ABSTRACT
The musculoskeletal system is important for balancing metabolic activity and maintaining health. Recent studies have shown that distortions in homeostasis of the intestinal microbiota are correlated with or may even contribute to abnormalities in musculoskeletal system function. Research has also shown that the intestinal flora and its secondary metabolites can impact the musculoskeletal system by regulating various phenomena, such as inflammation and immune and metabolic activities. Most of the existing literature supports that reasonable nutritional intervention helps to improve and maintain the homeostasis of intestinal microbiota, and may have a positive impact on musculoskeletal health. The purpose of organizing, summarizing and discussing the existing literature is to explore whether the intervention methods, including nutritional supplement and moderate exercise, can affect the muscle and bone health by regulating the microecology of the intestinal flora. More in-depth efficacy verification experiments will be helpful for clinical applications.
Introduction
With the increasing severity of global aging, aging-related diseases, including sarcopenia, osteoporosis and osteoarthritis, have become increasingly prominent, affecting at least 7–27% of the world’s population.Citation1–3 The incidence of sarcopenia ranges from 10 to 27%,Citation1 the prevalence of osteoporosis among women over 50 years of age is no less than 20%,Citation2 and the prevalence of osteoarthritis is approximately 7%.Citation3 Despite tremendous efforts to control such diseases, there is less effective treatment available. At present, functional improvement and symptom control with the aim of improving the quality of life of patients remain the main goals in the management of musculoskeletal disorders. To shift from symptom management to individualized interventions targeting its pathogenesis, there is an urgent need to explore new pathogenic pathways and mechanisms, which could provide new therapeutic targets for musculoskeletal health-related diseases. In view of the subtle relationship between intestinal flora and its balance with body inflammation and metabolic function, emerging research has focused on the relationship between intestinal flora and its microecology and musculoskeletal health related diseases.
The gut microbial community is a complex but delicately balanced microecosystem composed of archaea, bacteria and eukaryotes, which exist as symbiotic entities in the gut.Citation4 The intestinal flora is currently considered a new, complex organ composed of approximately 500 to 1000 species of microorganisms and 1014 bacteria, which is approximately 1.3 times the total number of human cells and more than 100 times the total number of human genes.Citation5,Citation6 It is affected by mode of birth, infant feeding, lifestyle, medication and host genetics. It is the largest microecosystem in the human body, and exhibits symbiosis with the host to maintain a dynamic equilibrium state of physiology.Citation7 Although clear definitions of probiotics and detrimental microbes within the human gut microbiota are debatable, researchers tend to classify intestinal microbiota into probiotic, detrimental bacterial and conditioned bacterial strains.Citation8,Citation9
Intestinal microbiota is closely related to body health. Microorganisms in the intestinal tract participate in the onset and progression of various diseases and affect the physiological functions.Citation10 Accumulating evidence shows that intestinal microorganisms and their metabolites exert a critical effect on the host immune system, nervous system, endocrine and metabolic functions. Thus, the intestinal flora is also called as “the second brain”.Citation11 Recently, with the boom in molecular biology and high-throughput sequencing technology, significant progress has been made in research on the gut microbiota.Citation5 Numerous studies have indicated that intestinal microecology plays an important role in maintaining gut homeostasis in the host and affects the progression of many diseases,Citation7,Citation12 such as neurodegenerative diseases,Citation13,Citation14 cardiovascular diseasesCitation15 and metabolic diseases.Citation16 These biological mechanisms involve inflammation, anabolic metabolism, insulin sensitivity, nutrient bioavailability, toxin release and energy metabolism.Citation17,Citation18
Of note, the microbiota in the human intestine is not static, and their variety can change with age.Citation19 Age-related changes in the gut microbiota are primarily manifested as intestinal ecological imbalance, a decline in bacterial diversity, an increase in opportunistic pathogens, and a reduction in the number of probiotics. It is not difficult to deduce that the gut microbiota participates in intricate mechanisms that lead to aging muscular dystrophy, such as sarcopenia.Citation20
With thorough investigation, the impact of the intestinal microbiota on the musculoskeletal system has gradually come to light and has attracted the attention of researchers. Moreover, some researchers have proposed the concept of a “gut-muscle axis”Citation21 and “gut-bone axis”.Citation22 The composition and multiformity of the intestinal microbiota may be a determinant of metabolism and function in the musculoskeletal system. Dysbiosis and homeostasis of the gut microbiota can affect energy metabolism, immune and endocrine status, synthesis of secondary metabolites and host musculoskeletal homeostasis through the gut-muscle axis and gut-bone axis.Citation23,Citation24 This suggests that the intestinal microbiota is tightly associated with the functions of the musculoskeletal system and even mediates musculoskeletal diseases such as sarcopenia and osteoporosis (OP). Available data support microbial dysregulation (i.e., adverse changes in gut microbiota diversity) as a cause of disorders associated with systemic chronic low-grade inflammation, which is an important contributor to the pathogenesis of musculoskeletal disorders,Citation25 while, the impact of intestinal microbiota on the musculoskeletal system, potential mechanisms and intervention methods have not been comprehensively and systematically reported. Based on the above considerations, we summarized and induced the interactions and intrinsic mechanisms between intestinal microbiota and the musculoskeletal system, such as muscle synthesis and breakdown, mitochondrial energy metabolism, oxidative stress injury, inflammation and osteoblast/osteoclast homeostasis. Following this, we summarized effective intervention measures to promote musculoskeletal health by regulating dysbiosis of the gut microbiota.
Effects and mechanism of the intestinal microbiota on the musculoskeletal system
Effect of “absence or presence of intestinal microbiota” on musculoskeletal system and its mechanism
Skeletal muscle
Experiments based on germ-free mice reveal the absence or presence of gut microbiota in vivo, which is crucial for the health of skeletal muscle by regulating material and energy metabolism pathways.Citation26 The absence or presence of the gut microbiota in the body is critical for skeletal muscle health. A healthy intestinal microbiota can affect skeletal muscle function and metabolism through multiple pathways and mediators.Citation26 Skeletal muscle mass was significantly lower in mice lacking gut microbiota, and the expression of myosin rejunction genes and skeletal muscle differentiation regulatory genes was significantly lower in germ-free (GF) mice than in normal germ-carrying mice, whereas the expression of muscle atrophy markers was significantly higher.Citation26,Citation27 Protein synthesis and degradation are important factors that affect skeletal muscle quality. The levels of insulin-like growth factor 1 (IGF-1), an important substance that promotes protein synthesis and enhances muscle function, decrease significantly in GF mice.Citation26,Citation28 Glucocorticoid-induced catabolism of branched amino acids is significantly increased in GF mice.Citation26,Citation29 Intestinal flora can also affect skeletal muscles by changing the patterns of energy metabolism. GF mice have lower glucose and insulin contents and significantly disrupted mitochondrial function, which affects the utilization of glucose by skeletal muscles, reduces the energy supply for muscle synthesis, and eventually affects the oxidative metabolic capacity of skeletal muscles.Citation26,Citation30,Citation31 In addition, the level of choline decreased in GF mice, resulting in the impairment of neuromuscular junction transmission.Citation26,Citation32,Citation33 Troponin is related to muscle fiber contractility and motor function in the skeletal muscles. The expression of the gene encoding troponin was reported to be markedly decreased in GF mouse skeletal muscle, indicating potential impairment of muscle fiber contractility.Citation26,Citation34 More importantly, after the intestinal microbiota was transplanted into GF mice, the results showed increased skeletal muscle mass, decreased muscle atrophy markers, improved muscle oxidative metabolism, and increased expression of neuromuscular junction assembly genes.Citation21,Citation26 This implies that intestinal microbiota plays a key role in maintaining normal skeletal muscle function.
Bone
The bone is continuously reconstructed through bone osteoblasts (OBs) and osteoclasts (OCLs). An imbalance in this process can lead to osteoporosis.Citation35 Early studies have found that the intestinal microbiota is also a major adjuster of bone mass, and its impact on bone mass is mediated through its effect on the immune system, which in turn adjusts osteoclast genesis.Citation36 Specifically, the loss of intestinal microbiota induces an abnormal increase in bone mass. Simultaneously, the number of osteoclast precursor cells and osteoclasts in the bone marrow of GF mice was reduced. Moreover, GF mice have decreased levels of gut-derived 5-hydroxytryptamine (5-HT) and inflammatory cytokines. Notably, colonization of GF mice with intestinal microbiota restored the lost bone mass. Of course, it cannot be excluded that other mechanisms may also involve in it.Citation37 This result implies that the gut microbiota has a negative impact on maintaining bone mass. However, a recent study showed that colonization with gut microbiota from mice or humans does not cause bone loss in GF Swiss Webster and/or C57BL/6 mice.Citation38 Furthermore, adult male GF BALB/c mice exhibit slower bone growth than normally raised mice.Citation39 Long-term colonization of GF mice with fecal microbiota results in a surprising increase in femur length and improvement in trabecular bone microarchitecture.Citation40 In addition, vitamin D has a great effect on improving intestinal calcium absorption to promote bone metabolism.Citation41 It has been suggested that GF mice have defective vitamin D metabolism, while GF mice colonized by the microbiota indicated the restoration of 1, 25-dihydroxyvitamin D and calcium levels.Citation42 Thus, the gut microbiota also has a beneficial effect on bone. These paradoxical results of gut microbiota on bone are partly due to differences in mouse strain, sex, age, content of colonization, or differences in antibiotic regimens, but the most likely reason may be the composition of the microbial community.Citation43 Considering the critical role of intestinal microbiota in regulating bone health status, it is of great significance to probe the communication mechanism between the intestinal microbiota and host bone. This transfer of functional extracellular vesicles from specific microorganisms to bone tissue may be a critical mechanism for the transgene-mediated regulation of bone health.Citation43,Citation44
Joint
Accumulating evidence indicates that the gut microbiome plays a critical role in the pathology of osteoarthritis (OA).Citation45 Earlier studies have shown that spontaneous T cell-dependent Rheumatoid Arthritis (RA) cannot be induced in IL-1αR−/−mice under GF conditions.Citation46 In toll-like receptor 4 (TLR4)-deficient mice, RA manifestations were also significantly inhibited, and the gut microbiota was thought to act as an antigen or adjuvant to induce or assist RA production.Citation46,Citation47 The gut microbiota also has an impact on the development of traumatic OA. Studies have found that OA caused by medial meniscus instability surgery is significantly reduced in GF mice, suggesting that the intestinal microbiota promotes the development of OA.Citation48 The gut microbiome has also been found to have a significant impact on the severity of injury-induced OA in a mouse model. After joint injury, GF mice had only mild OA symptoms, and the severity of the pathological manifestations was the lowest. Microbiome abundance in mice was positively correlated with inflammatory biomarker concentration, intestinal permeability and OA severity. This may be because, in the presence of joint injury, immune activation due to the gut microbiota (composed of more Fusobacterium and Faecalis and less Ruminococcaceae) may exacerbate the pathological process of OA.Citation45
These animal experiments results indicate that the “presence or absence” of intestinal microbiota multiformity is indeed a critical factor about affecting and maintenance the musculoskeletal system. In the following section, we further elaborate on the effects and mechanisms of intestinal microbiota on the fitness of the musculoskeletal system from the perspectives of microbiota imbalance and probiotics along with their secondary metabolites.
Effect of intestinal microbiota dysbiosis and its mechanism on musculoskeletal system
Intestinal microbiota dysbiosis refers to a state in which multiformity alternates within the intestinal microbiome. It mainly includes the bloom of pathobionts, reduction of normal flora and disappearance of the diversity of microbiota composition. Multiple factors, such as metabolic, autoimmune, inflammatory and neurodegenerative diseases, have been associated with intestinal dysbiosis.Citation49 The influence of microbiota dysregulation on the musculoskeletal system has attracted increasing attention.
Skeletal muscle
Many factors are relevant to the pathology of sarcopenia, including aging, inflammation, mitochondrial damage, and/or insulin resistance. Interestingly, almost all of these pathological processes trigger dysregulation of the intestinal microbiota.Citation49 In turn, microbiota disharmony also plays a crucial role in the decline in skeletal muscle mass and function. Since the gut microbiome is known to be responsible for the development and exacerbation of metabolic dysregulation phenotypes such as obesity or insulin resistance, skeletal muscle mass and function could partially regulate by gut microbiome.Citation23
The major phyla of healthy gut microbiota are Firmicutes, Bacteroidetes, and Actinobacteria, while Verrucomicrobia and Proteobacteria are less extent. Similar gut dysbiosis was observed between the elder and the undernourished, both showing an increase in Ruminococcus gnavus, which promotes inflammation, and a decrease in Roseburia intestinalis, a butyrate producer.Citation50 And Proteobacteria phylum seems to be more abundant in the gut microbiota of children with severe malnutrition.Citation51 Rapid loss of muscle mass and changes in gut microbiota composition also occur in cachexia individuals. In cancer patients, the abundance of Bifidobacterium, Lactobacillus, and Faecalibacterium decreased, while Enterobacteriaceae and Enterococcus increased. Similar microbial colon patterns have been found in other patients with non-cancer cachexia who have chronic kidney, heart, or liver disease.Citation23,Citation52 The analysis of gut microbiota composition in the elderly population showed that microbiota composition changes with age. Individuals with high frailty scores show a significant reduction in relative abundance of Lactobacilli, Bacteroides/Prevotella, and Faecalibacterium prausnitzii, and increased Enterobacteriaceae, of which Faecalibacterium prausnitzii is considered an indicator of good gut health because it produces health-promoting short-chain fatty acids such as butyrate.Citation53–55
The increase in circulating pro-inflammatory cytokines induced by gut dysbiosis can induce muscle atrophy via different mechanisms (insulin resistance, inflammation, and associated oxidative stress).Citation23,Citation56 Among them, muscle failure caused by inflammatory bowel disease (IBD)Citation57 and agingCitation58 are the two most typical examples of the gut microbiota dysbiosis. We use these two examples to specifically elaborate the intrinsic mechanism of sarcopenia induced by gut microbiota dysbiosis.
IBD is typically characterized by a dysregulation of the gut microbiota (primarily a decline in Firmicutes and Bacteroidetes, a relative heightened of Entero bacteriaceae) that disrupts the integrity of the intestinal barrier and then increases the pathogen penetration of intestinal tissues, thereby leading to lipopolysaccharide (LPS) translocation into the systemic circulation.Citation59 Studies have demonstrated that gut microbiota dysregulation is often accompanied by intestinal barrier damage and an increase in intestinal barrier permeability, which eases the entry of endotoxins and other bacterial metabolites into the circulatory system, thus increasing the levels of LPS and other inflammatory factors and inducing inflammatory responses in the body.Citation58,Citation60 A characteristic manifestation of LPS-induced inflammation is severe muscle atrophy caused by increased proteolytic degradation and decreased protein synthesis.Citation61 The diversity of intestinal microbiota may also lead to intestinal oxidative stress, intestinal mucosal inflammation and barrier dysfunction, thus causing immune dysfunction.Citation60 Oxidative stress, caused by high levels of reactive oxygen species (ROS), can cause skeletal muscle contraction disorders, resulting in muscle weakness and fatigue.Citation62 Therefore, inflammatory responses and oxidative stress caused by an imbalance in intestinal microbiota can negatively regulate muscle function. In a mouse model of colitis, skeletal muscle mass and muscle fiber cross-sectional area decreased and muscle protein content decreased in the quadriceps femoris and gastrocnemius muscles.Citation63 At the same time, muscle dysfunction worsens and the muscle growth markers Insulin-like growth factor 1 receptor (IGF-1 R) and phospho-mammalian target of rapamycin (mTOR) are downregulated.Citation64 Researchers believe that inflammation caused by dysregulation of the gut microbiota may be a triggering factor for skeletal muscle atrophy. The enhanced expression of muscle atrophy F-box (atrogin-1) and muscle ring finger protein 1 (Murf-1), which are related to the breakdown of myofibrils, mediates the accelerated breakdown of myofibrils.Citation65 Clinical studies have also found that muscle injury is a common pathological feature of chronic gastrointestinal diseases such as IBD. Sarcopenia occurs in 42% of IBD patients.Citation66 The intestinal microbiota stimulates mucosal immune cells to promote pro-inflammatory cytokines (interleukin 6 (IL-6), interleukin 10 (IL-10), tumor necrosis factor alpha (TNF-α), etc.), produce the general state of chronic low-grade inflammation, activate oxidative stress injury, and further influence insulin sensitivity, amino acid biosynthesis, mitochondria, biological generation, the synthesis and catabolism of muscle and the increase muscle attenuation-related molecular pathways. This can lead to musculoskeletal damage and weakness.Citation67–69
Similarly, the multifarious composition of the intestinal microbiota in frail or motile elderly also shows varying levels of dysregulation, with reduced species richness and an imbalance between opportunistic pathogens and anti-inflammatory flora.Citation53,Citation70 Dysregulation of the gut microbiota may be related to the complicated mechanisms of muscular atrophy.Citation58,Citation71 Specifically, the intestinal microbiota diversity decreases due to aging. Simultaneously, the ability to regulate the gut environment also decreases, the gut barrier function weakens, and the gut mucosal permeability increases, which collectively leads to increased absorption of bacterial products, including LPS, and activation of the inflammatory response in the body.Citation72 The gut microbiome associated with aging promotes inflammation so that levels of circulating inflammatory mediators increase, and reversing these age-related microbiome changes is a potential strategy to reduce age-related inflammation and accompanying morbidity.Citation72 LPS promotes inflammatory signaling, induces inflammation and insulin resistance in skeletal muscles, thereby contributing to the process of metabolic syndrome, and then induces skeletal muscle aging.Citation73–75 Studies showed that LPS-related cytokines decisively affect the ability to balance (i.e., synthesis and breakdown) proteins, and that increased cytokines with aging may induce muscle mass reduction.Citation76,Citation77 An enhanced inflammatory response can aggravate skeletal muscle mass loss. For example, in patients with sarcopenia, increased levels of pro-inflammatory factors such as IL-6 and TNF-α, among others, have been reported to induce a decrease in muscle mass.Citation78 Anti-TNF treatment can reverse age-related changes in the microbiota and therefore has potential anti-muscle aging effects.Citation72 The study also found that changes in the diversity and composition of the gut microbiota are associated with the disturbance of immune homeostasis during aging. The small intestines of young germ-free mice transplanted with the gut microbiota of older mice identified immune-related differential genetic signatures, including reduced antigen presentation and altered cytokine and chemokine production. These genes may play a potential role as markers of immune disorders during the aging of the gut microbiome.Citation79
Changes in the composition of the gut microbiota ecosystem due to various of the aforementioned causes (inflammation, malnutrition, cachexia, aging, etc.) may lead to intestinal leakage and the release of bacterial endotoxins (e.g., LPS) into the peripheral blood. LPS can trigger macrophages to produce inflammatory cytokines and ROS through TLR4 receptor. In skeletal muscle, TNF-α activates the expression of genes involved in the nuclear factor-κb (NF-κB) pathway, thereby reducing muscle cell differentiation and proliferation by inhibiting myogenin and myoD. IL-6 and IκB kinase can inhibit insulin receptor substrate 1, which is associated with the induction of insulin resistance, limiting the activation of muscle targets of rapamycin complex 1 (mTORC1), thereby limiting protein synthesis in muscle cells. In addition, the inhibitory effect on forkhead box O can no longer be exerted due to the inhibition of protein kinase B, which leads to increased expression of ubiquitin E3 ligases, Atrogin-1 and MuRF1, promoting muscle growth proteolysis. Similarly, autophagy activating kinase 1, which is not inhibited by mTORC1, further induced autophagy in skeletal muscle cells. When these regulatory mechanisms induced by gut dysbiosis are activated, an imbalance between protein breakdown/synthesis occurs and ultimately leads to muscle atrophy.Citation23
Other mechanisms include that the quorum-sensing peptide iAM373 produced by Enterobacter faecalis during bacterial dysregulation downregulated most skeletal muscle development and differentiation genes, reduced the metabolic activity of myoblast cells, and upregulated the proteasome degradation pathway in muscle ducts, which is a new inducer of sarcopenia.Citation80 Cohort research has suggested that sarcopenia is also correlated with a decrease in short-chain fatty acid (SCFAs)-producing gut microbiota, resulting in an imbalance in gut flora.Citation81,Citation82 Dysregulation of the intestinal flora may lead to skeletal muscle atrophy through upregulation of the primary bile acid-farnesoid X receptor pathway.Citation83
Bone
As the critical role of intestinal microbiota in the bone metabolic steady state is better understood, there is growing interest in the important role of gut microbiota in regulating bone healthCitation84. Researchers have shown a strong relationship between intestinal microbiota dysbiosis and bones, which suggests that the intestinal microbiota regulates bone metabolismCitation24,Citation85. Varying degrees of evidence, both from animal models and human studies, support the close connection between gut microbiota dysregulation and bone health or diseaseCitation86.
