ABSTRACT
The gut is a major source of bacteria and antigens that contribute to neuroinflammation after brain injury. Colonic epithelial cells (ECs) are responsible for secreting major cellular components of the innate defense system, including antimicrobial proteins (AMP) and mucins. These cells serve as a critical regulator of gut barrier function and maintain host-microbe homeostasis. In this study, we determined post-stroke host defense responses at the colonic epithelial surface in mice. We then tested if the enhancement of these epithelial protective mechanisms is beneficial in young and aged mice after stroke. AMPs were significantly increased in the colonic ECs of young males, but not in young females after experimental stroke. In contrast, mucin-related genes were enhanced in young females and contributed to mucus formation that maintains the distance between the host and gut bacteria. Bacterial community profiling was done using universal amplification of 16S rRNA gene sequences. The sex-specific colonic epithelial defense responses after stroke in young females were reversed with ovariectomy and led to a shift from a predominately mucin response to the enhanced AMP expression seen in males after stroke. Estradiol (E2) replacement prior to stroke in aged females increased mucin gene expression in the colonic ECs. Interestingly, we found that E2 treatment reduced stroke-associated neuronal hyperactivity in the insular cortex, a brain region that interacts with visceral organs such as the gut, in parallel to an increase in the composition of Lactobacillus and Bifidobacterium in the gut microbiota. This is the first study demonstrating sex differences in host defense mechanisms in the gut after brain injury.
Introduction
Ischemic stroke is a leading cause of mortality and leads to persistent neurological disability that is seen in many stroke survivors.Citation1 Importantly, epidemiological studies suggest that stroke is a sexually dimorphic disease, with differences in incidence between males and females across the lifespan.Citation2,Citation3 The significant contribution of sex hormones to host immune responses in infectious and autoimmune diseases has been well documented.Citation4–6 The role of sex hormones (e.g., estrogen) has been extensively studied in rodent stroke models. Numerous studies have shown that estrogen is neuroprotective by reducing infarct size, neuronal damage and hypoperfusion,Citation7–12 but some studies have reported a deleterious role of estrogen,Citation13–15 including in aged mice.Citation16,Citation17 In humans, a protective role of estrogen on stroke had been reported,Citation18–21 however several randomized double-blinded studies, including the large Women’s Health Initiative (WHI),Citation22,Citation23 found that hormone therapy (HT) can increase the risk of stroke without a reduction of mortality, especially in women over 65 y.Citation24–26 Further sub-analysis of the WHI results by age group, and more recent randomized control studies, including the Kronos Early Estrogen and Prevention Study (KEEPs) and Early versus Late Intervention Trial with Estradiol (ELITE),Citation27 demonstrate that the risk of adverse cardiovascular events for HT is low for women <60 y of age or within 10 y from menopause, showing the importance of the timing of initiation of hormone therapy on vascular risk. More recent investigations have revealed that both estrogens and gut microbiota can affect outcomes after stroke differently in males and females.Citation28,Citation29
It has become apparent that the state of the microbiome and the physiological health of the gut plays an important role in stroke outcome. A large body of evidence has identified a bidirectional system of communication between the gut and the brain after stroke.Citation30–33 Through “top-down” signaling of the brain-gut axis, stroke disrupts the normal function of the gut and alters the gut microbiota.Citation31,Citation32 Stroke leads to gut dysbiosis, defined as a pathological shift in the microbiota, and increased gut permeability, leading to an exacerbation of neuroinflammation.Citation31,Citation32,Citation34,Citation35 However, there are limited studies investigating if estrogen-mediated mechanisms are involved in the gut response to stroke. Whether estrogen contributes to gut barrier integrity and leads to a reduction in invading antigens and bacteria following stroke, is unknown.
In order to maintain intestinal homeostasis, intestinal epithelial cells (ECs) must respond to environmental signals. ECs have evolved protective mechanisms to maintain host barrier function to keep the microbiota at bay; by producing mucin (Muc) and antimicrobial proteins (AMPs).Citation36,Citation37 Mucins are secreted by goblet cells, a specialized type of ECs, and form a thick mucus layer that serves as a protective interface separating the luminal microbiota from the ECs.Citation38 AMPs, including regenerating islet-derived protein 3 genes (Reg3), are produced by specialized ECs and fortify the mucosal protective barrier through their antimicrobial effects.Citation36,Citation37
In this study, we hypothesized that stroke differentially alters the epithelial responses and the protective barrier function in a sexually dimorphic manner. We narrowed our focus to the colon since it harbors the greatest load and diversity of microbes.Citation39 As endogenous gonadal hormones are known to contribute to sex differences, and estrogens are potent neuroprotectants following experimental ischemic stroke,Citation40 we performed ovariectomy (OVX) in young females to examine the potential link between estrogens and EC-regulated gut protective mechanisms. Lastly, exogenous 17β-estradiol (E2) was given to aged female mice to determine if the female-specific protective mechanisms could be recapitulated in aged animals. We show that estrogen and the host microbiome play a coordinated role in stroke-induced colonic epithelial damage through mucin and AMP-regulated inflammatory responses that differ by sex.
Results
Stroke increases Reg3 family-specific antimicrobial genes in young male colonic ECs
We first examined the neurological deficit scores (NDS) of young males and females after stroke. One female mouse was excluded from the study as they died during the post-operative care period. As shown in , young females had lower NDS at post-MCAO day 7 compared to young males. In order to investigate potential sex differences in the epithelial antimicrobial responses in the colon after stroke, we isolated ECs and intraepithelial lymphocytes (IELs) from colonic tissue following a 60-minute middle cerebral artery occlusion (MCAO) and examined the expression of AMPs. As ECs and IELs both express genes for AMP proteins, e.g., Reg3γ,Citation41,Citation42 we separated ECs from IELs using Percoll density gradients, as in recent reports.Citation43,Citation44 We found that young male mice increased the mRNA expression of multiple Reg3 genes at day 7 post-stroke compared to young sham mice (). Reg3b, Reg3g and Reg4 increased ~24-fold (P = .0215), ~21-fold (P = .0161) and ~3-fold (P = .0659) on average, respectively. However, young female mice had no significant change in these AMP genes after stroke. We also evaluated the expression of other subtypes of AMPs that contribute to the protection of the epithelial barrier in the gut. Notably, stroke did not induce changes in Defa (for α-defensin) and Lyz1 (for lysozyme) genes in any of the groups (data not shown). These results indicate that AMP expression in young males is Reg3-specific after stroke.
Figure 1. Stroke increases Reg (males) and mucin-related genes (females) in colonic ECs.
