ABSTRACT
Fine particulate matter (PM2.5) in the atmosphere is easily accompanied by toxic and harmful substances, causing serious harm to human health, including cognitive impairment. Vitamin B12 (VitB12) is an essential micronutrient that is synthesized by bacteria and contributes to neurotransmitter synthesis as a nutrition and signaling molecule. However, the relationship between VitB12 attenuation of cognitive impairment and intestinal microbiota regulation in PM2.5 exposure has not been elucidated. In this study, we demonstrated that PM2.5 caused behavioral defects and neuronal damage in Caenorhabditis elegans (C. elegans), along with significant gene expression changes in neurotransmitter receptors and a decrease in VitB12 content, causing behavioral defects and neuronal damage in C. elegans. Methylcobalamin (MeCbl), a VitB12 analog, alleviated PM2.5-induced neurotoxicity in C. elegans. Moreover, using in vivo and in vitro models, we discovered that long-term exposure to PM2.5 led to changes in the structure of the gut microbiota, resulting in an imbalance of the VitB12-associated metabolic pathway followed by cognitive impairment. MeCbl supplementation could increase the diversity of the bacteria, reduce harmful substance contents, and restore the concentration of short-chain fatty acids (SCFAs) and neurotransmitters to the level of the control group to some degree. Here, a new target to mitigate the harm caused by PM2.5 was discovered, supplying MeCbl for relieving intestinal and intracellular neurotransmitter disorders. Our results also provide a reference for the use of VitB12 to target the adjustment of the human intestinal microbiota to improve metabolic disorders in people exposed to PM2.5.
Introduction
Air pollution is a complex mixture of particulate matter (PM), carbon monoxide, lead, nitrogen dioxide, ozone, and sulfur dioxide. Among them, atmospheric particulate matter with an aerodynamic diameter of less than 2.5 µm, namely, PM2.5, is the most harmful to human health. The composition of PM2.5 is complicated; it not only contains organic materials, such as benzo[a]pyrene, polycyclic aromatic hydrocarbons, nitro, and oxygen-based PAHs, but is also rich in inorganic chemicals, including sulfate, nitrate, and metals.Citation1,Citation2 Cumulative evidence over the past 20 years has shown that air pollution is closely associated with respiratory diseases such as asthma, cardiovascular diseases, metabolic disorders, and cancer,Citation3–6 ranking it as the 13th leading cause of death globally by the World Health Organization.Citation7 Among various air pollution components, PM2.5 is one of the main factors strongly associated with cognitive impairment.Citation8,Citation9 PM2.5 can cross the blood-brain barrier and enter the nervous system, producing excess reactive oxygen species (ROS) and inflammatory factors, leading to nerve cell apoptosis.Citation10,Citation11 Guo et al., demonstrated that organic extracts in PM2.5 induced ferroptosis of SH-SY5Y cells.Citation12 Autophagic-lysosomal dysfunction was also investigated in Neuro-2a cells exposed to PM2.5, leading to lysosome membrane permeabilization-associated cell death.Citation13 Nevertheless, the specific mechanism of neurotoxicity caused by PM2.5 remains unclear.
The gut contains diverse microbiotas that play important roles in human health.Citation14 The brain-gut axis is a bidirectional communication network between the gastrointestinal tract and the central nervous system. Related metabolites produced by gut microbes interact with the host to regulate central nervous system function and behavior. PM2.5 could induce the obvious decline of body weight of adult mice, accompanied by the ratio of Bacteroidetes/Firmicutes reduction.Citation15 Research has shown that in a mouse model, the α-diversity of bacterial genera increased in the PM2.5 exposure group, while Lactobacillus, which was observed in the control group, was absent in the PM2.5 exposure group.Citation16 Recent study has indicated that exposure to PM2.5 is associated with decreased Bacteroidetes and increased Proteobacteria, and black carbon is the most important element in the association.Citation17 The effect of PM2.5 on gut microbes has been widely concerned,Citation18 it is particularly essential to investigate specific relationship between PM2.5 and intestinal flora in-depth, which will help us to provide early prevention and new treatment for harmful effects of PM2.5 exposure.
Vitamin B12 (VitB12), also known as cobalamin, acts as a cofactor or coenzyme in many essential biochemical processes, and the physiological role of VitB12 revolves around central cobalt-mediated chemical reactions, particularly the ability of cobalt ions to form direct cobalt-carbon bonds.Citation19 Natural VitB12 can only be synthesized by microorganisms, most of which are bacteria and actinomycetes. Its biosynthesis process requires the participation of a variety of enzymes, and its synthesis pathway is extremely complex. Directional production and selective absorption of specific species of VitB12 can reduce competition with other bacteria, which is conducive to the establishment of mutualistic symbiosis between bacteria. Studies have found that early VitB12 supplementation can delay cognitive decline, reduce brain atrophy, and reduce the incidence of dementia and Alzheimer’s disease (AD) later in life.Citation20,Citation21 Our previous study found that methylcobalamin (MeCbl) supplementation alleviated cognitive impairment induced by PM2.5 in C57BL/6 mice.Citation22 However, there are few reports on the effect of PM2.5, through dysregulation of gut microbiota, on cognitive ability. The relationship between MeCbl attenuation of cognitive impairment and intestinal microbiota regulation in PM2.5 has not been elucidated.
In this study, C. elegans, an excellent model for determining the neurotoxicity of substances, was first used to evaluate the neurotoxicity induced by PM2.5. Moreover, mouse experiments and in vitro simulated colonic fermentation model experiments were combined to investigate the underlying mechanism of MeCbl in alleviating PM2.5-induced metabolic disorders. First, we aimed to illustrate the relationship among PM2.5, bacterial community composition and internal VitB12 levels. In addition, we aimed to understand the interactions between the intestinal flora and neurotransmitters affected by PM2.5 and VitB12. Our results provide a reference for further studies on the mechanism of MeCbl action and targeted regulation of the intestinal microbiota.
Results
PM2.5 exposure induced toxicity in C. elegans that attenuated by MeCbl
C. elegans has become a classic model organism in the field of aging research because of its clear genetic background, simple structure, short life cycle, and easy culture.Citation23 Recent studies have shown that nematodes are powerful tools for exploring microbe-host interactions, since the gut structure of C. elegans is similar to that of humans and harbors a rich and diverse microbiome.Citation24 Therefore, we firstly used C. elegans as the research model to explore the effects of MeCbl on PM2.5-induced neurotoxicity remission in vivo. The nervous system of nematode can regulate and control its motor behavior, and the motor behavior ability of nematode is usually evaluated and analyzed by head swing frequency, body bend frequency and pharyngeal pumping.Citation25 Compared with the control group, the PM2.5 group had a lower body bending frequency but higher head swing frequency, while MeCbl decreased the periodicity of head bends but not body bends (). Additionally, both PM2.5 or MeCbl supplementation had no obvious effect on the swallowing function of nematodes (). Furthermore, the survival ratio of worms exposed to PM2.5 apparently decreased, as half of the worms died on Day 9, while the control group maintained a 50% survival rate on Day 14. Intervention with MeCbl effectively improved the survival rate; a survival rate of approximately 70% was observed on the 9th day when worms were supplied with MeCbl (). Taken together, these data suggest that PM2.5-induced toxicity is moderated by MeCbl.
Figure 1. Effects of MeCbl on PM2.5-induced toxicity in C. elegans. (a-c) the impact of MeCbl intervention on body bending times (1 min), head swing times (20 s) and pharyngeal pumping rate (20 s) of worms. *p < .05; **p < .01; ns: not significant by Student’s t-test. (d) lifespan assay on worms with different treatments. (e) chemotaxis-mediated associative learning model; (f) chemotaxis learning behavior after 72 h PM2.5 exposure with or without MeCbl; (g) the schematic diagram for analyzing chemotaxis of C. elegans. (h) chemotaxis behavior of C. elegans after 72 h PM2.5 exposure with or without MeCbl; indole and decanal (dissolved in ethanol) were used for studying the sensitivity of nematodes to chemicals; (i) the schematic diagram for analyzing foraging behavior of C. elegans; (j) foraging behavior after 72 h PM2.5 exposure with or without MeCbl. Values indicated by the bars with different letters are significantly different (p < .05, one-way ANOVA). CI, chemotaxis index.
