ABSTRACT
Inflammatory bowel disease (IBD) is a chronic, recurrent inflammatory disease of the gastrointestinal tract. In addition to digestive symptoms, patients with IBD may also develop extra-intestinal manifestations (EIMs), the etiology of which remains undefined. The gut microbiota has been reported to exert a critical role in the pathogenesis of IBD, with a similar pattern of gut dysbiosis observed between patients with IBD and those with EIMs. Therefore, it is hypothesized that the gut microbiota is also involved in the pathogenesis of EIMs. The potential mechanisms are presented in this review, including: 1) impaired gut barrier: dysbiosis induces pore formation in the intestinal epithelium, and activates pattern recognition receptors to promote local inflammation; 2) microbial translocation: intestinal pathogens, antigens, and toxins translocate via the impaired gut barrier into extra-intestinal sites; 3) molecular mimicry: certain microbial antigens share similar epitopes with self-antigens, inducing inflammatory responses targeting extra-intestinal tissues; 4) microbiota-related metabolites: dysbiosis results in the dysregulation of microbiota-related metabolites, which could modulate the differentiation of lymphocytes and cytokine production; 5) immunocytes and cytokines: immunocytes are over-activated and pro-inflammatory cytokines are excessively released. Additionally, we summarize microbiota-related therapies, including probiotics, prebiotics, postbiotics, antibiotics, and fecal microbiota transplantation, to promote better clinical management of IBD-associated EIMs.
Introduction
Inflammatory bowel disease (IBD) is a chronic, recurrent, progressive, destructive inflammatory disorder of the gastrointestinal tract.Citation1 The rising incidence, especially in newly industrialized countries such as China, has caused a considerable burden on the society.Citation2 Crohn’s disease (CD) and ulcerative colitis (UC) are the two major subtypes of IBD. The main clinical features of CD include diarrhea, abdominal pain, and weight loss, whereas UC is characterized by mucopurulent bloody stools.Citation3 Currently, the most commonly accepted hypothesis regarding IBD etiology is that environmental factors acting on genetically susceptible populations result in a leaky gut barrier, microbiota dysbiosis, and ultimately overactivation of immune responses.Citation4 In particular, the intestinal microbiota has been shown to take a critical position.Citation5 Diet, 5-amino salicylic acid (5-ASA), corticosteroids, immunosuppressors, biologics, and small molecules are widely used for the management of IBD.Citation1,Citation6
Not only involving the gastrointestinal tract, IBD manifests in extra-intestinal organs in up to 50% IBD patients.Citation7 These EIMs are defined as inflammations beyond the digestive tract in patients with IBD, which develop either as expansion of intestinal inflammatory responses or with similar genetic or environmental predisposition to IBD.Citation7 Most EIMs develop in parallel with the disease activity of IBD and are associated with unfavorable prognosis, including medication escalation, surgery, and poor quality of life.Citation8 Thus, EIMs have been placed in an increasingly important position for better management of IBD. However, the pathophysiology of EIMs remains unclear and generally include genetic susceptibility, environmental factors, dysregulated immune responses, and microbiota dysbiosis.Citation7
Patients with IBD partly share genetic mutations, including that of nucleotide-binding oligomerization domain-containing protein 2 (NOD2)/caspase recruitment domain 15 (CARD15), with those who develop EIMs.Citation9 In addition, common environmental factors, including higher exposure to smoking, have been reported in IBD and EIMs.Citation10 Furthermore, a series of immunocytes, pro-inflammatory cytokines, and signaling pathways, such as interleukin (IL)-23 and IL-17, has been associated with the development of EIMs.Citation11 Accordingly, the gut microbiota, which interacts with genetic susceptibility and environmental factors and is highly responsible for immune activation, is thus hypothesized to take an essential role in the pathogenesis of EIMs.
In this review, we first introduce the overall pathophysiology of EIMs. Next, we summarize gut dysbiosis in some common EIMs and further explore the essential roles of microbiota in the pathogenesis. Finally, we discuss the potential treatment options based on microbiota for IBD-associated EIMs.
EIMs in IBD
According to the European Crohn’s and Colitis Organization, up to 50% of IBD patients experience at least one EIM.Citation7 Among the diverse types of EIMs, musculoskeletal system (e.g., peripheral arthritis, axial spondyloarthropathy), mucocutaneous system (e.g., psoriasis), ocular system (e.g., uveitis), hepatobiliary tract (e.g., primary sclerosing cholangitis [PSC]) and oral cavity (e.g., periodontitis) are most frequently affected (). Other systems may also be involved, such as the cardiovascular system (e.g., ischemic heart disease), respiratory system (e.g., interstitial pneumonia), pancreas, and urogenital system (e.g., renal insufficiency).Citation8,Citation33 Typically, EIMs manifest after IBD, while nearly 25% patients develop EIMs before the occurrence of IBD, particularly uveitis and axial spondyloarthropathy.Citation34
Table 1. Extra-intestinal manifestations of inflammatory bowel disease in adults: prevalence, susceptible groups and available treatments.
The occurrence of EIMs poses a great impact on the quality of life of patients with IBD. Most EIMs develop in parallel with disease activity () and may indicate a higher risk of treatment escalation.Citation35 Furthermore, the presence of EIMs could affect the original therapeutic approach. For example, vedolizumab, a highly gut-selective biological agent, should be cautiously administered in cases of EIMs. Confronted with EIMs independent of IBD disease activity, the benefit of other biologics such as infliximab would overwhelm that of vedolizumab.Citation36
The remarkable relationship between EIMs and IBD leads to the hypothesis that their underlying pathophysiological mechanisms share certain similarities. An overlap of susceptible gene loci between IBD and EIMs has been reported, and a higher concordance rate for EIMs has been observed in parent-child pairs and sibling pairs.Citation33,Citation37 Mutations in CARD15 contribute to the intracellular persistence of pathogens and a higher risk of joint inflammation. More specifically, encoded by CARD15, NOD2 recognizes bacterial cell wall components and activates nuclear factor-κB (NF-κB), which regulates the release of pro-inflammatory cytokines.Citation9 Additionally, a more frequent exposure to environmental factors such as smoking has been correlated with a higher prevalence of EIMs, while cessation is associated with a lower prevalence.Citation10 Gut dysbiosis has also been reported in EIMs (), in which microbiota, antigens, and toxins might translocate via the impaired intestinal barrier into other extra-intestinal sites, leading to the overactivation of inflammatory responses. As a result, inflammatory signaling pathways are up-regulated with aberrant immunocyte homing and excessive pro-inflammatory cytokine secretion. For instance, the tumor necrosis factor (TNF)-NF-κB pathway is up-regulated in patients in the context of erythema nodosum and pyoderma gangrenosum; correspondingly, anti-TNF therapy has been proven effective in the management of such patients.Citation70 Moreover, the expression of mucosal vascular addressin cell adhesion molecule 1 in the liver and bone marrow induces aberrant lymphocyte homing, triggering inflammation at these extra-intestinal sites.Citation11,Citation71
Table 2. Changes and roles of common gut microbiota that contributes to IBD-associated EIMs.
Mechanisms linking gut dysbiosis to IBD-associated EIMs
As is widely accepted, genes, environment, microbiota, and immune responses contribute to the development of IBD.Citation72,Citation73 Among these, gut dysbiosis plays an essential role. This may be corroborated by a finding that genetically susceptible mice raised in a germ-free environment manifest less colitis, whereas re-exposure to pro-inflammatory microbiota induces gut inflammation.Citation74 In patients with IBD, gut dysbiosis such as increased Bifidobacterium, Enterococcus faecalis, Escherichia coli, Klebsiella, Ruminococcus gnavus, Veillonella parvula and decreased Akkermansia muciniphila, Bacteroides, Clostridium, Coprococcus, Faecalibacterium prausnitzii, Lachnospira, Roseburia, Saccharomyces cerevisiae, have been reported.Citation38,Citation40,Citation43,Citation48,Citation58 The dys-regulated microbiota disrupts the gut barrier, disturbs the metabolism of bile acids (BA) and short-chain fatty acids (SCFA), promotes the hyper-activation of immunocytes and the over-production of pro-inflammatory cytokines, thus contributing to the development of IBD.Citation61
Similarly, in the context of EIMs, animal models that tend to spontaneously develop spondylarthritis (SpA)Citation75 or uveitisCitation76 exhibit less severity when raised in germ-free conditions. However, once the animals are re-exposed to a specific pathogen-free environment or microbial components, the EIMs manifest, which indicates microbiota’s essential effects in EIMs.Citation75,Citation76 Moreover, considering that multiple studies have observed the similar pattern of gut dysbiosis in IBD and various types of EIMs (), it is hypothesized that gut microbiota might exert a significant role with regard to the pathophysiology of EIMs. Afterwards, some of the underlying mechanisms between gut microbiota and EIMs has been discovered, which could be briefly summarized into five paths: 1) impaired gut barrier: dysbiosis induces pore formation in the intestinal epithelium, and activates pattern recognition receptors to promote local inflammation; 2) microbial translocation: intestinal pathogens, antigens, and toxins translocate via the impaired gut barrier into extra-intestinal sites; 3) molecular mimicry: certain microbial antigens share similar epitopes with self-antigens, inducing inflammatory responses targeting extra-intestinal tissues; 4) microbiota-related metabolites: dysbiosis results in the dysregulation of microbiota-related metabolites, which could modulate the differentiation of lymphocytes and cytokine production; 5) immunocytes and cytokines: immunocytes are over-activated and pro-inflammatory cytokines are excessively released ().Citation7
Figure 1. Roles of gut microbiota in the pathophysiology of IBD-associated EIMs. Generally, the intestinal epithelium is composed of enterocytes (the majority, defense and nutrition), goblet cells (scattered, secrete mucus), Paneth cells (crypt base, secrete anti-microbial peptides and regulate stem cells), stem cells (crypt base, proliferate), etc. Macrophages and DCs recognize microbial antigens, secrete pro-inflammatory cytokines or present antigens to other lymphocytes (e.g., naïve T cells, plasma cells), responsible for immune over-activation in the development of IBD and EIMs. (a) impaired gut barrier: transcellular hyper-permeability and paracellular hyper-permeability appear as a result of gut dysbiosis, respectively contributing to specific bacteria internalization and nonspecific microbiota translocation. Elevated expression of zonulin induces a down-regulation of occludin and E-cadherin. (b) translocation: increased levels of gut microbiota, relevant antigens (e.g., DNA) or toxins (e.g., LPS), antigen-loaded immunocytes are detected in the serum of patients with EIMs. (c) molecular mimicry: some bacterial antigens (e.g., Klebsiella nitrogenase) share similar epitopes with self-antigens (e.g., HLA-B27) and induce activation of auto-reactive immunocytes. (d) microbiota-related metabolites: dysregulated metabolism of BAs, SCFAs, MCFAs, etc., results in the impaired mucosa integrity, attenuation of anti-inflammatory response and promotes T cell trafficking toward extra-intestinal sites. (e) immunocytes and cytokines: aberrant expression of cell adhesion molecules and interaction with antigen-loaded macrophages are responsible for abnormal homing of lymphocytes. Activated APCs also favor differentiation of naïve T cells in the direction of pro-inflammatory Th17 cells. Treg, regulatory T cell; teff, effector T cell; LPS, lipopolysaccharide; iFABP, intestinal fatty acid binding protein; GFAP, glial fibrillary acidic protein; PBA, primary bile acid; SBA, secondary bile acid; SCFA, short-chain fatty acid; TGR5, transmembrane G protein-coupled receptor 5; FXR, farnesoid X receptor; ASBT, apical sodium-dependent bile acid transporter; FGF19, fibroblast growth factor 19; GPR43, G protein-coupled receptor 43; GPR109A, G protein-coupled receptor 109A; NLRP3, NOD-, LRR- and pyrin domain-containing 3; MAMPs, microbe associated molecular patterns; TLR, toll-like receptor; NLR, NOD-like receptor; APC, antigen-presenting cell; TNF-α, tumor necrosis factor-α; TGF-β, tumor growth factor-β.
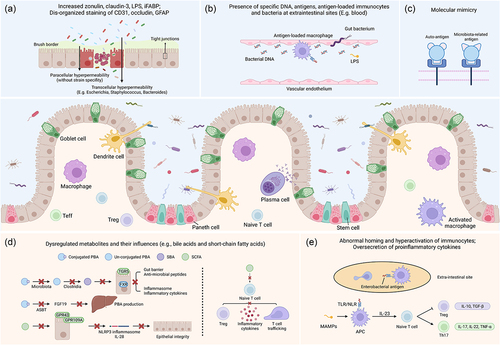
Impaired gut barrier
The gut barrier is primarily composed of digestive juice (e.g., gastric acid), commensal microbiota, antimicrobial peptides, epithelial cells, and local immunocytes.Citation77 Brush border and tight junctions of epithelial cells respectively serve as the transcellular barrier and the paracellular barrier against intestinal flora.Citation78 In terms of IBD and EIMs, an elevated abundance of mucin-degrading bacteria (e.g., R. gnavus) contributes to the disruption of epithelial integrity.Citation41,Citation79 Zonulin and enzymes released by gut microbiota down-regulate the expression of tight junction proteins, resulting in the impaired intestinal barrier.Citation45,Citation65 Furthermore, transcellular hyper-permeability occurs through the internalization of specific bacterial strains such as Escherichia coli and Bacteroides vulgatus.Citation78 Subsequently, paracellular hyper-permeability occurs, leading to nonspecific penetration of the microbiota.Citation78 In this way, a vicious cycle is created.
