ABSTRACT
The gut-joint axis, one of the mechanisms that mediates the onset and progression of joint and related diseases through gut microbiota, and shows the potential as therapeutic target. A variety of drugs exert therapeutic effects on rheumatoid arthritis (RA) through the gut-joint axis. However, the anti-inflammatory and immunomodulatory effect of novel photobiomodulatory therapy (PBMT) on RA need further validation and the involvement of gut-joint axis in this process remains unknown. The present study demonstrated the beneficial effects of PBMT on RA, where we found the restoration of gut microbiota homeostasis, and the related key pathways and metabolites after PBMT. We also discovered that the therapeutic effects of PBMT on RA mainly through the gut-joint axis, in which the amino acid metabolites (Alanine and N-acetyl aspartate) play the key role and rely on the activity of metabolic enzymes in the target organs. Together, the results prove that the metabolites of amino acid from gut microbiota mediate the regulation effect on the gut-joint axis and the therapeutic effect on rheumatoid arthritis of PBMT.
Introduction
Rheumatoid arthritis (RA) is a chronic autoimmune disease that predominantly affects the joints. Alterations in gut microbiota composition and metabolites have been demonstrated to exacerbate the development of RA in both clinical patients and animal models.Citation1,Citation2 The concept of the gut-joint axis was first proposed in 2006, when an increase in cross-reactive antibodies was discovered in the gut lavage fluid of patients with RA and was further found to be associated with RA.Citation3 In the past decade, dysbiosis of the gut microbiota has shaped the immune system, induced persistent immune inflammatory reactions, and contributed to the process of RA symptoms.Citation4 Differences in the microbial composition between patients with RA and healthy controls have been observed, and gut microbiota modulation can alleviate RA symptoms, suggesting that gut microbiota play a role in regulating immune responses and the pathogenesis of RA.Citation5 Moreover, metabolites are the primary components of the gut microbiota that exert their effects in the gut-joint axis.Citation6,Citation7 Various therapeutic drugs, probiotics, and fecal microbiota transplants can alleviate RA progression by modulating the dysbiosis and metabolism of the gut microbiota. Although the main mechanism of photobiomodulation therapy (PBMT) for regulating inflammation and immune imbalance has been demonstrated, the alterations in gut microbiota and the role of the gut-joint axis in RA remain unclear.
In this study, we identified the impact of PBMT on the RA process through the gut-joint axis. We revealed the main effector through which PBMT affects the process of RA via the gut-joint axis. Furthermore, we identified the crucial role of target organs in the gut-joint axis, thus providing insights into the gut-joint axis. This study provides new evidence and choice for the selection of appropriate metabolites for the treatment of RA and evaluation of treatment efficacy.
Materials and methods
Animal experiment
TNF-Tg and WT mice (Background C57BL/6J; 18–22 g; Age of 10w after birth) were used in this study. The mice were obtained from Cyagen Technologies Co., Ltd. (Jiangsu China). All experimental protocols involving mice were approved by the Animal Ethics and Welfare Committee of Harbin Medical University, Harbin, China (Number: HMUIRB2023003). LED device (CP-YL3213-630, Truwin Optoelectronic Medical, Beijing, China) was used to deliver 630 nm radiation.
The total of 38 mice were randomly assigned to four groups: WT group (n = 10), TNF-Tg group (n = 8), TNF-Tg+LED (irradiation with 630 nm LED; n = 10), and TNF-Tg + 2LED (irradiation with a double dose of 630 nm LED; n = 10). The LED radiation treatment lasted for 20 days and operated in dark. The LED irradiation time was 30 min, power density was 8.2 mW/cm2, and energy density was 14 J/cm2. Treatment was performed once or twice daily for 20 days.
In the confirmation experiment, a total of 21 mice were randomly assigned to four groups: WT group (n = 5), TNF-Tg group (n = 5), TNF-Tg+AA (administration with alanine and N-acetyl aspartic acid; n = 6), and TNF-Tg + 2LED (irradiation with a double dose of 630 nm LED; n = 5). The LED radiation and AA administration lasted for 14 days and operated in dark. The prepared amino acid solution consists of 3% L-alanine (L-alanine powder, MACKLIN, 99%)Citation8, 0.8% D-alanine (D-alanine powder, MACKLIN, cat 98%)Citation9 and 0.2% N-acetyl aspartic acid (N-acetyl aspartic acid powder, MACKLIN, 95%)Citation10. Dissolve in mice drinking water and drink freely.
The RA Score and paw swelling was evaluated in all groups every day. After the last treatment of LED irradiation or AA administration, the mice were sacrificed under deep anesthesia for collection of stool, joints, and paws.
Standard of paw thickness measurement and RA scoring
Those are modified according to Bonnefoy et al.Citation11 Paw thickness was measured in all four groups. Foreleg thickness was measured for each mouse. RA Score was measured in all four groups. Each mouse received 4 points for each paw, for a total of 16 points: 0 points, no change; 1 point for redness or swelling of the toes; redness and swelling of the toes; 3 points, more than 2 feet redness or swelling of the toes or swelling of the whole paw of the mice, but not involving the ankle joint; 4 points, lesions involving the ankle. Pearson correlation analysis of the relationship between paw swelling and RA score.
Cell culture with metabolites and LED irradiation
The human synovial fibroblast cell line MH7A was purchased from the Guan Dao Biological Research Center of Shanghai. The cells were cultured in DMEM supplemented with fetal bovine serum (10%) and penicillin/streptomycin solution (1%). Cultured cells were maintained at 37°C in a 5% CO2 atmosphere.
Cells were exposed through the bottom of plastic culture plates to the 630nm radiation 6 times. The time it took to irradiate once was 15 min to achieve a radiation energy of 9 J/cm2. The growth medium of all groups was replaced with new cell culture medium, whereas for TNF-α stimulation groups, TNF-α (10 ng/mL, PeproTech) was added before radiation. To clarify the effects of Alanine (ALA) and N-acetylaspartic acid (NAA) on TNF-induced inflammatory response in MH7A cells, the cells were incubated with ALA, NAA and 10 ng/ml TNF-α for 6 h. ALA and NAA were administered at concentration of 10 μM, and 20 μM. The cells of the experimental groups were irradiated at room temperature. The control groups (non-irradiated cells) were wrapped with aluminum foil and kept at room temperature while the experimental samples were irradiated.
Quantitative real-time PCR
Reverse transcriptase polymerase chain reaction (RT-PCR) was performed on the total RNA harvested from synovial fibroblast cells using a RNeasy mini kit (Hai Gene, Harbin, China). Equal amounts of total RNA (2 μg) were reverse-transcribed using a Thermo script RT-PCR system (Invitrogen, Carlsbad, CA, USA). The resultant cDNA was PCR-amplified for pro-inflammatory cytokines using human gene-specific sense and antisense primers based on sequences published in GenBank (Primer used in this study was provide in Table S2). PCR was carried out on a LightCycler® 96 thermal cycler instrument (Roche Applied Science, Penzberg, Germany). The program used for quantitative PCR amplification included a 5-min pre-denaturation step at 95°C, a 3-step amplification at 95°C for 10 s, 60°C for 20 s (for 45 cycles), and a dissociation step (10 s at 98°C, 60 s at 65°C, and 1 s at 97°C).
Fecal DNA extraction, 16s rDNA sequencing and bioinformatics analysis
A DNA extraction kit (DNeasy Power Soil Kit; Qubit dsDNA Assay Kit, Qiagen, Germany) was used to extract genomic DNA from fecal samples (n = 38). Specific primers with barcodes and a high-fidelity enzyme (Takara Ex Taq; Takara, Japan) were used for PCR according to the selected sequencing area. Samples were sequenced on an Illumina MiSeq platform (San Diego, CA, USA).
The original data were in the FASTQ format. Trimmomatic software (Illumina) was used to remove the clutter from the original double-ended sequence. The Vsearch software (https://github.com/torognes/vsearch) was used to classify the sequences into multiple Operational Taxonomic Unit (OTUs) according to sequence similarity. Representative sequences of each OTU were selected using the QIIME2 software package (http://qiime.sourceforge.net/), and all representative sequences were compared with the Silva (version 132) database for annotation.
Alpha and beta diversity calculations were performed using QIIME2 and R (v3.6.1). Principal Coordinates Analysis (PCoA) was based on unweighted UniFrac distance, and the P-value of analysis of similarities (ANOSIM) was obtained using a permutation test. We then conducted a linear discriminant analysis (LDA) effect size (LEfSe) analysis to determine the differential taxa. The microbial dysbiosis index (MDI) was used to evaluate microbial dysbiosis previously described in prior studies.Citation12 The MDI was defined as the log value of [total abundance of bacterial genera enriched in TNF-Tg mice] divided by [total abundance of bacterial genera depleted in TNF-Tg mice]. Phylogenetic investigation of communities by reconstruction of unobserved states 2 (PICRUST2) was applied to predict the function and metabolic enzymes of 16s rDNA. Metabolites were predicted by metabolic enzymes using the KEGG database. Subsequently, metabolic enzymes were predicted from the metabolites, and the relationships between pathways, enzymes, and metabolites were determined using a Sankey plot. Other graphs were produced using the R software.