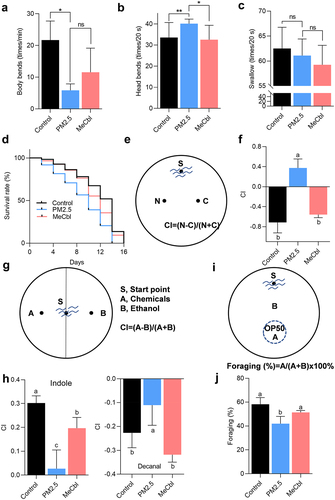
Effects of PM2.5 exposure on chemotaxis, feeding, learning and memory behavior in C. elegans
C. elegans can react to liquid and airborne chemicals, sensing water-soluble attractants and volatile substances in the micromolar concentration range, resulting in a tendency or avoidance behavior.Citation26–28 First, the chemotaxis-mediated learning and memory model was investigated. In this model, nematodes were cultured in an NGM medium with NaCl and no food for a period of time to integrate the NaCl signal with the starvation signal so that nematodes would avoid the NaCl concentration during the starvation treatment. Exposure to PM2.5 resulted in an increase in the number of nematodes in the NaCl region, and MeCbl intervention reversed this abnormality (). Indole and decanal were chosen for chemotaxis assays. In the control group, nematodes showed attraction to indole and repulsion to decal, but the absolute value of the CI index decreased after worms were exposed to PM2.5, which was partially recovered by MeCbl supplementation (). Feeding behavior is controlled by pharyngeal motor neurons, which directly coordinate the contraction and relaxation of the pharyngeal muscle to complete feeding behavior. PM2.5 induced an obvious reduction in foraging ability compared to the nonexposed group. Again, the foraging behavior of worms was restored after MeCbl prevention (). These results showed that MeCbl exhibited a protective effect against chemotaxis behavior sensitivity and obstacles to learning and memory behavior in C. elegans damaged by PM2.5.
Effect of PM2.5 exposure on neurons and neurotransmitter receptors in C. elegans
Neurotransmitters in C. elegans have a high degree of similarity with those in mammals, including synthesis, storage, transport, metabolism, and many other biological processes. With exposure to PM2.5, the fluorescence intensity of green fluorescent protein-labeled GABAergic (unc-47), cholinergic (unc-17), and glutamatergic (eat-4) neurons in nematodes decreased to varying degrees, suggesting that a series of neurons in nematodes were damaged by PM2.5 exposure. MeCbl treatment partially protected against PM2.5-induced neurotoxicity ().
Figure 2. Effects of MeCbl on GABAergic, cholinergic and glutamatergic neurons of EG1285, LX929and DA1240 after a 72 h PM2.5-exposure period. (a) Fluorescent photographs show the GABAergic neuron in worms when exposed to PM2.5 with or without MeCbl treatment. (b) Neuronal injury in EG1285 nematodes after PM2.5 and MeCbl treatment. (c) Fluorescent photographs show the cholinergic neuron in worms when exposed to PM2.5 with or without MeCbl treatment. (d) Neuronal injury in LX929nematodes after PM2.5 and MeCbl treatment. Degree of neuronal injury was classified into three categories, namely normal (good neuronal integrity), intermediate (degradation of a few neurons, less than 5), abnormal (more than 5 neurons degradation, discontinuous or misplacement). (e) Fluorescent photographs show the glutamatergic neuron in worms when exposed to PM2.5 with or without MeCbl treatment. (f) Relative fluorescence intensity of eat-4:GFP in C. elegans with indicated treatments. Values indicated by the bars with different letters are significantly different (p < .05, one-way ANOVA).
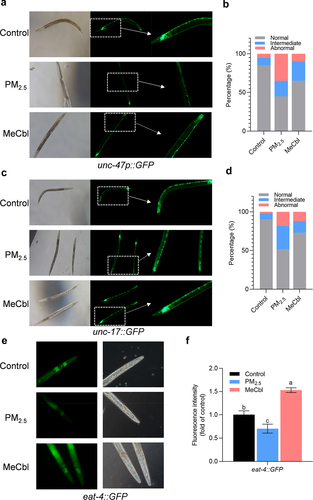
PM2.5 exposure altered the expression of genes involved in neurons and VitB12 metabolism in C. elegans
The methionine/S-adenosylmethionine (Met/SAM) cycle is a part of one-carbon metabolism, including folate metabolism and purine and thymine biosynthesis. VitB12 is an essential cofactor that participates in these metabolic pathways.Citation29,Citation30 Decreased gene expression levels of metr-1, mtrr-1, and sams-1 were detected in PM2.5-exposed C. elegans and were recovered by MeCbl replenishment (). These three genes were associated with the VitB12-dependent homocysteine (Hcy) depletion pathway (). Hcy is a nonprotein amino acid that can be recycled into methionine or cysteine with the assistance of B vitamins, the deficiency of which can lead to hyperhomocysteinemia. Low VitB12 leads to the accumulation of Hcy, inducing severe oxidative stress that is highly associated with neurodegenerative diseases. After the neurotransmitter is synthesized and released, it must bind to the corresponding receptor to play a role in the neuroregulation and transmission of nerve signals. To study the activity of neurotransmitters, it is important to detect the expression of the corresponding receptor gene. To further illustrate the effects of PM2.5 exposure and MeCbl intervention on neurotransmitters, the mRNA levels of nine related genes were measured. As shown in , when nematodes were exposed to PM2.5 for 72 h, the expression of the eat-4, glt-3, unc-30, unc-46 and unc-47 genes was significantly upregulated, while the expression of the gab-1 and acr-8 genes was significantly downregulated in the MeCbl-treated group compared to the nonexposed group. Interestingly, PM2.5 led to a stronger response of gene expression involved in neurotransmitter receptors than that of methyl conversion-related genes, implying another protection mechanism of MeCbl, in addition to one-carbon cycle participation. In C. elegans, acyl-CoA dehydrogenase acdh-1 is a well-established sensor gene for VitB12 levels,Citation31 and a lower VitB12 level triggers a higher expression of acdh-1. Pacdh-1:GFP worms (VL749) are usually used to indicate endogenous VitB12 levels. The reduced VitB12 levels in animals exposed to PM2.5 were investigated and recovered by MeCbl supplementation, implying that VitB12 deficiency may be one reason for abnormal behaviors in worms after treatment with PM2.5 ().
Figure 3. Effects of MeCbl on the mRNA levels of genes related with cobalamin metabolism and neurotransmitters in C. elegans exposed to PM2.5. (a) Levels of Met/SAM cycle genes affected by PM2.5 and MeCbl. (b) Cartoon of VitB12-related metabolic pathways in C. elegans. (c) Effect of MeCbl on neurotransmission-related gene expression in nematodes exposed to PM2.5. Relative gene expressions were normalized to act-1 gene. (d) Fluorescent photographs and relative fluorescence intensity of Pacdh1:GFP transgenic animals (VL749 strain) when exposed to PM2.5 with or without MeCbl treatment. The acyl-CoA dehydrogenase acdh-1 is a well-established sensor gene for B12 levels, and a lower B12 level triggers higher expression of acdh-1. Values indicated by the bars with different letters are significantly different (p < .05, one-way ANOVA).