In fact, down-regulated tight junction proteins and elevated zonulin have been reported in patients with ankylosing spondylitis (AS).Citation45 Additionally, microbiota isolated from ileal biopsies, such as E. coli, which is enriched in patients with IBD and those with SpA,Citation38,Citation46 promote the expression of zonulin in cultured epithelial cells.Citation45,Citation47 Elevated zonulin further down-regulates the levels of occludin, E-cadherin, and other tight junction proteins, thus impairing the gut vascular barrier.Citation45 Correspondingly, after applying antibiotics, the gut microbiome and the expression of tight junction proteins are restored.Citation45
Elevated intestinal fatty acid-binding protein, a predictor of the impaired gut barrier, seems positively correlated with disease severity in patients with psoriasis.Citation80 Enriched Campylobacter spp. and decreased A. muciniphila appear in patients with IBD psoriasis, and correlated with the hyper-permeability of gut mucosa.Citation40,Citation52,Citation55,Citation81,Citation82
In patients with uveitis, the intestinal epithelium is disorganized. In animal models of autoimmune uveitis, the course of impaired intestinal permeability coincides with the extent of gut dysbiosis and the degree of intestinal permeability corresponds with that of ocular inflammation.Citation83,Citation84
In the context of IBD and PSC, expansion of E. faecalis promotes matrix metalloproteinase (e.g., gelatinase) production, degrading E-cadherin, down-regulating trans-epithelial electrical resistance, and impairing mucosal integrity.Citation38,Citation65,Citation66 Furthermore, enriched Klebsiella pneumoniae could trigger pore formation in the gut epithelium in vitro.Citation43,Citation67
Notably, in animal models of periodontitis, berberine contributes to an increased abundance of butyrate-producing gut microbiota (e.g., Roseburia), which is reduced in IBD patients.Citation38,Citation85 Restoration of the gut microbiota improves the gut barrier, lowers the circulatory endotoxin levels, and ameliorates periodontitis, indicating the potential of gut dysbiosis in the pathogenesis of periodontal disease.Citation85
Translocation of bacteria, antigens, and toxins
Penetrating via the impaired gut barrier, harmful gastrointestinal bacteria or relevant toxins and antigens are translocated via the blood or the lymphatics into extra-intestinal sites, including the synovial tissue.Citation86,Citation87 Sometimes, such bacteria, antigens, or toxins can be engulfed and later presented on the surface by immunocytes such as dendritic cells (DCs) and macrophages.Citation86,Citation88 These engulfed bacteria might remain quiescent, or their lysed components (e.g., lipopolysaccharides [LPS]) are released to induce chronic inflammation. Moreover, lymphatic expansion has been confirmed in the context of gastrointestinal inflammation,Citation86 indicating its potential role in the translocation of the gut microbiota.
Although no living microbiota has been isolated from the joint fluid, antigens related to Yersinia enterocolitica and Salmonella enteritidis have been observed in patients with reactive arthritis. Similarly, their DNA have been detected in the intestinal samples and mesenteric lymph nodes (MLN) of patients with CD.Citation89,Citation90 In addition, serum bacterial products, such as LPS and intestinal fatty acid-binding proteins, are increased in patients with IBD-associated SpA.Citation91 Artificial injection of peptidoglycan-polysaccharide complexes successfully induces erosive peripheral arthritis in animal models,Citation92 further indicating a significant role of the gut microbiota.
Elevated serum levels of gut bacterial DNA (e.g., E. coli) have also been reported in patients with psoriasis.Citation87 Methylated DNA segments (e.g., CpG) might act as microbe-associated molecular patterns (MAMPs) to activate immunocytes, such as DCs, thus promoting inflammation in the skin. Accordingly, a higher quantity of microbial DNA typically corresponds with increased circulatory pro-inflammatory cytokine levels (e.g., IL-1β, IL-6, IL-12, TNF-α, and IFN-γ) via interacting with Toll-like receptor 9.Citation87
Although no living microbiota or relevant antigens have been detected in uvea of uveitis patients,Citation83 artificial injection of the group A streptococcus peptidoglycan-polysaccharide complex, which is elevated in IBD patients, successfully induced bilateral uveitis and polyarthritis in Lewis rats.Citation38,Citation92
Enriched Enterococcus gallinarum, and K. pneumoniae, have been observed in patients with IBD and PSC.Citation43,Citation67,Citation93 These bacteria have also been isolated from the MLN of gnotobiotic PSC/UC mice, which is derived from fecal samples from PSC/UC patients.Citation67
Similarly, oral – gut microbiota axis has been proposed to participate in both oral and gastrointestinal inflammation. As has been reported, Klebsiella and Enterobacter spp., originally isolated from the oral cavity, effectively elicit the activation of T helper cells and inflammasome, contributing to the development of colitis.Citation94,Citation95 In addition, elevated circulatory LPS has been reported in animal models of periodontitis. Interestingly, administration of berberine significantly reduces LPS levels, preventing hematogenous translocation of gut pathogens, and thus improving the degree of alveolar bone loss.Citation85
Molecular mimicry
Certain bacterial antigens might share similar amino acid sequences or molecular structures with self-antigens, such as major histocompatibility complex molecules, inducing over-activation of auto-reactive immunocytes targeting human tissues.Citation96
In the context of SpA, antigens related to Bacillus megaterium, E. coli, Klebsiella (nitrogenase), Pseudomonas aeruginosa, and Salmonella typhimurium share similar amino acid sequences (e.g., LRRYLENGK) with human leukocyte antigen class I molecule B27 (HLA-B27).Citation43,Citation96,Citation97 Consequently, T cells are highly activated in response to macrophages presenting peptides of either bacterial antigens or the endogenous HLA-B27 turnover product.Citation97 Chlamydia trachomatis, another arthritis related microbiota, expresses a specific DNA primase that shares a similar sequence with a dodecapeptide of HLA-B27.Citation98 Patients with IBD suffered a high risk of Chlamydia trachomatis infection,Citation99 which might contribute to the development of SpA. Similarities also exist between K. pneumonia nitrogenase reductase and HLA-B27 (QTDRED),Citation100 K. pneumonia pullulanase and HLA-B27 (DRED), K. pneumonia pullulanase and collagens type I, III and IV (“Gly-X-Pro”),Citation101 which reportedly participate in the development and progression of IBD and SpA.
Microbiota-related metabolites
Gut microbiota participates in the production and excretion of various metabolites. Therefore, as a result of gut dysbiosis, microbiota-related metabolites are dys-regulated, leading to disruption of their functions in maintaining the gut barrier, inhibiting microbiota translocation, modulating activation of inflammasomes or other inflammation-related signaling pathways, and directing differentiation and trafficking of immunocytes.Citation102
Bile acids
Primary bile acids (PBAs), primarily including chenodeoxycholic acid (CDCA) and cholic acid (CA), are produced in the liver. PBAs are conjugated to taurine or glycine, which are subsequently released to the intestinal lumen. Most are re-absorbed in the distal ileum via the apical sodium-dependent bile acid transporter, while the remainder is further metabolized in the colon. This process involves deconjugation via bile salt hydrolase, and subsequent de-hydroxylation by various microbiota, e.g., Bacteroides, Clostridium, and F. prausnitzii, ultimately generating secondary bile acids (SBAs, primarily including lithocholic acid [LCA], deoxycholic acid [DCA]).Citation103 Bile acids act by interacting with transmembrane G protein-coupled receptor 5 (TGR5), farnesoid X receptor (FXR), etc. Notably, CDCA has the highest affinity for FXR, while SBAs have a high affinity for TGR5.Citation104,Citation105 Activation of FXR prevents gut hyper-permeability, inhibits bacterial overgrowth and translocation, promotes antimicrobial peptide production, and down-regulates NF-κB associated TNF-α, IL-1β, and IL-6 production.Citation106,Citation107 Meanwhile, TGR5 activation modulates an M2-dominant anti-inflammatory macrophage phenotype transformation, inhibits NF-κB associated pro-inflammatory cytokine production via the cyclic adenosine monophosphate (cAMP)-protein kinase A (PKA) pathway, and blocks the production of IL-1β and the Nod-like receptor protein 3 (NLRP3) inflammasome. Hence, FXR and TGR5 activation both alleviate intestinal inflammation.Citation108,Citation109 Furthermore, SBAs promote the differentiation of anti-inflammatory regulatory T cells (Tregs).Citation108,Citation110,Citation111 In IBD, decreased Clostridium, and F. prausnitzii disturb the de-conjugation and de-hydroxylation processes, resulting in increased PBA and decreased SBA levels in serums and feces.Citation38,Citation103,Citation112,Citation113
In uveitis, SBAs are down-regulated and inhibit DC-secreted pro-inflammatory cytokines such as IL-1β, IL-6, IL-12, and TNF-α via the TGR5-cAMP-PKA-NF-κB signaling pathway, further directing the differentiation of naïve T cells in experimental autoimmune uveitis.Citation114
For patients with IBD-associated PSC, total BAs are significantly down-regulated in fecal samples, with non-significantly increased conjugated BAs. Moreover, levels of SBAs are negatively correlated with disease activity.Citation115 Additionally, the abundances of Clostridium spp. and Ruminococcus spp. are decreased in patients with PSC, which contribute to deconjugation and dihydroxylation of BAs and thus bio-transformation of PBAs to SBAs.Citation64,Citation103,Citation116
For patients with periodontitis, significantly increased conjugated BAs are detected in gingival tissues, which are positively correlated with periodontitis status.Citation117 Other forms of BAs, i.e., primary and secondary, conjugated and unconjugated, are also up-regulated in patients with periodontitis.Citation117
Short-chain fatty acids
Short-chain fatty acids (SCFAs), primarily including acetate, propionate, and butyrate, are synthesized from dietary fibers during the gut microbe metabolism. After interacting with G protein-coupled receptor 43 (GPR43) or GPR109A, SCFAs regulate the activation of the NLRP3 inflammasome and production of IL-18, participating in the repair of the intestinal barrier.Citation118 Butyrate also modulates the expression of MUC genes in goblet cells and thus the properties of mucus, enhancing the barrier function.Citation119 Most importantly, SCFAs inhibit the activity of histone deacetylase, promote the differentiation of naïve T cells toward Tregs instead of T helper type 17 (Th17) cells, and regulate the circulation of T cells toward extra-intestinal sites such as the eyes.Citation102,Citation120 Modulation of pro-inflammatory cytokine secretion and B cell responses, such as plasma cell differentiation and IgG production, has also been observed.Citation121 Moreover, SCFAs reportedly suppress DC maturation, down-regulate DC-secreted chemokines such as C-C chemokine ligand 5, and up-regulate anti-inflammatory IL-10 in DCs to further promote Treg function.Citation121 The anti-inflammatory effects of macrophages (e.g., IL-10 production) are also strengthened following SCFA supplementation.Citation121
For patients with IBD, SpA, psoriasis, uveitis, PSC, or periodontitis, the abundance of butyrate-producing bacteria (e.g., A. muciniphila, Clostridium XIVa, Coprococcus, F. prausnitzii, Lachnospira, Roseburia spp. and Ruminococcus spp.) was reduced.Citation38,Citation50,Citation52,Citation57,Citation62,Citation64,Citation69,Citation122 Meanwhile, fecal samples from patients with arthritis exhibit reduced butyrate levels.Citation123 Butyrate was also found to inhibit the proliferation of antigen-specific B lymphocytes and cytokine production of natural killer T cells in rodents. Hence, butyrate down-regulates the degree of inflammation and tissue degradation of the joint.Citation124
Other metabolites
Other microbiota-related metabolites have been linked to the pathogenesis of EIMs. Tryptophan, normally metabolized by microbiota in the indole pathway, activates the aryl hydrocarbon receptor, promotes Treg differentiation, and participates in the IL-10 and IL-22 pathways.Citation102 Decreased serum tryptophan levels and increased serum kynurenine levels have been observed in patients with AS.Citation125 Tryptophan metabolism has also been markedly disrupted (e.g., up-regulated L-kynureninase) in psoriasis.Citation126
Similarly, patients with IBD-associated rheumatoid arthritis exhibits further microbial tyrosine degradation.Citation127 In addition, lower levels of IgA and medium-chain fatty acids have been reported in the stool of patients with psoriasis, which is correlated with the decreased abundance of Akkermansia, Coprococcus, and Ruminococcus.Citation128 Enriched phenol and p-cresol suppress the expression of keratin 10 and dys-regulate the differentiation of keratinocytes as well as the skin barrier function.Citation129 Moreover, trimethylamine N-oxide is elevated in the blood of patients with psoriasis, inducing M1-type polarization of macrophages, promoting inflammation, and increasing the risk of psoriasis comorbidities.Citation130,Citation131 Decreased circulatory branched-chain amino acids and vitamin B6 have also been demonstrated in patients with PSC.Citation132