Protein extraction, LC-MS/MS analysis and bioinformatics analysis
A corresponding volume of 25 mM dl-dithiothreitol was added to each sample. Then, six times the volume of precooled acetone was added to precipitate the protein. After the precipitation, the sample was centrifuged to collect the precipitate. Then, 10 μl of TMT label reagent was added to each sample and incubated for 1 h. Finally, hydroxylamine was added to each sample and incubated for 15 min to terminate the reaction. Reversed-phase (RP) separation was performed using a 1100 HPLC System (Agilent). The separated peptides were lyophilized for mass spectrometry.
The peptides were subjected to a capillary source, followed by TimsTOF Pro (Bruker Daltonics) mass spectrometry. The resulting MS/MS data were processed using the MaxQuant search engine (v1.6.15.0). Tandem mass spectra were searched against the human SwissProt database (20422 entries) concatenated with a reverse-decoy database. Carbamidomethyl on Cys was specified as a fixed modification, and acetylation on the protein N-terminus and oxidation on Met were specified as variable modifications. Differential expression (DE) analysis was performed in R (v3.2.1) using EdgeR package v3.10.2, as previously described, except for the applied contrast. Paired DE analysis was performed to assess changes within the groups. The EdgeR analysis was based on section 3.5 of the Edge R user’s guide.
Molecular docking
The molecular docking method was used to investigate the interaction potential and binding efficacy of ALA and NAA toward the three-dimensional structure of the most important protein molecules of the proteome, such as p65, MMP9, Caspase3 and Caspase8. ChemBio3D Ultra 14.0 program was used to construct 3D structures of ALA and NAA, energy minimized, and converted to mol2 files. The X-ray crystal structures of Caspase3 (PDB ID:5IBP), Caspase8 (PDB ID:4ZBW), Mmp9 (PDB ID:6ESM) and P65 (PDB ID:4OT9) were obtained from the RCSB Protein Data Bank (www.rcsb.org/pdb). These structures were loaded into AutoDock Tools v1.5.6,Citation13 the atomic charge was added, the atomic type was assigned, and all flexible bonds could be rotated by default. The DEEPSITE was used to predict protein binding pockets online.Citation14 The binding mechanism of Caspase8, Caspase3, MMP9 and p65 to ALA and NAA were analyzed using Auto Dock Vina v1.1.2.Citation15 The docking process was calculated by the Genetic Algorithm. All docking run options were default values. Finally, the docking results with the highest scores were visualized by Discovery Studio 4.0 Visualizer (BIOVIA, CA, USA).
Statistical analyses
All data with error bars are represented as the mean ± SEM. For two-group comparisons, unpaired two-tailed Student’s t-test was performed. For more than two group comparisons, one-way ANOVA was performed. Statistical significance was set at P < 0.05. The original ab2022 (Origin Lab Corporation, Northampton, MA, USA) was used for the statistical analysis and figure production. Pearson’s correlation coefficient was used to calculate the relationship between paw and RA scores, gut microbiome and RA Score, and the co-occurrence network of the gut microbiome.
Result
PBMT alleviates RA progression and inflammatory response in TNF-Tg mice
To assess the effect of PBMT on RA progression, TNF-Tg mice were irradiated with 630 nm LED.
The TNF-Tg group exhibited significant changes in the degree of joint swelling and paw arthritis score as RA progressed over 20 days (P < 0.01, ). On the 20th day, the TNF-Tg group showed a significant increase in joint swelling and paw arthritis score compared to the WT group (P < 0.01, ). However, TNF-Tg+LED and TNF-Tg + 2LED groups demonstrated a slower increase in arthritis score, with significantly reduced arthritis scores and paw thickness changes compared to TNF-Tg groups (P < 0.05, ). Pearson correlation analysis indicated a positive correlation between the degree of digital joint swelling and paw arthritis score (P < 0.05, ).
Figure 1. The anti-rheumatic effect of 630nm LED irradiation on C57BL/6 TNF-Tg mice. (a) Representative images of mice paw on the 20 days after primary LED irradiation. (b) Clinical arthritis score of the mice (Age of 10w after birth). The inflammation levels of mice before PBM were displayed at day 0. * indicates TNF-Tg+LED vs TNF-Tg < 0.05, # indicates TNF-Tg + 2LED vs TNF-Tg (n = 8) <0.05, & indicates TNF-Tg+LED (n = 10) vs TNF-Tg + 2LED (n = 10) <0.05. WT (n = 10); (c) Hind paw thickness changes. (d) RA Score of mice paw on 20th day of LED irradiation. (e) Positive correlations of the RA Score to Paw swelling. Each dot indicates a subject. Solid blue line represents linear regression curve. (f) GSEA plots comparing the four groups. Leading-edge genes from gene sets are shown as black outlined dots. Shaded gray area depicts ART. (g) The proteomic shows the key proteins changes in the TNF-α pathway. P-values by White’s nonparametric t-test, Storey FDR correction.
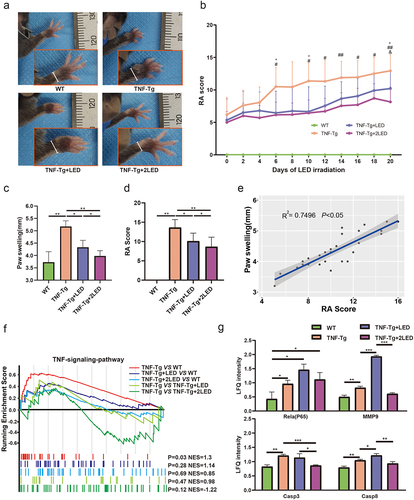
Given that the TNF signaling pathway mediates the activation of the TNF-Tg inflammatory response and its induction of the RA process,Citation16 the synovial tissue of the mice paw joints was subjected to proteomic and GSEA pathway enrichment analyses after LED irradiation. The GSEA results showed a significant activation of the TNF signaling pathway in the TNF-Tg groups (P < 0.05), while no significant difference was observed between the TNF-Tg + 2LED group and the WT group, indicating the activation of TNF signaling inhibited by PMBT in the treatment groups (). Furthermore, LED irradiation suppressed the expression of key inflammatory factors, such as Casp3, Casp8, Mmp9, and P65 in TNF signaling pathway (P < 0.05, ). In summary, these findings suggest that PBMT affects the RA process by inhibiting the TNF signaling pathway.
Microbiota diversity analysis of TNF-Tg mice after PBMT irradiation
In this study, we aimed to investigate the impact of PBMT on the inhibition of RA and its association with gut microbiota. The changes in gut microbiota induced by PBMT in RA were sequenced using 16s rDNA. Mice fecal DNA samples were collected from four groups of mice, including wild-type (WT; n = 10), TNF-transgenosis (TNF-Tg; n = 8), TNF-Tg mice receiving a single dose of LED irradiation (TNF-Tg+LED; n = 10), and TNF-Tg mice receiving a double dose of LED irradiation (TNF-Tg + 2LED; n = 10). After processing and quality control, 1,064 operational taxonomic units (OTUs) from 3,710,965 sequencing data were identified.
The α-diversity of the gut microbiota in the TNF-Tg groups was lower than that in the WT groups (P = 0.016, Wilcoxon rank-sum test; ). However, LED irradiation restored the α-diversity of the gut microbiota in TNF-Tg mice, similar to that in the WT group. PCoA based on weighted UniFrac distance showed differences in β-diversity of the gut microbiota among the TNF-Tg, WT, and TNF-Tg+LED groups (PERMANOVAR, P = 0.001, ). Notably, LED irradiation exhibited a dose-dependent effect on the composition of the gut microbiota. Moreover, microbiota that displayed a higher association with LED was identified (PERMANOVA, P = 0.001, ).
Figure 2. Analysis of the gut microbiota in the colon between four group. (a) Alpha diversity of the gut microbiota between the four groups. (b) Beta diversity of the gut microbiota between the four groups was assessed by principal coordinate analysis using the Bray – Curtis index. (c) The relative abundance of bacteria at the phylum level between the four groups is presented by the colorful columns. (d) The heatmap of relative abundance at phylum level.