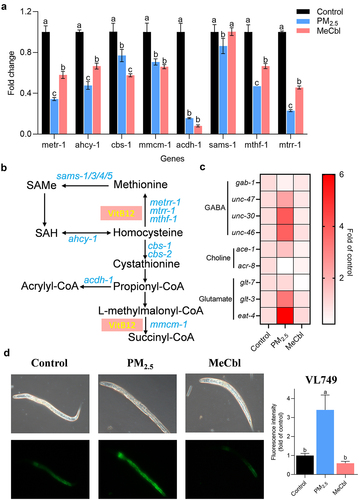
Alteration of intestinal bacterial composition in mice exposed to PM2.5
Neurotransmitters are a diverse group of compounds involved in the transmission of information at chemical synapses. Many gut bacteria can generate or consume neurotransmitters.Citation32 A previous study showed that MeCbl could restore the impaired learning and memory function caused by PM2.5. The role of MeCbl in neurotransmitter modulation to ameliorate PM2.5-induced toxicity has attracted our interest. MeCbl supplementation resulted in the recovery of the shortening of intestinal length and weight loss in mice exposed to PM2.5, although there was no significant difference in weight gain among the three groups (Fig. S1A-C). As shown in , PM2.5 exposure led to a decreased level of α-diversity of the intestinal microbiota in mice, which was recovered by MeCbl supplementation, indicating that MeCbl kept the intestinal microbiota more stable and balanced. In addition, there was no significant difference in the overall structure of the bacterial flora changes among the three groups (Fig. S1D). The gut microbiota was dominated by bacteria from two phyla, Firmicutes and Bacteroidetes.Citation33 At the phylum level (Fig. S1E), Firmicutes and Bacteroidetes were the dominant bacteria in the intestinal flora of mice, accounting for 87.70% of all phyla, on average. Verrucomicrobia was more abundant in the PM2.5 group in the second month and in the MeCbl group in the fourth and fifth months. In the later stage, Actinobacteria accounted for a larger proportion of the control group and PM2.5 group, while accounting for a smaller proportion of the MeCbl group. A heatmap was used to compare the genus levels among the groups (). Compared with the control group, the abundance of Lactobacillus and Parabacteroides increased in the PM2.5 group, while MeCbl decreased the abundance of these bacteria. In contrast, at a genus level, Bacteroides, Prevotella, Ruminococcus and Alloprevotella were decreased in the PM2.5 group, and vice versa in the MeCbl group, especially Bacteroides. Above bacteria such as Lactobacillus, Bacteroides, Prevotella, and Ruminococcus can utilize fiber substrate for short-chain fatty acids (SCFAs) productionCitation34 and Lactobacillus, Bacteroides, Parabacteroides and Ruminococcus have been reported to associated with neurotransmitters synthesis.Citation35
Figure 4. Effects of MeCbl on constitutes of intestinal bacteria and metabolite in mice exposed to PM2.5. (a) α-diversity-Shannon index of fecal microbiota; (b) Heatmap of relative abundance of bacterial genus in the intestinal tract of mice; (c) Principal component analysis of intestinal metabolites in mice (the difference in PCA1 and PCA2 was 53.43% and 45.75%); (d) Intestinal short-chain fatty acid concentration in mice; (e) metabolite heatmap of intestinal microbiome in mice; (f) Correlation analysis of metabolites and intestinal microflora in mice.
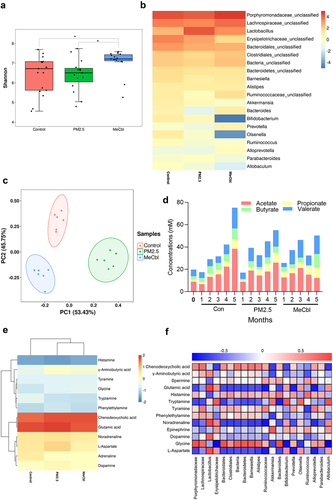
PM2.5 exposure altered the metabolomic profile in mice
The feces of the mice in the second month were selected for nontargeted metabolome analysis. Points from the same group were clustered together, whereas points in different groups were scattered, indicating good sample parallelism and obvious differences among the control, PM2.5 and MeCbl groups (). PM2.5 increased the levels of γ-aminobutyric acid (GABA), glycine, glutamic acid, norepinephrine, aspartic acid, and dopamine and decreased histamine and phenylethylamine levels ().
The concentrations of SCFAs in the mouse feces are shown in . In the 4th and 5th months, the acetic acid content in PM2.5-exposed mice was significantly lower than that of the control, while butyrate was lower in the PM2.5 group than in the control group for all months. Nevertheless, the addition of MebCl did not obviously change the contents of the two substances mentioned above in comparison with the PM2.5 group, except that the concentration of butyrate increased in the fifth month and was even higher than that in the control group. In the second and third months, the valerate contents of the MebCl groups were significantly higher than those of PM2.5. It can be concluded that exposure of mice to PM2.5 reduces the total concentration of SCFAs in the intestine, especially the concentrations of acetic acid and butyric acid. VitB12 intake could increase intestinal butyric acid and valerate concentrations but not acetic acid content.
Through the correlation analysis of bacterial flora and metabolites, the increase in the abundance of Lactobacillus and the decrease in Bacteroides abundance may be the main factors of glutamic acid, GABA, and dopamine content elevation when mice were exposed to PM2.5, which could be reversed by MeCbl ().
Alteration of the gut microbiome and metabolites in the in vitro colon simulation system with PM2.5 exposure and MeCbl supplementation
Considering that the gut microbiota of mice and humans is quite different, an in vitro simulated colonic fermentation model that can simulate the growth and metabolism of human intestinal flora was used for further research. The SCFA content of the colon cavity is shown in . Compared with PM2.5, an increase in butyric acid content was observed in the MeCbl-treated groups (MeCbl-M and MeCbl-H). Valeric acid was only present in the MeCbl-H group but not in any other group, and this result was consistent with that in the mucosa ( and S2A). The decrease in abundance of Faecalibacterium, Ruminococcus_2, Anaeroglobus and Bifidobacterium after treatment with PM2.5 might be one reason for lower butyric acid production ().
Figure 5. Effect of MeCbl on changes in the composition of bacteria and metabolites in gut lumen of the in vitro colon simulation system exposed to PM2.5. (a) histogram of changes in SCFAs in the gut lumen of the colon; (b) cartoon of relationship between butyrate production and gut flora changes impacted by PM2.5 and MeCbl; (c) heatmap of relative abundance of bacteria genus; (d) heatmap of secondary metabolic intensity of bacteria; (e) correlation analysis of metabolites and intestinal microflora; (f) canonical correlation analysis (CCA) between species and neurotransmitters. We firstly carry out detrended correspondence analysis (DCA) analysis on data and the DCA1 of relevant data in this study is 3.7928 that is greater than 3, so we choose a CCA model.
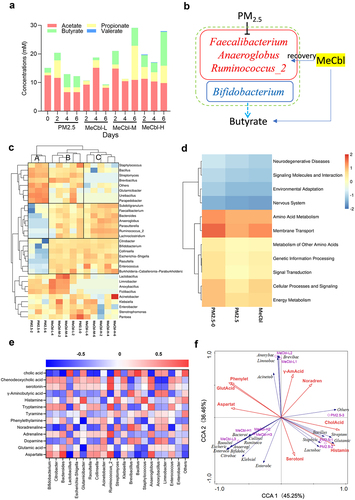
The addition of VitB12 resulted in clear changes in the structure of the phylum. Compared to the PM2.5 group, the relative abundance of Actinobacteria, Cyanobacteria, Acidobacteria, Armatimonadetes, Planctomycetes, Fibrobacters, Verrucomicrobia, Errucomicrobia, Patescibacteria, Chloroflexi, Gemmatimonadetes and BRC1 decreased in all three MeCbl-treated groups (Fig. S3). In summary, the structure of the phyla in the PM2.5 group was significantly different from that in the group supplemented with VitB12, and the structure of the phyla changed in the same trend with increasing time and concentration of VitB12, among which Verrucomicrobia showed a clear gradient change.
In the genus heatmap (), compared with the PM2.5-0 group, PM2.5 exposure increased the abundance of Bacillus, Streptomyces, Glutamicibacter, Ureibacillus, Parapedobacter and other bacterial genera. However, the addition of VitB12 reduced the abundance of these genera. The relative abundance of these genera decreased with prolonged VitB12 supplementation time and an increase in VitB12 concentration. Moderate and low concentrations of VitB12 reduced the abundance of these genera to a level similar to that in the PM2.5-0 group, whereas high concentrations of long-term VitB12 supplementation reduced the abundance of these genera. On the other hand, exposure to PM2.5 reduced the abundance of Subdoligranulum, Faecalibacterium, Bacteroides, Anaeroglobus, Parasutterella, Ruminococcus_2, Lachnoclostridium and other genera, which increased with the addition of VitB12. The relative abundance of these genera increased with time and VitB12 concentration. Additionally, MeCbl intake increased Escherichia-Shigella and decreased Lactobacillus as a result of VitB12 regulation through ribosomal switches in bacteria.Citation36,Citation37
Among the secondary metabolic pathways in the colonic lumen (), PM2.5 attenuated all secondary metabolic pathways, including neurodegenerative diseases, signaling molecules and interactions, environmental adaptation, nervous system, amino acid metabolism, membrane transport, metabolism of other amino acids, genetic information processing, signal transduction, cellular processes, metabolic intensity of signaling, and energy metabolism, which was also observed in the mucosa (Fig. S2B). While VitB12 enhanced part of the metabolic pathway, it could only partially restore the metabolic intensity but not completely restore it to the level of PM2.5-0. On the fourth day, we further analyzed the correlation between bacterial flora and metabolites in the lumen. As shown in , cholic acid was the most obviously enriched substance, which was most positively associated with Lactobacillus and Bacillus and negatively associated with Bifidobacterium, Bacteroides, Ruminococcus_2, and Anaeroglobus. Canonical correlation analysis (CCA) is a statistical analysis that describes the relationship between two variables. Here, the correlation between neurotransmitter concentrations and gut microbiota abundance was used for CCA. Most microbiota were in the third and fourth quadrants, whereas neurotransmitters were distributed in the first, second, and third quadrants ().