It was found that the damage of intestinal microbiota and poor microbial diversity in TLR5-deficient mice induce changes in whole bone strength;Citation87 In addition, the damage of intestinal flora caused by long-term use of antimicrobials (mainly characterized by the obvious decline of Bacteroides and the significant upregulation of Proteobacteria) lead to the damage of bone performance in mice, especially in the reduction of total bone strength.Citation88 Ovariectomy induces dysregulation of gut microbiota and leads to bone mass loss in mice, which is mediated by microbe-dependent T lymphocytes (such as T helper cell 17 (Th17) cells).Citation89
Changes in the intestinal microbiota have been observed in patients with skeletal disorders. Existing cross-sectional studies have shown an association between intestinal microbiota and the accumulation of bone mineral density.Citation90 Recently, a genome-wide study identified the gut bacterial taxa Clostridiales and Lachnospiraceae related to bone mass variability, revealing a causal link between microbiota and bone development.Citation91 The study found that patients with bacterial overgrowth had lower bone mass and higher rates of bone loss than healthy people.Citation92 Aggravated bone loss is a common complication of IBD, and dysregulation of the gut microbiota is also an important manifestation of IBD.Citation93,Citation94
Clinical studies have shown that the composition and abundance of the intestinal microbiota in individuals with low bone mineral density are significantly decreased. Bacterial groups, including Roseburia, Bifidobacterium and Lactobacillus were positively correlated with bone mineral density. However, in people with low bone mineral density, the microbiota associated with LPS synthesis is more abundant.Citation95 The Firmicutes/Bacteroidetes ratio usually represents the unbalance of intestinal flora in different degrees or states. The study found that the Firmicutes/Bacteroidetes ratio was negatively associated with bone mass, and the abundance of Actinobacteria and Bifidobacteriaceae was positively correlated with bone volume. Analysis of gut microbiota in OP patients using 16s RNA sequencing showed that gut microbiota diversity estimates were negatively correlated with bone mineral density.Citation96,Citation97 In patients with OP, an increase in the abundance of representative bacterial genera was observed, such as Actinomyces, Clostridium Cluster XlVa, Eggerthella, Blautia, Lactobacillus, Ruminococcaceae and Parabacteroides.Citation98,Citation99 Other studies have found that the diversity of intestinal microbiota in OP patients, particularly the quantity of Dialister and Faecalibacterium increased significantly.Citation100
The microbiota has a necessary effect on nutrient transport and absorption, which are required for bone health, such as calcium and vitamin D.Citation101 Dysregulation of gut microbiota may impair the transport of nutrients and calcium through the gut into the circulatory system. The decrease in intestinal absorption capacity of 1, 25(OH) 2-D3 and calcium increases with age, which is closely related to the dysregulation of intestinal microbiota.Citation102 This is one of the primary reasons that microbiota dysbiosis affects bone health.
In addition, the immune and inflammatory systems are thought to intervene between the gut microbiota and bone metabolism. The change in biodiversity and colonization of opportunistic pathogens leads to an increase in bacterial endotoxins, such as LPS,Citation103,Citation104 which is related to an increase in the intestinal inflammatory response, and the increase in inflammation is related to the activation of osteoclasts. Intestinal flora dysregulation mediates inflammation, especially Interleukin 1 (IL-1), TNF-α and IL-6, which play a key role in osteoclast activation as long as OP.Citation105,Citation106 The proliferation of intestinal microbiome-dependent Th17 cells and TNF-α-producing T cells produces a large number of pro-inflammatory cytokines (IL-17 and TNF-α), receptor activator of NF-κB ligand (RANKL),Citation107 and decreases the secretion of RANKL antagonists (RANKL induces osteoclast function while IL-17 reduces bone formation; TNF-α enhances the activity of RANKL and induces Th17 cell proliferation and activation), which may be the underlying immune regulatory mechanism in the process of OP.Citation108 Th17 cells are a population of osteoclasts that produces IL-17 and secretes TNF-α and RANKL. Interestingly, newly generated osteoclasts induced the generation of Treg cells.Citation109 It suppresses immune responses, induces and maintains immune tolerance, reduces inflammation through several pathways, and yields immunosuppressive cytokines, such as TGF-β and IL-10.Citation110 The subtle and complex relationship between Treg and Th17 cells can influence bone health. Crucially, gut bacteria are key to controlling this balance.Citation86
5-HT is classified into two types according to where it is synthesized: brain-derived and gut-derived 5-HT. Interestingly, these two 5-HT have different functions: gut-derived serotonin has a negative impact on bone formation, whereas brain-derived serotonin has an opposite influence.Citation111 In recent years, studies have found that gut flora not only induces cytokines to adjust bone metabolism, but also downregulates gut-borne serotonin levels by reducing the serotonin biosynthesis enzyme and increasing serotonin transporters, thereby regulating bone metabolism.Citation37
IGF-1 also plays a crucial role in the regulation of bone formation and growth. Gut microbiota may regulate overall bone quality by altering IGF-1 levels. For example, colonization of adult GF mice by conventionally specific intestinal microbiota can increase circulating IGF-1 and increase the formation and resorption of bone.Citation40,Citation112 Reduced gut microbiota diversity may also lead to a decrease in circulating estrogen, which in turn affects normal bone calcium deposition because the intestinal microbiota adjusts estrogen through β-glucosidase secretion, which breaks down estrogen into its active form.Citation113
According to the above research results, it is suggested that, similar to muscle health, bone health-related intestinal flora disorder is mainly manifested by the dominant intestinal flora, leading to excessive LPS production or causes more inflammatory reactions. Mechanisms mainly involve the subtly controlled osteogenic/osteoclastic balance of cytokines and Treg and Th17 cells. Difference from muscle health, the effect of gut dysbiosis on the effective absorption of calcium ions and the regulatory effects of gut-derived 5-HT, IGF-1, and estrogen are also highlighted here. Whether these mechanisms also have a role in muscle health may be a meaningful direction of exploration.
Joint
Dysbiosis of intestinal flora is involved in the pathogenesis of OA. Abnormalities in intestinal microbiota can seriously impair joint function.Citation25,Citation45
Fecal microbiota transplants from patients with impaired metabolism accelerate OA in mice, and dysregulation of the gut microbiota (e.g., abundance changes of Ruminococcus, Faecalis and Fusobacterium) may aggravate the severity of cartilage and subchondral bone pathology in OA mice.Citation45 In mice, variations in the intestinal flora induced by ampicillin and neomycin reduced serum LPS levels and suppressed inflammatory responses. This leads to improvement in OA after joint injury.Citation114 Chronic antibiotics also attenuate cartilage injury by altering the multiformity of the gut flora in mice with a spontaneous metabolic syndrome phenotype.Citation88
Population studies have found that the abundance of Lentisphaerae was inversely associated with the OA prevalent.Citation115 And the abundance of intestinal streptococci is correlated with intensive knee pain and inflammation.Citation116 But existing studies only weakly support the relationship between the gut microbiome and OA, particularly the association between certain groups or microbial products and inflammatory status and OA symptom severity (including knee pain), and there is a lack of high-quality studies on the potential role of the gut microbiome in OA-related pain.Citation117
Studies have suggested that the prevalence of OA is possibly due to the specific changes in gut microbiota composition observed in the gut ecosystem of the elderly, in particular an increase in opportunistic pro-inflammatory bacteria and a significant decline in symbiotic bacteria with anti-inflammatory properties.Citation118 Alterations in intestinal permeability may explain the influence of intestinal microbiota on the emergence and persistence of inflammatory diseases. Once the mucosal barrier is disturbed, components of the exogenous lumen invade the gut environment and mucosal inflammation and immune activation ensue.Citation119 Gut bacteria can bias the immune system toward a pro-inflammatory state, which can eventually lead to joint inflammation.Citation120 An increase in intestinal barrier permeability due to an imbalance in the intestinal microbiota was positively correlated with OA severity in mice.Citation45 Intestinal microbiota may mediate OA through the translocation of microbial molecules into the circulatory system. LPS is produced by gut microbiota and migrates to the circulatory system, contributing to low-grade inflammation. Metabolic endotoxemia, which involves the interaction between gut-derived LPS and TLR4, has recently been recognized as a common source of low-grade inflammation, including OA.Citation121 The study also confirmed that serum LPS levels were positively correlated with the number of activated macrophages in the knee synovium and the severity of arthritis.Citation122 The intestinal microbiota affects Tregs in the colon, suggesting that intestinal microbiota also modulates intestinal immune tolerance.Citation123 These interactions also modulate inflammation and autoimmune diseases such as OA.Citation124
In recent years, increasing evidence has reported that dysregulation of the intestinal microbiota is strongly associated with the occurrence and development of RA. Intestinal microbiota dysregulation has also been observed in RA in human and animal studies.Citation120,Citation125 Significant changes in gut microbial communities were observed in RA model mice induced by collagen emulsion, mainly characterized by a decline in Bacteroidetes and an increase in Firmicutes and Proteobacteria. The model also resulted in an imbalance of 14 intestinal bacteria and considerable interference with metabolites involved in tryptophan, fatty acids and secondary bile acids.Citation125
In patients with RA, various lactobacillus and Prevotella genera have been found to be more abundant; therefore, increased quantities of Prevotella and imbalance of gut flora have been suggested as latent resources for the development of RA.Citation126 Dysregulation of gut microbiota in RA patients was correlated with the severity of RA; Euryarchaeota was directly correlated with the severity of RA, becoming an independent risk factor for the pathogenesis of RA.Citation127 There was a strong correlation between disease and the presence of Prevotella and the loss of Bacteroides in the fecal microbiota of untreated patients with new-onset RA. Bacterial rRNA was isolated from the synovial tissue of patients with RA.Citation120 The quantity and multiformity of Lactic acid bacteria in the gut microbiota of RA patients were significantly increased.Citation126,Citation127 This is consistent with the data that reported an increase in Lactobacilli in mice with collagen-induced arthritis.Citation128 Paradoxically, Lactobacillus acidophilus and casei seem to be conducive to the improvement of RA, indicating that different Lactobacillus subsets may have different effects on the pathogenesis of RA.Citation129
Intestinal opportunistic pathogenic bacteria, such as Prevotella, may participate in the formation of a pro-inflammatory immune state by enhancing the apoptotic mechanism and disrupting gut barrier integrity, thereby inducing the occurrence and maintenance of arthropathy inflammation.Citation130 In addition, Th17 cells promote osteoclast differentiation by producing a series of inflammatory factors, which are thought to be responsible for the osteodestructive phase of RA.Citation131 The reduction of Bacteroides, also found in RA mice, may promote a localized inflammatory environment by reducing Treg cell differentiation.Citation125
The occurrence of OA may be due to specific changes in the composition of intestinal microbiota, especially the increase of opportunistic pro-inflammatory bacteria and the significant decrease of commensal bacteria with anti-inflammatory properties, the increase of intestinal permeability, and then LPS causes inflammatory and immune responses, inducing the activation of cascade signaling pathways, leading to joint lesions and even pain, but the relevant evidence is limited. However, the study of RA related to immune inflammation is relatively deeper, and the diversity of intestinal flora is richer, but it also leads to the more complexity of research results and biological mechanisms, which needs further exploration of the rules and truth.
Effect of probiotics along with their secondary metabolites and its mechanism on musculoskeletal system
The concept of ‘probiotics’ was first coined in 1974, that is, ‘living microbes that have health benefits. Gut probiotics are beneficial for host health and body function.Citation132 Here, we summarize the effects and mechanisms of probiotics and their secondary metabolites on the musculoskeletal system.
Skeletal muscle
The gut is linked to skeletal muscle due to the activity of the gut microbiome and regulates muscle function by regulating systemic/tissue inflammation, insulin sensitivity, etc. Probiotics combat loss of muscle mass and function by improving the diversity of the gut microbiota.Citation23 In mouse cancer models, Lactobacillus reuteri is able to inhibit the development of cachexia and is implicated in the preservation of muscle mass.Citation133 Lactobacillus plantarum HY7715 ameliorates sarcopenia by improving skeletal muscle mass and function in aged Balb/c mice.Citation134 Lactobacillus plantarum TWK10 supplementation improves exercise performance and increases muscle mass in mice.Citation135 Lactobacillus paracasei PS23 decelerated age-related muscle loss by ensuring mitochondrial function in SAMP8 mice.Citation136 Restoring specific lactobacilli levels decreases inflammation and muscle atrophy markers in an acute leukemia mouse modelCitation137. Other experiments have also found that probiotics LC122, BL986 and LPPS23 can improve intestinal microflora imbalance and prevent age-related loss of muscle mass and strength in elderly mice.Citation136,Citation138 In a word, recent reports suggest that there are at least seven probiotics (Lactobacillus casei LC122 (LC122), Saccharomyces boulardii (SB), Lactobacillus paracasei PS23 (LPPS23), Bifidobacterium longum BL986 (BL986), Lactobacillus plantarum TWK10 (LP10), Lactobacillus salivarius SA-03 (SA-03) and Bifidobacterium longum OLP-01 (OLP-01)) is beneficial to skeletal muscle mass and strength in mice.Citation139 Among them, the most widely used strains are Lactobacillus and Bifidobacterium, which can improve muscle mass, strength and endurance loss.Citation23,Citation132,Citation139 Although recent studies have shown that probiotics that can limit sarcopenia or promote health performance in rodents, In anabolic situations, the effects of probiotics may target different signaling or metabolic pathways and tissues, such as lower inflammation and stress, maintenance of muscle protein synthesis, and improved muscle strength. However, the data obtained after supplementation of probiotics in the catabolic animal model showed that the effect on muscle mass and function was almost small, and only a certain anti-inflammatory effect was observed.Citation23
In humans, it has been found that ingesting specific probiotics can alter the gut microbiota in favor of increased skeletal muscle mass.Citation140 For example, a six-week intake of TWK10 can improve endurance performance on running tests in young adults.Citation141 Studies of supplementation with Lactobacillus plantarum have shown that probiotics improve exercise performance, endurance, as well as body composition, reducing fat mass and increasing muscle mass.Citation142 However, it has also been reported that, to a certain extent, probiotic supplementation in the elderly population can lead to beneficial changes in the gut microbiota, reducing pathogens and improving constipation, but the impact on host health is relatively small.Citation23 Fermented milk containing Lactobacillus and Bifidobacteria strains, as well as Lactobacillus casei, has also been reported to have no effect on muscle condition in the elderly.Citation143 These findings suggest that the mechanism of action of probiotics is complex and requires further investigation. Therefore, a recent meta-analysis suggested that probiotic supplementation enhanced muscle mass and strength, however, no beneficial effects were observed in terms of total lean body mass. The study suggests that it is important to explore differences in physiological mechanisms among different aging populations and to explore supplementing with appropriate probiotic strains for optimal muscle mass and strength.Citation144 From the above studies, it is not difficult to find that, the scarcity of studies, variability of populations, and low reproducibility make it difficult to find specific probiotic strains for optimizing muscle mass and function, and further studies in more defined populations are needed to design personalized probiotic interventions.Citation23
Probiotics reduce the ratio of Firmicutes to Bacteroidetes, which improves muscle mass, endurance and strength in mice.Citation145 The addition of probiotics LP10 and CP2998 can play a positive role in muscle by inhibiting glucocorticoid receptor activation and improving glucose.Citation135 Supplementation with LC122 and BL986 decreased the expression of the inflammatory cytokines TNF-α, IL-6 and IL-1β and improved muscle protein synthesis.Citation136,Citation138 Oral administration of B. breve and Lactobacillus rhamnosus significantly reduced the levels of the pro-inflammatory cytokines IL-2, IL-4, IL-6 and TNF-α in mice, thereby suppressing inflammatory responses.Citation146 LPPS23 also alleviated muscle inflammation by increasing IL-10 levels.Citation136 The expression of antioxidant stress factors, such as superoxide dismutase and glutathione peroxidase, was higher in the muscle of aged mice administered LPPS23.Citation136 Supplements containing LP10 and Lactobacillus reuteri were also found to improve muscle mass in mice, which was associated with reduced inflammation and muscle atrophy marker expression.Citation135,Citation137,Citation147 Long-term probiotics (such as L. reuteri) boost the leucine absorption ratio from whey protein to enhance protein absorption, improve the protein utilization rate and eventually promote muscle synthesis and increase muscle mass.Citation148
Therefore, regulating the composition of the intestinal flora is one of the mechanisms of action of probiotics, and the activity and diversity of the microbial community may be a determinant of healthy muscle under various pathological conditions.Citation23 The beneficial effects of probiotics also occur through various mechanisms, including the induction of immune regulation, resistance to physiological stress, inhibition of pathogens and improvement of the barrier function of intestinal epithelial cells.Citation132 The potential mechanisms by which probiotics regulate muscle mainly include energy metabolism of glucose, lipids and proteins; inflammatory levels; mitochondrial function and neuromuscular connectivity; and molecular pathways of muscle synthesis and catabolism metabolites.Citation139 Gut probiotics break down carbohydrates, lipids and proteins to provide energy to their hosts. They have also been shown to limit insulin resistance, regulate metabolic pathways, or inhibit oxidative stress and inflammation. In addition, probiotic-derived SCFAs and bile acids are involved in regulating host metabolism.Citation7,Citation149,Citation150
The intestinal microbiota can also decompose nutrients into secondary metabolites through distinct metabolic pathways, regulate intestinal immunity and metabolic homeostasis, and maintain symbiotic and parasitic relationships between the host and the intestinal microbiota.Citation151 The primary effects and mechanisms of action of these secondary metabolites on the skeletal muscle metabolism are discussed below.
