ABSTRACT
Bacteria synchronize social behaviors via a cell-cell communication and interaction mechanism termed as quorum sensing (QS). QS has been extensively studied in monocultures and proved to be intensively involved in bacterial virulence and infection. Despite the role QS plays in pathogens during laboratory engineered infections has been proved, the potential functions of QS related to pathogenesis in context of microbial consortia remain poorly understood. In this review, we summarize the basic molecular mechanisms of QS, primarily focusing on pathogenic microbes driving gastrointestinal (GI) infections. We further discuss how GI pathogens disequilibrate the homeostasis of the indigenous microbial consortia, rebuild a realm dominated by pathogens, and interact with host under worsening infectious conditions via pathogen-biased QS signaling. Additionally, we present recent applications and main challenges of manipulating QS network in microbial consortia with the goal of better understanding GI bacterial sociality and facilitating novel therapies targeting bacterial infections.
Introduction
The human body is a large reservoir of microorganisms that colonize various parts of the body, including the skin, mouth, reproductive organs, and gastrointestinal (GI) tract. These microorganisms, primarily bacteria, have a close relationship with the human host and play a crucial role in maintaining a healthy state.Citation1 Particularly in the GI tract, these commensal microbes perform important functions such as providing nutrients to the host, developing the immune system, and preventing the colonization of pathogenic microbes.Citation2 The proper structure of the GI microbiota is essential for healthy gut functions, which rely on effective cell-cell communication and interaction. Quorum sensing (QS) is a universal communication system used by bacteria to coordinate social behaviors that shape the structure and function of polymicrobial communities. QS triggers changes in global gene expression by responding to different autoinducers (AIs) secreted by bacteria or even the host.Citation3–6 The accumulation of AIs in the human microbiota is closely associated with the bacterial composition. When a bacterial infection occurs in the human body, the invading pathogens disrupt the intrinsic balance of the signaling network and deploy their own AI signals to recruit other synergistic accomplices, forming denser polymicrobial biofilms and increasing toxin production, thereby worsening the infectious situation.Citation7,Citation8
Quorum sensing systems have been identified in various bacteria isolated from the human body, including pathogenic bacteria such as Fusobacterial nucleaum,Citation9 Helicobacter pylori,Citation10 Escherichia coli,Citation11 Salmonella enterica,Citation12 and probiotics like lactobacillus. Citation13 QS signaling systems have been extensively studied in these individual bacteria and have been shown to be involved in various processes related to bacterial colonization, virulence expression, and biofilm formation. However, it is still challenging to understand how these systems work together to establish an effective bacterial communication network and maintain bacterial community homeostasis in multispecies consortiums like the human gut microflora. Infection models have provided valuable insights into the QS-regulated social behaviors of bacteria in the context of multispecies communities. In this review, we will discuss the basic molecular mechanisms of QS in infectious pathogens and highlight the role of QS in exacerbating infectious conditions. Our aim is to demonstrate how these studies contribute to our current understanding of QS in bacteria associated with the human host, both in terms of fundamental knowledge and practical applications.
Classical autoinducers identified in GI microbiota
Quorum sensing (QS) is a regulatory mechanism that controls sporulation, biofilm production, virulence secretion, and interactive relationships of bacteria, including interspecies competition, cooperation, and kinship inference.Citation14–16 QS relies on the production, release, and detection of extracellular signaling molecules called autoinducers (AIs). Gram-negative (Gram−) bacteria primarily use species-specific acyl-homoserine lactone (AHL) as their AI, while Gram-positive (Gram+) bacteria mainly use autoinducing peptides (AIPs). Another universal language used by both Gram+ and Gram− bacteria is autoinducer-2 (AI-2), also known as furanosyl borate diester or tetrahydroxy furan. While numerous studies have revealed the regulatory role of bacterial AIs in controlling physiological functions in certain bacteria, we are particularly interested in exploring the role of bacterial AIs in inter-species and inter-kingdom communication and interaction, especially in the human GI tract.
AHL-based signaling facilitates bacterial adaptation in the GI tract
AHLs are the most common AIs utilized by Gram− bacteria.Citation17 These compounds consist of a core N-acylated homoserine lactone ring and a fatty acid chain of 4–18 carbon atoms in length. Moreover, AHLs can have various modifications on their acyl chains, adding further diversity to the signaling molecules.Citation18 AHLs are synthesized by LuxI type synthases via deriving the lactone moiety, an intermediate of fatty acid biosynthesis, from S-adenosylmethionine (SAM), and sensed by LuxR type receptor, a cytoplasmic transcription factor with two functional domains for AHL and DNA binding.Citation19 Usually, the LuxI and LuxR are coexisted in human pathogens, such as Pseudomonas aeruginosa,Citation20 Yersinia enterocolitica Citation21 and Burkholderia cepacia,Citation22 and they are crucial to produce virulence factors, colonization and infection, biofilm formation and antimicrobial resistance.Citation23–25
AHL type molecules have been detected in sputum and saliva of infected patients,Citation26,Citation27 infected woundsCitation28 and human feces of gastrointestinal disease patients as well as healthy subjects,Citation29,Citation30 which suggests a potential regulatory role of AHLs playing in human GI microbiota. Although the evidence of the AHL production by commensal bacteria are still lacking, however, there exist quantities of bacteria in the human gut possessing LuxR solos/orhpans, such as E. coli, S. enterica, Enterobacter spp., and Klebsiella pneumoniae.Citation31 These bacteria encode a LuxR homolog named SdiA, but do not encode a partner LuxI homolog or synthesize their own AHLs, which enables them to sense and respond to AHLs produced by other members in the microbiota. A recent study investigated the presence and expression of AHL synthases and receptor genes in human gut during IBD by in silico approach.Citation32 Although no known AHL synthase was identified, orphan LuxR homologs in human gut bacteria were observed and reported differential expressions in different Bacteroides in IBD against non-IBD group of people.Citation32 Perceiving exogenic AHLs might be an advanced survival strategy for bacteria, which facilitates them to sense and adjust to the changeable living environment. For example, an enterohemorrhagic E. coli (EHEC) O157:H7 would activate gene expression related with acid resistance when sensing surrounding AHLs.Citation33,Citation34 This may be the reason explaining how EHEC survive when passed through bovine acidic stomachs ().
AI-2 based signaling maintains the homeostasis of microbial barrier to against pathogen invasion
AI-2 molecules are widely accepted as a universal bacterial language allowing for interspecies communications and interactions. They are encoded by LuxS, which is ubiquitous and conserved in both Gram+ and Gram− bacteria.Citation35 Moreover, AI-2 molecules have also been detected in stools from healthy human, suggesting that AI-2 is normally present in human GI tract.Citation36
GI bacteria are expected to utilize AI-2 strengthening the beneficial interactions, thus structure homeostatic bacterial consortia and protect host against colonization of pathogens. It has been demonstrated that antibiotic treatment to mice would eliminate Firmicute in their intestine and increase the infection risk by Salmonella enterica serovar Typhimurium.Citation37,Citation38 Thereafter, the author introduces an engineered E. coli to the mice aiming in restoring the concentration of AI-2 in the mouse gut. The test result shows that the introduced E. coli increases intestinal AI-2 level, thereafter favors the expansion of Firmicutes phylum in the mouse gut.Citation38 This suggests the regulating role of AI-2 in maintaining homeostasis of GI flora. Besides, AI-2 can also strengthen intestinal barrier against the invasion by pathogens. As demonstrated by Hsiao, AI-2 produced by Ruminococcus obeum, a commensal GI bacterium, can protect mice against V. cholerae colonization ().Citation39 It is interesting to note that the expression of luxS gene in R. obeum was upregulated in response to V. cholerae, which reflects an immediate reaction on signaling communication by commensals when they get threated by unexpected invaders.
Competition between symbiotic and pathogenic bacteria based on AIP
AIP is a series of small, secreted peptides, mainly deployed by Gram+ for QS signaling. These peptides are encoded as a precursor from the QS operon, then processed and secreted by a specialized system named ATP-binding cassette transporter. Secreted AIPs accumulate with an increase of the bacteria density, subsequently be detected by the two-component sensor histidine kinases, such as Agr system in Streptococcus and Firmicutes.Citation17,Citation40 Interplay between AIP and sensor kinase would onset the phosphorylation of the cytoplasmic response regulator that controls the transcription of QS-regulated genes.
The interplay between AIP and its receptors is strongly species specific, that is, the non-cognate AIP can inhibit QS signaling in other strains.Citation41,Citation42 One recent study presents that AIPs produced by Agr system of commensal Staphylococcus simulans block the QS system in a methicillin-resistant strain Staphylococcus aureus, therefore protects host skin from damage.Citation43 The competing strategy via AIP is likely prevalent in GI microbiota, considering that the Agr system is evolutionarily ubiquitous across Firmicutes, including probiotics like Lactobacillus plantarum as well as human pathogens like Clostridium perfringens, Clostridium botulinum, Clostridium difficile, Listeria monocytogenes and Enterococcus faecalis ().Citation44 We envision that AIP signaling by commensal bacteria is another key factor influencing the structure and function of GI microbiota. Antibiotic treatment dramatically decreased the abundance of Firmicutes in mammalian gut,Citation37,Citation38 which means the intrinsic QS architectures and protective flora barrier would be destroyed. The interfered QS signaling can be recognized by pathogens and accelerate the colonization by them. That is one of the key reasons contributing to the severe risk of infection by C. difficile and S. typhimurium after antibiotic treatment.
AI-3 based signaling in the GI tract
AI-3 is another constantly discovered QS signal in GI tract. This chemical is synthesized by bacterial members in human GI flora, such as enterohemorrhagic E. coli. Citation36,Citation45 Although the pathway of AI-3 synthesis is still less defined, the threonine dehydrogenase (Tdh) and the aminoacyl-tRNA synthetases-related spontaneous cyclization are identified to be essential for AI-3 production.Citation45 AI-3 signal can be sensed by E. coli, S. typhimurium, and Enterococcus via a histidine kinase sensor QseC to regulate the expression of their virulence repertoire ().Citation36,Citation46 Including AI-3, QseC can sense host hormones like epinephrine (Epi) and norepinephrine (NE), which indicates a manipulating ability of host on GI microbiota via mammalian signal Epi/NE.Citation47 However, AI-3 and its analogs do not affect adrenergic signaling in human cells in vitro.Citation45
The effect of microbiota plays on host driven by QS
Evidence has shown that AI molecules such as AHL, AI-2 and AIP, which are frequently found in GI microbiota, can interact with intestinal mucosal cells and affect the inflammation and carcinogenesis process of host () (). On the other hand, perceiving AIs from the parasitic microbiota enables host to continuously monitor bacterial communications and bacterial infection dynamics. Clarifying the interkingdom interactions from the perspective of QS are essential for maintaining the homeostasis of the microbiota as well as the health of the host.
