ABSTRACT
Morphine addiction is closely associated with dysbiosis of the gut microbiota. miRNAs play a crucial role in regulating intestinal bacterial growth and are involved in the development of disease. Ginsenoside Rg1 exhibits an anti-addiction effect and significantly improves intestinal microbiota disorders. In pseudo-germfree mice, supplementation with Bacteroides vulgatus (B. vulgatus) synergistically enhanced Rg1 to alleviate morphine addiction. However, it is currently unknown the relationship between fecal miRNAs in morphine-exposed mice and their potential modulation of gut microbiome, as well as their role in mediating the resistance of ginsenoside Rg1 to drug addiction. Here, we studied the fecal miRNA abundance in mice treated with morphine to explore the different miRNAs expressed, their association with B. vulgatus and their role in the amelioration of morphine reward by ginsenoside Rg1. Our results indicated ginsenoside Rg1 attenuated the significant increase in miR-129-5p expression observed in the feces of morphine-treated mice. The miR-129-5p, specifically, inhibited the growth of B. vulgatus by modulating the transcript of the site-tag BVU_RS11835 and increased the levels of 5-hydroxytryptophan and indole-3-carboxaldehyde in vitro. Subsequently, we noticed that oral administration of synthetic miR-129-5p increased 5-HT levels in the hippocampus and inhibited the reversal effect of ginsenoside Rg1 both on the relative abundance of B. vulgatus in the feces and CPP effect induced by morphine exposure. In short, Ginsenoside Rg1 might play an indirect role in remodeling the B. vulgatus against morphine reward by suppressing miR-129-5p expression. These results highlight the role of miR-129-5p and B. vulgatus in morphine reward and the anti-morphine addiction of ginsenoside Rg1.
Introduction
Morphine is an opioid drug and one of the effective analgesics for the treatment of postoperative and cancer pain.Citation1 Long-term and repeated use of morphine can stimulate reward pathways in the brain, leading to altered brain plasticity, strong psychological demands and addictive drug behavior.Citation2–4 Opioid addiction treatment commonly used drugs are methadone, buprenorphine, naloxone.Citation5 These drugs still have problems such as poor rehabilitation effect or serious side effects.Citation5,Citation6 Therefore, it is still important to further study the mechanism of morphine addiction and develop new therapeutic strategies.
The gut microbiome plays an important role in the development of drug addiction. Drug addiction changes the composition of gut microbiota, and gut microbiota affects host’s addictive behavior. The chronic morphine treatment alters the gut microbial composition;Citation7 The bacteria from Bacteroides, Faecalibacterium were less abundant whereas those from Thauera, Prevotella were more abundant in substance use disorders compared to healthy controls;Citation8 Probiotics VSL#3 pretreatment attenuates morphine tolerance.Citation9 However, although it is widely considered that there is a bidirectional interaction between morphine-treated hosts and gut microbiota, the related mechanisms remain to be uncovered.
The gut microbiome interacts with the host via various routes, including host-gut microbiota metabolism,Citation10 immune regulation,Citation11 and intestinal homeostasis.Citation12 MicroRNAs (miRNA, miR) have been found to play an important role in mediating the interaction between gut microbiome and their hosts in recent years. Host-produced miRNAs could enter bacteria, specifically regulate the transcription of bacterial genes and affect bacterial growth.Citation13 The feces of multiple sclerosis patients were rich in miRNA, in which miR-30d suppressed experimental autoimmune encephalomyelitis symptoms by increasing Akkermansia muciniphila.Citation14 However, the modulation role of miRNAs on intestinal bacteria in morphine addiction has been disregarded.
Ginsenoside Rg1 is a major active ingredient extracted from the root of Panax ginseng C.A. Meyer. The pharmacological effects of ginsenoside Rg1 on the central nervous system include neuroprotective effects, improvement of learning and memory.Citation15–17 Ginsenosides Rg1 can be used to treat a variety of neurodegenerative diseases, such as Alzheimer’s disease and Parkinson’s disease.Citation15,Citation17 Studies have shown that ginsenoside Rg1 significantly improved spatial learning deficits induced by morphine administration and inhibited morphine-induced hyperactivity and conditioned place preference (CPP).Citation18,Citation19
In our previous study,Citation20 we found that the relative abundance of B. vulgatus in the feces of morphine-exposed mice was significantly decreased, whereas it was increased by ginsenoside Rg1 treatment. Also, we found that the Rg1-induced amelioration of CPP was not observed in mice when their gut microbiome was depleted by non-absorbable antibiotics, whereas B. vulgatus supplementation synergistically enhanced Rg1-alleviated morphine-exposed CPP in mice in pseudo-germfree mice. Therefore, in this project, the B. vulgatus was used as a target species to explore the interaction between host, gut microbiome and ginsenoside Rg1 mediated by miRNA under morphine reward.
We studied miRNAs in morphine-treated and ginsenoside Rg1-treated mice to explore different miRNAs expressed and their relations with B. vulgatus, as well as their effects on host received morphine. Our results indicated that morphine treatment reduced miR-129-5p expression in the hippocampus and feces of mice, which ginsenoside Rg1 reversed. Specifically, miR-129-5p inhibited the growth of B. vulgatus by affecting bacterial transcription and increased the content of 5-hydroxytryptophan, 3-formylindole. Furthermore, oral delivery of miR-129-5p decreased the abundance of the gut commensal B. vulgatus and further increased serotonin (5-HT) expression in the hippocampus of morphine-exposed mice. Oral dosing of miR-129-5p inhibits the ameliorative effect of ginsenoside Rg1 to play an anti-morphine reward role.
Results
Ginsenoside Rg1 inhibited the morphine-induced CPP in mice
In this study, the effect of ginsenoside Rg1 () on morphine reward was investigated in a morphine-induced CPP mouse model (). As shown in , there were no significant differences in CPP scores and movement trajectories among the groups of mice before training. Compared to the control mice, the morphine-treated mice showed a significant increase in residence time in the white box after subcutaneous injection of morphine and 14 days of training, suggesting that morphine successfully induced CPP in the experimental mice. Compared to the morphine-treated mice, the residence time and movement trajectories of the ginsenoside Rg1-treated mice in the white box decreased. Moreover, no significant changes in CPP scores and movement trajectories were observed in mice treated with ginsenoside Rg1 alone.