Immunocytes and cytokines
In addition to hyper-activation of immunocytes, such as macrophages, DCs, and lymphocytes, gut dysbiosis also participates in the aberrant homing of immunocytes toward extra-intestinal sites and the over-secretion of pro-inflammatory cytokines such as IL-1β, IL-6, and IL-17. MAMPs such as LPS stimulate Toll-like receptors and nucleotide-binding oligomerization domain (NOD)-like receptors.Citation133 Activation of Toll-like receptors and NOD-like receptors result in the differentiation of Th1 and Th17 cells, and the production of pro-inflammatory cytokines (TNF, IL-17, IL-22, etc.), which in turn worsens intestinal hyper-permeability and translocation.Citation134
In the context of SpA and psoriasis, once stimulated by dysbiosis, DCs and macrophages secrete IL-23, activating type 3 innate lymphoid cells and Th17 cells to produce and release pro-inflammatory cytokines, including IL-17, IL-22, and TNF-α.Citation11,Citation135,Citation136 Systemic administration of β-1,3-glucan (a fungal element) reportedly promotes mucosal dysfunction and cytokine secretion, leading to the SpA syndrome in an IL-23/IL-17 dependent pattern (i.e., IL-23 amplifies endoplasmic reticulum stress).Citation137 Similarly, the gut microbiota from arthritis-susceptible mice significantly up-regulate Th17 cells and down-regulate Tregs and DCs in the spleens of germ-free mice.Citation138 The introduction of segmented filamentous bacteria (SFB) could induce arthritis driven by Th17 cells, while neutralization of IL-17 successfully blocks the formation of germinal centers and the progression of arthritis in specific-pathogen-free K/BxN mice.Citation139 Moreover, SFB induces T follicular helper cell differentiation in Peyer’s patches via DC-mediated inhibition of the IL-2-related pathway and directs T follicular helper cell trafficking toward systemic lymphoid tissues responsible for auto-antibody production, which might contribute to SFB-induced arthritis.Citation140 Besides, enrichment of IgA-coated adherent-invasive E. coli (AIEC) was observed in feces of CD patients with SpA compared to those with CD alone, in which subsequent functional analysis showed that AIEC trigger Th17 cells activation to increase systemic immune responses, including joint and intestinal inflammation.Citation141 Parabacteroides distasonis, another intestinal bacterium reduced in patients with rheumatoid arthritis, could inhibit the expression of auto-antibodies and pro-inflammatory cytokines (IL-1β, IL-6, IL-17A, and TNF-α), increase the mRNA levels of anti-inflammatory cytokines (IL-10), reverse the Th17/Treg imbalance in MLN of mice with arthritis, and even induce M2-type polarization of macrophages via its products such as iso-lithocholic acid and 3-oxo-lithocholic acid.Citation142 Similarly, enriched Prevotella spp. have been detected in patients with rheumatoid arthritis, while administration of P. copri exacerbates joint inflammation in animal models of arthritis via over-producing succinate, and up-regulating pro-inflammatory responses in macrophages.Citation143 A special group of macrophages that are enriched in the gut mucosa and synovium, expresses the scavenger receptor CD163 and present bacterial antigens, potentially activating the corresponding T cell groups, and contributing to joint inflammation.Citation88 In addition, another T cell group specific to gut microbiota-related antigens has been isolated from the joint fluid and synovium of patients with reactive arthritis.Citation144 CD4+ T cells might become activated in the gut under the stimulus of macrophage-presenting bacterial antigens, travel into the joints, and induce inflammatory responses. The trafficking of such immunocytes depends on the selectively increased expression of vascular adhesion protein-1, CD44, very late antigen-4, and integrin α4β7, as well as the up-regulation of mucosal vascular addressin cell adhesion molecule 1 in the bone marrow.Citation11,Citation96 Correspondingly, a high expression of IL-17 has been detected in the gut and synovial fluid of patients with SpA. Downstream TNF-α induces synovial fibroblasts to release more matrix-degrading metalloproteinases and destroy tissues in the joints.Citation96
In animal models of uveitis, the intestinal microbiota is sufficient to activate T cells specific for disease development, regardless of the endogenous retinal auto-antigens (i.e., interphotoreceptor retinoid-binding protein). However, after heat inactivation or pre-treatment with Proteinase K, the intestinal contents lose the ability to induce uveal inflammation.Citation76 Furthermore, with the aid of specific transgenic mouse models, lymphocytes originally in the colon have been detected in the eye, supporting that lymphocyte trafficking may participate in uveitis progression.Citation120
In patients with IBD-associated PSC, increased abundance of Sphingomonas sp. and Veillonella sp. is reported. Consequently, more amino oxidases are produced, among which vascular adhesion protein-1 promotes the trafficking and the adherence of original gut lymphocytes toward the hepatic endothelium.Citation122,Citation145 IL-17-producing lymphocytes, such as Th17 cells, are also increased in the peri-ductal area. Moreover, increased recruitment of macrophages in the liver is associated with gut dysbiosis in dextran sodium sulfate-administered animal models of PSC.Citation146
As for periodontitis, it has been reported that translocation of Porphyromonas gingivalis from oral cavity to the intestinal tract could enhances Th17 cells differentiation, which could exacerbate periodontitis.Citation147 Furthermore, this study also found that Th17 cells derived from the intestinal microbiome could migrate to the oral cavity and induce oral inflammation.Citation147 In addition, serum TNF-α and IL-17A, as well as IL-17A+ cells in the alveolar bone are significantly increased compared with the control group, which are reversed upon repairing of the gut barrier, indicating the influence of gut microbiota-related pro-inflammatory cytokine over-secretion.Citation85
Clinical applications
Considering that gut dysbiosis has a significant role in the pathogenesis of EIMs, several microbiota-based therapies have been proposed. For reduced commensal microbiota, medical supplementation is one of the treatment strategies. Direct replenishment of anti-inflammatory microbiota and indirect addition of beneficial metabolites or food ingredients are all acceptable options. On the other hand, for those increased harmful pathogens, antibiotics could be adopted. Fecal microbiota transplantation (FMT) may sometimes be considered if the gut microbiota is greatly disturbed. In the future, more individualized and accurate approaches are expected, including genetically modified micro-organisms, to eradicate the “criminal” pathogens or deliver anti-inflammatory factors in need. Clinical trials and animal experiments exploring microbiota-related treatments of EIMs are shown in .
Table 3. Microbiota-related animal experiments and clinical trials in the management of EIMs.
Probiotics
According to the International Scientific Association for Probiotics and Prebiotics, probiotics are living micro-organisms that provide health benefits when consumed adequately.Citation178 After being introduced into the human body, probiotics not only produce anti-inflammatory metabolites to down-regulate inflammatory factors such as IL-6, IL-12, TNF-α, and relevant signaling pathways such as the NF-κB pathway, but also aid in inhibiting the growth of pathogens, repairing the gut barrier, and regulating the differentiation and proliferation of naïve lymphocytes.Citation134
In BALB/c mice, introduction of Lactobacillus casei prevents intestinal and articular inflammation, with down-regulation of IL-1β, IL-6, IL-17, IL-23, and TNF-α not only in the knees, but also in the mesenteric and popliteal lymph nodes.Citation148 In addition, after supplementation with Lactobacillus acidophilus and Lactobacillus salivarius for 4 weeks, the Bath Ankylosing Spondylitis Disease Activity Index and visual analogue scale were improved in a pilot study involving 18 patients with active AS.Citation151
Bifidobacterium infantis 35624 and a 1:1:1 mixture of probiotics (i.e., B. longum CECT 7347, B. lactis CECT 8145, Lactobacillus rhamnosus CECT 8361) either reduce pro-inflammatory TNF-α and plasma C-reactive protein or reduce the Psoriasis Area and Severity Index in patients after an 8- to 12-week course of treatment.Citation153,Citation154 In BALB/c mice treated with imiquimod, Lactobacillus pentosus GMNL-77 significantly reduced erythematous scaling lesions and mRNA levels of pro-inflammatory cytokines such as IL-23 and IL-27.Citation152 Interestingly, supplement of Bifidobacterium breve CCFM683 effectively down-regulated keratin 16/17, IL-17, and TNF-α expression, improving psoriasis via regulating the FXR/NF-κB pathway and the keratinocyte proliferation.Citation179
Moreover, a combination of L. casei, L. acidophilus, L. reuteri, Bifidobacterium bifidum, and Streptococcus thermophilus successfully reduced retinal histological scores in C57BL/6 mice immunized with interphotoreceptor retinoid-binding protein, an animal model of autoimmune uveitis.Citation156
In the context of periodontitis, introduction of Bifidobacterium lactis HN019 or Lactobacilli reuteri significantly improved the clinical index of periodontitis, including reduced probing depth, less bleeding on probing, and lower surgical risk.Citation157,Citation158 Importantly, no serious adverse events were reported in the clinical trials or the animal experiments described above.
Furthermore, next-generation probiotics such as F. prausnitzii and A. muciniphila have been proposed, and their therapeutic efficiency for IBD has been confirmed. These probiotics reduce infiltrating macrophages, suppress NF-κB signaling pathways, reduce IL-8 production, and eventually down-regulate the severity of colitis.Citation51,Citation82 Considering the similar pattern of gut dysbiosis between IBD and common EIMs, the application of next-generation probiotics in the management of EIMs might also be promising.
Prebiotics
As suggested by the International Scientific Association for Probiotics and Prebiotics, prebiotics refer to substrates that are selectively utilized by micro-organisms to bring health benefits.Citation180 Microbial fermentation of prebiotics, such as inulin and oligofructose, generates metabolites (e.g., SCFAs) that further regulate the gut micro-ecological system and the immune response.Citation180
In the context of SpA, oral administration of long-chain inulin and oligofructose has been reported to significantly reduce the incidence of colitis and arthritis in HLA-B27 transgenic rats.Citation159
As for animal models of periodontitis, oral supplementation of mannan oligosaccharide successfully protects against alveolar bone loss, reduces the expression of IL-10 and IFN-γ, down-regulates levels of TNF-α and IL-1β, and notably restores intestinal villi as well as crypt depth.Citation160
Notably,prebiotics owns widely acceptable safety profile with few serious adverse events reported.
Postbiotics
Under the guidance of the International Scientific Association for Probiotics and Prebiotics, postbiotics refer to dead micro-organisms or their components that benefit the host, including SCFAs, SBAs, etc.Citation181
In the context of SpA, direct exogenous supplementation with SCFAs attenuates arthritis severity in various animal models.Citation121 Besides, oral administration of SCFAs also prevents the activation of effector T cells and the trafficking of immunocytes toward the spleen and cervical lymph nodes, ultimately down-regulating the severity of uveitis in C57BL/6J and Kaede transgenic mouse models.Citation120
Research on PSC in this field is relatively abundant. Several phase II clinical trials have been launched with a course of 12–24 weeks, reporting that either norursodeoxycholic acid (a derivative of SBAs), obeticholic acid (an FXR ligand), or cilofexor (an FXR agonist) significantly reduce alkaline phosphatase in the serum of patients with PSC (with or without IBD).Citation164–166 Furthermore, in C57BL/6J mice with IBD-related liver injury, the addition of milk fat globule membrane is associated with decreased pro-inflammatory cytokines, restoration of Faccalibacumum and Roseburia, attenuated colitis and liver injury, and re-activation of the glutathione transferase pathway.Citation167
For patients with periodontitis, oral intake of heat-killed Lactobacillus plantarum L-137 has effectively reduced the depth of probing in patients who simultaneously underwent supportive periodontal therapy and had a depth no less than 4 mm at baseline.Citation168 Moreover, berberine promotes butyrate production, improves the gut barrier, decreases circulatory LPS and pro-inflammatory cytokine levels, and down-regulates pro-inflammatory cells in alveolar bone, eventually ameliorating alveolar bone loss in animal models of periodontitis.Citation85 Similarly, no severe adverse events have been reported in postbiotics.
Antibiotics
Clinically, antibiotics are used to kill pathogenic bacteria or to inhibit their proliferation.