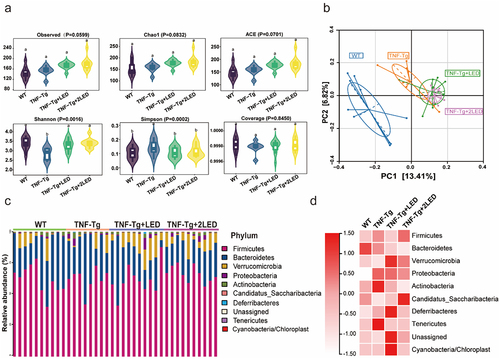
Moreover, the composition and relative abundance of the gut microbiota at the phylum level were examined. Firmicutes (62.60%), Bacteroidetes (27.18%), Verrucomicrobia (7.13%), and Proteobacteria (1.34%) were the predominant phyla accounting for approximately 98.17% of the gut microbiota. Compared to the WT group, the TNF-Tg group exhibited an increase in Firmicutes, Actinobacteria, Proteobacteria, and Tenericutes (P < 0.05), and a decrease in Verrucomicrobia (P < 0.05) (). However, LED irradiation led to an increase in Verrucomicrobia and a decrease in Actinobacteria (P < 0.05) compared with TNF-Tg mice. Additionally, after LED irradiation, the relative abundance of Verrucomicrobia, Proteobacteria, Actinobacteria, Candidatus_Saccharibacteria, and Tenericutes tended to approach that of WT mice ().
In addition, the gut microbiota was analyzed at the genus level. Notably, a significant difference in MDI was observed between the WT and TNF-Tg groups (P < 0.01), indicating microbial dysbiosis in the TNF-Tg groups. However, LED irradiation resulted in a reduction in the MDI compared to the TNF-Tg group (P < 0.05), and the MDI of the TNF-Tg + 2LED group exhibited no significant difference compared to that of the WT group (P > 0.05, ). These results provide evidence that PBMT can modulate dysbiosis of the gut microbiome in a dose-dependent manner.
Figure 3. Analysis of the gut bacteria co-occurrence network between the WT group, TNF-Tg group, TNF-Tg+LED group and TNF-Tg+2LED group. (a) Nodes of the network are colored by the degree corresponds to relative abundance of the bacteria. (b) The analysis of four groups co-occurrence network of gut bacteria. (c) The microbial dysbiosis index (MDI) of four groups mice.
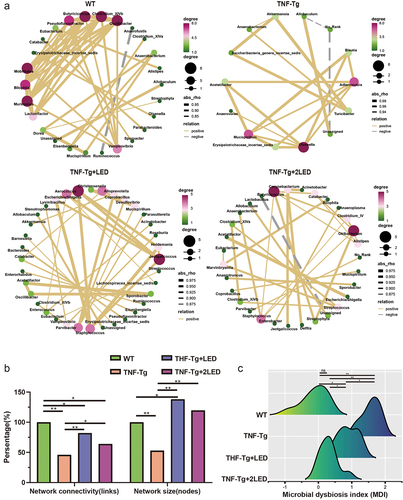
Moreover, our findings demonstrate that after PBMT, the gut microbiota reached PBMT-specific homeostasis in TNF-Tg mice, characterized by Firmicute and Verrucomicrobia (). PBMT was found to regulate the abundance of beneficial phyla, leading to a significant recovery of TNF-Tg gut microbiota dysbiosis at the genus level. Notably, the TNF-Tg + 2LED was completely restored compared with the WT group.
PBMT promotes new homeostasis of gut microbiota in TNF-Tg mice
To clarify the bacterial co-occurrence network and homeostasis of the gut microbiota in TNF-Tg mice after LED irradiation, a bacterial co-occurrence network was constructed with the gut microbiota at the genus level using Pearson correlation analysis. The bacterial co-occurrence networks of the four groups were analyzed (). The results of the gut microbiota co-occurrence network showed a significant decrease in the network correlation and size of the gut microbiota in the TNF-Tg groups (P < 0.01). After LED irradiation, the network correlation and size of the gut microbiota in TNF-Tg mice were restored (Spearman Rho > 0.5, P < 0.01; ). These results indicate that although LED irradiation restored the stability of the gut microbiota co-occurrence network, the genera that make up the network changed. Additionally, the above results confirmed that after irradiation, the stability of the co-occurrence network was restored, and the genus that made up the network changed compared to the TNF-Tg groups, suggesting that LED irradiation promotes homeostasis in the gut microbiota.
Identification of RA-associated genus in gut microbiota after PBMT irradiation
To investigate the effect of PBMT on bacterial genus related to RA, linear discriminant analysis effect size (LEfSe) was employed to compare the differential genus between the four groups. The TNF-Tg group showed an increase in Prevotella, Aerococcus, and Staphylococcus, whereas the abundance of Parasutterella and Clostridim_XIVa decreased (P <.05).
Upon receiving single and double doses of LED irradiation, the abundance of Eisenbergiella and Turicibacter increased, while the abundance of Parasutterella and Olsenella decreased compared to the TNF-Tg group (). LED irradiation also recovered the abundance of Aerococcus, Odoribacter, Alistipes, and Clostridium to the abundance of the WT group (P < 0.01; ).
Figure 4. The changes of gut microbial composition at the genus level in WT、TNF-Tg、TNF-Tg+LED and TNF-Tg +2LED group. Chart showing significantly differentially abundant genus (P < 0.01), where genus is grouped and colored by phylum along the y-axis. The x-axis indicates the fold-change in three compared groups as baseline. (b) Boxplots indicating the percentage relative abundance of taxa showing differential abundance among the four groups (P < 0.01). (c) Integrated correlation-based analysis of microbes and RA Score. Pearson’s correlation analysis from the in the three group. (d) The Venn shows the different genus related to LED and RA.
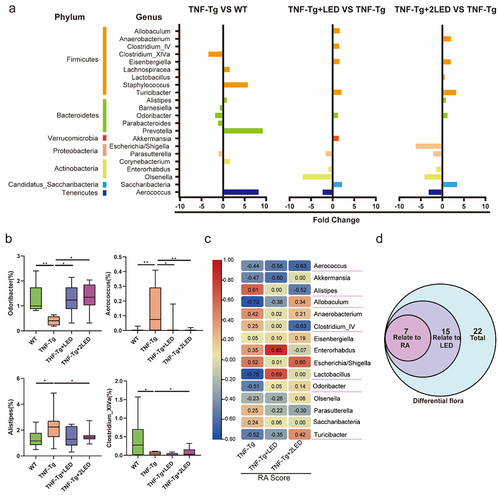
Furthermore, Pearson correlation analysis showed that among the 22 differential genus, 15 genera were associated with LED irradiation and 7 genera were linked to RA scores after LED irradiation. Among these microbial genera, Alistipes, Aerococcus, Allobaculum, Clostridium_IV, Lactobacillus, Odoribacter and Turicibacter were correlated with RA Score ().
PBMT alters metabolic pathways and metabolites of gut microbiota in TNF-Tg mice
To assess the alterations in metabolic pathways of 15 LED irradiation-related bacterial genera and 7 RA-related bacterial genera, as well as their potential impact on the process of RA, PICRUSt2 was used to predict the function of the gut microbiota among the four groups.
Notably, 94 pathways exhibited differential expression among the four groups in 15 LED irradiation-related bacterial genera (). A Venn diagram was used to select 11 synthetic and metabolic pathways recovered after irradiation, including those involved in alanine, aspartate, and glutamate metabolism; D-glutamine and D-glutamate metabolism; thiamine synthesis; streptomycin biosynthesis; penicillin and cephalosporin biosynthesis; unsaturated fatty acid biosynthesis; ascorbic acid and aldehyde metabolism-related pathways; linoleic acid metabolism-related pathways; styrene degradation pathway; two-component system; and sulfur relay system. Intriguingly, these 11 pathways exhibited differences between the WT and TNF-Tg groups and were reversed after irradiation (). PBMT may affect the gut microbiota via these 11 pathways, thereby influencing the inflammatory response in TNF-Tg mice ().
Figure 5. The effect of the gut microbiota modifications on predicted functional metabolic pathways obtained from PICRUSt2 analysis of 16S rRNA sequencing data.
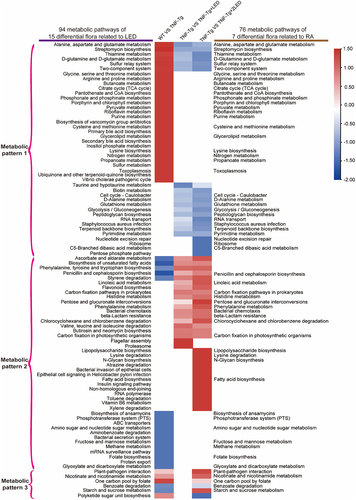
Figure 6. The functional prediction of PICRUSt2 the gut microbiota in mices. (a-b) The Venn shows the numbers of pathways related to LED irradiation and RA process. Each box plot indicates the mean proportion of sequences assigned to pathways in each group. P-values by White’s nonparametric t-test, Storey FDR correction.