Alteration of toxin concentration, enzyme activity and VitB12 content in fermentations exposed to PM2.5
The accumulation of lipopolysaccharide (LPS) was accelerated by PM2.5, and the intervention of MeCbl slowed this change, even lower than that in the control group one week after fermentation (). The variation trend of Shiga toxin content was similar to that of the control group, whereas the MeCbl group showed a lower level (). These results suggest that MeCbl intake can reduce intestinal toxin content and the risk of disease. Protease activity decreased significantly on the second day in the PM2.5-treated group, and the intervention of MeCbl caused protease activity to remain at the same level as that of the control group on the second day of fermentation. With continuous fermentation, the enzyme activity in the PM2.5-treated group gradually returned to normal. This implies that MeCbl is beneficial for stabilizing the metabolic level of protease activity during intestinal disturbances induced by environmental stimulation (). PM2.5 decreased amylase activity on the second day of fermentation, but with the progress of fermentation, the amylase activity in the MeCbl intervention group increased on Days 4 to 6 (). There are two main active forms in the body: adenocobalamin in the mitochondria and MeCbl in the cytoplasm, which are necessary for maintaining the homeostasis of methylmalonic acid (MMA) and homocysteine (Hcy). On the other part, cyanocobalamin is easy to crystallize and not easily oxidized by air, so it is often used as a food additive form of VitB12. Hydroxocobalamin is more easily converted to the active form than cyanocobalamin, has a strong affinity for cyanide ions, and can be used as an antidote for cyanide poisoning. Our results showed that the main form of VitB12 in the simulated intestinal fermentation broth was adenocobalamin, which decreased during the fermentation process with the addition of PM2.5. Meanwhile, the addition of MeCbl greatly increased the contents of all types of VitB12 ().
Figure 6. Toxin, enzyme activity and VitB12 contents of in in vitro colon simulation system at different periods of fermentation. (a) LPS contents; (b) Shiga toxin contents; (c) protease activity; (d) amylase activity; (e-h) line chart of four types of VitB12 (cyanocobalamin, methylcobalamin, Adenosylcobalamin and hydroxocobalamin) content changes.
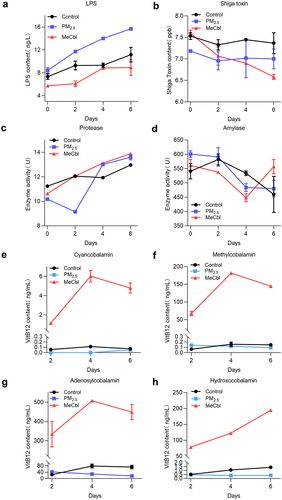
MeCbl restored PM2.5-induced disorder of VitB12 synthesis metabolism and transportation
In contrast to the control and MeCbl groups, the microbiome in the PM2.5 group enhanced the expression of CobC, CobG, CobS, CobT, and CobN, which are involved in the aerobic pathway of cobyrinate synthesis from Uroporphyrinogen III (). Meanwhile, the microbiome in the control and MeCbl groups increased the gene expression of the anaerobic pathway of cobyrinate and the synthesis of adenosyl-cobalamin (). Additionally, combined with changes in the microbiota, PM2.5 exposure led to an obvious downregulation of Faecalibacterium, Bacteroides, Ruminococcus_2, Lachnoclostridium, Citrobacter, Collinsella, and Escherichia-Shigella, which participate in VitB12 synthesis regardless of de novo biosynthesis or the salvaging pathwayCitation38 (). It is worth noting that Bacillus megaterium is a VitB12 producer that is considered a candidate for industrial application.Citation39 Given that the abundance of this bacterium in humans is very low and there are no other reports on Bacillus for VitB12 production, we did not classify Bacillus as being able to synthesize VitB12. These results indicate that PM2.5 supplementation significantly destroys the anaerobic synthesis capability of cobalamin and inhibits the concentration of adenosyl-cobalamin, which is involved in the metabolism of amino acids and some neurotransmitters. The addition of MeCbl could recover such a disturbance by enhancing the abundance of the bacteria mentioned above or directly increasing the VitB12 concentration. BtuB is regarded as a transport protein found in the outer membrane of Escherichia coli that is responsible for absorbing VitB12. TonB, a transperiplasmic protein that forms a complex with two inner membrane proteins, ExbB and ExbD, helps VitB12 transport across the outer membrane into the periplasm.Citation40,Citation41 According to metatranscriptome data analysis, the relative abundance of BtuB, TonB, ExbD, and ExbB obviously decreased in the PM2.5-treated group, indicating an impaired microbial VitB12 transport system. ExbB levels were recovered by MeCbl treatment, which is important for energy supply ().
Figure 7. Changes of cobalamin metabolism and transport in vitro colon simulation system (lumen) by KEGG pathways analysis (day 4). (a) genes of cobalamin metabolic enzymes up-regulated by PM2.5 exposure; (b) genes of cobalamin metabolic enzymes down-regulated by PM2.5 exposure; MeCbl solution was added to the fermenter at the final concentration of 0.0125 mg/mL (MeCbl-L) and 1.25 mg/mL (MeCbl-H); (c) key pathways associated with cobalamin metabolism. Up-regulated genes were marked in red and down-regulated genes were marked in blue after PM2.5 treatment, while all these marked genes level were significantly reversed by MeCbl supplementation; (d) flowchart of key pathway of bacterial B12 synthesis. Bacteria listed here was significant changed in PM2.5 and MeCbl-treated groups; (e) diagram of the VitB12 transport machinery in E. coli. (f) relative transcriptional levels of BtuB, TonB, ExbD and ExbB that involved in VitB12 transport in fermentation at day 4. Values indicated by the bars with different letters are significantly different (p < .05, one-way ANOVA).
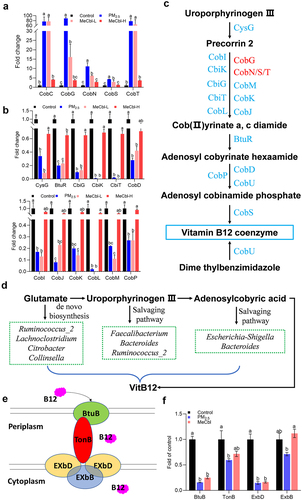
Discussion
Our results show that exposure to PM2.5 leads to VitB12 metabolism disturbance which is associated with unbalance of gut microbes. Importantly, VitB12 is identified as a new potential target by PM2.5 intervention in this study. Previous studies have indicated that chronic exposure to PM2.5 resulted in cognitive decline both in animal models and clinical studies, but nerve cell experiments cannot give an entire answer.Citation42 A series of studies have been proved that PM2.5 could affect the composition and abundance of microflora both in humans and animal models, however no one concerned the alternation of neurotransmitter produced by microbiota.Citation18,Citation43 Nevertheless, the association between PM2.5, micronutrients, and the gut microenvironment remains unclear. In this study, we evaluated the mechanism by which PM2.5 affects the gut microbiota, metabolites, and harmful markers in vivo and in vitro. Our results give solid evidence for the effectiveness of PM2.5 on neurotoxicity based on the “gut-brain axis”.