Figure 2. The effect of microbiota plays on host driven by QS.
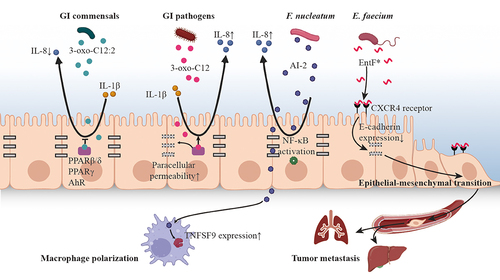
Table 1. QS mediated inter-species and inter-kingdom communications in GI tract (mammalian host).
AHL-elicited immunomodulatory activity
AHLs are fatty acid-based signaling molecules that are chemically similar to lipidic and steroid hormones in eukaryotes.Citation65 As one of the signaling molecules produced by the infamous P. aeruginosa, 3-oxo-dodecanoyl-l-homoserine lactone (3OC12-HSL) has been frequently detected from sputum, saliva and feces collected from human host,Citation26,Citation27,Citation29 and its role in inter-kingdom signaling has been fully studied. Injection of 3OC12-HSL into mouse skin increases the expression of cytokines IL-1α and IL-6, which in turn leads to subsequent inflammation of the host.Citation49 On the other hand, this molecule can suppress immune activity by inhibiting cytokine production and lymphocyte proliferation,Citation50,Citation51 disrupting NF-κB functions,Citation52 inducing immune cell deathCitation53 and decreasing antibody responses in mammalian cells.Citation66 A newly discovered AHL in human gut, 3-oxo-C12:2 is significantly more frequent in fecal samples from healthy subjects comparing with that in IBD patients. This molecule exerts an anti-inflammatory effect on Caco-2 cells by decreasing IL-1β induced IL-8 secretion. In contrast, 3OC12-HSL increases paracellular permeability but 3-oxo-C12:2 does not (), which indicates the protective role of 3-oxo-C12:2 plays on both gut microbiota and intestinal epithelial cells.
Receptors for AHL and related signaling pathways in mammalian host are still poorly understood. Nevertheless, the peroxisome proliferators-activated receptor PPARβ/δ and PPARγ are suspected to be putative mammalian 3OC12-HSL receptors, participating the expression of proinflammatory genes.Citation67 Another host receptor, aryl hydrocarbon receptor (AhR), can detect the type and quantity of quorum-sensing molecules of P. aeruginosa including AHL, quinolones, and phenazines (). Through the recognition of different signal molecules by AhR, the host judges the degree of bacterial infection, thereafter adjust the immunologic response.Citation68 This mechanism may explain why an opportunistic pathogen can be tolerated by the host at low density but become harmful once a threshold of tolerability has been exceeded. Host receptors having similar function to AhR may widely existed, therefore the immunologic defense would be specifically focused on harmful traits instead of harmless traits, allowing the host to mobilize the most appropriate defense mechanism according to the severity of threat.
Proinflammatory effects caused by AI-2
AI-2 is also involved in inflammatory process of host cells as a response to a challenge with bacterial pathogens. AI-2 is able to affect the proinflammatory responses of host to GI pathogens, like F. nucleatum,Citation69 P. gingivalis,Citation70 and H. pylori.Citation56 The AI-2 concentration in both colorectal tissue and stool of CRC patients was significantly higher compared with that in colorectal adenoma (AD) and normal colon mucosa (NC),Citation71 witch suggests an intense interaction between the secreted AI-2 and the immunity of colorectal cancer (CRC). An in vitro study reveals that the AI-2 signaling molecule elicits inflammatory by inducing IL-8 expression in HCT-8 colon cancer cells ().Citation54 Moreover, AI-2 extracted from F. nucleatum promotes macrophage polarization via stimulating the expression of TNFSF9 (), which was mainly derived from the tumor microenvironment.Citation71 This result suggests the AI-2 produced by gut microbiota may play a role in the carcinogenesis of CRC through immune cells of the gut.Citation71
Although the above-mentioned research has demonstrated that AI-2 can be produced by the GI microbiota and exists in the intestinal environment, it can also be sensed by intestinal epithelial cells and immune cells, triggering intracellular signal transduction pathways that influence immune responses. However, details regarding which receptors in host cells, particularly intestinal epithelial cells, can recognize AI-2 signal molecules remain uncertain.
AIP participate in promoting metastasis of cancer cells
The autoinducing peptides (AIPs) synthesized by human microbiota were found to influence mammalian cells (), such as promoting metastasis of cancer cellsCitation59,Citation60 and penetrating the blood-brain barrier.Citation59 Certain AIP, such as Phr0662 of Bacillus sp., EntF-metabolite of Enterococcus faecium, and EDF-derived peptides of E. coli, could initiate HCT-8/E11 colon cancer cell invasion, with Phr0662 also promoting angiogenesis.Citation57 Taking the QS peptide EntF* produced by E. faecium as an example, it is found to be naturally present in mice bloodstream. Its role in promoting colorectal cancer metastasis was also observed in vivo, with metastatic lesions found in both liver and lung tissues, using an orthotopic mice model. Further in vitro tests suggest that EntF* can be sensed by CXCR4 receptors, thereafter inhibits the expression of E-cadherin in the colorectal cells and consequently results in the epithelial-mesenchymal transition (). This is likely to be one of the main mechanisms that pathogens promote tumor metastasis.Citation60 In addition to CXCR4 receptors, the epidermal growth factor receptor (EGFR) overexpressed in many types of cancer cells are also potential receptors responsible for binding with AIP and activating intracellular signaling cascade that resulting in tumor metastasis.Citation57
At present, it is still in the early stage to explore the relationship among QS signaling, intestinal microbiota homeostasis and human health. It is imperative to clarify the characteristics of QS signaling (i.e., type and concentration of AIs) under different health conditions, which will provide us with new concept for disease prevention, diagnosis, and therapy by selective modulation on the gut microbiota.
The fight back against bacterial QS signaling by host
Host-mediated AHL degrading and modifying
To disrupt bacterial QS, many bacteria have developed the ability to interfere with or manipulate of QS pathways, which termed as quorum quenching (QQ). In multispecies microflora, lactonase, acylase and oxidoreductase are the most frequently investigated enzymes to inactivate AHLs by bacteria. Intriguingly, the eukaryotic cytoplasmic paraoxonases (PONs) can act as lactonases, being able to degrade AHL 3-oxo-C12 ().Citation72,Citation73 The PON family (PON1–3) was initially proved to subvert the QS signaling of P. aeruginosa and its biofilm formation in vitro.Citation63,Citation64 Later, Stoltz proved the degrading function on AHL by PON1 using a transgenic Drosophila melanogaster overexpressing the enzyme, which protect the host from P. aeruginosa lethality due to the lactonase activity of PON1 ().Citation74 Another modification mechanism was found in Hydra vulgaris, which can modify 3-oxo-C12 into the 3-OH-C12 counterparts, resulting in a phenotypic switch in their bacterial colonizers.Citation75 Given Hydra is far less advanced than mammals, we suspect the modification of bacterial signals may also exist in the more sophisticated human host. In this way, the host can control or modify the behaviors of its bacterial colonizers for its own benefit.
Figure 3. The fight back against bacterial QS signaling by host.
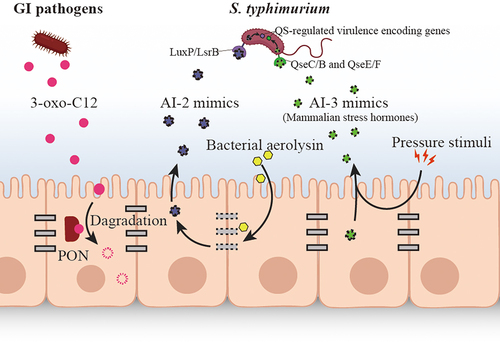
Synthesis of QS signal mimics by host cells
AI-2 mimics
Interkingdom communication between eukaryotic cells and bacteria can rely on the AI-2-based QS. Mammalian epithelial cells from colon tissues (Caco-2), lung tissues (A549), and cervical tissues (HeLa) can produce AI-2 mimics in response to bacteria.Citation61 These AI-2 mimics can be detected by traditional AI-2 receptors, LuxP/LsrB, and activate QS-controlled gene expression in the enteric pathogen S. typhimurium () ().Citation61 Considering that AI-2 is a universal nonspecific QS signal, synthesizing AI-2 mimics could be a strategy for host to maximally manipulate bacterial behaviors in a mixed population like GI microbiota.
AI-3 mimics
Gut hormones such as epinephrine (E) and norepinephrine (NE) that contribute to gut motility, potassium and chloride secretion, epithelial barrier function, and inflammation,Citation76,Citation77 can also be perceived by microbial QS receptors and interfere with bacterial behaviors. Hormones E and NE are reported to signal through the same pathway in bacteria with QS signal AI-3, a two-component systems QseC/B and QseE/F.Citation36,Citation46,Citation78–80 E and NE are reported to be the host-derived AI-3 mimics, which can affect the signal reception of AI-3 and activate the virulence of Escherichia coli O157:H7 and Salmonella () ().Citation45,Citation46 Another mammalian stress hormone dynorphin in mice intestinal mucosa was reported to activate QS signaling in P. aeruginosa, and therefore enhanced its virulence in vivo.Citation62 Although there is still limited number of in vivo studies about cross-talk between host and bacteria, we expect more in vivo evidence to explain the underlying mechanisms.
QS functions as allay or enemy to host?