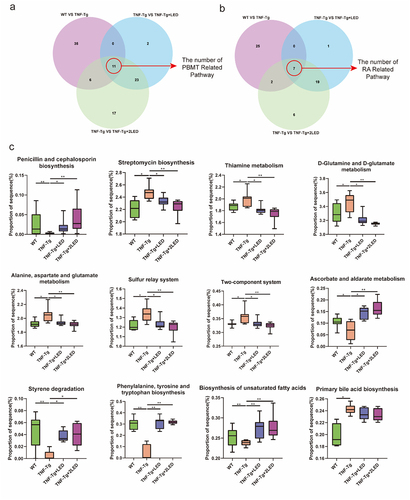
PICRUSt2 was used to predict changes in gut microbiota pathways, and 76 different pathways were analyzed among the four groups (). Based on the correlation between pathway abundance and RA Score, we divided 76 pathways into three groups: positive correlation between LED treatment and RA Score (Metabolic pattern 1), negative correlation between LED treatment and RA Score (Metabolic pattern 2), and others (Metabolic pattern 3). To characterize the inflammatory pathways of TNF-Tg groups that were reversed after LED irradiation (Metabolic pattern 1&2), we selected seven synthetic and metabolic pathways related to RA after irradiation by drawing a Venn diagram, including pathways related to alanine, aspartate, and glutamate metabolism; D-glutamine and D-glutamate metabolism; thiamine synthesis signaling pathway; streptomycin biosynthesis pathway; and penicillin and cephalosporin biosynthesis signaling pathway. These pathways showed significant differences between the WT and TNF-Tg groups and were reversed in the TNF-Tg+LED groups (). These findings suggest that PBMT may affect the gut microbiota through these pathways and influence the RA process in TNF-Tg mice ().
To investigate alterations in metabolites in these pathways, PICRUSt2 was used to predict metabolic enzymes in five synthetic and metabolic pathways. Subsequently, Pearson correlation was used to analysis between the enzymes in five pathways and the RA Score (), which led to the identification of 10 enzymes correlated with RA (|rho| > 0.5, P < 0.05). Notably, these 10 enzymes were significantly different between the WT and TNF-Tg groups, which was reversed after LED irradiation (P < 0.05; ). Using the KEGG enzyme database, we discovered that 10 enzymes in the five pathways induced changes in 11 metabolites present in the gut microbiota of TNF-Tg mice after irradiation, including amino acid metabolites such as L/D-aspartate, N-acetyl-aspartate (NAA), L-glutamine, L/D-alanine (ALA), antibiotic metabolites such as cephalosporin and streptomycin, nucleosides, and sulfur-containing amino acids. A Sankey diagram was employed to depict the relationship between the pathways, enzymes, and metabolites (), and the metabolites were associated with the attenuation of inflammation, indicating that PBMT has an impact on the RA process through these 11 metabolites.
Figure 7. RA related metabolic enzymes, pathways and metabolites of gut microbiota changed in the mice. (a) Each box plot indicates the mean proportion of sequences assigned to the metabolic enzymes in each group-values by White’s nonparametric t-test, Storey FDR correction. (b) Integrated correlation-based analysis of metabolic enzymes in five pathways and RA Score. Pearson’s correlation analysis from the in the three groups. (c) The Sankey diagram shows the correction among the enzyme, pathway, classification and products after the 630 nm LED irradiation.
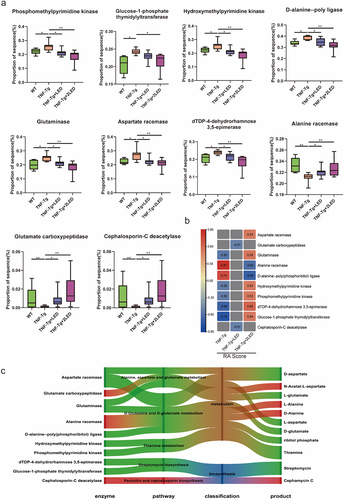
The gut metabolites mediated PBMT anti-inflammatory effects and gut-joint axis regulation
In this study, we investigated the transfer of gut microbiota metabolites and their anti-inflammatory effects in mice joints. The levels of inflammatory cytokines (IL-1β, IL-6, and IL-10) were assessed using RT-qPCR (). After induction with TNF-α, MH7A synovial cells exhibited increased IL-1β and IL-6 levels and decreased IL-10 levels (P < 0.05). The expression of IL-1β decreased and IL-10 increased after LED irradiation (P < 0.05), indicating that PBMT may inhibit TNF-α inflammation by suppressing IL-1β and upregulating IL-10. Treatment with 10 mM ALA resulted in a decrease in IL-6 levels and an increase in IL-10 levels (P < 0.05). Similarly, 10 mM NAA treatment led to decreased IL-6 and IL-1β levels (P < 0.05). This suggests that ALA and NAA inhibit inflammation by upregulating IL-10, similarly to PBMT. Notably, no significant changes in IL-6 expression were observed after LED irradiation. In contrast, IL-6 expression levels decreased significantly after NAA treatment (P < 0.05), indicating that PBMT may regulate IL-6 production through gut microbiota metabolites. Both PBMT and gut microbiota metabolites were found to regulate IL-1β and IL-10 expression.
Figure 8. The gut metabolites mediated PBMT effects and its mechanism in the RA process of TNF-Tg mice. (a) The relative expression of IL-1β, IL-6 and IL- 10 after the irradiation of LED or gut metabolites. (b-h) Optimal conformation of molecular docking of alanine (ALA) and N-acetyl-aspartate (NAA) with Caspase3, Caspase8, MMP9 and P65. (i) The proteomic analysis the changes of metabolic enzymes in mice joint synovium. P-values by White’s nonparametric t-test, Storey FDR correction.
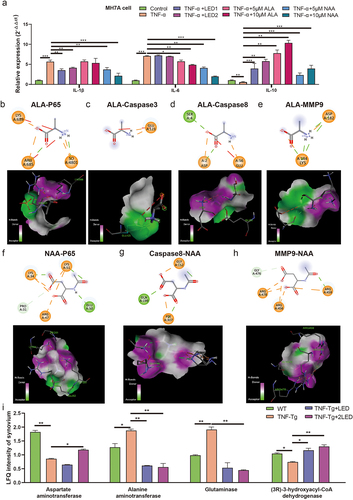
To investigate the effect of ALA and NAA on inflammation-related proteins within the TNF signaling pathway after LED irradiation (), we conducted molecular docking experiments with four target proteins: Caspase3, Caspase8, MMP9, and P65. Molecular docking results revealed that ALA exhibited binding free energies of − 4.7, −4.5, −3.9, and −4.1 kcal/mol with Caspase3 protein (PDB ID:5IBP), Caspase8 protein (PDB ID:4ZBW), MMP9 protein (PDB ID:6ESM), and P65 protein (PDB ID:4OT9), respectively. Similarly, NAA exhibited binding free energies of − 3.3, −3.0, and − 4.9 kcal/mol with Caspase8, MMP9 and P65, respectively. The bond lengths of the key hydrogen bonds were shown in (Figure S1, Table S3). These results demonstrated that ALA and NAA have the potential to bind Caspase3, Caspase8, MMP9, and P65 proteins, thus facilitating favorable interactions ().
Subsequently, to determine whether ALA and NAA can function within the mice synovial membrane, amino acid and unsaturated fatty acid metabolizing enzymes in the proteomics analysis of the four groups were analyzed. The results indicated that after LED irradiation, the LDA of aspartate aminotransferase increased, while the LDA of alanine aminotransferase and glutamine synthetase decreased. Specifically, the levels of alanine increased, whereas the levels of aspartate, glutamine, and arginine decreased in mice synovial metabolites (). Since the metabolism of TNF-Tg mice gut microbiota was upregulated in unsaturated fatty acid biosynthesis after irradiation, the LDA of fatty acid β-oxidation enzymes increased in synovial tissue, indicating a decrease in unsaturated fatty acids. Therefore, it may not exert anti-inflammatory effects on the synovial joints of mice. The changes in amino acid-metabolizing enzymes suggest that PBMT may act on joints through ALA and NAA, while being influenced by the host’s metabolizing enzyme levels.
To investigate the effect of ALA and NAA, metabolites of gut microbes associated with the therapeutic efficacy of PBMT, on the inflammatory process of RA. TNF-Tg mice were administrated with PBMT and a mixed amino acid solution consisting of ALA & NAA(AA). Changes in the degree of joint swelling and RA score were monitored daily.
Two different groups were treated by double dose of LED and AA simultaneously. On the 14th day after treatment, TNF-Tg mice showed significantly altered joint swelling and higher RA scores compared with the WT group (P < 0.01, ). After LED irradiation, the degree of joint swelling and RA Scores of TNF-Tg mice were significantly decreased compared with the untreated group (P < 0.01, ). Mixed amino acid (AA) treatment resulted in a significant reduction in RA score and joint swelling in TNF-Tg mice compared with the untreated group (P < 0.05, ). Comparison between groups revealed that the protective effect of AA was weaker than LED irradiation on TNF-Tg mice (P < 0.05, ). Pearson correlation analysis suggested a positive correlation between the degree of digital joint swelling and RA score (P < 0.05, ).
