than 0.05. #FDR-P < .1, *FDR-P < .05, **FDR-P < .01, ***FDR-P < .001. Abbreviations: FC, fold change.
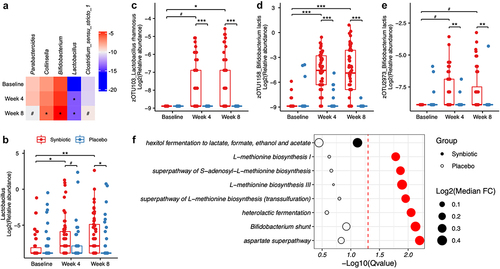
Spearman correlation analysis indicated a significant negative correlation between the decrease of Parabacteroides and the growth of plasma IL-10 in the synbiotic group (r = −0.208, P = .041). Moreover, the enrichments of several pathways concerning the amino acids or SCFAs biosynthesis were positively correlated with the increase of anti-inflammatory markers (plasma IL-10 or stool sIgA) (all P < .05) (). There was no measurable distinction in the modifications of stool acetate, propionate, butyrate, or total SCFAs between the two groups (Table S4 in Supplementary material).
Figure 4. Synbiotic-induced improvements in immune parameters were associated with alternations in gut microbiota. Correlation coefficients and significances were evaluated by Spearman correlation analysis between the alternations in immune markers and the changes in microbial genera or functional pathways by treatment group. Abbreviations: IL, interleukin; sIga, secretory IgA.
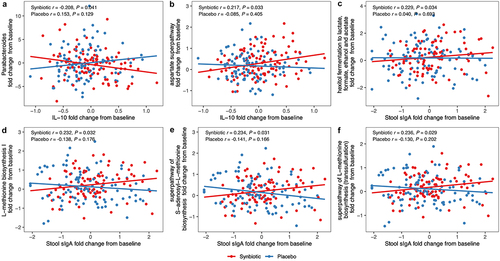
Synbiotic effects were modified by microbial enterotypes
The log-transformed pre-treatment P/B ratio exhibited a bimodal distribution (P = .006, Figure S6 in Supplementary material). Accordingly, all the subjects were stratified into two microbial enterotypes, low P/B ratio (LP, n = 65) and high P/B ratio (HP, n = 40), with a cutoff value of 0.1. Throughout the intervention period, no significant effect of synbiotic products on the P/B ratio was observed. At baseline, the HP group had a higher BMI (P = .031) but lower plasma high-density lipoprotein cholesterol (P = 0.031), saliva sIgA (P = 0.017), and stool sIgA (P < .001) than LP (Table S5 in Supplementary material).
As depicted in , plasma CRP continuously declined in the synbiotic_HP group (week 4: mean FC = 0.69, P = .002; week 8: mean FC = 0.61, P < .001). Moreover, the reduction was more pronounced in the synbiotic_HP group than that in the placebo (P = .006) and synbiotic_LP (P = .017). Similarly, synbiotic supplementation induced a drop of 19% in plasma IFN-γ in the HP group (P = .010), which was significantly different from the placebo (P = .017, ). Although no marked change was observed in plasma IL-10 in the synbiotic_HP group, it decreased by 17% in the placebo group (P < .010). Consequently, there was a rise in IL-10 (P = .002) in synbiotic_HP compared to the placebo (). At the end of the intervention, stool sIgA increased by 67% within synbiotic_HP (P = .007), resulting in a greater increase in the synbiotic_HP than that in the placebo (P = .008, ). However, no detectable difference in immune parameters was found between the synbiotic_LP and placebo. Furthermore, we observed an inverse correlation between CRP change and the baseline P/B ratio in the synbiotic group (r = −0.302, P = .003, ).
Figure 5. Differential responses to synbiotic supplementation in individuals with low or high pretreatment P/B ratio. (a-d) Comparison of the change from baseline in plasma CRP (a), IFN-gamma (b), IL-10 (c), and stool sIga (d) among the whole placebo (n = 53), synbiotic_LP (n = 31), and synbiotic_HP (n = 22). The point indicated adjusted mean fold changes from week 4 or week 8 to baseline, which was the least square means estimated using generalized linear models, with time (week 0, 4, 8), subgroup (placebo, synbiotic_LP, synbiotic_HP), and subgroup-by-time as fixed effects, subjects as a random effect, and baseline value as a covariate. Error bars indicated 95% confidence intervals. Due to similar variations of LP and HP in placebo group, the placebo group was analyzed as a whole to avoid small sample size of each enterotype group. (e) correlation between the CRP change and the pretreatment P/B ratio by treatment group. Coefficients and significances were evaluated by Spearman correlation analysis. (f) significantly altered genera following synbiotic supplementation in all the participants. Fold change was identified as the ratio of the relative abundance at week 8 and that at baseline. +FDR-P < 0.2, #FDR-P < .1, NOTE: *FDR-P < .05. Abbreviations: CRP, C-reactive protein; IFN, interferon; IL, interleukin; sIga, secretory IgA; synbiotic_LP, low P/B ratio group supplemented the synbiotic; synbiotic_HP, high P/B ratio group supplemented the synbiotic.