C. elegans has been successfully used in the toxicity assessment of PM2.5, and heavy metals potentially contribute to PM2.5 toxicity.Citation44,Citation45 In this study, chemotaxis behavior sensitivity and obstacles to learning and memory behavior of C. elegans were significantly damaged by PM2.5, which was restored by MeCbl supplementation. The transgenic nematode with GABAergic, cholinergic, and glutamatergic neurons labeled by green fluorescent protein was introduced in this research. The transgenic nematode EG1285 with 26 GABAergic neurons showed a relative low expression of GFP, which meant that in C. elegans PM2.5 decreased the concentrations of GABA, an inhibitory and excitatory neurotransmitter, leading to the body-bending activity of nematodes. These similar results were also reported by other researchers, exposure to heavy metals such as Pb, Hg, Cu, Cr, Cd, and Mn can cause axonal degeneration and neuronal loss in GABAergic neurons of C. elegans.Citation46 After experiment, we also found that GFP expression in the DA1240 (glutamatergic neurons) and LX929(cholinergic neurons) strains was impaired in the presence of PM2.5, but upregulated by MeCbl treatment. Glutamate is an important excitatory neurotransmitter in the central nervous system. Excessive glutamate in the brain manifests as long-term excitability enhancement, which leads to brain cell damage, anxiety, depression, and other manifestations. Similarly, acetylcholine (Ach) is essential for controlling the stimulus of the body wall and pharyngeal muscles and hence is desired for locomotion and feeding.Citation47 All of these neurotransmitters are synthesized or degraded by the microbiome via MeCbl regulation.
VitB12 is an elementary cofactor for methylmalonyl coenzyme A mutase and methionine synthase, which are vital for the one-carbon cycle and normal functioning of nerves. Interestingly, in our research the genes involved in these pathways were not significantly changed by PM2.5 and MeCbl intervention in the C. elegans model. Thus, we have reasons to believe that the regulation of gut microbes made contributions to PM2.5-induced neurotoxicity. Mouse models and in vitro microbial culture systems were used to investigate the relationships between PM2.5, MeCbl, and microbial homeostasis. Some intestinal microorganisms have the ability to synthesize VitB12, and microbiota interactions are necessary for the maintenance of VitB12 levels in the body.Citation48 Levels of Faecalibacterium, Bacteroides, Ruminococcus_2, Lachnoclostridium, Citrobacter, Collinsella, and Escherichia-Shigella, which participate in VitB12 synthesis, were drastically decreased after damage by PM2.5, leading to a very low VitB12 content in the intestinal environment, in which the similar results were reported by our previous researches.Citation49,Citation50 Consequently we found that PM2.5 exposure caused the disturbance of the VitB12 metabolic pathway, resulting in abnormal secretion of neurotransmitters, thereby causing cognitive impairment in nematodes. These abnormal phenotypes can be partially compensated by MeCbl supplementation.
SCFAs are mainly produced by the bacterial fermentation of carbohydrates (dietary fiber) and can affect central processes through a variety of mechanisms. It can interact with the brain-gut axis to alter gene expression by binding to receptors expressed by cells throughout the communication network, including neurons, enteroendocrine cells, and immune cells.Citation51,Citation52 In this study, MeCbl increased butyrate concentration, resulting from an increased abundance of butyrate-producing bacteria, such as Faecalibacterium, Ruminococcus_2 and Anaeroglobus. Bifidobacterium can provide a suitable environment for butyrate-producing bacterial growth, although it cannot directly produce butyric acid.
According to our previous microbiome and metabolome analysis data, the signaling pathway related to neurodegenerative disease has aroused great concern because of the protective effect of MeCbl on the abnormal secretion of neurometabolic substances caused by PM2.5. Evidence from this study shows that the gut microbiota can also influence host neurotransmitters and their associated pathways, with consequent effects on host physiology and behavior. Neurotransmitters secreted by bacteria in the gut can induce epithelial cells to release molecules that further regulate signals in the enteric nervous system, thereby regulating cognitive function in the brain.Citation35 Increased levels of glutamate were found in the PM2.5-exposed groups of our research, regardless of the mouse or in vitro microbial culture system, which is regarded as one of the underlying causes of neurotoxicity. This suggests that an increased level of Glutamicibacter in the PM2.5-exposed groups is one factor for glutamate accumulation.
The number of metabolites depends on cross-feeding, and microbial cooperation is likely to play a primary role in intestinal microbial ecology. Long exposure to PM2.5 led to an imbalance in the gut microenvironment followed by abnormal production of VitB12, butyrate, and neurotransmitters, ultimately causing cognitive impairment and neurotoxicity. Supplementation with MeCbl restores the balance of the flora, thereby maintaining normal concentrations of relevant metabolites and consequently improving cognitive disorders. It is critical for the application of micronutrients to human health, especially in environments where pollutants accumulate, to understand how MeCbl works as a beneficial factor for preventing the damage caused by PM2.5. Due to the rapid environmental changes in our country, the collection of population data has not been completed, and there is a lack of relevant data from population experiments that long-term exposure to PM2.5 to further verify the conclusions of this study.
Conclusion
In summary, PM2.5 exposure resulted in neurobehavioral abnormalities that could be rescued by MeCbl supplementation. Further study provided evidence that PM2.5 led to disturbance of VitB12 synthesis, metabolism, and transportation, associated with the percent change of Bacteroides spp., Bifidobacterium spp., Ruminococcaceae spp., and Faecalibacterium spp. MeCbl intake can maintain homeostasis of the relevant metabolism. In addition, MeCbl restored the concentration of neurotransmitters to the level of the control group to a certain extent, which is related to maintaining the balance of gut microbiota, including Lactobacillus and Bacteroides abundance, as well as by participating in neurotransmitter synthesis. This is followed by alleviation of neurotransmitter disorder and remission of cognitive impairment. Pathways of fatty acid, histidine, and phenylalanine metabolism were also involved in the improvement effect of MeCbl. The current findings may provide new clues for targeted regulation of the intestinal microbiota by VitB12, implying that the harmful effects of long-term exposure to PM2.5 can be alleviated by regulating the intestinal microbiota by adjusting the dietary structure.
Materials and methods
Maintenance, synchronization and treatments of C. elegans
Worms were cultured in a standard nematode growth medium (NGM) (3 g/L NaCl, 2.5 g/L peptone, 17 g/L agar, 25 mM potassium phosphate, 1 mM CaCl2 .2 H2O, 1 mM MgSO4 .7 H2O, and 12.9 mM cholesterol) containing Escherichia coli OP50 at 20°C. When most worms reached the L4 stage, they were collected with M9 buffer and lysed to obtain eggs via synchronization. For the treatment, synchronized worms were exposed to PM2.5 (0.1 mg/mL) with/without MeCbl (0.5 μg/mL) except for the control group. Indicators, including motility, animal behavior, and corresponding fluorescence intensities, were measured after 72 h of exposure. The PM2.5 sample was kindly provided by Huanhuan Wang (Hangzhou Normal University, China). The most abundant inorganic components were sulfur, potassium, and chloride, whereas the most abundant organic components were benzo(b)fluoranthene, indeno(1,2,3-cd)pyrene and chrysene.Citation22,Citation53
Lifespan assay
Synchronized young adult worms were grown in NGM with 5-fluoro-2′-deoxy-β-uridine (5-FudR, 50 μg/mL) and OP50 containing different agents throughout their lifespan. A total of 30–40 young adults were cultured on each NGM, and worms were counted every other day. Worms that did not move when gently touched with a platinum wire were considered dead. All experiments were repeated three times.
Movement assays
Synchronous nematodes (L4-stage) were transferred to NGM plates under different treatments. On the 3rd day, the nematodes were inspected using a stereomicroscope. The movement of one wavelength of the nematode relative to the long axis of its body was recorded as body bending. The head of a nematode can swing from side to side; therefore, recovery is considered a head swing. The number of body bends was counted within 60 s, and the head swing times were counted within 20 s, with no less than 30 nematodes in each group.