Figure 9. The anti-rheumatic effect of AA (ALA&NAA) administration or LED irradiation on C57BL/6 TNF-Tg mice. (a) Representative images of mice paw on the 14days after primary ALA&NAA administration or LED irradiation. (b) RA Score of the mice on the 14 days after primary ALA&NAA administration or LED irradiation. * indicates TNF-Tg+LED vs TNF-Tg < 0.05, & indicates TNF-Tg+AA vs TNF-Tg (n = 5) <0.05, # indicates TNF-Tg+AA (n = 6) vs TNF-Tg + 2LED (n = 5) <0.05; WT (n = 5. (c) Hind paw thickness changes. (d) RA Score of mice paw on 14th day of LED irradiation. (e) Positive correlations of the RA Score to paw swelling. Each dot indicates a subject. Solid blue line represents linear regression curve.
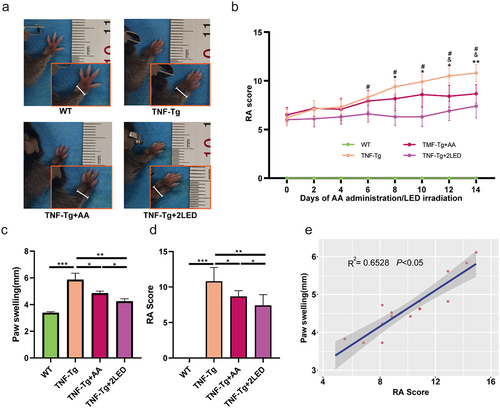
Discussion
Based on the foundation of our previous study on the anti-inflammatory effects of PBMT,Citation17,Citation18 the present study demonstrated the altered composition and symbiosis of the microbiota of TNF-Tg mice after PBMT, which resulted in the formation of a new homeostasis of gut microbes. Alanine and N-acetylaspartate were identified as amino acid metabolites that exerted anti-inflammatory effects in the gut microbiota after PBMT. Finally, the ability of the amino acid metabolites to inhibit TNF-α-induced inflammation in human synoviocytes as well as in TNF-Tg mice was experimentally confirmed, and the mechanism by which the amino acid metabolites of gut microbiota mediate the therapeutic effects of PBMT in rheumatoid arthritis as well as its modulation of the gut-joint axis was demonstrated.
Animal studies of RA typically use CIA-induced arthritis. The CIA was established by immunogenetically susceptible (DBA/1) mice to type II collagen, resulting in polyarthritis that is clinically and histologically similar to RA.Citation19 In 1991, Kollias established the TNF-Tg model, which is also used in recent years in the study of RA due to its characteristic of 100% development of chronic erosive polyarthritis, with macroscopic and histologic symptoms very close to those of the human rheumatoid arthritis.Citation20 We found that 630 nm LED can alleviate the inflammatory progression of RA by inhibiting inflammatory pathways and other pathways in CIA model mice.Citation17,Citation18 In this study, we applied the TNF-Tg model for the first time to demonstrated the role of PBMT in the treatment of arthritis, the alteration of gut microbiota homeostasis, and the role of the gut-joint axis in mediating the pathogenesis and PBMT therapeutic effect on RA.
A set of RA-specific microbiota is present in both animal models and clinical patients during the process of RA. Clinical studies have shown that rheumatoid arthritis leads to a decrease in the diversity of gut microbiota, which is manifested at the level of the phylum by an increase in the abundance of Firmicutes, Proteobacteria and Tenericutes and a decrease in the abundance of Bacteroidetes. At the genus level, Prevotellaceae, Escherichia, Lachnospiraceae and Ruminococcaceae showed elevated abundance, whereas Bacteroidaceae, Clostridium, Bifidobacteria, and Lactobacillus decreased in abundance.Citation1,Citation2,Citation21–27 In TNF-Tg mice used in this study, elevated RA-associated inflammatory bacterial genera were similarly observed, suggesting that the TNF-Tg mice are a valid model for studying the gut microbiota of rheumatoid arthritis, and are able to respond to changes of clinical RA patients.
Glucocorticoids, non-steroidal anti-inflammatory drugs (NSAIDs), DMARDs and monoclonal antibodies targeting inflammatory factors are used in the clinical management of RA. The drugs restored the diversity of the gut microbiota in RA patients along with the relief of clinical symptoms, as evidenced by an increase in the abundance of Firmicutes, Lactobacillus, Odoribacter, Alistipes, Bifidobacteria, Lachnospiraceae and Clostridium and a decrease in the abundance of Veillonellaceae, Escherichia and Aerococcus. The correlation with clinical indicators suggests that these changes are associated with the symptoms of the disease and an improvement of treatment.Citation28–32 In comparison with the RA disease group, the changes of genera were restored to the level of the control group (Table S1). Taken together, the results suggest that changes of Clostridium, Escherichia, Lactobacillus, Aerococcus, Odoribacter, Alistipes and Bifidobacteria reflect the therapeutic effects of RA.
PBM refers to the cellular and metabolic responses of living cells to light energy, including electromagnetic radiation in the visible, near-infrared, and ultraviolet spectra.Citation18 PBM has been used in the treatment and rehabilitation of RA, and PBMT has emerged as a potential noninvasive and side-effect-free treatment method that can complement existing therapeutic strategies. In contrast to the side effects of RA therapeutic drugs, PBMT has been shown to control pain, inflammation, and immune regulation, and promote tissue regeneration.Citation33,Citation34 Research has demonstrated that PBM utilizing near-infrared light can boost the abundance of the probiotic Allobaculum in mice by nearly 10,000 times.Citation35 However, it remains unclear whether PBMT can exert its effects by regulating gut microbiota, despite recent studies indicating the potential of PBMT to improve the progression of Alzheimer’s disease by targeting the gut microbiota.Citation36
Our study also found that PBM increased the abundance of Allobaculum and restored the microbiota diversity in TNF-Tg mice. At the phylum level, PBM rectified gut microbiota dysbiosis by upregulating Verrucomicrobia and downregulating Actinobacteria and Tenericutes. At the genus level, the increase in Allobaculum, Clostridium, Odoribacter, Turicibacter, Lactobacillus and the decrease in Escherichia, Alistipes and Aerococcus. Among them, Alistipes, Clostridium, Odoribacter, and Lactobacillus were consistent with the changes in gut microbiota induced by RA therapeutic agents. Escherichia and Aerococcus were significantly altered after PBMT, which had not been reported in previous studies. The above results suggest that PBMT is capable of restoring the homeostasis of RA gut microbiota. Aerococcus is a gram-negative bacterium in gut.Citation37 In the osteoarthritis model, the abundance of Aerococcus was higher than that of the control, suggesting that Aerococcus may serve as a biomarker in osteoarthritis.Citation38 At the genus level, Odoribacter, a beneficial bacterium, is decreased in patients with RA. Odoribacter inhibits inflammation by producing butyrate.Citation39 However, this study did not observe a significant increase in butyrate levels after PBMT, suggesting that the production of butyrate may be influenced by the host environment and intervention strategies, and its effects may be exerted through other metabolites. Alistipes is a gram-negative bacterium associated with colorectal cancer and cardiovascular disease and plays a pivotal role in inflammation and disease.Citation40 Akkermansia increased in abundance after PBMT, and studies have shown that intestinal Akkermansia regulate L-aspartate metabolism via the gut-hepatic axis and ameliorate the disease progression of metabolic dysfunction-associated fatty liver.Citation41 In this study, we found an increase in the abundance of the gut microbiota metabolite N-acetyl aspartate after PBMT, suggesting that the amino acid metabolites of gut microbiota mediate the therapeutic effects of PBMT in rheumatoid arthritis as well as the mechanism of modulation of the gut-hepatic axis. Taken together, these findings suggest that the increase in Prevotella, Escherichia and Streptococcus, as well as the decrease in probiotics such as Clostridium_IV, Lactobacillus and Odoribacter, are the main drivers of pathogenesis and progression in RA. Lactobacillus, a host-friendly probiotic, has been shown to improve symptoms in an RA model by applying L. casei and L. delbrueckii.Citation42 Prevotella and Escherichia can induce the production of bacterial antibodies that accelerate the progression of RA.Citation43 In this study, PBMT increased the abundance of beneficial bacteria such as Clostridium_IV, Lactobacillus and Odoribacter. The therapeutic effect of drugs such as PBMT primarily operates by increasing the abundance of beneficial bacteria and indirectly regulating harmful bacteria. The anti-inflammatory and anti-RA effects of PBMT mediated by the gut-joint axis are same as the concept of “Fu zheng” in traditional Chinese medicine.