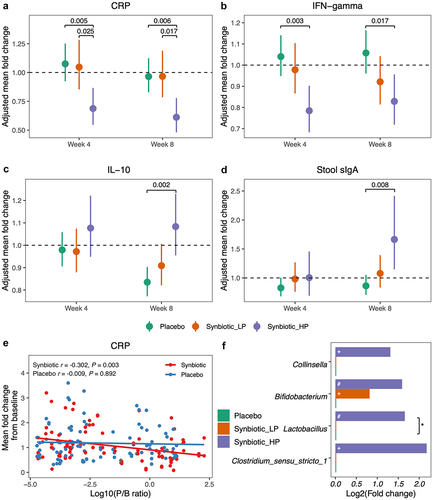
There was no substantial difference in alpha diversity before and after intervention in both HP and LP groups. However, principal component analysis of gut microbiota composition revealed a divergence following synbiotic supplementation in the HP group (week 4: P = .042; week 8: P = .033), but not in the LP group, suggesting a maladaptation of gut microbiota in LP (Figure S7 in Supplementary material). Furthermore, significant increases of Clostridium_sensu_stricto_1, Lactobacillus, and Collinsella observed in the whole synbiotic group were only found in HP (). Notably, Lactobacillus enhanced much more in synbiotic_HP than that in the placebo (FDR-P = .011). None of these bacteria significantly altered in both the synbiotic_LP group and the placebo group. Besides, synbiotic supplementation tended to induce a higher growth of Bifidobacterium in HP than that in the LP or placebo group, but their between-group differences were non-significant. No measurable difference was found in the functional capacity among synbiotic_HP, synbiotic_LP, and the whole placebo.
Discussion
In the current study, we found that daily supplementation of synbiotic supplements for 8 weeks altered systemic inflammation markers (plasma CRP, IFN-γ, and IL-10) and mucosal immunity markers (stool sIgA) in healthy adults. We also found that synbiotic supplementation increased beneficial bacteria (Collinsella, Lactobacillus, Bifidobacterium, and Clostridium_sensu_stricto_1) as well as functional pathways related to the biosynthesis of amino acids or SCFAs, whereas reduced certain potential pro-inflammation bacteria (Parabacteroides) compared to baseline. Moreover, alternations in IL-10 and stool sIgA were correlated with microbial variations induced by synbiotic supplementation. Importantly, the aforementioned synbiotic effects were more favorable in subjects with a higher pre-treatment P/B ratio.
Our results showed that synbiotic supplementation decreased pro-inflammatory biomarkers (CRP and IFN-γ) and increased anti-inflammatory cytokine (IL-10) among healthy adults. Existing evidence regarding the impacts of synbiotics on inflammatory markers in healthy populations is limited and has yielded inconsistent results. Some studies suggested that synbiotic supplementation did not alter systemic CRP, IL-10, or IFN-γ,Citation18–20 while others found an improvement in CRP or IL-10 following the synbiotic intervention.Citation21–23 However, it should be noted that the synbiotic formula used in the above-mentioned studies differs from the one applied in our study, and the efficacy of its major ingredients (probiotics) has been validated to be strain-specific. Previous evidence supported the immunity-enhancing properties of L. rhamnosus HN001 and B. lactis HN019, which represented the main components of the synbiotic used in our study. B. lactis HN019 has been extensively reported to enhance systemic cellular immunity, including T lymphocytes, polymorphonuclear cell phagocytosis, and NK cell killing activity.Citation16,Citation24 Likewise, L. rhamnosus HN001 has also been suggested to enhance natural and acquired immunity in animal and human experiments.Citation14,Citation25 Additionally, we also observed an increase in the concentration of fecal sIgA, which was widely used as a marker of mucosal immunity.Citation26 In line with this, several clinical trials have reported that daily supplementation of synbiotics increased fecal sIgA among healthy infants,Citation27 children,Citation28 adults,Citation29 and the elder population.Citation30 Hence, our results provided a better understanding of the ability of synbiotics to regulate systemic inflammation and mucosal immunity.
This study also found that synbiotic supplementation altered the gut microbiota composition and functional potentials. Firstly, zOTUs within L. rhamnosus or B. lactis dramatically increased in the synbiotic group, indirectly indicating the successful survival of the ingested B. lactis HN019 and L. rhamnosus HN001. This finding is consistent with previous probiotic intervention trials in infantsCitation31 or elderly subjects.Citation32 Secondly, the synbiotic intervention significantly increased several beneficial bacteria, including Bifidobacterium, Lactobacillus, Collinsella, and Clostridium_sensu_stricto_1. Bifidobacterium and Lactobacillus were widely used as probiotics for their perceived health-promoting effects,Citation33 including inhibiting pathogens, increasing the production of SCFA, and optimizing colonic function.Citation34 Collinsella and Clostridium_sensu_stricto_1 were SCFA-producing bacteria,Citation35 and indirectly involved in the process of immunoregulation.Citation36,Citation37 Thirdly, synbiotic consumption reduced Parabacteroides, which was associated with pro-inflammatory responses in Crohn’s disease and potentially involved in the pathogenesis of diabetes cardiovascular disease.Citation38 Lastly, synbiotic supplementation enriched metabolic pathways facilitating the biosynthesis of L-methionine, S-adenosyl-L-methionine, and SCFAs. These alternations were positively correlated with changes in anti-inflammatory biomarkers such as IL-10 and sIgA. Correspondingly, the microbial biosyntheses of L-methionine and S-adenosyl-L-methionine were involved in cognitive and metabolic health.Citation39,Citation40 SCFAs, particularly butyrate, played a crucial role in maintaining intestinal homeostasis through anti-inflammatory actions.Citation41,Citation42 Collectively, our results highlighted the beneficial impacts of synbiotic supplementation on gut microbiota, with the observed microbial variations being associated with the immunoregulatory changes induced by synbiotic supplementation.