Figure 1. Ginsenoside Rg1 inhibited the morphine-induced CPP in mice. (a) Structural formula of ginsenoside Rg1. (b) Diagram of experimental design for conditioned place preference (CPP) testing. (c) time spent in the drug compartment during pre-conditioning and post-conditioning (n=6). (d) Representative head trajectories of mice in CPP apparatus in pre- or post-conditioning phases. The groups were: the control group (C), the morphine group (M), the morphine + ginsenoside Rg1 group (MR), the ginsenoside Rg1 group (CR). Data were analyzed with one-way ANOVA. Values are mean ± SEM. ***p < .001 vs the control group; ###p < .001 vs the morphine group.
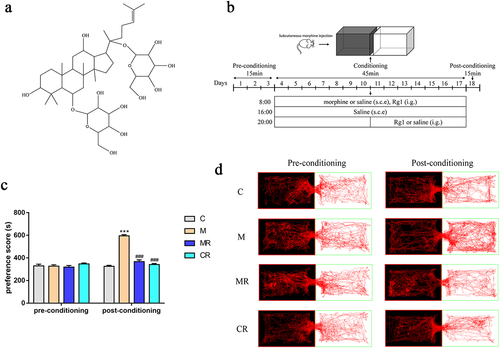
Morphine altered miRNA expression that was reversed by ginsenoside Rg1
Hippocampus and feces were found to be rich in miRNAs. To identify which miRNAs were generated during morphine addiction, we performed small RNA sequencing, in which we measured hippocampal and fecal RNA from control, morphine-exposed and ginsenoside Rg1-treated mice. We input the sequence of 50 most abundant miRNAs (, S1 and S2) and the whole genome sequence of B. vulgatus to RNA22 v2Citation21 to search for potential targets of miRNAs. Some of the miRNA targeting prediction results were shown in the Table S2. We selected four (miR-129-5p, miR-125a-5p, miR-30d-5p, and miR-let-7c-5p) of these miRNAs and verified the expression by qPCR (). In hippocampus, we found that miR-129-5p and miR-let-7c-5p expression were increased in the morphine group compared to the control and ginsenoside Rg1 treatment groups. In feces, we found that the miR-129-5p, miR-125a-5p and miR-30d-5p were increased in the morphine group compared to the control group and ginsenoside Rg1 treated group. The results showed that morphine altered miRNA expression that was reversed by ginsenoside Rg1 in hippocampus and feces of CPP mice.
Figure 2. Morphine altered miRNA expression that was reversed by ginsenoside Rg1. (a) the 50 most abundant miRnas in mice feces of control group. (b-e) relative expression of miR-let-7c-5p, miR-30d-5p, miR-125a-5p, and miR-129-5p in mice feces were verified by qPCR. Data were analyzed with one-way ANOVA. Values are mean ± SEM, n = 6. *p < .05 vs the control group; #p < .05 vs the morphine group.
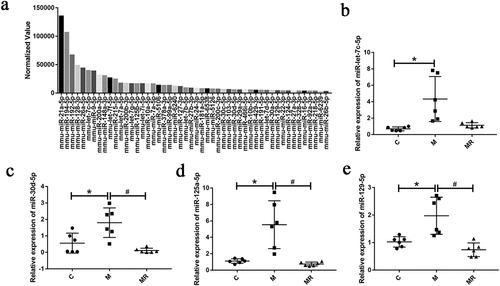
miR-129-5p and ginsenoside Rg1 affected the growth of B. vulgatus in vitro
Further, we need to determine whether specific miRNAs can affect B. vulgatus directly in vitro. Accordingly, we cultured B. vulgatus and Escherichia coli (E. coli) with synthesized miRNA mimics of four miRNAs in vitro. We found that miR-129-5p inhibited the growth of B. vulgatus (), while they did not have any effect on the growth of E. coli (Figure S3a). To explore how miR-129-5p regulates B. vulgatus, we performed transcriptome sequencing on the sediments of B. vulgatus. There were some changes in the transcriptome of miR-129-5p scramble-treated B. vulgatus, but slight (Figure S3C-D). The miR-129-5p-treated B. vulgatus was screened 116 differentially expressed genes, with 47 down-regulated and 69 up-regulated (). The differentially expressed genes were mainly concentrated in metabolism, enriched in histidine metabolism, glycine, serine and threonine metabolism, two-component system ( and Table S3). Among them, the expression of genes related to amino acid metabolism was down-regulated and in the two-component system related genes were up-regulated. We found that the expression of BVU_RS11835 (TolC family protein), a potential target gene of miR-129-5p predicted by RNA22 v2, was upregulated (Table S3) and we further predicted the secondary binding structure using RNAhybridCitation22 (). BVU_RS11835 might be the target gene of miR-129-5p acting on B. vulgatus.
Figure 3. Ginsenoside Rg1 and miR-129-5p affected the growth of B. vulgatus in vitro. (a) B. vulgatus was grown in culture media with 2 μM miR-129-5p mimics, miR-125a-5p mimics, miR-30d-5p mimics, miR-let-7c-5p mimics. Growth was monitored as absorbance at 600 nm (OD600) once per hour (n=3). (b) B. vulgatus was grown in culture media with 2 μM miR-129-5p and miR-let-7c-5p mimics, scramble and inhibitor. Growth was monitored as absorbance at 600 nm (OD600) once per hour (n=3). (c) B. vulgatus was grown in culture media with ginsenoside Rg1 of different concentrations. Growth was monitored as absorbance at 600 nm (OD600) once per hour (n=5). (d)B. vulgatus was cultured in the presence of 2 μM Cy3-labeled (red) miR-129-5p. (e) B. vulgatus gene (BVU_RS11835) were predicted to be targeted by miR-129-5p by RNA22 v2 and predicted for secondary structure property (minimum free energy, mfe) by RNAhybrid. (f-g) Volcano plot of differentially expressed genes in the miR-129-5p-treated and ginsenoside Rg1-treated B. vulgatus compared to the control B. vulgatus (n=3). (h-i) statistical graph of KEGG functional enrichment analysis in the miR-129-5p-treated and ginsenoside Rg1-treated B. vulgatus compared to the control B. vulgatus (n=3).