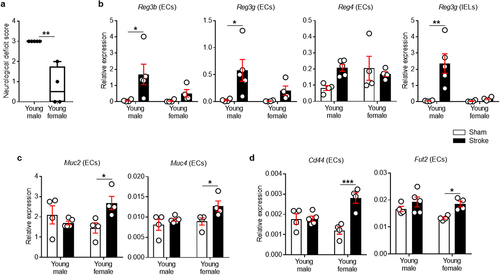
Young male lymphocytes within the colonic epithelium had increased expression of proinflammatory genes after stroke
IELs are a lymphocyte population intercalated between ECs in the intestinal epithelium. Both ECs and IELs play an essential role in host-microbiota homeostasis in the gut. In injured epithelia, IELs control the paracellular penetration of commensal bacteria by inducing both antibacterial and pro-inflammatory responses.Citation45 Thus, we asked if the closer contact of colonic epithelial surface with luminal bacteria in young males provokes immune responses from IELs after stroke. We isolated IELs from the same mice which were used for the EC study and quantified the expression of genes including Reg3g, Cxcl9, Il1b and Cxcl2/Mip2a. These have been previously recognized as microbiota-associated factors that are produced by IELs following epithelial damage.Citation45,Citation46 As shown in , only young male mice increased antimicrobial Reg3g expression after stroke (P = .0011). Further, young male mice had significantly higher expression of pro-inflammatory and chemotactic cytokines including Cxcl2/Mip2a (P = .0171), but not Cxcl9 and Il1b, after stroke, which was not seen in young females (Supplementary Figure S1). Given that IELs are situated on the basolateral side of ECs near tight junctions, our data supports the idea that the antigens approach and penetrate the host epithelium after stroke, resulting in the activation of IELs in response to the invading bacteria in young males after stroke.
Stroke enhances mucin-related genes in young female colonic ECs
Mucins provide the gut with a densely packed physical barrier that serves as the primary line of defense in mucosal protection.Citation47 Young male mice had no increase in Muc2 or Muc4 genes in their colonic ECs. However, in young female mice, there was a significant increase in mucin genes after stroke (, P = .0234 for Muc2 and P = .0434 for Muc4). CD44 signaling is critical for promoting both EC proliferationCitation48 and mucus secretion,Citation49 and therefore, we examined CD44 gene expression in colonic ECs. Stroke induced an increase in colonic epithelial Cd44 expression only in young female mice (P = .0003; ). To further elucidate the stroke-induced changes specific to young female mice, epithelial glycosylation was examined. The expression level of Fut2, a key enzyme that regulates the process of fucosylation,Citation50 was significantly increased in colonic ECs from young female mice (P = .0330; ). In contrast, there was no significant change in Fut2 mRNA levels in young males after stroke.
Young females maintain larger spatial separation between the colonic epithelium and commensal bacteria after stroke compared with young males
As mucin gene expression was significantly increased in young female mice after stroke, we hypothesized that the mucus layer would be thicker in the colonic region of young females, providing an increased barrier between the host and the luminal bacteria in the colon. For direct and comprehensive visualization of the entire bacterial population in the colon, bacterial fluorescence in situ hybridization (FISH) was performed by a blinded investigator, using a universal probe EUB338, which is specific for the bacterial 16S rRNA gene.Citation51 After stroke, young male mice failed to maintain this distance, and had more disruption of colonic crypt structures (). The colonic ECs in male mice were in close contact with bacteria, while in young females the crypt epithelial architecture remained intact and the distance between host and bacteria was maintained (). In addition, we performed Periodic acid – Schiff (PAS) staining to assess the number of goblet cells and their mucin production within the intestinal epithelium. demonstrates that young female mice had a thicker mucus layer compared to sham counterparts, suggesting that goblet cell function was not affected after stroke in young females, further supporting our bacteria FISH data shown in . The number of goblet cells remained the same across the experimental groups (data not shown).
Figure 2. Spatial segregation between the host colonic epithelium and commensal microbiota and fecal microbiota profiles differ in young male and female mice.
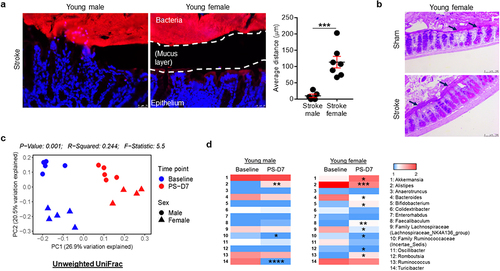
Stroke induces shifts in the gut microbiota composition in both young females and males
We employed 16S rRNA sequencing (16S rRNA-seq) using fecal samples to assess the gut microbiota composition and diversity in young mice of both sexes, before and after stroke (post-stroke day 7; PS-D7). Importantly, upon visualization of beta-diversity or between-samples diversity, with unweighted UniFrac distance by principal coordinate analysis (PCoA), we observed a notable clustering effect between the groups (). To further explore specific microbiota differences, we compared the 16S rRNA-seq data and analyses of relative abundances were limited to taxa classified at the genus level in the metagenomics datasets. When analyzing the data with a criterion of taxa with a P < .2 in either male or female groups at the genus level, we identified the 14 bacterial taxa using a Mann–Whitney U test (). We found that Alistipes was increased; whereas Turicibacter and Family Ruminococcaceae (Incertae_Sedis) were reduced in young males after stroke (). In contrast, young female mice showed changes of different genera including Akkermansia, Alistipes, Bacteroides, Bifidobacterium, Faecalibaculum, Family Lachnospiraceae (Lachnospiraceae_NK4A136_group), Family Ruminococcaceae (Incertae_Sedis), Romboutsia and Ruminococcus after stroke (unadjusted P < .05; ). Taken together, these results indicate that the gut microbiome dynamically responds to stroke-induced gut dysfunction in a sex-specific manner.
