ABSTRACT
In many Vibrio species, virulence is regulated by quorum sensing, which is regulated by a complex, multichannel, two-component phosphorelay circuit. Through this circuit, sensor kinases transmit sensory information to the phosphotransferase LuxU via a phosphotransfer mechanism, which in turn transmits the signal to the response regulator LuxO. For Vibrio parahaemolyticus, type III secretion system 1 (T3SS1) is required for cytotoxicity, but it is unclear how quorum sensing regulates T3SS1 expression. Herein, we report that a hybrid histidine kinase, ArcB, instead of LuxU, and sensor kinase LuxQ and response regulator LuxO, collectively orchestrate T3SS1 expression in V. parahaemolyticus. Under high oxygen conditions, LuxQ can interact with ArcB directly and phosphorylates the Hpt domain of ArcB. The Hpt domain of ArcB phosphorylates the downstream response regulator LuxO instead of ArcA. LuxO then activates transcription of the T3SS1 gene cluster. Under hypoxic conditions, ArcB autophosphorylates and phosphorylates ArcA, whereas ArcA does not participate in regulating the expression of T3SS1. Our data provides evidence of an alternative regulatory path involving the quorum sensing phosphorelay and adds another layer of understanding about the environmental regulation of gene expression in V. parahaemolyticus.
Introduction
Vibrio parahaemolyticus is a leading cause of seafood-derived vibriosis, which is characterized by acute gastroenteritis.Citation1,Citation2 Such infections can lead to septicemia, which is life-threatening to those with preexisting medical conditions.Citation3 The pandemic V. parahaemolyticus strain RIMD 2210633 expresses multiple virulence determinants including type III secretion system 1 (T3SS1) and system 2 (T3SS2).Citation4 The V. parahaemolyticus T3SS2 is found in clinical isolates, is critical for the diarrhea‐inducing activity and can cause fluid accumulation, epithelial damage, and inflammation in the intestine.Citation4–6 In contrast, T3SS1 is present in all V. parahaemolyticus isolates, contributes to V. parahaemolyticus-induced cytotoxicity in vitro and is involved in lethal activity in a murine infection model in vivo.Citation7,Citation8 Therefore, T3SS1 may be related to V. parahaemolyticus-induced septicemia in humans.
The expression of T3SS1 is regulated by the ExsACDE operon. When V. parahaemolyticus contacts host cells (such as HeLa or Caco-2 cells), or when cultured in Dulbecco’s modified Eagle’s medium (DMEM), T3SS1 gene expression is upregulated.Citation9 Under these inducing conditions, ExsA activates the expression of T3SS1 related genes. ExsD can directly interact with ExsA to prevent this activation,Citation10 while ExsC can directly bind ExsD to free ExsA and permit the expression of T3SS1 genes.Citation11 ExsE binding to ExsC disrupts the complex between ExsC and ExsD, thereby allowing free ExsD to bind ExsA.Citation12
Vibrio sp. coordinate gene expression using a process of intercellular communication called quorum sensing (QS), which involves synthesis, excretion, and responses to signaling molecules called autoinducers (AIs).Citation13 V. parahaemolyticus produces three AIs, including harveyi autoinducer 1 (HAI-1), autoinducer 2 (AI-2) and cholerae autoinducer 1 (CAI-1), which are detected by sensor kinases (SKs) LuxN, LuxP/LuxQ and CqsS respectively.Citation14 After an AI binds to its corresponding receptor, signal transduction coordinates a phenotypic response across a bacterial population, such as formation of biofilms, initiation of infection, or bioluminescence.Citation15 QS is important for controlling T3SS1 expression in V. parahaemolyticus RIMD 2210633, including the QS regulator OpaR,Citation16 which inhibits T3SS1 expression, and AphA, which activates T3SS1 gene transcription at low cell densities.Citation14,Citation17 A third QS regulator, QsvR, interacts with the regulatory regions of aphA and opaR to inhibit or activate their transcription, respectively.Citation18
The master regulators of QS in V. parahaemolyticus are LuxO and LuxU.Citation19 LuxU is a histidine-containing phosphotransfer (Hpt) protein that acts as both a phosphate donor and a phosphate receiver. LuxO is a response regulator (RR) that affects the expression of QS regulators. The signal transduction cascades involving LuxU and LuxO of QS circuits in V. parahaemolyticus have been summarized previously.Citation20 Briefly, at low cell densities, SKs work by transferring phosphates from their response regulator domains to LuxU. Phosphate from LuxU is transferred to the response regulator (RR), LuxO. LuxO-P induces transcription of small quorum regulatory RNAs (Qrrs). Aided by the RNA chaperone Hfq, Qrrs activate the translation of the AphA regulator and inhibits the translation of the OpaR regulator. At a high cell density, SKs become LuxO phosphatases, producing an inactive form of LuxO and Qrrs.Citation21–23 Thus, OpaR, but not AphA, is highly translated. Together these two transcriptional regulators control hundreds of target genes.Citation14,Citation16,Citation17,Citation24 The number of SKs that converge on LuxU usually varies.Citation20, Citation25–31 V. parahaemolyticus employs three SKs, LuxN, LuxQ, and CqsS, which act through LuxU to regulate the phosphorylation/dephosphorylation of LuxO.Citation32
ArcB/ArcA, a two-component signal transduction system involved in the metabolic shift from anaerobic to aerobic conditions, has been the subject of extensive research using an Escherichia coli model.Citation33 A redox signal associated with the plasma membrane is thought to stimulate the autophosphorylation of sensor kinase ArcB at the expense of Adenosine triphosphate (ATP).Citation34 Phosphorylated ArcB catalyzes the transphosphorylation of the response regulator ArcA to control the response to anaerobic growth.Citation35–37 ArcB/ArcA regulates important cellular functions, such as surface adherence, stress response, metabolism, biofilm formation, bioluminescence and DNA replication.Citation38–43 In addition, the ArcAB two-component system is implicated in pathogenesis of Salmonella enterica serovar Typhimurium,Citation44 and V. cholerae.Citation45 ArcB not only transfers phosphate groups to its cognate regulatory factor ArcA, but also conducts cross signaling with other two-component systems through the carboxy-terminal Hpt domain.Citation46–50 At present, there are no reports about the function of ArcB in V. parahaemolyticus.
In this study, we examined QS in V. parahaemolyticus by probing the phosphorylation cascade of the upstream elements that regulate T3SS1. We found that among the three SKs, only LuxQ plays a role in regulating T3SS1 but independently of LuxU. Instead, a tripartite hybrid kinase ArcB functions downstream of LuxQ to control T3SS1 expression, which represents a novel cross-regulation and cascade regulation. This unique signal transduction pathway can propagate multiple signals and form complex regulatory networks.
Materials and methods
Bacterial strains, plasmids, and media
The bacterial strains and plasmids used in the present study are listed in Table S1. wild-type V. parahaemolyticus strain RIMD 2210633 (wild-type, WT) is a pandemic O3:K6 strain that was isolated from a patient with diarrhea in 1996.Citation4 All Vibrio strains were grown in heart infusion broth (HI; BD, USA) with 1% (w/v) NaCl or on thiosulfate citrate bile salts sucrose (TCBS; Huankai, China) agar plates at 37°C. Under aerobic growth conditions, bacteria were incubated in a 12-ml culture tube with a loose cap with shaking at 200 rpm (high O2). Under anaerobic growth conditions, bacteria were incubated in tightly closed 12-ml tubes with 3 -ml medium without shaking (low O2).Citation44
E. coli S17–1 λpir was used for conjugation in gene deletion experiments. E. coli BL21(DE3) was used as the host for the expression and purification of LuxQ, ArcB, and LuxO. The E. coli strains were cultured overnight in Luria-Bertani (LB; BD, USA) medium at 37°C. The suicide plasmid pDM4Citation51 was used to generate gene knockouts, and the expression vectors pMMB207Citation52 or pBBR1MCS–1Citation53 were used for complementation experiments. The pCold I vector was used for cold shock-induced expression and purification of LuxQ, ArcB and LuxO.Citation54 V. parahaemolyticus was cultured with ampicillin (100 μg ml−1) or chloramphenicol (5 μg ml−1) as necessary. Ampicillin (100 μg ml−1), chloramphenicol (25 μg ml−1), and kanamycin (50 μg ml−1) were added to the E. coli culture as required.
Construction of deletion mutants
To generate null mutants, target regions were deleted from the WT strain using the suicide plasmid pDM4 and homologous recombination following the methods of Milton et al.Citation51 Briefly, for ΔarcB construction, using chromosomal DNA of V. parahaemolyticus RIMD 2210633 as a template, a 526 bp DNA fragment immediately upstream of arcB and a 552 bp DNA fragment in the immediate downstream region of arcB were amplified by PCR using the primer pairs ArcB_A1_XbaI/ArcB_A1_R and ArcB_A2_F/ArcB_A2_XbaI, respectively. Using primer pairs for ArcB_A1_XbaI/ArcB_A2_XbaI, overlapping extension PCR was implemented to fuse the two DNA fragments together. The DNA fragment was ligated into the pDM4 suicide vector digested with XbaI using a ClonExpress II one-step cloning kit (Vazyme, China). The resulting plasmid was designated as pDM4-ΔarcB. Upon verification by sequencing, the recombinant vector (containing the deletion allele, sacB conferring sensitivity to sucrose, and a chloramphenicol resistance gene) was introduced into E. coli S17–1 and transferred into RIMD 2210633 via conjugation. V. parahaemolyticus cells with chromosomal integration were resistant to chloramphenicol. Candidate cells were then spread onto agar plates containing 10% sucrose. Mutant strains with the excision of the integrated plasmid through a second round of allelic exchange were identified by their resistance to 10% sucrose and sensitivity to chloramphenicol and further verified by PCR. One clone with a PCR-confirmed arcB deletion was designated ∆arcB. To construct arcBHpt, the primer pairs arcB_hpt_pDM4_XbaI_A1_F/arcB_hpt_pDM4_A1_R and arcB_hpt_pDM4_A2_F/arcB_hpt_pDM4_XbaI_R were used to amplify the Hpt domain of arcB with the start codon. The subsequent steps are the same as those described above.