Forging behavior
E. coli OP50 was cultivated within a 0.5 cm radius from the center in 9 cm agar medium, and 1 μL of 1 M NaN3 was applied to the bacterial colony for anesthetization. Next, the exposed worms were placed 4 cm away from the OP50 center. Each test was performed with 60 worms in the test medium and replicated three times. After 6 h of incubation at 20°C, the number of nematodes that reached OP50 colonies was counted in each plate. The attainment level of C. elegans was calculated by dividing the number of worms that reached the bacterial colony by the total number of worms on the plate.Citation54
Chemotaxis (CTX) assays
For normal CTX assays, 5 μL of an odorous substance was dropped at point A, and the same solvent was dropped at point B. For the memory associative learning assay, a piece of NaCl agar (100 mM) was placed 3 cm away from the center, and the opposite corresponding position was set as a control. The medium was stored for 14 h at 4°C to obtain a concentration gradient of NaCl. Fifteen minutes before the assay, the NaCl agar was removed, and individuals were placed at the center of the plate. After 4 h of cultivation, animals within a 2 cm radius of either spot were counted, and the chemotactic index (CI) was obtained by subtracting the number of animals at the control spot, followed by the division of the total number of animals on the plate. A positive CI indicated an attraction to salt, and a negative CI indicated an aversion, as described in the literature. Each concentration was replicated three times.
Imaging and fluorescence quantification
Strains EG1285 [unc-47p:GFP+lin-15(+)], DA1240 [eat-4:sGFP+lin-15(+)], and LX929[unc-17:GFP] were separately used to evaluate GABAergic, glutamatergic, and cholinergic neurons. VL749 [acdh-1p:GFP + unc-119(+)] worms were used to indicate endogenous VitB12 levels. When synchronized worms reached L1 stage, they were exposed to PM2.5 (0.1 mg/mL) with/without MeCbl (0.5 μg/mL) except for the control group. After 72 h exposure, randomly selected worms with different treatments were transferred to slides containing M9 buffer with 0.2% levamisole and captured using a fluorescence microscope with fixed exposure parameters. At least 20 worms per group were used to measure the fluorescence intensity, and each experiment was performed in triplicate. Fluorescence was quantified using Image-Pro Plus 6.0 and the results are presented as relative fluorescence units normalized to the average fluorescence intensity of control animals.
Real-time quantitative PCR (RT‒qPCR)
Synchronized wild-type N2 worms were exposed to PM2.5 with or without MeCbl at the young adult stage for 72 h. Then, all nematodes were collected, and RNA was extracted, followed by cDNA synthesis in accordance with the instructions of commercial kits. qPCR was performed using the SYBR-Green PCR kit (Yeasen, Shanghai, China), and the results were analyzed using the 2−ΔΔCt method. Relative mRNA expression of the gene of interest was measured in relation to act-1 expression.
Mice and treatments
Forty-eight male C57BL/6 wild-type mice (8 weeks old) were purchased from Shanghai Slack Laboratory Animal Co., Ltd. Before the experiment, mice were acclimated for 7 days (12-hour light/dark cycle) at 25 ± 1°C and 55 ± 5% humidity and provided with standard experimental food and drinking water. The mice were randomly divided into three groups and fed for five months after the adaptation period. The experimental chamber of the control group was filled with filtered air (PM2.5 content was equal to zero), and the PM2.5 group and the MeCbl group were exposed to environmental PM2.5 in the ambient PM2.5 chronic real-time exposure chamber. The MeCbl group was fed sterile 0.125 mg/L MeCbl nutrient solution daily, and the other groups were fed sterile ultrapure water. Mice were weighed on Day 0 and monthly, and feces were collected. Five months later, the mice were sacrificed, and the length of the colon was measured. Feces were stored at − 80°C for DNA extraction and short-chain fatty acid determination. Animal experiments were carried out according to The Guide for Care and Use of Laboratory Animals and were approved by The Animal Care and Use Committee of the School of Medicine, Hangzhou Normal University (Approval NO. HSD20170301).
In vitro colon fermentation simulation
The in vitro colonic simulation system of Zhejiang Gongshang University was used to simulate colon fermentation.Citation50 After connecting the tubes of the machine, 300 mL intestinal culture medium and 15 mucosal beads were added to each fermenter. Fecal microbes were inoculated at 10% to 4 fermenters (three donors provided feces to eliminate individual differences). By setting the parameters of the intestinal fermentation system, 0.5 M NaOH solution and 0.5 M HCl were automatically added to adjust the pH to 5.5, and the temperature was constant at 37°C, which simulated the fermentation environment of the human ascending colon. Nitrogen was pumped into the fermentation tanks to maintain an anaerobic environment. After 24 h of inoculation, 100 mL of new intestinal culture medium was supplied daily, and fermentation was discharged from the same volume of tanks to simulate human ingestion and digestion. Five new mucosal beads were used daily to replace the old ones in the tank to simulate mucosal regeneration.
The fecal microbes were inoculated and fermented continuously for approximately one week to stabilize the microbial structure, and then the required samples were added. A sterile syringe was used to add 28.7 μL of 100 mg/mL PM2.5 solution to the medium in a 2 L feeding tank. MeCbl solution was added to the fermenter at final concentrations of 0.0125 mg/mL, 0.125 mg/mL and 1.25 mg/mL, and fermentation was stopped after 7 days. The fermentation broth and mucous globules of each fermenter were collected from Days 0–7 of the second stage, and the samples were stored at −20°C.
Detection of digestive enzymes and toxins
An ultrasonic cell crusher was used to break the bacteria and set the machine’s working power to 450 W, the working time to 1 min, and the ultrasonic time and interval time to 5 s. The crushed bacterial liquid was centrifuged at 8000 rpm for 10 min at 4°C, and the supernatant was used for the detection of related enzymes and toxins.
Amylase activity was detected using the iodine-starch colorimetric method, and protease activity was measured using the Folin-Lowry protein assay. The activities of the two digestive enzymes were calculated based on the dilution ratio of the standard curve.
The Shiga toxin ELISA test kit and LPS ELISA test kit were used to detect the Shiga toxin and lipopolysaccharide contents. A standard curve was constructed according to the standard solution provided in the kit. According to the standard curve, the concentrations of the two toxins were calculated using the dilution ratio.
Determination of cobalamin content
Four types of VitB12 standard sample solutions, hydroxocobalamin, cyanocobalamin, adenocobalamin, and methylcobalamin, were precisely measured and prepared. The retention times of the samples were determined using high-performance liquid chromatography (HPLC), and a standard curve was drawn.
After 8 mL of the bacterial solution was measured and centrifuged for 10 min at 8000 rpm at 4°C, the medium precipitation was removed, and the supernatant was collected. The separation and purification of VitB12 were performed by solid-phase extraction. The solid-phase extraction column (Sep-Pak C18, Waters) was activated and bond with the collected supernatant, after rinsing five column volumes with pure water, eluent was eluted twice with 3 mL methanol and collected. The methanol was completely evaporated by a nitrogen blower at 37°C, and the obtained solid substance was placed in 1 mL of ultra-pure water for testing.
All chromatographic separations were performed at room temperature. A flow of 1.0 mL/min, 0.1% formic acid aqueous solution (A) and pure acetonitrile (B), which were degassed using an ultrasonic water bath, were used as mobile phases, and the gradient elution was programmed as follows: 0–5 min 90% A; 5–25 min 90–70% A; 25–30 min 70–30% A; 30–40 min 30–90% A; 40–50 min 90% A. The injection volume was 20 μL, and the column eluate was monitored at 361 nm. The chromatographic column was a Venusil XBP C18 (4.5 × 250, 5 μm, Agela Technologies).
Metatranscriptome sequencing analysis
Colon samples (5 mL) from Day 6 of the simulated fermentation were collected and centrifuged at 800 rpm for 5 min at 4°C. The bottom solid medium was discarded, and the supernatant was transferred to a new centrifuge tube. The liquid was centrifuged at 6000 rpm for 10 min at 4°C, the supernatant was removed, and the precipitate was removed. The samples were stored at −80°C with a 2 mL RNA fixer and sent to Shanghai Meiji Biomedicine Technology Co., Ltd. for macro transcriptome sequencing.
Statistical analysis
All data are presented as the mean ± standard deviation (SD). One-way ANOVA with a post hoc multiple range significant difference (Duncan) test and t test were performed to determine statistical significance using SPSS 22.0. The significance of significant differences is indicated as *p < .05 and **p < .01. According to the OTU table, we calculated the Shannon index and Simpson index to estimate the α-diversity of the flora and performed principal component analysis (PCA) based on the sequencing data. Correlation heatmaps were generated by using MeV. 4-9-0. and Origin8.0 were used to draw the correlation histogram. In addition, PICRUSt was used to analyze the sequence data obtained from 16S rRNA sequencing to predict gene functions and metabolic pathways.
