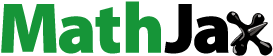
ABSTRACT
Bifidobacterium is a widely distributed commensal bacterial genus that displays beneficial pro-homeostatic and anti-inflammatory immunomodulatory properties. Depletion or absence of Bifidobacterium in humans and model organisms is associated with autoimmune responses and impaired immune homeostasis. At the cellular level, Bifidobacterium upregulates suppressive regulatory T cells, maintains intestinal barrier function, modulates dendritic cell and macrophage activity, and dampens intestinal Th2 and Th17 programs. While there has been a large volume of literature characterizing the probiotic properties of various Bifidobacterial species, the likely multifactorial mechanisms underlying these effects remain elusive, in particular, its immune tolerogenic effect. However, recent work has shed light on Bifidobacterium surface structural polysaccharide and protein elements, as well as its metabolic products, as commensal mediators of immune homeostasis. This review aims to discuss several mechanisms Bifidobacterium utilizes for immune modulation as well as their indirect impact on the regulation of gut microbiome structure and function, from structural molecules to produced metabolites. These mechanisms are pertinent to an increasingly networked understanding of immune tolerance and homeostasis in health and disease.
PART I. Overview of Bifidobacterium immune modulatory abilities
Bifidobacterium is a gram-positive, anaerobic, saccharoclastic, non-motile commensal bacterial genus. It contains 10 phylogenetic clusters and has a broad host range within the mammalian gastrointestinal tract.Citation1,Citation2 In vitro culture of Bifidobacteria require highly specific conditions given their requirement for an anaerobic environment and metabolic precussors.Citation3,Citation4 Bifidobacterium exhibits genomic differences that partly reflect the gene acquisition events required to thrive in different host ecological niches. Bifidobacterium tropism and niche adaption underpin its diverse role in host immune regulation.
Bifidobacterium has been implicated as an immunomodulator or biomarker of human disease, serving as both a driver and protector. Various strains are commonly used as live biotherapeuticsCitation5 and demonstrate beneficial immunomodulatory and anti-inflammatory properties. These include upregulation of suppressive Foxp3+ regulatory T cells (Tregs),Citation6 improvement of intestinal barrier function,Citation7 and dampening of intestinal Th2 and Th17 programs.Citation8 On the other hand, absence or reduction of Bifidobacterium species has been implicated in multiple autoimmune and autoinflammatory conditions in humans. For instance, decreased gut levels of several Bifidobacterium species in humans are associated with the microbiome fingerprint of treatment-naïve Crohn’s disease (CD).Citation9 Decreased levels of gut Bifidobacterium infantis are correlated with Guillain-Barré Syndrome in humans.Citation10 Mice treated with B. pseudolongum ATCC25526 and receiving allogeneic heterotopic heart transplants with long-term immunosuppression display improved long-term graft survival and decreased allograft inflammation.Citation11 Contrary to its classical protective role, enrichment of Bifidobacterium is also associated with Parkinson’s disease in a meta-analysis of patient gut microbiome data.Citation12 Similarly, Bifidobacterium is enriched in the stool of patients with ulcerative colitis (UC) compared to healthy controls.Citation13 These results suggest that Bifidobacteria possess both multifactorial immunomodulatory mechanisms and diverse strain-mediated immune effects.
Bifidobacteria also modulate immune responses at the level of the gut mucosa. In mice treated with Bifidobacterium adolescentis ATCC15703, the levels of pro-inflammatory cytokines TNFα, IL-6, IL- 1β, IL-18, IL-22, and IL-9 in colon homogenates are lower than controls,Citation14 while anti-inflammatory IL-10 and Th2-type cytokines IL-4 and IL-5 are higher. Tregs are also increased in the colons of colitic mice receiving B. adolescentis ATCC15703. Similarly, germ-free mice colonized with Bifidobacterium bifidum strain PRI1 have increased Tregs in the colonic lamina propria.Citation6 This effect is facilitated by colon lamina propria dendritic cells (DCs), which have increased mRNA expression of IL-10, GM-CSF, TGFβ1, Indoleamine 2,3-dioxygenase, PTGS2, and PD-1, as well as the co-stimulatory molecules CD86 and CD40 after treatment with PRI1. In vitro treatment of DCs with PRI1 followed by co-culture with naïve CD4 T cells leads to enhanced Treg induction and IL-10 production.Citation6
Bifidobacterium immunomodulatory programs vary in phenotype and intensity at the species and strain levels, including pro-inflammatory effects.Citation15 The immunomodulatory effect of Bifidobacterium was shown to be independent of its phylogeny.Citation16 Bone marrow-derived DCs co-cultured with Bifidobacterium animalis subsp. lactis 5764 (Bl 5764) display high levels of maturation and costimulatory molecules CD40, CD86, and MHC II in comparison to non-stimulated cells. When pre-treated DCs are co-cultured with naïve CD4 T cells, there is increased pro-inflammatory IL-17A and IL-17F production compared to control and groups treated with different bacterial species.Citation17 Co-culture of the THP-1 human monocyte cell line with Bifidobacterium breve strains UCC2003 and JCM7017 results in MyD88-dependent NFκB and TNF expression compared to untreated controls.Citation18 Murine bone marrow derived macrophages express increased TNFα after co-culture with these B. breve strains, favoring an anti-inflammatory phenotype. While this co-culture model provides a controlled environment to investigate potential interactions between Bifidobacterium and immune cells, the findings require careful interpretation and further validation using more sophisticated ex vivo or in vivo models. Strain-specific immunomodulation is likely rooted in niche adaption due to the different mammalian gut microenvironments from which each strain has been isolated. Thus, it is important to understand the properties of specific Bifidobacterium strains when planning for therapeutic application.