SCFAs are metabolic products produced in response to dietary fiber fermentation by intestinal flora and primarily include butyrate, propionate and acetate.Citation152 SCFAs play an important role in glucose and lipid homeostasis, adjustment of inflammation, and connections between the gut and other distant tissues.Citation23 SCFAs play an important role in regulating intestinal environmental homeostasis, improving glucose metabolism, promoting calcium and phosphorus absorption, and alleviating oxidative stress and inflammatory responses, which are of great significance for regulating skeletal muscle functions.Citation153,Citation154 Skeletal muscle injury was partially reversed upon treatment with SCFAs in GF mice (SCFAs decreased atrogin-1 expression and increased MyoD and muscle mass and function), suggesting that SCFAs produced by the gut flora play a critical role in regulating skeletal muscle function.Citation26,Citation155 In a study of female C57BL/6 mice, atrophy of the hind limb muscle was completely or partially ameliorated in response to butyrate treatment, whereas the mass of the hind limb muscle was significantly lower in aging C57BL/6 female mice fed a regular diet.Citation156 Butyrate was shown to not only preserve muscle mass, but also improve glucose tolerance in mice, whereas it exerted no significant effect on insulin tolerance. In addition, butyrate increases the levels of the mitochondrial protein porin and mitochondrial transcription factor A and significantly improves mitochondrial function in skeletal muscle cells.Citation156,Citation157 Moreover, butyrate treatment reduces oxidative stress expression and markers of apoptosis in mice and alters the activity of antioxidant enzymes, thus preventing skeletal muscle damage caused by oxidative stress.Citation156 Butyrate is an important SCFA and histone deacetylase inhibitor that regulates age-related muscle loss. Butyrate has been shown to promote muscle synthesis by inhibiting histone deacetylase expression and improving muscle mass and cross-sectional area in aged mice.Citation156,Citation158 The addition of acetate can promote glucose absorption and glycogen generation in rabbit skeletal muscle and reduce intramuscular lipid production by increasing fatty acid mobilization and oxidation.Citation159 Additionally, SCFAs induce IGF-1 production, which promotes muscle anabolism.Citation40 These metabolic impacts of SCFAs may act directly on skeletal muscle or may be produced indirectly by stimulating glucagon-like peptide 1 (GLP-1) secretion. Other indirect effects of SCFAs on muscles include accelerated blood flow effects.Citation160
Tryptophan is an indispensable aromatic amino acid in humans. Metabolites rich in indole and indole derivatives produced during the decomposition of tryptophan by the intestinal microbiota play a critical role in maintaining homeostasis in the intestinal environment and the diversity of intestinal flora.Citation161 Indole metabolites derived from the intestinal microbiota can enhance body IL-10 levels, and IL-10 has an anti-inflammatory effect in regulating the inflammatory state of the host.Citation162 Evidence from other studies suggests that the tryptophan metabolite indole acrylic acid promotes intestinal barrier function and suppresses the inflammatory response by downregulating inflammation- and oxidative stress-related gene expression. The anti-inflammatory effects of tryptophan metabolites are particularly important when the body is in a chronic inflammatory state and muscle protein synthesis is limited.Citation163
Bile acids are small metabolic molecules that are produced in the liver and secreted into the intestine, and are involved in dietary lipid absorption. Intestinal flora can change the structure, bioavailability and bioactivity of bile acids, thus affecting bile acid metabolism and host metabolic homeostasis.Citation164 Intestinal anaerobes have been shown to transform primary bile acids into secondary bile acids.Citation165 The Lachnospiraceae family can also produce SCFAs and switch from primary bile acids to secondary bile acids.Citation166 Intestinal flora may be involved in bile acid metabolism and the FXR-FGF19 signaling pathway (Evidence suggests that FGF19 activation can inhibit the protein expression of muscle atrophy markers, enhance the molecules associated with myogenic differentiation, and regulate skeletal muscle protein balance,Citation83 which in turn affects skeletal muscle metabolism.Citation167,Citation168 Secondary bile acids can also enhance muscle function by contributing to lipid and glucose metabolism.Citation169
Vitamins are essential micronutrients for humans and the body cannot synthesize most of them. Experimental evidence has confirmed that intestinal probiotics promote the synthesis of vitamins in the body, which have a significant impact on skeletal muscle function. For example, Bifidobacterium and Lactobacillus have been shown to synthesize vitamin B groups (including folate, riboflavin and vitamin B12).Citation170 B-group vitamins are water-soluble vitamins directly involved in energy metabolism, and their deficiency has been reported to cause myocardial damage and heart failure.Citation171 Vitamin B12 deficiency increases homocysteine levels and causes muscle damage.Citation172
Bone
Previous studies have shown that probiotics have been found to benefit intestinal homeostasis and play a key role in the prevention and treatment of bone mass loss.Citation173 The upregulation of Lactobacillus in the gastrointestinal tract may be crucial for promoting the symbiosis of intestinal microbiota and intestinal barrier function, as Lactobacillus can produce effective bactericides and organic acids, which can inhibit pathogenic Escherichia coli.Citation24 The increased abundance of Lactobacillus and Lactococcus in the gut flora can effectively improve gut microbiota composition, thus promoting bone growth.Citation174 Supplements with L. reuteri can significantly improve any imbalance in intestinal microflora and enhance intestinal barrier function to prevent bone loss.Citation175 In a randomized controlled trial, postmenopausal women aged 75–80 years with low bone mass treated with a daily dose of L. reuteri, the proportion of bone mineral density loss after 12 months was obviously lower than that in the control group, suggesting that probiotics reduced bone mass loss.Citation176 Supplementation with B. vulgatus can also reduce LPS concentration to suppress the inflammatory response and prevent bone loss.Citation177 L. acidophilusCitation178 and Bacillus clausiiCitation179 can maintain bone homeostasis by balancing the levels of inflammatory cytokines.Citation24 B. longum has also been shown to prevent and treat osteoporosis, improve bone loss by enhancing osteoblast activity, and inhibiting osteoclast formation.Citation180 Additionally, probiotics can improve bone health by regulating mineral absorption. For example, B. longum can enhance bone density by improving the absorption of minerals, such as calcium, phosphate and magnesium.Citation181 L. reuteri has been confirmed to alleviate bone mass loss by inhibiting osteoclasts.Citation182
SCFAs also participate in bone metabolism and affect bone formation and resorption.Citation24 The protective effect of SCFAs on bone mass is related to the suppression of bone resorption. In terms of the underlying mechanism, butyrate and propionate induce the osteoclasts metabolic reprogramming, enhance glycolysis, downregulate critical osteoclast genes, and significantly reduce the number of osteoclasts, thereby inhibiting bone resorption. Therefore, SCFAs are effective adjusters of osteoclast metabolism and bone homeostasis, and play important roles in promoting bone formation.Citation183 In a study in mice, butyrate was found to promote bone anabolism and increase bone mass by mobilizing the Wnt signaling pathway in osteoblasts.Citation110 Moreover, butyrate can protect osteoblast precursor cells from damage induced by hydrogen peroxide and promote mineralization and differentiation of osteoblasts. It primarily maintains the balance of bone metabolism by enhancing the activity of cellular antioxidant enzymes, promoting ATP production, and reducing ROS levels.Citation184 Additionally, SCFAs induce IGF-1 production. IGF-1, in addition to promoting skeletal muscle function, and plays a crucial role in bone metabolism. IGF-1 participates in bone formation and absorption and regulates the balance of bone metabolism.Citation40 Thus, SCFAs play a critical role in maintaining homeostasis during bone metabolism.
Tryptophan metabolites are closely related to bone metabolism. Kynurenine, a tryptophan metabolite, is the first stable metabolite formed after the enzymatic degradation of tryptophan.Citation185 The key function of kynurenine in bone metabolism appears to be the acceleration of bone loss and the mediation of adverse effects on the bone. Kynurenine content increases during aging, and its adverse effect on bone may be due to its effect on osteoclast activation, resulting in increased bone fragility and an imbalance in bone remodeling.Citation186 Other studies have shown that increased kynurenine levels accelerate bone aging by impairing osteoblast differentiation and increasing osteoclast resorption.Citation187
Low vitamin B12 levels can also inhibit osteoblast activity, thus increasing osteoporosis risk and even inducing fracture.Citation188 Probiotics, such as lactic acid bacteria and Bifidobacteria play a critical role in promoting the formation of riboflavin and folic acid.Citation170,Citation189 Riboflavin and folic acid are important for promoting vitamin synthesis and regulating the inflammatory response. Studies have shown that riboflavin plays an anti-inflammatory role to an extent, and its intake inhibits TNF-α release in rat leukocytes.Citation190 Riboflavin also regulates oxidative stress as a cofactor for antioxidant enzymes.Citation191 Folic acid has been implicated in regulating insulin resistance and suppressing the pro-inflammatory cytokines IL-6, IL-8 and TNF-α.Citation192 It is not difficult to find that the functions of vitamin B group such as anti-inflammatory, antioxidant and participating in energy metabolism are indispensable in the maintenance of skeletal health.Citation86 The absorption of calcium and vitamin D is particularly crucial for healthy bone maintenance, probiotics reduce intestinal PH and improve calcium absorption to enhance bone function.Citation193
Secondary bile acids also modulate bone homeostasis by regulating signal transduction between osteoblasts and osteoclasts.Citation194 In addition, secondary bile acids induce the production of GLP-1, which regulates glucose homeostasis and stimulates osteoblast differentiationCitation24,Citation195 and can further enhance the functions of the skeletal system.
Joint
In a rat model of OA, the probiotic complex attenuated the development of osteoarthritis by inhibiting pro-inflammatory cytokines and cartilage destruction.Citation196 In a human trial, 537 OA patients were randomized to either L. casei or a placebo, and systemic inflammation was significantly lowered after 6 months in the experimental group compared to that in the control group.Citation197 Oral intake of Streptococcus thermophilus improves OA degeneration.Citation198 Oral administration of Clostridium butyricum can effectively preserve knee cartilage and synovium in OA rats, significantly reduce the amount of fibrous tissue, and significantly reduce the serum concentrations of various inflammatory and metabolic markers of bone and cartilage.Citation199 Lactobacillus casei alleviates joint inflammatory damage by downregulating pro-inflammatory cytokines.Citation200
Probiotics have recently been added to the list of treatment agents for joint inflammation, as L. casei was shown to inhibit joint swelling, reduce RA, and prevent bone destruction in rats with joint inflammation.Citation201 Lactobacillus acidophilus and Lactobacillus casei are also commonly used as relievers to manage RA.Citation129 It has also been suggested that the availability of probiotic supplements to relieve RA-related outcomes is still weak, and the impact of probiotics on RA appears to be flora specific.Citation202
SCFAs can reduce arthritis severity by regulating IL-10 secretion from Treg cells.Citation203 Butyric acid produced by Lactobacillus inhibits OA by controlling chondrocyte autophagy and inflammatory cell death.Citation204 Probiotic-derived butyrate has been shown to inhibit mouse arthritis by influencing T and B cell progression.Citation205
As for probiotics and their secondary metabolites, current research results are limited. However, its anti-inflammatory and immunomodulatory properties warrant further investigation to determine its roles and biological mechanisms in the musculoskeletal system. In summary, we present the primary mechanisms discussed above in concise form in . We also summarize the findings of related studies published in recent years on the influence of common intestinal microbiota on the musculoskeletal system, as shown in .
Figure 1. Intestinal microbiota and the metabolites they produce play a crucial role in the musculoskeletal system. Probiotics promote short-chain fatty acids (SCFAs) production, which enhance the function of the musculoskeletal system by improving energy metabolism, promoting IGF-1 production, and inhibiting inflammation and oxidative stress. Vitamin B promotes musculoskeletal development by reducing inflammation and oxidative stress, whereas vitamin D enhances bone function by promoting bone formation. Indoles promote musculoskeletal development by reducing inflammation. Prebiotics enhance the function of probiotics function. However, in addition to the positive effects, imbalances in the intestinal microbiota can trigger a series of negative effects. First, an imbalance in intestinal permeability promotes the increase in the ROS and LPS levels in circulation and causes oxidative stress and inflammation, which damages the musculoskeletal systems and joints. Second, harmful bacteria can activate the inflammatory response and suppress musculoskeletal function. Kynurenine can enhance osteoclast function, increase bone resorption, and promote bone loss. Created with BioRender.com.
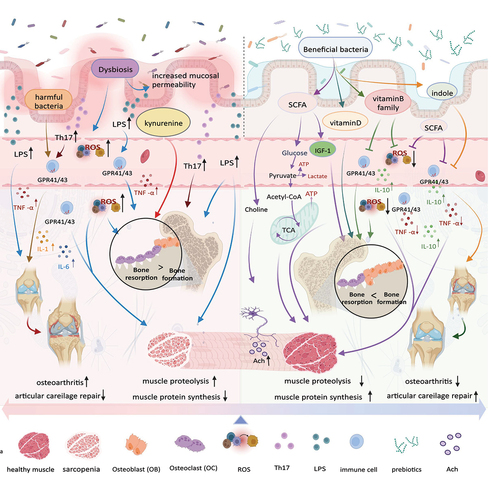
Table 1. The influence of common intestinal microbiota on the musculoskeletal system.
Effective intervention of musculoskeletal health based on intestinal microbiota
Intestinal microbiota is closely related to the normal metabolism of the musculoskeletal system. Appropriate interventions can be adopted to promote the optimal regulatory role of intestinal probiotics ().
Figure 2. The intestinal microbiota is generally divided into three categories: probiotic, detrimental bacteria, and conditioned bacteria. Different lifestyles exert varied effects on the intestinal microbiota. Aging, the decreased secretion of sex hormones, high-fat diet intake, high-protein diet intake, and a sedentary lifestyle or over exercising can exert negative impacts on the intestinal microbiota. These cause an increase in the proportion of detrimental bacteria, inducing mucosal permeability and triggering a series of negative reactions. Meanwhile, a healthy diet, moderate exercise, photobiological regulation, supplementation with prebiotics and probiotics, vitamin intake, Ca2+, sex hormones, and melatonin can promote positive changes in the intestinal microbiota, thereby enhancing musculoskeletal system function. Created with BioRender.com.
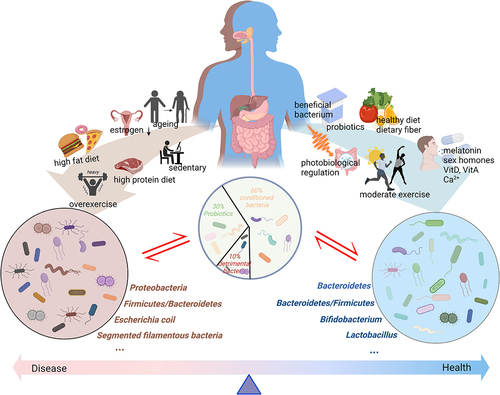
Supplementation with prebiotics, vitamins D and dietary calcium promotes musculoskeletal system health
Prebiotics are nutrients that are degraded by intestinal flora and support symbiote survival.Citation213 Prebiotics are indigestible dietary fibers that are generally considered a type of dietary fiber. The intestinal microbiota can utilize prebiotics to rebuild the bacteria community.Citation139 These provide beneficial physiological effects on the host musculoskeletal system by selectively stimulating the activity or growth of certain intestinal microbial strains. Lactobacillus and Bifidobacterium are common target bacteria for prebiotics, and inulin and oligosaccharides have been widely studied as prebiotics.Citation189 Prebiotics improve intestinal barrier function and host immunity and reduce the abundance of potentially pathogenic bacteria such as Clostridium. Prebiotic supplements have been shown to promote positive changes in the skeletal muscles. Delzenne et al. showed that circulating LPS levels and inflammation decreased and muscle mass increased in mice fed prebiotic fiber oligosaccharides.Citation214 In addition, prebiotic supplementation improved Bifidobacterium and Lactobacillus levels and prebiotic supplementation also affected the proportion of Bacteroidetes and Firmicutes.Citation215 Concurrently, prebiotics, as a type of dietary fiber, can also promote the generation of SCFAs.Citation189
Vitamin D is a key factor in increasing bone mass. Besides directly affecting calcium absorption, vitamin D also regulates intestinal mucosal homeostasis by maintaining the integrity of the intestinal barrier, which affects immune system function and inflammatory responses.Citation216,Citation217 Vitamin D improves homeostasis of the intestinal microbiome and facilitates muscle anabolismCitation218. Vitamin D can inhibit the levels of pro-inflammatory factors and promote the anti-inflammatory factors secretion of body.Citation219,Citation220 Findings from specific studies suggest that vitamin D supplements can regulate gut flora and increase its diversity in women. In addition to the Bacteroidetes to Firmicutes ratio, the relative abundances of Akkermansia and Bifidobacterium were increased in response to vitamin D supplementation.Citation221 Vitamin A has been shown to exhibit a similar function in maintaining the intestinal barrier function and regulating immune responses and bacterial diversity to maintain intestinal homeostasis.Citation222 It has also been reported that vitamin D supplementation did not improve any sarcopenia index in older people in the community and may impair some aspects of bodily function. More experimental evidence is needed to clarify the role of Vitamin D.Citation223 Therefore, the evidence from clinical trials needs to be strengthened.
Calcium is the most common mineral in the body, and its intake is related to the healthy development of bones. Calcium is also the most important element in maintaining the normal excitatory and contractile functions of skeletal muscles. Dietary calcium intake can cause changes in the gut microbiota. For example, mice fed with dietary calcium showed a significant increase in intestinal microbiota diversity and higher levels of Bifidobacterium, Bacteroides, Ruminococcaceae and Akkermansia.Citation224,Citation225 In addition, dietary calcium appears to have a protective effect on the gut barrier, increasing buffering capacity and promoting bone mass by increasing the absorption of dietary calcium in the host body.Citation224 In summary, dietary calcium regulates the intestinal microbiota, establishes cross-dialogue with the host, promotes metabolism, and promotes musculoskeletal health.
Diet regulates intestinal microbiota homeostasis and promotes musculoskeletal health
The intestinal microbiota has recently been defined as a “sensor” of host nutritional signals. The type and diversity of food consumed are closely associated with the composition of gut microbiota.Citation226 Evidence from previous studies has shown that long-term changes in dietary patterns can induce changes in intestinal flora.Citation227 Short-term dietary modifications can also lead to variations in the gut microbiota. For example, after switching from a plant-based diet to an animal-based diet, the concentrations of acetate and butyrate in the intestinal tract decreases significantly.Citation228 Additionally, an animal-based diet increased Bacteroidetes and decreased Firmicutes by.Citation229
Skeletal muscle mass is affected by muscle protein synthesis and breakdown, and changes in the intestinal microbiota with age are also affected by dietary protein intake.Citation230 In addition, the intake of a protein-rich diet is positively correlated with the diversity of gut microbiota and can improve the abundance of Bacteroidetes in the gut microbiota.Citation231 Notably, long-term high-protein diets do not always exert positive effects on muscles, and the long-term administration of beef protein supplements to endurance athletes was shown to decrease the abundance of beneficial intestinal bacteria such as Bifidobacteria.Citation232 Moreover, compounds produced by fermentation of undigested protein residues in the colon exert potential negative effects on intestinal, immune and metabolic functions.Citation233
Studies of high-fat diet mice have shown that the diet increases body weight and inflammatory marker expression and decreases glucose tolerance;Citation234 more importantly, the circulating levels of LPS in high-fat diet-fed mice increased by two or three times, which led to an increase in intestinal permeability, thus triggering an inflammatory response that damages muscle mass.Citation235 High-fat diet obesity caused by intake may also lead to excessive production of ROS and oxidative stress responses in the body and an accompanying increase in the adipokine population and TNF-α expression, thus increasing the chronic inflammatory response in the body and affecting skeletal muscle function.Citation236 Moreover, high-fat diets can reduce SCFAs production and increase the proportion of Proteobacteria.Citation229
In addition, higher carbohydrate intake was closely associated with a decreased diversity of intestinal flora. Although Bifidobacterium content increased, Lactobacillus and Streptococcus contents decreased. Coffee, tea and red wine are rich in polyphenols, which are associated with prebiotic abundance and Bifidobacterium activity.Citation237 Dietary polyphenols can increase the bloom of SCFA-producing bacteria and inhibit the growth of LPS-producing bacteria, thus regulating the gut microbiotaCitation238 and affecting musculoskeletal system health. The intake of a Mediterranean diet helps maintain healthy gut flora because the diet incorporates a balanced intake of high-quality protein and complex carbohydrates and higher levels of fiber and polyphenols.Citation20 Therefore, a healthy diet can improve the relative proportion of probiotics and regulate inflammation, whereas an unhealthy diet can lead to dysregulation of the intestinal flora, oxidative stress, inflammation, and other adverse effects, ultimately damaging the health of the musculoskeletal system.