Large number of studies have shown, through 16S rDNA sequencing results, that some genus in RA different from those in WT and that homeostasis is restored after treatment. However, the effect of gut metabolites on RA treatment following changes in genus abundance has not been explored. Characteristic changes in microbiota metabolism in RA patients include increased bile acid and tryptophan biosynthesis as well as decreased unsaturated fatty acid biosynthesis.Citation6 These changes were also observed in TNF-Tg mice. Furthermore, amino acid biosynthesis was inhibited in TNF-Tg mice, consistent with the clinical fecal metabolomics of patients with RA.Citation43 The study found that PBMT can regulate the composition and metabolism of the gut microbiota, thereby indirectly improving the process of RA. Pearson correlation analysis of RA identified seven bacterial genera related to PBMT, including Alistipes, Allobaculum, Aerococcus, Clostridium_IV, Lactobacillus, Odoribacter, and Turicibacter. PICRUSt2 analysis revealed that PBMT mainly affected the gut microbiota by increasing amino acids (glutamate, aspartate, and alanine), thiamine metabolism, and antibiotic biosynthesis (streptomycin and cephalosporin C), and decreasing streptomycin biosynthesis. Our study found that ALA and NAA were upregulated, whereas glutamate and tryptophan were downregulated. Amino acids have been shown to inhibit the formation of inflammatory factors, modulate immune cell activity, and regulate inflammatory signaling pathways.Citation44 In this study, ALA and NAA inhibited inflammatory factors in human synovial cells by binding to upstream P65 protein and MMP9, thereby exerting an inhibitory effect in the process of RA. A significant correlation between ALA and tryptophan in the feces of Type II collagen induced arthritis (CIA) mice and RA has been observed.Citation45 ALA has anti-inflammatory properties, such as inhibiting expression of IL-8 induced by TNF-α in colon cancer cells.Citation46 Additionally, it can inhibit the activation of NF-κB, IL-6, and TNF-α during intestinal inflammation.Citation47 An increase in NAA was observed in this study, which can inhibit the activation of COX-2 protein and NF-κB in STTG cells stimulated by IL-1βCitation48. Alanine produced by the gut microbiota after FMT can significantly improve the survival rate of spinal cord injury mice with SCI.Citation49 In our study, we found that PBMT inhibited RA inflammation by promoting the synthesis of ALA and NAA. Glutamic and aspartic acids are acidic amino acids. Excess production of glutamic acid leads to severe neurotoxicity and accelerates the onset of neuroinflammation.Citation50 Excessive aspartic acid promotes IL-1β expression in macrophages and accelerates the inflammatory response.Citation51 Liu et al. observed upregulation of glutamic acid in the plasma of CIA mice.Citation52 Kim et al. observed an increase in aspartic acid and phosphocholine in the synovial fluid and gut metabolites of RA patients, suggesting that changes in amino acids may play a key role in the process of RA.Citation53 In our study, PBM irradiation resulted in downregulation of glutamic and aspartic acid biosynthesis by the gut microbiota, which might inhibit inflammation. Similarly, changes in metabolites, such as antibiotics, were observed to be involved in the regulation of microbiota; however, they were not further studied, which may be a limitation of this study.
Molecular docking is a method used to study intermolecular interactions.Citation54 In this study, molecular docking experiments confirmed that two amino acid metabolites could bind P65 protein and MMP upstream of inflammatory factors and exert an inhibitory effect on the inflammatory pathway and inflammatory mediators in RA. And the anti-inflammatory effects of alanine and N-acetyl aspartate were confirmed in TNF-Tg mice model and human synovial cells, respectively. These results demonstrated that ALA&NAA could effectively ameliorate the inflammatory progression of RA in the TNF-Tg mice. It is suggested that the metabolites of amino acid from gut microbiota mediate the gut-joint axis and PBMT therapeutic effect on rheumatoid arthritis.
In this study, we aim to investigate the potential anti-inflammatory effects of gut microbiota metabolites on target organs. Specifically, we evaluated the levels of metabolizing enzymes in the synovium using proteomic analysis. Our results demonstrated a decrease in the levels of alanine aminotransferase and aspartate aminotransferase after PBMT, suggesting a potential role for ALA and NAA in the synovium of mice. Previous studies have focused on the anti-inflammatory effects of SCFAs in relieving inflammatoryCitation55 but have not considered the effects of target organs on metabolites. The PICRUSt2 results revealed no significant changes in the unsaturated fatty acid biosynthesis pathway, indicating that SCFAs may not play an important role in RA during PBMT. Clinical studies have demonstrated that lipid deposition occurs in the joints of RA patients.Citation56 The content of fatty acid β-oxidation enzymes decreases, leading to an increase in synovial lipid synthesis.Citation57 Proteomic analysis revealed a decrease in the content of fatty acid β-oxidation enzymes, which increased after PBMT. This finding suggests that unsaturated fatty acids may not exert anti-inflammatory effects after PBMT, despite SCFAs being important anti-inflammatory metabolites. PBMT affects the expression of β-oxidation enzymes in the target organs, which causes SCFA consumption. Our findings suggest that different treatments have an impact on the metabolic enzymes of the target organs and affect the effects of metabolites. Given the influence of intervention mode, types of metabolites, and target organ metabolizing enzyme status on PBMT, it is necessary to evaluate the overall effect of PBMT and its different mechanisms of action.
This study revealed that PBMT not only modulates the inflammatory response of RA by adjusting the TNF signaling pathway but also through amino acid metabolites in the gut-joint axis. The gut-joint axis refers to the interaction between the gut and joints, which involves the transfer of inflammatory factors, neural connections, and metabolites such as SCFA and tryptophan from the gut to the joints. This concept has emerged from recent studies that gut microbiota can affect the regulation of inflammatory response, which has a potential impact on joint health. Dysbiosis of the gut microbiota causes damage to the intestinal barrier. Intestinal inflammation has been implicated in systemic inflammation and in accelerating the process of RA. This study provides new insights into the gut-joint axis, including the fact that RA can affect the gut-joint axis, and various interventions such as (PBMT) can improve gut microbiota dysbiosis through the gut-joint axis. Gut metabolites can be involved in the metabolism of target organs and are simultaneously affected by target organ metabolic enzymes at the same time. We provided a basis for selecting appropriate metabolites for RA treatment and efficacy evaluation.
Conclusion
Our study demonstrated that the gut-joint axis is involved in regulating the TNF-induced RA process and the rehabilitative effect of PBMT. The efficacy of PBMT was manifested by the formation of a new homeostasis of the gut microbiota, including an increase in the abundance of beneficial bacteria, Clostridium_IV, Lactobacillus, and Odoribacter, and an enhancement of the stability of the symbiotic network. Amino acid metabolites of the gut microbiota are the main components of the PBMT-regulated RA gut-joint axis, and the effects of the gut-joint axis are influenced by the mode of intervention, the type of metabolites, and the activity of metabolic enzymes in target organs.
Highlights
The gut-joint axis mediates the pathogenesis of TNF-α induced rheumatoid arthritis and the PBMT effects on RA.
The amino acid are main metabolites of gut microbiota for regulating of PBMT effects on RA.
The role of gut microbiota metabolites in the gut-joint axis was determined by the level of metabolic enzymes in the joint.
The effect of PBMT is mainly recovery of microbiota homeostasis, which characterized by the increase of Clostridium_IV, Lactobacillus and Odoribacter.
Authors’ contributions
Q.M., M.L., and W.S. contributed equally to this study. Q.M., M.L., and W.S. Conceptualization, Data curation, Formal analysis, Methodology, Visualization, writing – original draft. J.W., Y.Y., and W.G. Methodology, Resources, Writing – review & editing. P.H., G.C., and Z.S. performed the experiments; P.X., X.D. and H.L. analyzed the data; and all authors approved the final version to be published. F.Z. and H.L. Conceptualization, Funding acquisition, Investigation, Methodology, Resources, Writing – review & editing.
Supplemental Material
Download Zip (1.1 MB)Disclosure statement
No potential conflict of interest was reported by the authors.
Data availability statement
All data generated or analyzed during the current study are included in this published article [and supplementary information files] or are available in the NCBI SRA repository, PRJNA1044260.
Supplementary material
Supplemental data for this article can be accessed online at https://doi.org/10.1080/19490976.2023.2281382
Additional information
Funding
References
- Alpizar-Rodriguez D, Lesker TR, Gronow A, Gilbert B, Raemy E, Lamacchia C, Gabay C, Finckh A, Strowig T. Prevotella copri in individuals at risk for rheumatoid arthritis. Ann Rheum Dis. 2019 May;78(5):590–22. doi:10.1136/annrheumdis-2018-214514.
- Scher JU, Sczesnak A, Longman RS, Segata N, Ubeda C, Bielski C, Rostron T, Cerundolo V, Pamer EG, Abramson SB, et al. Expansion of intestinal Prevotella copri correlates with enhanced susceptibility to arthritis. Elife. 2013 Nov 5;2:e01202. doi:10.7554/eLife.01202.