Ovariectomy worsens neurological deficits and alters epithelial defense mechanisms of young female mice after stroke
Estrogens, primarily estradiol (E2), have a protective role in experimental stroke,Citation52,Citation53 and this neuroprotective effect can be abolished by OVX and restored with exogenous E2 replacement.Citation54 Therefore, to understand the effect of ovarian hormones on the colonic epithelial responses to stroke, and to determine whether the mucin response is mediated by hormones, we ovariectomized young female mice 14 d prior to stroke and isolated colonic ECs at post-stroke day 7. Two female mice with both OVX and MCAO surgeries were excluded from the study as they died during the post-operative care period. We first examined if the removal of gonadal hormones affects post-stroke neurological deficits in young female mice. We found that OVX females had worse neurological deficits at post-stroke day 7, compared with non-OVX females (P = .0449; ). More interestingly, OVX led to the loss of the stroke-induced increase in mucin-related genes () that was seen in intact females after stroke. Instead, ovariectomized female mice had an up-regulation of AMP genes such as Reg3b and Reg3g (P = .0177 for Reg3b and P = .0228 for Reg3g; ), similar to the post-stroke response seen in young males. This implies that the lack of gonadal hormones (hormone-driven) or OVX-induced microbiota alteration (microbiota-driven) may cause female mice to initiate an epithelial response similar to that observed in males. To address these two possibilities and to determine if these changes were mediated specifically by hormonal effects on the epithelium in young females, we performed 16S rRNA-seq using fecal samples from OVX stroke mice and compared with the microbiome of non-OVX stroke mice. Interestingly, the microbiome between young males, young females and OVX females was distinctly clustered in PCoA analysis at day 7 after MCAO (). These data suggest that female gonadal hormones are a critical factor driving colonic mucin-related gene expression after stroke in young female mice and that sex differences in the protective strategies mounted by males (AMPs) and females (mucins) are hormonally controlled in young mice. The changes in gene expression in post-MCAO colonic ECs in both young males and females () and in OVX females () were further compared (see Supplementary Figure S2). When analyzed our 16S rRNA-seq data to detect similarities at the genus level in young males and young OVX females compared to young ovary-intact females after stroke, the relative abundance of Alistipes, Bifidobacterium, Dubosiella and Turicibacter was different between young males and young ovary-intact females. In addition, the relative abundance of Akkermansia, Alistipes, Bacteroides, Bifidobacterium, Colidextribacter, Faecalibaculum, Monoglobus, Oscillibacter, Roseburia and Turicibacter was different between OVX females and ovary-intact females (Supplementary Figure S3), further supporting that the microbiota changes could be involved in the gut response to ischemic brain injury.
Figure 3. Ovariectomy worsened neurological deficits and switches the protective mechanisms in the colonic epithelium of young females to a male-like pattern.
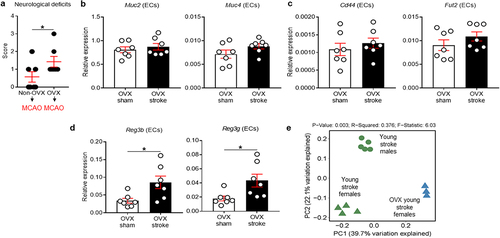
E2 replacement enhances mucin gene expression in the colonic epithelium of aged females after stroke
Finally, to determine if the female-specific colonic defense mechanisms were also seen in aged animals, and if these were mediated by estrogen, we examined gut epithelial mucin expression in reproductively senescent female mice. Aged females (18–20 months), which have low E2 levels compared to young and middle-aged female mice,Citation55 were supplemented with E2Citation16 for 2 weeks. The mice were subjected to MCAO and were euthanized at day 7. Five aged female mice (two MCAO mice with oil treatment and three MCAO mice with E2 treatment) were excluded from the study as they died during the post-operative care period. Next, we isolated colonic ECs from vehicle- and E2-treated aged stroke females and examined the expression levels of mucin genes. Interestingly, E2-replaced stroke mice showed higher expression of Muc2 (P = .0156) and Muc4 (P = .0003), compared with the vehicle-treated group (). Taken together, our data supports the idea that estrogen exerts beneficial effects on the gut after stroke.
Figure 4. Replacement of E2 restores mucin gene expression in colonic ECs, alters microbiome profiles, and reduces a marker of neuronal hyperactivity in the brain of aged stroke females.
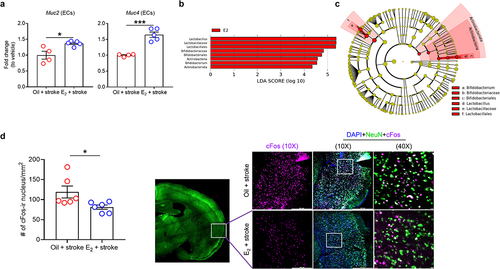
E2 replacement alters the gut microbiota composition after stroke and reduces a marker of neuronal activity in the insular cortex in aged stroke females
To further investigate whether the gut microbiota composition is associated with E2 levels in aged females after stroke, we used linear discriminant analysis (LDA) effect size to identify bacterial taxa that were differently enriched in fecal samples between mice treated with oil (vehicle) and E2 prior to stroke (P < .05, LDA score (log10) > 4.0). Across the two groups, a total of 8 enriched bacterial taxa were identified (). In the E2-treated mice, Lactobacilus, Lactobacillaceae, Lactobacillales, Bifidobacteriaceae, Bifidobacteriales, Actinobacteria, Bifidobacterium and Actinobacteriota were higher compared to the oil-treated group. All bacterial taxa identified across the two groups are listed from the highest to the lowest LDA scores and filtered based on the significance level (P < .05). represents the LEfSe results as a cladogram. When analyzed to identify any similarities in microbiota compositions of both young intact and E2-treated aged stroke female mice, we found a trend toward an increase in the relative abundance of Bifidobacterium in both groups when compared to their control groups (Supplementary Figure S4). Of note, Bifidobacterium species are well-known mucus enhancers in the colon by secreting beneficial metabolites such as short-chain fatty acids and neurotransmitters.Citation56,Citation57
It is known that estrogen can regulate neuronal activity in the insular cortex after stroke.Citation58 In addition, this region is highly associated with gut inflammation.Citation59 Therefore, we measured cFos in the insular cortex.Citation60 We found that aged female mice with E2 supplementation had significantly reduced cFos-positive neurons compared to the oil (vehicle)-treated group (P < .05; ), suggesting a dampening of an aberrant neuronal activation in the post-stroke brain.
Discussion
The intestinal epithelium is a critical interface for host-microbe symbiosis as it mediates the crosstalk between microbes and immune cells. We found that cells in the colonic epithelium (ECs and IELs) actively participated in stroke-induced and microbe-associated gut alterations. Estrogen reduces brain injury in experimental stroke via neuroprotective and anti-inflammatory actions.Citation40,Citation61 In this study, we extend the beneficial effects of estrogen to the gut. Our data shows that female gonadal hormones drive gut defense mechanisms in young female mice after stroke. Further, we demonstrated that loss of gonadal hormones altered gut defense mechanisms in female mice after stroke. Removal of the ovaries led to a loss of the stroke-induced increase of mucin genes, and instead, led to a significant increase in AMP genes, similar to males. Most importantly, this appears to be mediated by E2, as estrogen supplementation in aged female mice prior to stroke led to enhanced expression of Muc2 and Muc4 in the gut epithelium, similar to that seen in ovary-intact females.