Availability of data and material
All data generated or analyzed during the current study are included in this published article [and supplementary information files] or are available in the NCBI SRA repository, PRJNA889242.
Supplemental Material
Download MS Word (1.9 MB)Disclosure statement
No potential conflict of interest was reported by the author(s).
Supplementary material
Supplemental data for this article can be accessed online at https://doi.org/10.1080/19490976.2023.2267186
Additional information
Funding
References
- Craig L, Brook JR, Chiotti Q, Croes B, Gower S, Hedley A, Krewski D, Krupnick A, Krzyzanowski M, Moran MD, et al. Air pollution and public health: a guidance document for risk managers. J Toxicol Env Heal A. 2008;71(9–10):588–21. doi:10.1080/15287390801997732.
- Yue H, Yun Y, Gao R, Li G, Sang N. Winter polycyclic aromatic hydrocarbon-bound particulate matter from peri-urban North China promotes lung cancer cell metastasis. Environ Sci Technol. 2015;49(24):14484–14493. doi:10.1021/es506280c.
- Eze IC, Hemkens LG, Bucher HC, Hoffmann B, Schindler C, Künzli N, Schikowski T, Probst-Hensch NM. Association between ambient air pollution and diabetes mellitus in Europe and North America: systematic review and meta-analysis. Environ Health Perspect. 2015;123(5):381–389. doi:10.1289/ehp.1307823.
- Hamra GB, Guha N, Cohen A, Laden F, Raaschou-Nielsen O, Samet JM, Vineis P, Forastiere F, Saldiva P, Yorifuji T, et al. Outdoor particulate matter exposure and lung cancer: a systematic review and meta-analysis. Environ Health Perspect. 2014;122(9):906–911. doi:10.1289/ehp/1408092.
- Brook RD, Rajagopalan S, Pope CA, Brook JR, Bhatnagar A, Diez-Roux AV, Holguin F, Hong Y, Luepker RV, Mittleman MA, et al. Particulate matter air pollution and cardiovascular disease. Circulation. 2010;121(21):2331–2378. doi:10.1161/CIR.0b013e3181dbece1.
- Villeneuve PJ, Chen L, Rowe BH, Coates F. Outdoor air pollution and emergency department visits for asthma among children and adults: a case-crossover study in northern Alberta, Canada. Environ Health. 2007;6(1):40. doi:10.1186/1476-069X-6-40.
- Araujo JA. Are ultrafine particles a risk factor for cardiovascular diseases? Rev Esp Cardiol. 2011;64(8):642–645. doi:10.1016/j.recesp.2011.05.002.
- Ailshire JA, Clarke P. Fine particulate matter air pollution and cognitive function among U.S. older adults. J Gerontol B. 2015;70(2):322–328. doi:10.1093/geronb/gbu064.
- Gatto NM, Henderson VW, Hodis HN, St. John JA, Lurmann F, Chen J, Mack WJ. Components of air pollution and cognitive function in middle-aged and older adults in Los Angeles. Neurotoxicology. 2014;40:1–7. doi:10.1016/j.neuro.2013.09.004.
- Thiankhaw K, Chattipakorn N, Chattipakorn SC. PM2.5 exposure in association with AD-related neuropathology and cognitive outcomes. Environ Pollut. 2022;292(Pt A):118320. doi:10.1016/j.envpol.2021.118320.
- Liu F, Liu C, Liu Y, Wang J, Wang Y, Yan B. Neurotoxicity of the air-borne particles: from molecular events to human diseases. J Hazard Mater. 2023;457:131827. doi:10.1016/j.jhazmat.2023.131827.
- Guo C, Lyu Y, Xia S, Ren X, Li Z, Tian F, Zheng J. Organic extracts in PM2.5 are the major triggers to induce ferroptosis in SH-SY5Y cells. Ecotoxicol Environ Saf. 2023;249:114350. doi:10.1016/j.ecoenv.2022.114350.
- Wei M, Bao G, Li S, Yang Z, Cheng C, Le W. PM2.5 exposure triggers cell death through lysosomal membrane permeabilization and leads to ferroptosis insensitivity via the autophagy dysfunction/p62-KEAP1-NRF2 activation in neuronal cells. Ecotoxicol Environ Saf. 2022;248:114333. doi:10.1016/j.ecoenv.2022.114333.
- Basic M, Dardevet D, Abuja PM, Bolsega S, Bornes S, Caesar R, Calabrese FM, Collino M, De Angelis M, Gérard P, et al. Approaches to discern if microbiome associations reflect causation in metabolic and immune disorders. Gut Microbes. 2022;14(1):2107386. doi:10.1080/19490976.2022.2107386.
- Dai S, Wang Z, Yang Y, Du P, Li X. PM2.5 induced weight loss of mice through altering the intestinal microenvironment: mucus barrier, gut microbiota, and metabolic profiling. J Hazard Mater. 2022;431:128653. doi:10.1016/j.jhazmat.2022.128653.
- Mutlu EA, Comba IY, Cho T, Engen PA, Yazıcı C, Soberanes S, Hamanaka RB, Niğdelioğlu R, Meliton AY, Ghio AJ, et al. Inhalational exposure to particulate matter air pollution alters the composition of the gut microbiome. Environ Pollut. 2018;240:817–830. doi:10.1016/j.envpol.2018.04.130.
- Li S, Guo B, Dong K, Huang S, Wu J, Zhou H, Wu K, Han X, Liang X, Pei X, et al. Association of long-term exposure to ambient PM2.5 and its constituents with gut microbiota: evidence from a China cohort. Sci Total Environ. 2023;884:163577. doi:10.1016/j.scitotenv.2023.163577.
- Qin J, Wang J. Research progress on the effects of gut microbiome on lung damage induced by particulate matter exposure. Environ Res. 2023;233:116162. doi:10.1016/j.envres.2023.116162.
- Smith AD, Warren MJ, Refsum H. Chapter six - Vitamin B12. In: Eskin NAM, editor. Advances in food and nutrition research. Vol. 2018. Academic Press; 2018. p. 215–279. doi:10.1016/bs.afnr.2017.11.005.
- Mccarter SJ, Coon EA, Savica R, St Louis EK, Bower JH, Benarroch EE, Sandroni P, Low P, Singer W. Lower Vitamin B12 level at multiple system atrophy diagnosis is associated with shorter survival. Mov Disord. 2020;35(8):1462–1466. doi:10.1002/mds.28070.
- Mccarter SJ, Teigen LM, Mccarter AR, Benarroch EE, St LE, Savica R. Low Vitamin B12 and Parkinson disease: potential link to reduced cholinergic transmission and severity of disease. Mayo Clin Proc. 2019;94(5):757–762. doi:10.1016/j.mayocp.2019.01.039.
- Ji X, Li C, Zhu X, Yu W, Cai Y, Zhu X, Lu L, Qian Q, Hu Y, Zhu X, et al. Methylcobalamin alleviates neuronal apoptosis and cognitive decline induced by PM2.5 exposure in mice. J Alzheimer Dis. 2022;86(4):1783–1796. doi:10.3233/JAD-215384.
- Zhang J, Holdorf AD, Walhout AJ. C. elegans and its bacterial diet as a model for systems-level understanding of host–microbiota interactions. Curr Opin Biotechnol. 2017;46:74–80. doi:10.1016/j.copbio.2017.01.008.
- Backes C, Martinez-Martinez D, Cabreiro F. C. elegans: a biosensor for host–microbe interactions. Lab Anim (NY). 2021;50(5):127–135. doi:10.1038/s41684-021-00724-z.
- Xu T, Zhang M, Hu J, Li Z, Wu T, Bao J, Wu S, Lei L, He D. Behavioral deficits and neural damage of Caenorhabditis elegans induced by three rare earth elements. Chemosphere. 2017;181:55–62. doi:10.1016/j.chemosphere.2017.04.068.