- Hvatum M, Kanerud L, Hällgren R, Brandtzaeg, Per. The gut-joint axis: cross reactive food antibodies in rheumatoid arthritis. Gut. 2006 Sep;55(9):1240–1247. doi:10.1136/gut.2005.076901.
- Zaiss MM, Joyce Wu HJ, Mauro D, Schett G, Ciccia F. The gut–joint axis in rheumatoid arthritis. Nat Rev Rheumatol. 2021 Apr;17(4):224–237. doi:10.1038/s41584-021-00585-3.
- Horta-Baas G, Sandoval-Cabrera A, Romero-Figueroa MDS. Modification of gut microbiota in inflammatory arthritis: highlights and future challenges. Curr Rheumatol Rep. 2021 Jul 3;23(8):67. doi:10.1007/s11926-021-01031-9.
- Xu X, Wang M, Wang Z, Chen Q, Chen X, Xu Y, Dai M, Wu B, Li Y. The bridge of the gut–joint axis: gut microbial metabolites in rheumatoid arthritis. Front Immunol. 2022;13:1007610. doi:10.3389/fimmu.2022.1007610.
- Chu Y, Sun S, Huang Y, Gao Q, Xie X, Wang P, Li J, Liang L, He X, Jiang Y, et al. Metagenomic analysis revealed the potential role of gut microbiome in gout. NPJ Biofilms Microbio. 2021 Aug 9;7(1):66. doi:10.1038/s41522-021-00235-2.
- Wood KD, Freeman BL, Killian ME, Lai WS, Assimos D, Knight J, Fargue S. Effect of alanine supplementation on oxalate synthesis. Biochimica et Biophysica Acta (BBA) - Molecular Basis of Disease. 2021 Jan 1;1867(1):165981. doi:10.1016/j.bbadis.2020.165981.
- Kimura-Ohba S, Asaka MN, Utsumi D, Takabatake Y, Takahashi A, Yasutomi Y, Isaka Y, Kimura T. D-alanine as a biomarker and a therapeutic option for severe influenza virus infection and COVID-19. Biochim Biophys Acta Mol Basis Dis. 2023 Jan 1;1869(1):166584. doi:10.1016/j.bbadis.2022.166584.
- Hofer DC, Zirkovits G, Pelzmann HJ, Huber K, Pessentheiner AR, Xia W, Uno K, Miyazaki T, Kon K, Tsuneki H, et al. N-acetylaspartate availability is essential for juvenile survival on fat-free diet and determines metabolic health. FASEB J. 2019 Dec;33(12):13808–13824. doi:10.1096/fj.201801323R.
- Bonnefoy F, Daoui A, Valmary-Degano S, Toussirot E, Saas P, Perruche S. Apoptotic cell infusion treats ongoing collagen-induced arthritis, even in the presence of methotrexate, and is synergic with anti-TNF therapy. Arthritis Res Ther. 2016 Aug 11;18(1):184. doi:10.1186/s13075-016-1084-0.
- Gevers D, Kugathasan S, Denson LA, Vázquez-Baeza Y, Van Treuren W, Ren B, Schwager E, Knights D, Song S, Yassour M, et al. The treatment-naive microbiome in new-onset crohn’s disease. Cell Host & Microbe. 2014 Mar 12;15(3):382–392. doi:10.1016/j.chom.2014.02.005.
- Morris GM, Huey R, Lindstrom W, Sanner MF, Belew RK, Goodsell DS, Olson AJ. AutoDock4 and AutoDockTools4: Automated docking with selective receptor flexibility. J Comput Chem. 2009 Dec;30(16):2785–2791. doi:10.1002/jcc.21256.
- Jiménez J, Doerr S, Martínez-Rosell G, Rose AS, De Fabritiis G. DeepSite: protein-binding site predictor using 3D-convolutional neural networks. Bioinformatics. 2017 Oct 1;33(19):3036–3042. doi:10.1093/bioinformatics/btx350.
- Trott O, Olson AJ. AutoDock Vina: improving the speed and accuracy of docking with a new scoring function, efficient optimization, and multithreading. J Comput Chem. 2010 Jan 30;31(2):455–461. doi:10.1002/jcc.21334.
- van Loo G, Bertrand MJM. Death by TNF: a road to inflammation. Nat Rev Immunol. 2023 May;23(5):289–303. doi: 10.1038/s41577-022-00792-3
- Song W, Zhang H, Pan Y, Xia Q, Liu Q, Wu H, Du S, Zhang F, Liu H. LED irradiation at 630 nm alleviates collagen-induced arthritis in mice by inhibition of NF-κB-mediated MMPs production. Photochem Photobiol Sci. 2023 Oct;22(10):2271–2283. doi:10.1007/s43630-023-00449-7.
- Li Y, Wei S, Zhang K, Fang Y, Liu H, Jin Z, Guo Q, He J, Song W, Zhang F, et al. The inflammation and reactive oxygen species regulated by Nrf2 and NF-κB signaling pathways in 630-nm light-emitting diode irradiation treated THP-1 monocytes/macrophages. Lasers Med Sci. 2021 Sep;36(7):1411–1419. doi:10.1007/s10103-020-03172-2.
- Brand DD, Kang AH, Rosloniec EF. The mouse model of collagen-induced arthritis. Methods Mol Med. 2004;102:295–312. doi:10.1385/1-59259-805-6:295.
- Kollias G, Douni E, Kassiotis G, Kontoyiannis D. On the role of tumor necrosis factor and receptors in models of multiorgan failure, rheumatoid arthritis, multiple sclerosis and inflammatory bowel disease. Immunol Rev. 1999 Jun;169(1):175–194. doi:10.1111/j.1600-065X.1999.tb01315.x.
- Li ZD, Qi FY, Li F. Integrating 16S sequencing and metabolomics study on anti-rheumatic mechanisms against collagen-induced arthritis of Wantong Jingu Tablet. Chin J Nat Med. 2022 Feb;20(2):120–132. doi:10.1016/S1875-5364(21)60080-8.
- Sun Y, Chen Q, Lin P, Xu R, He D, Ji W, Bian Y, Shen Y, Li Q, Liu C, et al. Characteristics of gut microbiota in patients with rheumatoid arthritis in Shanghai, China. Front Cell Infect Microbiol. 2019 Oct 23;9:369. doi:10.3389/fcimb.2019.00369.
- Chen J, Wright K, Davis JM, Jeraldo P, Marietta EV, Murray J, Nelson H, Matteson EL, Taneja V. An expansion of rare lineage intestinal microbes characterizes rheumatoid arthritis. Genome Med. 2016 Apr 21;8(1):43. doi:10.1186/s13073-016-0299-7.
- Zhang X, Zhang D, Jia H, Feng Q, Wang D, Liang D, Wu X, Li J, Tang L, Li Y, et al. The oral and gut microbiomes are perturbed in rheumatoid arthritis and partly normalized after treatment. Nat Med. 2015 Aug;21(8):895–905. doi:10.1038/nm.3914.
- Vaahtovuo J, Munukka E, Korkeamäki M, Luukkainen R, Toivanen P. Fecal microbiota in early rheumatoid arthritis. J Rheumatol. 2008 Aug;35(8):1500–1505. Epub 2008 Jun 1. PMID: 18528968.
- Breban M, Tap J, Leboime A, Said-Nahal R, Langella P, Chiocchia G, Furet J-P, Sokol H. Faecal microbiota study reveals specific dysbiosis in spondyloarthritis. Ann Rheum Dis. 2017 Sep;76(9):1614–1622. doi:10.1136/annrheumdis-2016-211064.
- Kishikawa T, Maeda Y, Nii T, Motooka D, Matsumoto Y, Matsushita M, Matsuoka H, Yoshimura M, Kawada S, Teshigawara S, et al. Metagenome-wide association study of gut microbiome revealed novel aetiology of rheumatoid arthritis in the Japanese population. Ann Rheum Dis. 2020 Jan;79(1):103–111. doi:10.1136/annrheumdis-2019-215743.
- Rodrigues GSP, Cayres LCF, Gonçalves FP, Fernanda T. Detection of increased relative expression units of bacteroides and Prevotella, and decreased Clostridium leptum in stool samples from Brazilian rheumatoid arthritis patients: a pilot study. Microorganisms. 2019 Oct 1;7(10):413. doi:10.3390/microorganisms7100413.
- Kanerud L, Scheynius A, Nord CE, Hafström I. Effect of sulphasalazine on gastrointestinal microflora and on mucosal heat shock protein expression in patients with rheumatoid arthritis. Br J Rheumatol. 1994 Nov;33(11):1039–1048. doi:10.1093/rheumatology/33.11.1039.