In the context of SpA, oral administration of meropenem and vancomycin effectively inhibits the development of peripheral enthesitis, accompanied by reduced Th1 and Th17 cells in the spleens of BALB/c and SKG mice.Citation169 As for uveitis, metronidazole or vancomycin has been shown to down-regulate uveal inflammation and increase the abundance of Tregs in extra-intestinal lymphoid tissues of B10.RIII mice pre-treated with interphotoreceptor retinoid-binding protein.Citation170 As for patients with PSC, application of vancomycin, metronidazole, and minocycline resulted in the improvement of liver enzymes and Mayo risk scores.Citation175
Moreover, amoxicillin plus metronidazole, metronidazole alone and azithromycin have been reported to effectively improve clinical outcomes in patients with periodontitis, among which amoxicillin plus metronidazole performs the best to reduce probing pocket depth, bleeding on probing and improve clinical attachment level.Citation176
Fecal microbiota transplantation
Considering the significant involvement of the gut microbiota in the pathogenesis of EIMs, a complete reset of the microbiota would be an option. FMT refers to the therapy transplanting microbiota from healthy human feces into the alimentary tract of patients, enabling rapid restoration of the gut micro-ecologic system.Citation82 However, FMT in the context of EIMs is rare and is mainly reported in patients with PSC (). Notably, the effect of FMT is disturbed by various factors, including the fecal quality of donors (e.g., proportion of SCFA-producing microbiota), the preparation procedure of fecal material, the administration approach and frequency, the individualized gut micro-organism composition before FMT (including bacteria, fungi, and viruses), and the operator techniques.Citation82 Recently, enriched Bacteroides spp. (donor [D]), Eubacterium hallii (patient [P]), Roseburia inulivorans (P), reduced Streptococcus spp. (D), and up-regulated SCFAs and SBAs (P) have been correlated with better prognosis after FMT in patients with IBD.Citation182 Given the similar pathogenesis between IBD and EIMs, possible correlations among such microbiota and the FMT effect in patients with various EIMs could be explored in further studies.
Conclusions and perspectives
In the context of IBD-associated EIMs, elevated mucin-degrading microbiota, pore-inducing microbiota, reduced SCFA-producing microbiota, and SBAs-transforming microbiota are all responsible for the impaired gut barrier, which could promote the translocation of gut microbiota, antigens, or toxins into extra-intestinal sites, including the blood, lymph nodes, synovial fluid, cutaneous tissues, liver, and oral cavity. Translocated microbiota can be engulfed and then presented by DCs and macrophages to promote lymphocyte activation and secretion of pro-inflammatory cytokines, especially IL-23 and IL-17. Moreover, certain bacterial antigens that share similar amino acid sequences or molecular structures with self-antigens, activate auto-reactive immunocytes targeting human tissues. In addition, dys-regulated microbiota-related metabolites, such as BAs, SCFAs, tryptophan, medium-chain fatty acids, and trimethylamine N-oxides modulate the activation of the NLRP3 inflammasome, secretion of inflammation-related cytokines, differentiation of naïve T cells toward Tregs or Th17, trafficking of lymphocytes into extra-intestinal sites, and in turn mediate the gut microbiota composition. Over-activation of the IL-23/Th17/IL-17 axis also takes an influential position in this process. Given the essential roles of microbiota, relevant therapies, including probiotics, prebiotics, postbiotics, antibiotics, and FMT, have been explored to optimize the prognosis for patients with EIMs. ()
Figure 2. Summary of the roles of gut microbiota and relevant therapeutic applications in four common EIMs. EIM, extra-intestinal manifestation; PSC, primary sclerosing cholangitis; MLN, mesenteric lymph node; BA, bile acid; PBA, primary bile acid; SBA, secondary bile acid; DCA, deoxycholic acid; CA, cholic acid; AAU, acute anterior uveitis; LPS, lipopolysaccharide; SCFA, short-chain fatty acid; iFABP, intestinal fatty acid binding protein; TMAO, trimethylamine N-oxide; TNF-α, tumor necrosis factor-α; IL-17, interleukin-17.
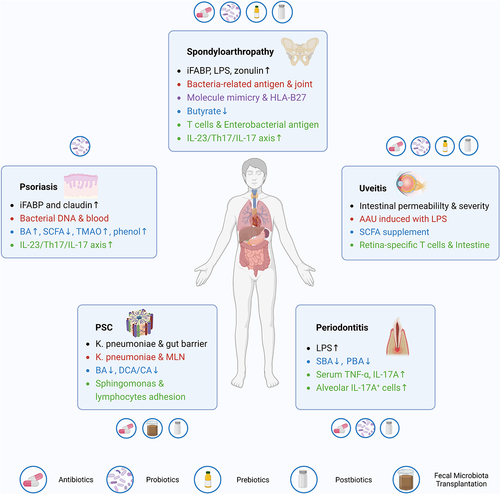
However, current research on EIMs is not always strictly limited to IBD. Abnormal changes in the gut microbiota and metabolites might not be found in patients with coincident IBD and EIMs. Furthermore, EIMs studies focusing on the functions of gut microbiota are confined mainly to SpA, psoriasis, PSC, and periodontitis, leaving the microbiota-related pathogenesis of other EIMs, such as erythema nodosum and pyoderma gangrenosum to be explored. Few animal experiments and clinical trials of EIMs have been conducted for microbiota-related therapies. Further studies are warranted for a clear and comprehensive understanding of the pathophysiology of IBD-associated EIMs to better guide clinical management. In addition, the development of precision medicine and gene-modifying technology would facilitate the identification of the gut microbiome of individuals and offer more effective, convenient, and safer therapeutic options.
Acknowledgments
We acknowledge Biorender, which is used to create schematic Figures.
Disclosure statement
No potential conflict of interest was reported by the author(s).
Additional information
Funding
References
- Agrawal M, Spencer EA, Colombel J-F, Ungaro RC. Approach to the management of recently diagnosed inflammatory bowel disease patients: a user’s guide for adult and pediatric gastroenterologists. Gastroenterology. 2021;161(1):47–24. doi:10.1053/j.gastro.2021.04.063.
- Ng SC, Shi HY, Hamidi N, Underwood FE, Tang W, Benchimol EI, Panaccione R, Ghosh S, Wu JCY, Chan FKL, et al. Worldwide incidence and prevalence of inflammatory bowel disease in the 21st century: a systematic review of population-based studies. Lancet. 2017;390(10114):2769–2778. doi:10.1016/S0140-6736(17)32448-0.
- Mowat C, Cole A, Windsor A, Ahmad T, Arnott I, Driscoll R, Mitton S, Orchard T, Rutter M, Younge L, et al. Guidelines for the management of inflammatory bowel disease in adults. Gut. 2011;60(5):571–607. doi:10.1136/gut.2010.224154.
- Chang JT, Longo DL. Pathophysiology of inflammatory bowel diseases. N Engl J Med. 2020;383(27):2652–2664. doi:10.1056/NEJMra2002697.
- Ni J, Wu GD, Albenberg L, Tomov VT. Gut microbiota and IBD: causation or correlation? Nat Rev Gastro Hepat. 2017;14(10):573–584. doi:10.1038/nrgastro.2017.88.
- Baumgart DC, Le Berre C, Ingelfinger JR, Ingelfinger JR. Newer Biologic and small-molecule therapies for inflammatory bowel disease. N Engl J Med. 2021;385(14):1302–1315. doi:10.1056/NEJMra1907607.
- Hedin CRH, Vavricka SR, Stagg AJ, Schoepfer A, Raine T, Puig L, Pleyer U, Navarini A, van der Meulen-de Jong AE, Maul J, et al. The pathogenesis of Extraintestinal manifestations: implications for IBD Research, Diagnosis, and therapy. J Crohn’s Colitis. 2019;13(5):541–554. doi:10.1093/ecco-jcc/jjy191.
- Harbord M, Annese V, Vavricka SR, Allez M, Barreiro-de AM, Boberg KM, Burisch J, De Vos M, De Vries A-M, Dick AD, et al. The first European evidence-based consensus on extra-intestinal manifestations in inflammatory bowel disease. J Crohn’s Colitis. 2016;10(3):239–254. doi:10.1093/ecco-jcc/jjv213.
- Bandinelli F, Manetti M, Ibba-Manneschi L. Occult spondyloarthritis in inflammatory bowel disease. Clin Rheumatol. 2016;35(2):281–289. doi:10.1007/s10067-015-3074-z.
- Severs M, van Erp SJ, van der Valk ME, Mangen MJ, Fidder HH, van der Have M, van Bodegraven AA, de Jong DJ, van der Woude CJ, Romberg-Camps MJL, et al. Smoking is associated with extra-intestinal manifestations in inflammatory bowel disease. J Crohn’s Colitis. 2016;10(4):455–461. doi:10.1093/ecco-jcc/jjv238.
- Ciccia F, Guggino G, Rizzo A, Saieva L, Peralta S, Giardina A, Cannizzaro A, Sireci G, De Leo G, Alessandro R, et al. Type 3 innate lymphoid cells producing IL-17 and IL-22 are expanded in the gut, in the peripheral blood, synovial fluid and bone marrow of patients with ankylosing spondylitis. Ann Rheum Dis. 2015;74(9):1739–1747. doi:10.1136/annrheumdis-2014-206323.
- Schwartzman M, Ermann J, Kuhn KA, Schwartzman S, Weisman MH. Spondyloarthritis in inflammatory bowel disease cohorts: systematic literature review and critical appraisal of study designs. RMD Open. 2022;8(1):e001777. doi:10.1136/rmdopen-2021-001777.
- Rothfuss KS, Stange EF, Herrlinger KR. Extraintestinal manifestations and complications in inflammatory bowel diseases. World J Gastroentero. 2006;12(30):4819–4831. doi:10.3748/wjg.v12.i30.4819.
- Vavricka SR, Schoepfer A, Scharl M, Lakatos PL, Navarini A, Rogler G. Extraintestinal manifestations of inflammatory bowel disease. Inflamm Bowel Dis. 2015;21(8):1982–1992. doi:10.1097/MIB.0000000000000392.
- Greuter T, Rieder F, Kucharzik T, Peyrin-Biroulet L, Schoepfer AM, Rubin DT, Vavricka SR. Emerging treatment options for extraintestinal manifestations in IBD. Gut. 2021;70(4):796–802. doi:10.1136/gutjnl-2020-322129.
- Orchard TR, Wordsworth BP, Jewell DP. Peripheral arthropathies in inflammatory bowel disease: their articular distribution and natural history. Gut. 1998;42(3):387–391. doi:10.1136/gut.42.3.387.
- Alinaghi F, Tekin HG, Burisch J, Wu JJ, Thyssen JP, Egeberg A. Global prevalence and bidirectional Association between psoriasis and inflammatory bowel disease—A systematic review and meta-analysis. J Crohn’s Colitis. 2020;14(3):351–360. doi:10.1093/ecco-jcc/jjz152.
- Jansen FM, Vavricka SR, den Broeder AA, de Jong EM, Hoentjen F, van Dop WA. Clinical management of the most common extra-intestinal manifestations in patients with inflammatory bowel disease focused on the joints, skin and eyes. United Eur Gastroenterol J. 2020;8(9):1031–1044. doi:10.1177/2050640620958902.
- Greenstein AJ, Janowitz HD, Sachar DB. The extra-intestinal complications of crohnʼs disease and ulcerative colitis: a study of 700 patients. Med. 1976;55(5):401–412. doi:10.1097/00005792-197609000-00004.
- Farhi D, Cosnes J, Zizi N, Chosidow O, Seksik P, Beaugerie L, Aractingi S, Khosrotehrani K. Significance of erythema nodosum and pyoderma gangrenosum in inflammatory bowel diseases: a cohort study of 2402 patients. Med. 2008;87(5):281–293. doi:10.1097/MD.0b013e318187cc9c.
- Pérez-Garza DM, Chavez-Alvarez S, Ocampo-Candiani J, Gomez-Flores M. Erythema nodosum: a practical approach and diagnostic algorithm. Am J Clin Dermatol. 2021;22(3):367–378. doi:10.1007/s40257-021-00592-w.
- Alavi A, French LE, Davis MD, Brassard A, Kirsner RS. Pyoderma gangrenosum: an update on pathophysiology, diagnosis and treatment. Am J Clin Dermatol. 2017;18(3):355–372. doi:10.1007/s40257-017-0251-7.
- States V, O’Brien S, Rai JP, Roberts HL, Paas M, Feagins K, Pierce EJ, Baumgartner RN, Galandiuk S. Pyoderma gangrenosum in inflammatory bowel disease: a systematic review and meta-analysis. Dig Dis Sci. 2020;65(9):2675–2685. doi:10.1007/s10620-019-05999-4.
- Li JX, Chiang CC, Chen SN, Lin JM, Tsai YY. The prevalence of ocular extra-intestinal manifestations in adults inflammatory bowel disease: a systematic review and meta-analysis. Int J Environ Res Public Health. 2022;19(23):15683. doi:10.3390/ijerph192315683.
- Trikudanathan G, Venkatesh PG, Navaneethan U. Diagnosis and therapeutic management of extra-intestinal manifestations of inflammatory bowel disease. Drugs. 2012;72(18):2333–2349. doi:10.2165/11638120-000000000-00000.