Positive effects of exercise on the intestinal microbiota promote musculoskeletal health
Moderate exercise can also improve the muscles, bones and joints. Our findings showed that exercise intervention can effectively improve muscle mass and function in elderly people aged >60 years, and the effect of exercise is significantly greater than that of nutritional supplements.Citation239 Exercise can also effectively increase the mineral density of bone, improve bone strength, and reduce the risk of bone mass loss and incidence of falls and fractures.Citation240 Similarly, exercise can relieve pain, enhance joint function, and improve the quality of life of people with osteoarthritis.Citation241
Exercise can also improve musculoskeletal health by improving the intestinal microbiota. Exercise has been found to promote positive changes in intestinal microbiota, thus improving musculoskeletal function in both human and animal experiments. High-intensity training prevented obesity-related intestinal microbiome dysregulation and maintained intestinal microbiota diversity in high fat diet-induced obese mice. The Bacteroides to Firmicutes ratio in non-obese mice also changes after exercise, and the abundance of Bacteroides increases significantly after exercise.Citation242 In a study on exercise and diet in obese rats, exercise exerted a stronger and more stable effect on the intestinal microbiota over time and could more effectively promote intestinal mucosal integrity and metabolic function.Citation243
Similar results have been obtained from human studies on the relationship between exercise and gut microbiota. The relative abundance of the gut microbiota was significantly higher in elite athletes who underwent intensive training than in sedentary adults.Citation231 Professional rugby players have a higher diversity of intestinal microbiota than nonprofessional athletes, and the intestinal microbiota of professional athletes primarily includes 22 phyla of bacteria, whereas the low body mass index (BMI) and high-BMI control groups only have 11 and 9 phyla of bacteria, respectively. Professional athletes also had lower levels of inflammatory cytokines than nonprofessional athletes.Citation244 Additionally, research has shown that the intestinal microbiota (Villanella) can also affect athletic performance by converting lactic acid produced during exercise into propionic acid to prolong running time and improve athletic performance.Citation245
The equilibrium between Bacteroidetes and Firmicutes in humans during aerobic exercise is critical for health maintenance, and disruption of the balance in bacterial colonization in the gut can lead to inflammation and metabolic or neurological diseases.Citation246 A Japanese study of aerobic exercise intervention in the intestinal microbiota of older women showed that a 12-week aerobic exercise program not only increased the relative abundance of Bacteroides but also improved cardiorespiratory health. Concurrently, the abundance of Bacteroides was remarkably higher in subjects who increased their brisk walking time by >20 min.Citation247 Therefore, moderate exercise can enhance musculoskeletal function by improving the composition of the gut microbiota, enhancing gut mucosal function, suppressing inflammatory responses, and maintaining a variety of intestinal flora.Citation248
The potential effects of exercise on gut microbiota mediate the process of osteoarthritis.Citation249 In an animal study, researchers administered rats with a high-sugar, high-fat diet along with aerobic exercise, prebiotics, and a combination of both and found that the combination of the two interventions completely prevented knee injury in obese rats.Citation250 Interestingly, another study showed that a combination of aerobic exercise and prebiotics improved metabolic disorders in obese rats but did not improve preexisting osteoarthritis damage in the knee.Citation251 Therefore, further research is required for a thorough investigation.
Excessive exercise may limit muscle formation by promoting inflammation and nutritional limitations as well as oxidative and metabolic stress. Other negative effects of overtraining include intestinal ischemia, increased intestinal barrier permeability, and oxidative stress, which lead to an imbalance in the intestinal microecology, an increase in inflammatory responses, increased catabolism, and deterioration of muscle function.Citation252 Regular training appears to be associated with better biodiversity and beneficial effects on intestinal microbiota.Citation253 Evidence from certain studies supports the idea that training for exhaustion may be linked to harmful microbial consequences.Citation254,Citation255 Therefore, the influence of exercise on the intestinal flora may depend on the intensity and duration of exercise.
The effects of supplementation with estrogen and melatonin on the intestinal microbiota promotes musculoskeletal health
The ratio of gut bacteria to human cells differs between genders, with women having a higher ratio than men. The ratio of bacteria to human cells was 1.3 for men and 2.2 for womenCitation256. Women also have a higher diversity of gut microbes. Akkermansia muciniphila is especially abundant in females. Premenopausal women had a higher ratio of Firmicutes/Bacteroidetes, and relatively more abundant Lachnospira and Roseburia than postmenopausal women. The relative abundance of Prevotella, Parasubacter and Bilophila were lower in premenopausal women than in postmenopausal women, as well as lower IL-6 and monocyte chemoattractant protein-1 plasma levels, which represent inflammation levels.Citation257 This suggests that estrogen may affect the regulation of intestinal microbial homeostasis and immunity.Citation258 Conversely, an imbalance in the intestinal flora also affects estrogen activity. Reportedly, a significant decline in estrogen levels after menopause can cause damage to the gut barrier and bone health.Citation216 As previously mentioned, the gut microbiota regulates estrogen by secreting β-glucosidase, and when this process is impaired by dysregulation of the gut flora (which is characterized by reduced microbial multiformity), it results in a decrease in circulating estrogen, which affects bone metabolism.Citation113 It has been reported that non-ovarian estrogen is influenced more by the gut microbiome,Citation259 which may be one of the reasons why postmenopausal women are more prone to OP. Therefore, the maintenance of intestinal homeostasis is essential for the normal secretion of estrogen and the balance of bone metabolism. Although the interaction between estrogen and intestinal microbiota has not been fully described, it is strongly suggested that the intervention of estrogen in intestinal microbiota may promote musculoskeletal health; however, direct evidence is still needed.
A recent review provides the latest evidence on how sleep disorders are associated with and contribute to changes in gut microbiome composition across the life cycle, offering an insight into the causes of sarcopenia through sleep disorders. It is thought that the loss of musculoskeletal health is related to sleep disorders. Meanwhile, in the older adults, shorter sleep duration was associated with an increase in pro-inflammatory bacteria, whereas improved sleep quality was positively correlated with an increase in beneficial Warts microbacteria and Flatcoccus. In young adults, the effect of sleep disruption on gut microbial composition, particularly the ratio of beneficial Firmicutes to Bacteroidetes, remains paradoxical and unclear. This study would not only deepen the understanding of the diverse contributing factors to sarcopenia but also offer a more comprehensive view of this complex condition. When sleep is poor, gut microbiome composition often changes, which may mediate the pro-inflammatory state between sleep disorders and sarcopenia.Citation260 These findings not only suggested the important role of gut microbiota in the correlation between sleep quality and sarcopenia, but also implied that hormones regulating sleep, such as melatonin, may be one of the effective intervention targets.
Melatonin is another endogenous hormone that regulates sleep and circadian rhythms and exhibits antiaging, anti-inflammatory and antioxidant properties. Recently, it has been found to be a safe dietary supplement for disease treatment and skeletal muscle quality improvement.Citation261 Melatonin reduces oxidative stress and inflammation and protects skeletal muscles from oxidative damage.Citation262 Additionally, it can improve muscle mitochondrial function during aging.Citation263 Studies in the elderly have also shown a significant correlation between melatonin levels and muscle strength.Citation264 Interestingly, SD mice displayed weakened intestinal flora and a limited number of probiotic species, such as Akkermansia, Bacteroides and Faecalibacterium.Citation265 Interestingly, melatonin treatment reversed this abnormal microbiome composition. Melatonin has also recently been shown to improve the intestinal microbiota in animals and humans. Oral melatonin supplementation can reduce lipid accumulation, reverse gut microbiota imbalance, and improve the diversity of the intestinal flora. SCFAs levels were significantly lowered in the intestinal tract of high fat diet-fed mice but were restored after melatonin supplementation.Citation266 In addition, melatonin supplementation in high-fat diet-fed mice can effectively improve the intestinal ecological imbalance, and melatonin can change the Firmicutes to Bacteroidetes ratio and enhance intestinal mucosal function in obese mice. Meanwhile, melatonin supplementation alleviated insulin resistance caused by low-grade inflammation and high-fat diet intake in mice.Citation267 Melatonin has been shown to regulate insulin sensitivityCitation268 and thus has a critical effect in maintaining glucose homeostasis and regulating glucose metabolism. Melatonin is involved in bone metabolism. Melatonin can also be used to treat inflammatory osteolysis via the inhibition of osteoclast formation through the activation of the nuclear factor erythroid 2-related factor 2 (Nrf2)/catalase signaling pathway.Citation269 Therefore, we can infer that melatonin can enhance the function of the gut mucosa, improve lipid and glucose metabolism, and promote the generation of SCFAs by regulating the dysregulation of the intestinal microbiota, thus eventually enhancing the function of the musculoskeletal system.
Photobiomodulation (PBM) of the intestinal microbiota promotes musculoskeletal health and other interventions
As a topical treatment, PBM is used clinically for various conditions, including muscle fatigue, joint and tendon inflammation and wound and fracture healing.Citation270 Recently, a close relationship has been reported between PBM and intestinal microbiota. After PBM was administered via irradiation to the abdomen of healthy mice, the gut microbiota of mice changed significantly, and the diversity of intestinal flora also increased significantly. The effect was most pronounced in mice treated with red light three times a week, but not in mice subjected to a single red-light treatmentCitation271. NIR light exerted a more significant effect than red light. After NIR irradiation, the ratio of probiotics in the intestinal microbiota of mice increased significantly, and the treatment also regulated the abundance of bacteria related to the imbalance in intestinal microbiota; this effect could be attributed to the anti-inflammatory and redox signaling effects of PBM on the intestinal microbiota.Citation270 Ultraviolet radiation has also been shown to affect the intestinal microbiota structure and function in rat models of bone loss.Citation272 In addition to its anti-inflammatory effects, UV radiation may also regulate bone metabolism by inducing vitamin D synthesis and intestinal calcium absorption, thereby promoting bone formation, decreasing bone absorption, and enhancing bone mineral density.Citation273 This evidence suggests that although there is not much direct evidence, PBM still shows a potential role in maintaining musculoskeletal system homeostasis by regulating the imbalance of intestinal flora. PBM has the potential to act as adjunct therapy (along with diet and exercise) to balance the microbiome and promote musculoskeletal health.
Lifestyle interventions including exercise, electroacupuncture, and supplementation with probiotics have a direct impact on the gut microbiome, altering its composition and function, which opens up an innovative avenue for new treatment opportunities for patients with a wide range of chronic diseases. Based on a large number of relevant literatures, recent studies have combed through the role of lifestyle intervention in regulating intestinal flora and thereby interfering with chronic pain, and found that direct evidence is still relatively less, but it is confirmed that these methods can improve pain and quality of life to a certain extentCitation274.
Future directions and clinical implications
The correlation between the modifying effects of the intestinal microbiota and musculoskeletal health is not well understood. In addition to the damage caused by the cascade of inflammation induced by endotoxins and oxidative stress, whether the intestinal flora will also secrete other bioactive substances, such as isoenzymes,Citation275 to further interfere with the musculoskeletal health of the host had not been deeply explored. Moreover, the application of non-drug treatments, such as exercise intervention, to promote the health of the intestinal flora and musculoskeletal system, and the reasonable selection of exercise intensity and duration and other parameters need to be explored further. In addition, methods for identifying and targeting the key microbiota contributing to musculoskeletal health, improving the functions of these microbial strains and their metabolites, and constructing a clearer microbial-musculoskeletal system interaction model are essential for future investigations.Citation144 Furthermore, the combined application of various reasonable intervention methods constitutes a meaningful exploration.
Recent systematic reviews have shown that most of the experimental results in animal trials support the direct improvement of musculoskeletal health through interventions such as nutritional regulation, probiotics supplementation, and fecal bacteria transplantation. The relevant mechanisms discussed in this paper are also confirmed. In contrast, clinical studies are currently few and the results obtained are not consistent, especially the lack of high-quality clinical studiesCitation139. The reason may be closely related to the difference of flora and individual differences of population.Citation144 Personalized treatment for intestinal flora regulation is both difficult and promising for the treatment of musculoskeletal system diseases.
In the clinical studies of fecal transplantation, the existing meta-analysis summarizing the review of the therapeutic effect of fecal transplantation on 85 diseases found that the most studies were conducted in infectious diseases and intestinal diseases, and very few studies directly related to musculoskeletal health. However, the paper also clearly points out the therapeutic value and vision of fecal bacteria transplantation in chronic low-grade inflammation-related diseases (such as diabetes and obesity), which needs to be confirmed by more rigorous clinical studies with larger samples.Citation276 This will bring hope for the treatment of musculoskeletal system diseases by fecal bacteria transplantation.
Nevertheless, the gut microbiota has a profound impact on musculoskeletal health. The current clinical application has just begun, considering the complexity of the microflora and individual differences, whether it is suitable for the screening of bacteria to promote musculoskeletal health, or individual treatment based on human fecal bacteria transplantation; Whether it is the means and methods of intestinal microecological intervention, or the effect of intestinal microecological intervention, it still needs a lot of clinical evidence to support, which needs joint efforts to achieve.
Conclusion
In conclusion, the potential mechanisms by which the gut microbiota regulates musculoskeletal health include protein, energy, blood lipids, glucose metabolism, inflammation levels, neuromuscular connectivity, and mitochondrial function. The composition and metabolic variation of the intestinal microbiota may affect the functions of the musculoskeletal system. Imbalances in the intestinal microbiota increase the levels of pro-inflammatory factors, activate oxidative stress pathways, and reduce muscle mass, and affect bone formation and absorption. The positive effects of intestinal probiotics on the musculoskeletal system are primarily mediated through direct or indirect effects that promote muscle synthesis and balance in the bone metabolism. In addition, intestinal probiotics can reduce inflammation and oxidative stress.
Based on the mechanism of action of the intestinal microbiota in the musculoskeletal system, different interventions, such as probiotics, prebiotics, vitamins and dietary calcium, can be used to improve the composition and metabolism of the gut microbiota and enhance musculoskeletal system function. Lifestyle interventions including exercise have a direct impact on the gut microbiome, altering its composition and function, which opens up an innovative avenue for new treatment opportunities for patients with a wide range of chronic diseases. Recent studies have combed through the role of lifestyle intervention in regulating intestinal flora and found that direct evidence is still relatively less. Furthermore, emerging approaches, such as estrogen and melatonin supplementation, and photobiological regulation, have shown potential in the regulation of intestinal microbiota and promotion of musculoskeletal health, especially when used in combination. In the current mechanism and application research, although there is many evidence in animal experiments and clinical research, there is a lack of higher quality clinical research, especially applied research.
List of acronyms
Acronym | = | The full name |
AIA | = | Adjuvant induced arthritis |
ATP | = | Adenosine triphosphate |
atrogin-1 | = | muscle atrophy F-box |
BMI | = | Body mass index |
CFU | = | Colony-forming units |
GF | = | Germ-free |
GLP-1 | = | glucagon-like peptide 1 |
hs-CRP | = | hypersensitive-c-reactive-protein |
IBD | = | Inflammatory bowel disease |
IFN-γ | = | Interferon gamma |
IGF-1 | = | Insulin-like growth factor 1 |
IGF-1R | = | Insulin-like growth factor 1 receptor |
IL-1 | = | Interleukin 1 |
IL-2 | = | Interleukin 2 |
IL-4 | = | Interleukin 4 |
IL-6 | = | Interleukin 6 |
IL-8 | = | Interleukin 8 |
IL-10 | = | Interleukin 10 |
IL-17 | = | Interleukin 17 |
LPS | = | Lipopolysaccharide |
mTOR | = | muscle target of rapamycin |
mTORC1 | = | muscle target of rapamycin complex 1 |
Murf-1 | = | Muscle ring finger protein 1 |
MyoD | = | myogenic regulatory factors D |
NIR | = | Near infrared |
NF-κB | = | nuclear factor-κb |
Nrf2 | = | Nuclear factor erythroid 2-related factor 2 |
OA | = | Osteoarthritis |
OBs | = | osteoclasts |
OCLs | = | osteoclasts |
OP | = | Osteoporosis |
PBM | = | Photobiomodulation |
PF | = | Pathogen- free |
RA | = | Rheumatoid arthritis |
RANKL | = | receptor activator of NF-κB ligand |
RNA | = | Ribonucleic Acid |
ROS | = | Reactive oxygen species |
SCFA | = | Short-chain fatty acids |
Th-17 | = | T helper cell 17 |
TLR | = | Toll like receptor |
TNF-α | = | Tumor necrosis factor alpha |
UV | = | Ultraviolet |
5-HT | = | 5-hydroxytryptamine |
Disclosure statement
No potential conflict of interest was reported by the author(s).
Additional information
Funding
References
- Petermann-Rocha F, Balntzi V, Gray SR, Lara J, Ho FK, Pell JP, Celis‐Morales C. Global prevalence of sarcopenia and severe sarcopenia: a systematic review and meta-analysis. J Cachexia Sarcopenia Muscle. 2022;13(1):86–35. doi:10.1002/jcsm.12783.
- Ayers C, Kansagara D, Lazur B, Fu R, Kwon A, Harrod C. Effectiveness and safety of treatments to prevent fractures in people with low bone mass or primary osteoporosis: a living systematic review and network meta-analysis for the American college of physicians. Ann Intern Med. 2023;176(2):182–195. doi:10.7326/M22-0684.
- Wei J, Zhang Y, Hunter D, Zeng C, Lei G. The gut microbiome-joint axis in osteoarthritis. Sci Bull (Beijing). 2023;68(8):759–762. doi:10.1016/j.scib.2023.03.024.
- Dey P, Chaudhuri SR, Efferth T, Pal S. The intestinal 3M (microbiota, metabolism, metabolome) zeitgeist - from fundamentals to future challenges. Free Radic Biol Med. 2021;176:265–285. doi:10.1016/j.freeradbiomed.2021.09.026.
- Gilbert JA, Blaser MJ, Caporaso JG, Jansson JK, Lynch SV, Knight R. Current understanding of the human microbiome. Nat Med. 2018;24(4):392–400. doi:10.1038/nm.4517.
- Turnbaugh PJ, Ley RE, Hamady M, Fraser-Liggett CM, Knight R, Gordon JI. The human microbiome project. Nature. 2007;449(7164):804–810. doi:10.1038/nature06244.
- Fan Y, Pedersen O. Gut microbiota in human metabolic health and disease. Nat Rev Microbiol. 2021;19(1):55–71. doi:10.1038/s41579-020-0433-9.
- Delzenne NM, Neyrinck AM, Backhed F, Cani PD. Targeting gut microbiota in obesity: effects of prebiotics and probiotics. Nat Rev Endocrinol. 2011;7(11):639–646. doi:10.1038/nrendo.2011.126.
- Ma Q, Li Y, Li P, Wang M, Wang J, Tang Z, Wang T, Luo L, Wang C, Wang T, et al. Research progress in the relationship between type 2 diabetes mellitus and intestinal flora. Biomed Pharmacother. 2019;117:109138. doi:10.1016/j.biopha.2019.109138.