Our results indicated a sex-specific response in immune parameters and gut microbiota composition to the synbiotic intervention. Although sex differences have been observed in immune responsesCitation43 and gut microbiota,Citation44 there are limited studies investigating the influence of gender on the immunomodulatory effect of synbiotics or probiotics. In an animal study, the expression of TNF-α was increased by a high-fat diet in male rats and then decreased with medium concentration following probiotic intervention.Citation45 Furthermore, sex differences have also been found in microbial effects of pre- and probiotic treatments in animal studies.Citation46 However, in a double-blind, placebo-controlled study involving patients with nonalcoholic fatty liver disease, probiotic supplementation reduced CRP levels, without any differences between men and women.Citation47 Notably, the sex-specific difference in response to synbiotics/probiotics might be attributed to sex hormones, which are associated with microbiota differences and systemic immunity.Citation46 Therefore, there is a need for more gender-focused research to gain valuable insights into sex-specific responses and comprehensively understand the underlying mechanisms.
Although several immune parameters were improved by synbiotic supplementation, the response effects showed larger individual differences. As reported in previous studies, baseline gut microbiota influenced the potential responsiveness to the same diet.Citation48 In this context, we observed that the synbiotic supplement exerted a more favorable effect on immune parameters and gut microbiota in individuals with a higher pre-treatment P/B ratio. To date, there are few studies investigating the effect of the pre-treatment P/B ratio on responses to the synbiotic supplement. Sandberg et al reported that individuals with a higher P/B ratio displayed a lower insulin response and a lower IL-6 concentration, independently of the intervention.Citation49 Song et al showed that probiotics intervention exerted a better effect on obesity-related biomarkers, which is associated with chronic low-grade inflammation,Citation50 in the obese population with Prevotella-dominant enterotype.Citation51 Some studies have proposed that individuals with Prevotella enterotype mainly depend on SCFA production to promote health,Citation52,Citation53 while individuals with Bacteroides enterotype are more likely to improve health via bile acids modification.Citation54 However, no remarkable difference in fecal SCFA shifts was detected between the synbiotics and placebo group in our study. Additional studies with metagenomics analyses are warranted to comprehensively understand the clinical relevance of the pre-treatment P/B ratio and its implications on the response to synbiotic supplementation.
There are several strengths of the present study. Firstly, to the best of our knowledge, this is the first study to find that the immunoregulatory and microbial effects of the synbiotic supplements are modified by the pre-treatment P/B ratio. Secondly, the participants were instructed to maintain their routine diet and physical activity. This was ensured by closely monitoring their dietary intakes and levels of physical exercise, thereby promoting stability in these lifestyle factors. However, there are certain limitations to acknowledge in this study. Firstly, although our results have demonstrated the beneficial effects of an 8-week duration of synbiotic supplementation, further investigations are required to assess the long-term effects of this intervention. Secondly, the 16S rRNA gene amplicon sequencing approach provided limited insights into the species or strain levels. Although we observed microbial changes at the zOTU level, additional comprehensive metagenomic studies would be desirable to gain insights into the gut microbiota dynamics.
In conclusion, an 8-week synbiotic supplementation altered immune parameters and modified gut microbiota in healthy adults. Furthermore, changes in anti-inflammatory markers (IL-10 and sIgA) were associated with microbial variations triggered by the synbiotic supplementation. Moreover, the immunomodulatory and microbial effects of synbiotic supplements were modified by enterotypes based on the basal P/B ratio. These findings provide evidence for the use of synbiotics to improve the immune function of healthy adults. The use of basal P/B ratio holds great promise for personalized supplementation of synbiotics for immunomodulation, but additional randomized controlled trials with larger numbers of participants are needed to confirm these findings.
Methods
Study participants
This study recruited 170 volunteers via advertisements in Tongji Medical School of Huazhong University of Science and Technology, Wuhan, China in October 2020. Inclusion criteria were: 18–65 years old, and BMI less than 35 kg/m2. Exclusion criteria were: known congenital or acquired immune deficiency diseases, allergic diseases, gastrointestinal disorders, and other acute or chronic diseases requiring treatment; use of the immunosuppressive drug, antibiotics, probiotics, prebiotics, synbiotics, or drug active on gastrointestinal motility within the preceding month; vaccination against influenza within the last 12 months; pregnancy or lactation; more than 5% weight change over the past three months; enrollment or planned enrollment in other research. After screening through a questionnaire regarding medical history, drug use, and other information, a total of 106 eligible participants were included in the study.
The study was approved by the Medical Ethics Committee of Tongji Medical School. Written informed consent was obtained from all subjects before enrollment. The clinical trial was registered on clinicaltrials.gov as NCT04960878.
Study design
The present study was a randomized, double-blind, placebo-controlled intervention study. Eligible participants were equally and randomly assigned into two groups using computer-generated random numbers after stratification by gender and BMI (<24 or ≥24 kg/m2) without a predetermined block size. Then, one group was administered a sachet of synbiotic powder (1.5 g): B. lactis HN019 1.5 × 108 CFU, L. rhamnosus HN001 7.5 × 107 CFU, 500 mg fructooligosaccharide, and a maltodextrin carrier, and the other group was administered a sachet of placebo powder (1.5 g) containing maltodextrin. During the intervention periods, participants were instructed to take one sachet dissolved in warm water (≤37°C) before breakfast every day for 8 weeks. Participants and outcome assessors were blind to trial allocation. To conceal allocation, all the supplements were identical in appearance, taste, and package.