The role of QS in the construction of microbial barrier
Although there are much more studies presenting the role QS plays in pathogenicity, evidence proving QS signaling in commensal flora is arising. The human intestine hosts trillions of microorganisms, which construct a complex microbial community playing multiple anti-infectious, anti-inflammatory, and immune modulating roles decisive for intestinal homeostasis.Citation81 In healthy human gut, the commensal flora has attached to the intestinal mucus, occupying all available spaces. They are suspected to recognize and cooperate with each other via QS systems, and then construct a protective barrier against enteropathogenic colonization. Recent study has shown that AI-2 produced by the commensal R.obeum inhibits virulence gene expression by Vibrio cholerae and restricts V. cholerae colonization in mice ().Citation39 In another study, the Firmicutes phylum previously eliminated by streptomycin was recovered by replenishing AI-2 in the mouse gut.Citation38 This demonstrates that AI-2 has great potential assisting commensal bacteria to maintain the homeostasis of gut microbiota, the balance of which is known to influence human health. AI-2 signaling has been identified in the probiotics E coli Nissle 1917, Bifidobacterium and Lactobacillus species, allowing adhesion and enrichment of them in intestines by regulating biofilm formation.Citation82–84 However, its role in maintaining homeostasis of commensal flora is still lacking evidence in vivo. In addition, other type QS signal derived from commensals (like 3-oxo-C12:2) in strengthening commensal intestinal bacteria cooperation to resist colonization by invaders, and keeping the dynamic balance of the microbiota also requires further exploration.
How QS accelerate dysbiosis of microbiota during infection
As previous mentioned, the dynamic balance and symbiotic cooperation in GI microbiota is protected by QS. However, when the intrinsic concordant QS network of commensal microbiota gets interfered or impaired due to alterations of host diet, physiology, and immunological processes, the resident pathobionts or external entero-pathogens are highly likely to multiply uncontrollably. In this unbalanced microbiome environment, enteropathogens, such as E. coli, Clostridia, Listeria, and Pseudomonas species, utilize their own QS systems to strengthen colonization and invasion of the intestinal mucus layer, thereby promoting bacterial virulence expression, host inflammation, systemic translocation, and ultimately leading to GI or systemic infections.Citation85–88
Colonization attraction
Some pathogens take advantages of host and microbiota-derived QS signals to selectively colonize in gut. Usually, epithelia cells of intestine directly interact with colonizing bacteria, protecting host from pathogen invading. But, when treated with stress signal, like the bacterial aerolysin, the epithelial tight junctions will be disrupted, and activate production of an AI-2 mimic, which is can activate QS to regulate mechanisms in enteric pathogens, such as S. typhimurium ().Citation61 The release of mammalian AI-2 mimic after epithelial tight-junction damage may call for the cell healing, such as the production of a polysaccharide called PSA, which is an ameliorative bacterially-produced molecule by intestinal commensals.Citation89 Besides that, the pathogenic residents that possessing bacterial AI-2 receptors, such as F. nucleaum, C. difficile, and E. coli, are also supposed to sense the AI-2 mimic,Citation61 thereby aggregate on the epithelial tight-junction damaging site and lead to an aggravating damage of intestinal barrier. Mammalian stress hormones, epinephrine (Epi), noradrenaline (NE), and dynorphin that induced by stress can affect the response of GI system, including the susceptibility of GI microbiota to pathogenic bacteria.Citation45,Citation46 As shown in an in vivo experiment, the dopamine β-hydroxylase knockout (Dbh−/−) disabled in producing Epi or NE, exhibits reduced susceptibility to S. typhimurium infection, which proved the attracting function of several host metabolites to enteric pathogens.Citation90 Similar attraction effect has been observed in P. aeruginosa by dynorphin in colonizing in mice intestinal mucosa.Citation47
Bacteria coaggregation and polymicrobial biofilm formation
Most of pathogens well characterized so far have QS signaling systems, that is, the reason why pathogens may precisely anchor to the impairing sites of the epithelial barrier based on signals released by cell damage. Especially when commensal bacterial consortium is obliterated with antibiotic treatment, pathogens can quickly take over the living space of intestinal tract, recruiting and aggregating with cooperative bacteria by QS, and ultimately construct a pathogen-dominated polymicrobial biofilm. In a notable study, the visualization of E. coli aggregates in murine intestinal contents has provided the first in vivo evidence of how chemotaxis toward AI-2 facilitates ecological niche segregation and stable co-existence of different E. coli strains.Citation91 This study sheds light on the important role of QS in shaping bacterial communities in a living host. Taking F. nucleatum as another example, it can induce biofilm growth of single and dual species and coaggregation between itself and the “red complex”, which suggests the QS signal AI-2 plays an important role in inter- and intraspecies interactions between periodontopathogens.Citation92 Whilst in the intestinal environment, F. nucleaum can aggregate with C. difficile and synergistically enhance the robust biofilm formation, however, whether QS involved in the process remains to be explored.Citation93.
Once a biofilm dominated by pathogens is formed, the infectious healing will be negatively affected. Biofilms provide their inhabitants with competitive advantages, like efficient nutrients exchanging and increased resistance to environmental stress.Citation94 Members in biofilm communicate and interact more frequently using QS due the proximal distance, thus drive collective behavior resulting in distinct physiologies from planktonic cells, like enhanced expression of virulent factors.Citation95 Meanwhile, the horizontal gene transfer is promoted in biofilm microbiota, which contributes to spreading of antibiotic-resistance genes.Citation96 In addition to enhancing information exchange and collaboration, the biofilm itself is a dense physical barrier rich in proteins and sugars that protects the bacteria inside from antibiotic, antimicrobial substances and host immune system, thereby exacerbating the infection.Citation97,Citation98 In addition, QS molecules secreted by bacteria are more likely to be retained in compact and hydrophobic biofilm, and then regulate host immune response by indirectly regulating the function of related microflora or directly acting on host cells.Citation95 It has accepted that the pathogen-driven polymicrobial biofilm appears to be a tipping point between a healthy and diseased state of gut mucosa. Biofilms have been frequently recognized in several conditions, such as gut wounds,Citation99,Citation100 IBD,Citation101,Citation102 and CRC,Citation103,Citation104 which are supposed to participate in the pathogenesis and disease manifestation.Citation105,Citation106
QS signaling molecules, including AHL, AI-2, and AIP are reported possessing immunomodulatory effect on host tissues (). As illustrated in the former section, 3OC12-HSL demonstrates immunomodulatory activities on T lymphocyte, macrophage and antibody responses in mammalian cells,Citation52,Citation66 while it can also suppress immune activity by inhibiting cytokine production and lymphocyte proliferation,Citation50,Citation51 disrupting NF-κB functions,Citation52 and inducing immune cell death.Citation53 AI-2 can affect the proinflammatory responses of host to GI pathogens, like F. nucleatum,Citation69 P. gingivalis,Citation70 and H. pylori.Citation56 AI-2 could induce IL-8 expression in HCT-8 colon cancer cells.Citation54 The autoinducing peptides (AIPs) synthesized by human microbiota were found to influence mammalian cells, promoting metastasis of cancer cellsCitation57,Citation60 and penetrate the blood-brain barrier.Citation57 Although the specific mechanism(s) through which AIs influence mammalian cells is unclear, modified immune responses by AIs unravel another circuit of how pathogens establish an infection.Citation107
Leveraging QS to manipulate the microbiota
Quorum sensing interference as an anti-infectious therapy
Given the association between pathogens and the role they play in pathogenic process, QSI strategy, for instance, using QS signaling molecule analogues or degradation enzymes, has been raised as a promising therapeutic strategy replacing antibiotic to migrate microbial infections and resistance from human host. Up to date, plenty of efficient QSIs derived from food and plant that target human pathogens have been discovered using model pathogens by in vitro and in vivo animal models (). For example, some natural organic acids, fatty acids, vitamin C, furanone, flavone and its derivations perform anti-QS activities due to their structural similarity to QS signaling molecules.Citation130,Citation131 These QSI agents derived from plant-based foods, once taken into human body, are hypothesized to participate in the homeostasis maintenance of GI microbial communities by inhibiting pathogens’ QS signaling to avoid invasion. This may be one of the key reasons why the risk of intestinal inflammation is generally lower with plant-based foods compared to meat-based foods.Citation132 However, to our knowledge, no direct evidence has been raised proving the validity of QSI strategies in protecting microbial barrier in human gut. In vivo test in murine infection models demonstrated the efficiency of QSI agent in protecting host skin barrier integrity by against S. aureus,Citation133 which shows us the great potential for QSI to be used in the treatment of infectious diseases in mammalian host. We envision that the development of food-based anti-infection strategies targeting pathogens’ QS systems is a promising adjuvant or alternative to antibiotic.
Table 2. Efficient QSIs derived from food and plant that target human pathogens by in vitro and in vivo animal models.
Engineering the QS systems of probiotics and symbiotic bacteria for disease diagnosis and treatment
In addition to the usage of QSI analogs to mitigate virulence from infectious pathogens, more studies try to design and engineer the QS pathway of probiotics and symbiotic bacteria for disease diagnosis and treatment. Mao et al.Citation127 and Holowko et al.Citation128 made the first attempt using engineered probiotic Lactococcus lactis and nonpathogenic E.coli to mediate cholera resistance in animal models. Holowko created a synthetic genetic CAI-1 sensing system with a sensor module and an inverter module. In the absence of quorum-sensing molecule CAI-1 that unique to the genus Vibrio, gene cassette, including CqsS, LuxU and LuxO are constitutively expressed and phosphorylated, thereafter, the phosphorylated LuxO activates Qrr4 promoter which expresses gRNA to further joins constitutively expressed dCas9 to repress GFP expression. While when V. cholerae reaches a high-density state, CAI-1 dephosphorylates CqsS will ultimately dephosphorylates LuxO. Dephosphorylated LuxO does not activate the Qrr4 promoter preventing gRNA expression therefore GFP can be expressed efficiently without gRNA inhibition. In the latter study, Mao advanced the QS based diagnosis in vitro close to the stage for dietary interventions with natural and engineered probiotics as an alternative strategy to combat the spread of cholera in vulnerable populations using a probiotic L. lactis (). The engineered L. lactis can detect V. cholerae and trigger expression of its enzymatic reporter that is readily detected in situ fecal samples. Such living diagnostics based on bioengineering of QS represents a promising approach to achieve near real-time surveillance of multiple pathological conditions.Citation127 Recently, there have been significant advances in the engineering of QS devices for dynamically controlling bacterial populations and developing potential clinical therapies. Wu and Dang have conducted comprehensive reviews of these recent achievements.Citation134,Citation135
Figure 4. Leveraging QS to manipulate the microbiota.