- Shah J, Shah A, Hassman L, Gutierrez A. Ocular manifestations of inflammatory bowel disease. Inflamm Bowel Dis. 2021;27(11):1832–1838. doi:10.1093/ibd/izaa359.
- Troncoso LL, Biancardi AL, de Moraes HV Jr., Zaltman C. Ophthalmic manifestations in patients with inflammatory bowel disease: A review. World J Gastroentero. 2017;23(32):5836–5848. doi:10.3748/wjg.v23.i32.5836.
- Barberio B, Massimi D, Cazzagon N, Zingone F, Ford AC, Savarino EV. Prevalence of primary sclerosing cholangitis in patients with inflammatory bowel disease: A systematic review and meta-analysis. Gastroenterology. 2021;161(6):1865–1877. doi:10.1053/j.gastro.2021.08.032.
- Zhang L, Gao X, Zhou J, Chen S, Zhang J, Zhang Y, Chen B, Yang J. Increased risks of dental caries and periodontal disease in Chinese patients with inflammatory bowel disease. Int Dent J. 2020;70(3):227–236. doi:10.1111/idj.12542.
- Chau SF, Lee CY, Huang JY, Chou MC, Chen HC, Yang SF. The existence of periodontal disease and subsequent ocular diseases: a population-based cohort study. Medicina (Kaunas, Lithuania). 2020;56(11):621. doi:10.3390/medicina56110621.
- Madsen GR, Bertl K, Pandis N, Stavropoulos A, Burisch J. The impact of periodontitis on inflammatory bowel disease activity. Inflamm Bowel Dis. 2023;29(3):396–404. doi:10.1093/ibd/izac090.
- Kwon T, Lamster IB, Levin L. Current Concepts in the management of periodontitis. Int Dent J. 2021;71(6):462–476. doi:10.1111/idj.12630.
- Rogler G, Singh A, Kavanaugh A, Rubin DT. Extraintestinal manifestations of inflammatory bowel disease: current concepts, treatment, and implications for disease management. Gastroenterology. 2021;161(4):1118–1132. doi:10.1053/j.gastro.2021.07.042.
- Vavricka SR, Rogler G, Gantenbein C, Spoerri M, Prinz VM, Navarini AA, French LE, Safroneeva E, Fournier N, Straumann A, et al. Chronological order of appearance of extraintestinal manifestations relative to the time of IBD diagnosis in the Swiss inflammatory bowel disease cohort. Inflamm Bowel Dis. 2015;21(8):1794–1800. doi:10.1097/MIB.0000000000000429.
- Burisch J, Jess T, Egeberg A. Incidence of immune-mediated inflammatory diseases among patients with inflammatory bowel diseases in Denmark. Clin Gastroenterol Hepatol. 2019;17(13):2704–12.e3. doi:10.1016/j.cgh.2019.03.040.
- Ferretti F, Monico MC, Cannatelli R, Carmagnola S, Lenti MV, Di Sabatino A, Conforti F, Pastorelli L, Caprioli F, Bezzio C, et al. The impact of biologic therapies on extra-intestinal manifestations in inflammatory bowel disease: a multicenter study. Front Med. 2022;9:933357. doi:10.3389/fmed.2022.933357.
- Satsangi J, Grootscholten C, Holt H, Jewell DP. Clinical patterns of familial inflammatory bowel disease. Gut. 1996;38(5):738–741. doi:10.1136/gut.38.5.738.
- Dahal RH, Kim S, Kim YK, Kim ES, Kim J. Insight into gut dysbiosis of patients with inflammatory bowel disease and ischemic colitis. Front Microbiol. 2023;14:1174832. doi:10.3389/fmicb.2023.1174832.
- Reichardt N, Duncan SH, Young P, Belenguer A, McWilliam Leitch C, Scott KP, Flint HJ, Louis P. Phylogenetic distribution of three pathways for propionate production within the human gut microbiota. ISME J. 2014;8(6):1323–1335. doi:10.1038/ismej.2014.14.
- Png CW, Lindén SK, Gilshenan KS, Zoetendal EG, McSweeney CS, Sly LI, McGuckin MA, Florin THJ. Mucolytic bacteria with increased prevalence in IBD mucosa augment in vitro utilization of mucin by other bacteria. Am J Gastroenterol. 2010;105(11):2420–2428. doi:10.1038/ajg.2010.281.
- Breban M, Tap J, Leboime A, Said-Nahal R, Langella P, Chiocchia G, Furet J-P, Sokol H. Faecal microbiota study reveals specific dysbiosis in spondyloarthritis. Ann Rheum Dis. 2017;76(9):1614–1622. doi:10.1136/annrheumdis-2016-211064.
- Henke MT, Kenny DJ, Cassilly CD, Vlamakis H, Xavier RJ, Clardy J. Ruminococcus gnavus, a member of the human gut microbiome associated with Crohn’s disease, produces an inflammatory polysaccharide. Proc Natl Acad Sci U S A. 2019;116(26):12672–12677. doi:10.1073/pnas.1904099116.
- Dorofeyev AE, Vasilenko IV, Rassokhina OA. Joint extraintestinal manifestations in ulcerative colitis. Dig Dis (Basel, Switzerland). 2009;27(4):502–510. doi:10.1159/000233289.
- Lee IA, Kim DH. Klebsiella pneumoniae increases the risk of inflammation and colitis in a murine model of intestinal bowel disease. Scand J Gastroenterol. 2011;46(6):684–693. doi:10.3109/00365521.2011.560678.
- Ciccia F, Guggino G, Rizzo A, Alessandro R, Luchetti MM, Milling S, Saieva L, Cypers H, Stampone T, Di Benedetto P, et al. Dysbiosis and zonulin upregulation alter gut epithelial and vascular barriers in patients with ankylosing spondylitis. Ann Rheum Dis. 2017;76(6):1123–1132. doi:10.1136/annrheumdis-2016-210000.
- Cardoneanu A, Cozma S, Rezus C, Petrariu F, Burlui AM, Rezus E. Characteristics of the intestinal microbiome in ankylosing spondylitis. Exp Ther Med. 2021;22(1):676. doi:10.3892/etm.2021.10108.
- El Asmar R, Panigrahi P, Bamford P, Berti I, Not T, Coppa GV, Catassi C, Fasano A. Host-dependent zonulin secretion causes the impairment of the small intestine barrier function after bacterial exposure. Gastroenterology. 2002;123(5):1607–1615. doi:10.1053/gast.2002.36578.
- Gevers D, Kugathasan S, Denson LA, Vázquez-Baeza Y, Van Treuren W, Ren B, Schwager E, Knights D, Song S, Yassour M, et al. The treatment-naive microbiome in new-onset Crohn’s disease. Cell Host & Microbe. 2014;15(3):382–392. doi:10.1016/j.chom.2014.02.005.
- Machiels K, Joossens M, Sabino J, De Preter V, Arijs I, Eeckhaut V, Ballet V, Claes K, Van Immerseel F, Verbeke K, et al. A decrease of the butyrate-producing species Roseburia hominis and Faecalibacterium prausnitzii defines dysbiosis in patients with ulcerative colitis. Gut. 2014;63(8):1275–1283. doi:10.1136/gutjnl-2013-304833.
- Min HK, Na HS, Jhun J, Lee SY, Choi SS, Park GE, Lee JS, Um IG, Lee SY, Seo H, et al. Identification of gut dysbiosis in axial spondyloarthritis patients and improvement of experimental ankylosing spondyloarthritis by microbiome-derived butyrate with immune-modulating function. Front Immunol. 2023;14:1096565. doi:10.3389/fimmu.2023.1096565.
- Sokol H, Pigneur B, Watterlot L, Lakhdari O, Bermúdez-Humarán LG, Gratadoux JJ, Blugeon S, Bridonneau C, Furet J-P, Corthier G, et al. Faecalibacterium prausnitzii is an anti-inflammatory commensal bacterium identified by gut microbiota analysis of Crohn disease patients. Proc Natl Acad Sci U S A. 2008;105(43):16731–16736. doi:10.1073/pnas.0804812105.
- Schade L, Mesa D, Faria AR, Santamaria JR, Xavier CA, Ribeiro D, Hajar FN, Azevedo VF. The gut microbiota profile in psoriasis: a Brazilian case-control study. Lett Appl Microbiol. 2022;74(4):498–504. doi:10.1111/lam.13630.
- Verma R, Verma AK, Ahuja V, Paul J. Real-time analysis of mucosal flora in patients with inflammatory bowel disease in India. J Clin Microbiol. 2010;48(11):4279–4282. doi:10.1128/JCM.01360-10.
- Kakiyama G, Pandak WM, Gillevet PM, Hylemon PB, Heuman DM, Daita K, Takei H, Muto A, Nittono H, Ridlon JM, et al. Modulation of the fecal bile acid profile by gut microbiota in cirrhosis. J Hepatol. 2013;58(5):949–955. doi:10.1016/j.jhep.2013.01.003.
- Plovier H, Everard A, Druart C, Depommier C, Van Hul M, Geurts L, Chilloux J, Ottman N, Duparc T, Lichtenstein L, et al. A purified membrane protein from Akkermansia muciniphila or the pasteurized bacterium improves metabolism in obese and diabetic mice. Nat Med. 2017;23(1):107–113. doi:10.1038/nm.4236.
- Ansaldo E, Slayden LC, Ching KL, Koch MA, Wolf NK, Plichta DR, Brown EM, Graham DB, Xavier RJ, Moon JJ, et al. Akkermansia muciniphila induces intestinal adaptive immune responses during homeostasis. Sci. 2019;364(6446):1179–1184. doi:10.1126/science.aaw7479.
- Eppinga H, Sperna WCJ, Thio HB, van der Woude CJ, Nijsten TE, Peppelenbosch MP, Konstantinov SR. Similar depletion of protective Faecalibacterium prausnitzii in psoriasis and inflammatory bowel disease, but not in Hidradenitis Suppurativa. J Crohn’s Colitis. 2016;10(9):1067–1075. doi:10.1093/ecco-jcc/jjw070.
- Sokol H, Leducq V, Aschard H, Pham HP, Jegou S, Landman C, Cohen D, Liguori G, Bourrier A, Nion-Larmurier I, et al. Fungal microbiota dysbiosis in IBD. Gut. 2017;66(6):1039–1048. doi:10.1136/gutjnl-2015-310746.
- Eppinga H, Thio HB, Schreurs MWJ, Blakaj B, Tahitu RI, Konstantinov SR, Peppelenbosch MP, Fuhler GM. Depletion of Saccharomyces cerevisiae in psoriasis patients, restored by Dimethylfumarate therapy (DMF). PloS One. 2017;12(5):e0176955. doi:10.1371/journal.pone.0176955.
- Sivignon A, de Vallée A, Barnich N, Denizot J, Darcha C, Pignède G, Vandekerckove P, Darfeuille-Michaud A. Saccharomyces cerevisiae CNCM I-3856 prevents colitis induced by AIEC bacteria in the transgenic mouse model Mimicking Crohnʼs disease. Inflamm Bowel Dis. 2015;21(2):276–286. doi:10.1097/MIB.0000000000000280.
- Hu Y, Chen Z, Xu C, Kan S, Chen D. Disturbances of the gut microbiota and microbiota-derived metabolites in inflammatory bowel disease. Nutrients. 2022;14(23):14. doi:10.3390/nu14235140.
- Kalyana CS, Jayasudha R, Sai PG, Ali MH, Sharma S, Tyagi M, Shivaji S. Dysbiosis in the gut bacterial microbiome of patients with uveitis, an inflammatory disease of the eye. Indian J Microbiol. 2018;58(4):457–469. doi:10.1007/s12088-018-0746-9.
- Pan M, Barua N, Ip M. Mucin-degrading gut commensals isolated from healthy faecal donor suppress intestinal epithelial inflammation and regulate tight junction barrier function. Front Immunol. 2022;13:1021094. doi:10.3389/fimmu.2022.1021094.
- Bajer L, Kverka M, Kostovcik M, Macinga P, Dvorak J, Stehlikova Z, Brezina J, Wohl P, Spicak J, Drastich P, et al. Distinct gut microbiota profiles in patients with primary sclerosing cholangitis and ulcerative colitis. World J Gastroentero. 2017;23(25):4548–4558. doi:10.3748/wjg.v23.i25.4548.
- Steck N, Hoffmann M, Sava IG, Kim SC, Hahne H, Tonkonogy SL, Mair K, Krueger D, Pruteanu M, Shanahan F, et al. Enterococcus faecalis metalloprotease compromises epithelial barrier and contributes to intestinal inflammation. Gastroenterology. 2011;141(3):959–971. doi:10.1053/j.gastro.2011.05.035.
- Liwinski T, Zenouzi R, John C, Ehlken H, Rühlemann MC, Bang C, Groth S, Lieb W, Kantowski M, Andersen N, et al. Alterations of the bile microbiome in primary sclerosing cholangitis. Gut. 2020;69(4):665–672. doi:10.1136/gutjnl-2019-318416.