Participants received a four-week dose of synbiotic or placebo at baseline and week 4, then they were instructed to return unused sachets at the next visit to evaluate compliance. If someone missed more than 10% of the supplement dose, he or she was defined as noncompliant and excluded from the study. During the intervention period, all subjects were required to maintain their routine diet and physical activity, other than restrict products containing prebiotics and/or probiotics according to labeling. A three-day 24-h dietary record survey and an international physical activity questionnaire (short form) were completed at baseline, week 4, and week 8 to confirm whether dietary intake and physical activity were stable during the study. Information on medications, supplement use, and adverse events were self-reported by participants throughout the intervention. The primary outcome of this study was the CRP change. In addition, we evaluated changes in other immune parameters and gut microbial alterations as secondary outcomes.
Assessments of clinical outcomes
Fasting blood samples, stool samples, and saliva samples were collected at baseline (day 0), week 4 (day 28), and week 8 (day 56). More details were described in the Supplementary material.
Plasma concentrations of CRP were determined with commercial ELISAs kits (R&D System Inc., MN, USA). Plasma levels of IFN-γ, IL-1β, IL-6, IL-8, IL-10, and TNF-α were detected using the MSD V-Plex Proinflammatory Panel 1 human Kits (Meso Scale Discovery, Rockville, MD, USA) and read using the MESO sector S 600 (Meso Scale Discovery). The levels of sIgA in saliva and stool samples were measured utilizing ELISA kits (EAGLE BIOSCIENCES, INC., Nashua, NH) according to the manufacturer’s instructions.
Flow cytometric analysis was performed to assess the absolute counts of leukocyte subpopulations. Firstly, 50 μL of each well-homogenized whole blood sample was mixed with 20 μL of anti-CD3-PercpCy5.5/anti-CD19-APC/anti-CD56-PE/anti-CD14-FITC/anti-CD45-APC-Cy7 regent in a TruCount Tube, which contained a lyophilized pallet that released a known number of fluorescent beads during sample preparation. All antibodies were purchased from BD Biosciences (San Jose, CA, USA). Secondly, the samples were incubated for 15 min at room temperature in the dark after being thoroughly vortexed. Thirdly, 450 μL of FACS lysis solution was added to each tube, and the tubes were incubated for 15 min at room temperature in the dark. Finally, data were acquired on a BD FACSCAria III flow cytometer (BD Life Science, San Jose, CA, USA). The counts of total white blood cells (CD45+), T cells (CD3+), B cells (CD3-CD19+), natural killer (NK) cells (CD3−CD56+), and monocyte (CD14+) were analyzed with FlowJo (Tree Star, Ashland, OR, USA).
The methodologies for assessing the occurrence, duration, and severity of URTI episodes, physiological and biochemical markers, and SCFAs were detailed in the Supplementary material.
16S rRNA gene sequencing and microbial analysis
The fecal microbiota was measured using 16S rRNA gene amplicon sequencing as previously described.Citation55 Briefly, fecal bacteria DNA was extracted using a QIAamp Fast DNA Stool Mini Kit (Qiagen, Valencia, CA, USA), and the V3-V4 region of the 16S rRNA gene was amplified with barcode-indexed primers (338F and 806 R), then sequencing was performed using the Illumina MiSeq platform (Illumina, San Diego, CA, USA). Raw sequencing data can be available from NCBI Sequence Read Archive (SRA) database under the BioProject accession number PRJNA903962.
Sequencing data were processed using the Quantitative Insights Into Microbiota Ecology platform (QIIME, Boulder, CO, USA, V.1.9.1).Citation56 Paired-end sequencing reads were merged, trimmed of primers and distal bases, quality filtered, and chimeras were removed using USEARCH V11.0.Citation57 Then, reads were denoised into zOTUs utilizing UNOISE3.Citation58 Taxonomy was assigned a taxonomical classification using the SILVA database (version 138) with a cutoff of 0.8. For significantly altered zOTUs during the study, we further searched the sequences using BLAST against the reference database from NCBI RefSeq (Accession date: January 10, 2023), with more than 99% identity to determine their assigned species. The zOTUs table was rarefied to the lowest read number. Based on the rarefied zOTUs table, α-diversity (the observed zOTUs and Shannon’s index) and β-diversity quantified Euclidean or Weighted UniFrac distances in each group were calculated using the vegan and phyloseq R package. The function prediction was performed using PICRUSt2Citation59 and MetaCyc database at the pathway level.
Statistical analysis
At a two-sided 0.05 significance level and with 80% statistical power, sample size calculation was based on a 0.1 difference in the CRP change with a standard deviation (SD) of 0.17 in a repeated measure design,Citation21 using the degree of correlation between successive measures of 0.8, and a sample size of 88 was required. Due to potential dropouts (20%), the number of enrolled subjects was increased to 106.
Continuous baseline characteristics were presented as mean ± SD or median and interquartile range (IQR) according to the normality, which was tested by the Shapiro-Wilk test and Q-Q plots. Categorical characteristics were summarized as numbers (percentages). Treatment effects on immune parameters, physiological and biochemical markers, and stool SCFAs were assessed by generalized linear mixed models, which included the change from each follow-up visit to baseline as the dependent variable, time (week 0, 4, 8), group (synbiotic, placebo), and group-by-time as fixed effects, subjects as a random effect, and baseline value of each variable as a covariate. Least square means from group-by-time interaction were adopted to estimate within-group changes and between-group differences. Due to more than 30% of samples being below the detection limit, plasma IL-1β was excluded from the following statistical analysis. For variables in skewed distribution, the ln-transformation was applied to alleviate the skewed distributions, and results were described as fold change from each follow-up visit to baseline.