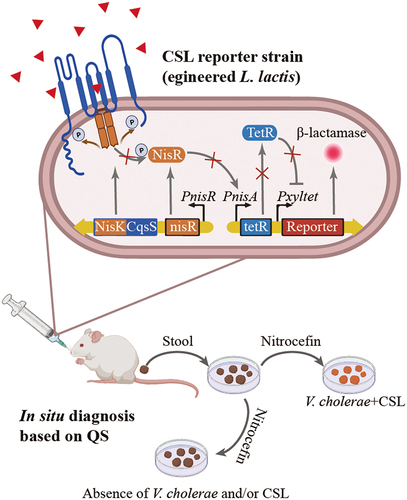
Complementary to the living therapies based on bacterial interactions, Sedlmayer and his colleagues designed a synthetic mammalian cell (human HEK-293 cell) based microbial-control device that detects microbial chemotactic formyl peptides through a formyl peptide sensor (FPS) and responds by releasing AI-2.Citation129 In details, N-formyl peptides activate the sensor FPR1, which was rewired to the constitutively expressed promiscuous human G protein subunit Gα16 and relays to calcium-triggered expression of LuxS. In the assistance with MTAN, LuxS generates AI-2 which can be further released outside the mammalian cell and sensed by AI-2 responsive pathogens, such as V. harveyi and Candida albicans. The biosensor-based circuits in implanted human designer cells programmed to interface with infection-related molecules and to mitigate microbial colonization may pave the way for next-generation antimicrobials in the post-antibiotic era.Citation129,Citation136
Preliminary attempt to shape the assembly of human microbiota by manipulating QS communication network
In recent years, more research tries to exploit novel strategies to promote gastrointestinal homeostasis from the aspect of microbiota-modulating. Because of the large diversity of bacteria in the gut, multiple QS pathways have been mined out by in silico search.Citation137 These QS systems are suspected to play vital roles regulating microbe-microbe interactions to maintain the harmonious of human gut microbiome.Citation6,Citation138 In the previous study, Thompson et al. used an engineered E. coli to restore the level of AI-2 in antibiotic-treated mice, and ultimately relanced the ratio of Bacteroidetes to Firmicutes phyla in the murine gut bacterial community,Citation38 which demonstrated the AI-2 availability in shaping the antibiotic-ruined GI microbiota. Nevertheless, very little is currently known with respect to the role of QS in complex microbial communities and their use in the detection, prevention, and treatment of acute infections remains underexplored. In our recent work (unpublished), we developed and in vitro multispecies (more than 300 species) oral biofilm assembly model, based on which attempting to revealing the shaping power of QS network in the assembly process by performing time serial metagenomic analysis and QS-interfering experiment. We constituted a full-scale longitudinal QS network via the QS hubs of Streptococcus, the Veillonella-Megasphaera group, and the Prevotella-Fusobacteria group for information delivery, and experimentally validated the directional shaping power of the longitudinal QS network in the microbiota. This work attempts to illustrate the role of longitudinal QS network in shaping human microbiota assembly, which could lead to more in targeted interventions for manipulating human microbiota through QS network intervention.
Challenges in applying QS based interventions for microbiota manipulation
Before the widespread adoption of QS therapies in the treatment of individuals, there are still several overarching challenges that need to be addressed. The first significant obstacle is assessing how QS functions in host environments that involve fluid flow and complex geometries. Flow is present in all living systems, such as, intestinal digestion and urination. Flow environment drives heterogeneously distributed QS signals in specific locations, therefore leading to spatial fate decisions which allows genetically identical bacterial to undertake distinct biological functions.Citation8,Citation15,Citation95 Additionally, surface topography is another realistic factor influencing QS dynamics, which is constantly found to drive non-uniform bacterial behavior conjunction with flow. Kim et al. applied microfluidic chambers with cervices to mimic intestinal crypts, revealing the heterogeneous QS activities in various localized bacteria.Citation95 Outside of the crevices, the QS activity were repressed due to constant flow, while bacteria that colonized inside the crevices exhibited heightened QS activity in response to signal accumulation. It is crucial to investigate how QS is deployed under realistic circumstances, such as clinical and industrial settings.
QS as a mechanism that regulate communication and gene expression in bacteria, plays a crucial role in the mutual antagonism between probiotics and pathogens in the gut microbiota. When probiotics detect the presence of pathogens in their environment, they can release signaling molecules to inhibit the QS system of the pathogens, suppressing their growth and the production of virulence factors. This competition slows down or prevents the pathogenicity of the pathogens and contribute to maintain a healthy balance in the gut. However, some pathogens can also utilize the QS system to counteract probiotics. In response to the presence of competitors like probiotics, pathogens can adjust gene expression, produce antimicrobial substances, or generate toxic metabolites to inhibit the growth and activity of probiotics. This antagonistic behavior helps pathogens establish a competitive advantage in the gut and enhances their ability to infect the host. Under normal circumstances, there is a dynamic equilibrium between probiotics and pathogens. This balance helps to maintain a stable gut environment, preventing the excessive proliferation of pathogens and protecting the host from disease invasion. However, deciphering how probiotics and pathogens decode the information in different combinations of signaling molecules produced by cooperating and competing bacteria remains a significant challenge.
While bacterial infections are well-known, it is important to note that fungal infections of the gastrointestinal tract can also occur, especially in individuals with compromised immune systems or other underlying health conditions. One of the most common fungal species involved in gastrointestinal infections is Candida, specifically Candida albicans. C. albicans utilizes QS based on farnesol to mediate metabolisms, such as morphogenesis, biofilm development, mating, drug efflux, and apoptosis.Citation139–143 QS in C. albicans also plays a role in inter-kingdom crosstalk, such as repressing the production of the quinolone QS signal in P. aeruginosa Citation144 and triggering the release of neutrophil extracellular traps.Citation145 These findings indicates that QS communication is involved in a broad range of interactions within microbiota and between microbes and the host. It is crucial for future studies to explore QS mechanisms regarding more realistic contexts, including the presence of flow and the inclusion of fungi, viruses and eukaryotic hosts, to further enhance our understanding and application of the QS communication mechanism.
Concluding remarks and future considerations
The misuse and overuse of antibiotics have led to the emergence of multidrug-resistant bacterial strains, posing a global health threat and limiting the effectiveness of conventional antibiotic treatments. As a result, researchers have been actively exploring new strategies to combat bacterial infections. Quorum sensing (QS) has emerged as an attractive target due to its regulatory effects on bacterial virulence. In recent decades, numerous studies have summarized and demonstrated the QS-based approaches alleviating and preventing infections.Citation134,Citation135,Citation146,Citation147 The growing number of studies that focus on QS-based applications shows a bright future for this field. However, our understanding of how to leverage QS to precisely manipulate microbiota is still in the early stage, and there are still many significant challenges that need to be addressed. First, the complex and dynamically changing environment can significantly impact the QS signaling cascade, leading to a heterogeneity in microbiota using QS regulatory pathway. Therefore, it is crucial to investigate how QS is deployed in realistic scenarios, such as clinical and industrial settings, which involve fluid flow and complex geometries. Second, understanding how probiotics and pathogens decode the information contained in blends of AIs produced by cooperating bacteria versus competing bacteria is essential. This knowledge is vital for selecting appropriate QS based interference strategy to target specific pathogens without interfering with the QS network inherent in the symbiotic microbiota. This is crucial in order to avoid causing undesirable flora dysbiosis and diseases. Third, the QS regulatory mechanisms not only facilitate communication among bacterial populations, but also play a role in the cross-talk between bacteria, fungi, and the mammalian host. Therefore, further investigations are urgently needed to decipher the QS molecules and pathways involved in inter-kingdom communication. Finally, to ensure the efficiency and safety of QS based applications, it is necessary to conduct more in vivo test rather than relying solely on in vitro experiments. To sum up, future studies exploring the QS communication network in complex, natural, multispecies consortia will enhance our understanding of the mutualistic symbiotic relationship between the microbiota and the host. This knowledge will provide opportunities for the development of more precise therapeutics to combat bacterial infections and enhance host immune defenses.
Consent for publication
All authors consent to the publication of this manuscript and declare no conflicts of interest.
Disclosure statement
No potential conflict of interest was reported by the author(s).
Data availability statement
Data sharing is not applicable to this article as no new data were created or analyzed in the review.
Additional information
Funding
References
- Rooks MG, Veiga P, Wardwell-Scott LH, Tickle T, Segata N, Michaud M, Gallini CA, Beal C, van Hylckama-Vlieg JE, Ballal SA, et al. Gut microbiome composition and function in experimental colitis during active disease and treatment-induced remission. ISME J. 2014;8(7):1403–22. doi:10.1038/ismej.2014.3.
- Gordon JI, Klaenhammer TR. A rendezvous with our microbes. PNAS. 2011;108:4513–4515. doi:10.1073/pnas.1101958108.
- Kumar V, Rawat J. Quorum sensing: the microbial linguistic. In: Recent Advancements in Microbial Diversity. Academic Press; 2020 p. 233–250. doi:10.1016/B978-0-12-821265-3.00010-4.
- Uhlig F, Grundy L, Garcia-Caraballo S, Brierley SM, Foster SJ, Grundy D. Identification of a quorum sensing-dependent communication pathway mediating bacteria-gut-brain cross talk. iScience. 2020;23:101695. doi:10.1016/j.isci.2020.101695.
- Wu L, Luo Y. Bacterial quorum-sensing systems and their role in intestinal bacteria-host crosstalk. Front Microbiol. 2021;12:611413. doi:10.3389/fmicb.2021.611413.
- Wu S, Xu C, Liu J, Liu C, Qiao J. Vertical and horizontal quorum-sensing-based multicellular communications. Trends Microbiol. 2021;29(12):1130–1142. doi:10.1016/j.tim.2021.04.006.
- Cvitkovitch DG, Li YH, Ellen RP. Quorum sensing and biofilm formation in streptococcal infections. J Clin Invest. 2003;112(11):1626–1632. doi:10.1172/JCI200320430.