- Nakamoto N, Sasaki N, Aoki R, Miyamoto K, Suda W, Teratani T, Suzuki T, Koda Y, Chu P-S, Taniki N, et al. Gut pathobionts underlie intestinal barrier dysfunction and liver T helper 17 cell immune response in primary sclerosing cholangitis. Nat microbiol. 2019;4(3):492–503. doi:10.1038/s41564-018-0333-1.
- Lemoinne S, Kemgang A, Ben BK, Straube M, Jegou S, Corpechot C, Chazouillères O, Housset C, Sokol H. Fungi participate in the dysbiosis of gut microbiota in patients with primary sclerosing cholangitis. Gut. 2020;69(1):92–102. doi:10.1136/gutjnl-2018-317791.
- Wu L, Han J, Nie JY, Deng T, Li C, Fang C, Xie W-Z, Wang S-Y, Zeng X-T. Alterations and correlations of gut microbiota and fecal metabolome characteristics in experimental periodontitis rats. Front Microbiol. 2022;13:865191. doi:10.3389/fmicb.2022.865191.
- Vavricka SR, Galván JA, Dawson H, Soltermann A, Biedermann L, Scharl M, Schoepfer AM, Rogler G, Prinz Vavricka MB, Terracciano L, et al. Expression patterns of TNFα, MAdCAM1, and STAT3 in intestinal and skin manifestations of inflammatory bowel disease. J Crohn’s Colitis. 2018;12(3):347–354. doi:10.1093/ecco-jcc/jjx158.
- Grant AJ, Lalor PF, Hübscher SG, Briskin M, Adams DH. MAdCAM-1 expressed in chronic inflammatory liver disease supports mucosal lymphocyte adhesion to hepatic endothelium (MAdCAM-1 in chronic inflammatory liver disease). Hepatol. 2001;33(5):1065–1072. doi:10.1053/jhep.2001.24231.
- Roda G, Chien Ng S, Kotze PG, Argollo M, Panaccione R, Spinelli A, Kaser A, Peyrin-Biroulet L, Danese S. Crohn’s disease. Nat Rev Dis Primers. 2020;6(1):22. doi:10.1038/s41572-020-0156-2.
- Kobayashi T, Siegmund B, Le Berre C, Wei SC, Ferrante M, Shen B, Bernstein CN, Danese S, Peyrin-Biroulet L, Hibi T, et al. Ulcerative colitis. Nat Rev Dis Primers. 2020;6(1):74. doi:10.1038/s41572-020-0205-x.
- Schaubeck M, Clavel T, Calasan J, Lagkouvardos I, Haange SB, Jehmlich N, et al. Dysbiotic gut microbiota causes transmissible Crohn’s disease-like ileitis independent of failure in antimicrobial defence. Gut. 2016;65:225–237. doi:10.1136/gutjnl-2015-309333.
- Taurog JD, Richardson JA, Croft JT, Simmons WA, Zhou M, Fernández-Sueiro JL, Balish E, Hammer RE. The germfree state prevents development of gut and joint inflammatory disease in HLA-B27 transgenic rats. J Exp Med. 1994;180(6):2359–2364. doi:10.1084/jem.180.6.2359.
- Horai R, Zárate-Bladés CR, Dillenburg-Pilla P, Chen J, Kielczewski JL, Silver PB, Jittayasothorn Y, Chan C-C, Yamane H, Honda K, et al. Microbiota-dependent activation of an autoreactive T cell receptor provokes autoimmunity in an immunologically privileged site. Immunity. 2015;43(2):343–353. doi:10.1016/j.immuni.2015.07.014.
- Camilleri M. Leaky gut: mechanisms, measurement and clinical implications in humans. Gut. 2019;68(8):1516–1526. doi:10.1136/gutjnl-2019-318427.
- Yu LC. Microbiota dysbiosis and barrier dysfunction in inflammatory bowel disease and colorectal cancers: exploring a common ground hypothesis. J Biomed Sci. 2018;25(1):79. doi:10.1186/s12929-018-0483-8.
- Tailford LE, Crost EH, Kavanaugh D, Juge N. Mucin glycan foraging in the human gut microbiome. Front Genet. 2015;6:81. doi:10.3389/fgene.2015.00081.
- Sikora M, Stec A, Chrabaszcz M, Waskiel-Burnat A, Zaremba M, Olszewska M, Rudnicka L. Intestinal fatty acid binding protein, a biomarker of intestinal barrier, is associated with severity of psoriasis. J Clin Med. 2019;8(7):1021. doi:10.3390/jcm8071021.
- Olejniczak-Staruch I, Ciążyńska M, Sobolewska-Sztychny D, Narbutt J, Skibińska M, Lesiak A. Alterations of the skin and gut microbiome in Psoriasis and Psoriatic arthritis. Int J Mol Sci. 2021;22(8):22. doi:10.3390/ijms22083998.
- Liu S, Zhao W, Lan P, Mou X. The microbiome in inflammatory bowel diseases: from pathogenesis to therapy. Protein Cell. 2021;12(5):331–345. doi:10.1007/s13238-020-00745-3.
- Parthasarathy R, Santiago F, McCluskey P, Kaakoush NO, Tedla N, Wakefield D. The microbiome in HLA-B27-associated disease: implications for acute anterior uveitis and recommendations for future studies. Trends Microbiol. 2022;31(2):142–158. doi:10.1016/j.tim.2022.08.008.
- Janowitz C, Nakamura YK, Metea C, Gligor A, Yu W, Karstens L, Rosenbaum JT, Asquith M, Lin P. Disruption of intestinal homeostasis and intestinal microbiota during experimental autoimmune uveitis. Invest Ophthalmol Visual Sci. 2019;60(1):420–429. doi:10.1167/iovs.18-24813.
- Jia X, Jia L, Mo L, Yuan S, Zheng X, He J, Chen V, Guo Q, Zheng L, Yuan Q, et al. Berberine Ameliorates periodontal bone loss by regulating gut microbiota. J Dent Res. 2019;98(1):107–116. doi:10.1177/0022034518797275.
- Berthelot JM, Claudepierre P. Trafficking of antigens from gut to sacroiliac joints and spine in reactive arthritis and spondyloarthropathies: Mainly through lymphatics? Joint Bone Spine. 2016;83(5):485–490. doi:10.1016/j.jbspin.2015.10.015.
- Ramírez-Boscá A, Navarro-López V, Martínez-Andrés A, Such J, Francés R, Horga DLPJ, Asín-Llorca M. Identification of bacterial DNA in the peripheral blood of patients with active psoriasis. JAMA Dermatol. 2015;151(6):670–671. doi:10.1001/jamadermatol.2014.5585.
- Baeten D, Demetter P, Cuvelier CA, Kruithof E, Van Damme N, De Vos M, Veys EM, De Keyser F. Macrophages expressing the scavenger receptor CD163: a link between immune alterations of the gut and synovial inflammation in spondyloarthropathy. J Pathol. 2002;196(3):343–350. doi:10.1002/path.1044.
- Fragoulis GE, Liava C, Daoussis D, Akriviadis E, Garyfallos A, Dimitroulas T. Inflammatory bowel diseases and spondyloarthropathies: from pathogenesis to treatment. World J Gastroentero. 2019;25(18):2162–2176. doi:10.3748/wjg.v25.i18.2162.
- Lamps LW, Madhusudhan KT, Havens JM, Greenson JK, Bronner MP, Chiles MC, Dean, P J., Scott, M A. Pathogenic Yersinia DNA is detected in bowel and mesenteric lymph nodes from patients with Crohn’s disease. The American Journal Of Surgical Pathology. 2003;27(2):220–227. doi:10.1097/00000478-200302000-00011.
- Luchetti MM, Ciccia F, Avellini C, Benfaremo D, Rizzo A, Spadoni T, Svegliati S, Marzioni D, Santinelli A, Costantini A, et al. Gut epithelial impairment, microbial translocation and immune system activation in inflammatory bowel disease–associated spondyloarthritis. Rheumatology (Oxford). 2021;60(1):92–102. doi:10.1093/rheumatology/keaa164.
- Wells A, Pararajasegaram G, Baldwin M, Yang CH, Hammer M, Fox A. Uveitis and arthritis induced by systemic injection of streptococcal cell walls. Invest Ophthalmol Visual Sci. 1986;27:921–925.
- Wohlgemuth S, Keller S, Kertscher R, Stadion M, Haller D, Kisling S, Jahreis G, Blaut M, Loh G. Intestinal steroid profiles and microbiota composition in colitic mice. Gut Microbes. 2011;2(3):159–166. doi:10.4161/gmic.2.3.16104.
- Atarashi K, Suda W, Luo C, Kawaguchi T, Motoo I, Narushima S, Kiguchi Y, Yasuma K, Watanabe E, Tanoue T, et al. Ectopic colonization of oral bacteria in the intestine drives TH1 cell induction and inflammation. Sci. 2017;358(6361):359–365. doi:10.1126/science.aan4526.
- Kitamoto S, Nagao-Kitamoto H, Jiao Y, Gillilland MG 3rd, Hayashi A, Imai J, Sugihara K, Miyoshi M, Brazil JC, Kuffa P, et al. The intermucosal connection between the mouth and gut in commensal pathobiont-driven colitis. Cell. 2020;182(2):447–62.e14. doi:10.1016/j.cell.2020.05.048.
- Fantini MC, Pallone F, Monteleone G. Common immunologic mechanisms in inflammatory bowel disease and spondylarthropathies. World J Gastroentero. 2009;15(20):2472–2478. doi:10.3748/wjg.15.2472.
- Scofield RH, Kurien B, Gross T, Warren WL, Harley JB, Scofield RH, Harley JB, Scofield RH, Harley JB. HLA-B27 binding of peptide from its own sequence and similar peptides from bacteria: implications for spondyloarthropathies. Lancet. 1995;345(8964):1542–1544. doi:10.1016/S0140-6736(95)91089-1.
- Ramos M, Alvarez I, Sesma L, Logean A, Rognan D, López de Castro JA. Molecular mimicry of an HLA-B27-derived ligand of arthritis-linked subtypes with chlamydial proteins. J Biol Chem. 2002;277(40):37573–37581. doi:10.1074/jbc.M205470200.
- Orda R, Samra Z, Levy Y, Shperber Y, Scapa E. Chlamydia trachomatis and inflammatory bowel disease–a coincidence? J R Soc Med. 1990;83(1):15–17. doi:10.1177/014107689008300108.
- Schwimmbeck PL, Yu DT, Oldstone MB. Autoantibodies to HLA B27 in the sera of HLA B27 patients with ankylosing spondylitis and Reiter’s syndrome. Molecular mimicry with Klebsiella pneumoniae as potential mechanism of autoimmune disease. J Exp Med. 1987;166(1):173–181. doi:10.1084/jem.166.1.173.
- Fielder M, Pirt SJ, Tarpey I, Wilson C, Cunningham P, Ettelaie C, Binder A, Bansal S, Ebringer A. Molecular mimicry and ankylosing spondylitis: possible role of a novel sequence in pullulanase of Klebsiella pneumoniae. FEBS Lett. 1995;369(2–3):243–248. doi:10.1016/0014-5793(95)00760-7.
- Lavelle A, Sokol H. Gut microbiota-derived metabolites as key actors in inflammatory bowel disease. Nat Rev Gastro Hepat. 2020;17(4):223–237. doi:10.1038/s41575-019-0258-z.
- Heinken A, Ravcheev DA, Baldini F, Heirendt L, Fleming RMT, Thiele I. Systematic assessment of secondary bile acid metabolism in gut microbes reveals distinct metabolic capabilities in inflammatory bowel disease. Microbiome. 2019;7(1):75. doi:10.1186/s40168-019-0689-3.
- Parks DJ, Blanchard SG, Bledsoe RK, Chandra G, Consler TG, Kliewer SA, Stimmel JB, Willson TM, Zavacki AM, Moore DD, et al. Bile acids: natural ligands for an orphan nuclear receptor. Sci. 1999;284(5418):1365–1368. doi:10.1126/science.284.5418.1365.
- Kawamata Y, Fujii R, Hosoya M, Harada M, Yoshida H, Miwa M, Fukusumi S, Habata Y, Itoh T, Shintani Y, et al. A G protein-coupled receptor responsive to bile acids. J Biol Chem. 2003;278(11):9435–9440. doi:10.1074/jbc.M209706200.
- Vavassori P, Mencarelli A, Renga B, Distrutti E, Fiorucci S. The bile acid receptor FXR is a modulator of intestinal innate immunity. J Immunol. 2009;183(10):6251–6261. doi:10.4049/jimmunol.0803978.
- Inagaki T, Moschetta A, Lee YK, Peng L, Zhao G, Downes M, Yu RT, Shelton JM, Richardson JA, Repa JJ, et al. Regulation of antibacterial defense in the small intestine by the nuclear bile acid receptor. Proc Natl Acad Sci U S A. 2006;103(10):3920–3925. doi:10.1073/pnas.0509592103.