The variation in beta diversity was evaluated using PERMANOVA with the adonis function in the vegan package and visualized on principal coordinates analysis using the phyloseq package. For alpha diversity indices, microbiota taxa, and functional pathways, treatment effects were calculated using a linear mixed model with lme4 package, and within-group differences were assessed utilizing the Wilcoxon signed-rank test. The correlation between significantly altered immune markers and significantly modified microbiota taxa and pathways was evaluated by Spearman’s rank test. FDR was estimated using the Benjamini-Hochberg method to control for multiple testing, and a cutoff of 0.1 was considered to be statistically significant unless stated otherwise.
The pre-treatment log10-transformed P/B ratio was calculated using the relative abundance of Prevotella and Bacteroides genera. According to the bimodal distribution of log10 (P/B) ratio,Citation60 all the participants were stratified into two microbial enterotypes, low (LP) and high P/B ratio (HP), with a cutoff value of 0.1. One individual was excluded for no detectable Bacteroides. The bimodal distribution of the log10 (P/B) ratio was tested by the diptest package. Because LP and HP in the placebo did not differ in variation in all clinical outcomes and gut microbiota composition, we treated the placebo group as a whole to avoid a small sample size in each enterotype subgroup. Differential responses to synbiotic supplementation in LP and HP were evaluated with generalized linear mixed models, with time, subgroup (synbiotic_LP, synbiotic_HP, the whole placebo), and subgroup-by-time as a fixed effect. The statistical significance was set at two-sided P<0.05. Statistical analyses were performed with SAS version 9.4 (SAS Institute Inc., Cary, NC, USA) and R version 4.1.3 (The R Foundation for Statistical Computing, Vienna, Austria).
Contributors
XQL: conceptualization, formal analysis, data curation, and writing-original draft; SH: formal analysis and methodology; JWY: data curation and revising-original draft; XBP and LK: data curation, revising-original draft, and supervision; LYL, ZHX, LZ, and ZP: formal analysis and data curation; XLZ, XGZ, QCH, and ZLS: revising-original draft; LGL: conceptualization and supervision. All authors contributed to the article and approved the submitted version.
Supplemental Material
Download MS Word (1.2 MB)Acknowledgments
We would like to thank all the participants for their active cooperation. We would also like to thank Life-Space Global Microbiome Center for providing the synbiotic products and placebo used in this study at no cost.
Disclosure statement
No potential conflict of interest was reported by the author(s).
Data availability statement
Sequencing data were deposited in the NCBI Sequence Read Archive database under BioProject PRJNA903962. The remaining individual participant data will be available from the corresponding authors upon reasonable request.
Supplementary material
Supplemental data for this article can be accessed online at https://doi.org/10.1080/19490976.2023.2247025
Additional information
Funding
References
- Zheng D, Liwinski T, Elinav E. Interaction between microbiota and immunity in health and disease. Cell Res. 2020;30(6):492–16. doi:10.1038/s41422-020-0332-7.
- Libertucci J, Young VB. The role of the microbiota in infectious diseases. Nat Microbiol. 2019;4(1):35–45. doi:10.1038/s41564-018-0278-4.
- Swanson KS, Gibson GR, Hutkins R, Reimer RA, Reid G, Verbeke K, Scott KP, Holscher HD, Azad MB, Delzenne NM, et al. The international scientific association for probiotics and prebiotics (ISAPP) consensus statement on the definition and scope of synbiotics. Nat Rev Gastroenterol Hepatol. 2020;17(11):687–701. doi:10.1038/s41575-020-0344-2.
- Fernandes R, Do Rosario VA, Mocellin MC, Kuntz MGF, Trindade E. Effects of inulin-type fructans, galacto-oligosaccharides and related synbiotics on inflammatory markers in adult patients with overweight or obesity: a systematic review. Clin Nutr. 2017;36(5):1197–1206. doi:10.1016/j.clnu.2016.10.003.
- Kazemi A, Soltani S, Ghorabi S, Keshtkar A, Daneshzad E, Nasri F, Mazloomi SM. Effect of probiotic and synbiotic supplementation on inflammatory markers in health and disease status: A systematic review and meta-analysis of clinical trials. Clin Nutr. 2020;39(3):789–819. doi:10.1016/j.clnu.2019.04.004.
- Zheng HJ, Guo J, Jia Q, Huang YS, Huang WJ, Zhang W, Zhang F, Liu WJ, Wang Y. The effect of probiotic and synbiotic supplementation on biomarkers of inflammation and oxidative stress in diabetic patients: a systematic review and meta-analysis of randomized controlled trials. Pharmacol Res. 2019;142:303–313. doi:10.1016/j.phrs.2019.02.016.
- Zheng HJ, Guo J, Wang Q, Wang L, Wang Y, Zhang F, Huang WJ, Zhang W, Liu WJ, Wang Y. Probiotics, prebiotics, and synbiotics for the improvement of metabolic profiles in patients with chronic kidney disease: A systematic review and meta-analysis of randomized controlled trials. Crit Rev Food Sci Nutr. 2021;61(4):577–598. doi:10.1080/10408398.2020.1740645.
- McLoughlin RF, Berthon BS, Jensen ME, Baines KJ, Wood LG. Short-chain fatty acids, prebiotics, synbiotics, and systemic inflammation: a systematic review and meta-analysis. Am J Clin Nutr. 2017;106(3):930–945. doi:10.3945/ajcn.117.156265.
- O’Connor LE, Gahche JJ, Herrick KA, Davis CD, Potischman N, Vargas AJ. Nonfood prebiotic, probiotic, and synbiotic use has increased in US adults and children from 1999 to 2018. Gastroenterology. 2021;161(2):476–486.e473. doi:10.1053/j.gastro.2021.04.037.