- Lynch SV, Pedersen O, Phimister EG. The human intestinal microbiome in health and disease. N Engl J Med. 2016;375(24):2369–2379. doi:10.1056/NEJMra1600266.
- Ridaura V, Belkaid Y. Gut microbiota: the link to your second brain. Cell. 2015;161(2):193–194. doi:10.1016/j.cell.2015.03.033.
- Chen Y, Zhou J, Wang L. Role and mechanism of gut microbiota in human disease. Front Cell Infect Microbiol. 2021;11:625913. doi:10.3389/fcimb.2021.625913.
- Dinan TG, Cryan JF. Brain-gut-microbiota axis and mental health. Psychosom Med. 2017;79(8):920–926. doi:10.1097/PSY.0000000000000519.
- Hu X, Wang T, Jin F. Alzheimer’s disease and gut microbiota. Sci China Life Sci. 2016;59(10):1006–1023. doi:10.1007/s11427-016-5083-9.
- Witkowski M, Weeks TL, Hazen SL. Gut microbiota and cardiovascular disease. Circ Res. 2020;127(4):553–570. doi:10.1161/CIRCRESAHA.120.316242.
- Qin J, Li Y, Cai Z, Li S, Zhu J, Zhang F, Liang S, Zhang W, Guan Y, Shen D, et al. A metagenome-wide association study of gut microbiota in type 2 diabetes. Nature. 2012;490(7418):55–60. doi:10.1038/nature11450.
- Moludi J, Maleki V, Jafari-Vayghyan H, Vaghef-Mehrabany E, Alizadeh M. Metabolic endotoxemia and cardiovascular disease: a systematic review about potential roles of prebiotics and probiotics. Clin Exp Pharmacol Physiol. 2020;47(6):927–939. doi:10.1111/1440-1681.13250.
- Koh A, De Vadder F, Kovatcheva-Datchary P, Backhed F. From dietary fiber to host physiology: short-chain fatty acids as key bacterial metabolites. Cell. 2016;165(6):1332–1345. doi:10.1016/j.cell.2016.05.041.
- Bana B, Cabreiro F. The microbiome and aging. Annu Rev Genet. 2019;53(1):239–261. doi:10.1146/annurev-genet-112618-043650.
- Strasser B, Wolters M, Weyh C, Kruger K, Ticinesi A. The effects of lifestyle and diet on gut microbiota composition, inflammation and muscle performance in our aging society. Nutrients. 2021;13(6):2045. doi:10.3390/nu13062045.
- Ticinesi A, Lauretani F, Milani C, Nouvenne A, Tana C, Del Rio D, Maggio M, Ventura M, Meschi T. Aging gut microbiota at the cross-road between Nutrition, physical frailty, and sarcopenia: is there a gut–muscle axis? Nutrients. 2017;9(12):1303. doi:10.3390/nu9121303.
- Zaiss MM, Jones RM, Schett G, Pacifici R. The gut-bone axis: how bacterial metabolites bridge the distance. J Clin Invest. 2019;129(8):3018–3028. doi:10.1172/JCI128521.
- Giron M, Thomas M, Dardevet D, Chassard C, Savary-Auzeloux I. Gut microbes and muscle function: can probiotics make our muscles stronger? J Cachexia Sarcopenia Muscle. 2022;13(3):1460–1476. doi:10.1002/jcsm.12964.
- Behera J, Ison J, Tyagi SC, Tyagi N. The role of gut microbiota in bone homeostasis. Bone. 2020;135:115317. doi:10.1016/j.bone.2020.115317.
- Biver E, Berenbaum F, Valdes AM, Araujo de Carvalho I, Bindels LB, Brandi ML, Calder PC, Castronovo V, Cavalier E, Cherubini A, et al. Gut microbiota and osteoarthritis management: an expert consensus of the European society for clinical and economic aspects of osteoporosis, osteoarthritis and musculoskeletal diseases (ESCEO). Ageing Res Rev. 2019;55:100946. doi:10.1016/j.arr.2019.100946.
- Lahiri S, Kim H, Garcia-Perez I, Reza MM, Martin KA, Kundu P, Cox LM, Selkrig J, Posma JM, Zhang H, et al. The gut microbiota influences skeletal muscle mass and function in mice. Sci Transl Med. 2019;11(502):11. doi:10.1126/scitranslmed.aan5662.
- Tintignac LA, Lagirand J, Batonnet S, Sirri V, Leibovitch MP, Leibovitch SA. Degradation of MyoD mediated by the SCF (MAFbx) ubiquitin ligase. J Biol Chem. 2005;280(4):2847–2856. doi:10.1074/jbc.M411346200.
- Yoshida T, Delafontaine P. Mechanisms of IGF-1-Mediated regulation of skeletal muscle hypertrophy and atrophy. Cells. 2020;9(9):1970. doi:10.3390/cells9091970.
- Waddell DS, Baehr LM, van den Brandt J, Johnsen SA, Reichardt HM, Furlow JD, Bodine SC. The glucocorticoid receptor and FOXO1 synergistically activate the skeletal muscle atrophy-associated MuRF1 gene. Am J Physiol Endocrinol Metab. 2008;295(4):E785–97. doi:10.1152/ajpendo.00646.2007.
- Woldt E, Sebti Y, Solt LA, Duhem C, Lancel S, Eeckhoute J, Hesselink MKC, Paquet C, Delhaye S, Shin Y, et al. Rev-erb-α modulates skeletal muscle oxidative capacity by regulating mitochondrial biogenesis and autophagy. Nat Med. 2013;19(8):1039–1046. doi:10.1038/nm.3213.
- Goodpaster BH, Sparks LM. Metabolic flexibility in health and disease. Cell Metab. 2017;25(5):1027–1036. doi:10.1016/j.cmet.2017.04.015.
- Jope RS, Jenden DJ. The utilization of choline and acetyl coenzyme a for the synthesis of acetylcholine. J Neurochem. 1980;35(2):318–325. doi:10.1111/j.1471-4159.1980.tb06267.x.
- Fambrough DM. Control of acetylcholine receptors in skeletal muscle. Physiol Rev. 1979;59(1):165–227. doi:10.1152/physrev.1979.59.1.165.
- Gomes AV, Potter JD, Szczesna-Cordary D. The role of troponins in muscle contraction. IUBMB Life (Int Union Biochem Mol Biol: Life). 2002;54(6):323–333. doi:10.1080/15216540216037.
- Ohlsson C, Sjogren K. Effects of the gut microbiota on bone mass. Trends Endocrinol Metab. 2015;26(2):69–74. doi:10.1016/j.tem.2014.11.004.
- Evans L. Bone: gut immune status affects bone mass in mice. Nat Rev Endocrinol. 2012;8(6):318. doi:10.1038/nrendo.2012.51.
- Sjogren K, Engdahl C, Henning P, Lerner UH, Tremaroli V, Lagerquist MK, Bäckhed F, Ohlsson C. The gut microbiota regulates bone mass in mice. J Bone Miner Res. 2012;27(6):1357–1367. doi:10.1002/jbmr.1588.
- Quach D, Collins F, Parameswaran N, McCabe L, Britton RA, Ellermeier CD. Microbiota reconstitution does not cause bone loss in germ-free mice. mSphere. 2018;3(1):3. doi:10.1128/mSphereDirect.00545-17.
- Schwarzer M, Makki K, Storelli G, Machuca-Gayet I, Srutkova D, Hermanova P, Martino ME, Balmand S, Hudcovic T, Heddi A, et al. Lactobacillus plantarum strain maintains growth of infant mice during chronic undernutrition. Sci. 2016;351(6275):854–857. doi:10.1126/science.aad8588.
- Yan J, Herzog JW, Tsang K, Brennan CA, Bower MA, Garrett WS, Sartor BR, Aliprantis AO, Charles JF. Gut microbiota induce IGF-1 and promote bone formation and growth. Proc Natl Acad Sci U S A. 2016;113(47):E7554–E63. doi:10.1073/pnas.1607235113.
- Holick MF. Vitamin D deficiency. N Engl J Med. 2007;357(3):266–281. doi:10.1056/NEJMra070553.
- Bora SA, Kennett MJ, Smith PB, Patterson AD, Cantorna MT. The gut microbiota regulates endocrine vitamin D metabolism through fibroblast growth factor 23. Front Immunol. 2018;9:408. doi:10.3389/fimmu.2018.00408.
- Liu JH, Chen CY, Liu ZZ, Luo ZW, Rao SS, Jin L, Wan T-F, Yue T, Tan Y-J, Yin H, et al. Extracellular vesicles from child gut microbiota enter into bone to preserve bone mass and strength. Adv Sci. 2021;8(9):2004831. doi:10.1002/advs.202004831.
- Liu H, Zhang H, Han Y, Hu Y, Geng Z, Su J. Bacterial extracellular vesicles-based therapeutic strategies for bone and soft tissue tumors therapy. Theranostics. 2022;12(15):6576–6594. doi:10.7150/thno.78034.
- Huang Z, Chen J, Li B, Zeng B, Chou CH, Zheng X, Xie J, Li H, Hao Y, Chen G, et al. Faecal microbiota transplantation from metabolically compromised human donors accelerates osteoarthritis in mice. Ann Rheum Dis. 2020;79(5):646–656. doi:10.1136/annrheumdis-2019-216471.
- Abdollahi-Roodsaz S, Joosten LA, Roelofs MF, Radstake TR, Matera G, Popa C, van der Meer JWM, Netea MG, van den Berg WB. Inhibition of Toll-like receptor 4 breaks the inflammatory loop in autoimmune destructive arthritis. Arthritis & Rheumatism. 2007;56(9):2957–2967. doi:10.1002/art.22848.
- van den Berg WB, van Lent PL, Joosten LA, Abdollahi-Roodsaz S, Koenders MI. Amplifying elements of arthritis and joint destruction. Ann Rheum Dis. 2007;66(Suppl 3):iii45–8. doi:10.1136/ard.2007.079830.
- Ulici V, Kelley KL, Azcarate-Peril MA, Cleveland RJ, Sartor RB, Schwartz TA, Loeser RF. Osteoarthritis induced by destabilization of the medial meniscus is reduced in germ-free mice. Osteoarthritis Cartilage. 2018;26(8):1098–1109. doi:10.1016/j.joca.2018.05.016.
- Levy M, Kolodziejczyk AA, Thaiss CA, Elinav E. Dysbiosis and the immune system. Nat Rev Immunol. 2017;17(4):219–232. doi:10.1038/nri.2017.7.
- Haran JP, Bucci V, Dutta P, Ward D, McCormick B. The nursing home elder microbiome stability and associations with age, frailty, nutrition and physical location. J Med Microbiol. 2018;67(1):40–51. doi:10.1099/jmm.0.000640.
- Gupta SS, Mohammed MH, Ghosh TS, Kanungo S, Nair GB, Mande SS. Metagenome of the gut of a malnourished child. Gut Pathog. 2011;3(1):7. doi:10.1186/1757-4749-3-7.
- Genton L, Mareschal J, Charretier Y, Lazarevic V, Bindels LB, Schrenzel J. Targeting the gut microbiota to treat cachexia. Front Cell Infect Microbiol. 2019;9:305. doi:10.3389/fcimb.2019.00305.
- Jackson MA, Jeffery IB, Beaumont M, Bell JT, Clark AG, Ley RE, O’Toole PW, Spector TD, Steves CJ. Signatures of early frailty in the gut microbiota. Genome Med. 2016;8(1):8. doi:10.1186/s13073-016-0262-7.
- Claesson MJ, Jeffery IB, Conde S, Power SE, O’Connor EM, Cusack S, Harris HMB, Coakley M, Lakshminarayanan B, O’Sullivan O, et al. Gut microbiota composition correlates with diet and health in the elderly. Nature. 2012;488(7410):178–184. doi:10.1038/nature11319.
- Zhang L, Liao J, Chen Q, Chen M, Kuang Y, Chen L, He W. Characterization of the gut microbiota in frail elderly patients. Aging Clin Exp Res. 2020;32(10):2001–2011. doi:10.1007/s40520-019-01385-2.
- Illiano P, Brambilla R, Parolini C. The mutual interplay of gut microbiota, diet and human disease. FEBS J. 2020;287(5):833–855. doi:10.1111/febs.15217.
- Nardone OM, de Sire R, Petito V, Testa A, Villani G, Scaldaferri F, Castiglione F. Inflammatory bowel diseases and sarcopenia: the role of inflammation and gut microbiota in the development of muscle failure. Front Immunol. 2021;12:694217. doi:10.3389/fimmu.2021.694217.
- Grosicki GJ, Fielding RA, Lustgarten MS. Gut microbiota contribute to age-related changes in skeletal muscle size, composition, and function: biological basis for a gut-muscle axis. Calcif Tissue Int. 2018;102(4):433–442. doi:10.1007/s00223-017-0345-5.
- Human Microbiome Project C. Structure, function and diversity of the healthy human microbiome. Nature. 2012;486:207–214.
- Wang H, Wang G, Banerjee N, Liang Y, Du X, Boor PJ, Hoffman KL, Khan MF. Aberrant gut microbiome contributes to intestinal oxidative stress, barrier dysfunction, inflammation and systemic autoimmune responses in MRL/lpr mice. Front Immunol. 2021;12:651191. doi:10.3389/fimmu.2021.651191.
- Callahan LA, Supinski GS. Sepsis-induced myopathy. Crit Care Med. 2009;37:S354–67. doi:10.1097/CCM.0b013e3181b6e439.
- Powers SK, Jackson MJ. Exercise-induced oxidative stress: cellular mechanisms and impact on muscle force production. Physiol Rev. 2008;88(4):1243–1276. doi:10.1152/physrev.00031.2007.
- Saul D, Kosinsky RL. Dextran Sodium Sulfate-induced colitis as a model for sarcopenia in mice. Inflamm Bowel Dis. 2020;26(1):56–65. doi:10.1093/ibd/izz127.
- Kishimoto Y, Zhu W, Hosoda W, Sen JM, Mattson MP. Chronic mild gut inflammation accelerates brain neuropathology and motor dysfunction in α-synuclein mutant mice. Neuromolecular Med. 2019;21(3):239–249. doi:10.1007/s12017-019-08539-5.
- Puleo F, Meirelles K, Navaratnarajah M, Fitzpatrick L, Shumate ML, Cooney RN, Lang CH. Skeletal muscle catabolism in trinitrobenzene sulfonic acid–induced murine colitis. Metabolism. 2010;59(11):1680–1690. doi:10.1016/j.metabol.2010.03.021.
- Ryan E, McNicholas D, Creavin B, Kelly ME, Walsh T, Beddy D. Sarcopenia and inflammatory bowel disease: a systematic review. Inflamm Bowel Dis. 2019;25(1):67–73. doi:10.1093/ibd/izy212.
- Scaldaferri F, Pizzoferrato M, Lopetuso LR, Musca T, Ingravalle F, Sicignano LL, Mentella M, Miggiano G, Mele MC, Gaetani E, et al. Nutrition and IBD: malnutrition and/or sarcopenia? A practical guide. Gastroenterology Research And Practice. 2017;2017:1–11. doi:10.1155/2017/8646495.
- Pizzoferrato M, de Sire R, Ingravalle F, Mentella MC, Petito V, Martone AM, Landi F, Miggiano GAD, Mele MC, Lopetuso LR, et al. Characterization of sarcopenia in an IBD population attending an Italian gastroenterology tertiary center. Nutrients. 2019;11(10):11. doi:10.3390/nu11102281.
- de Sire R, Rizzatti G, Ingravalle F, Pizzoferrato M, Petito V, Lopetuso L, Graziani C, de Sire A, Mentella MC, Mele MC, et al. Skeletal muscle-gut axis: emerging mechanisms of sarcopenia for intestinal and extra intestinal diseases. Minerva Gastroenterol Dietol. 2018;64(4):351–362. doi:10.23736/S1121-421X.18.02511-4.
- Maffei VJ, Kim S, Blanchard E, Luo M, Jazwinski SM, Taylor CM, Welsh DA. Biological aging and the human gut microbiota. J Gerontol A Biol Sci Med Sci. 2017;72(11):1474–1482. doi:10.1093/gerona/glx042.
- Ticinesi A, Nouvenne A, Cerundolo N, Catania P, Prati B, Tana C, Meschi T. Gut microbiota, muscle mass and function in aging: a focus on physical frailty and sarcopenia. Nutrients. 2019;11(7):11. doi:10.3390/nu11071633.
- Thevaranjan N, Puchta A, Schulz C, Naidoo A, Szamosi JC, Verschoor CP, Loukov D, Schenck LP, Jury J, Foley KP, et al. Age-associated microbial dysbiosis promotes intestinal permeability, systemic inflammation, and macrophage dysfunction. Cell Host & Microbe. 2017;21(4):455–466.e4. doi:10.1016/j.chom.2017.03.002.
- Ghosh S, Lertwattanarak R, Garduno Jde J, Galeana JJ, Li J, Zamarripa F, Lancaster JL, Mohan S, Hussey S, Musi N, et al. Elevated muscle TLR4 expression and metabolic endotoxemia in human aging. J Gerontol A Biol Sci Med Sci. 2015;70(2):232–246. doi:10.1093/gerona/glu067.
- Cevenini E, Caruso C, Candore G, Capri M, Nuzzo D, Duro G, Rizzo C, Colonna-Romano G, Lio D, Carlo D, et al. Age-related inflammation: the contribution of different organs, tissues and systems. How to face it for therapeutic approaches. Curr Pharm Des. 2010;16(6):609–618. doi:10.2174/138161210790883840.
- Jensen GL. Inflammation: roles in aging and sarcopenia. JPEN J Parenter Enteral Nutr. 2008;32(6):656–659. doi:10.1177/0148607108324585.
- Li YP, Chen Y, John J, Moylan J, Jin B, Mann DL, Reid MB. TNF-α acts via p38 MAPK to stimulate expression of the ubiquitin ligase atrogin1/MAFbx in skeletal muscle. FASEB J. 2005;19(3):362–370. doi:10.1096/fj.04-2364com.
- Cuthbertson D, Smith K, Babraj J, Leese G, Waddell T, Atherton P, Wackerhage H, Taylor PM, Rennie MJ. Anabolic signaling deficits underlie amino acid resistance of wasting, aging muscle. FASEB J. 2005;19(3):422–424. doi:10.1096/fj.04-2640fje.
- Patel HP, Al-Shanti N, Davies LC, Barton SJ, Grounds MD, Tellam RL, Stewart CE, Cooper C, Sayer AA. Lean mass, muscle strength and gene expression in community dwelling older men: findings from the Hertfordshire Sarcopenia Study (HSS). Calcif Tissue Int. 2014;95(4):308–316. doi:10.1007/s00223-014-9894-z.
- Giannos P, Prokopidis K, Isanejad M, Wright HL. Markers of immune dysregulation in response to the ageing gut: insights from aged murine gut microbiota transplants. BMC Gastroenterol. 2022;22(1):533. doi:10.1186/s12876-022-02613-2.
- De Spiegeleer A, Wynendaele E, Descamps A, Debunne N, Braeckman BP, De Mey M, Coudenys J, Crombez L, Verbeke F, Janssens Y, et al. The bacterial quorum sensing peptide iAM373 is a novel inducer of sarcopenia. Clin Transl Med. 2022;12(10):e1053. doi:10.1002/ctm2.1053.