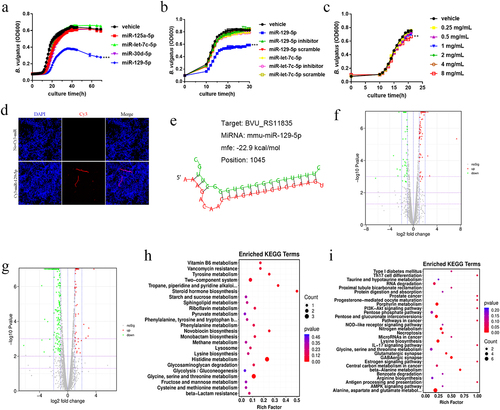
We found that ginsenoside Rg1 inhibited the growth of B. vulgatus () and E. coli (Figure S3B) in vitro. The ginsenoside Rg1-treated B. vulgatus was screened 219 differentially expressed genes, with 159 down-regulated and 60 up-regulated (). The differentially expressed genes were mainly concentrated in metabolism, enriched in glycine, serine and threonine metabolism, and alanine, aspartate and glutamate metabolism ( and Table S4). A previous studyCitation20 by our group found that ginsenoside Rg1 increased the abundance of B. vulgatus in feces of morphine-exposed mice, the same as our results in this animal experiment (). The opposite results of in vivo and in vitro experiments suggested to us that the modulatory effect of ginsenoside Rg1 on the intestinal flora of morphine-exposed mice may be indirect. Oral administration of ginsenoside Rg1 might indirectly affect the abundance of B. vulgatus in feces by regulating the expression of miR-129-5p in morphine-exposed mice.
miR-129-5p and ginsenoside Rg1 affected the metabolism of B. vulgatus
In order to further explore the relationship between ginsenoside Rg1 and miR-129-5p and B. vulgatus, we studied the changes of metabolites in each group of B. vulgatus. There were some changes in the metabolism of miR-129-5p scramble-treated B. vulgatus, but slight (Figure S4A-B). The miR-129-5p-treated B. vulgatus was screened 78 differential metabolites, with 54 up-regulated and 24 down-regulated (). The up-regulated metabolites were mainly involved in the pathways including tyrosine metabolism, styrene degradation, and phenylalanine metabolism; The down-regulated metabolites were mainly involved in the pathways including D-amino acid metabolism, aminoacyl-tRNA biosynthesis, biosynthesis of amino acids (). We found that the content of 5-hydroxytryptophan (5-HTP) and indole-3-carboxaldehyde, which are related to tryptophan metabolism, increased significantly in miR-129-5p-treated B. vulgatus (). 5-HTP is effective in increasing the level of 5-HT in the central nervous system and has been shown to be effective in treating a variety of diseases.Citation23,Citation24
Figure 4. Ginsenoside Rg1 and miR-129-5p affected the metabolism of B. vulgatus. (a-b) Hierarchical clustering results of different metabolites in the miR-129-5p-treated, or ginsenoside Rg1-treated compared to the control B. vulgatus. (c-d) DAscore plots of overall, down-regulated metabolic pathways in the miR-129-5p-treated, or ginsenoside Rg1-treated compared to the control B. vulgatus. (e) the content of 5-hydroxytryptophan and indole-3-carboxaldehyde in each group (n=3). (f) the content of ginsenoside-related metabolites in ginsenoside Rg1-treated B. vulgatus (n=3). Data were analyzed with one-way ANOVA. n = 3. *p < .05, **p < .01 vs the control groups.
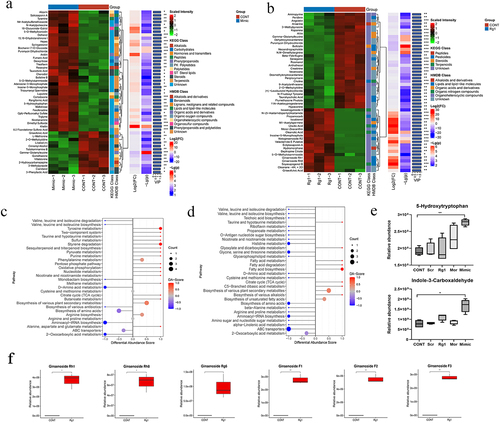
The ginsenoside Rg1-treated B. vulgatus was screened 137 differential metabolites, with 63 up-regulated and 74 were down-regulated. Up-regulated metabolites were mainly involved in pathways including fatty acid biosynthesis, fatty acid metabolism, taurine and hypotaurine metabolism; The down-regulated metabolites were mainly involved in the pathways including histidine metabolism, glycine, serine and threonine metabolism, ABC transporters, and biosynthesis of amino acids (). We found that Rg1-treated B. vulgatus produced a large number of secondary metabolites of ginsenoside, such as ginsenoside F1, Rh1, F2, Rh8 (). Ginsenoside Rg1 might be metabolized by B. vulgatus.
Oral administration of miR-129-5p decreased the relative abundance of B. vulgatus and inhibited the amelioration of ginsenoside Rg1 on morphine-induced CPP in mice
To investigate whether miR-129-5p affects the inhibitory effect of ginsenoside Rg1 on morphine-induced CPP in mice, we synthesized miR-129-5p and gavaged mice with miR-129-5p for seven consecutive days (). We observed that the level of miR-129-5p was significantly increased in the feces after 4 h of oral gavage (), suggesting that miR-129-5p could reach the feces. Then, we found that the relative abundance of B. vulgatus was significantly decreased in the feces of mice treated with miR-129-5p alone. In addition, we found that the relative abundance of B. vulgatus in the feces of the morphine-treated, morphine+miR-129-5p+Rg1-treated, morphine+ miR-129-5p-treated mice was significantly decreased compared to the control mice (). Compared with the morphine-treated mice, the relative abundance of B. vulgatus in the Rg1-treated mice was significantly increased and that of B. vulgatus in the morphine+ miR-129-5p-treated mice was significantly decreased. Similarly, the relative abundance of B. vulgatus was significantly decreased in the morphine+miR-129-5p+Rg1-treated mice compared to the Rg1-treated group. Oral administration of miR-129-5p might reduce the relative abundance of B. vulgatus in feces. Peroral delivery of miR-129-5p inhibited the reversal effect of ginsenoside Rg1 on the relative abundance of B. vulgatus in the feces of morphine-exposed mice.