- Costea PI, Hildebrand F, Arumugam M, Backhed F, Blaser MJ, Bushman FD, de Vos WM, Ehrlich SD, Fraser CM, Hattori M, et al. Enterotypes in the landscape of gut microbial community composition. Nat Microbiol. 2018;3(1):8–16. doi:10.1038/s41564-017-0072-8.
- Hjorth MF, Christensen L, Larsen TM, Roager HM, Krych L, Kot W, Nielsen DS, Ritz C, Astrup A. Pretreatment Prevotella-to-Bacteroides ratio and salivary amylase gene copy number as prognostic markers for dietary weight loss. Am J Clin Nutr. 2020;111(5):1079–1086. doi:10.1093/ajcn/nqaa007.
- Hjorth MF, Roager HM, Larsen TM, Poulsen SK, Licht TR, Bahl MI, Zohar Y, Astrup A. Erratum: Pre-treatment microbial Prevotella-to-Bacteroides ratio, determines body fat loss success during a 6-month randomized controlled diet intervention. Int J Obes (Lond). 2018;42(2):284. doi:10.1038/ijo.2018.1.
- Braat H, van den Brande J, van Tol E, Hommes D, Peppelenbosch M, van Deventer S. Lactobacillus rhamnosus induces peripheral hyporesponsiveness in stimulated CD4+ T cells via modulation of dendritic cell function. Am J Clin Nutr. 2004;80(6):1618–1625. doi:10.1093/ajcn/80.6.1618.
- Gill HS, Rutherfurd KJ, Prasad J, Gopal PK. Enhancement of natural and acquired immunity by Lactobacillus rhamnosus (HN001), Lactobacillus acidophilus (HN017) and Bifidobacterium lactis (HN019). Br J Nutr. 2000;83(2):167–176. doi:10.1017/s0007114500000210.
- Chiang BL, Sheih YH, Wang LH, Liao CK, Gill HS. Enhancing immunity by dietary consumption of a probiotic lactic acid bacterium (Bifidobacterium lactis HN019): optimization and definition of cellular immune responses. Eur J Clin Nutr. 2000;54(11):849–855. doi:10.1038/sj.ejcn.1601093.
- Miller LE, Lehtoranta L, Lehtinen MJ. The effect of Bifidobacterium animalis ssp. lactis HN019 on cellular immune function in healthy elderly subjects: systematic review and meta-analysis. Nutrients. 2017;9(3). doi:10.3390/nu9030191.
- Drescher LS, Thiele S, Mensink GB. A New index to measure healthy food diversity better reflects a healthy diet than traditional measures ,2. J Nutr. 2007;137(3):647–651. doi:10.1093/jn/137.3.647.
- Macfarlane S, Cleary S, Bahrami B, Reynolds N, Macfarlane GT. Synbiotic consumption changes the metabolism and composition of the gut microbiota in older people and modifies inflammatory processes: a randomised, double-blind, placebo-controlled crossover study. Aliment Pharmacol Ther. 2013;38(7):804–816. doi:10.1111/apt.12453.
- Gomes AC, de Sousa RG, Botelho PB, Gomes TL, Prada PO, Mota JF. The additional effects of a probiotic mix on abdominal adiposity and antioxidant status: A double-blind, randomized trial. Obesity (Silver Spring). 2017;25(1):30–38. doi:10.1002/oby.21671.
- Quero CD, Manonelles P, Fernandez M, Abellan-Aynes O, Lopez-Plaza D, Andreu-Caravaca L, Hinchado MD, Galvez I, Ortega E. Differential Health effects on inflammatory, immunological and stress parameters in professional soccer players and sedentary individuals after consuming a synbiotic. A triple-blinded, randomized, placebo-controlled pilot study. Nutrients. 2021;13(4). doi:10.3390/nu13041321.
- Costabile A, Bergillos-Meca T, Rasinkangas P, Korpela K, de Vos WM, Gibson GR. Effects of soluble corn fiber alone or in synbiotic combination with Lactobacillus rhamnosus GG and the pilus-deficient derivative GG-PB12 on fecal microbiota, metabolism, and markers of immune function: a randomized, double-blind, placebo-controlled, Crossover study in healthy elderly (Saimes study). Front Immunol. 2017;8:1443. doi:10.3389/fimmu.2017.01443.
- Khabbazi A, Ahangari Maleki M, Soltani-Zangbar MS, Yousefi M, Malek Mahdavi A. Effects of synbiotic supplementation on regulatory T cells’ response in patients with axial spondyloarthritis: a randomized double-masked placebo-controlled trial. Food Funct. 2022;13(24):12733–12741. doi:10.1039/d2fo01377k.
- Widhani A, Djauzi S, Suyatna FD, Dewi BE. Changes in gut microbiota and systemic inflammation after synbiotic supplementation in patients with systemic lupus erythematosus: a randomized, double-blind, placebo-controlled trial. Cells. 2022;11(21). doi:10.3390/cells11213419.
- Cheng J, Laitila A, Ouwehand AC. Bifidobacterium animalis subsp. lactis HN019 effects on gut Health: a review. Front Nutr. 2021;8:790561. doi:10.3389/fnut.2021.790561.
- Sheih YH, Chiang BL, Wang LH, Liao CK, Gill HS. Systemic immunity-enhancing effects in healthy subjects following dietary consumption of the lactic acid bacterium Lactobacillus rhamnosus HN001. J Am Coll Nutr. 2001;20(2 Suppl):149–156. doi:10.1080/07315724.2001.10719027.
- Gutzeit C, Magri G, Cerutti A. Intestinal IgA production and its role in host-microbe interaction. Immunol Rev. 2014;260(1):76–85. doi:10.1111/imr.12189.