- Ticinesi A, Mancabelli L, Tagliaferri S, Nouvenne A, Milani C, Del Rio D, Lauretani F, Maggio MG, Ventura M, Meschi T, et al. The gut-muscle axis in older Subjects with low muscle mass and performance: a proof of concept study exploring fecal microbiota composition and function with shotgun metagenomics sequencing. Int J Mol Sci. 2020;21(23):21. doi:10.3390/ijms21238946.
- Kang L, Li P, Wang D, Wang T, Hao D, Qu X. Alterations in intestinal microbiota diversity, composition, and function in patients with sarcopenia. Sci Rep. 2021;11(1):4628. doi:10.1038/s41598-021-84031-0.
- Mancin L, Wu GD, Paoli A. Gut microbiota–bile acid–skeletal muscle axis. Trends Microbiol. 2022;31(3):322. doi:10.1016/j.tim.2023.01.003.
- Zmora N, Suez J, Elinav E. You are what you eat: diet, health and the gut microbiota. Nat Rev Gastroenterol Hepatol. 2019;16(1):35–56. doi:10.1038/s41575-018-0061-2.
- Charles JF, Ermann J, Aliprantis AO. The intestinal microbiome and skeletal fitness: connecting bugs and bones. Clin Immunol. 2015;159(2):163–169. doi:10.1016/j.clim.2015.03.019.
- Chen Y, Wang X, Zhang C, Liu Z, Li C, Ren Z. Gut microbiota and bone diseases: a growing partnership. Front Microbiol. 2022;13:877776. doi:10.3389/fmicb.2022.877776.
- Guss JD, Horsfield MW, Fontenele FF, Sandoval TN, Luna M, Apoorva F, Lima SF, Bicalho RC, Singh A, Ley RE, et al. Alterations to the gut microbiome impair bone strength and tissue material properties. J Bone Miner Res. 2017;32(6):1343–1353. doi:10.1002/jbmr.3114.
- Guss JD, Taylor E, Rouse Z, Roubert S, Higgins CH, Thomas CJ, Baker SP, Vashishth D, Donnelly E, Shea MK, et al. The microbial metagenome and bone tissue composition in mice with microbiome-induced reductions in bone strength. Bone. 2019;127:146–154. doi:10.1016/j.bone.2019.06.010.
- Yu M, Pal S, Paterson CW, Li JY, Tyagi AM, Adams J, Coopersmith CM, Weitzmann MN, Pacifici R. Ovariectomy induces bone loss via microbial-dependent trafficking of intestinal TNF+ T cells and Th17 cells. J Clin Invest. 2021;131(4):131. doi:10.1172/JCI143137.
- Cheng S, Qi X, Ma M, Zhang L, Cheng B, Liang C, Liu L, Li P, Kafle OP, Wen Y, et al. Assessing the relationship between gut microbiota and bone mineral density. Front Genet. 2020;11:6. doi:10.3389/fgene.2020.00006.
- Ni JJ, Yang XL, Zhang H, Xu Q, Wei XT, Feng GJ, Zhao M, Pei Y-F, Zhang L. Assessing causal relationship from gut microbiota to heel bone mineral density. Bone. 2021;143:115652. doi:10.1016/j.bone.2020.115652.
- Mitsui T, Shimaoka K, Takagi C, Goto Y, Kagami H, Ito A. Small bowel bacterial overgrowth may not affect bone mineral density in older people. Clin Nutr. 2005;24(6):920–924. doi:10.1016/j.clnu.2005.05.022.
- Compston JE, Judd D, Crawley EO, Evans WD, Evans C, Church HA, Reid EM, Rhodes J. Osteoporosis in patients with inflammatory bowel disease. Gut. 1987;28(4):410–415. doi:10.1136/gut.28.4.410.
- Semeao EJ, Jawad AF, Stouffer NO, Zemel BS, Piccoli DA, Stallings VA. Risk factors for low bone mineral density in children and young adults with Crohn’s disease. J Pediatr. 1999;135(5):593–600. doi:10.1016/S0022-3476(99)70058-2.
- Li C, Huang Q, Yang R, Dai Y, Zeng Y, Tao L, Li X, Zeng J, Wang Q. Gut microbiota composition and bone mineral loss—epidemiologic evidence from individuals in Wuhan, China. Osteoporos Int. 2019;30(5):1003–1013. doi:10.1007/s00198-019-04855-5.
- Qin J, Li R, Raes J, Arumugam M, Burgdorf KS, Manichanh C, Nielsen T, Pons N, Levenez F, Yamada T, et al. A human gut microbial gene catalogue established by metagenomic sequencing. Nature. 2010;464(7285):59–65. doi:10.1038/nature08821.
- Eckburg PB, Bik EM, Bernstein CN, Purdom E, Dethlefsen L, Sargent M, Gill SR, Nelson KE, Relman DA. Diversity of the human intestinal microbial flora. Sci. 2005;308(5728):1635–1638. doi:10.1126/science.1110591.
- Wang J, Wang Y, Gao W, Wang B, Zhao H, Zeng Y, Ji Y, Hao D. Diversity analysis of gut microbiota in osteoporosis and osteopenia patients. PeerJ. 2017;5:e3450. doi:10.7717/peerj.3450.
- Das M, Cronin O, Keohane DM, Cormac EM, Nugent H, Nugent M, Molloy C, O’Toole PW, Shanahan F, Molloy MG, et al. Gut microbiota alterations associated with reduced bone mineral density in older adults. Rheumatology (Oxford). 2019;58(12):2295–2304. doi:10.1093/rheumatology/kez302.
- Xu Z, Xie Z, Sun J, Huang S, Chen Y, Li C, Sun X, Xia B, Tian L, Guo C, et al. Gut microbiome reveals specific dysbiosis in primary osteoporosis. Front Cell Infect Microbiol. 2020;10:160. doi:10.3389/fcimb.2020.00160.
- DeLuca HF. Evolution of our understanding of vitamin D. Nutr Rev. 2008;66:S73–87. doi:10.1111/j.1753-4887.2008.00105.x.
- Yatsonsky Ii D, Pan K, Shendge VB, Liu J, Ebraheim NA. Linkage of microbiota and osteoporosis: a mini literature review. World J Orthop. 2019;10(3):123–127. doi:10.5312/wjo.v10.i3.123.
- Smith BJ, Lerner MR, Bu SY, Lucas EA, Hanas JS, Lightfoot SA, Postier RG, Bronze MS, Brackett DJ. Systemic bone loss and induction of coronary vessel disease in a rat model of chronic inflammation. Bone. 2006;38(3):378–386. doi:10.1016/j.bone.2005.09.008.
- Chongwatpol P, Rendina-Ruedy E, Stoecker BJ, Clarke SL, Lucas EA, Smith BJ. Implications of compromised zinc status on bone loss associated with chronic inflammation in C57BL/6 mice. J Inflamm Res. 2015;8:117–128. doi:10.2147/JIR.S82261.
- Quach D, Britton RA. Gut microbiota and Bone Health. Adv Exp Med Biol. 2017;1033:47–58.
- Belkaid Y, Hand TW. Role of the microbiota in immunity and inflammation. Cell. 2014;157(1):121–141. doi:10.1016/j.cell.2014.03.011.
- Kong YY, Feige U, Sarosi I, Bolon B, Tafuri A, Morony S, Capparelli C, Li J, Elliott R, McCabe S, et al. Activated T cells regulate bone loss and joint destruction in adjuvant arthritis through osteoprotegerin ligand. Nature. 1999;402(6759):304–309. doi:10.1038/46303.
- D’Amelio P, Grimaldi A, Di Bella S, Brianza SZM, Cristofaro MA, Tamone C, Giribaldi G, Ulliers D, Pescarmona GP, Isaia G, et al. Estrogen deficiency increases osteoclastogenesis up-regulating T cells activity: a key mechanism in osteoporosis. Bone. 2008;43(1):92–100. doi:10.1016/j.bone.2008.02.017.
- Ibanez L, Abou-Ezzi G, Ciucci T, Amiot V, Belaid N, Obino D, Mansour A, Rouleau M, Wakkach A, Blin-Wakkach C, et al. Inflammatory osteoclasts prime TNFα-producing CD4+T cells and express CX 3 CR1. J Bone Miner Res. 2016;31(10):1899–1908. doi:10.1002/jbmr.2868.
- Tyagi AM, Yu M, Darby TM, Vaccaro C, Li JY, Owens JA, Hsu E, Adams J, Weitzmann MN, Jones RM, et al. The microbial metabolite butyrate stimulates bone formation via T regulatory cell-mediated regulation of WNT10B expression. Immunity. 2018;49(6):1116–1131.e7. doi:10.1016/j.immuni.2018.10.013.
- Pawlak D, Domaniewski T, Znorko B, Oksztulska-Kolanek E, Lipowicz P, Doroszko M, Karbowska M, Pawlak K. The impact of peripheral serotonin on leptin-brain serotonin axis, bone metabolism and strength in growing rats with experimental chronic kidney disease. Bone. 2017;105:1–10. doi:10.1016/j.bone.2017.08.004.
- Shen S, Prame Kumar K, Wen SW, Shim R, Wanrooy BJ, Stanley D, Moore RJ, Van TTH, Robert R, Hickey MJ, et al. Deficiency of dietary fiber modulates gut microbiota composition, neutrophil recruitment and worsens experimental colitis. Front Immunol. 2021;12:619366. doi:10.3389/fimmu.2021.619366.
- Baker JM, Al-Nakkash L, Herbst-Kralovetz MM. Estrogen-gut microbiome axis: physiological and clinical implications. Maturitas. 2017;103:45–53. doi:10.1016/j.maturitas.2017.06.025.
- Guan Z, Jia J, Zhang C, Sun T, Zhang W, Yuan W, Leng H, Song C. Gut microbiome dysbiosis alleviates the progression of osteoarthritis in mice. Clin Sci (Lond). 2020;134(23):3159–3174. doi:10.1042/CS20201224.
- Jackson MA, Verdi S, Maxan ME, Shin CM, Zierer J, Bowyer RCE, Martin T, Williams FMK, Menni C, Bell JT, et al. Gut microbiota associations with common diseases and prescription medications in a population-based cohort. Nat Commun. 2018;9(1):2655. doi:10.1038/s41467-018-05184-7.
- Boer CG, Radjabzadeh D, Medina-Gomez C, Garmaeva S, Schiphof D, Arp P, Koet T, Kurilshikov A, Fu J, Ikram MA, et al. Intestinal microbiome composition and its relation to joint pain and inflammation. Nat Commun. 2019;10(1):4881. doi:10.1038/s41467-019-12873-4.
- Sanchez Romero EA, Melendez Oliva E, Alonso Perez JL, Martin Perez S, Turroni S, Marchese L, Villafañe JH. Relationship between the gut microbiome and osteoarthritis pain: review of the literature. Nutrients. 2021;13(3):716. doi:10.3390/nu13030716.
- Santoro A, Ostan R, Candela M, Biagi E, Brigidi P, Capri M, Franceschi C. Gut microbiota changes in the extreme decades of human life: a focus on centenarians. Cell Mol Life Sci. 2018;75(1):129–148. doi:10.1007/s00018-017-2674-y.
- Kayama H, Okumura R, Takeda K. Interaction between the microbiota, Epithelia, and immune cells in the intestine. Annu Rev Immunol. 2020;38(1):23–48. doi:10.1146/annurev-immunol-070119-115104.
- Van de Wiele T, Van Praet JT, Marzorati M, Drennan MB, Elewaut D. How the microbiota shapes rheumatic diseases. Nat Rev Rheumatol. 2016;12(7):398–411. doi:10.1038/nrrheum.2016.85.
- Boutagy NE, McMillan RP, Frisard MI, Hulver MW. Metabolic endotoxemia with obesity: is it real and is it relevant? Biochimie. 2016;124:11–20. doi:10.1016/j.biochi.2015.06.020.
- Huang Z, Kraus VB. Does lipopolysaccharide-mediated inflammation have a role in OA? Nat Rev Rheumatol. 2016;12(2):123–129. doi:10.1038/nrrheum.2015.158.
- Tanoue T, Atarashi K, Honda K. Development and maintenance of intestinal regulatory T cells. Nat Rev Immunol. 2016;16(5):295–309. doi:10.1038/nri.2016.36.
- Opazo MC, Ortega-Rocha EM, Coronado-Arrazola I, Bonifaz LC, Boudin H, Neunlist M, Bueno SM, Kalergis AM, Riedel CA. Intestinal microbiota influences non-intestinal related autoimmune diseases. Front Microbiol. 2018;9:432. doi:10.3389/fmicb.2018.00432.
- Zaiss MM, Joyce Wu HJ, Mauro D, Schett G, Ciccia F. The gut–joint axis in rheumatoid arthritis. Nat Rev Rheumatol. 2021;17(4):224–237. doi:10.1038/s41584-021-00585-3.
- Liu X, Zou Q, Zeng B, Fang Y, Wei H. Analysis of fecal Lactobacillus community structure in patients with early rheumatoid arthritis. Curr Microbiol. 2013;67(2):170–176. doi:10.1007/s00284-013-0338-1.
- Picchianti-Diamanti A, Panebianco C, Salemi S, Sorgi ML, Di Rosa R, Tropea A, Sgrulletti M, Salerno G, Terracciano F, D’Amelio R, et al. Analysis of gut microbiota in rheumatoid arthritis patients: disease-related dysbiosis and modifications induced by Etanercept. Int J Mol Sci. 2018;19(10):2938. doi:10.3390/ijms19102938.
- Liu X, Zeng B, Zhang J, Li W, Mou F, Wang H, Zou Q, Zhong B, Wu L, Wei H, et al. Role of the gut microbiome in modulating arthritis progression in mice. Sci Rep. 2016;6(1):30594. doi:10.1038/srep30594.
- Alipour B, Homayouni-Rad A, Vaghef-Mehrabany E, Sharif SK, Vaghef-Mehrabany L, Asghari-Jafarabadi M, Nakhjavani MR, Mohtadi‐Nia J. Effects of Lactobacillus casei supplementation on disease activity and inflammatory cytokines in rheumatoid arthritis patients: a randomized double-blind clinical trial. Int J Rheum Dis. 2014;17(5):519–527. doi:10.1111/1756-185X.12333.
- Kalinkovich A, Livshits G. A cross talk between dysbiosis and gut-associated immune system governs the development of inflammatory arthropathies. Semin Arthritis Rheum. 2019;49(3):474–484. doi:10.1016/j.semarthrit.2019.05.007.
- Sato K, Suematsu A, Okamoto K, Yamaguchi A, Morishita Y, Kadono Y, Tanaka S, Kodama T, Akira S, Iwakura Y, et al. Th17 functions as an osteoclastogenic helper T cell subset that links T cell activation and bone destruction. J Exp Med. 2006;203(12):2673–2682. doi:10.1084/jem.20061775.
- Suez J, Zmora N, Segal E, Elinav E. The pros, cons, and many unknowns of probiotics. Nat Med. 2019;25(5):716–729. doi:10.1038/s41591-019-0439-x.
- Varian BJ, Gourishetti S, Poutahidis T, Lakritz JR, Levkovich T, Kwok C, Teliousis K, Ibrahim YM, Mirabal S, Erdman SE, et al. Beneficial bacteria inhibit cachexia. Oncotarget. 2016;7(11):11803–11816. doi:10.18632/oncotarget.7730.
- Lee K, Kim J, Park SD, Shim JJ, Lee JL. Lactobacillus plantarum HY7715 ameliorates sarcopenia by improving skeletal muscle mass and function in aged Balb/c mice. Int J Mol Sci. 2021;22(18):22. doi:10.3390/ijms221810023.
- Chen YM, Wei L, Chiu YS, Hsu YJ, Tsai TY, Wang MF, Huang C-C. Lactobacillus plantarum TWK10 supplementation improves exercise performance and increases muscle mass in mice. Nutrients. 2016;8(4):205. doi:10.3390/nu8040205.
- Chen LH, Huang SY, Huang KC, Hsu CC, Yang KC, Li LA, Chan C-H, Huang H-Y. Lactobacillus paracasei PS23 decelerated age-related muscle loss by ensuring mitochondrial function in SAMP8 mice. Aging (Albany NY). 2019;11(2):756–770. doi:10.18632/aging.101782.
- Bindels LB, Beck R, Schakman O, Martin JC, De Backer F, Sohet FM, Dewulf EM, Pachikian BD, Neyrinck AM, Thissen J-P, et al. Restoring specific lactobacilli levels decreases inflammation and muscle atrophy markers in an acute leukemia mouse model. PloS One. 2012;7(6):e37971. doi:10.1371/journal.pone.0037971.
- Ni Y, Yang X, Zheng L, Wang Z, Wu L, Jiang J, Yang T, Ma L, Fu Z. Lactobacillus and Bifidobacterium improves physiological function and cognitive ability in aged mice by the regulation of gut microbiota. Mol Nutr Food Res. 2019;63(22):e1900603. doi:10.1002/mnfr.201900603.
- Liu C, Cheung WH, Li J, Chow SK, Yu J, Wong SH, Ip M, Sung JJY, Wong RMY. Understanding the gut microbiota and sarcopenia: a systematic review. J Cachexia Sarcopenia Muscle. 2021;12(6):1393–1407. doi:10.1002/jcsm.12784.
- Daily JW, Park S. Sarcopenia is a cause and consequence of metabolic dysregulation in aging humans: effects of gut dysbiosis, glucose dysregulation, diet and lifestyle. Cells. 2022;11(3):11. doi:10.3390/cells11030338.
- Huang WC, Hsu YJ, Li H, Kan NW, Chen YM, Lin JS, Hsu, TK, Tsai, TY, Chiu, YS, Huang, CC. Effect of Lactobacillus plantarum TWK10 on improving endurance performance in humans. Chin J Physiol. 2018;61(3):163–170. doi:10.4077/CJP.2018.BAH587.
- Huang WC, Lee MC, Lee CC, Ng KS, Hsu YJ, Tsai TY, Young S-L, Lin J-S, Huang C-C. Effect of Lactobacillus plantarum TWK10 on exercise physiological adaptation, performance, and body composition in healthy humans. Nutrients. 2019;11(11):11. doi:10.3390/nu11112836.
- Neto JV, de Melo CM, Ribeiro SM. Effects of three-month intake of synbiotic on inflammation and body composition in the elderly: a pilot study. Nutrients. 2013;5(4):1276–1286. doi:10.3390/nu5041276.
- Prokopidis K, Giannos P, Kirwan R, Ispoglou T, Galli F, Witard OC, Triantafyllidis KK, Kechagias KS, Morwani‐Mangnani J, Ticinesi A, et al. Impact of probiotics on muscle mass, muscle strength and lean mass: a systematic review and meta-analysis of randomized controlled trials. J Cachexia Sarcopenia Muscle. 2023;14(1):30–44. doi:10.1002/jcsm.13132.
- Hsu YJ, Huang WC, Lin JS, Chen YM, Ho ST, Huang CC, Tung Y-T. Kefir supplementation modifies gut microbiota composition, reduces physical fatigue, and improves exercise performance in mice. Nutrients. 2018;10(7):10. doi:10.3390/nu10070862.
- Sagar S, Morgan ME, Chen S, Vos AP, Garssen J, van Bergenhenegouwen J, Boon L, Georgiou NA, Kraneveld AD, Folkerts G, et al. Bifidobacterium breve and Lactobacillus rhamnosus treatment is as effective as budesonide at reducing inflammation in a murine model for chronic asthma. Respir Res. 2014;15(1):46. doi:10.1186/1465-9921-15-46.