The luxU deletion mutant was constructed in the same manner using the primers LuxU_A1_XbaI, LuxU_A1_R, LuxU_A2_F, and LuxU_A2_XbaI. One PCR-confirmed clone was isolated and designated ΔluxU. A double mutant strain was generated by conjugating the plasmid pDM4-ΔluxU from E. coli S17 to the ΔarcB strain, and one PCR-confirmed clone was isolated and designated ∆arcB∆luxU. The triple mutants were constructed using a similar procedure, except that the recombinant plasmid was transformed into a double mutant. The construction of other deletion mutants was performed in the same manner using the corresponding primers. The primers used in this study are listed in Table S2.
Construction of complemented strains
For complementation experiments, the promoter regions with the complete open reading frame (ORF) of each indicated target gene were inserted into pBBR1MCS–1.Citation53 To construct ΔluxQ:BBR-luxQ, the primers LuxQ_BBR_A1_XbaI and LuxQ_BBR_A1_R were used to amplify the sequence containing the luxQ native promoter. Primers LuxQ_BBR_A2_F and LuxQ_BBR_A2_XbaI were used to amplify the complete luxQ sequence. Subsequently, the primers LuxQ_BBR_A1_XbaI and LuxQ_BBR_A2_XbaI were used to connect the two fragments. After XbaI digestion, the pBBR1MCS–1 plasmid and native promoter-controlled luxQ sequence were cloned using the ClonExpress II one-step cloning kit. Plasmid pBBR1MCS-1_luxQ was transformed into E. coli S17 λpir, resulting in the strain S17:pMMB207_luxQ, and was then conjugated from E. coli S17 λpir into ΔluxQ, ΔluxQΔarcB and ΔluxQΔluxO strains, resulting in ΔluxQ:pBBR1MCS1-luxQ, ΔluxQΔarcB:pBBR1MCS1-luxQ and ΔluxQΔluxO:pBBR1MCS1-luxQ. Site-directed mutagenesis of luxQ was performed using the Mut Express II Fast Mutagenesis Kit V2 (Vazyme, China) in the pBBR1MCS-1_luxQ vector. Transformation and conjugation were performed as previously described. Wild-type or mutant arcB and luxO complement strains were constructed in the same manner.
For western blot analysis, ORFs were amplified using gene-specific primers and a Flag tag was added at the C-terminus. The PCR product was ligated into BamHI-digested pMMB207.Citation52 The resulting plasmid was transformed into E. coli S17 λpir and then conjugated into the corresponding mutant strains. The complemented strain was selected on agar plates containing carbenicillin and chloramphenicol and verified by PCR analysis. The primers used in this study are listed in Table S2.
LDH release assay
HeLa cells (ATCC CCL-2) were grown in high-glucose Dulbecco’s modified Eagle’s medium (DMEM; Gibco, USA) supplemented with 10% fetal bovine serum (FBS; Gibco, USA) at 37°C with 5% CO2. To quantify cytotoxic effects, confluent HeLa cell monolayers in a 96-well plate were washed three times with warm PBS and then infected with a multiplicity of infection (MOI) of 12.5 for 2 h. We reduced the MOI to 5 to improve imaging of cytotoxicity during experiments lasting 7-hours. Supernatants from the infected cultures were removed after centrifuged for 5 min. Each supernatant was then analyzed using the LDH cytotoxicity detection kitPLUS (Roche, Germany), and the absorbance of each sample was measured at 490 nm using a Tecan Spark microplate reader. As described in the kit instructions, the percent cytotoxicity was calculated for infected cultures after subtraction of the background LDH release values from uninfected HeLa cells. Triton X-100 was used to determine the maximal LDH release. The vcrD1 gene encodes a T3SS1 inner membrane protein.Citation55 As such, ΔvcrD1 is a T3SS1-deficient strain. Uninfected cells were used as negative controls.
Fluorescence microscopy
HeLa cells plated on glass coverslips were fixed at the indicated time points in 4% paraformaldehyde in 1× PBS for 10 min at room temperature and then permeabilized in cold acetone (≤-20°C) for 5 min. Rhodamine phalloidin (200-μL; TRITC, 100 nM) was added to label actin filaments of the cytoskeleton, and 4′,6-diamidino-2-phenylindole (DAPI) was used to stain the nucleus following the manufacturer’s recommendations (Solarbio Technology Ltd, China). Slides were sealed with ProLong™ Glass Antifade Mountant (Invitrogen, USA) and examined under a fluorescence microscope (Carl Zeiss, Germany).
Construction of recombinant protein-expression plasmids
To construct plasmids for overproduction of LuxQ, V. parahaemolyticus RIMD 2210633 genomic DNA was used as a template, and the luxQ sequence was PCR-amplified (amino acid residues 1–34, which constitute the transmembrane segments, were omitted) with primers Cold-LuxQ-F-HA-SacI and Cold-LuxQ-R-EcoRI with an additional HA-tag added at the N-terminus. The arcB sequence (amino acid residues 1 to 77, which constitute the transmembrane segment, were omitted) with an additional Flag-tag on the N-terminus and the luxO sequence with an additional Myc-tag on the N-terminus were amplified with primer pairs Cold-ArcB-F-Flag-SacI/Cold-ArcB-R-EcoRI and Cold-LuxO-F-Myc-SacI/Cold-LuxO-R-EcoRI, respectively. The DNA fragments were ligated into the pCold vector digested with SacI and EcoRI using a Vazyme cloning kit to generate protein expression plasmids. Plasmids were transformed into E. coli strain DH5α for storage and into E. coli BL21 (DE3) strain for protein expression and purification.
Protein expression and purification
E. coli BL21(DE3) strains harboring pCold plasmids containing the recombinant protein were grown in a shaker at 37°C in LB broth supplemented with ampicillin (100 μg ml−1). When the optical density of the bacterial culture reached 0.6 at 600 nm, the cultures were quickly cooled to 20°C in ice water and left at this temperature for 30 min. Isopropyl-β-D-thiogalactoside (IPTG) was then added at a final concentration of 1.0 mM followed by incubation (with shaking) at 20°C for 24 h. Aliquots (5–10 ml) of the induced cultures were collected by centrifugation (5,000 xg) for 20 min. Cells were resuspended in 5-mL BugBuster Master Mix (Millipore, USA) per gram of wet mass and incubated for 15 min at room temperature on a shaking platform at a slow speed. Following lysis, the cell lysates were cleared by centrifugation (16,000 xg) for 20 min at 4°C. The supernatant was incubated with 2-ml Ni-NTA Agarose (Qiagen, DE, USA) for 12 h at 4°C with gentle agitation. The mixture containing the recombinant protein was loaded onto a column and purified by Ni-NTA affinity chromatography. The recovery and purity of the proteins in each fraction were checked using SDS-PAGE and pooled. Purified protein samples were concentrated and exchanged into final storage buffers (50 mM Tris-HCI [pH 7.6], 0.1 mM EDTA, 100 mM KCI, 10 mM MgCl2, and 30% glycerol) using Amicon ultracentrifugal filters (Millipore, USA). Protein concentration was estimated using a protein assay kit (Bio-Rad, USA), and purity was verified by SDS-PAGE.
SDS-PAGE and western blots
Proteins of different strains were isolated using the BugBuster Master Mix according to the manufacturer’s protocol. For western blotting, protein samples in loading buffer were denatured at 100°C for 10 min and separated using SDS-PAGE. Protein bands were transferred to polyvinylidene difluoride (PVDF) membranes (Millipore, Billerica, MA, USA) and blocked overnight in 5% nonfat milk. Anti-Flag, anti-HA, or anti-Myc antibodies (Proteintech, USA) were used as the primary antibodies in a 1:2,000 dilution with 0.5% nonfat milk in 1× Tris-buffered saline (TBS). The secondary antibody (1:5,000 dilution) was goat anti-mouse IgG (H1L)-horseradish peroxidase conjugate (Proteintech, USA). Three washes were performed in 1× TBS-Tween 20 for 10 min each, and the blots were visualized using SuperSignal™ West Pico PLUS Chemiluminescent Substrate (Thermo Fisher Scientific, USA) and photographed using FluorChem® E (Cell Biosciences, USA). To quantify the extent of phosphorylation of LuxU, blot images were analyzed with ImageJ. Intensities of both phosphorylated and unphosphorylated LuxU bands were used to calculate the fraction of LuxU∼P.
Phosphorylation assays
In vivo phosphorylation was confirmed using a Phos-tag assay. The strains were streaked onto TCBS agar plates containing chloramphenicol. Single colonies were cultured in HI broth to an OD600 of 0.5. To determine the phosphorylation of LuxO, single colonies were cultured to an OD600 of 0.2 ~ 0.3. Protein expression was induced by culturing with IPTG (0.5 mM) for 3 h. Proteins were isolated using BugBuster Master Mix according to the manufacturer’s protocol and resolved on 8% SDS-PAGE with 25 mM Phos-tag acrylamide (Wako, JPN). Proteins in the Phos-tagged gels were transferred onto PVDF membranes, and the protein mobility shift was determined by western blotting using an anti-FLAG antibody.
All in vitro biochemical assays were performed at room temperature. For autophosphorylation reactions, purified LuxQ and ArcB were diluted to 1 μM with kinase buffer (50 mM Tris-HCl [pH 7.6], 100 mM KCl, and 10 mM MgCl2), and the phosphorylation reaction was initiated by adding ATP at a final concentration of 10 mM.Citation56 A 15-μl sample was removed after 10 min and mixed with a 5×SDS-PAGE loading buffer (NCM, China) to stop the reaction. Proteins were resolved by using 8% SDS-PAGE with 25 mM Phos-tag acrylamide and further validated by western blotting.
For the phosphotransfer assays, the phosphorylated forms of LuxQ and ArcB were prepared as described above. Before the phosphotransfer experiments, excess ATP was removed by desalting and the concentration of phosphorylated protein was determined. Next, two-fold excess ArcB and LuxO were added to the LuxQ~P sample or two-fold excess LuxO was added to ArcB~P to initiate the phosphorylation transfer reaction, and 15-μl samples were taken after 60 s and analyzed as described above.
Bacterial two-hybrid assays
Proteins of interest were fused to the T18 and T25 split domains of the Bordetella adenylate cyclase as described previously.Citation57 The two plasmids encoding the fusion proteins were cotransformed into the reporter strain BTH101 (Table S1). pKT25-zip and pUT18C-zip were used as the positive controls. Three independent colonies for each transformation were inoculated into 1-mL LB medium. After incubation at 30°C for 12 h, 10-μL of each culture was spotted onto LB plates supplemented with ampicillin, kanamycin, IPTG, and X-Gal and incubated for 12 h at 30°C.