- Whiteley M, Diggle SP, Greenberg EP. Progress in and promise of bacterial quorum sensing research. Nature. 2017;551(7680):313–320. doi:10.1038/nature24624.
- Frias J, Olle E, Alsina M, O’Brien AD. Periodontal pathogens produce quorum sensing signal molecules. Infect Immun. 2001;69(5):3431–3434. doi:10.1128/IAI.69.5.3431-3434.2001.
- Rader BA, Campagna SR, Semmelhack MF, Bassler BL, Guillemin K. The quorum-sensing molecule autoinducer 2 regulates motility and flagellar morphogenesis in Helicobacter pylori. J Bacteriol. 2007;189(17):6109–6117. doi:10.1128/JB.00246-07.
- Walters M, Sperandio V. Quorum sensing in Escherichia coli and Salmonella. Int J Med Microbiol. 2006;296(2–3):125–131. doi:10.1016/j.ijmm.2006.01.041.
- Bearson BL, Bearson SM. The role of the QseC quorum-sensing sensor kinase in colonization and norepinephrine-enhanced motility of Salmonella enterica serovar typhimurium. Microb Pathog. 2008;44(4):271–278. doi:10.1016/j.micpath.2007.10.001.
- Sturme MH, Francke C, Siezen RJ, de Vos WM, Kleerebezem M. Making sense of quorum sensing in lactobacilli: a special focus on Lactobacillus plantarum WCFS1. Microbiol (Russ Acad Sci). 2007;153:3939–3947. doi:10.1099/mic.0.2007/012831-0.
- Miller MB, Bassler BL. Quorum sensing in bacteria. Annu Rev Microbiol. 2001;55(1):165–199. doi:10.1146/annurev.micro.55.1.165.
- Mukherjee S, Bassler BL. Bacterial quorum sensing in complex and dynamically changing environments. Nat Rev Microbiol. 2019;17(6):371–382. doi:10.1038/s41579-019-0186-5.
- Ng W-L, Bassler BL. Bacterial quorum-sensing network architectures. Annu Rev Genet. 2009;43(1):197. doi:10.1146/annurev-genet-102108-134304.
- Waters CM, Bassler BL. Quorum sensing: cell-to-cell communication in bacteria. Annu Rev Cell Dev Biol. 2005;21(1):319–346. doi:10.1146/annurev.cellbio.21.012704.131001.
- Decho AW, Frey RL, Ferry JL. Chemical challenges to bacterial AHL signaling in the environment. Chem Rev. 2011;111(1):86–99. doi:10.1021/cr100311q.
- Nealson KH, Platt T, Hastings JW. Cellular control of the synthesis and activity of the bacterial luminescent system. J Bacteriol. 1970;104(1):313–322. doi:10.1128/jb.104.1.313-322.1970.
- Brint JM, Ohman DE. Synthesis of multiple exoproducts in Pseudomonas aeruginosa is under the control of RhlR-RhlI, another set of regulators in strain PAO1 with homology to the autoinducer-responsive LuxR-LuxI family. J Bacteriol. 1995;177(24):7155–7163. doi:10.1128/jb.177.24.7155-7163.1995.
- Atkinson S, Chang CY, Sockett RE, Camara M, Williams P. Quorum sensing in Yersinia enterocolitica controls swimming and swarming motility. J Bacteriol. 2006;188(4):1451–1461. doi:10.1128/JB.188.4.1451-1461.2006.
- Lewenza S, Conway B, Greenberg EP, Sokol PA. Quorum sensing in Burkholderia cepacia: identification of the LuxRI homologs CepRI. J Bacteriol. 1999;181(3):748–756. doi:10.1128/JB.181.3.748-756.1999.
- Dyszel JL, Smith JN, Lucas DE, Soares JA, Swearingen MC, Vross MA, Young GM, Ahmer BM. Salmonella enterica serovar typhimurium can detect acyl homoserine lactone production by Yersinia enterocolitica in mice. J Bacteriol Parasitol. 2010;192(1):29–37. doi:10.1128/JB.01139-09.
- Papenfort K, Bassler BL. Quorum sensing signal–response systems in Gram-negative bacteria. Nat Rev Microbiol. 2016;14(9):576. doi:10.1038/nrmicro.2016.89.
- Huber B, Riedel K, Hentzer M, Heydorn A, Gotschlich A, Givskov M, Molin S, Eberl L. The cep quorum-sensing system of Burkholderia cepacia H111 controls biofilm formation and swarming motility. Microbiology. 2001;147(9):2517–2528. doi:10.1099/00221287-147-9-2517.
- Erickson DL, Endersby R, Kirkham A, Stuber K, Vollman DD, Rabin HR, Mitchell I, Storey DG. Pseudomonas aeruginosa quorum-sensing systems may control virulence factor expression in the lungs of patients with cystic fibrosis. Infect Immun. 2002;70(4):1783–1790. doi:10.1128/IAI.70.4.1783-1790.2002.
- Kumari A, Pasini P, Daunert S. Detection of bacterial quorum sensing N-acyl homoserine lactones in clinical samples. Anal Bioanal Chem. 2008;391(5):1619–1627. doi:10.1007/s00216-008-2002-3.
- Buch PJ, Chai Y, Goluch ED. Bacterial chatter in chronic wound infections. Wound Repair Regen. 2021;29(1):106–116. doi:10.1111/wrr.12867.
- Kumari A, Pasini P, Deo SK, Flomenhoft D, Shashidhar H, Daunert S. Biosensing systems for the detection of bacterial quorum signaling molecules. Anal Chem. 2006;78(22):7603–7609. doi:10.1021/ac061421n.
- Landman C, Grill JP, Mallet JM, Marteau P, Humbert L, Le Balc’h E, Maubert MA, Perez K, Chaara W, Brot L, et al. Inter-kingdom effect on epithelial cells of the N-acyl homoserine lactone 3-oxo-C12: 2, a major quorum-sensing molecule from gut microbiota. PLoS One. 2018;13(8):e0202587. doi:10.1371/journal.pone.0202587.
- Hudaiberdiev S, Choudhary KS, Vera Alvarez R, Gelencsér Z, Ligeti B, Lamba D, Pongor S. Census of solo LuxR genes in prokaryotic genomes. Front Cell Infect Microbiol. 2015;5:20. doi:10.3389/fcimb.2015.00020.
- Grellier N, Suzuki MT, Brot L, Rodrigues AMS, Humbert L, Escoubeyrou K, Rainteau D, Grill JP, Lami R, Seksik P. Impact of IBD-Associated dysbiosis on bacterial quorum sensing mediated by acyl-homoserine lactone in human gut microbiota. Int J Mol Sci. 2022;23(23):23. doi:10.3390/ijms232315404.
- Hughes DT, Terekhova DA, Liou L, Hovde CJ, Sahl JW, Patankar AV, Gonzalez JE, Edrington TS, Rasko DA, Sperandio V. Chemical sensing in mammalian host–bacterial commensal associations. PNAS. 2010;107(21):9831–9836. doi:10.1073/pnas.1002551107.
- Yan Y, Nguyen LH, Franzosa EA, Huttenhower C. Strain-level epidemiology of microbial communities and the human microbiome. Genome Med. 2020;12(1):71. doi:10.1186/s13073-020-00765-y.
- Pereira CS, Thompson JA, Xavier KB. AI-2-mediated signalling in bacteria. FEMS Microbiol Rev. 2013;37(2):156–181. doi:10.1111/j.1574-6976.2012.00345.x.
- Sperandio V, Torres AG, Jarvis B, Nataro JP, Kaper JB. Bacteria–host communication: the language of hormones. PNAS. 2003;100(15):8951–8956. doi:10.1073/pnas.1537100100.
- Sekirov I, Tam NM, Jogova M, Robertson ML, Li Y, Lupp C, Finlay BB. Antibiotic-induced perturbations of the intestinal microbiota alter host susceptibility to enteric infection. Infect Immun. 2008;76(10):4726–4736. doi:10.1128/IAI.00319-08.
- Thompson JA, Oliveira RA, Djukovic A, Ubeda C, Xavier KB. Manipulation of the quorum sensing signal AI-2 affects the antibiotic-treated gut microbiota. Cell Rep. 2015;10(11):1861–1871. doi:10.1016/j.celrep.2015.02.049.
- Hsiao A, Ahmed AM, Subramanian S, Griffin NW, Drewry LL, Petri WA Jr., Haque R, Ahmed T, Gordon JI. Members of the human gut microbiota involved in recovery from Vibrio cholerae infection. Nature. 2014;515(7527):423–426. doi:10.1038/nature13738.
- Zschiedrich CP, Keidel V, Szurmant H. Molecular mechanisms of two-component signal transduction. J Mol Biol. 2016;428(19):3752–3775. doi:10.1016/j.jmb.2016.08.003.
- Lyon GJ, Wright JS, Muir TW, Novick RP. Key determinants of receptor activation in the agr autoinducing peptides of Staphylococcus aureus. Biochemistry. 2002;41(31):10095–10104. doi:10.1021/bi026049u.
- Geisinger E, Muir TW, Novick RP. Agr receptor mutants reveal distinct modes of inhibition by staphylococcal autoinducing peptides. PNAS. 2009;106(4):1216–1221. doi:10.1073/pnas.0807760106.
- Brown MM, Kwiecinski JM, Cruz LM, Shahbandi A, Todd DA, Cech NB, Horswill AR. Novel peptide from commensal Staphylococcus simulans blocks methicillin-resistant Staphylococcus aureus quorum sensing and protects host skin from damage. Antimicrob Agents Chemother. 2020;64(6):64. doi:10.1128/AAC.00172-20.
- Atkinson S, Williams P. Quorum sensing and social networking in the microbial world. J R Soc Interface. 2009;6(40):959–978. doi:10.1098/rsif.2009.0203.
- Kim CS, Gatsios A, Cuesta S, Lam YC, Wei Z, Chen H, Russell RM, Shine EE, Wang R, Wyche TP. Characterization of autoinducer-3 structure and biosynthesis in E. coli. ACS Cent Sci. 2020;6(2):197–206. doi:10.1021/acscentsci.9b01076.
- Clarke MB, Hughes DT, Zhu C, Boedeker EC, Sperandio V. The QseC sensor kinase: a bacterial adrenergic receptor. PNAS. 2006;103(27):10420–10425. doi:10.1073/pnas.0604343103.