- Bindels LB, Neyrinck AM, Claus SP, Le Roy CI, Grangette C, Pot B, Martinez I, Walter J, Cani PD, Delzenne NM, et al. Synbiotic approach restores intestinal homeostasis and prolongs survival in leukaemic mice with cachexia. ISME J. 2016;10(6):1456–1470. doi:10.1038/ismej.2015.209.
- Maathuis AJ, Keller D, Farmer S. Survival and metabolic activity of the GanedenBC30 strain of Bacillus coagulans in a dynamic in vitro model of the stomach and small intestine. Benef Microbes. 2010;1(1):31–36. doi:10.3920/BM2009.0009.
- Krautkramer KA, Fan J, Backhed F. Gut microbial metabolites as multi-kingdom intermediates. Nat Rev Microbiol. 2021;19(2):77–94. doi:10.1038/s41579-020-0438-4.
- Frampton J, Murphy KG, Frost G, Chambers ES. Short-chain fatty acids as potential regulators of skeletal muscle metabolism and function. Nat Metab. 2020;2(9):840–848. doi:10.1038/s42255-020-0188-7.
- McCarville JL, Chen GY, Cuevas VD, Troha K, Ayres JS. Microbiota metabolites in health and disease. Annu Rev Immunol. 2020;38(1):147–170. doi:10.1146/annurev-immunol-071219-125715.
- Cummings JH, Pomare EW, Branch WJ, Naylor CP, Macfarlane GT. Short chain fatty acids in human large intestine, portal, hepatic and venous blood. Gut. 1987;28(10):1221–1227. doi:10.1136/gut.28.10.1221.
- Wang Y, Dilidaxi D, Wu Y, Sailike J, Sun X, Nabi XH. Composite probiotics alleviate type 2 diabetes by regulating intestinal microbiota and inducing GLP-1 secretion in db/db mice. Biomed Pharmacother. 2020;125:109914. doi:10.1016/j.biopha.2020.109914.
- Markowiak-Kopec P, Slizewska K. The effect of probiotics on the production of Short-chain fatty acids by human intestinal microbiome. Nutrients. 2020;12(4):12. doi:10.3390/nu12041107.
- Canfora EE, Jocken JW, Blaak EE. Short-chain fatty acids in control of body weight and insulin sensitivity. Nat Rev Endocrinol. 2015;11(10):577–591. doi:10.1038/nrendo.2015.128.
- Walsh ME, Bhattacharya A, Sataranatarajan K, Qaisar R, Sloane L, Rahman MM, Kinter M, Van Remmen H. The histone deacetylase inhibitor butyrate improves metabolism and reduces muscle atrophy during aging. Aging Cell. 2015;14(6):957–970. doi:10.1111/acel.12387.
- Gao Z, Yin J, Zhang J, Ward RE, Martin RJ, Lefevre M, Cefalu WT, Ye J. Butyrate improves insulin sensitivity and increases energy expenditure in mice. Diabetes. 2009;58(7):1509–1517. doi:10.2337/db08-1637.
- Al-Harbi NO, Nadeem A, Ahmad SF, Alotaibi MR, AlAsmari AF, Alanazi WA, Al-Harbi MM, El-Sherbeeny AM, Ibrahim KE. Short chain fatty acid, acetate ameliorates sepsis-induced acute kidney injury by inhibition of NADPH oxidase signaling in T cells. Int Immunopharmacol. 2018;58:24–31. doi:10.1016/j.intimp.2018.02.023.
- Liu L, Fu C, Li F. Acetate affects the process of lipid metabolism in rabbit liver, skeletal muscle and adipose tissue. Anim (Basel). 2019;9(10):9. doi:10.3390/ani9100799.
- Gizard F, Fernandez A, De Vadder F. Interactions between gut microbiota and skeletal muscle. Nutr Metab Insights. 2020;13:1178638820980490. doi:10.1177/1178638820980490.
- Agus A, Planchais J, Sokol H. Gut microbiota regulation of tryptophan metabolism in health and disease. Cell Host & Microbe. 2018;23(6):716–724. doi:10.1016/j.chom.2018.05.003.
- Alexeev EE, Lanis JM, Kao DJ, Campbell EL, Kelly CJ, Battista KD, Gerich ME, Jenkins BR, Walk ST, Kominsky DJ, et al. Microbiota-derived indole metabolites promote human and murine intestinal homeostasis through regulation of Interleukin-10 receptor. Am J Pathol. 2018;188(5):1183–1194. doi:10.1016/j.ajpath.2018.01.011.
- Wlodarska M, Luo C, Kolde R, d’Hennezel E, Annand JW, Heim CE, Krastel P, Schmitt EK, Omar AS, Creasey EA, et al. Indoleacrylic acid produced by commensal peptostreptococcus species suppresses inflammation. Cell Host & Microbe. 2017;22(1):25–37.e6. doi:10.1016/j.chom.2017.06.007.
- de Aguiar Vallim TQ, Tarling EJ, Edwards PA. Pleiotropic roles of bile acids in metabolism. Cell Metab. 2013;17(5):657–669. doi:10.1016/j.cmet.2013.03.013.
- Ridlon JM, Kang DJ, Hylemon PB, Bajaj JS. Bile acids and the gut microbiome. Curr Opin Gastroenterol. 2014;30(3):332–338. doi:10.1097/MOG.0000000000000057.
- Vacca M, Celano G, Calabrese FM, Portincasa P, Gobbetti M, De Angelis M. The controversial role of human gut Lachnospiraceae. Microorganisms. 2020;8(4):8. doi:10.3390/microorganisms8040573.
- Arora T, Backhed F. The gut microbiota and metabolic disease: current understanding and future perspectives. J Intern Med. 2016;280(4):339–349. doi:10.1111/joim.12508.
- Qiu Y, Yu J, Li Y, Yang F, Yu H, Xue M, Zhang F, Jiang X, Ji X, Bao Z, et al. Depletion of gut microbiota induces skeletal muscle atrophy by FXR-FGF15/19 signalling. Ann Med. 2021;53(1):508–522. doi:10.1080/07853890.2021.1900593.
- Kobayashi Y, Hara N, Sugimoto R, Mifuji-Moroka R, Tanaka H, Eguchi A, Iwasa M, Hasegawa H, Iwata K, Takei Y, et al. The associations between circulating bile acids and the muscle volume in patients with Non-Alcoholic Fatty Liver Disease (NAFLD). Intern Med. 2017;56(7):755–762. doi:10.2169/internalmedicine.56.7796.
- LeBlanc JG, Milani C, de Giori GS, Sesma F, van Sinderen D, Ventura M. Bacteria as vitamin suppliers to their host: a gut microbiota perspective. Curr Opin Biotechnol. 2013;24(2):160–168. doi:10.1016/j.copbio.2012.08.005.
- Piquereau J, Boitard SE, Ventura-Clapier R, Mericskay M. Metabolic therapy of heart failure: is there a future for B vitamins? Int J Mol Sci. 2021;23(1):23. doi:10.3390/ijms23010030.
- Mithal A, Bonjour JP, Boonen S, Burckhardt P, Degens H, El Hajj Fuleihan G, Josse R, Lips P, Morales Torres J, Rizzoli R, et al. Impact of nutrition on muscle mass, strength, and performance in older adults. Osteoporos Int. 2013;24(5):1555–1566. doi:10.1007/s00198-012-2236-y.
- Schepper JD, Irwin R, Kang J, Dagenais K, Lemon T, Shinouskis A, Parameswaran N, McCabe, LR. Probiotics in gut-bone signaling. Adv Exp Med Biol. 2017;1033:225–247.
- Zhao X, Ai J, Mao H, Gao X. Effects of Eclipta prostrata on gut microbiota of SAMP6 mice with osteoporosis. J Med Microbiol. 2019;68(3):402–416. doi:10.1099/jmm.0.000936.
- Schepper JD, Collins FL, Rios-Arce ND, Raehtz S, Schaefer L, Gardinier JD, Britton RA, Parameswaran N, McCabe LR. Probiotic Lactobacillus reuteri prevents postantibiotic bone loss by reducing intestinal dysbiosis and preventing barrier disruption. J Bone Miner Res. 2019;34(4):681–698. doi:10.1002/jbmr.3635.
- Nilsson AG, Sundh D, Backhed F, Lorentzon M. Lactobacillus reuteri reduces bone loss in older women with low bone mineral density: a randomized, placebo-controlled, double-blind, clinical trial. J Intern Med. 2018;284(3):307–317. doi:10.1111/joim.12805.
- Yuan S, Shen J. Bacteroides vulgatus diminishes colonic microbiota dysbiosis ameliorating lumbar bone loss in ovariectomized mice. Bone. 2021;142:115710. doi:10.1016/j.bone.2020.115710.
- Dar HY, Shukla P, Mishra PK, Anupam R, Mondal RK, Tomar GB, Sharma V, Srivastava RK. Lactobacillus acidophilus inhibits bone loss and increases bone heterogeneity in osteoporotic mice via modulating Treg-Th17 cell balance. Bone Rep. 2018;8:46–56. doi:10.1016/j.bonr.2018.02.001.
- Dar HY, Pal S, Shukla P, Mishra PK, Tomar GB, Chattopadhyay N, Srivastava RK. Bacillus clausii inhibits bone loss by skewing Treg-Th17 cell equilibrium in postmenopausal osteoporotic mice model. Nutrition. 2018;54:118–128. doi:10.1016/j.nut.2018.02.013.
- Parvaneh K, Ebrahimi M, Sabran MR, Karimi G, Hwei AN, Abdul-Majeed S, Ahmad Z, Ibrahim Z, Jamaluddin R. Probiotics (Bifidobacterium longum) increase bone mass density and upregulate sparc and bmp-2 genes in rats with bone loss resulting from Ovariectomy. Biomed Res IntBiomed Res Int. 2015;2015:1–10. doi:10.1155/2015/897639.
- Rodrigues FC, Castro AS, Rodrigues VC, Fernandes SA, Fontes EA, de Oliveira TT, Martino HSD, de Luces Fortes Ferreira CL. Yacon flour and Bifidobacterium longum modulate bone health in rats. J Med Food. 2012;15(7):664–670. doi:10.1089/jmf.2011.0296.
- Britton RA, Irwin R, Quach D, Schaefer L, Zhang J, Lee T, Parameswaran N, McCabe LR. Probiotic L. reuteri treatment prevents bone loss in a menopausal ovariectomized mouse model. J Cell Physiol. 2014;229(11):1822–1830. doi:10.1002/jcp.24636.
- Lucas S, Omata Y, Hofmann J, Bottcher M, Iljazovic A, Sarter K, Albrecht O, Schulz O, Krishnacoumar B, Krönke G, et al. Short-chain fatty acids regulate systemic bone mass and protect from pathological bone loss. Nat Commun. 2018;9(1):55. doi:10.1038/s41467-017-02490-4.
- Tang X, Ma S, Li Y, Sun Y, Zhang K, Zhou Q, Yu R. Evaluating the activity of sodium butyrate to prevent osteoporosis in rats by promoting osteal GSK-3β/Nrf2 signaling and mitochondrial function. J Agric Food Chem. 2020;68(24):6588–6603. doi:10.1021/acs.jafc.0c01820.
- Metz R, Smith C, DuHadaway JB, Chandler P, Baban B, Merlo LM, Pigott E, Keough MP, Rust S, Mellor AL, et al. IDO2 is critical for IDO1-mediated T-cell regulation and exerts a non-redundant function in inflammation. Int Immunol. 2014;26(7):357–367. doi:10.1093/intimm/dxt073.
- Kim BJ, Hamrick MW, Yoo HJ, Lee SH, Kim SJ, Koh JM, Isales CM. The detrimental effects of Kynurenine, a tryptophan metabolite, on human bone metabolism. J Clin Endocrinol Metab. 2019;104(6):2334–2342. doi:10.1210/jc.2018-02481.
- Refaey ME, McGee-Lawrence ME, Fulzele S, Kennedy EJ, Bollag WB, Elsalanty M, Zhong Q, Ding K-H, Bendzunas NG, Shi X-M, et al. Kynurenine, a tryptophan metabolite that accumulates with age, induces bone loss. J Bone Miner Res. 2017;32(11):2182–2193. doi:10.1002/jbmr.3224.
- Kim GS, Kim CH, Park JY, Lee KU, Park CS. Effects of vitamin B12 on cell proliferation and cellular alkaline phosphatase activity in human bone marrow stromal osteoprogenitor cells and UMR106 osteoblastic cells. Metabolism. 1996;45(12):1443–1446. doi:10.1016/S0026-0495(96)90171-7.
- Slavin J. Fiber and prebiotics: mechanisms and health benefits. Nutrients. 2013;5(4):1417–1435. doi:10.3390/nu5041417.
- Al-Harbi NO, Imam F, Nadeem A, Al-Harbi MM, Iqbal M, Ahmad SF. Carbon tetrachloride-induced hepatotoxicity in rat is reversed by treatment with riboflavin. Int Immunopharmacol. 2014;21(2):383–388. doi:10.1016/j.intimp.2014.05.014.
- LeBlanc JG, Levit R, Savoy de Giori G, de Moreno de LeBlanc A. Application of vitamin-producing lactic acid bacteria to treat intestinal inflammatory diseases. Appl Microbiol Biotechnol. 2020;104(8):3331–3337. doi:10.1007/s00253-020-10487-1.
- Dehkordi EH, Sattari F, Khoshdel A, Kasiri K. Effect of folic acid and metformin on insulin resistance and inflammatory factors of obese children and adolescents. J Res Med Sci. 2016;21(1):71. doi:10.4103/1735-1995.189669.
- Wallace TC, Marzorati M, Spence L, Weaver CM, Williamson PS. New frontiers in fibers: innovative and emerging research on the gut microbiome and bone health. J Am Coll Nutr. 2017;36(3):218–222. doi:10.1080/07315724.2016.1257961.
- Cho SW, An JH, Park H, Yang JY, Choi HJ, Kim SW, Park YJ, Kim SY, Yim M, Baek W-Y, et al. Positive regulation of osteogenesis by bile acid through FXR. J Bone Miner Res. 2013;28(10):2109–2121. doi:10.1002/jbmr.1961.
- Sandoval DA, D’Alessio DA. Physiology of proglucagon peptides: role of glucagon and GLP-1 in health and disease. Physiol Rev. 2015;95(2):513–548. doi:10.1152/physrev.00013.2014.
- Kwon JY, Lee SH, Jhun J, Choi J, Jung K, Cho KH, Kim SJ, Yang CW, Park S-H, Cho M-L, et al. The combination of probiotic complex, Rosavin, and zinc improves pain and cartilage destruction in an osteoarthritis rat model. J Med Food. 2018;21(4):364–371. doi:10.1089/jmf.2017.4034.
- Lei M, Guo C, Wang D, Zhang C, Hua L. The effect of probiotic Lactobacillus casei Shirota on knee osteoarthritis: a randomised double-blind, placebo-controlled clinical trial. Benef Microbes. 2017;8(5):697–703. doi:10.3920/BM2016.0207.
- Lyu JL, Wang TM, Chen YH, Chang ST, Wu MS, Lin YH, Lin Y-H, Kuan C-M. Oral intake of Streptococcus thermophil us improves knee osteoarthritis degeneration: a randomized, double-blind, placebo-controlled clinical study. Heliyon. 2020;6(4):e03757. doi:10.1016/j.heliyon.2020.e03757.
- Sim BY, Choi HJ, Kim MG, Jeong DG, Lee DG, Yoon JM, Kang D-J, Park S, Ji J-G, Joo I-H, et al. Effects of ID-CBT5101 in preventing and alleviating osteoarthritis symptoms in a monosodium Iodoacetate-induced rat model. J Microbiol Biotechnol. 2018;28(7):1199–1208. doi:10.4014/jmb.1803.03032.
- Amdekar S, Singh V, Singh R, Sharma P, Keshav P, Kumar A. Lactobacillus casei reduces the inflammatory joint damage associated with collagen-induced arthritis (CIA) by reducing the pro-inflammatory cytokines: lactobacillus casei: COX-2 inhibitor. J Clin Immunol. 2011;31(2):147–154. doi:10.1007/s10875-010-9457-7.
- Pan H, Guo R, Ju Y, Wang Q, Zhu J, Xie Y, Zheng Y, Li T, Liu Z, Lu L, et al. A single bacterium restores the microbiome dysbiosis to protect bones from destruction in a rat model of rheumatoid arthritis. Microbiome. 2019;7(1):107. doi:10.1186/s40168-019-0719-1.
- Dourado E, Ferro M, Sousa Guerreiro C, Fonseca JE. Diet as a modulator of intestinal microbiota in rheumatoid arthritis. Nutrients. 2020;12(11):12. doi:10.3390/nu12113504.
- Smith PM, Howitt MR, Panikov N, Michaud M, Gallini CA, Bohlooly YM, Glickman JN, Garrett WS. The microbial metabolites, short-chain fatty acids, regulate colonic Treg cell homeostasis. Sci. 2013;341(6145):569–573. doi:10.1126/science.1241165.
- Cho KH, Na HS, Jhun J, Woo JS, Lee AR, Lee SY, Lee JS, Um IG, Kim SJ, Park S-H, et al. Lactobacillus (LA-1) and butyrate inhibit osteoarthritis by controlling autophagy and inflammatory cell death of chondrocytes. Front Immunol. 2022;13:930511. doi:10.3389/fimmu.2022.930511.
- Lee S, Koh J, Chang Y, Kim HY, Chung DH. Invariant NKT cells functionally link microbiota-induced butyrate production and joint inflammation. J Immunol. 2019;203(12):3199–3208. doi:10.4049/jimmunol.1801314.
- Lee MC, Hsu YJ, Chuang HL, Hsieh PS, Ho HH, Chen WL, Chiu Y-S, Huang C-C. In vivo ergogenic properties of the Bifidobacterium longum OLP-01 isolated from a weightlifting gold medalist. Nutrients. 2019;11(9):11. doi:10.3390/nu11092003.
- Huang WC, Wei CC, Huang CC, Chen WL, Huang HY. The beneficial effects of Lactobacillus plantarum PS128 on high-intensity, exercise-induced oxidative stress, inflammation, and performance in triathletes. Nutrients. 2019;11(2):11. doi:10.3390/nu11020353.
- Lee MC, Hsu YJ, Ho HH, Hsieh SH, Kuo YW, Sung HC, Huang C-C. Lactobacillus salivarius subspecies salicinius SA-03 is a new probiotic capable of enhancing exercise performance and decreasing fatigue. Microorganisms. 2020;8(4):8. doi:10.3390/microorganisms8040545.
- Toda K, Yamauchi Y, Tanaka A, Kuhara T, Odamaki T, Yoshimoto S, Xiao J-Z. Heat-killed Bifidobacterium breve B-3 enhances muscle functions: possible involvement of increases in muscle mass and mitochondrial biogenesis. Nutrients. 2020;12(1):12. doi:10.3390/nu12010219.
- Zhang J, Motyl KJ, Irwin R, MacDougald OA, Britton RA, McCabe LR. Loss of bone and Wnt10b expression in male type 1 diabetic mice is blocked by the probiotic Lactobacillus reuteri. Endocrinology. 2015;156(9):3169–3182. doi:10.1210/EN.2015-1308.
- Li JY, Chassaing B, Tyagi AM, Vaccaro C, Luo T, Adams J, Darby TM, Weitzmann MN, Mulle JG, Gewirtz AT, et al. Sex steroid deficiency–associated bone loss is microbiota dependent and prevented by probiotics. J Clin Invest. 2016;126(6):2049–2063. doi:10.1172/JCI86062.