- Song X, Sun X, Oh SF, Wu M, Zhang Y, Zheng W, Geva-Zatorsky N, Jupp R, Mathis D, Benoist C, et al. Microbial bile acid metabolites modulate gut RORγ+ regulatory T cell homeostasis. Nature. 2020;577(7790):410–415. doi:10.1038/s41586-019-1865-0.
- Yoneno K, Hisamatsu T, Shimamura K, Kamada N, Ichikawa R, Kitazume MT, Mori M, Uo M, Namikawa Y, Matsuoka K, et al. TGR 5 signalling inhibits the production of pro-inflammatory cytokines by in vitro differentiated inflammatory and intestinal macrophages in Crohn’s disease. Immunology. 2013;139(1):19–29. doi:10.1111/imm.12045.
- Campbell C, McKenney PT, Konstantinovsky D, Isaeva OI, Schizas M, Verter J, Mai C, Jin W-B, Guo C-J, Violante S, et al. Bacterial metabolism of bile acids promotes generation of peripheral regulatory T cells. Nature. 2020;581(7809):475–479. doi:10.1038/s41586-020-2193-0.
- Hang S, Paik D, Yao L, Kim E, Trinath J, Lu J, Ha S, Nelson BN, Kelly SP, Wu L, et al. Bile acid metabolites control T(H)17 and T(reg) cell differentiation. Nature. 2019;576(7785):143–148. doi:10.1038/s41586-019-1785-z.
- Murakami M, Iwamoto J, Honda A, Tsuji T, Tamamushi M, Ueda H, Monma T, Konishi N, Yara S, Hirayama T, et al. Detection of gut dysbiosis due to reduced Clostridium Subcluster XIVa using the fecal or serum bile acid profile. Inflamm Bowel Dis. 2018;24(5):1035–1044. doi:10.1093/ibd/izy022.
- Bamba S, Inatomi O, Nishida A, Ohno M, Imai T, Takahashi K, Naito Y, Iwamoto J, Honda A, Inohara N, et al. Relationship between the gut microbiota and bile acid composition in the ileal mucosa of Crohn’s disease. Intest Res. 2022;20(3):370–380. doi:10.5217/ir.2021.00054.
- Hu J, Wang C, Huang X, Yi S, Pan S, Zhang Y, Yuan G, Cao Q, Ye X, Li H, et al. Gut microbiota-mediated secondary bile acids regulate dendritic cells to attenuate autoimmune uveitis through TGR5 signaling. Cell Rep. 2021;36(12):109726. doi:10.1016/j.celrep.2021.109726.
- Torres J, Palmela C, Brito H, Bao X, Ruiqi H, Moura-Santos P, Pereira da Silva J, Oliveira A, Vieira C, Perez K, et al. The gut microbiota, bile acids and their correlation in primary sclerosing cholangitis associated with inflammatory bowel disease. United Eur Gastroenterol J. 2018;6(1):112–122. doi:10.1177/2050640617708953.
- Rühlemann M, Liwinski T, Heinsen FA, Bang C, Zenouzi R, Kummen M, Thingholm L, Tempel M, Lieb W, Karlsen T, et al. Consistent alterations in faecal microbiomes of patients with primary sclerosing cholangitis independent of associated colitis. Aliment Pharmacol Ther. 2019;50(5):580–589. doi:10.1111/apt.15375.
- Yang R, Yu W, Lin L, Jin M, Hu S, Jiang B, Mao C, Li G, Tang J, Gu Y, et al. Profiling of bile acids and activated receptor S1PR2 in gingival tissues of periodontitis patients. J Periodontol. 2023;94(4):564–574. doi:10.1002/JPER.22-0398.
- Macia L, Tan J, Vieira AT, Leach K, Stanley D, Luong S, Maruya M, Ian McKenzie C, Hijikata A, Wong C, et al. Metabolite-sensing receptors GPR43 and GPR109A facilitate dietary fibre-induced gut homeostasis through regulation of the inflammasome. Nat Commun. 2015;6(1):6734. doi:10.1038/ncomms7734.
- Gaudier E, Jarry A, Blottière HM, de Coppet P, Buisine MP, Aubert JP, Laboisse C, Cherbut C, Hoebler C. Butyrate specifically modulates MUC gene expression in intestinal epithelial goblet cells deprived of glucose. Am J Physiol Gastrointest Liver Physiol. 2004;287(6):G1168–74. doi:10.1152/ajpgi.00219.2004.
- Nakamura YK, Janowitz C, Metea C, Asquith M, Karstens L, Rosenbaum JT, Lin P. Short chain fatty acids ameliorate immune-mediated uveitis partially by altering migration of lymphocytes from the intestine. Sci Rep. 2017;7(1):11745. doi:10.1038/s41598-017-12163-3.
- Yang W, Cong Y. Gut microbiota-derived metabolites in the regulation of host immune responses and immune-related inflammatory diseases. Cell Mol Immunol. 2021;18(4):866–877. doi:10.1038/s41423-021-00661-4.
- Little R, Wine E, Kamath BM, Griffiths AM, Ricciuto A. Gut microbiome in primary sclerosing cholangitis: a review. World J Gastroentero. 2020;26(21):2768–2780. doi:10.3748/wjg.v26.i21.2768.
- Rosser EC, Piper CJM, Matei DE, Blair PA, Rendeiro AF, Orford M, Alber DG, Krausgruber T, Catalan D, Klein N, et al. Microbiota-derived metabolites suppress arthritis by amplifying Aryl-Hydrocarbon receptor activation in regulatory B cells. Cell Metab. 2020;31(4):837–51.e10. doi:10.1016/j.cmet.2020.03.003.
- Bungau SG, Behl T, Singh A, Sehgal A, Singh S, Chigurupati S, Vijayabalan S, Das S, Palanimuthu VR. Targeting probiotics in rheumatoid arthritis. Nutrients. 2021;13(10):3376. doi:10.3390/nu13103376.
- Eryavuz OD, Sivrikaya A, Isik K, Abusoglu S, Albayrak GI, Humeyra YF, Abusoglu G, Unlu A, Tezcan D. Altered kynurenine pathway metabolism in patients with ankylosing spondylitis. Int Immunopharmacol. 2021;99:108018. doi:10.1016/j.intimp.2021.108018.
- Harden JL, Lewis SM, Lish SR, Suárez-Fariñas M, Gareau D, Lentini T, Johnson-Huang LM, Krueger JG, Lowes MA. The tryptophan metabolism enzyme L-kynureninase is a novel inflammatory factor in psoriasis and other inflammatory diseases. J Allergy Clin Immunol. 2016;137(6):1830–1840. doi:10.1016/j.jaci.2015.09.055.
- Muñiz DA, Chen J, Hillmann B, Jeraldo P, Al-Ghalith G, Taneja V, Davis JM, Knights D, Nelson H, Faubion WA, et al. An increased abundance of clostridiaceae characterizes arthritis in inflammatory bowel disease and rheumatoid arthritis: a cross-sectional study. Inflamm Bowel Dis. 2019;25(5):902–913. doi:10.1093/ibd/izy318.
- Scher JU, Ubeda C, Artacho A, Attur M, Isaac S, Reddy SM, Marmon S, Neimann A, Brusca S, Patel T, et al. Decreased bacterial diversity characterizes the altered gut microbiota in patients with psoriatic arthritis, resembling dysbiosis in inflammatory bowel disease. Arthritis Rheumatol. 2015;67(1):128–139. doi:10.1002/art.38892.
- Miyazaki K, Masuoka N, Kano M, Iizuka R. Bifidobacterium fermented milk and galacto-oligosaccharides lead to improved skin health by decreasing phenols production by gut microbiota. Benef Microbes. 2014;5(2):121–128. doi:10.3920/BM2012.0066.
- Sikora M, Stec A, Chrabaszcz M, Giebultowicz J, Samborowska E, Jazwiec R, Dadlez M, Olszewska M, Rudnicka L. Clinical implications of intestinal barrier damage in psoriasis. J Inflamm Res. 2021;14:237–243. doi:10.2147/JIR.S292544.
- Wu K, Yuan Y, Yu H, Dai X, Wang S, Sun Z, Wang F, Fei H, Lin Q, Jiang H, et al. The gut microbial metabolite trimethylamine N-oxide aggravates GVHD by inducing M1 macrophage polarization in mice. Blood. 2020;136(4):501–515. doi:10.1182/blood.2019003990.
- Kummen M, Thingholm LB, Rühlemann MC, Holm K, Hansen SH, Moitinho-Silva L, Liwinski T, Zenouzi R, Storm-Larsen C, Midttun Ø, et al. Altered gut microbial metabolism of essential Nutrients in primary sclerosing cholangitis. Gastroenterology. 2021;160(5):1784–98.e0. doi:10.1053/j.gastro.2020.12.058.
- Baumgart DC, Sandborn WJ. Crohn’s disease. The Lancet. 2012;380(9853):1590–1605. doi:10.1016/S0140-6736(12)60026-9.
- Glassner KL, Abraham BP, Quigley EMM. The microbiome and inflammatory bowel disease. J Allergy Clin Immunol. 2020;145(1):16–27. doi:10.1016/j.jaci.2019.11.003.
- De Francesco MA, Caruso A. The gut microbiome in psoriasis and Crohn’s disease: Is its perturbation a common denominator for their pathogenesis? Vaccines (Basel). 2022;10(2):10. doi:10.3390/vaccines10020244.
- Kabeerdoss J, Sandhya P, Danda D. Gut inflammation and microbiome in spondyloarthritis. Rheumatol Int. 2016;36(4):457–468. doi:10.1007/s00296-015-3414-y.
- Benham H, Rehaume LM, Hasnain SZ, Velasco J, Baillet AC, Ruutu M, Kikly K, Wang R, Tseng H-W, Thomas GP, et al. Interleukin-23 mediates the intestinal response to microbial β-1,3-glucan and the development of spondyloarthritis pathology in SKG mice. Arthritis Rheumatol. 2014;66(7):1755–1767. doi:10.1002/art.38638.
- Liu X, Zeng B, Zhang J, Li W, Mou F, Wang H, Zou Q, Zhong B, Wu L, Wei H, et al. Role of the gut microbiome in modulating arthritis progression in mice. Sci Rep. 2016;6(1):30594. doi:10.1038/srep30594.
- Wu HJ, Ivanov II, Darce J, Hattori K, Shima T, Umesaki Y, Littman DR, Benoist C, Mathis D. Gut-residing segmented filamentous bacteria drive autoimmune arthritis via T helper 17 cells. Immunity. 2010;32(6):815–827. doi:10.1016/j.immuni.2010.06.001.
- Teng F, Klinger CN, Felix KM, Bradley CP, Wu E, Tran NL, Umesaki Y, Wu H-J. Gut microbiota drive autoimmune arthritis by promoting differentiation and migration of Peyer’s Patch T follicular helper cells. Immunity. 2016;44(4):875–888. doi:10.1016/j.immuni.2016.03.013.
- Viladomiu M, Kivolowitz C, Abdulhamid A, Dogan B, Victorio D, Castellanos JG, Woo V, Teng F, Tran NL, Sczesnak A, et al. IgA-coated E. coli enriched in Crohn’s disease spondyloarthritis promote TH 17-dependent inflammation. Sci Transl Med. 2017;9(376):eaaf9655. doi:10.1126/scitranslmed.aaf9655.
- Sun H, Guo Y, Wang H, Yin A, Hu J, Yuan T, Zhou S, Xu W, Wei P, Yin S, et al. Gut commensal Parabacteroides distasonis alleviates inflammatory arthritis. Gut. 2023;72(9):1664–1677. doi:10.1136/gutjnl-2022-327756.
- Jiang L, Shang M, Yu S, Liu Y, Zhang H, Zhou Y, Wang M, Wang T, Li H, Liu Z, et al. A high-fiber diet synergizes with Prevotella copri and exacerbates rheumatoid arthritis. Cell Mol Immunol. 2022;19(12):1414–1424. doi:10.1038/s41423-022-00934-6.
- Jacques P, Elewaut D. Joint expedition: linking gut inflammation to arthritis. Mucosal Immunol. 2008;1(5):364–371. doi:10.1038/mi.2008.24.
- Shah A, Macdonald GA, Morrison M, Holtmann G. Targeting the gut microbiome as a treatment for primary sclerosing cholangitis: a conceptional framework. Am J Gastroenterol. 2020;115(6):814–822. doi:10.14309/ajg.0000000000000604.
- Mathies F, Steffens N, Kleinschmidt D, Stuhlmann F, Huber FJ, Roy U, Meyer T, Luetgehetmann M, von Petersdorff M, Seiz O, et al. Colitis promotes a pathological condition of the liver in the absence of Foxp3(+) regulatory T cells. J Immunol. 2018;201(12):3558–3568. doi:10.4049/jimmunol.1800711.