- Beghin L, Tims S, Roelofs M, Rouge C, Oozeer R, Rakza T, Chirico G, Roeselers G, Knol J, Roze JC, et al. Fermented infant formula (with Bifidobacterium breve C50 and Streptococcus thermophilus O65) with prebiotic oligosaccharides is safe and modulates the gut microbiota towards a microbiota closer to that of breastfed infants. Clin Nutr. 2021;40(3):778–787. doi:10.1016/j.clnu.2020.07.024.
- Nocerino R, Paparo L, Terrin G, Pezzella V, Amoroso A, Cosenza L, Cecere G, De Marco G, Micillo M, Albano F, et al. Cow’s milk and rice fermented with Lactobacillus paracasei CBA L74 prevent infectious diseases in children: A randomized controlled trial. Clin Nutr. 2017;36(1):118–125. doi:10.1016/j.clnu.2015.12.004.
- Childs CE, Roytio H, Alhoniemi E, Fekete AA, Forssten SD, Hudjec N, Lim YN, Steger CJ, Yaqoob P, Tuohy KM, et al. Xylo-oligosaccharides alone or in synbiotic combination with Bifidobacterium animalis subsp. lactis induce bifidogenesis and modulate markers of immune function in healthy adults: a double-blind, placebo-controlled, randomised, factorial cross-over study. Br J Nutr. 2014;111(11):1945–1956. doi:10.1017/S0007114513004261.
- Ouwehand AC, Tiihonen K, Saarinen M, Putaala H, Rautonen N. Influence of a combination of Lactobacillus acidophilus NCFM and lactitol on healthy elderly: intestinal and immune parameters. Br J Nutr. 2009;101(3):367–375. doi:10.1017/s0007114508003097.
- Murphy R, Morgan XC, Wang XY, Wickens K, Purdie G, Fitzharris P, Otal A, Lawley B, Stanley T, Barthow C, et al. Eczema-protective probiotic alters infant gut microbiome functional capacity but not composition: sub-sample analysis from a RCT. Benef Microbes. 2019;10(1):5–17. doi:10.3920/BM2017.0191.
- Ahmed M, Prasad J, Gill H, Stevenson L, Gopal P. Impact of consumption of different levels of Bifidobacterium lactis HN019 on the intestinal microflora of elderly human subjects. J Nutr Health Aging. 2007;11:26–31.
- Andrade RC, Figueredo CS, de Carvalho Alves J, Roselino MN. Evidence and updates on non-dairy synbiotic beverage development. Recent Pat Biotechnol. 2022;16(3):214–225. doi:10.2174/1872208316666220303095807.
- Rinninella E, Raoul P, Cintoni M, Franceschi F, Miggiano GAD, Gasbarrini A, Mele MC. What is the healthy gut microbiota composition? A changing ecosystem across age, environment, diet, and diseases. Microorganisms. 2019;7(1). doi:10.3390/microorganisms7010014.
- Qin P, Zou Y, Dai Y, Luo G, Zhang X, Xiao L. Characterization a novel butyric acid-producing bacterium Collinsella aerofaciens subsp. Shenzhenensis subsp. Nov. Microorganisms. 2019;7(3). doi:10.3390/microorganisms7030078.
- Matson V, Fessler J, Bao R, Chongsuwat T, Zha Y, Alegre ML, Luke JJ, Gajewski TF. The commensal microbiome is associated with anti-PD-1 efficacy in metastatic melanoma patients. Sci. 2018;359(6371):104–108. doi:10.1126/science.aao3290.
- Gao X, Du L, Randell E, Zhang H, Li K, Li D. Effect of different phosphatidylcholines on high fat diet-induced insulin resistance in mice. Food Funct. 2021;12(4):1516–1528. doi:10.1039/d0fo02632h.
- Ezeji JC, Sarikonda DK, Hopperton A, Erkkila HL, Cohen DE, Martinez SP, Cominelli F, Kuwahara T, Dichosa AEK, Good CE, et al. Parabacteroides distasonis: intriguing aerotolerant gut anaerobe with emerging antimicrobial resistance and pathogenic and probiotic roles in human health. Gut Microbes. 2021;13(1):1922241. doi:10.1080/19490976.2021.1922241.
- Anstee QM, Day CP. S-adenosylmethionine (SAMe) therapy in liver disease: a review of current evidence and clinical utility. J Hepatol. 2012;57(5):1097–1109. doi:10.1016/j.jhep.2012.04.041.
- Sharma A, Gerbarg P, Bottiglieri T, Massoumi L, Carpenter LL, Lavretsky H, Muskin PR, Brown RP, Mischoulon D, Work Group of the American Psychiatric Association Council on R. S-Adenosylmethionine (SAMe) for neuropsychiatric disorders: A clinician-oriented review of research. J Clin Psychiatry. 2017;78(6):e656–e667. doi:10.4088/JCP.16r11113.
- Parada Venegas D, De la Fuente MK, Landskron G, Gonzalez MJ, Quera R, Dijkstra G, Harmsen HJM, Faber KN, Hermoso MA. Short Chain Fatty Acids (SCFAs)-mediated gut Epithelial and immune regulation and its Relevance for inflammatory bowel diseases. Front Immunol. 2019;10:277. doi:10.3389/fimmu.2019.00277.
- Yao Y, Cai X, Fei W, Ye Y, Zhao M, Zheng C. The role of short-chain fatty acids in immunity, inflammation and metabolism. Crit Rev Food Sci Nutr. 2022;62(1):1–12. doi:10.1080/10408398.2020.1854675.