- Ishihara T, Iino Y, Mohri A, Mori I, Gengyo-Ando K, Mitani S, Katsura I. HEN-1, a secretory protein with an LDL receptor motif, regulates sensory integration and learning in Caenorhabditis elegans. Cell. 2002;109(5):639–649. doi:10.1016/S0092-8674(02)00748-1.
- Soares MV, Charão MF, Jacques MT, Dos Santos ALA, Luchese C, Pinton S, Ávila DS. Airborne toluene exposure causes germline apoptosis and neuronal damage that promotes neurobehavioural changes in Caenorhabditis elegans. Environ Pollut. 2020;256:113406. doi:10.1016/j.envpol.2019.113406.
- Wu T, He K, Zhan Q, Ang S, Ying J, Zhang S, Zhang T, Xue Y, Tang M. MPA-capped CdTe quantum dots exposure causes neurotoxic effects in nematode Caenorhabditis elegans by affecting the transporters and receptors of glutamate, serotonin and dopamine at the genetic level, or by increasing ROS, or both. Nanoscale. 2015;7(48):20460–20473. doi:10.1039/C5NR05914C.
- Lam AB, Kervin K, Tanis JE. Vitamin B12 impacts amyloid beta-induced proteotoxicity by regulating the methionine/S-adenosylmethionine cycle. Cell Rep. 2021;36(13):109753. doi:10.1016/j.celrep.2021.109753.
- Giese GE, Walker MD, Ponomarova O, Zhang H, Li X, Minevich G, Walhout AJ. Caenorhabditis elegans methionine/S-adenosylmethionine cycle activity is sensed and adjusted by a nuclear hormone receptor. Elife. 2020;9:e60259. doi:10.7554/eLife.60259.
- Qin S, Wang Y, Li L, Liu J, Xiao C, Duan D, Hao W, Qin C, Chen J, Yao L, et al. Early-life vitamin B12 orchestrates lipid peroxidation to ensure reproductive success via SBP-1/SREBP1 in Caenorhabditis elegans. Cell Rep. 2022;40(12):111381. doi:10.1016/j.celrep.2022.111381.
- Strandwitz P. Neurotransmitter modulation by the gut microbiota. Brain Res. 2018;1693:128–133. doi:10.1016/j.brainres.2018.03.015.
- Landini L, Dadson P, Gallo F, Honka M, Cena H. Microbiota in anorexia nervosa – potential for treatment. Nutr Res Rev. 2022;1–51. doi:10.1017/S0954422422000130.
- Dalile B, Van Oudenhove L, Vervliet B, Verbeke K. The role of short-chain fatty acids in microbiota–gut–brain communication. Nat Rev Gastroenterol Hepatol. 2019;16(8):461–478. doi:10.1038/s41575-019-0157-3.
- Chen Y, Xu J, Chen Y. Regulation of neurotransmitters by the gut microbiota and effects on cognition in neurological disorders. Nutrients. 2021;13(6):2099. doi:10.3390/nu13062099.
- Bernhardt C, Zhu X, Schütz D, Fischer M, Bisping B. Cobalamin is produced by Acetobacter pasteurianus DSM 3509. Appl Microbiol Biotechnol. 2019;103(9):3875–3885. doi:10.1007/s00253-019-09704-3.
- Zhu X, Xiang S, Feng X, Wang H, Tian S, Xu Y, Shi L, Yang L, Li M, Shen Y, et al. Impact of cyanocobalamin and methylcobalamin on inflammatory bowel disease and the intestinal microbiota composition. J Agric Food Chem. 2019;67(3):916–926. doi:10.1021/acs.jafc.8b05730.
- Guetterman HM, Huey SL, Knight R, Fox AM, Mehta S, Finkelstein JL. Vitamin B-12 and the gastrointestinal microbiome: a systematic review. Adv Nutr. 2022;13(2):530–558. doi:10.1093/advances/nmab123.
- Mohammed Y, Lee B, Kang Z, Du G. Development of a two-step cultivation strategy for the production of vitamin B12 by Bacillus megaterium. Microb Cell Fact. 2014;13(1):102. doi:10.1186/s12934-014-0102-7.
- Yao L, Wang Y, Qin S, Zhu S, Wu L. The antidiabetic drug metformin aids bacteria in hijacking vitamin B12 from the environment through RcdA. Commun Biol. 2023;6(1):96. doi:10.1038/s42003-023-04475-0.
- Nilaweera TD, Nyenhuis DA, Cafiso DS. Structural intermediates observed only in intact Escherichia coli indicate a mechanism for TonB-dependent transport. Elife. 2021;10:e68548. doi:10.7554/eLife.68548.
- Thiankhaw K, Chattipakorn N, Chattipakorn SC. PM2.5 exposure in association with AD-related neuropathology and cognitive outcomes. Environ Pollut. 2022;292:118320. doi:10.1016/j.envpol.2021.118320.
- Bailey MJ, Holzhausen EA, Morgan ZEM, Naik N, Shaffer JP, Liang D, Chang HH, Sarnat J, Sun S, Berger PK, et al. Postnatal exposure to ambient air pollutants is associated with the composition of the infant gut microbiota at 6-months of age. Gut Microbes. 2022;14(1):2105096. doi:10.1080/19490976.2022.2105096.
- Sun L, Wu Q, Liao K, Yu P, Cui Q, Rui Q, Wang D. Contribution of heavy metals to toxicity of coal combustion related fine particulate matter (PM2.5) in Caenorhabditis elegans with wild-type or susceptible genetic background. Chemosphere. 2016;144:2392–2400. doi:10.1016/j.chemosphere.2015.11.028.
- Yilmaz LS, Walhout AJM. Worms, bacteria, and micronutrients: an elegant model of our diet. Trends Genet. 2014;30(11):496–503. doi:10.1016/j.tig.2014.07.010.
- Du M, Wang D. The neurotoxic effects of heavy metal exposure on GABAergic nervous system in nematode Caenorhabditis elegans. Environ Toxicol Pharmacol. 2009;27(3):314–320. doi:10.1016/j.etap.2008.11.011.
- Treinin M, Jin Y. Cholinergic transmission in C. elegans: functions, diversity, and maturation of ACh‐activated ion channels. J Neurochem. 2021;158(6):1274–1291. doi:10.1111/jnc.15164.
- Roth W, Mohamadzadeh M. Vitamin B12 and gut-brain homeostasis in the pathophysiology of ischemic stroke. EBioMedicine. 2021;73:103676. doi:10.1016/j.ebiom.2021.103676.
- Zhu X, Xiang S, Feng X, Wang H, Tian S, Xu Y, Shi L, Yang L, Li M, Shen Y, et al. Impact of cyanocobalamin and methylcobalamin on inflammatory bowel disease and the intestinal microbiota composition. J Agric Food Chem. 2019;67(3):916–926. doi:10.1021/acs.jafc.8b05730.
- Xu Y, Xiang S, Ye K, Zheng Y, Feng X, Zhu X, Chen J, Chen Y. Cobalamin (vitamin B12) induced a shift in microbial composition and metabolic activity in an in vitro colon simulation. Front Microbiol. 2018;9:2780. doi:10.3389/fmicb.2018.02780.
- Yan F, Xiang S, Shi L, Zhu X. Synthesis of queuine by colonic gut microbiome via cross-feeding. Food Front. 2023. doi:10.1002/fft2.307.
- O’Riordan KJ, Collins MK, Moloney GM, Knox EG, Aburto MR, Fülling C, Morley SJ, Clarke G, Schellekens H, Cryan JF, et al. Short chain fatty acids: microbial metabolites for gut-brain axis signalling. Mol Cell Endocrinol. 2022;546:111572. doi:10.1016/j.mce.2022.111572.
- Shou Y, Zhu X, Zhu D, Yin H, Shi Y, Chen M, Lu L, Qian Q, Zhao D, Hu Y, et al. Ambient PM2.5 chronic exposure leads to cognitive decline in mice: from pulmonary to neuronal inflammation. Toxicol Lett. 2020;331:208–217. doi:10.1016/j.toxlet.2020.06.014.
- Zhang X, Ye Y, Sun J, Wang JS, Tang L, Xu Y, Ji J, Sun X. Abnormal neurotransmission of GABA and serotonin in Caenorhabditis elegans induced by Fumonisin B1. Environ Pollut. 2022;304:119141. doi:10.1016/j.envpol.2022.119141.