- Nagao JI, Kishikawa S, Tanaka H, Toyonaga K, Narita Y, Negoro-Yasumatsu K, Tasaki S, Arita-Morioka K-I, Nakayama J, Tanaka Y, et al. Pathobiont-responsive Th17 cells in gut-mouth axis provoke inflammatory oral disease and are modulated by intestinal microbiome. Cell Rep. 2022;40(10):111314. doi:10.1016/j.celrep.2022.111314.
- Noto LM, Sarnacki SH, Aya CMR, Bernal MI, Giacomodonato MN, Cerquetti MC. Consumption of Lactobacillus casei fermented milk prevents Salmonella reactive arthritis by modulating IL-23/IL-17 expression. PloS One. 2013;8(12):e82588. doi:10.1371/journal.pone.0082588.
- Fan Z, Ross RP, Stanton C, Hou B, Zhao J, Zhang H, Yang B, Chen W. Lactobacillus casei CCFM1074 alleviates collagen-induced arthritis in rats via balancing Treg/Th17 and modulating the metabolites and gut microbiota. Front Immunol. 2021;12:680073. doi:10.3389/fimmu.2021.680073.
- Marietta EV, Murray JA, Luckey DH, Jeraldo PR, Lamba A, Patel R, Luthra HS, Mangalam A, Taneja V. Suppression of inflammatory arthritis by human gut-derived Prevotella histicola in humanized mice. Arthritis Rheumatol. 2016;68(12):2878–2888. doi:10.1002/art.39785.
- Sanges M, Valente G, Rea M, Della GR, De Franchis G, Sollazzo R, D’Arienzo A. Probiotics in spondyloarthropathy associated with ulcerative colitis: a pilot study. Eur Rev Med Pharmacol Sci. 2009;13:233–234.
- Chen YH, Wu CS, Chao YH, Lin CC, Tsai HY, Li YR, Chen Y-Z, Tsai W-H, Chen Y-K. Lactobacillus pentosus GMNL-77 inhibits skin lesions in imiquimod-induced psoriasis-like mice. J Food Drug Anal. 2017;25(3):559–566. doi:10.1016/j.jfda.2016.06.003.
- Groeger D, O’Mahony L, Murphy EF, Bourke JF, Dinan TG, Kiely B, Shanahan F, Quigley EMM. Bifidobacterium infantis 35624 modulates host inflammatory processes beyond the gut. Gut Microbes. 2013;4(4):325–339. doi:10.4161/gmic.25487.
- Navarro-López V, Martínez-Andrés A, Ramírez-Boscá A, Ruzafa-Costas B, Núñez-Delegido E, Carrión-Gutiérrez MA, Prieto-Merino D, Codoñer-Cortés F, Ramón-Vidal D, Genovés-Martínez S, et al. Efficacy and safety of oral administration of a mixture of probiotic Strains in patients with psoriasis: a randomized controlled clinical trial. Acta Derm Venereol. 2019;99:1078–1084. doi:10.2340/00015555-3305.
- Dusek O, Fajstova A, Klimova A, Svozilkova P, Hrncir T, Kverka M, Coufal S, Slemin J, Tlaskalova-Hogenova H, Forrester JV, et al. Severity of experimental autoimmune uveitis is reduced by pretreatment with live probiotic Escherichia coli Nissle 1917. Cells. 2020;10(1):10. doi:10.3390/cells10010023.
- Kim J, Choi SH, Kim YJ, Jeong HJ, Ryu JS, Lee HJ, Kim T, Im S-H, Oh J, Kim M, et al. Clinical effect of IRT-5 probiotics on immune Modulation of Autoimmunity or Alloimmunity in the Eye. Nutrients. 2017;9(11):1166. doi:10.3390/nu9111166.
- Invernici MM, Salvador SL, Silva PHF, Soares MSM, Casarin R, Palioto DB, Souza SLS, Taba M, Novaes AB, Furlaneto FAC, et al. Effects of Bifidobacterium probiotic on the treatment of chronic periodontitis: A randomized clinical trial. J Clin Periodontol. 2018;45(10):1198–1210. doi:10.1111/jcpe.12995.
- Tekce M, Ince G, Gursoy H, Dirikan Ipci S, Cakar G, Kadir T, Yılmaz S. Clinical and microbiological effects of probiotic lozenges in the treatment of chronic periodontitis: a 1-year follow-up study. J Clin Periodontol. 2015;42(4):363–372. doi:10.1111/jcpe.12387.
- Hoentjen F, Welling GW, Harmsen HJ, Zhang X, Snart J, Tannock GW, Lien K, Churchill TA, Lupicki M, Dieleman LA, et al. Reduction of colitis by prebiotics in HLA-B27 transgenic rats is associated with microflora changes and immunomodulation. Inflamm Bowel Dis. 2005;11(11):977–985. doi:10.1097/01.MIB.0000183421.02316.d5.
- Levi Y, Novais GS, Dias RB, Andraus RAC, Messora MR, Neto HB, Ervolino E, Santinoni CS, Maia LP. Effects of the prebiotic mannan oligosaccharide on the experimental periodontitis in rats. J Clin Periodontol. 2018;45(9):1078–1089. doi:10.1111/jcpe.12987.
- Li ZY, Zhou JJ, Luo CL, Zhang LM. Activation of TGR5 alleviates inflammation in rheumatoid arthritis peripheral blood mononuclear cells and in mice with collagen II‑induced arthritis. Mol Med Rep. 2019;20(5):4540–4550. doi:10.3892/mmr.2019.10711.
- Hasan H, Ismail H, El-Orfali Y, Khawaja G. Therapeutic benefits of indole-3-Carbinol in adjuvant-induced arthritis and its protective effect against methotrexate induced-hepatic toxicity. BMC Complement Altern Med. 2018;18(1):337. doi:10.1186/s12906-018-2408-1.
- Ebringer A, Wilson C. The use of a low starch diet in the treatment of patients suffering from ankylosing spondylitis. Clin Rheumatol. 1996;15(Suppl 1):62–66. doi:10.1007/BF03342649.
- Fickert P, Hirschfield GM, Denk G, Marschall HU, Altorjay I, Färkkilä M, Schramm C, Spengler U, Chapman R, Bergquist A, et al. norUrsodeoxycholic acid improves cholestasis in primary sclerosing cholangitis. J Hepatol. 2017;67(3):549–558. doi:10.1016/j.jhep.2017.05.009.
- Kowdley KV, Vuppalanchi R, Levy C, Floreani A, Andreone P, LaRusso NF, Shrestha R, Trotter J, Goldberg D, Rushbrook S, et al. A randomized, placebo-controlled, phase II study of obeticholic acid for primary sclerosing cholangitis. J Hepatol. 2020;73(1):94–101. doi:10.1016/j.jhep.2020.02.033.
- Trauner M, Gulamhusein A, Hameed B, Caldwell S, Shiffman ML, Landis C, Eksteen B, Agarwal K, Muir A, Rushbrook S, et al. The nonsteroidal farnesoid X receptor Agonist Cilofexor (GS-9674) improves markers of cholestasis and liver injury in patients with primary sclerosing cholangitis. Hepatol. 2019;70(3):788–801. doi:10.1002/hep.30509.
- Wu Z, Liu X, Huang S, Li T, Zhang X, Pang J, Zhao J, Chen L, Zhang B, Wang J, et al. Milk fat globule membrane attenuates acute colitis and secondary liver injury by improving the mucus barrier and regulating the gut microbiota. Front Immunol. 2022;13:865273. doi:10.3389/fimmu.2022.865273.
- Iwasaki K, Maeda K, Hidaka K, Nemoto K, Hirose Y, Deguchi S. Daily Intake of heat-killed Lactobacillus plantarum L-137 decreases the probing depth in patients undergoing supportive periodontal therapy. Oral Health Prev Dent. 2016;14(3):207–214. doi:10.3290/j.ohpd.a36099.
- Tabuchi Y, Katsushima M, Nishida Y, Shirakashi M, Tsuji H, Onizawa H, Kitagori K, Akizuki S, Nakashima R, Murakami K, et al. Oral dextran sulfate sodium administration induces peripheral spondyloarthritis features in SKG mice accompanied by intestinal bacterial translocation and systemic Th1 and Th17 cell activation. Arthritis Res Ther. 2022;24(1):176. doi:10.1186/s13075-022-02844-4.
- Nakamura YK, Metea C, Karstens L, Asquith M, Gruner H, Moscibrocki C, Lee I, Brislawn CJ, Jansson JK, Rosenbaum JT, et al. Gut microbial Alterations associated with Protection from autoimmune uveitis. Invest Ophthalmol Visual Sci. 2016;57(8):3747–3758. doi:10.1167/iovs.16-19733.
- Tabibian JH, Weeding E, Jorgensen RA, Petz JL, Keach JC, Talwalkar JA, Lindor KD. Randomised clinical trial: vancomycin or metronidazole in patients with primary sclerosing cholangitis - a pilot study. Aliment Pharmacol Ther. 2013;37(6):604–612. doi:10.1111/apt.12232.
- Rahimpour S, Nasiri-Toosi M, Khalili H, Ebrahimi-Daryani N, Nouri-Taromlou MK, Azizi Z. A triple blinded, randomized, placebo-controlled clinical trial to evaluate the efficacy and safety of oral vancomycin in primary sclerosing cholangitis: a pilot study. J Gastrointestin Liver Dis. 2016;25(4):457–464. doi:10.15403/jgld.2014.1121.254.rah.
- Färkkilä M, Karvonen AL, Nurmi H, Nuutinen H, Taavitsainen M, Pikkarainen P, Kärkkäinen P. Metronidazole and ursodeoxycholic acid for primary sclerosing cholangitis: a randomized placebo-controlled trial. Hepatol. 2004;40(6):1379–1386. doi:10.1002/hep.20457.
- Silveira MG, Torok NJ, Gossard AA, Keach JC, Jorgensen RA, Petz JL, Lindor KD. Minocycline in the treatment of patients with primary sclerosing cholangitis: results of a pilot study. Am J Gastroenterol. 2009;104(1):83–88. doi:10.1038/ajg.2008.14.
- Shah A, Crawford D, Burger D, Martin N, Walker M, Talley NJ, Tallis C, Jones M, Stuart K, Keely S, et al. Effects of antibiotic therapy in primary sclerosing cholangitis with and without inflammatory bowel disease: a systematic review and meta-analysis. Semin Liver Dis. 2019;39(4):432–441. doi:10.1055/s-0039-1688501.
- Teughels W, Feres M, Oud V, Martín C, Matesanz P, Herrera D. Adjunctive effect of systemic antimicrobials in periodontitis therapy: a systematic review and meta-analysis. J Clinic Periodontology. 2020;47(Suppl S22):257–281. doi:10.1111/jcpe.13264.
- Allegretti JR, Kassam Z, Carrellas M, Mullish BH, Marchesi JR, Pechlivanis A, Smith M, Gerardin Y, Timberlake S, Pratt DS, et al. Fecal microbiota transplantation in patients with primary sclerosing cholangitis: a pilot clinical trial. Am J Gastroenterol. 2019;114(7):1071–1079. doi:10.14309/ajg.0000000000000115.
- Hill C, Guarner F, Reid G, Gibson GR, Merenstein DJ, Pot B, Morelli L, Canani RB, Flint HJ, Salminen S, et al. Expert consensus document. The International Scientific Association for probiotics and prebiotics consensus statement on the scope and appropriate use of the term probiotic. Nat Rev Gastro Hepat. 2014;11(8):506–514. doi:10.1038/nrgastro.2014.66.
- Chen X, Chen Y, Stanton C, Ross RP, Zhao J, Chen W, Yang B. Dose–response efficacy and mechanisms of orally administered Bifidobacterium breve CCFM683 on IMQ-Induced psoriasis in mice. Nutrients. 2023;15(8):15. doi:10.3390/nu15081952.
- Gibson GR, Hutkins R, Sanders ME, Prescott SL, Reimer RA, Salminen SJ, Scott K, Stanton C, Swanson KS, Cani PD, et al. Expert consensus document: The International Scientific Association for probiotics and Prebiotics (ISAPP) consensus statement on the definition and scope of prebiotics. Nat Rev Gastro Hepat. 2017;14(8):491–502. doi:10.1038/nrgastro.2017.75.
- Salminen S, Collado MC, Endo A, Hill C, Lebeer S, Quigley EMM, Sanders ME, Shamir R, Swann JR, Szajewska H, et al. The International Scientific Association of Probiotics and Prebiotics (ISAPP) consensus statement on the definition and scope of postbiotics. Nat Rev Gastro Hepat. 2021;18(9):649–667. doi:10.1038/s41575-021-00440-6.
- Paramsothy S, Nielsen S, Kamm MA, Deshpande NP, Faith JJ, Clemente JC, Paramsothy R, Walsh AJ, van den Bogaerde J, Samuel D, et al. Specific bacteria and metabolites associated with response to fecal microbiota transplantation in patients with ulcerative colitis. Gastroenterology. 2019;156(5):1440–54.e2. doi:10.1053/j.gastro.2018.12.001.