Figure 5. Oral administration of miR-129-5p inhibited the amelioration of ginsenoside Rg1 on morphine-induced CPP in mice. (a) Diagram of experimental design for CPP testing. (b) relative B. vulgatus abundance using real-time qPCR in feces (n=6). (c) relative abundance of miR-129-5p in feces after oral administration (n=5). (d) Representative head trajectories of mice in CPP apparatus in pre- or post-conditioning phases. (e) time spent in the drug compartment during pre-conditioning and post-conditioning (n = 8). The groups were: the control group (C), the miR-129-5p scramble group (CC), the miR-129-5p mimic group (CM), the morphine group (M), the morphine + ginsenoside Rg1 group (MR), the morphine + ginsenoside Rg1 + miR-129-5p mimic group (MMR), the morphine + miR-129-5p mimic group (MM). Data were analyzed with one-way ANOVA. Values are mean ± SEM. *p < .05, **p < .01, ***p < .001 vs the control group; #p < .05, ##p < .01, ###p < .001 vs the morphine group; ▲p <.05, ▲▲p <.01, ▲▲▲p <.001 vs the MR group; ●p <.05 vs the MMR group.
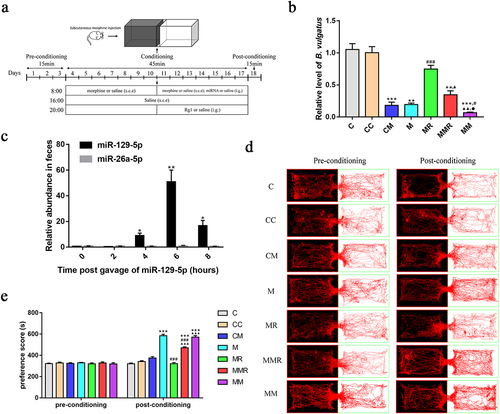
We used the Smart 3.0 software (Panlab) to track the mice heads and record their trajectories and residence time within 15 min (). During the pre-conditioning phase, no significant difference was observed in the trajectories or CPP scores between the control and other groups. Compared with the control group and baseline, mice in the morphine group showed a significant increase in their residence time and trajectory in the white box after subcutaneous morphine injection and training in the black and white box for 14 days, indicating the successful establishment of the mice morphine-induced CPP model. Compared with the morphine-treated mice, the residence time and trajectory of Rg1-treated mice and morphine+miR-129-5p+Rg1-treated mice in the white box were significantly reduced. However, the residence time and trajectory of mice in the white box were significantly increased in the morphine+miR-129-5p+Rg1-treated mice compared to the Rg1-treated mice. Administration of miR-129-5p in conjunction with ginsenoside Rg1 administration attenuated the inhibitory effect of ginsenoside Rg1 on morphine-induced CPP in mice.
miR-129-5p reduced the amelioration of ginsenoside Rg1 on morphine addiction
Current research suggests that morphine-based drug addiction can cause neurotransmitter changes in the brain.Citation25 To investigate the role of miR-129-5p in the alleviation of morphine addiction by ginsenoside Rg1, we measured the levels of GABA, 5-HT, and DA in hippocampus and serum (). We found that oral administration of miR-129-5p increased 5-HT content in the hippocampus of morphine-exposed mice. The regulatory effects of ginsenoside Rg1 on GABA, 5-HT, and DA in morphine-exposed mice were inhibited by miR-129-5p treatment. Ginsenoside Rg1 increased the expression of GAD1 and GAD2 in the hippocampus, but this regulatory effect was inhibited by miR-129-5p ().
Figure 6. MiR-129-5p reduced the amelioration of ginsenoside Rg1 on morphine reward. (a and d) Concentration of GABA in serum and hippocampus. (b and e) Concentration of 5-HT in serum and hippocampus. (c and f) Concentration of dopamine in serum and hippocampus. (g-h) the relative expression of GAD1 and GAD2 were verified by qPCR. (i) the protein levels of GAD1 and GAD2. Data were analyzed with one-way ANOVA. The groups were: the control group (C), the miR-129-5p scramble group (CC), the miR-129-5p mimic group (CM), the morphine group (M), the morphine + ginsenoside Rg1 group (MR), the morphine + ginsenoside Rg1 + miR-129-5p mimic group (MMR), the morphine + miR-129-5p mimic group (MM). Values are mean ± SEM, n = 3 for protein qualification and n = 6 for other data. *p < .05, ** p < .01, ***p < .001 vs. The control group; #p < .05, ##p < .01, vs the morphine group; ▲p <.05, ▲▲p <.01, ▲▲▲p <.001 vs. The MR group.
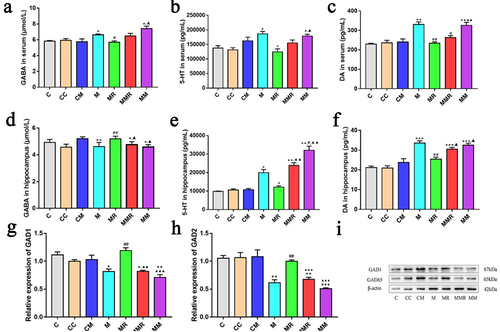
Discussion
Opioid addiction is a public health threat worldwide.Citation5 Morphine is the representative drug of opioids. It is widely believed that morphine addiction significantly affects the composition of the gut microbiota.Citation8,Citation20 Morphine tolerance is attenuated in germfree mice,Citation9 suggesting that the microbiota plays a critical role in morphine addiction.
MiRNAs were found to play an important role in mediating the interaction between gut microbiome and host. A large number of miRNAs are present in the intestinal lumen and feces of humans and animals.Citation13,Citation26 miRNAs can be absorbed by intestinal microbes and subsequently alter bacterial gene expression.Citation12 Here, we found a significant increase in miR-129-5p expression in the feces of morphine-exposed mice. Through growth curve and laser confocal microscopy experiments, we demonstrated that exogenous miR-129-5p was internalized by B. vulgatus and inhibited the growth of B. vulgatus. The transcriptome sequencing results showed that miR-129-5p regulated the gene expression of B. vulgatus. MiR-129-5p might inhibit the growth of B. vulgatus by upregulating site-tagged transcripts of BVU_RS11835.