- Parvaneh M, Karimi G, Jamaluddin R, Ng MH, Zuriati I, Muhammad SI. Lactobacillus helveticus (ATCC 27558) upregulates Runx2 and Bmp2 and modulates bone mineral density in ovariectomy-induced bone loss rats. Clin Interv Aging. 2018;13:1555–1564. doi:10.2147/CIA.S169223.
- Davani-Davari D, Negahdaripour M, Karimzadeh I, Seifan M, Mohkam M, Masoumi SJ, Berenjian A, Ghasemi Y. Prebiotics: definition, types, sources, mechanisms, and clinical applications. Foods. 2019;8(3):92. doi:10.3390/foods8030092.
- Cani PD, Possemiers S, Van de Wiele T, Guiot Y, Everard A, Rottier O, Geurts L, Naslain D, Neyrinck A, Lambert DM, et al. Changes in gut microbiota control inflammation in obese mice through a mechanism involving GLP-2-driven improvement of gut permeability. Gut. 2009;58(8):1091–1103. doi:10.1136/gut.2008.165886.
- Everard A, Lazarevic V, Derrien M, Girard M, Muccioli GG, Neyrinck AM, Possemiers S, Van Holle A, François P, de Vos WM, et al. Responses of gut microbiota and glucose and lipid metabolism to prebiotics in genetic obese and diet-induced leptin-resistant mice. Diabetes. 2011;60(11):2775–2786. doi:10.2337/db11-0227.
- Li R, Boer CG, Oei L, Medina-Gomez C. The gut microbiome: a new frontier in musculoskeletal research. Curr Osteoporos Rep. 2021;19(3):347–357. doi:10.1007/s11914-021-00675-x.
- Farre R, Fiorani M, Abdu Rahiman S, Matteoli G. Intestinal permeability, inflammation and the role of Nutrients. Nutrients. 2020;12(4):12. doi:10.3390/nu12041185.
- Jamshidi S, Masoumi SJ, Abiri B, Vafa M. The effects of synbiotic and/or vitamin D supplementation on gut-muscle axis in overweight and obese women: a study protocol for a double-blind, randomized, placebo-controlled trial. Trials. 2022;23(1):631. doi:10.1186/s13063-022-06598-x.
- Sigmundsdottir H, Pan J, Debes GF, Alt C, Habtezion A, Soler D, Butcher, E C. Dcs metabolize sunlight-induced vitamin D3 to ‘program’ T cell attraction to the epidermal chemokine CCL27. Nat Immunol. 2007;8(3):285–293. doi:10.1038/ni1433.
- Elias KM, Laurence A, Davidson TS, Stephens G, Kanno Y, Shevach EM, O’Shea JJ. Retinoic acid inhibits Th17 polarization and enhances FoxP3 expression through a stat-3/Stat-5 independent signaling pathway. Blood. 2008;111(3):1013–1020. doi:10.1182/blood-2007-06-096438.
- Singh P, Rawat A, Alwakeel M, Sharif E, Al Khodor S. The potential role of vitamin D supplementation as a gut microbiota modifier in healthy individuals. Sci Rep. 2020;10(1):21641. doi:10.1038/s41598-020-77806-4.
- Cantorna MT, Snyder L, Arora J. Vitamin a and vitamin D regulate the microbial complexity, barrier function, and the mucosal immune responses to ensure intestinal homeostasis. Crit Rev Biochem Mol Biol. 2019;54(2):184–192. doi:10.1080/10409238.2019.1611734.
- Prokopidis K, Giannos P, Katsikas Triantafyllidis K, Kechagias KS, Mesinovic J, Witard OC, Scott D. Effect of vitamin D monotherapy on indices of sarcopenia in community-dwelling older adults: a systematic review and meta-analysis. J Cachexia Sarcopenia Muscle. 2022;13(3):1642–1652. doi:10.1002/jcsm.12976.
- Chaplin A, Parra P, Laraichi S, Serra F, Palou A. Calcium supplementation modulates gut microbiota in a prebiotic manner in dietary obese mice. Mol Nutr Food Res. 2016;60(2):468–480. doi:10.1002/mnfr.201500480.
- Nadeem Aslam M, Bassis CM, Zhang L, Zaidi S, Varani J, Bergin IL, Pazienza V. Calcium reduces liver injury in mice on a high-fat diet: alterations in microbial and bile acid profiles. PloS One. 2016;11(11):e0166178. doi:10.1371/journal.pone.0166178.
- Shanahan F, van Sinderen D, O’Toole PW, Stanton C. Feeding the microbiota: transducer of nutrient signals for the host. Gut. 2017;66(9):1709–1717. doi:10.1136/gutjnl-2017-313872.
- Wu GD, Chen J, Hoffmann C, Bittinger K, Chen YY, Keilbaugh SA, Bewtra M, Knights D, Walters WA, Knight R, et al. Linking long-term dietary patterns with gut microbial enterotypes. Sci. 2011;334(6052):105–108. doi:10.1126/science.1208344.
- David LA, Maurice CF, Carmody RN, Gootenberg DB, Button JE, Wolfe BE, Ling AV, Devlin AS, Varma Y, Fischbach MA, et al. Diet rapidly and reproducibly alters the human gut microbiome. Nature. 2014;505(7484):559–563. doi:10.1038/nature12820.
- Simpson HL, Campbell BJ. Review article: dietary fibre-microbiota interactions. Aliment Pharmacol Ther. 2015;42(2):158–179. doi:10.1111/apt.13248.
- Ni Lochlainn M, Bowyer RCE, Steves CJ. Dietary protein and muscle in aging people: the potential role of the gut microbiome. Nutrients. 2018;10(7):929.
- Clarke SF, Murphy EF, O’Sullivan O, Lucey AJ, Humphreys M, Hogan A, Hayes P, O’Reilly M, Jeffery IB, Wood-Martin R, et al. Exercise and associated dietary extremes impact on gut microbial diversity. Gut. 2014;63(12):1913–1920. doi:10.1136/gutjnl-2013-306541.
- Moreno-Perez D, Bressa C, Bailen M, Hamed-Bousdar S, Naclerio F, Carmona M, Pérez M, González-Soltero R, Montalvo-Lominchar M, Carabaña C, et al. Effect of a protein supplement on the gut microbiota of endurance athletes: a randomized, controlled, double-blind Pilot study. Nutrients. 2018;10(3):10. doi:10.3390/nu10030337.
- Karlund A, Gomez-Gallego C, Turpeinen AM, Palo-Oja OM, El-Nezami H, Kolehmainen M. Protein supplements and their relation with Nutrition, microbiota composition and health: is more protein always better for sportspeople? Nutrients. 2019;11(4):11. doi:10.3390/nu11040829.
- Gulhane M, Murray L, Lourie R, Tong H, Sheng YH, Wang R, Kang A, Schreiber V, Wong KY, Magor G, et al. High fat diets induce colonic epithelial cell stress and inflammation that is reversed by IL-22. Sci Rep. 2016;6(1):28990. doi:10.1038/srep28990.
- Guo S, Al-Sadi R, Said HM, Ma TY. Lipopolysaccharide causes an increase in intestinal tight junction permeability in vitro and in vivo by inducing enterocyte membrane expression and localization of TLR-4 and CD14. Am J Pathol. 2013;182(2):375–387. doi:10.1016/j.ajpath.2012.10.014.
- Maurizi G, Della Guardia L, Maurizi A, Poloni A. Adipocytes properties and crosstalk with immune system in obesity-related inflammation. J Cell Physiol. 2018;233(1):88–97. doi:10.1002/jcp.25855.
- Zhernakova A, Kurilshikov A, Bonder MJ, Tigchelaar EF, Schirmer M, Vatanen T, Mujagic Z, Vila AV, Falony G, Vieira-Silva S, et al. Population-based metagenomics analysis reveals markers for gut microbiome composition and diversity. Sci. 2016;352(6285):565–569. doi:10.1126/science.aad3369.
- Liu J, He Z, Ma N, Chen ZY. Beneficial effects of dietary polyphenols on high-fat diet-induced obesity Linking with modulation of gut microbiota. J Agric Food Chem. 2020;68(1):33–47. doi:10.1021/acs.jafc.9b06817.
- Beaudart C, Dawson A, Shaw SC, Harvey NC, Kanis JA, Binkley N, Reginster JY, Chapurlat R, Chan DC, Bruyère O, et al. Nutrition and physical activity in the prevention and treatment of sarcopenia: systematic review. Osteoporos Int. 2017;28(6):1817–1833. doi:10.1007/s00198-017-3980-9.
- Kohrt WM, Bloomfield SA, Little KD, Nelson ME, Yingling VR. American college of Sports M. American college of sports medicine position stand: physical activity and bone health. Med Sci Sports Exerc. 2004;36(11):1985–1996. doi:10.1249/01.MSS.0000142662.21767.58.
- Regnaux JP, Lefevre-Colau MM, Trinquart L, Nguyen C, Boutron I, Brosseau L, Ravaud P. High-intensity versus low-intensity physical activity or exercise in people with hip or knee osteoarthritis. Cochrane Database Of Systematic Reviews. 2015;2015(10):CD010203. doi:10.1002/14651858.CD010203.pub2.
- Denou E, Marcinko K, Surette MG, Steinberg GR, Schertzer JD. High-intensity exercise training increases the diversity and metabolic capacity of the mouse distal gut microbiota during diet-induced obesity. Am J Physiol Endocrinol Metab. 2016;310(11):E982–93. doi:10.1152/ajpendo.00537.2015.
- Welly RJ, Liu TW, Zidon TM, Rowles JL 3rd, Park YM, Smith TN, SWANSON KS, PADILLA J, VIEIRA-POTTER VJ. Comparison of diet versus exercise on metabolic function and gut microbiota in obese rats. Med Sci Sports Exerc. 2016;48(9):1688–1698. doi:10.1249/MSS.0000000000000964.
- O’Sullivan O, Cronin O, Clarke SF, Murphy EF, Molloy MG, Shanahan F, Cotter PD. Exercise and the microbiota. Gut Microbes. 2015;6(2):131–136. doi:10.1080/19490976.2015.1011875.
- Scheiman J, Luber JM, Chavkin TA, MacDonald T, Tung A, Pham LD, Wibowo MC, Wurth RC, Punthambaker S, Tierney BT, et al. Meta-omics analysis of elite athletes identifies a performance-enhancing microbe that functions via lactate metabolism. Nat Med. 2019;25(7):1104–1109. doi:10.1038/s41591-019-0485-4.
- Allen JM, Mailing LJ, Niemiro GM, Moore R, Cook MD, White BA, HOLSCHER HD, WOODS JA. Exercise alters gut microbiota composition and function in lean and obese humans. Med Sci Sports Exerc. 2018;50(4):747–757. doi:10.1249/MSS.0000000000001495.
- Morita E, Yokoyama H, Imai D, Takeda R, Ota A, Kawai E, Hisada T, Emoto M, Suzuki Y, Okazaki K. Aerobic exercise training with brisk walking increases intestinal Bacteroides in healthy elderly women. Nutrients. 2019;11(4):11. doi:10.3390/nu11040868.
- de Sire A, de Sire R, Petito V, Masi L, Cisari C, Gasbarrini A, Scaldaferri F, Invernizzi M. Gut–joint axis: the role of physical exercise on gut microbiota modulation in older people with osteoarthritis. Nutrients. 2020;12(2):12. doi:10.3390/nu12020574.
- Hao X, Shang X, Liu J, Chi R, Zhang J, Xu T. The gut microbiota in osteoarthritis: where do we stand and what can we do? Arthritis Res Ther. 2021;23(1):42. doi:10.1186/s13075-021-02427-9.
- Rios JL, Bomhof MR, Reimer RA, Hart DA, Collins KH, Herzog W. Protective effect of prebiotic and exercise intervention on knee health in a rat model of diet-induced obesity. Sci Rep. 2019;9(1):3893. doi:10.1038/s41598-019-40601-x.
- Rios JL, Hart DA, Reimer RA, Herzog W. Prebiotic and exercise do not alter knee osteoarthritis in a rat model of established obesity. Cartilage. 2021;13(2_suppl):1456S–1466S. doi:10.1177/1947603520959399.
- Przewlocka K, Folwarski M, Kazmierczak-Siedlecka K, Skonieczna-Zydecka K, Kaczor JJ. Gut-muscle AxisExists and May affect skeletal muscle adaptation to training. Nutrients. 2020;12(5):12. doi:10.3390/nu12051451.
- Hearris MA, Hammond KM, Fell JM, Morton JP. Regulation of muscle glycogen metabolism during exercise: implications for endurance performance and training adaptations. Nutrients. 2018;10(3):10. doi:10.3390/nu10030298.
- Sohail MU, Yassine HM, Sohail A, Thani AAA. Impact of physical exercise on gut microbiome, inflammation, and the pathobiology of metabolic disorders. Rev Diabet Stud. 2019;15(1):35–48. doi:10.1900/RDS.2019.15.35.
- Karl JP, Margolis LM, Madslien EH, Murphy NE, Castellani JW, Gundersen Y, Hoke AV, Levangie MW, Kumar R, Chakraborty N, et al. Changes in intestinal microbiota composition and metabolism coincide with increased intestinal permeability in young adults under prolonged physiological stress. Am J Physiol Gastrointest Liver Physiol. 2017;312(6):G559–G571. doi:10.1152/ajpgi.00066.2017.
- Sender R, Fuchs S, Milo R. Revised estimates for the number of human and bacteria cells in the body. PLoS Biol. 2016;14(8):e1002533. doi:10.1371/journal.pbio.1002533.
- Sinha T, Vich Vila A, Garmaeva S, Jankipersadsing SA, Imhann F, Collij V, Bonder MJ, Jiang X, Gurry T, Alm EJ, et al. Analysis of 1135 gut metagenomes identifies sex-specific resistome profiles. Gut Microbes. 2019;10(3):358–366. doi:10.1080/19490976.2018.1528822.
- Yoon K, Kim N. Roles of sex hormones and gender in the gut microbiota. J Neurogastroenterol Motil. 2021;27(3):314–325. doi:10.5056/jnm20208.
- Flores R, Shi J, Fuhrman B, Xu X, Veenstra TD, Gail MH, Gajer P, Ravel J, Goedert JJ. Fecal microbial determinants of fecal and systemic estrogens and estrogen metabolites: a cross-sectional study. J Transl Med. 2012;10(1):253. doi:10.1186/1479-5876-10-253.
- Morwani-Mangnani J, Giannos P, Belzer C, Beekman M, Eline Slagboom P, Prokopidis K. Gut microbiome changes due to sleep disruption in older and younger individuals: a case for sarcopenia? Sleep. 2022;45(12):zsac239. doi:10.1093/sleep/zsac239.
- Stacchiotti A, Favero G, Rodella LF. Impact of melatonin on skeletal muscle and exercise. Cells. 2020;9(2):288. doi:10.3390/cells9020288.
- Leonardo-Mendonca RC, Ocana-Wilhelmi J, de Haro T, de Teresa-Galvan C, Guerra-Hernandez E, Rusanova I, Fernández-Ortiz M, Sayed RKA, Escames G, Acuña-Castroviejo D, et al. The benefit of a supplement with the antioxidant melatonin on redox status and muscle damage in resistance-trained athletes. Appl Physiol Nutr Metab. 2017;42(7):700–707. doi:10.1139/apnm-2016-0677.
- Rodriguez MI, Carretero M, Escames G, Lopez LC, Maldonado MD, Tan DX, Reiter RJ, Acuña-Castroviejo D. Chronic melatonin treatment prevents age-dependent cardiac mitochondrial dysfunction in senescence-accelerated mice. Free Radic Res. 2007;41(1):15–24. doi:10.1080/10715760600936359.
- Obayashi K, Saeki K, Maegawa T, Iwamoto J, Sakai T, Otaki N, Kataoka H, Kurumatani N. Melatonin secretion and muscle strength in elderly individuals: a cross-sectional study of the HEIJO-KYO cohort. J Gerontol A Biol Sci Med Sci. 2016;71(9):1235–1240. doi:10.1093/gerona/glw030.
- Gao T, Wang Z, Dong Y, Cao J, Lin R, Wang X, Yu Z, Chen Y. Role of melatonin in sleep deprivation-induced intestinal barrier dysfunction in mice. J Pineal Res. 2019;67(1):e12574. doi:10.1111/jpi.12574.
- Yin J, Li Y, Han H, Chen S, Gao J, Liu G, Wu X, Deng J, Yu Q, Huang X, et al. Melatonin reprogramming of gut microbiota improves lipid dysmetabolism in high-fat diet-fed mice. J Pineal Res. 2018;65(4):e12524. doi:10.1111/jpi.12524.
- Xu P, Wang J, Hong F, Wang S, Jin X, Xue T, Jia L, Zhai Y. Melatonin prevents obesity through modulation of gut microbiota in mice. J Pineal Res. 2017;62(4):62. doi:10.1111/jpi.12399.
- She M, Deng X, Guo Z, Laudon M, Hu Z, Liao D, Hu X, Luo Y, Shen Q, Su Z, et al. NEU-P11, a novel melatonin agonist, inhibits weight gain and improves insulin sensitivity in high-fat/high-sucrose-fed rats. Pharmacol Res. 2009;59(4):248–253. doi:10.1016/j.phrs.2009.01.005.
- Zhu X, Zhang Y, Yang H, He F, Lin J. Melatonin suppresses ti-particle-induced inflammatory osteolysis via activation of the Nrf2/Catalase signaling pathway. Int Immunopharmacol. 2020;88:106847. doi:10.1016/j.intimp.2020.106847.
- Liebert A, Bicknell B, Johnstone DM, Gordon LC, Kiat H, Hamblin MR. “Photobiomics”: can light, including Photobiomodulation, alter the microbiome? Photobiomodul Photomed Laser Surg. 2019;37(11):681–693. doi:10.1089/photob.2019.4628.
- Bicknell B, Liebert A, Johnstone D, Kiat H. Photobiomodulation of the microbiome: implications for metabolic and inflammatory diseases. Lasers Med Sci. 2019;34(2):317–327. doi:10.1007/s10103-018-2594-6.
- Cui J, Fu Y, Yi Z, Dong C, Liu H. The beneficial effects of ultraviolet light supplementation on bone density are associated with the intestinal flora in rats. Appl Microbiol Biotechnol. 2021;105(9):3705–3715. doi:10.1007/s00253-021-11282-2.
- Guo R, Du Y, Zhang S, Liu H, Fu Y. The effects of ultraviolet supplementation to the artificial lighting on rats’ bone metabolism, bone mineral density, and skin. J Photochem Photobiol B. 2018;188:12–18. doi:10.1016/j.jphotobiol.2018.08.020.
- Gonzalez-Alvarez ME, Sanchez-Romero EA, Turroni S, Fernandez-Carnero J, Villafane JH. Correlation between the altered gut microbiome and lifestyle interventions in chronic widespread pain patients: a systematic review. Medicina (Kaunas). 2023;59(2):59. doi:10.3390/medicina59020256.
- Wang K, Zhang Z, Hang J, Liu J, Guo F, Ding Y, Li M, Nie Q, Lin J, Zhuo Y, et al. Microbial-host-isozyme analyses reveal microbial DPP4 as a potential antidiabetic target. Sci. 2023;381(6657):eadd5787. doi:10.1126/science.add5787.
- Wang Y, Zhang S, Borody TJ, Zhang F. Encyclopedia of fecal microbiota transplantation: a review of effectiveness in the treatment of 85 diseases. Chin Med J (Engl). 2022;135(16):1927–1939. doi:10.1097/CM9.0000000000002339.