It is well documented that young female mice are protected after experimental stroke compared to young and aged male mice and aged females.Citation62 In the gut, we found that ischemic stroke provoked a colonic response, and that these responses were sex-specific. Young male mice showed enhanced Reg-specific AMPs, e.g., Reg3b and Reg3g, while young females showed increased mucin-related gene expressions, e.g., Muc2 and Muc4. In addition, we profiled the bacterial compositions of the gut in young mice of both sexes after stroke to identify a possible role of the microbiome in stroke-initiated brain to gut signaling. Our data showed that stroke induces highly unique alterations in the fecal microbiota of mice in a sex-dependent manner. Young female mice preserve their colonic epithelial barrier by enhancing mucins after stroke. This host-bacteria interaction may be secondary to E2-enhanced mucin production in the colonic epithelium after stroke.
Previous studies have shown that the decline in estrogen levels after menopause reduces Lactobacilli dominance in the vaginal microenvironment, and estrogen replacement therapy restores this dominance.Citation63,Citation64 Here, we found that E2 supplementation increases the fecal composition of Lactobacillus and Bifidobacterium in aged stroke mice. Interestingly, these gut microbes produce β-glucosidase enzymes that can convert inactive estrogens into their active forms through deconjugation.Citation65,Citation66 Estrogens regulate immune and epithelial cell responses by regulating the release of bacterial metabolites to dampen inflammation, and by blocking the activation of endotoxins such as lipopolysaccharides. Taken together, our findings suggest that estrogens can reshape the gut microbiome and protect female-specific colonic epithelial cell responses to stroke.
The insular cortex regulates the connectivity between afferent and efferent fibers of visceral organs.Citation67 Increasing evidence suggests that the insula regulates the inflammatory status of the gut.Citation59 Previous studies have shown that estrogen reduces neuronal excitability and stroke-induced cell death in the insular cortex; however, these studies only examined changes at acute time points and only assessed young animals, and gut responses were not evaluated.Citation58 Here, we found that E2 supplementation in aged female mice prior to stroke has the potential to reduce the levels of cFos in the ipsilateral insula, suggesting that reduced neuronal excitability might be associated with the alterations in colonic EC responses regulated by E2 after stroke.
It is well known that estrogen is neuroprotective;Citation40 one important caveat of this study is that some of the colonic changes seen may be related to the size of the infarct. In addition, post-stroke outcomes and functional recovery are also mediated by the sex chromosome compliment (XX vs. XY).Citation68 However, sex-specific epithelial responses in the gut have not been previously investigated, adding considerable novelty to our findings. Thus, future investigations of how infarct size and location contribute to the colonic epithelial responses after stroke and examination of the changes in EC responses at chronic time points after stroke are needed. Future studies examining post-stroke colonic epithelial responses that incorporate a mouse model such as the four-core genotype model (i.e., XX and XY males and XX and XY females) to differentiate the role of gonadal hormones vs. the sex chromosome complement are also warranted. Other limitations of the study include 1) Changes were analyzed at only one timepoint (post-stroke day 7); 2) The lack of deep microbiome profiling, using shotgun sequencing; 3) The neuronal activity was analyzed using one marker (cFos); and 4) The possibility of involvement of other brain areas and detailed electrophysiological studies are still needed.
In conclusion, our current study demonstrates that the colonic epithelium, which contributes to both mechanical and immunologic responses that regulate host-microbiota crosstalk in the gut, dynamically responds to brain injury. Importantly, several key chemical and biological niches in the colonic epithelium that protect the host against harmful factors initiated by brain injury, including mucins and AMPs were significantly altered in mice after stroke. These changes in the host colon, and in the microbiota composition, were sex-specific and were estrogen dependent. Our findings demonstrate the importance of studying both sexes and further highlight potential interactions between sex, the gut microbiome and immunity in age-related neurological diseases such as stroke.
Materials and methods
Mice
All animal protocols were approved by the Institutional Animal Care and Use Committee at the University of Texas Health Science Center at Houston (UTHealth). This study was performed in accordance with the guidelines provided by the National Institutes of Health (NIH) and followed RIGOR guidelines.Citation69 Young male and female C57BL/6J mice (2-month) were purchased from Jackson Laboratory and acclimatized for a minimum of 1 month before use. Aged female C57BL/6J mice (18–20 months) were raised in our animal facilities. All mice were housed in ventilated cages with irradiated corncob bedding (1/8”), mice were received filtered tap water and irradiated and nutritionally balanced rodent diet (PicoLab® rodent diet 20 5053, LabDiet). To determine the effect of female gonadal hormones, one cohort of young female mice was ovariectomized 2 weeks prior to stroke. Another cohort of aged female mice was subcutaneously implanted with E2 pellets (Sigma-Aldrich) or sesame oil as a vehicle 2 weeks prior to stroke.Citation70
Experimental stroke
A 60-minute middle cerebral artery occlusion (MCAO) was achieved by inserting a silicone coated suture (0.21 or 0.23 mm diameter for young and aged mice respectively) into the external carotid artery (ECA).Citation33,Citation71 The suture was advanced into the circle of Willis of mice to occlude the MCA. The animal was awakened from anesthesia and intra-ischemic focal deficits were confirmed. After 60 minutes, the mouse was re-anesthetized and the suture was removed allowing for reperfusion. Sham control mice underwent the same surgical procedure and exposure of the ECA but the suture was not inserted into the MCA. All mice were randomly assigned to stroke or sham cohorts. All surgeries were conducted using isoflurane anesthesia with temperature maintenance.
Isolation of colonic ECs and intraepithelial lymphocytes (IELs)
Colonic ECs and IELs were isolated at post-stroke day 7, as previously described.Citation43,Citation44 Briefly, colonic tissue was harvested from sham and stroke mice at day 7 and analyzed by an investigator blinded to treatment group. After removal of the cecum, the tissues were opened longitudinally and rinsed with ice-cold RPMI-1640 (Corning). The tissue was incubated with RPMI-1640 containing 2 mM EDTA (Invitrogen) and 10% FBS (Gibco) for 30 min at 37°C. The resulting solution containing digested tissues was passed through a nylon mesh (70 μm, Falcon). The filtrate was applied to Percoll (GE Healthcare) density gradients: 25%, 40% and 75%. After centrifugation at 500 g for 20 min, the interface between gradients were collected to obtain ECs (25% and 40%) and IELs (40% and 75%).
Quantification of mRNAs
Total RNA was isolated from the colonic ECs and IELs using Trizol (Invitrogen). cDNA was synthesized from 500 ng of RNA using iScript Reverse Transcription Supermix for RT-qPCR kit (Bio-Rad). Beta-actin and glyceraldehyde-3-phosphate dehydrogenase (GAPDH) were used as internal controls to normalize the expression level of ECs and IELs mRNAs, respectively. Real-time quantitative PCR (RT-qPCR) using cDNA was performed by using SsoAdvancedTM Universal SYBRⓇ Green Supermix (Bio-Rad) in CFX96 TouchTM Real-Time PCR Detection System (Bio-Rad). The list of primers used in this study along with their sequences are listed in Supplementary Table S1.