Morphine addiction may be closely related to the Bacteroides in the intestine. It was found that the number of Bacteroides in the feces of morphine induced CPP model mice decreased and showed a trend of recovery after drug discontinuation;Citation27 the number of Bacteroides in the feces of patients using opioid agonists decreased.Citation28 B. vulgatus, the dominant species of the mouse intestinal Bacteroides, had reduced abundance in the feces of morphine-exposed mice.Citation20 In this study, we found that oral administration of miR-129-5p decreased the relative abundance of B. vulgatus in the feces and increased the levels of 5-HT in the hippocampus. By non-targeted metabolomics, we found that miR-129-5p increased the content of 5-HTP and indole-3-carboxaldehyde in B. vulgatus in vitro. It was found that 5-HTP from the gut microbiota could be absorbed into the bloodstream and then cross the blood-brain barrier to increase 5-HT levels in the brain.Citation24 5-HT is a major neurotransmitter associated with depression, addiction, and other neuropsychiatric illnesses.Citation29 Serotonin was effective in stimulating the communication of hippocampal neurons, promoting the formation and enhancement of memory.Citation30 Systemic morphine produced significant increases in extracellular 5-HT in the hippocampus.Citation31 These studies suggest that the morphine-exposed host might increase the 5-HTP level of B. vulgatus through miR-129-5p and further increase the 5-HT level in the hippocampus to help the host maintain addiction to drugs.
Panax ginseng C.A. Meyer is widely used in drug treatment, and its active ingredient is ginsenoside.Citation32 It is difficult for ginsenosides to exert direct pharmacological effects, and intestinal microbiota plays a decisive role in the biological activity of ginsenosides.Citation33 Our previous study found that depletion of the gut microbiota influenced ginsenoside Rg1 to exert its anti-morphine addiction role, while B. vulgatus synergistically enhanced the effect of ginsenoside Rg1 to alleviate morphine addiction in mice.Citation20 Here, our results indicated that morphine altered miR-129-5p expression that was reversed by ginsenoside Rg1. Oral administration of miR-129-5p reduced the relative abundance of B. vulgatus in the feces of ginsenoside Rg1-treated morphine-exposed mice and further inhibited the ameliorative effect of ginsenoside Rg1 on morphine reward. Ginsenoside Rg1 might play an indirect role in remodeling B. vulgatus against morphine reward by inhibiting miR-129-5p expression.
In conclusion, ginsenosides and intestinal microbiota were in an interactive relationship. Ginsenoside Rg1 might play a role in reshaping the intestinal microbiota by affecting the expression of miRNA in morphine-exposed host; the intestinal microbiota was involved in the biotransformation of ginsenoside Rg1. Notably, we identified a highly expressed miRNA in morphine-exposed mice that could be involved in addiction by affecting the growth of specific bacteria and the production of its associated metabolites. The results presented here provide insights into miRNA enriched in the feces of morphine-exposed mice, which might contribute to the understanding of the relationship between drug reward, ginsenoside Rg1 and intestinal microbiota.
Material and methods
Animals
Animal procedures were approved by the Science and Technology Committee and the Institutional Animal Care and Use Committee of Southern Medical University. BALB/c mice were from the Experimental Animal Center of Southern Medical University (Guangzhou, China) (Guangdong, SCXK 2021–0041) and acclimated in the local animal facility for at least 5 days prior to study initiation. Otherwise specified, all mice used were male and 6–7 weeks old at the initiation of study, and they were randomly allocated into groups. Mice were housed under specific pathogen-free conditions at the Laboratory Animal Management Center of the Southern Medical University (Guangzhou, China) (Guangdong, SCXK 2021–0167).
Drugs
Morphine hydrochloride was provided by the PLA General Logistics Department supply station. Ginsenoside Rg1 (purity ≥98%) was purchased from Chengdu Herbpurify CO., LTD.
Establishment of the morphine-induced CPP model
The place conditioning apparatus were made by our research group, as shown in Chen’s article.Citation20 The CPP apparatus were created using a divider to divide one box into black and white smaller boxes with equal volumes (20 cm long × 13 cm wide × 10 cm deep). After the divider was lifted, the mice could freely move around between the white and black boxes.
The CPP tests () consisted of three stages and took place over 14 days.Citation34 In the first stage (Days 1 to 3), the mice were allowed to freely move around the CPP box and the time spent by each mouse in the black and white boxes within 15 min was recorded, and the mice with significant preference for the white box were excluded. There remaining mice were randomized into three groups (n = 6): the control group, morphine (9 mg/kg) group (M), the morphine + ginsenoside Rg1 (200 mg/kg) (MR) group, and ginsenoside Rg1 (200 mg/kg) (CR) group. On days 4–10 of the CPP experiment (Stage 2), a divider was used to separate the black and white boxes, and the mice underwent CPP training twice per day continuously for 7 days. The specific protocol was as follows: every morning at 08:00, the mice in the model, and MR groups received subcutaneous injections of morphine (9 mg/kg), while an equal volume of subcutaneous injections containing physiological saline was administered to the control group and CR group. Subsequently, the mice were placed in a white box for 1 h of training. Every afternoon at 16:00, an equal volume of physiological saline was administered to the mice in the four groups before they were placed in a black box for 1 h of training. On days 11–17, every night at 20:00, ginsenoside Rg1 was administered through gavage to the MR group, which conducted continuously for seven days. On day 18 of the experiment (Stage 3), the mice were not administered morphine for 24 h before CPP was administered. The test procedure was similar to that of the baseline measurement process. After the CPP test was completed, fecal samples were collected immediately upon defecation. At last, the mice were anesthetized, and decapitated after entering deep anesthesia and the hippocampus were removed.
Isolation of RNA from feces and hippocampus
According to the manufacturer’s recommendation, QIAgen miRNeasy Mini Kit (Qiagen, Valencia, CA, USA) was used for total RNA extraction from fecal samples. Total RNA extracted from hippocampus was using TRIzol reagent (AG Accurate Biology, Hunan, China). RNA quality was assessed by A260/A280 ratios using DS-11 Spectrophotometer (DeNovix, USA). The purity of RNA was A260/A280: 1.8 ~ 2.0, A260/A230: ≥1.
Small RNA sequencing
Three micrograms of total RNA per sample was used as input for the small RNA library. The small RNA library was checked for quality with Agilent 2200 TapeStation and were sequenced with the Illumina HiSeqTM 2500 platform. Data was mapped to the reference sequence using BWA.Citation35 Normalization expression were performed with the edgeR.Citation36
miRNA target prediction
We selected the 50 most abundant miRNAs in the blank group, and these miRNAs were expressed in the feces of mice in the control, morphine and ginsenoside Rg1 treatment mice. (, S1 and S2). The sequence of 50 most abundant miRNAs were paired with the whole genome sequence (NC_009614.1) of B. vulgatus using the RNA22 v2.Citation21 RNAhybridCitation22 was used to calculate the secondary binding structure between miR-129-5p and potential targeting B. vulgatus sequence.