β-galactosidase assays
BTH101 cultures were grown overnight at 30°C in Luria-Bertani (LB) broth supplemented with ampicillin, kanamycin, and IPTG. The overnight cultures were diluted to OD600 = 0.05 in the same medium and further incubated until the cell density reached 0.5. Cell pellets from 200-μl cultures were resuspended in 1-ml Z buffer (60 mM Na2HPO4, 40 mM NaH2PO4, 10 mM KCl, 1 mM MgSO4, pH 7.0) and 25-μl chloroform and 25-μl 0.1% SDS were added, followed by vigorous mixing to lyse the bacteria. To start the reaction, 200-μl of o-nitrophenyl-β-D-galactoside (4 mg ml−1) was added. When the suspension started to turn yellow, the reaction was stopped by the addition of 0.5-ml 1 M Na2CO3. The mixture was centrifuged, and absorbance data (OD420) were collected from the supernatant.
Quantitative real-time PCR (qRT-PCR)
V. parahaemolyticus strains were cultured aerobically in HI supplemented with ampicillin or chloramphenicol for 5 h. Culture (1.5-ml) was centrifuged (10,000 × g for 2 min) and the pellet was resuspended in 15-ml DMEM supplemented with 1% FBS. After 3 h of aerobic incubation, total RNA was extracted using TRIzol (Invitrogen, USA), treated with DNase I (Thermo Fisher Scientific, USA), and transcribed into cDNA using a cDNA Synthesis Kit (Bio-Rad, USA). Real-time PCR was performed using a LightCycler 96 real-time quantitative PCR system (Roche, Germany) with SYBR Green qPCR Master Mix (Takara, JPN). The average Ct values were normalized using rpoA as the reference gene, and relative fold changes in gene expression were calculated according to the 2−ΔΔCT method.Citation58
Statistical analysis
Data were presented as mean ± standard deviation. Multiple t test was used for comparison between two groups. ANOVA was used for comparisons among multiple groups. P-values <0.0005 were considered statistically significant. Analyses were conducted using GraphPad Prism Software (version 8.3.0).
Results
ArcB functions in the same pathway with LuxQ and LuxO to regulate V. parahaemolyticus cytotoxicity
Our previous research found that only LuxQ regulates the biofilm within the QS circuit (LuxQ, LuxN, and CqsS) of V. parahaemolyticus.Citation59 Herein we explored whether these SKs are involved in the regulation of T3SS1. Deletion mutants were co-cultured with HeLa cells and after two hours of infection the WT, ΔcqsS, and ΔluxN strains exhibited a cytotoxic phenotype as evidenced by cytoskeletal changes and nuclear condensation (). In contrast, HeLa cells infected with ΔluxQ maintained a normal cell appearance, similar to cells infected with a ΔvcrD1 strain that is defective for T3SS1 activity (). The lactate dehydrogenase (LDH) release assays further supported these morphological observations for which the LDH level of the ΔluxQ strain was significantly lower than that of the WT strain (). In contrast, deletion of luxN and cqsS did not affect cytotoxicity induced by V. parahaemolyticus (). These data are consistent with LuxQ, but not LuxN and CqsS, contributing to the regulation of V. parahaemolyticus-induced cytotoxicity.
Figure 1. ArcB functions in the same pathway with LuxQ and LuxO to regulate V. parahaemolyticus cytotoxicity. (a) schematic diagram of V. parahaemolyticus LuxQ, LuxN, CqsS, LuxO and ArcB. LuxQ includes a predicted transmembrane (TM) (12–23), a periplasm (40–277), a His kinase a (HisKA) (481–546), a histidine kinase-like ATPases (HATPase_c) (593–710), and a receiver (REC) (734–846) domain. LuxN includes eight predicted domains including a TM (15–273), a HisKA (461–530), a HATPase_c (574–683), and a REC (720–830). LuxN includes eight predicted domains including TM (15–273), a HisKA (461–530), a HATPase_c (574–683), and a REC (720–830). CqsS includes six predicted domains including a TM (13–168), a HisKA (180–260), a HATPase_c (306–414), and a REC (564–678) domains. LuxU includes a single histidine phosphotransfer (Hpt) (673–779) domain. LuxO includes REC (1–108), and AAA – ATPases (153–296) domains. ArcB includes two predicted TMs (20–77), a per-arnt-sim (PAS) (155–221), a Motif C-terminal to PAS motifs (PAC) (227–269), a HisKA (282–347), a HATPase_c (394–510), a REC (527–638) and a Hpt (673–779) domain. (b) HeLa cells infected with different strains under aerobic conditions before infection. Uninfected cells were used as a negative control. Cells were visualized under a fluorescence microscope using rhodamine phalloidin to stain actin (red) and DAPI to stain nuclei (blue). Scale bar is 20 μm. The data are representative of three independent experiments. (c, d) LDH assay results (% cytotoxicity) for HeLa cells infected with different strains, including WT strain, indicated mutant strains, and complementation strains. The pBBR1MCS–1 plasmid was used for all complemented strains. All strains were incubated under aerobic conditions before infection. The data are the means ± SD from a representative experiment repeated in triplicate. Statistical significance was calculated using one-way ANOVA analysis. *** indicate significance compared to WT (P < .0005); n.S. indicate not statistically significant for complementation strains compared to double-deletion strains.
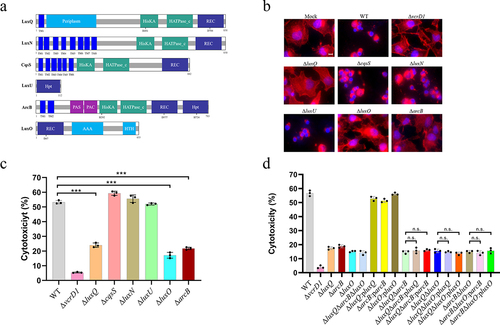
LuxU and LuxO are recognized regulatory factors from the QS pathways in Vibrio sp.Citation21 Morphological observation and LDH release assays showed that deletion of luxO resulted in a significant reduction in cell rounding and LDH release from infected HeLa cells (), although this reduction was not as severe as the ΔvcrD1 strain, which may be due to T3SS1 being regulated by other pathways besides LuxO, such as H-NS and VbrR. Complementation with luxO (ΔluxQ:pBBRMCS1-luxQ) restored the ability of the ΔluxO strain to efficiently induce cytotoxicity ( and S1). Interestingly, LuxU has an Hpt domain that shuttles phosphoryl groups between SK LuxQ and RR LuxO (), but the deletion of luxU did not influence the cytotoxicity of HeLa cells ().
Figure 2. Phosphorylation is essential for the LuxQ-ArcB-LuxO pathway to regulate V. parahaemolyticus cytotoxicity. (a) HeLa cells were infected with different strains from V. parahaemolyticus. The pBBR1MCS–1 plasmid was used for all complemented strains. All strains were incubated under aerobic conditions before infection. At the indicated time points, the culture supernatants were measured for the release of LDH, followed by the calculation of cytotoxicity as a percentage of total cellular lysis. The data are the means ± SD from a representative experiment repeated in triplicate. Statistical significance was calculated using one-way ANOVA analysis. n.s. indicate not statistically significant for complementation strains compared to mutant strains. (b, c) in vivo phosphorylation assays. The pMMB207 plasmid was used for the construction of all western blot analysis strains, and a Flag tag was added at the C-terminus. All V. parahaemolyticus strains were incubated under aerobic conditions. Western blot analysis of V. parahaemolyticus cell lysates using Phos-tag™ to detection phosphorylation (left panels; LuxQ~P, ArcB~P and LuxO~P) and using conventional SDS-PAGE (right panels). The data are representative of three independent experiments.
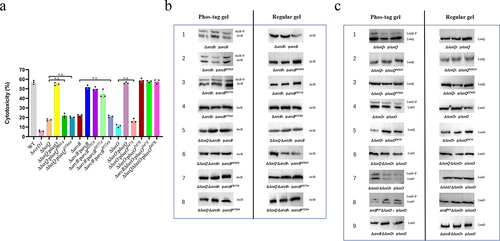
Because LuxU is an important component of the QS circuit,Citation60 we hypothesized that another Hpt protein may link LuxQ and LuxO in V. parahaemolyticus. To test this hypothesis, we identified eight proteins containing Hpt domains (TorS, VPA1100, BarA, VPA0736, ArcB, VP1472, VP2127, and VPA0744) by analyzing the genome sequence of the WT strain and generating corresponding gene deletion mutants. Cytotoxicity assays for the arcB-deleted strain showed a significant decrease in cell rounding and LDH release compared to the WT and other deletion strains ( and S2).
To determine whether ArcB functions in the same signaling pathway as LuxQ and LuxO to regulate V. parahaemolyticus cytotoxicity, three double-deletion strains (ΔluxQΔarcB, ΔarcBΔluxO, and ΔluxQΔluxO) and a triple-deletion strain (ΔluxQΔarcBΔluxO) were constructed and evaluated for their cytotoxicity against HeLa cells. As expected, LDH assays showed that the double- and triple-deletion mutants were attenuated and similar to the single deletion strains (). Importantly, complementation of luxQ, arcB or luxO alone in double-deletion strains did not restore cytotoxicity (). These results suggest that SK LuxQ, Hpt ArcB, and RR LuxO likely function together in the same signaling pathway to regulate the cytotoxicity of V. parahaemolyticus.
Phosphorylation is essential for the LuxQ-ArcB-LuxO pathway to regulate V. parahaemolyticus cytotoxicity
ArcB belongs to the subfamily of tripartite hybrid kinases. In addition to the C-terminal transmitter domain (Hpt), it also possesses an orthodox transmitter domain and a central receiver domain (). To determine whether all domains of ArcB play a role in the regulation of cytotoxicity, we performed site-directed mutagenesis of conserved amino acid residues (histidine 292, aspartate 577, and histidine 724) in the three domains of ArcB, and assessed the effect of these residues by complementation with mutated ArcB. Complementation of ArcBH292A and ArcBD577A restored the cytotoxic phenotype of ΔarcB strain, but complementation with the ArcBH724A strain did not restore cytotoxicity ().