- Wilkinson NM, Chen HC, Lechner MG, Su MA. Sex differences in immunity. Annu Rev Immunol. 2022;40:75–94. doi:10.1146/annurev-immunol-101320-125133.
- Dominianni C, Sinha R, Goedert JJ, Pei Z, Yang L, Hayes RB, Ahn J, Wilson BA. Sex, body mass index, and dietary fiber intake influence the human gut microbiome. PLoS One. 2015;10(4):e0124599. doi:10.1371/journal.pone.0124599.
- Choi Y, Choi SI, Kim N, Nam RH, Jang JY, Na HY, Shin CM, Lee DH, Min H, Kim YR, et al. Effect of Clostridium butyricum on high-fat diet-induced intestinal inflammation and production of short-chain fatty acids. Dig Dis Sci. 2023;68(6):2427–2440. doi:10.1007/s10620-023-07835-2.
- Vemuri R, Sylvia KE, Klein SL, Forster SC, Plebanski M, Eri R, Flanagan KL. The microgenderome revealed: sex differences in bidirectional interactions between the microbiota, hormones, immunity and disease susceptibility. Semin Immunopathol. 2019;41(2):265–275. doi:10.1007/s00281-018-0716-7.
- Derosa G, Guasti L, D’Angelo A, Martinotti C, Valentino MC, Di Matteo S, Bruno GM, Maresca AM, Gaudio GV, Maffioli P. Probiotic Therapy with VSL#3((R)) in patients with NAFLD: A randomized clinical trial. Front Nutr. 2022;9:846873. doi:10.3389/fnut.2022.846873.
- Hou Q, Zhao F, Liu W, Lv R, Khine WWT, Han J, Sun Z, Lee YK, Zhang H. Probiotic-directed modulation of gut microbiota is basal microbiome dependent. Gut Microbes. 2020;12(1):1736974. doi:10.1080/19490976.2020.1736974.
- Sandberg J, Kovatcheva-Datchary P, Bjorck I, Backhed F, Nilsson A. Abundance of gut Prevotella at baseline and metabolic response to barley prebiotics. Eur J Nutr. 2019;58(6):2365–2376. doi:10.1007/s00394-018-1788-9.
- Santoro A, Guidarelli G, Ostan R, Giampieri E, Fabbri C, Bertarelli C, Nicoletti C, Kadi F, de Groot L, Feskens E, et al. Gender-specific association of body composition with inflammatory and adipose-related markers in healthy elderly Europeans from the NU-AGE study. Eur Radiol. 2019;29(9):4968–4979. doi:10.1007/s00330-018-5973-2.
- Song EJ, Han K, Lim TJ, Lim S, Chung MJ, Nam MH, Kim H, Nam YD. Effect of probiotics on obesity-related markers per enterotype: a double-blind, placebo-controlled, randomized clinical trial. EPMA J. 2020;11(1):31–51. doi:10.1007/s13167-020-00198-y.
- Hjorth MF, Blaedel T, Bendtsen LQ, Lorenzen JK, Holm JB, Kiilerich P, Roager HM, Kristiansen K, Larsen LH, Astrup A. Prevotella-to-Bacteroides ratio predicts body weight and fat loss success on 24-week diets varying in macronutrient composition and dietary fiber: results from a post-hoc analysis. Int J Obes (Lond). 2019;43(1):149–157. doi:10.1038/s41366-018-0093-2.
- Christensen L, Roager HM, Astrup A, Hjorth MF. Microbial enterotypes in personalized nutrition and obesity management. Am J Clin Nutr. 2018;108(4):645–651. doi:10.1093/ajcn/nqy175.
- Gu Y, Wang X, Li J, Zhang Y, Zhong H, Liu R, Zhang D, Feng Q, Xie X, Hong J, et al. Analyses of gut microbiota and plasma bile acids enable stratification of patients for antidiabetic treatment. Nat Commun. 2017;8(1):1785. doi:10.1038/s41467-017-01682-2.
- Li X, Yin J, Zhu Y, Wang X, Hu X, Bao W, Huang Y, Chen L, Chen S, Yang W, et al. Effects of whole Milk supplementation on gut microbiota and cardiometabolic biomarkers in subjects with and without Lactose Malabsorption. Nutrients. 2018;10(10). doi:10.3390/nu10101403.
- Kuczynski J, Stombaugh J, Walters WA, Gonzalez A, Caporaso JG, Knight R. Using QIIME to analyze 16S rRNA gene sequences from microbial communities. Curr Protoc Microbiol. 2012;27(1): Chapter 1:Unit 1E 5. doi:10.1002/9780471729259.mc01e05s27.
- Edgar RC. Search and clustering orders of magnitude faster than BLAST. Bioinformatics. 2010;26(19):2460–2461. doi:10.1093/bioinformatics/btq461.
- Edgar RC. UNOISE2: Improved error-correction for Illumina 16S and ITS amplicon sequencing. bioRxiv. 2016. doi:10.1101/081257.
- Douglas GM, Maffei VJ, Zaneveld JR, Yurgel SN, Brown JR, Taylor CM, Huttenhower C, Langille MGI. Picrust2 for prediction of metagenome functions. Nat Biotechnol. 2020;38(6):685–688. doi:10.1038/s41587-020-0548-6.
- Roager HM, Licht TR, Poulsen SK, Larsen TM, Bahl MI. Microbial enterotypes, inferred by the prevotella-to-bacteroides ratio, remained stable during a 6-month randomized controlled diet intervention with the new Nordic diet. Appl Environ Microbiol. 2014;80(3):1142–1149. doi:10.1128/AEM.03549-13.