- Lustri BC, Sperandio V, Moreira CG, Andrews-Polymenis HL. Bacterial chat: intestinal metabolites and signals in host-microbiota-pathogen interactions. Infect Immun. 2017;85(12). doi:10.1128/IAI.00476-17.
- Nguyen YN, Sheng H, Dakarapu R, Falck JR, Hovde CJ, Sperandio VJI, Bäumler AJ. The acyl-homoserine lactone synthase YenI from Yersinia enterocolitica modulates virulence gene expression in enterohemorrhagic Escherichia coli O157: H7. Infect Immun. 2013;81(81):4192–4199. doi:10.1128/IAI.00889-13.
- Smith RS, Harris SG, Phipps R, Iglewski B. The Pseudomonas aeruginosa quorum-sensing molecule N-(3-oxododecanoyl) homoserine lactone contributes to virulence and induces inflammation in vivo. J Bacteriol. 2002;184(4):1132–1139. doi:10.1128/jb.184.4.1132-1139.2002.
- Ritchie AJ, Yam AO, Tanabe KM, Rice SA, Cooley MA. Modification of in vivo and in vitro T- and B-Cell-mediated immune responses by the Pseudomonas aeruginosa quorum-sensing molecule N -(3-oxododecanoyl)- l -homoserine lactone. Infect Immun. 2003;71(8):4421–4431. doi:10.1128/IAI.71.8.4421-4431.2003.
- Chhabra SR, Harty C, Hooi DS, Daykin M, Williams P, Telford G, Pritchard DI, Bycroft BW. Synthetic analogues of the bacterial signal (quorum sensing) molecule N-(3-oxododecanoyl)-L-homoserine lactone as immune modulators. J Med Chem. 2003;46(1):97–104. doi:10.1021/jm020909n.
- Kravchenko Kvv, GF MJ, Scott DA, Katz AZ, Grauer DC, Lehmann M, Meijler MM, Janda KD, Ulevitch R, Ulevitch RJ. Modulation of gene expression via disruption of NF-κB signaling by a bacterial small molecule. Sci. 2008;321(5886):259–263. doi:10.1126/science.1156499.
- Song D, Meng J, Cheng J, Fan Z, Chen P, Ruan H, Tu Z, Kang N, Li N, Xu Y. Pseudomonas aeruginosa quorum-sensing metabolite induces host immune cell death through cell surface lipid domain dissolution. Nature Microbiol. 2019;4(1):97–111. doi:10.1038/s41564-018-0290-8.
- Zargar A, Quan DN, Carter KK, Guo M, Sintim HO, Payne GF, Bentley WE, Rubin EJ. Bacterial secretions of nonpathogenic Escherichia coli elicit inflammatory pathways: a closer investigation of interkingdom signaling. MBio. 2015;6(2):e00025–15. doi:10.1128/mBio.00025-15.
- Wu J, Li K, Peng W, Li H, Li Q, Wang X, Peng Y, Tang X, Fu X. Autoinducer-2 of Fusobacterium nucleatum promotes macrophage M1 polarization via TNFSF9/IL-1β signaling. Int Immunopharmacol. 2019;74:105724–. doi:10.1016/j.intimp.2019.105724.
- Wen Y, Huang H, Tang T, Yang H, Wang X, Huang X, Gong Y, Zhang X, She F. AI-2 represses CagA expression and bacterial adhesion, attenuating the Helicobacter pylori -induced inflammatory response of gastric epithelial cells. Helicobacter. 2021;26(2):e12778. doi:10.1111/hel.12778.
- Wynendaele E, Verbeke F, D’Hondt M, Hendrix A, Van De Wiele C, Burvenich C, Peremans K, De Wever O, Bracke M, De Spiegeleer B. Crosstalk between the microbiome and cancer cells by quorum sensing peptides. Peptides. 2015;64:40–48. doi:10.1016/j.peptides.2014.12.009.
- Debunne N, De Spiegeleer A, Depuydt D, Janssens Y, Descamps A, Wynendaele E, De Spiegeleer B. Influence of blood collection mthods and long-term plasma storage on quorum-sensing peptide stability. ACS Omega. 2020;5(26):16120–16127. doi:10.1021/acsomega.0c01723.
- Wynendaele E, Verbeke F, Stalmans S, Gevaert B, Janssens Y, Van De Wiele C, Peremans K, Burvenich C, De Spiegeleer B, Tharakan B. Quorum sensing peptides selectively penetrate the blood-brain barrier. PLoS One. 2015;10(11):e0142071. doi:10.1371/journal.pone.0142071.
- Debunne N, Wynendaele E, Janssens Y, De Spiegeleer A, Verbeke F, Tack L, Van Welden S, Goossens E, Knappe D, Hoffmann R, et al. The quorum sensing peptide Entf* promotes colorectal cancer metastasis in mice: a new factor in the microbiom-host interaction. BMC Biol. 2020;20(1):1–16. doi:10.1186/s12915-022-01317-z.
- Ismail AS, Valastyan JS, Bassler BL. A host-produced autoinducer-2 mimic activates bacterial quorum sensing. Cell Host & Microbe. 2016;19(4):470–480. doi:10.1016/j.chom.2016.02.020.
- Zaborina O, Lepine F, Xiao G, Valuckaite V, Chen Y, Li T, Ciancio M, Zaborin A, Petroff E, Turner JR, et al. Dynorphin activates quorum sensing quinolone signaling in Pseudomonas aeruginosa. PLoS Path. 2007;3(3):e35. doi:10.1371/journal.ppat.0030035.
- Khersonsky O, Tawfik DS. Structure−reactivity studies of serum paraoxonase PON1 suggest that its native activity is lactonase. Biochemistry. 2005;44(16):6371–6382. doi:10.1021/bi047440d.
- Draganov DI, Teiber JF, Speelman A, Osawa Y, Sunahara R, La Du BN. Human paraoxonases (PON1, PON2, and PON3) are lactonases with overlapping and distinct substrate specificities. J Lipid Res. 2005;46(6):1239–1247. doi:10.1194/jlr.M400511-JLR200.
- Hughes DT, Sperandio V. Inter-kingdom signalling: communication between bacteria and their hosts. Nat Rev Microbiol. 2008;6(2):111–120. doi:10.1038/nrmicro1836.
- Skindersoe ME, Zeuthen LH, Brix S, Fink LN, Lazenby J, Whittall C, Williams P, Diggle SP, Froekiaer H, Cooley M. Pseudomonas aeruginosa quorum-sensing signal molecules interfere with dendritic cell-induced T-cell proliferation. FEMS Immunol Med Microbiol. 2009;55(3):335–345. doi:10.1111/j.1574-695X.2008.00533.x.
- Jahoor A, Patel R, Bryan A, Do C, Krier J, Watters C, Wahli W, Li G, Williams SC, Rumbaugh KP. Peroxisome proliferator-activated receptors mediate host cell proinflammatory responses to Pseudomonas aeruginosa autoinducer. J Bacteriol. 2008;190(13):4408–4415. doi:10.1128/JB.01444-07.
- Moura-Alves P, Puyskens A, Stinn A, Klemm M, Guhlich-Bornhof U, Dorhoi A, Furkert J, Kreuchwig A, Protze J, Lozza L, et al. Host monitoring of quorum sensing during Pseudomonas aeruginosa infection. Science. 2019;366(6472). doi:10.1126/science.aaw1629.
- Wu J, Wang Y, Jiang Z. Immune induction identified by TMT proteomics analysis in Fusobacterium nucleatum autoinducer-2 treated macrophages. Expert Rev Proteomics. 2020;17(2):175–185. doi:10.1080/14789450.2020.1738223.
- Scheres N, Lamont RJ, Crielaard W, Krom BP. LuxS signaling in Porphyromonas gingivalis-host interactions. Anaerobe. 2015;35:3–9. doi:10.1016/j.anaerobe.2014.11.011.
- Li Q, Peng W, Wu J, Wang X, Ren Y, Li H, Peng Y, Tang X, Fu X. Autoinducer-2 of gut microbiota, a potential novel marker for human colorectal cancer, is associated with the activation of TNFSF9 signaling in macrophages. Oncoimmunology. 2019;8(10):e1626192. doi:10.1080/2162402X.2019.1626192.
- Yang F, Wang L-H, Wang J, Dong Y-H, Hu JY, Zhang L-H. Quorum quenching enzyme activity is widely conserved in the sera of mammalian species. FEBS Lett. 2005;579(17):3713–3717. doi:10.1016/j.febslet.2005.05.060.
- Chun CK, Ozer EA, Welsh MJ, Zabner J, Greenberg EP. Inactivation of a Pseudomonas aeruginosa quorum-sensing signal by human airway epithelia. PNAS. 2004;101(10):3587–3590. doi:10.1073/pnas.0308750101.
- Stoltz DA, Ozer EA, Taft PJ, Barry M, Liu L, Kiss PJ, Moninger TO, Parsek MR, Zabner J. Drosophila are protected from Pseudomonas aeruginosa lethality by transgenic expression of paraoxonase-1. J Clin Invest. 2008;118(9):3123–3131. doi:10.1172/JCI35147.
- Pietschke C, Treitz C, Foret S, Schultze A, Kunzel S, Tholey A, Bosch TCG, Fraune S. Host modification of a bacterial quorum-sensing signal induces a phenotypic switch in bacterial symbionts. PNAS. 2017;114(40):E8488–E97. doi:10.1073/pnas.1706879114.
- Eisenhofer G, Åneman A, Friberg P, Hooper D, Fåndriks L, Lonroth H, Hunyady B, Mezey E. Substantial production of dopamine in the human gastrointestinal tract. J Clin Endocrinol Metab. 1997;82(11):3864–3871. doi:10.1210/jcem.82.11.4339.
- Asano Y, Hiramoto T, Nishino R, Aiba Y, Kimura T, Yoshihara K, Koga Y, Sudo N. Critical role of gut microbiota in the production of biologically active, free catecholamines in the gut lumen of mice. Am J Physiol-Gastr L. 2012;303(11):G1288–G95. doi:10.1152/ajpgi.00341.2012.