To independently confirm the phosphorylation of ArcB, we overexpressed C-terminal FLAG-tagged ArcB or point mutant ArcB in the ArcB deletion strain or in the double LuxQ and ArcB deletion strain using the pMMB207 vector. Phos-tag™ gels showed two bands for ArcB, ArcBH292A and ArcBD577A ( line 1–3) whereas only one band was visible for ArcBH724A when it was expressed in the ArcB deletion strain ( line 4). Furthermore, no phosphorylated band was detected regardless of which ArcB version was expressed in the ΔluxQΔarcB strain ( line 5–8). To further confirm phosphate transfer between LuxQ and ArcB, arcB was expressed under the control of the native promoter to avoid overexpression. This experiment confirmed that in the absence of LuxQ, phosphorylation of ArcB did not occur, even if wild-type arcB was expressed (Fig. S3). As a negative control, only one band was observed using a conventional polyacrylamide gel for all strains. These data indicate that ArcB phosphorylation is dependent on the phosphate groups received from LuxQ under the test conditions used in this study, and that this event is important for regulatory functions in V. parahaemolyticus cytotoxicity.
The transmission of phosphate groups requires the joint participation of several conserved domains in SK and RR,Citation61 so we tested whether the conserved amino acid residues of phosphate group transfer in LuxQ and LuxO are involved in their regulatory activity. We found that mutations of histidine 491 within the histidine-containing phosphoacceptor (HisKA) domain, aspartic acid 784 within the receiver (REC) domain of LuxQ, and aspartic acid 47 within the REC domain of LuxO caused the loss of their complementation phenotype, indicating that these amino acids are essential for regulatory function (). Similarly, Phos-tag gels showed that LuxQ and LuxO were no longer phosphorylated when these residues were mutated ( line 2, 3 and 5). Furthermore, LuxO was not phosphorylated in the absence of LuxQ or ArcB ( line 6 and 9), whereas LuxO was still phosphorylated in the absence of LuxU ( line 7). We also constructed a complemented mutant strain with a mutation of aspartic acid 47 to glutamic acid within luxO (ΔluxO:pluxOD47E), which mimics the phosphorylated state and locks luxO in a constitutively active state.Citation62 The ΔluxO:pluxOD47E was as cytotoxic as the WT strain, and this cytotoxic effect was independent of the presence of ArcB or LuxQ (). Simultaneously, we constructed a deletion mutant that only retains the Hpt domain (containing the start codon) by deleting other domains in arcB. Using this strain with complemented luxO knockout (arcBHptΔluxO:pluxO) showed that LuxO was still phosphorylated ( line 8), consistent with LuxO being the downstream response regulator of LuxQ and ArcB. Collectively, it is evident that phosphorylation occurs at the conserved sites of LuxQ, ArcB, and LuxO, and is required to regulate V. parahaemolyticus cytotoxicity.
Phosphotransfer cascade within the LuxQ-ArcB-LuxO pathway in vitro
To further investigate the phosphorelay between LuxQ, ArcB, and LuxO, we expressed and purified recombinant LuxQ, ArcB, and LuxO proteins with different tags in their N-termini using an E. coli expression system and conducted autophosphorylation and phosphotransfer assays in vitro. Phos-tag gel results showed that an extra band was present above the LuxQ band when ATP was added (, upper panel), and western blotting further confirmed that the up-shifted band was LuxQ (anti-HA antibody) (, lower panel), indicating that LuxQ can be autophosphorylated in the presence of ATP. Phosphorelay assays were performed from phosphorylated LuxQ to ArcB and LuxO (). Phosphorylated LuxQ was obtained from the autophosphorylation assay and excess ATP was completely removed. As shown in , in the presence of phosphorylated LuxQ there were shifted bands above the ArcB and LuxO bands after phos-tag gel separation (upper panel), and band specificity was confirmed by western blotting using antibodies against their N-terminus tags (lower panel). Similarly, autophosphorylation of ArcB () and transfer of the phosphoryl group from ArcB to LuxO () occurred as expected. Together, these in vitro data demonstrated that phosphorelay occurs in the LuxQ-ArcB-LuxO pathway, and we speculate that ArcB functions as an Hpt protein by acquiring a phosphoryl group from LuxQ and phosphorylating the non-cognate response regulator LuxO.
Figure 3. Phosphotransfer cascade within the LuxQ-ArcB-LuxO pathway in vitro. (a, b) Phos-tag SDS-PAGE of the LuxQ and ArcB autophosphorylation reactions. LuxQ or ArcB protein samples after autophosphorylation reactions were loaded onto 8% SDS-PAGE with 25 mM Phos-tag acrylamide (upper panel) and immunoblotting with the anti-HA (indicating LuxQ) and the anti-Flag (indicating ArcB) antibody (lower panels). The data are representative of three independent experiments. (c, d) Phos-tag SDS-PAGE of the LuxQ/ArcB/LuxO and ArcB/LuxO phosphotransfer reactions. Purified ArcB and LuxO are added to LuxQ-P or ArcB-P to initiate phosphorylation transfer reactions. The reacted samples were loaded onto 8% SDS-PAGE with 25 mM Phos-tag acrylamide (upper panel) and immunoblotting with the anti-HA (indicating LuxQ), the anti-Flag (indicating ArcB) and the anti-Myc (indicating LuxO) antibody (lower panels). The data are representative of three independent experiments.
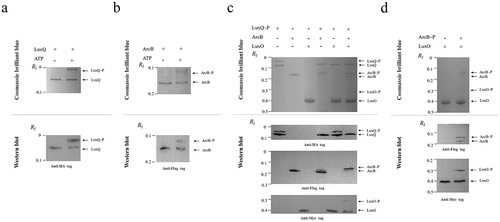
The Hpt domain of ArcB is required for its interaction with LuxQ
Based on these findings, we surmised that LuxQ, ArcB, and LuxO act in the same signaling pathway, and ArcB serves as a mediator between SK LuxQ and RR LuxO. To further investigate the presumptive ArcB–LuxQ and ArcB–LuxO interactions, a bacterial two-hybrid system confirmed a probable interaction between ArcB and LuxQ, but there was no evidence for an interaction between ArcB and LuxO (). This result was verified by subsequent assays for β-galactosidase activity (), confirming an interaction between ArcB and LuxQ.
Figure 4. The Hpt domain of ArcB is required for its interaction with LuxQ. (a) plasmid vectors carrying the indicated T18 and T25 constructs were transformed to BTH101. Individual colonies were grown in LB at 30°C for 12 h under aerobic growth conditions and then patched on LB medium supplemented with amp, Kan, X-Gal, and 0.5 mM IPTG. Plates were incubated at room temperature for at least 12 h. T25-zip & T18-zip were used as positive controls, and T25 & T18 empty vectors were used as negative controls. The data are representative of three independent experiments. (b) determination of β-galactosidase activity. Bacteria were cultured to a cell density of 0.5 (OD600) under aerobic conditions, and aliquots from cultures were resuspended with Z buffer. Cultures were lysed with SDS and chloroform. The reaction was stopped by the addition of Na2CO3 before OD420 measurements were taken. The Miller unit was defined as β-galactosidase activity unit of the culture. T25-zip & T18-zip were used as positive controls, and T25 & T18 empty vectors were used as negative controls. The data are the means ± SD from a representative experiment repeated in triplicate. Statistical significance was calculated using one-way ANOVA analysis. *** indicate significance compared to negative controls (P < .0005). (c) LDH assay results (% cytotoxicity) for HeLa cells infected with different strains, including WT strain, indicated mutant strains, and complementation strains. The pBBR1MCS–1 plasmid was used for all complemented strains. All strains were incubated under aerobic conditions before infection. The data are the means ± SD from a representative experiment repeated in triplicate. Statistical significance was calculated using one-way ANOVA analysis. *** indicate significance compared to ΔarcB (P < .0005); n.s. indicate not statistically significant for complementation strains compared to ΔarcB.
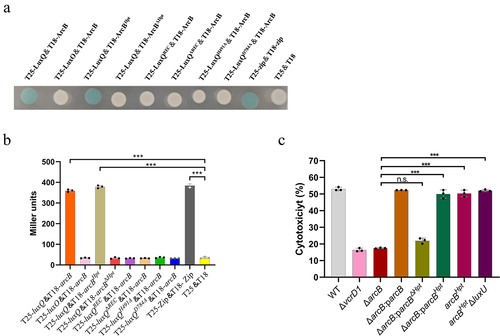
Because LuxQ-induced cytotoxicity required only histidine H724 in ArcB, but not histidine 292 or aspartic acid 577, we cloned this domain and assessed its complementation. The Hpt protein alone (ΔarcB:207-arcBHpt) permitted LuxQ-induced cytotoxicity in the ΔarcB strain. In contrast, the N-terminus of ArcB (ΔarcB:207-arcBΔHpt) did not complement ArcB deletion. Moreover, in mutant strains that retained only the Hpt domain of ArcB (arcBHpt), cytotoxicity was not affected, ().
Previous data suggested that LuxQ, a hybrid SK with two predicted phosphorylation sites, does not contain an Hpt domain (). Thus, we hypothesized that LuxQ donates phosphoryl groups to the Hpt domain of ArcB, which then passes the phosphoryl groups downstream to RR, LuxO. To test this hypothesis, we generated a bacterial two-hybrid system expressing LuxQ and either the N-terminal domain of ArcB (lacking its Hpt domain) or the C-terminal Hpt domain of ArcB. Our data showed that LuxQ clearly interacts with the Hpt domain of ArcB, but not with ArcB that lacks the Hpt domain (). Notably, we found that this interaction between LuxQ and ArcB may require the intact LuxQ protein because the interaction disappears when LuxQ only contains the REC domain or when the key amino acid sites undergo mutations (). Overall, these data indicate that the Hpt domain of ArcB is necessary for the transfer of phosphate groups from LuxQ to LuxO.
LuxU is still phosphorylated but not involved into regulation of V. parahaemolyticus cytotoxicity
Although our data revealed that ArcB, but not LuxU, is connected to SK LuxQ and RR LuxO to regulate Vibrio parahaemolyticus cytotoxicity, we cannot rule out the possibility of partial redundancy of LuxU with ArcB. To assess this possibility, we generated a double-deletion strain of luxU and arcB (∆arcB∆luxU) and two complemented strains using the single genes arcB or luxU (∆arcB∆luxU:parcB and ∆arcB∆luxU:pluxU). To observe the changes in cytotoxicity throughout the entire infection more clearly (7 h assays), we have reduced the multiplicity of infection for these experiments. The ∆arcB∆luxU strain exhibited cytotoxicity similar to that of the ∆arcB strain, which was lower than that of the WT and ∆luxU strains within 5 h of infection (). Complementation of luxU in ∆arcB∆luxU had no observable effect on cytotoxicity, while complementation of arcB restored cytotoxicity of the ∆arcB∆luxU strain (). These observations are consistent with LuxU not being involved in the regulation of V. parahaemolyticus cytotoxicity.