Bacterial fluorescence in situ hybridization (FISH) and PAS staining
Bacterial FISH was performed as previously described.Citation72 Briefly, colonic tissue was isolated from sham and stroke mice at day 7 and fixed in Carnoy’s solution. Paraffin-embedded tissues were sectioned at 5 μm. For bacterial FISH, the sections were hybridized with Cy3-labeled EUB338 probe (primer sequence: GCTGCCTCCCGTAGGAG) at 51°C overnight (Integrated DNA Technologies). The hybridized sections were co-stained with 4’,6-diamidino-2-phenylindole (DAPI, Sigma-Aldrich). The slides were analyzed by confocal microscopy at a wavelength of 330 to 700 nm (Leica DMi8 and TCS SPE microsystems) by a blinded investigator. For PAS staining, colonic tissue sections were subjected to periodic acid (0.5% solution) for 5 min and Schiff reagent (basic fuchsin, sodium metabisulfite and 1N hydrochloric acid in distilled water) for 15 min. The slides were analyzed by bright field microscopy by a blinded investigator.
16S ribosomal RNA sequencing (16S rRNA-seq) and analysis
Fecal samples (i.e., stool samples) were collected from sham and stroke mice between 9 and 10 am at baseline and at post-stroke day 7 and immediately frozen at −80°C until the analysis. Fecal DNA was extracted using MO BIO’s PowerMag Soil DNA Isolation kit (MO BIO). Using high-throughput sequencing (Illumina MiSeq platform; Illumina, San Diego, CA), bacterial taxa from the fecal samples were analyzed via amplification of the V4 region of the 16S rRNA gene.Citation32,Citation73 The data was analyzed using the Agile Toolkit for Incisive Microbial Analyses (ATIMA), a software developed by the Alkek Center for Metagenomics and Microbiome Research (CMMR) at the Baylor College of Medicine.Citation74 Galaxy online interface Version 1.0 (https://huttenhower.sph.harvard.edu/galaxy/) was used to perform linear discriminant analysis (LDA) and to generate cladogram and LDA effect size (LEfSe) plots.Citation75
Immunohistochemistry
Brain tissues were collected from perfused mice and sectioned at a 30-micron thickness. Free floating brain sections were incubated in blocking solution followed by antigen retrieval (BioGenex, HK086-9K) as per manufacturer’s instructions. Sections were incubated in blocking solution (2% donkey normal serum + 0.1% BSA + 0.2% Triton X-100 in PBS) for 1 h at room temperature, followed by the incubation with anti-cFos (Oncogene Research Products, PC38; 1:20,000); Alexa Fluor 488 pre-conjugated anti-NeuN (EMD Millipore, MAB377X; 1:500) in blocking solution overnight at room temperature. The sections were washed with PBS three times for 5 min, and then Alexa Fluor 647 pre-conjugated donkey anti-rabbit IgG (H+L) (Jackson ImmunoResearch Laboratories, 711-605-152; 1:2,000) was used as secondary antibody to detect the cFos expression. The sections were mounted on slides and then stained with DAPI (Sigma-Aldrich, F6057). Sections were imaged using Leica Thunder Imager DMi8 microscope (Leica, Heerbrugg, Switzerland). Cell counts from images were analyzed using ImageJ by an investigator blinded to treatment condition.
Statistics
Two-way ANOVA was used to analyze data with two factors including sex and control/stroke, followed by the post-hoc stroke vs control group comparison within males and females, respectively. Multiple testing adjustment was performed by Sidak method. For two group comparisons, Student’s t-test or Mann–Whitney U test were used and the normality of data was confirmed by the Shapiro–Wilk normality test. P value less than 0.05 was considered as significant. GraphPad Prism 7.03 was used for all data analysis.
Supplemental Material
Download MS Word (237.2 KB)Acknowledgments
We thank Dr Eunyoung Lee for her help with the statistical review.
Disclosure statement
No potential conflict of interest was reported by the author(s).
Data availability statement
The raw reads of Illumina sequencing have been submitted to the Sequence Read Archive (SRA) in the National Center for Biotechnology Information (NCBI) under BioProject accession number PRJNA628128 https://www.ncbi.nlm.nih.gov/bioproject/PRJNA628128. All data sets and supporting material details of this study are available from the corresponding or first author of the article upon reasonable request from qualified investigators.
Supplementary material
Supplemental data for this article can be accessed online at https://doi.org/10.1080/19490976.2023.2271629.
Additional information
Funding
References
- Mozaffarian D, Benjamin EJ, Go AS, Arnett DK, Blaha MJ, Cushman M, de Ferranti S, Després J-P, Fullerton HJ, Howard VJ, et al. Heart disease and stroke statistics—2015 update. Circulation. 2015;131(4):e29. doi:10.1161/CIR.0000000000000152.
- Chauhan A, Moser H, McCullough Louise D. Sex differences in ischaemic stroke: potential cellular mechanisms. Clin Sci. 2017;131(7):533–14. doi:10.1042/CS20160841.
- Spychala MS, Honarpisheh P, McCullough LD. Sex differences in neuroinflammation and neuroprotection in ischemic stroke. J Neurosci Res. 2017;95(1–2):462–471. doi:10.1002/jnr.23962.
- Markle JG, Frank DN, Mortin-Toth S, Robertson CE, Feazel LM, Rolle-Kampczyk U, von Bergen M, McCoy KD, Macpherson AJ, Danska JS, et al. Sex differences in the gut microbiome drive hormone-dependent regulation of autoimmunity. Sci. 2013;339(6123):1084–1088. doi:10.1126/science.1233521.
- Yurkovetskiy L, Burrows M, Khan AA, Graham L, Volchkov P, Becker L, Antonopoulos D, Umesaki Y, Chervonsky A. Gender bias in autoimmunity is influenced by microbiota. Immunity. 2013;39(2):400–412. doi:10.1016/j.immuni.2013.08.013.
- Klein SL. The effects of hormones on sex differences in infection: from genes to behavior. Neurosci Biobehav Rev. 2000;24(6):627–638. doi:10.1016/S0149-7634(00)00027-0.
- Dhandapani KM, Brann DW. Protective effects of estrogen and selective estrogen receptor modulators in the brain. Biol Reprod. 2002;67(5):1379–1385. doi:10.1095/biolreprod.102.003848.
- Green PS, Simpkins JW. Neuroprotective effects of estrogens: potential mechanisms of action. Int J Dev Neurosci. 2000;18:347–358. doi:10.1016/S0736-5748(00)00017-4.