MiRNA measurement by qPCR
Quantitative PCR (qPCR) was performed to verify the relative level of miRNAs that were identified in small RNA-Seq. The primers for miRNAs and internal reference U6 were designed using the Bulge-loop™ miRNA qRT-PCR Primer Sets from Ribobio Co. Ltd. (Guangzhou, China). RNA was input for miRNA cDNA synthesis using Evo M-MLV RT Kit (AG Accurate Biology, Hunan, China), which the primers in the kit were replaced with bulge-loop primers. MiRNA cDNAs were then quantified by real-time PCR using SYBR Green Premix Pro Taq HS qPCR Kit (AG Accurate Biology, Hunan, China) following the manufacturer’s protocol.
In vitro bacteria growth measurements
B. vulgatus (ATCC 8428, Microbiologics, USA) and Escherichia coli (E. coli, provided by Wei Fenghuan research group of Southern Medical University) were cultured respectively in Difco-Reinforced Clostridial Medium (BD Bioscience, New Jersey, USA) and LB medium (Hopebio, Shandong, China) supplemented with miRNA mimics, scramble miRNAs, inhibitor miRNAs and ginsenoside Rg1 at 37°C and monitored as absorbance at 600 nm (OD600).
Detection of miRNA entering bacteria by confocal
B. vulgatus was cultured in the presence of 2 μM synthesized Cy3-labeled (red) miR-129-5p, and B. vulgatus cultured without Cy3-labeled miR-129-5p was used as a control. The bacterial suspension obtained from the culture was centrifuged at 12,000 r/min for 1 min and the precipitate was taken. After washing the precipitate twice with cold PBS, 10 μL of the bacterial suspension was aspirated and applied on the slide. After the bacterial suspension was dried, it was fixed with 2% PFA for 10 min, and then washed several times with cold PBS. Subsequently, B. vulgatus was stained with 10 μg/mL of DAPI (blue) for 10 min. At last, one drop of anti-fluorescence quencher was added and the coverslip was covered. The above steps were done under light-proof conditions. Samples were observed under a laser confocal microscope using a 63× objective.
Preparation of bacterial sequencing samples
B. vulgatus was grown in culture media with 2 μM miR-129-5p mimics (Mimic), 2 μM miR-129-5p scramble (Scr), 20 mg/mL ginsenoside Rg1 (Rg1) to logarithmic growth phase. Meanwhile, a blank control group (CONT) was set up. The bacterial suspensions were centrifuged, and the bacterial fluids and bacterial sediments were collected separately. The bacterial sediments were washed several times with PBS and snap frozen in liquid nitrogen, and stored at −80°C. The bacterial sediment was used for transcriptome sequencing, and the bacterial fluid was used for LC-MS untargeted metabolomics.
Transcriptome sequencing
Extraction of total RNA from bacterial sediment by Trizol. Sequencing libraries were generated using NEBNextő Ultra II Directional RNA Library Prep Kit for Illumina (NEB, Ipswich, MA, USA). The qualified cDNA library used Illumina HiSeq 2500 platform for PE150 sequencing. Quality control of sequencing data and reference genome alignment were performed. Further, differential expression analysis and functional annotation analysis were performed.
Non-targeted metabolomics
The 1 mL bacterial fluid was accurately weighed, and the metabolites were extracted using 1 mL of pre-cooled methanol/acetonitrile/water (2:2:1, v/v/v). The mixture was allowed to settle at −20°C and treated with ultrasound at 40 KHz for 1 h, and then placed at −20°C for 2 h. After centrifugation at 16,000 × g and 4°C for 20 min, the supernatant was transferred to sample vials for LC-MS analysis. Nexera X2 LC-30AD ultra-high performance liquid chromatography system (Shimadzu, Japan) was used for chromatographic separation, and QE Plus mass spectrometer (Thermo Fisher Scientific,USA) was used for mass spectrometry collection. The metabolite structures were identified and searched using public databases such as HMDB, MassBank and the local metabolite standards library of Bioprofile Biotechnology Co. (Shanghai, China). Further, the differentially expressed metabolites were analyzed and KEGG enrichment analysis was performed.
Oral administration of miR-129-5p combined with the CPP test
The mice were randomly divided into seven treatment groups: the control group (C), the miR-129-5p scramble group (500 pmol, ig) (CC), the miR-129-5p mimic group (500 pmol, ig) (CM), the morphine group (9 mg/kg, sc) (M), the morphine + ginsenoside Rg1 group (200 mg/kg, ig) (MR), the morphine + ginsenoside Rg1 + miR-129-5p mimic group (200 mg/kg + 500 pmol, ig) (MMR), the morphine + miR-129-5p mimic group (500 pmol, ig) (MM). CPP training using the methods described in this chapter. In the second stage (days 11–17), the CM, MMR and MM groups were gavaged with miR-129-5p mimic at a dose of 500 pmol once a day for 1 week.
Dynamics of oral administered miR-129-5p in the feces
Synthetic miR-129-5p was orally administered to mice and feces were collected at the gavage (0 hour), and every 2 hours post administration. The levels of miRNA were quantified with qPCR using the approach described in this chapter.
Quantification of B. vulgatus in feces
Fecal DNA was extracted and isolated using the TIANamp Stool DAN Kit (TIANGEN, Shanghai, China), according to the manufacturer’s instructions. Using the universal 16S rDNA (all bacteria) as an internal reference, the operational taxonomic units were quantified using qPCR. Primers used for detecting the abundance of B. vulgatus were listed in Table S1. The relative quantity was calculated using the comparative CT method normalizing to the amount of all bacteria in the sample.
Enzyme linked immunosorbent assay
The concentrations of 5-hydroxytryptamine and dopamine in hippocampus and serum were quantified using Mouse 5-Hydroxytryptamine (5-HT) ELISA Kit and Mouse dopamine (DA) ELISA Kit (CUSABIO, Wuhan, China), according to the manufacturer’s instructions. The concentrations of γ-aminobutyric acid in hippocampus and serum were quantified using Mouse γ-aminobutyric (GABA) ELISA Kit (MEIMIAN, Jiangsu, China), according to the manufacturer’s instructions.