Figure 5. LuxU is still phosphorylated but not involved into regulation of V. parahaemolyticus cytotoxicity. (a) the trend of LDH (% cytotoxicity) in HeLa cells infected with different strains over time. At the indicated time points, the culture supernatants were measured for the release of LDH, followed by the calculation of cytotoxicity as a percentage of total cellular lysis. Including WT strain, indicated mutant strains, and complementation strain. The pBBR1MCS–1 plasmid was used for all complemented strains. All strains were incubated under aerobic conditions before infection. The data are the means ± SD from a representative experiment repeated in triplicate. Statistical significance was calculated using one-way ANOVA analysis. (b) in vivo phosphorylation detection of ArcB and LuxU. All V. parahaemolyticus strains were incubated under aerobic conditions. Western blot analysis of V. parahaemolyticus cell lysates using Phos-tag™ to detection phosphorylation (left panels; LuxU~P, ArcB~P) and using conventional SDS-PAGE (right panels). The protein amounts was calibrated by RNA-Pol β. The data are representative of three independent experiments.
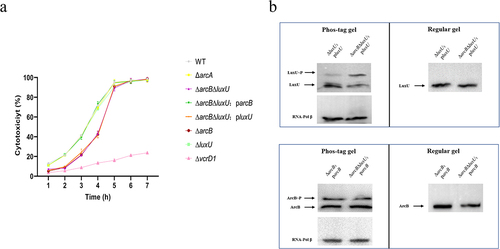
LuxU, a well-known Hpt protein, is an important component of Vibrio QS circuits and has been linked to many biological phenotypesCitation19. Although LuxU did not interact with LuxQ and LuxO to participate in the regulation of V. parahaemolyticus cytotoxicity, we also monitored the phosphorylation status of LuxU under conditions in which phosphorylation of ArcB was detected. Phos-tag gels revealed LuxU phosphorylation in the luxU complemented strain. Interestingly, the magnitude of phosphorylated LuxU increased by about two-fold in the absence of arcB (∆arcB∆luxU strain) when compared to the presence of arcB (∆luxU strain) (, upper panel). After excluding other sensor kinases (LuxN and CqsS) that can potentially phosphorylate LuxU, we monitored the phosphorylation status of LuxU again and obtained similar results. When all SK in the QS pathway are knocked out, LuxU is no longer phosphorylated (Fig. S4). In contrast, the presence or absence of luxU did not affect the phosphorylation level of ArcB (, lower panel). These data suggest that ArcB outcompetes LuxU for the phosphate groups delivered by LuxQ.
Loss of ArcA, the cognate response regulator of ArcB, does not affect cytotoxicity
Earlier work with E. coli characterized ArcB as a sensory kinase that controls the anaerobic repression of several operons by phosphorylating its response regulator ArcA.Citation36,Citation63 However, our findings show that ArcB is involved in the regulation of V. parahaemolyticus cytotoxicity, but it remains to be determined if ArcB plays a canonical role in this process. After showing that deletion of arcA did not affect cytotoxicity (), we assessed how ArcB/ArcA responded under different growth conditions. Under anaerobic growth conditions, both ArcB and ArcA underwent phosphorylation regardless of the presence of LuxQ (); however, ArcA was not phosphorylated when ArcB was deleted (). In contrast, under aerobic growth conditions, ArcB was phosphorylated in the presence of LuxQ, and no phosphorylation bands were observed in the luxQ deletion background (). Under aerobic conditions there was no evidence of phosphorylation of ArcA regardless of the presence of ArcB or LuxQ (). We also found that under aerobic conditions the phosphorylation level of ArcB was dependent on cell density, which is consistent with the cell density-dependent regulation of QS by LuxQ (). Furthermore, ArcA does not affect the expression of T3SS1 gene (regulator gene exsA and structural gene vcrD1) under both high and low oxygen conditions (Fig. S5). These results indicate that under anaerobic-like conditions, V. parahaemolyticus uses an ArcB/ArcA TCS whereby ArcB is autophosphorylated before it transphosphorylates ArcA. In contrast, under aerobic conditions V. parahaemolyticus uses the LuxQ-ArcB-LuxO pathway in which the Hpt domain of ArcB receives phosphate groups from LuxQ and transmits them to LuxO, but further transfer to ArcA does not occur.
Figure 6. ArcA, the response regulatory factor of ArcB, is not linked with the regulation of cytotoxicity. (a) in vivo phosphorylation detection of ArcB phosphorylation levels under high and low O2 conditions. Western blot analysis of V. parahaemolyticus cell lysates using Phos-tag™ to detection phosphorylation (left panels; ArcB~P) and using conventional SDS-PAGE (right panels). The data are representative of three independent experiments. (b) in vivo phosphorylation detection of ArcA phosphorylation levels under high and low O2 conditions. Western blot analysis of V. parahaemolyticus cell lysates using Phos-tag™ to detection phosphorylation (left panels; ArcA~P) and using conventional SDS-PAGE (right panels). The data are representative of three independent experiments. (c) in vivo phosphorylation of ArcB at different growth stages under aerobic conditions. The phosphorylation of ArcB at different growth stages (OD600 of 0.05, 0.20, 0.40, 0.60, 0.80, 1.00) under aerobic conditions was detected by strain ΔarcB:parcB, and the protein amounts was calibrated by RNA-Pol β. The data are representative of three independent experiments.
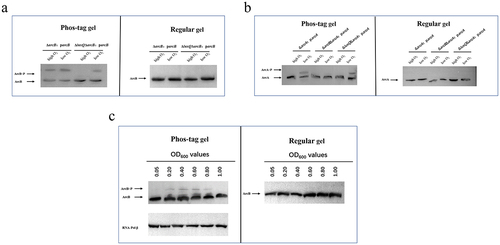
LuxQ-ArcB-LuxO pathway exerts cytotoxicity by regulating T3SS1 expression
T3SS1 is mainly responsible for V. parahaemolyticus cytotoxicity. To better understand how the LuxQ-ArcB-LuxO pathway regulates the cytotoxicity of V. parahaemolyticus under aerobic conditions, we selected several T3SS1 genes for transcriptional analysis, including those that encode the translocon proteins VopB1 and VopD1,Citation64 the T3SS1 regulators ExsA and ExsD,Citation11 the effector proteins VopS and VopQ,Citation65 and the structural proteins VscC1 and VcrD1.Citation55 Transcription levels for these genes were significantly downregulated in the deletion strains (ΔluxQ, ΔluxO, ΔarcB, ΔarcBΔluxQ, ΔluxQΔluxO, and ΔarcBΔluxO) compared to those in the WT strain and the complemented strains (ΔluxQ:BBR-luxQ, ΔluxO:BBR-luxO, and ΔarcB:BBR-arcB) ( and S6). Mutation of the conserved amino acid residues of phosphate group transfer in LuxQ, LuxO, and ArcB (ΔluxQ:BBR-luxQH491A, ΔluxQ:BBR-luxQD784A, ΔluxO:BBR-luxOD47A, and ΔarcB:BBR-arcBH724A) resulted in significantly reduced transcription. Mutation of the first conserved histidine (H292A) or conserved aspartic acid (D577A) of the ΔarcB strain did not negatively affect complementation ( and S6). Analogous results were obtained when assessing whether these mutant proteins could complement the decreased cytotoxicity associated with arcB deletion (). The transcription level of T3SS1 genes in a constitutively active mutant (ΔluxO:BBR-luxOD47E) was consistent with the WT phenotype, and was not affected by deletion of luxQ or arcB. Furthermore, the absence of arcA and luxU did not affect the transcription of these genes ( and S6). Collectively, our findings characterize a novel pathway that controls cytotoxicity by regulating the expression of T3SS1 through the Hpt domain of ArcB.
Figure 7. LuxQ-ArcB-LuxO pathway exerts cytotoxicity by regulating T3SS1 expression. Expression levels (qRT-PCR) for T3SS1 genes exsA and vcrD1 for the indicated strains. The data were normalized to rpoA expression. The pBBR1MCS–1 plasmid was used for all the complemented strains. Before extracting total RNA, all strains were incubated under aerobic conditions. The data are the means ± SD from a representative experiment repeated in triplicate. Statistical significance was calculated using one-way ANOVA analysis. *** indicate significance compared to gene expression in WT (P < .0005).
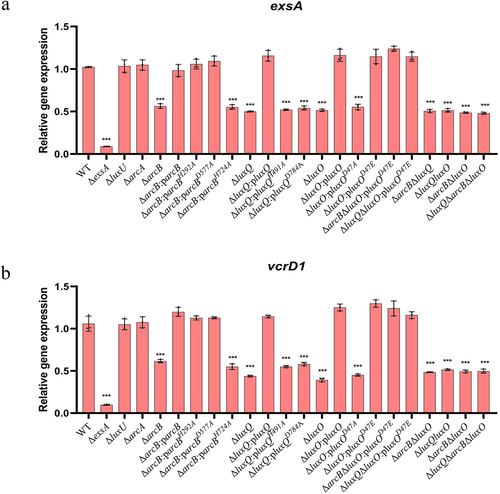
Discussion
Our results suggest that LuxQ and LuxO are the only QS components involved in the regulation of T3SS1. This previously undescribed QS-dependent response appears to require the participation of the V. parahaemolyticus SK ArcB. Our previous research reported that the LuxQ-LuxU-LuxO pathway regulates biofilm formation by V. parahaemolyticus at 24 h (Liu M et al. 2021), but in this study, LuxU did not affect cytotoxicity during in vitro infection (). Furthermore, the absence of luxU did not act synergistically with the loss of cytotoxicity attributed to expression of arcB. Therefore, we surmised that LuxQ cannot phosphorylate LuxU when LuxQ binds to ArcB, whereas when ArcB is not expressed or degraded, unbound LuxQ phosphorylates LuxU. Consistent with this hypothesis, subsequent in vivo phosphorylation assays demonstrated that LuxU was more likely to be phosphorylated in the absence of ArcB, whereas phosphorylation of ArcB was not affected by LuxU.