- Clarke MB, Sperandio V. Transcriptional regulation of flhDC by QseBC and σ 28 (FliA) in enterohaemorrhagic Escherichia coli. Mol Microbiol. 2005;57(6):1734–1749. doi:10.1111/j.1365-2958.2005.04792.x.
- Hughes DT, Clarke MB, Yamamoto K, Rasko DA, Sperandio V, Stebbins CE. The QseC adrenergic signaling cascade in enterohemorrhagic E. coli (EHEC). PLoS Path. 2009;5:e1000553. doi:10.1371/journal.ppat.1000553.
- Reading NC, Rasko DA, Torres AG, Sperandio V. The two-component system QseEF and the membrane protein QseG link adrenergic and stress sensing to bacterial pathogenesis. PNAS. 2009;106(14):5889–5894. doi:10.1073/pnas.0811409106.
- Lin L, Zhang J. Role of intestinal microbiota and metabolites on gut homeostasis and human diseases. BMC Immunol. 2017;18(1):2. doi:10.1186/s12865-016-0187-3.
- Lebeer S, Verhoeven TL, Perea Vélez M, Vanderleyden J, De Keersmaecker SC. Impact of environmental and genetic factors on biofilm formation by the probiotic strain Lactobacillus rhamnosus GG. Appl Environ Microbiol. 2007;73(21):6768–6775. doi:10.1128/AEM.01393-07.
- Jacobi CA, Grundler S, Hsieh C-J, Frick JS, Adam P, Lamprecht G, Autenrieth IB, Gregor M, Malfertheiner P. Quorum sensing in the probiotic bacterium Escherichia coli Nissle 1917 (Mutaflor)–evidence that furanosyl borate diester (AI-2) is influencing the cytokine expression in the DSS colitis mouse model. Gut Pathog. 2012;4(1):1–10. doi:10.1186/1757-4749-4-8.
- Sun Z, He X, Brancaccio VF, Yuan J, Riedel CU, Kaufmann GF. Bifidobacteria exhibit LuxS-dependent autoinducer 2 activity and biofilm formation. PLoS One. 2014;9(2):e88260. doi:10.1371/journal.pone.0088260.
- Iacob S, Iacob DG, Luminos LM. Intestinal microbiota as a host defense mechanism to infectious threats. Front Microbiol. 2018;9:3328. doi:10.3389/fmicb.2018.03328.
- Chen J, Ma M, Uzal FA, McClane BA. Host cell-induced signaling causes Clostridium perfringens to upregulate production of toxins important for intestinal infections. Gut Microbes. 2014;5(1):96–107. doi:10.4161/gmic.26419.
- Pinheiro J, Lisboa J, Pombinho R, Carvalho F, Carreaux A, Brito C, Pöntinen A, Korkeala H, dos Santos NMS, Morais-Cabral JH, et al. MouR controls the expression of the Listeria monocytogenes Agr system and mediates virulence. Nucleic Acids Res. 2018;46:9338–9352. doi:10.1093/nar/gky624.
- Asfour HZ. Anti-quorum sensing natural compounds. J Microsc Ultrastruct. 2018;6(1):1. doi:10.4103/JMAU.JMAU_10_18.
- Shen Yue. California Institute of Technology. Interkingdom communication of a bacterial mutualist and its mammalian host. 2012. doi:10.7907/56YV-8J33.
- Moreira CG, Weinshenker D, Sperandio V. QseC mediates Salmonella enterica serovar typhimurium virulence in vitro and in vivo. Infect Immun. 2010;78:914–926. doi:10.1128/IAI.01038-09.
- Laganenka L, Lee JW, Malfertheiner L, Dieterich CL, Fuchs L, Piel J, von Mering C, Sourjik V, Hardt WD. Chemotaxis and autoinducer-2 signalling mediate colonization and contribute to co-existence of Escherichia coli strains in the murine gut. Nature Microbiol. 2023;8(2):204–217. doi:10.1038/s41564-022-01286-7.
- Jang Y-J, Choi Y-J, Lee S-H, Jun H-K, Choi B-K. Autoinducer 2 of Fusobacterium nucleatum as a target molecule to inhibit biofilm formation of periodontopathogens. Arch Oral Biol. 2013;58(1):17–27. doi:10.1016/j.archoralbio.2012.04.016.
- Engevik MA, Danhof HA, Auchtung J, Endres BT, Ruan W, Basseres E, Engevik AC, Wu Q, Nicholson M, Luna RA, et al. Fusobacterium nucleatum adheres to Clostridioides difficile via the RadD adhesin to enhance biofilm formation in intestinal mucus. Gastroenterology. 2021;160(4):1301–14 e8. doi:10.1053/j.gastro.2020.11.034.
- Otto M. Physical stress and bacterial colonization. FEMS Microbiol Rev. 2014;38(6):1250–1270. doi:10.1111/1574-6976.12088.
- Kim MK, Ingremeau F, Zhao A, Bassler BL, Stone HA. Local and global consequences of flow on bacterial quorum sensing. Nature Microbiol. 2016;1(1):1. doi:10.1038/nmicrobiol.2015.5.
- Balcázar JL, Subirats J, Borrego CM. The role of biofilms as environmental reservoirs of antibiotic resistance. Front Microbiol. 2015;6:1216. doi:10.3389/fmicb.2015.01216.
- Flemming HC, Wingender J, Szewzyk U, Steinberg P, Rice SA, Kjelleberg S. Biofilms: an emergent form of bacterial life. Nat Rev Microbiol. 2016;14(9):563–575. doi:10.1038/nrmicro.2016.94.
- Lebeaux D, Ghigo JM, Beloin C. Biofilm-related infections: bridging the gap between clinical management and fundamental aspects of recalcitrance toward antibiotics. Microbiol Mol Biol Rev. 2014;78(3):510–543. doi:10.1128/MMBR.00013-14.
- Bertesteanu S, Triaridis S, Stankovic M, Lazar V, Chifiriuc MC, Vlad M, Grigore R. Polymicrobial wound infections: pathophysiology and current therapeutic approaches. Int J Pharm. 2014;463(2):119–126. doi:10.1016/j.ijpharm.2013.12.012.
- Alam A, Leoni G, Quiros M, Wu H, Desai C, Nishio H, Jones RM, Nusrat A, Neish AS. The microenvironment of injured murine gut elicits a local pro-restitutive microbiota. Nature Microbiol. 2016;1(2):1–8. doi:10.1038/nmicrobiol.2015.21.
- Swidsinski A, Ladhoff A, Pernthaler A, Swidsinski S, Loening–Baucke V, Ortner M, Weber J, Hoffmann U, Schreiber S, Dietel M. Mucosal flora in inflammatory bowel disease. Gastroenterology. 2002;122(1):44–54. doi:10.1053/gast.2002.30294.
- Swidsinski A, Weber J, Loening-Baucke V, Hale LP, Lochs H. Spatial organization and composition of the mucosal flora in patients with inflammatory bowel disease. J Clin Microbiol. 2005;43(7):3380–3389. doi:10.1128/JCM.43.7.3380-3389.2005.
- Drewes JL, White JR, Dejea CM, Fathi P, Iyadorai T, Vadivelu J, Roslani AC, Wick EC, Mongodin EF, Loke MF, et al. High-resolution bacterial 16S rRNA gene profile meta-analysis and biofilm status reveal common colorectal cancer consortia. NPJ Biofilms Microbiomes. 2017;3(1):34. doi:10.1038/s41522-017-0040-3.
- Dejea CM, Fathi P, Craig JM, Boleij A, Taddese R, Geis AL, Wu X, Shields D CE, Hechenbleikner EM, Huso DL. Patients with familial adenomatous polyposis harbor colonic biofilms containing tumorigenic bacteria. Science. 2018;359(6375):592–597. doi:10.1126/science.aah3648.
- Tytgat HLP, Nobrega FL, van der Oost J, de Vos WM. Bowel biofilms: tipping points between a healthy and compromised gut? Trends Microbiol. 2019;27(1):17–25. doi:10.1016/j.tim.2018.08.009.
- Hoarau G, Mukherjee P, Gower-Rousseau C, Hager C, Chandra J, Retuerto M, Neut C, Vermeire S, Clemente J, Colombel J-F, et al. Bacteriome and mycobiome interactions underscore microbial dysbiosis in familial Crohn’s disease. MBio. 2016;7(5):e01250–16. doi:10.1128/mBio.01250-16.
- Lazar V, Ditu LM, Pircalabioru GG, Gheorghe I, Curutiu C, Holban AM, Picu A, Petcu L, Chifiriuc MC. Aspects of gut microbiota and immune system interactions in infectious diseases, immunopathology, and cancer. Front Immunol. 2018;9:1830. doi:10.3389/fimmu.2018.01830.
- Ludwig S, Patrice F, Adrien F, Antoine H, Manuela T, David H, James C, Patrick L, Jacques S. Impact of oleic acid (cis-9-octadecenoic acid) on bacterial viability and biofilm production in Staphylococcus aureus. FEMS Microbiol Lett. 2008;149–155.
- Abdel-Aziz MM, Emam TM, Raafat MM. Hindering of cariogenic Streptococcus mutans biofilm by fatty acid array derived from an endophytic Arthrographis kalrae strain. Biomolecules. 2020;10(5):10. doi:10.3390/biom10050811.
- El-Mowafy SAS, El Galil MIA, H K. Sodium ascorbate as a quorum sensing inhibitor of Pseudomonas aeruginosa. J Appl Microbiol. 2014;117(5):1388–1399. doi:10.1111/jam.12631.
- Pandit S, Ravikumar V, Abdel-Haleem AM, Derouiche A, Mokkapati V, Sihlbom C, Mineta K, Gojobori T, Gao X, Westerlund F, et al. Low concentrations of vitamin C reduce the synthesis of extracellular polymers and destabilize bacterial biofilms. Front Microbiol. 2017;8:2599. doi:10.3389/fmicb.2017.02599.
- Gutiérrez-Barranquero JA, Reen FJ, Mccarthy RR, O’Gara F. Deciphering the role of coumarin as a novel quorum sensing inhibitor suppressing virulence phenotypes in bacterial pathogens. Appl Microbiol Biotechnol. 2015;99:3303–3316. doi:10.1007/s00253-015-6436-1.