- Hurn PD, Macrae IM. Estrogen as a neuroprotectant in stroke. J Cereb Blood Flow Metab. 2000;20:631–652. doi:10.1097/00004647-200004000-00001.
- Murphy S, McCullough L, Littleton-Kearney M, Hurn P. Estrogen and selective estrogen receptor modulators: neuroprotection in the women’s Health initiative era. Endocrine. 2003;21:17–26. doi:10.1385/ENDO:21:1:17.
- Wise PM, Dubal DB. Estradiol protects against ischemic brain injury in middle-aged rats. Biol Reprod. 2000;63(4):982–985. doi:10.1095/biolreprod63.4.982.
- McCullough LD, Alkayed NJ, Traystman RJ, Williams MJ, Hurn PD. Postischemic estrogen reduces hypoperfusion and secondary ischemia after experimental stroke. Stroke. 2001;32(3):796–802. doi:10.1161/01.STR.32.3.796.
- Harukuni I, Hurn PD, Crain BJ. Deleterious effect of β-estradiol in a rat model of transient forebrain ischemia. Brain Res. 2001;900(1):137–142. doi:10.1016/S0006-8993(01)02278-8.
- Wang L, Kitano H, Hurn PD, Murphy SJ. Estradiol attenuates neuroprotective benefits of isoflurane preconditioning in ischemic mouse brain. J Cereb Blood Flow Metab. 2008;28:1824–1834. doi:10.1038/jcbfm.2008.70.
- Santizo RA, Xu HL, Ye S, Baughman VL, Pelligrino DA. Loss of benefit from estrogen replacement therapy in diabetic ovariectomized female rats subjected to transient forebrain ischemia. Brain Res. 2002;956(1):86–95. doi:10.1016/S0006-8993(02)03484-4.
- Liu F, Benashski SE, Xu Y, Siegel M, McCullough LD. Effects of chronic and acute oestrogen replacement therapy in aged animals after experimental stroke. J Neuroendocrinol. 2012;24(2):319–330. doi:10.1111/j.1365-2826.2011.02248.x.
- Cai M, Ma YL, Qin P, Li Y, Zhang LX, Nie H, Peng Z, Dong H, Dong H-L, Hou W-G, et al. The loss of estrogen efficacy against cerebral ischemia in aged postmenopausal female mice. Neurosci Lett. 2014;558:115–119. doi:10.1016/j.neulet.2013.11.007.
- Paganini-Hill A, Ross RK, Henderson BE. Postmenopausal oestrogen treatment and stroke: a prospective study. BMJ ( Clinical research ed). 1988;297(6647):519–522. doi: 10.1136/bmj.297.6647.519.
- Hunt K, Vessey M, McPherson K. Mortality in a cohort of long-term users of hormone replacement therapy: an updated analysis. Br J Obstet Gynaecol. 1990;97(12):1080–1086. doi:10.1111/j.1471-0528.1990.tb02494.x.
- Finucane FF, Madans JH, Bush TL, Wolf PH, Kleinman JC. Decreased risk of stroke among postmenopausal hormone users. Results From a National Cohort. Arch Intern Med. 1993;153(1):73–79. doi:10.1001/archinte.153.1.73.
- Falkeborn M, Persson I, Terént A, Adami HO, Lithell H, Bergström R. Hormone replacement therapy and the risk of stroke. Follow-up of a population-based cohort in Sweden. Arch Intern Med. 1993;153(10):1201–1209. doi:10.1001/archinte.1993.00410100035005.
- Rossouw JE, Anderson GL, Prentice RL, LaCroix AZ, Kooperberg C, Stefanick ML, Jackson RD, Beresford SAA, Howard BV, Johnson KC et al. Group for the Women's Health Initiative Investigators. Risks and benefits of estrogen plus progestin in healthy postmenopausal women: principal results from the women’s Health initiative randomized controlled trial. JAMA. 2002;288(3):321–333. doi:10.1001/jama.288.3.321.
- Anderson GL, Limacher M, Assaf AR, Bassford T, Beresford SA, Black H, Bonds D, Brunner R, Brzyski R, Caan B et al. Women's Health Initiative Steering Committee. Effects of conjugated equine estrogen in postmenopausal women with hysterectomy: the women’s Health initiative randomized controlled trial. JAMA. 2004;291(14):1701–1712.
- Mohammed K, Abu Dabrh AM, Benkhadra K, Al Nofal A, Carranza Leon BG, Prokop LJ, Montori VM, Faubion SS, Murad MH. Oral vs Transdermal estrogen therapy and vascular events: a systematic review and meta-analysis. J Clin Endocr Metab. 2015;100(11):4012–4020. doi:10.1210/jc.2015-2237.
- Marjoribanks J, Farquhar C, Roberts H, Lethaby A, Lee J. Long term hormone therapy for perimenopausal and postmenopausal women Cochrane Database Syst Rev. 2017; 1(1): Cd004143. doi:10.1002/14651858.
- Viscoli CM, Brass LM, Kernan WN, Sarrel PM, Suissa S, Horwitz RI. A clinical trial of estrogen-replacement therapy after ischemic stroke. N Engl J Med. 2001;345(17):1243–1249. doi:10.1056/NEJMoa010534.
- Hodis HN, Mack WJ, Henderson VW, Shoupe D, Budoff MJ, Hwang-Levine J, Li Y, Feng M, Dustin L, Kono N, et al. Vascular effects of Early versus late postmenopausal treatment with Estradiol. N Engl J Med. 2016;374(13):1221–1231. doi:10.1056/NEJMoa1505241.
- Park MJ, Pilla R, Panta A, Pandey S, Sarawichitr B, Suchodolski J, Sohrabji F. Reproductive senescence and ischemic stroke remodel the gut microbiome and modulate the effects of estrogen treatment in female rats. Transl Stroke Res. 2020;11(4):812–830. doi:10.1007/s12975-019-00760-5.
- Ahnstedt H, Patrizz A, Chauhan A, Roy-O’Reilly M, Furr JW, Spychala MS, D’Aigle J, Blixt FW, Zhu L, Bravo Alegria J, et al. Sex differences in T cell immune responses, gut permeability and outcome after ischemic stroke in aged mice. Brain Behav Immun. 2020;87:556–567. doi:10.1016/j.bbi.2020.02.001.
- Benakis C, Brea D, Caballero S, Faraco G, Moore J, Murphy M, Sita G, Racchumi G, Ling L, Pamer EG, et al. Commensal microbiota affects ischemic stroke outcome by regulating intestinal γδ T cells. Nat Med. 2016;22(5):516. doi:10.1038/nm.4068.