- Picchianti-Diamanti A, Panebianco C, Salemi S, Sorgi M, Di Rosa R, Tropea A, Sgrulletti M, Salerno G, Terracciano F, D’Amelio R, et al. Analysis of gut microbiota in rheumatoid arthritis patients: disease-related dysbiosis and modifications induced by etanercept. Int J Mol Sci. 2018 Sep 27;19(10):2938. doi:10.3390/ijms19102938.
- Maeda Y, Matsushita M, Yura A, Teshigawara S, Katayama M, Yoshimura M, Watanabe A, Tanaka E, Tsuji S, Kitatobe A, et al. OP0191 the fecal microbiota of rheumatoid arthritis patients differs from that of healthy volunteers and is considerably altered by treatment with biologics. Ann Rheum Dis. 2013;72(Suppl 3):A117–A117. doi:10.1136/annrheumdis-2013-eular.396.
- Bungau SG, Behl T, Singh A, Sehgal A, Singh S, Chigurupati S, Vijayabalan S, Das S, Palanimuthu VR. Targeting probiotics in rheumatoid arthritis. Nutrients. 2021 Sep 26;13(10):3376. doi:10.3390/nu13103376.
- Ailioaie C, Lupusoru-Ailioaie LM. Beneficial effcts of laser therapy in the early stages of rheumatoid arthritis onset. Laser Therapy. 1999;11(2):79–87. doi:10.5978/islsm.11.79.
- Salehpour F, Rasta SH. The potential of transcranial photobiomodulation therapy for treatment of major depressive disorder. Rev Neurosci. 2017 May 24;28(4):441–453. doi:10.1515/revneuro-2016-0087.
- Bicknell B, Liebert A, Johnstone D, Kiat H. Photobiomodulation of the microbiome: implications for metabolic and inflammatory diseases. Lasers Med Sci. 2019 Mar;34(2):317–327. doi:10.1007/s10103-018-2594-6.
- Chen Q, Wu J, Dong X, Yin H, Shi X, Su S, Che B, Li Y, Yang J. Gut flora-targeted photobiomodulation therapy improves senile dementia in an aß-induced Alzheimer’s disease animal model. J Photochem Photobiol B. 2021 Mar;216:112152. doi:10.1016/j.jphotobiol.2021.112152.
- Everard A, Matamoros S, Geurts L, Delzenne NM, Cani PD. Saccharomyces boulardii administration changes gut microbiota and reduces hepatic steatosis, low-grade inflammation, and fat mass in obese and type 2 diabetic db/db mice. mBio. 2014 Jun 10;5(3):e01011–14. doi:10.1128/mBio.01011-14.
- Yan Y, Yi X, Duan Y, Jiang B, Huang T, Inglis BM, Zheng B, Si W. Alteration of the gut microbiota in rhesus monkey with spontaneous osteoarthritis. BMC Microbiol. 2021 Nov 27;21(1):328. doi:10.1186/s12866-021-02390-0.
- He J, Chu Y, Li J, Meng Q, Liu Y, Jin J, Wang Y, Wang J, Huang B, Shi L, et al. Intestinal butyrate-metabolizing species contribute to autoantibody production and bone erosion in rheumatoid arthritis. Sci Adv. 2022 Feb 11;8(6):eabm1511. doi:10.1126/sciadv.abm1511.
- Parker BJ, Wearsch PA, Veloo ACM, Rodriguez-Palacios A. The genus Alistipes: gut bacteria with emerging implications to inflammation, cancer, and mental health. Front Immunol. 2020 Jun 9;11:906. doi:10.3389/fimmu.2020.00906.
- Rao Y, Kuang Z, Li C, Guo S, Xu Y, Zhao D, Hu Y, Song B, Jiang Z, Ge Z, et al. Gut Akkermansia muciniphila ameliorates metabolic dysfunction-associated fatty liver disease by regulating the metabolism of L-aspartate via gut-liver axis. Gut Microbes. 2021 Jan-Dec;13(1):1–19. doi:10.1080/19490976.2021.1927633.
- Kano H, Kaneko T, Kaminogawa S. Oral intake of Lactobacillus delbrueckii subsp. bulgaricus OLL1073R-1 prevents collagen-induced arthritis in mice. J Food Protection. 2002 Jan;65(1):153–160. doi:10.4315/0362-028X-65.1.153.
- Yu D, Du J, Pu X, Zheng L, Chen S, Wang N, Li J, Chen S, Pan S, Shen B, et al. The gut microbiome and metabolites are altered and interrelated in patients with rheumatoid arthritis. Front Cell Infect Microbiol. 2021 Jan 25;11:763507. doi:10.3389/fcimb.2021.763507.
- He F, Wu C, Li P, Li N, Zhang D, Zhu Q, Ren W, Peng Y. Functions and signaling pathways of amino acids in intestinal inflammation. Biomed Res Int. 2018;2018:1–13. doi:10.1155/2018/9171905.
- Shan J, Peng L, Qian W, Xie T, Kang A, Gao B, Di L. Integrated serum and fecal metabolomics study of collagen-induced arthritis rats and the therapeutic effects of the zushima tablet. Front Pharmacol. 2018 Aug;9:891. doi:10.3389/fphar.2018.00891.
- Li P, Yin YL, Li D, Woo Kim S, Wu G. Amino acids and immune function. Br J Nutr. 2007 Aug 3;98(2):237–252. doi:10.1017/S000711450769936X.
- Raspé C, Czeslick E, Weimann A, Schinke C, Leimert A, Kellner P, Simm A, Bucher M, Sablotzki A. Glutamine and alanine-induced differential expression of intracellular IL-6, IL-8, and TNF-α in LPS-stimulated monocytes in human whole-blood. Cytokine. 2013 Apr;62(1):52–57. doi:10.1016/j.cyto.2013.02.020.
- Rael LT, Thomas GW, Bar-Or R, Craun ML, Bar-Or D. An anti-inflammatory role for N-acetyl aspartate in stimulated human astroglial cells. Biochem Biophys Res Commun. 2004 Jul 2;319(3):847–853. doi:10.1016/j.bbrc.2004.04.200.
- Jing Y, Bai F, Wang L, Yang D, Yan Y, Wang Q, Zhu Y, Yu Y, Chen Z, et al. Fecal microbiota transplantation exerts neuroprotective effects in a mouse spinal cord injury model by modulating the microenvironment at the lesion site. Microbiol Spectr. 2022 Jun 29;10(3):e0017722. doi:10.1128/spectrum.00177-22.
- Haroon E, Miller AH, Sanacora G. Inflammation, glutamate, and Glia: a trio of trouble in mood disorders. Neuropsychopharmacology. 2017 Jan;42(1):193–215. doi:10.1038/npp.2016.199.
- Wang H, Zheng X, Liu B, Xia Y, Xin Z, Deng B, He L, Deng J, Ren W. Aspartate metabolism facilitates IL-1β production in inflammatory macrophages. Front Immunol. 2021 Oct 21;12:753092. doi:10.3389/fimmu.2021.753092.
- Liu Y, Xie Y. Metabolomics approach to the exploration of amino acids metabolism changes associated with disease progression in a rat model of adjuvant-induced arthritis. J Environ Pathol Toxicol Oncol. 2021;40(4):43–52. doi:10.1615/JEnvironPatholToxicolOncol.2021039409.
- Kim S, Hwang J, Xuan J, Jung YH, Cha H-S, Kim KH. Global metabolite profiling of synovial fluid for the specific diagnosis of rheumatoid arthritis from other inflammatory arthritis. PloS One. 2014 Jun 2;9(6):e97501. doi:10.1371/journal.pone.0097501.
- Pinzi L, Rastelli G. Molecular docking: shifting paradigms in drug discovery. Int J Mol Sci. 2019 Sep 4;20(18):4331. doi:10.3390/ijms20184331.
- Yao Y, Cai X, Fei W, Ye Y, Zhao M, Zheng C. The role of short-chain fatty acids in immunity, inflammation and metabolism. Crit Rev Food Sci Nutr. 2022;62(1):1–12. doi:10.1080/10408398.2020.1854675.
- Song IH, Hermann KG, Haibel H, Althoff CE, Poddubnyy D, Listing J, Weiß A, Freundlich B, Lange E, Rudwaleit M, et al. Inflammatory and fatty lesions in the spine and sacroiliac joints on whole-body MRI in early axial spondyloarthritis—3-Year data of the ESTHER trial. Semin Arthritis Rheum. 2016 Feb;45(4):404–410. doi:10.1016/j.semarthrit.2015.08.005.
- Olsen RK, Pourfarzam M, Morris AA, Dias RC, Knudsen I, Andresen BS, Gregersen N, Olpin SE. Lipid-storage myopathy and respiratory insufficiency due to ETFQO mutations in a patient with late-onset multiple acyl-CoA dehydrogenation deficiency. J Inherit Metab Dis. 2004;27(5):671–678. doi:10.1023/B:BOLI.0000042986.10291.e9.