Determination of gene expression by quantitative real-time PCR
Total RNA was isolated from the hippocampus using TRIzol reagent (AG Accurate Biology, Hunan, China). cDNA was synthesized using the Evo M-MLV RT Kit (AG Accurate Biology, Hunan, China). Quantitative real-time PCR was performed using SYBR Green Premix Pro Taq HS qPCR Kit (AG Accurate Biology, Hunan, China), as previously described. The primers used in this experiment were designed by BGI Tech Solutions Co. Ltd., (Beijing, China) (Table S1). Data were normalized to the housekeeping gene GAPDH and analyzed using the 2−ΔΔCT method. Data are presented as the mean ± standard error.
Western blotting analysis
Proteins from the hippocampus were extracted by RIPA lysate (Beyotime, Shanghai, China). The primary antibodies used were beta Actin (Servicebio, Wuhan, China), GAD1 and GAD2 (Abcam, Cambridge, MA, USA). Secondary antibody was HRP conjugated Goat Anti-Rabbit IgG (Servicebio, Wuhan, China). Chemiluminescence was performed by Immobilon Western HRP substrate (Millipore, Massachusetts, USA).
Statistical analysis
The GraphPad Prism software (version 8.0) was used for statistical analysis. Datasets were tested for normality. For data that met the orthogonal distribution, differences between groups were treated by one-way ANOVA; for data that did not meet the orthogonal distribution, a nonparametric test was used. A two-sided P-value of ≤ 0.05 was considered as significant.
Authors’ contributions
C.L., Y.L. and R.L. contributed equally to this work; C.L. and Z.M. conceived the concept and designed the study; C.L., Y.L. and R.L. performed the research; Z.C. and Q.Z. analyzed and interpreted the data; C.L. and Z.M. drafted the article; C.-H. L. reviewed the manuscript; Z.M. obtained funding and supervised the study.
Ethics statement
The ethics of the study protocol and experimental procedures were approved by the Science and Technology Committee and the Institutional Animal Care and Use Committee of Southern Medical University.
Supplemental Material
Download Zip (8 MB)Disclosure statement
No potential conflict of interest was reported by the author(s).
Data availability statement
Small RNA sequencing data and transcriptome sequencing data were deposited in Sequence Read Archive (SRA) (https://www.ncbi.nlm.nih.gov/sra) under the accession number PRJNA941221 and PRJNA1013199. The Non-targeted metabolomics data was uploaded to MetaboLights (https://www.ebi.ac.uk/metabolights/) under the accession number MTBLS7341.
Supplementary material
Supplemental data for this article can be accessed online at https://doi.org/10.1080/19490976.2023.2254946
Additional information
Funding
References
- Dowell D, Ragan KR, Jones CM, Baldwin GT, Chou R. CDC clinical practice guideline for prescribing opioids for pain—United States, 2022. MMWR Recomm Rep. 2022;71(3):1–14. doi:10.15585/mmwr.rr7103a1.
- Kim J, Ham S, Hong H, Moon C, Im HI. Brain reward circuits in morphine addiction. Mol Cells. 2016;39:645–653. doi:10.14348/molcells.2016.0137.
- Beltrán-Campos V, Silva-Vera M, García-Campos ML, Díaz-Cintra S. Effects of morphine on brain plasticity. Neurología. 2015;30(3):176–180. doi:10.1016/j.nrl.2014.08.004.
- Listos J, Łupina M, Talarek S, Mazur A, Orzelska-Górka J, Kotlińska J. The mechanisms involved in morphine addiction: an overview. Int J Mol Sci 2019 20. 20(17): 4302. doi:10.3390/ijms20174302.
- Volkow ND, Jones EB, Einstein EB, Wargo EM. Prevention and treatment of opioid misuse and addiction: a review. JAMA Psychiarty. 2019;76:208–216. doi:10.1001/jamapsychiatry.2018.3126.
- Kreek MJ, Reed B, Butelman ER. Current status of opioid addiction treatment and related preclinical research. Sci Adv. 2019;5(10):eaax9140. doi:10.1126/sciadv.aax9140.
- Banerjee S, Sindberg G, Wang F, Meng J, Sharma U, Zhang L, Dauer P, Chen C, Dalluge J, Johnson T, et al. Opioid-induced gut microbial disruption and bile dysregulation leads to gut barrier compromise and sustained systemic inflammation. Mucosal Immunol. 2016;9(6):1418–1428. doi:10.1038/mi.2016.9.
- Xu Y, Xie Z, Wang H, Shen Z, Guo Y, Gao Y, Chen X, Wu Q, Li X, Wang K, et al. Bacterial diversity of intestinal microbiota in patients with substance use disorders revealed by 16S rRNA gene deep sequencing. Sci Rep. 2017;7(1):3628. doi:10.1038/s41598-017-03706-9.
- Zhang L, Meng J, Ban Y, Jalodia R, Chupikova I, Fernandez I, Brito N, Sharma U, Abreu MT, Ramakrishnan S, et al. Morphine tolerance is attenuated in germfree mice and reversed by probiotics, implicating the role of gut microbiome. Proc Natl Acad Sci U S A. 2019;116(27):13523–13532. doi:10.1073/pnas.1901182116.
- Nicholson JK, Holmes E, Kinross J, Burcelin R, Gibson G, Jia W, Pettersson S. Host-gut microbiota metabolic interactions. Sci. 2012;336(6086):1262–1267. doi:10.1126/science.1223813.
- Hooper LV, Littman DR, Macpherson AJ. Interactions between the microbiota and the immune system. Sci. 2012;336(6086):1268–1273. doi:10.1126/science.1223490.
- Kamada N, Chen GY, Inohara N, Núñez G. Control of pathogens and pathobionts by the gut microbiota. Nat Immunol. 2013;14(7):685–690. doi:10.1038/ni.2608.
- Liu S, da Cunha AP, Rezende RM, Cialic R, Wei Z, Bry L, Comstock L, Gandhi R, Weiner H. The host shapes the gut microbiota via fecal microRNA. Cell Host & Microbe. 2016;19(1):32–43. doi:10.1016/j.chom.2015.12.005.
- Liu S, Rezende RM, Moreira TG, Tankou SK, Cox LM, Wu M, Song A, Dhang FH, Wei Z, Costamagna G, et al. Oral administration of miR-30d from feces of MS patients suppresses MS-like symptoms in mice by expanding Akkermansia muciniphila. Cell Host & Microbe. 2019;26(6):779–94.e8. doi:10.1016/j.chom.2019.10.008.