SKs use ATP to autophosphorylate a conserved histidine residue in response to an external stimulus. The phosphoryl group is then transferred from histidine to an aspartic acid residue of a separate RR protein, which initiates an appropriate cellular responseCitation61. Consistent with this, we found that a histidine residue (His 491) in the transmitter domain and an aspartate residue (Asp 784) in the REC domain of LuxQ were indispensable for cytotoxicity by V. parahaemolyticus. Similarly, we also showed the essential role of the aspartate residue (Asp 47) in the REC domain of LuxO in cytotoxicity (). More complex phosphate transfer mechanisms employ an Hpt domain as a mediator (or alternative transmitter) to transfer the phosphoryl group from the SK to the RR.Citation66 We found that as an unorthodox SK (Hpt domain of ArcB) is required for the regulation of T3SS1 genes expression under aerobic conditions. And under aerobic conditions, phosphorylation of ArcB requires the presence of LuxQ, while retaining only the Hpt domain of ArcB is sufficient to phosphorylate LuxO. Consequently, in the aerobic mediated cytotoxicity regulation pathway, it appears that LuxQ functionally replaces the enzymatic activity of ArcB and only the Hpt domain of ArcB influences the activity of the downstream RR LuxO. This more complex phosphate transfer likely provides opportunities to integrate multiple signals at the intermediate step of phosphotransfer.
Previous work demonstrated that ArcB-dependent phosphotransfer reactions are complex and that ArcB may participate in more than one signaling pathway,Citation67 consistent with the Hpt domain of ArcB acting not only as a phosphate donor for its cognate effector protein, ArcA, but also as a phosphate donor for chemotactic proteins, CheY,Citation50 and the osmoregulator, OmpR.Citation48 In Actinobacillus actinomycetemcomitans, ArcB may work together with LuxS to control the adaptation of iron-limiting conditions and its growth under such conditions.Citation68 This indicates that ArcB is at the intersection of multiple signaling pathways and may mediate a broad range of cellular responses to various environmental stimuli.
ArcAB is a two-component system that responds to the redox state of the cell by sensing an oxygen-limited signal for altered gene expression.Citation69 However, some groups suggest different modulatorsCitation37,Citation70–72, which underscores that this system may be active in multiple conditions, including aerobiosis. Our results suggest that the V. parahaemolyticus ArcAB plays a canonical role in responding to redox stress (), but under aerobic conditions, the Hpt domain of ArcB replaces LuxU as a phosphodonor for LuxO in the regulation of cytotoxicity of V. parahaemolyticus. Under this scenario, ArcA, the cognate response regulator of ArcB, did not play a role. This indicates that domains within the same signaling network could have discrete roles depending on environmental conditions surrounding the cell. The specificity of ArcB mediated phosphorylation flow for LuxO or ArcA may be due to the plasticity in regulatory patterns of TCSs.Citation73 TCS connect stimuli to specific responses by exploiting allostery and protein plasticity.Citation74 When bacteria sense specific external stimuli or signals, TCSs quickly remodel signal transduction pathways and corresponding function in a specific regulatory pathway. This capability helps bacteria to rapidly shift gene expression in an economical manner to better adapt to different conditions. Our evidence for the function of ArcB under aerobic conditions further proves this system not only serves as strictly an anaerobic global regulator, and justifies additional study of ArcB functionality in this context.
V. parahaemolyticus is influenced by several factors in the marine environment and in the human host. Low calcium levels induce T3SS1 gene expressionCitation12, which is regulated by QS regulatory cascade. In this study, we demonstrated ArcB, a known oxygen sensing protein, is involved in coordination between QS and T3SS1-induced cytotoxicity. This implies that the role of T3SS1 is weakened in the hypoxic environment of the intestine. In previous studies, it was demonstrated that TTSS1 was involved in the cytotoxicity of the RIMD 2210633 strain to HeLa cell.Citation55 Intraperitoneal injection of V. parahaemolyticus into mice has demonstrated that T3SS1 contribute to lethality during systemic infection.Citation7 On the other hand, T3SS2 has been demonstrated to be involved in enterotoxicity in the rabbit ileal loop test and T3SS2 is necessary to cause gastrointestinal clinical signs in newborn piglets.Citation6,Citation55 These results implicate T3SS1 in V. parahaemolyticus-induced septicemia in humans, rather than serving as the dominant contributor to the enterocolitis. Although the relationship between cytotoxicity and enteritis remains unclear, these two traits seem to act synergistically during conventional food-borne vibriosis. In the model of infant rabbits oro-gastrically inoculated with V. parahaemolyticus, T3SS2 is necessary for V. parahaemolyticus to colonize and cause disease in the small intestine, but T3SS1 also regulates the virulence of V. parahaemolyticus in the intestine,Citation75 which may be achieved by defending against attacks of some intestinal cells. Therefore, during human infection, T3SS2 may play a major role in causing the initial infection and diarrheal disease, while T3SS1 acts synergistically. Once V. parahaemolyticus disseminates out of the intestine and into deeper tissues, T3SS1 may be required for survival from immune clearance and contributes to subsequent sepsis and septic shock.
The level of T3SS1 expression is strongly linked to its cytotoxicity against host cells in V. parahaemolyticus.Citation10 In this study, we found that the deletion of LuxQ, ArcB and LuxO or the mutation of their key amino acid sites resulted in a significant decrease in the transcription of T3SS1 genes (), which is consistent with reduced cytotoxicity. How does this three-component signal transduction system exert regulatory effects on the expression of V. parahaemolyticus T3SS1 genes? Previous work demonstrated that the transcription of T3SS1 genes is directly regulated by the ExsACDE regulatory cascade in V. parahaemolyticus.Citation11 H-NS represses T3SS1-related gene expression by suppressing exsA gene expression.Citation12 In addition, VbrR, the RR that harbors DNA-binding domains in the two-component pair VbrK/VbrR, binds to the exsC promoter region to regulate T3SS1.Citation76 It has been shown that LuxO is phosphorylated at low cell density, and the phosphorylated LuxO activates the expression of genes encoding Qrrs. These Qrrs control the expression of two master QS regulators, AphA and OpaR.Citation17 AphA and OpaR are transcription factors that are abundantly expressed at low cell density and high cell density stages, respectively, and they modulate the expression of various genes.Citation77 Importantly, AphA activates the transcription of T3SS1 genes via direct activation of the transcription of exsBAD-vscBCD,Citation17,Citation78 while OpaR negatively regulates T3SS1 production by repressing the transcription of the aphA gene.Citation14 Thus, we surmise that this novel signal transduction system achieves the regulation of T3SS1 gene expression by coordinating the master QS regulators, AphA and OpaR, in V. parahaemolyticus.
In summary, this study describes the interaction between a hybrid SK ArcB in V. parahaemolyticus and a novel multicomponent signal transduction system involving two previously identified QS components (). We propose that under low cell density and aerobic conditions, SK LuxQ senses an environmental signal and autophosphorylates a conserved histidine within the HisKA domain using ATP as the phosphoryl donor. This phosphoryl group is then transferred to the second phosphorylation site in the covalently attached REC domain. Subsequently, the phosphate group is donated to the histidine in the C-terminal Hpt domain of ArcB, which in turn passes it to a conserved aspartate in the REC domain within RR LuxO. Phosphorylated LuxO then regulates T3SS1 expression by controlling the ExsACDE operon.
Figure 8. Models of the LuxQ/ArcB/LuxO three-component signal transduction system in Vibrio parahaemolyticus. The details of signal transduction for the new signal transduction system of V. parahaemolyticus have been described in the text. Gray font indicates the cessation of relevant regulatory cascades. The green arrows indicate phosphoryl group transfers under high oxygen conditions, while the blue arrow indicates the transfer of phosphate groups under low oxygen conditions. Solid single arrows indicate direct inducing effects and dotted single arrows indicate indirect inducing effects. Abbreviations: H, histidine; D, aspartate; HTH, helix-turn-helix; P, periplasm; IM, inner membrane; C, cytoplasm.
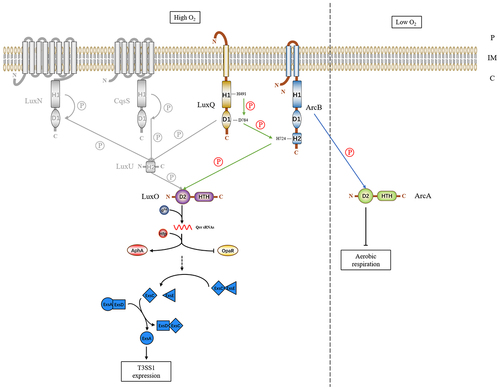
Author contributions
Zhe Zhao, Ming Liu, and Ce Zhang conceived and designed the research; Ce Zhang, Min Liu, Ming Liu, and Ying Wu performed the experiments; Xixi Li and Chen Zhang analyzed the data; Ce Zhang, Zhe Zhao, and Ming Liu wrote the paper; Douglas R. Call advised on the project and provided comments on the manuscript.
Supplemental Material
Download Zip (12.5 MB)Disclosure statement
No potential conflict of interest was reported by the author(s).
Data availability statement
The authors confirm that the data supporting the findings of this study are available in the article and its supplementary material.
Supplementary material
Supplemental data for this article can be accessed online at https://doi.org/10.1080/19490976.2023.2281016
Additional information
Funding
References
- Daniels NA, MacKinnon L, Bishop R, Altekruse S, Ray B, Hammond RM, Thompson S, Wilson S, Bean NH, Griffin PM, et al. Vibrio parahaemolyticus infections in the United States, 1973-1998. J Infect Dis. 2000;181(5):1661–20. doi:10.1086/315459.
- McLaughlin JB, DePaola A, Bopp CA, Martinek KA, Napolilli NP, Allison CG, Murray SL, Thompson EC, Bird MM, Middaugh JP. Outbreak of Vibrio parahaemolyticus gastroenteritis associated with Alaskan oysters. N Engl J Med. 2005;353(14):1463–1470. doi:10.1056/NEJMoa051594.
- Su YC, Liu C. Vibrio parahaemolyticus: a concern of seafood safety. Food Microbiol. 2007;24(6):549–558. doi:10.1016/j.fm.2007.01.005.