- Proctor CR, Mccarron PA, Ternan NG. Furanone quorum-sensing inhibitors with potential as novel therapeutics against Pseudomonas aeruginosa. J Med Microbiol. 2020;69(2):195–206. doi:10.1099/jmm.0.001144.
- Singh VK, Kavita K, Prabhakaran R, Jha B. Cis-9-octadecenoic acid from the rhizospheric bacterium Stenotrophomonas maltophilia BJ01 shows quorum quenching and anti-biofilm activities. Biofouling. 2013;29(7):855–867. doi:10.1080/08927014.2013.807914.
- Nicol M, Alexandre S, Luizet JB, Skogman M, Jouenne T, Salcedo SP, De E. Unsaturated fatty acids affect quorum sensing communication system and inhibit motility and biofilm formation of Acinetobacter baumannii. Int J Mol Sci. 2018;19(1):214. doi:10.3390/ijms19010214.
- Markus V, Share O, Terali K, Ozer N, Marks RS, Kushmaro A, Golberg K. Anti-quorum sensing activity of stevia extract, stevioside, rebaudioside a and their aglycon steviol. Molecules. 2020;25(22):25. doi:10.3390/molecules25225480.
- Hou HM, Jiang F, Zhang GL, Wang JY, Zhu YH, Liu XY. Inhibition of Hafnia alvei H4 biofilm formation by the food additive dihydrocoumarin. J Food Protection. 2017;80(5):842–847. doi:10.4315/0362-028X.JFP-16-460.
- Defoirdt T, Crab R, Wood TK, Sorgeloos P, Verstraete W, Bossier P. Quorum sensing-disrupting Brominated Furanones protect the gnotobiotic brine shrimp artemia franciscana from pathogenic Vibrio harveyi , Vibrio campbellii , and Vibrio parahaemolyticus Isolates. Appl Environ Microbiol. 2006;72(9):6419–6423. doi:10.1128/AEM.00753-06.
- Rodriguez-Lopez P, Barrenengoa AE, Pascual-Saez S, Cabo ML. Efficacy of synthetic furanones on Listeria monocytogenes biofilm formation. Foods. 2019;8(12):647. doi:10.3390/foods8120647.
- Ben Amara H, Song HY, Ryu E, Park JS, Schwarz F, Kim BM, Choi BK, Koo KT. Effects of quorum-sensing inhibition on experimental periodontitis induced by mixed infection in mice. Eur J Oral Sci. 2018;126(6):449–457. doi:10.1111/eos.12570.
- Almasoud A, Hettiarachchy N, Rayaprolu S, Babu D, Kwon YM, Mauromoustakos A. Inhibitory effects of lactic and malic organic acids on autoinducer type 2 (AI-2) quorum sensing of Escherichia coli O157: H7 and Salmonella typhimurium. LWT-Food Sci Technol. 2016;O157:560–564. doi:10.1016/j.lwt.2015.11.013.
- Soni KA, Jesudhasan P, Cepeda M, Widmer K, Jayaprakasha GK, Patil BS, Hume ME, Pillai SD. Identification of ground beef–derived fatty acid Inhibitors of autoinducer-2–Based cell signaling. J Food Protection. 2008;71(1):134–138. doi:10.4315/0362-028X-71.1.134.
- Shivaprasad D, Taneja NK, Lakra A, Sachdev D. In vitro and in situ abrogation of biofilm formation in E. coli by vitamin C through ROS generation, disruption of quorum sensing and exopolysaccharide production. Food Chem. 2020;341:341. doi:10.1016/j.foodchem.2020.128171.
- Ellermann M, Jimenez AG, Pifer R, Ruiz N, Sperandio V, Skaar E, McFall-Ngai MJ. The canonical long-chain fatty acid sensing machinery processes arachidonic acid to inhibit virulence in enterohemorrhagic Escherichia coli. mBio. 2021;12(1):12. doi:10.1128/mBio.03247-20.
- An SQ, Murtagh J, Twomey KB, Gupta MK, Tang JL, Ingram R, Valvano MA, Tang J-L. Modulation of antibiotic sensitivity and biofilm formation in Pseudomonas aeruginosa by interspecies signal analogues. Nat Commun. 2019;10(1):10. doi:10.1038/s41467-019-10271-4.
- Kim HS, Cha E, Ham SY, Park JH, Nam S, Kwon H, Byun Y, Park HD. Linoleic acid inhibits Pseudomonas aeruginosa biofilm formation by activating diffusible signal factor-mediated quorum sensing. Biotechnol Bioeng. 2021;118(1):82–93. doi:10.1002/bit.27552.
- Mao N, Cubillos-Ruiz A, Cameron DE, Collins JJ. Probiotic strains detect and suppress cholera in mice. Sci Transl Med. 2018;10(445):10. doi:10.1126/scitranslmed.aao2586.
- Holowko MB, Wang H, Jayaraman P, Poh CL. Biosensing Vibrio cholerae with genetically engineered Escherichia coli. ACS Synth Biol. 2016;5(11):1275–1283. doi:10.1021/acssynbio.6b00079.
- Sedlmayer F, Hell D, Muller M, Auslander D, Fussenegger M. Designer cells programming quorum-sensing interference with microbes. Nat Commun. 2018;9(1):1822. doi:10.1038/s41467-018-04223-7.
- Falà AK, Álvarez-Ordóñez A, Filloux A, Gahan CG, Cotter PD. Quorum sensing in human gut and food microbiomes: Significance and potential for therapeutic targeting. Front Microbiol. 2022;13:1002185. doi:10.3389/fmicb.2022.1002185.
- Xie Y, Chen J, Wang B, Peng AY, Mao ZW, Xia W. Inhibition of quorum-sensing Regulator from Pseudomonas aeruginosa using a flavone derivative. Molecules. 2022;27(8):27. doi:10.3390/molecules27082439.
- Ananthakrishnan AN, Khalili H, Konijeti GG, Higuchi LM, De Silva P, Korzenik JR, Fuchs CS, Willett WC, Richter JM, Chan AT. A prospective study of long-term intake of dietary fiber and risk of Crohn’s disease and Ulcerative colitis. Gastroenterology. 2013;145(5):970–977. doi:10.1053/j.gastro.2013.07.050.
- Brown MM, Todd D, Cech N, Horswill A. 525 novel peptide from commensal Staphylococcus simulans blocks MRSA quorum sensing and protects host skin from damage. J Invest Dermatol. 2019;139(5):139. doi:10.1016/j.jid.2019.03.601.
- Wu S, Liu J, Liu C, Yang A, Qiao J. Quorum sensing for population-level control of bacteria and potential therapeutic applications. Cell Mol Life Sci. 2020;77(7):1319–1343. doi:10.1007/s00018-019-03326-8.
- Dang Z, Gao M, Wang L, Wu J, Guo Y, Zhu Z, Huang H, Kang G. Synthetic bacterial therapies for intestinal diseases based on quorum-sensing circuits. Biotechnol Adv. 2023;65:108142. doi:10.1016/j.biotechadv.2023.108142.
- Contreras-Ramos M, Mansell TJ. Leveraging quorum sensing to manipulate microbial dynamics. Curr Opin Biomed Eng. 2021;19:100306. doi:10.1016/j.cobme.2021.100306.
- Wu S, Feng J, Liu C, Wu H, Qiu Z, Ge J, Sun S, Hong X, Li Y, Wang X, et al. Machine learning aided construction of the quorum sensing communication network for human gut microbiota. Nat Commun. 2022;13(1):3079. doi:10.1038/s41467-022-30741-6.
- Wu S, Yang S, Wang M, Song N, Feng J, Wu H, Yang A, Liu C, Li Y, Guo F, et al. Quorum sensing-based interactions among drugs, microbes, and diseases. Sci China Life Sci. 2023;66(1):137–151.
- Dumitru R, Navarathna DH, Semighini CP, Elowsky CG, Dumitru RV, Dignard D, Whiteway M, Atkin AL, Nickerson KW. In vivo and in vitro anaerobic mating in Candida albicans. Eukaryot Cell. 2007;6(3):465–472. doi:10.1128/EC.00316-06.
- Hornby JM, Jensen EC, Lisec AD, Tasto JJ, Jahnke B, Shoemaker R, Dussault P, Nickerson KW. Quorum sensing in the dimorphic fungus Candida albicans is mediated by farnesol. Appl Environ Microbiol. 2001;67(7):2982–2992. doi:10.1128/AEM.67.7.2982-2992.2001.
- Ramage G, Saville SP, Wickes BL, López-Ribot JL. Inhibition of Candida albicans biofilm formation by farnesol, a quorum-sensing molecule. Appl Environ Microbiol. 2002;68(11):5459–5463. doi:10.1128/AEM.68.11.5459-5463.2002.
- Sharma M, Prasad R. The quorum-sensing molecule farnesol is a modulator of drug efflux mediated by ABC multidrug transporters and synergizes with drugs in Candida albicans. Antimicrob Agents Chemother. 2011;55(10):4834–4843. doi:10.1128/AAC.00344-11.
- Shirtliff ME, Krom BP, Meijering RA, Peters BM, Zhu J, Scheper MA, Harris ML, Jabra-Rizk MA. Farnesol-induced apoptosis in Candida albicans. Antimicrob Agents Chemother. 2009;53(6):2392–2401. doi:10.1128/AAC.01551-08.
- Cugini C, Calfee MW, Farrow JM, Morales D3rd, Pesci EC, Hogan DA, Hogan DA. Farnesol, a common sesquiterpene, inhibits PQS production in Pseudomonas aeruginosa. Mol Microbiol. 2007;65(4):896–906. doi:10.1111/j.1365-2958.2007.05840.x.
- Zawrotniak M, Wojtalik K, Rapala-Kozik M. Farnesol, a quorum-sensing molecule of Candida Albicans triggers the release of neutrophil extracellular traps. Cells. 2019;8(12):1611. doi:10.3390/cells8121611.
- LaSarre B, Federle MJ. Exploiting quorum sensing to confuse bacterial pathogens. Microbiol Mol Biol Rev. 2013;77(1):73–111. doi:10.1128/MMBR.00046-12.
- Hawver LA, Jung SA, Ng WL, Shen A. Specificity and complexity in bacterial quorum-sensing systems. FEMS Microbiol Rev. 2016;40(5):738–752. doi:10.1093/femsre/fuw014.