- Singh V, Roth S, Llovera G, Sadler R, Garzetti D, Stecher B, Dichgans M, Liesz A. Microbiota dysbiosis controls the neuroinflammatory response after stroke. J Neurosci. 2016;36(28):7428. doi:10.1523/JNEUROSCI.1114-16.2016.
- Spychala MS, Venna VR, Jandzinski M, Doran SJ, Durgan DJ, Ganesh BP, Ajami NJ, Putluri N, Graf J, Bryan RM, et al. Age-related changes in the gut microbiota influence systemic inflammation and stroke outcome. Ann Neurol. 2018;84(1):23–36. doi:10.1002/ana.25250.
- Lee J, d’Aigle J, Atadja L, Quaicoe V, Honarpisheh P, Ganesh BP, Hassan A, Graf J, Petrosino J, Putluri N, et al. Gut microbiota–derived short-chain fatty acids promote poststroke recovery in aged mice. Circ Res. 2020;127(4):453–465. doi:10.1161/CIRCRESAHA.119.316448.
- Crapser J, Ritzel R, Venna VR, Liu F, Chauhan A, Koellhoffer E, Koellhoffer E, Patel A, Ricker A, Maas K, et al. Ischemic stroke induces gut permeability and enhances bacterial translocation leading to sepsis in aged mice. Aging. 2016;8(5):1049–1063. doi:10.18632/aging.100952.
- Stanley D, Mason LJ, Mackin KE, Srikhanta YN, Lyras D, Prakash MD, Nurgali K, Venegas A, Hill MD, Moore RJ, et al. Translocation and dissemination of commensal bacteria in post-stroke infection. Nat Med. 2016;22(11):1277–1284. doi:10.1038/nm.4194.
- Artis D. Epithelial-cell recognition of commensal bacteria and maintenance of immune homeostasis in the gut. Nat Rev Immunol. 2008;8(6):411. doi:10.1038/nri2316.
- Ouellette AJ. Paneth cells and innate mucosal immunity. Curr Opin Gastroenterol. 2010;26(6):547–553. doi:10.1097/MOG.0b013e32833dccde.
- Pelaseyed T, Bergström JH, Gustafsson JK, Ermund A, Birchenough GMH, Schütte A, van der Post S, Svensson F, Rodríguez‐Piñeiro AM, Nyström EEL, et al. The mucus and mucins of the goblet cells and enterocytes provide the first defense line of the gastrointestinal tract and interact with the immune system. Immunol Rev. 2014;260(1):8–20. doi:10.1111/imr.12182.
- Pereira FC, Berry D. Microbial nutrient niches in the gut. Environ Microbiol. 2017;19(4):1366–1378. doi:10.1111/1462-2920.13659.
- McCullough LD, Hurn PD. Estrogen and ischemic neuroprotection: an integrated view. Trends Endocrinol Metab. 2003;14(5):228–235. doi:10.1016/S1043-2760(03)00076-6.
- Vaishnava S, Yamamoto M, Severson KM, Ruhn KA, Yu X, Koren O, Ley R, Wakeland EK, Hooper LV. The antibacterial lectin RegIIIγ promotes the spatial segregation of microbiota and host in the intestine. Sci. 2011;334(6053):255–258. doi:10.1126/science.1209791.
- Ismail AS, Severson KM, Vaishnava S, Behrendt CL, Yu X, Benjamin JL, Ruhn KA, Hou B, DeFranco AL, Yarovinsky F, et al. γδ intraepithelial lymphocytes are essential mediators of host–microbial homeostasis at the intestinal mucosal surface. Proc Natl Acad Sci USA. 2011;108(21):8743–8748. doi:10.1073/pnas.1019574108.
- Lee J, Park EJ, Yuki Y, Ahmad S, Mizuguchi K, Ishii KJ, Shimaoka M, Kiyono H. Profiles of microRNA networks in intestinal epithelial cells in a mouse model of colitis. Sci Rep. 2015;5(1):18174. doi:10.1038/srep18174.
- Lee J, Mohsen A, Banerjee A, McCullough LD, Mizuguchi K, Shimaoka M, Kiyono H, Park EJ. Distinct age-specific miRegulome profiling of isolated small and large intestinal epithelial cells in mice. Int J Mol Sci. 2021;22(7):3544. doi:10.3390/ijms22073544.
- Ismail AS, Behrendt CL, Hooper LV. Reciprocal interactions between commensal bacteria and γδ intraepithelial lymphocytes during mucosal injury. J Immunol. 2009;182(5):3047–3054. doi:10.4049/jimmunol.0802705.
- Cash HL, Whitham CV, Behrendt CL, Hooper LV. Symbiotic bacteria direct expression of an intestinal bactericidal lectin. Sci. 2006;313(5790):1126–1130. doi:10.1126/science.1127119.
- AP C. Mucins: a biologically relevant glycan barrier in mucosal protection. Biochim et Biophys Acta (BBA) - Gen Subj. 2015;1850(1):236–252. doi:10.1016/j.bbagen.2014.05.003.
- Riehl TE, Santhanam S, Foster L, Ciorba M, Stenson WF. CD44 and TLR4 mediate hyaluronic acid regulation of Lgr5+ stem cell proliferation, crypt fission, and intestinal growth in postnatal and adult mice. Am J Physiol Gastrointest Liver Physiol. 2015;309(11):G874–87. doi:10.1152/ajpgi.00123.2015.
- Yu H, Li Q, Zhou X, Kolosov VP, Perelman JM. Role of hyaluronan and CD44 in reactive oxygen species-induced mucus hypersecretion. Mol Cell Biochem. 2011;352(1–2):65–75. doi:10.1007/s11010-011-0740-6.
- Magalhães A, Rossez Y, Robbe-Masselot C, Maes E, Gomes J, Shevtsova A, Bugaytsova J, Borén T, Reis CA. Muc5ac gastric mucin glycosylation is shaped by FUT2 activity and functionally impacts Helicobacter pylori binding. Sci Rep. 2016;6(1):25575. doi:10.1038/srep25575.
- Harmsen H, Prieur D, Jeanthon C. Group-specific 16S rRNA-targeted oligonucleotide probes to identify thermophilic bacteria in marine hydrothermal vents. Appl Environ Microb. 1997;63(10):4061–4068. doi:10.1128/aem.63.10.4061-4068.1997.
- Alkayed NJ, Harukuni I, Kimes AS, London ED, Traystman RJ, Hurn PD. Gender-linked brain injury in experimental stroke. Stroke ( discussion 66). 1998;29(1):159–165. doi: 10.1161/01.STR.29.1.159.
- Liu F, Benashski SE, Xu Y, Siegel M, McCullough LD. Effects of chronic and acute oestrogen replacement therapy in aged animals after experimental stroke. J Neuroendocrinol. 2011;24(2):319–330. doi:10.1111/j.1365-2826.2011.02248.x.