- Makino K, Oshima K, Kurokawa K, Yokoyama K, Uda T, Tagomori K, Iijima Y, Najima M, Nakano M, Yamashita A, et al. Genome sequence of Vibrio parahaemolyticus: a pathogenic mechanism distinct from that of V cholerae. Lancet. 2003;361(9359):743–749. English. doi:10.1016/S0140-6736(03)12659-1.
- de Souza Santos M, Orth K, Miller JF. Intracellular Vibrio parahaemolyticus escapes the vacuole and establishes a replicative niche in the cytosol of epithelial cells. mBio. 2014;5(5):e01506–01514. doi:10.1128/mBio.01506-14.
- Piñeyro P, Zhou X, Orfe LH, Friel PJ, Lahmers K, Call DR. Development of two animal models to study the function of Vibrio parahaemolyticus type III secretion systems. Infect Immun. 2010;78(11):4551–4559. doi:10.1128/IAI.00461-10.
- Hiyoshi H, Kodama T, Iida T, Honda T. Contribution of Vibrio parahaemolyticus virulence factors to cytotoxicity, enterotoxicity, and lethality in mice. Infect Immun. 2010;78(4):1772–1780. doi:10.1128/IAI.01051-09.
- Ono T, Park KS, Ueta M, Iida T, Honda T. Identification of proteins secreted via Vibrio parahaemolyticus type III secretion system 1. Infect Immun. 2006;74(2):1032–1042. doi:10.1128/IAI.74.2.1032-1042.2006.
- Hueck CJ. Type III protein secretion systems in bacterial pathogens of animals and plants. Microbiol Mol Biol Rev. 1998;62(2):379–433. doi:10.1128/MMBR.62.2.379-433.1998.
- Zhou X, Shah DH, Konkel ME, Call DR. Type III secretion system 1 genes in Vibrio parahaemolyticus are positively regulated by ExsA and negatively regulated by ExsD. Mol Microbiol. 2008;69(3):747–764. doi:10.1111/j.1365-2958.2008.06326.x.
- Zhou X, Konkel ME, Call DR. Regulation of type III secretion system 1 gene expression in Vibrio parahaemolyticus is dependent on interactions between ExsA, ExsC, and ExsD. Virulence. 2010;1(4):260–272. doi:10.4161/viru.1.4.12318.
- Kodama T, Yamazaki C, Park KS, Akeda Y, Iida T, Honda T. Transcription of Vibrio parahaemolyticus T3SS1 genes is regulated by a dual regulation system consisting of the ExsACDE regulatory cascade and H-NS. FEMS Microbiol Lett. 2010;311(1):10–17. doi:10.1111/j.1574-6968.2010.02066.x.
- Miller MB, Bassler BL. Quorum sensing in bacteria. Annu Rev Microbiol. 2001;55(1):165–199. doi:10.1146/annurev.micro.55.1.165.
- Zhang Y, Qiu Y, Tan Y, Guo Z, Yang R, Zhou D, Mukhopadhyay P. Transcriptional regulation of opaR, qrr2–4 and aphA by the Master quorum-sensing regulator OpaR in Vibrio parahaemolyticus. PloS One. 2012;7(4):e34622. doi:10.1371/journal.pone.0034622.
- Ng WL, Bassler BL. Bacterial quorum-sensing network architectures. Annu Rev Genet. 2009;43(1):197–222. doi:10.1146/annurev-genet-102108-134304.
- Gode-Potratz CJ, McCarter LL. Quorum sensing and silencing in Vibrio parahaemolyticus. J Bacteriol. 2011;193(16):4224–4237. doi:10.1128/JB.00432-11.
- Sun F, Zhang Y, Wang L, Yan X, Tan Y, Guo Z, Qiu J, Yang R, Xia P, Zhou D, et al. Molecular characterization of direct target genes and cis-acting consensus recognized by quorum-sensing regulator AphA in Vibrio parahaemolyticus. PloS One. 2012;7(9):e44210. doi:10.1371/journal.pone.0044210.
- Zhang Y, Hu L, Qiu Y, Osei-Adjei G, Tang H, Zhang Y, Zhang R, Sheng X, Xu S, Yang W, et al. QsvR integrates into quorum sensing circuit to control Vibrio parahaemolyticus virulence. Environ Microbiol. 2019;21(3):1054–1067. doi:10.1111/1462-2920.14524.
- Milton DL. Quorum sensing in vibrios: complexity for diversification. Int J Med Microbiol. 2006;296(2–3):61–71. doi:10.1016/j.ijmm.2006.01.044.
- Henke JM, Bassler BL. Three parallel quorum-sensing systems regulate gene expression in Vibrio harveyi. J Bacteriol. 2004c;186(20):6902–6914. English. doi: 10.1128/JB.186.20.6902-6914.2004.
- Henke JM, Bassler BL. Quorum Sensing Regulates Type III Secretion in Vibrio harveyi and Vibrio parahaemolyticus. J Bacteriol. 2004a;186(12):3794–3805. English. doi: 10.1128/JB.186.12.3794-3805.2004.
- Lenz DH, Mok KC, Lilley BN, Kulkarni RV, Wingreen NS, Bassler BL. The small RNA chaperone hfq and multiple small RNAs control quorum sensing in Vibrio harveyi and Vibrio cholerae. Cell. 2004;118(1):69–82. English. doi: 10.1016/j.cell.2004.06.009.
- Tu KC, Bassler BL. Multiple small RNAs act additively to integrate sensory information and control quorum sensing in Vibrio harveyi. Genes Dev. 2007;21(2):221–233. doi:10.1101/gad.1502407.
- van Kessel JC, Rutherford ST, Shao Y, Utria AF, Bassler BL. Individual and combined roles of the master regulators AphA and LuxR in control of the Vibrio harveyi quorum-sensing regulon. J Bacteriol. 2013;195(3):436–443. doi:10.1128/JB.01998-12.
- Chen X, Schauder S, Potier N, Van Dorsselaer A, Pelczer I, Bassler BL, Hughson FM. Structural identification of a bacterial quorum-sensing signal containing boron. Nature. 2002;415(6871):545–549. doi:10.1038/415545a.
- Croxatto A, Pride J, Hardman A, Williams P, Cámara M, Milton DL. A distinctive dual-channel quorum-sensing system operates in Vibrio anguillarum. Mol Microbiol. 2004;52(6):1677–1689. doi:10.1111/j.1365-2958.2004.04083.x.
- Gilson L, Kuo A, Dunlap PV. AinS and a new family of autoinducer synthesis proteins. J Bacteriol. 1995;177(23):6946–6951. doi:10.1128/jb.177.23.6946-6951.1995.
- Henke JM, Bassler BL. Three parallel quorum-sensing systems regulate gene expression in Vibrio harveyi. J Bacteriol. 2004b;186(20):6902–6914. doi:10.1128/JB.186.20.6902-6914.2004.
- Kawase T, Miyoshi S, Sultan Z, Shinoda S. Regulation system for protease production in Vibrio vulnificus. FEMS Microbiol Lett. 2004;240(1):55–59. doi:10.1016/j.femsle.2004.09.023.
- Miller MB, Skorupski K, Lenz DH, Taylor RK, Bassler BL. Parallel quorum sensing systems converge to regulate virulence in Vibrio cholerae. Cell. 2002;110(3):303–314. doi:10.1016/S0092-8674(02)00829-2.
- Ruby EG, Urbanowski M, Campbell J, Dunn A, Faini M, Gunsalus R, Lostroh P, Lupp C, McCann J, Millikan D, et al. Complete genome sequence of Vibrio fischeri: a symbiotic bacterium with pathogenic congeners. Proc Natl Acad Sci USA. 2005;102(8):3004–3009. doi:10.1073/pnas.0409900102.
- Liu AC, Thomas NA. Transcriptional profiling of Vibrio parahaemolyticus exsA reveals a complex activation network for type III secretion. Front Microbiol. 2015;6:1089. doi:10.3389/fmicb.2015.01089.
- Alvarez AF, Georgellis D. In vitro and in vivo analysis of the ArcB/A redox signaling pathway. Methods Enzymol. 2010;471:205–228.
- Iuchi S, Chepuri V, Fu HA, Gennis RB, Lin EC. Requirement for terminal cytochromes in generation of the aerobic signal for the arc regulatory system in Escherichia coli: study utilizing deletions and lac fusions of cyo and cyd. J Bacteriol. 1990;172(10):6020–6025. doi:10.1128/jb.172.10.6020-6025.1990.
- Georgellis D, Kwon O, Lin EC. Quinones as the redox signal for the arc two-component system of bacteria. Sci. 2001;292(5525):2314–2316. doi:10.1126/science.1059361.
- Kwon O, Georgellis D, Lin EC. Phosphorelay as the sole physiological route of signal transmission by the arc two-component system of Escherichia coli. J Bacteriol. 2000;182(13):3858–3862. doi:10.1128/JB.182.13.3858-3862.2000.
- Rolfe MD, Ter Beek A, Graham AI, Trotter EW, Asif HM, Sanguinetti G, de Matto JT, Poole RK, Green J, de Mattos JT. Transcript profiling and inference of Escherichia coli K-12 ArcA activity across the range of physiologically relevant oxygen concentrations. J Biol Chem. 2011;286(12):10147–10154. doi:10.1074/jbc.M110.211144.
- Bose JL, Kim U, Bartkowski W, Gunsalus RP, Overley AM, Lyell NL, Visick KL, Stabb EV. Bioluminescence in Vibrio fischeri is controlled by the redox-responsive regulator ArcA. Mol Microbiol. 2007;65(2):538–553. doi:10.1111/j.1365-2958.2007.05809.x.
- Giaouris E, Samoilis G, Chorianopoulos N, Ercolini D, Nychas GJ. Differential protein expression patterns between planktonic and biofilm cells of Salmonella enterica serovar Enteritidis PT4 on stainless steel surface. Int J Food Microbiol. 2013;162(1):105–113. English. doi: 10.1016/j.ijfoodmicro.2012.12.023.
- Gunsalus RP, Park SJ. Aerobic-anaerobic gene regulation in Escherichia coli: control by the ArcAB and fnr regulons. Res Microbiol. 1994;145(5–6):437–450. doi:10.1016/0923-2508(94)90092-2.
- Lee YS, Han JS, Jeon Y, Hwang DS. The arc two-component signal transduction system inhibits in vitro Escherichia coli chromosomal initiation. J Biol Chem. 2001;276(13):9917–9923. doi:10.1074/jbc.M008629200.