- Yang SJ, Wang JJ, Cheng P, Chen LX, Hu JM, Zhu GQ. Ginsenoside Rg1 in neurological diseases: from bench to bedside. Acta Pharmacol Sin. 2023;44(5):913–930. doi:10.1038/s41401-022-01022-1.
- Zarneshan SN, Fakhri S, Khan H. Targeting Akt/CREB/BDNF signaling pathway by ginsenosides in neurodegenerative diseases: a mechanistic approach. Pharmacol Res. 2022;177:106099. doi:10.1016/j.phrs.2022.106099.
- Sun Y, Yang Y, Liu S, Yang S, Chen C, Lin M, Zeng Q, Long J, Yao J, Yi F, et al. New therapeutic approaches to and mechanisms of ginsenoside Rg1 against neurological diseases. Cells. 2022;11(16):2529. doi:10.3390/cells11162529.
- Qi D, Zhu Y, Wen L, Liu Q, Qiao H. Ginsenoside Rg1 restores the impairment of learning induced by chronic morphine administration in rats. J Psychopharmacol. 2009;23(1):74–83. doi:10.1177/0269881107082950.
- Kim HS, Hong YT, Jang CG. Effects of the ginsenosides Rg1 and Rb1 on morphine-induced hyperactivity and reinforcement in mice. J Pharm Pharmacol. 1998;50(5):555–560. doi:10.1111/j.2042-7158.1998.tb06198.x.
- Chen Z, Lin Y, Zhou Q, Xiao S, Li C, Lin R, Li J, Chen Y, Luo C, Mo Z, et al. Ginsenoside Rg1 mitigates morphine dependence via regulation of gut microbiota, tryptophan metabolism, and serotonergic system function. Biomed Pharmacother. 2022;150:112935. doi:10.1016/j.biopha.2022.112935.
- Miranda KC, Huynh T, Tay Y, Ang YS, Tam WL, Thomson AM, Lim B, Rigoutsos I. A pattern-based method for the identification of MicroRNA binding sites and their corresponding heteroduplexes. Cell. 2006;126(6):1203–1217. doi:10.1016/j.cell.2006.07.031.
- Krüger J, Rehmsmeier M. Rnahybrid: microRNA target prediction easy, fast and flexible. Nucleic Acids Res. 2006;34:W451–4. doi:10.1093/nar/gkl243.
- Javelle F, Lampit A, Bloch W, Häussermann P, Johnson SL, Zimmer P. Effects of 5-hydroxytryptophan on distinct types of depression: a systematic review and meta-analysis. Nutr Rev. 2020;78:77–88. doi:10.1093/nutrit/nuz039.
- Zhang ZW, Gao CS, Zhang H, Yang J, Wang YP, Pan LB, Yu H, He C-Y, Luo H-B, Zhao Z-X, et al. Morinda officinalis oligosaccharides increase serotonin in the brain and ameliorate depression via promoting 5-hydroxytryptophan production in the gut microbiota. Acta Pharm Sin B. 2022;12(8):3298–3312. doi:10.1016/j.apsb.2022.02.032.
- Tahsili-Fahadan P, Aston-Jones G. Neurobiology of opioid addiction. In: eds., Encyclopedia of behavioral neuroscience. Oxford: Academic Press. 2010, pp. 393–403. 10.1016/B978-0-08-045396-5.00074-9.
- Francavilla A, Ferrero G, Pardini B, Tarallo S, Zanatto L, Caviglia GP, Sieri S, Grioni S, Francescato G, Stalla F, et al. Gluten-free diet affects fecal small non-coding RNA profiles and microbiome composition in celiac disease supporting a host-gut microbiota crosstalk. Gut Microbes. 2023;15(1):2172955. doi:10.1080/19490976.2023.2172955.
- Zhang J, Deji C, Fan J, Chang L, Miao X, Xiao Y, Zhu Y, Li S. Differential alteration in gut microbiome profiles during acquisition, extinction and reinstatement of morphine-induced CPP. Prog Neuropsychopharmacol Biol Psychiatry. 2021;104:110058. doi:10.1016/j.pnpbp.2020.110058.
- Gicquelais RE, Bohnert ASB, Thomas L, Foxman B. Opioid agonist and antagonist use and the gut microbiota: associations among people in addiction treatment. Sci Rep. 2020;10(1):19471. doi:10.1038/s41598-020-76570-9.
- Kim DY, Camilleri M. Serotonin: a mediator of the brain–gut connection. Am J Gastroenterol. 2000;95(10):2698–2709. doi:10.1111/j.1572-0241.2000.03177.x.
- Teixeira CM, Rosen ZB, Suri D, Sun Q, Hersh M, Sargin D, Dincheva I, Morgan AA, Spivack S, Krok AC, et al. Hippocampal 5-HT input regulates memory formation and Schaffer collateral excitation. Neuron. 2018;98(5):992–1004.e4. doi:10.1016/j.neuron.2018.04.030.
- Tao R, Auerbach SB. Increased extracellular serotonin in rat brain after systemic or intraraphe administration of morphine. J Neurochem. 1994;63(2):517–524. doi:10.1046/j.1471-4159.1994.63020517.x.
- Kim HC, Shin EJ, Jang CG, Lee MK, Eun JS, Hong JT, Oh K-W. Pharmacological action of Panax ginseng on the behavioral toxicities induced by psychotropic agents. Arch Pharm Res. 2005;28(9):995–1001. doi:10.1007/bf02977391.
- Yang L, Zou H, Gao Y, Luo J, Xie X, Meng W, Zhou H, Tan Z. Insights into gastrointestinal microbiota-generated ginsenoside metabolites and their bioactivities. Drug Metab Rev. 2020;52(1):125–138. doi:10.1080/03602532.2020.1714645.
- Cunningham CL, Gremel CM, Groblewski PA. Drug-induced conditioned place preference and aversion in mice. Nat Protoc. 2006;1(4):1662–1670. doi:10.1038/nprot.2006.279.
- Li H, Durbin R. Fast and accurate short read alignment with burrows–wheeler transform. Bioinf. 2009;25(14):1754–1760. doi:10.1093/bioinformatics/btp324.
- Gao D, Kim J, Kim H, Phang TL, Selby H, Tan AC, Tong T. A survey of statistical software for analysing RNA-seq data. Hum Genomics. 2010;5(1):56–60. doi:10.1186/1479-7364-5-1-56.