- Dubal DB, Zhu H, Yu J, Rau SW, Shughrue PJ, Merchenthaler I, Kindy MS, Wise PM. Estrogen receptor α, not β, is a critical link in estradiol-mediated protection against brain injury. Proc Natl Acad Sci U S A. 2001;98(4):1952–1957. doi:10.1073/pnas.98.4.1952.
- Manwani B, Liu F, Scranton V, Hammond MD, Sansing LH, McCullough LD. Differential effects of aging and sex on stroke induced inflammation across the lifespan. Exp Neurol. 2013;249:120–131. doi:10.1016/j.expneurol.2013.08.011.
- Engevik MA, Luk B, Chang-Graham AL, Hall A, Herrmann B, Ruan W, Endres BT, Shi Z, Garey KW, Hyser JM, et al. Bifidobacterium dentium fortifies the intestinal mucus layer via autophagy and calcium signaling pathways. mBio. 2019;10(3):10. doi:10.1128/mBio.01087-19.
- Schroeder BO, Birchenough GMH, Ståhlman M, Arike L, Johansson MEV, Hansson GC, Bäckhed F. Bifidobacteria or fiber protects against diet-induced microbiota-mediated colonic mucus deterioration. Cell Host & Microbe. 2018;23(1):27–40.e7. doi:10.1016/j.chom.2017.11.004.
- Saleh TM, Connell BJ, Legge C, Cribb AE. Estrogen attenuates neuronal excitability in the insular cortex following middle cerebral artery occlusion. Brain Res. 2004;1018(1):119–129. doi:10.1016/j.brainres.2004.05.074.
- Koren T, Yifa R, Amer M, Krot M, Boshnak N, Ben-Shaanan TL, Azulay-Debby H, Zalayat I, Avishai E, Hajjo H, et al. Insular cortex neurons encode and retrieve specific immune responses. Cell. 2021;184(24):5902–15.e17. doi:10.1016/j.cell.2021.10.013.
- Bullitt E. Expression of c-fos-like protein as a marker for neuronal activity following noxious stimulation in the rat. J Comp Neurol. 1990;296(4):517–530. doi:10.1002/cne.902960402.
- McCullough LD, Blizzard K, Simpson ER, Öz OK, Hurn PD. Aromatase cytochrome P450 and extragonadal estrogen play a role in ischemic neuroprotection. J Neurosci. 2003;23(25):8701. doi:10.1523/JNEUROSCI.23-25-08701.2003.
- Liu F, Yuan R, Benashski SE, McCullough LD. Changes in experimental stroke outcome across the life span. J Cereb Blood Flow Metab. 2009;29(4):792–802. doi:10.1038/jcbfm.2009.5.
- Amabebe E, Anumba DOC. The vaginal microenvironment: the physiologic role of lactobacilli. Front Med. 2018;5:181. doi:10.3389/fmed.2018.00181.
- Gupta S, Kumar N, Singhal N, Kaur R, Manektala U. Vaginal microflora in postmenopausal women on hormone replacement therapy. Indian J Pathol Microbiol. 2006;49:457–461.
- Flores R, Shi J, Gail MH, Gajer P, Ravel J, Goedert JJ, Highlander SK. Association of fecal microbial diversity and taxonomy with selected enzymatic functions. PloS One. 2012;7(6):e39745. doi:10.1371/journal.pone.0039745.
- Acharya KD, Gao X, Bless EP, Chen J, Tetel MJ. Estradiol and high fat diet associate with changes in gut microbiota in female ob/ob mice. Sci Rep. 2019;9(1):20192. doi:10.1038/s41598-019-56723-1.
- Montalbano MJ, Shane Tubbs R. Lateralization of the insular cortex. In: Turgut M, Yurttaş C Tubbs R, editors Island of Reil (insula) in the human brain: anatomical, functional, clinical and surgical aspects. Cham: Springer International Publishing; 2018. pp. 129–132.
- McCullough LD, Mirza MA, Xu Y, Bentivegna K, Steffens EB, Ritzel R, Liu F. Stroke sensitivity in the aged: sex chromosome complement vs. gonadal hormones. Aging (Albany NY). 2016;8(7):1432–1441. doi:10.18632/aging.100997.
- Lapchak PA, Zhang JH, Noble-Haeusslein LJ. RIGOR guidelines: escalating STAIR and STEPS for effective translational research. Transl Stroke Res. 2013;4(3):279–285. doi:10.1007/s12975-012-0209-2.
- McCullough LD, Zeng Z, Blizzard KK, Debchoudhury I, Hurn PD. Ischemic nitric oxide and poly (ADP-ribose) polymerase-1 in cerebral ischemia: male toxicity, female protection. J Cereb Blood Flow Metab. 2005;25:502–512. doi:10.1038/sj.jcbfm.9600059.
- McCullough LD, Roy-O’Reilly M, Lai YJ, Patrizz A, Xu Y, Lee J, Holmes A, Kraushaar DC, Chauhan A, Sansing LH, et al. Exogenous inter-α inhibitor proteins prevent cell death and improve ischemic stroke outcomes in mice. J Clin Invest. 2021;131(17):131. doi:10.1172/JCI144898.
- Ganesh BP, Hall A, Ayyaswamy S, Nelson JW, Fultz R, Major A, Haag A, Esparza M, Lugo M, Venable S, et al. Diacylglycerol kinase synthesized by commensal Lactobacillus reuteri diminishes protein kinase C phosphorylation and histamine-mediated signaling in the mammalian intestinal epithelium. Mucosal Immunol. 2017;11(2):380–393. doi:10.1038/mi.2017.58.
- Honarpisheh P, Reynolds CR, Blasco Conesa MP, Moruno Manchon JF, Putluri N, Bhattacharjee MB, Urayama A, McCullough LD, Ganesh BP. Dysregulated gut homeostasis observed prior to the accumulation of the brain amyloid-β in Tg2576 mice. Int J Mol Sci. 2020;21(5):1711. doi:10.3390/ijms21051711.
- Rosshart SP, Vassallo BG, Angeletti D, Hutchinson DS, Morgan AP, Takeda K, Hickman HD, McCulloch JA, Badger JH, Ajami NJ, et al. Wild mouse gut microbiota promotes host fitness and improves disease resistance. Cell. 2017;171(5):1015–28.e13. doi:10.1016/j.cell.2017.09.016.
- Segata N, Izard J, Waldron L, Gevers D, Miropolsky L, Garrett WS, Huttenhower C. Metagenomic biomarker discovery and explanation. Genome Biol. 2011;12(6):R60. doi:10.1186/gb-2011-12-6-r60.