- Longo P, Ota-Tsuzuki C, Nunes A, Fernandes B, Mintz K, Fives-Taylor P, Mayer M. Aggregatibacter actinomycetemcomitans arcB influences hydrophobic properties, biofilm formation and adhesion to hydroxyapatite. Braz J Microbiol. 2009;40(3):550–562. doi:10.1590/S1517-83822009000300018.
- Wang Z, Sun J, Xia T, Liu Y, Fu J, Lo YK, Chang C, Yan A, Liu X. Proteomic Delineation of the ArcA Regulon in Salmonella Typhimurium During Anaerobiosis. Molecular & Cellular Proteomics: MCP. 2018;17(10):1937–1947. doi:10.1074/mcp.RA117.000563.
- Jiang L, Feng L, Yang B, Zhang W, Wang P, Jiang X, Wang L, Slauch JM. Signal transduction pathway mediated by the novel regulator LoiA for low oxygen tension induced Salmonella Typhimurium invasion. PLoS Pathog. 2017;13(6):e1006429. doi:10.1371/journal.ppat.1006429.
- Sengupta N, Paul K, Chowdhury R. The global regulator ArcA modulates expression of virulence factors in Vibrio cholerae. Infect Immun. 2003;71(10):5583–5589. doi:10.1128/IAI.71.10.5583-5589.2003.
- Appleby JL, Parkinson JS, Bourret RB. Signal transduction via the multi-step phosphorelay: not necessarily a road less traveled. Cell. 1996;86(6):845–848. doi:10.1016/S0092-8674(00)80158-0.
- Ishige K, Nagasawa S, Tokishita S, Mizuno T. A novel device of bacterial signal transducers. EMBO J. 1994;13(21):5195–5202. doi:10.1002/j.1460-2075.1994.tb06850.x.
- Matsubara M, Kitaoka SI, Takeda SI, Mizuno T. Tuning of the porin expression under anaerobic growth conditions by his-to-Asp cross-phosphorelay through both the EnvZ-osmosensor and ArcB-anaerosensor in Escherichia coli. Genes To Cells. 2000;5(7):555–569. doi:10.1046/j.1365-2443.2000.00347.x.
- Perraud AL, Weiss V, Gross R. Signalling pathways in two-component phosphorelay systems. Trends Microbiol. 1999;7(3):115–120. doi:10.1016/S0966-842X(99)01458-4.
- Yaku H, Kato M, Hakoshima T, Tsuzuki M, Mizuno T. Interaction between the CheY response regulator and the histidine-containing phosphotransfer (HPt) domain of the ArcB sensory kinase in Escherichia coli. FEBS Lett. 1997;408(3):337–340. doi:10.1016/S0014-5793(97)00459-6.
- Milton DL, O’Toole R, Horstedt P, Wolf-Watz H. Flagellin a is essential for the virulence of Vibrio anguillarum. J Bacteriol. 1996;178(5):1310–1319. doi:10.1128/jb.178.5.1310-1319.1996.
- Morales VM, Bäckman A, Bagdasarian M. A series of wide-host-range low-copy-number vectors that allow direct screening for recombinants. Gene. 1991;97(1):39–47. doi:10.1016/0378-1119(91)90007-X.
- Kovach ME, Elzer PH, Hill DS, Robertson GT, Farris MA, Roop RM 2nd, Peterson KM. Four new derivatives of the broad-host-range cloning vector pBBR1MCS, carrying different antibiotic-resistance cassettes. Gene. 1995;166(1):175–176. doi:10.1016/0378-1119(95)00584-1.
- Qing G, Ma LC, Khorchid A, Swapna GV, Mal TK, Takayama MM, Xia B, Phadtare S, Ke H, Acton T, et al. Cold-shock induced high-yield protein production in Escherichia coli. Nat Biotechnol. 2004;22(7):877–882. doi:10.1038/nbt984.
- Park KS, Ono T, Rokuda M, Jang MH, Okada K, Iida T, Honda T. Functional characterization of two type III secretion systems of Vibrio parahaemolyticus. Infect Immun. 2004;72(11):6659–6665. doi:10.1128/IAI.72.11.6659-6665.2004.
- Kinoshita E, Kinoshita-Kikuta E, Shiba A, Edahiro K, Inoue Y, Yamamoto K, Yoshida M, Koike T. Profiling of protein thiophosphorylation by Phos-tag affinity electrophoresis: evaluation of adenosine 5’-O-(3-thiotriphosphate) as a phosphoryl donor in protein kinase reactions. Proteomics. 2014;14(6):668–679. doi:10.1002/pmic.201300533.
- Liang X, Moore R, Wilton M, Wong MJ, Lam L, Dong TG. Identification of divergent type VI secretion effectors using a conserved chaperone domain. Proc Natl Acad Sci USA. 2015;112(29):9106–9111. doi:10.1073/pnas.1505317112.
- Livak KJ, Schmittgen TD. Analysis of relative gene expression data using real-time quantitative PCR and the 2−ΔΔCT method. Methods. 2001;25(4):402–408. doi:10.1006/meth.2001.1262.
- Liu M, Zhu X, Zhang C, Zhao Z. LuxQ-LuxU-LuxO pathway regulates biofilm formation by Vibrio parahaemolyticus. Microbiol Res. 2021;250:126791. doi:10.1016/j.micres.2021.126791.
- Freeman JA, Bassler BL. Sequence and function of LuxU: a two-component phosphorelay protein that regulates quorum sensing in Vibrio harveyi. J Bacteriol. 1999b;181(3):899–906. doi:10.1128/JB.181.3.899-906.1999.
- Gao R, Stock AM. Biological insights from structures of two-component proteins. Annu Rev Microbiol. 2009;63(1):133–154. doi:10.1146/annurev.micro.091208.073214.
- Freeman JA, Bassler BL. A genetic analysis of the function of LuxO, a two-component response regulator involved in quorum sensing in Vibrio harveyi. Mol Microbiol. 1999a;31(2):665–677. doi:10.1046/j.1365-2958.1999.01208.x.
- Iuchi S, Lin EC. arcA (dye), a global regulatory gene in Escherichia coli mediating repression of enzymes in aerobic pathways. Proc Natl Acad Sci USA. 1988;85(6):1888–1892. doi:10.1073/pnas.85.6.1888.
- Shimohata T, Mawatari K, Iba H, Hamano M, Negoro S, Asada S, Aihara M, Hirata A, Su Z, Takahashi A. VopB1 and VopD1 are essential for translocation of type III secretion system 1 effectors of Vibrio parahaemolyticus. Can J Microbiol. 2012;58(8):1002–1007. doi:10.1139/w2012-081.
- Burdette DL, Seemann J, Orth K. Vibrio VopQ induces PI3-kinase-independent autophagy and antagonizes phagocytosis. Mol Microbiol. 2009;73(4):639–649. doi:10.1111/j.1365-2958.2009.06798.x.
- Mizuno T. His-Asp phosphotransfer signal transduction. J Biochem. 1998;123(4):555–563. doi:10.1093/oxfordjournals.jbchem.a021972.
- Matsushika A, Mizuno T. A dual-signaling mechanism mediated by the ArcB hybrid sensor kinase containing the histidine-containing phosphotransfer domain in Escherichia coli. J Bacteriol. 1998;180(15):3973–3977. doi:10.1128/JB.180.15.3973-3977.1998.
- Fong KP, Gao L, Demuth DR. luxS and arcB control aerobic growth of Actinobacillus actinomycetemcomitans under iron limitation. Infect Immun. 2003;71(1):298–308. doi:10.1128/IAI.71.1.298-308.2003.
- Salmon KA, Hung SP, Steffen NR, Krupp R, Baldi P, Hatfield GW, Gunsalus RP. Global gene expression profiling in Escherichia coli K12: effects of oxygen availability and ArcA. J Biol Chem. 2005;280(15):15084–15096. doi:10.1074/jbc.M414030200.
- Alvarez AF, Rodriguez C, Georgellis D. Ubiquinone and menaquinone electron carriers represent the yin and yang in the redox regulation of the ArcB sensor kinase. J Bacteriol. 2013;195(13):3054–3061. doi:10.1128/JB.00406-13.
- Loui C, Chang AC, Lu S. Role of the ArcAB two-component system in the resistance of Escherichia coli to reactive oxygen stress. BMC Microbiol. 2009;9(1):183. doi:10.1186/1471-2180-9-183.
- van Beilen JW, Hellingwerf KJ, van Beilen JWA. All three endogenous quinone species of Escherichia coli are involved in controlling the activity of the aerobic/anaerobic response regulator ArcA. Front Microbiol. 2016;7:1339. doi:10.3389/fmicb.2016.01339.
- Xie Y, Li J, Ding Y, Shao X, Sun Y, Xie F, Liu S, Tang S, Deng X. An atlas of bacterial two-component systems reveals function and plasticity in signal transduction. Cell Rep. 2022;41(3):111502. doi:10.1016/j.celrep.2022.111502.
- Imelio JA, Trajtenberg F, Buschiazzo A. Allostery and protein plasticity: the keystones for bacterial signaling and regulation. Biophys Rev. 2021;13(6):943–953. doi:10.1007/s12551-021-00892-9.
- Ritchie JM, Rui H, Zhou X, Iida T, Kodoma T, Ito S, Davis BM, Bronson RT, Waldor MK, Valdivia RH. Inflammation and disintegration of intestinal villi in an experimental model for Vibrio parahaemolyticus-induced diarrhea. PLoS Pathog. 2012;8(3):e1002593. doi:10.1371/journal.ppat.1002593.
- Gu D, Zhang Y, Wang Q, Zhou X. S-nitrosylation-mediated activation of a histidine kinase represses the type 3 secretion system and promotes virulence of an enteric pathogen. Nat Commun. 2020;11(1):5777. doi:10.1038/s41467-020-19506-1.
- Lu R, Osei-Adjei G, Huang X, Zhang Y. Role and regulation of the orphan AphA protein of quorum sensing in pathogenic Vibrios. Future Microbiol. 2018;13(3):383–391. doi:10.2217/fmb-2017-0165.
- Wang L, Ling Y, Jiang H, Qiu Y, Qiu J, Chen H, Yang R, Zhou D. AphA is required for biofilm formation, motility, and virulence in pandemic Vibrio parahaemolyticus. Int J Food Microbiol. 2013;160(3):245–251. doi:10.1016/j.ijfoodmicro.2012.11.004.