PART II. Bifidobacterium modulates immune responses by affecting gut microbiome
Microbial species interact through the metabolites they consume and secrete, a process known as cross-feeding. Bifidobacteria play an essential role in producing metabolites utilized by other genera and even among different species and strains of Bifidobacterium.Citation19–26 For example, Anaerostipes caccae L1–92 is an important butyrate producer that relies on metabolites produced by Bifidobacterium in order to establish itself in the infant gut. A. caccae L1–92 is unable to grow in monoculture, but co-culture with B. infantis ATCC15697 enables A. caccae L1–92 growth, which utilizes glucose and galactose along with the acetate generated by B. infantis ATCC15697 to produce butyrate.Citation20 Faecalibacterium prausnitzii S3/L3 or A2–165 and B. adolescentis L2–32;F. prausnitzii A2–165 or ATCC 27,768 and B. catenulatum KCTC 3221; as well as Eubacterium rectale, ATCC 33,656, and B. longum NCC2705 share similar cross-feeding relationships. In these co-cultures, Bifidobacterium is necessary for either the establishment of a butyrate producer or for enhancing butyrate production through the metabolism of human milk oligosaccharides (HMOs) into monosaccharides and the production of acetate.Citation19,Citation21,Citation22
This butyrogenic effect also results from the cross-feeding interaction between Bifidobacteria and Clostridiales, which negatively correlates with inflammatory bowel disorders.Citation19,Citation21,Citation27–29 Short-chain fatty acids (SCFAs), particularly butyrate, are important for colonic Treg homeostasis.Citation30,Citation31 Singh et al. demonstrated the role of butyrate and niacin in the suppression of colonic inflammation and carcinogenesis through the activation of Gpr109a. This stimulated DCs and macrophages to produce IL-10, leading to enhanced differentiation of Tregs.Citation32 Furthermore, co-culture of F. prausnitzii A2–165 or ATCC 27,768 and B. catenulatum KCTC 3221 results in the reduction of pro-inflammatory cytokines produced by HT-29 human colorectal adenocarcinoma cells and RAW 264.7 murine macrophages in vitro as well as decreased IL-8 in the colons of colitic mouse models.Citation22 These studies indicate the important role of Bifidobacterium metabolism in modulating immune homeostasis directly or via cross-feeding. Furthermore, Bifidobacterium does not possess polyamine biosynthetic machinery for putrescene, a known immunomodulator, but administration of B. animalis subsp. lactis LKM512 increases the concentration of luminal putrescene by sufficiently acidifying the intestine and allowing for activation of polyamine biosynthesis by endogenous gut microbiota.Citation33
From a cooperativity standpoint, Bifidobacteria can also negatively regulate the presence of pro-inflammatory metabolites such as trimethylamine N-oxide (TMAO), derived from gut microbiota-produced choline, which is associated with the development of atherosclerosis.Citation34 Supplementation with B. breve Bb4 as well as B. longum BL1 and BL7 decreases plasma TMAO levels in mice.Citation35 These pathways exemplify the complex interactions between different Bifidobacterium species and the host intestinal environment, including available carbohydrate nutrient sources and other members of the colonic microbiota, and their subsequent downstream effects on host immune responses.
Cross-feeding relationships also exist among various species of Bifidobacterium (), which subsequently affect metabolic production and immune properties. Different Bifidobacterium strains within the same species play different cross-feeding roles as well. B. breve UCC200 lacks the enzymes necessary for mucin degradation. Yet it proliferates to a greater extent in mucin-based media, similar to the human colon, when co-cultured with mucin-degrading B. bifidum PRL2010.Citation23 Isolated B. pseudocatelatum strains LH9, LH11, LH13, LH14 that express fucosidase support the growth of B. longum strain LH12, which cannot degrade fucosylated HMOs.Citation24 B. bifidum PRL2010 cannot grow in monoculture with xylan or starch-supplemented media, but grows in the same media when co-cultured with B. adolescentis 22 L, B. breve 12 L, or B. thermophilum JCM 1207.Citation25 When co-cultured, B. magnum and B. cuniculi proliferate in starch-supplemented media, whereas co-culture with xylan results in B. magnum modulation of xylan-degrading genes, which supports B. cuniculi.Citation26 This mutualism highlights the intricate relationships between various bacterial species in the gut microbiome and demonstrates that these direct and indirect interactions are all critical for determining the overall environment for immune stimulation versus homeostasis. The diverse applications of Bifidobacterium as a live biotherapeutic are contingent upon an array of strain-specific traits as well as interactions within the host milieu. This encompasses the interplay between host and gut microbiota, metabolic functionalities, adherence to intestinal epithelial cells, resilience to gastric acids and bile, immunomodulatory capacity, and competitive antagonism with pathogenic bacteria. Consequently, the functional dynamics of individual Bifidobacterium strains emerge from a multifaceted interplay of these distinct attributes.
Table 1. Cross-feeding relationships between Bifidobacterium and other microbiota species.
PART III. Bifidobacterium promotes a tolerogenic environment in various disease states
Solid organ transplant
Given its role as a marker and inducer of anti-inflammatory and pro-tolerogenic immune effects, Bifidobacterium has been studied in solid organ transplant models. Mice receiving allogeneic heart transplants and Bifidobacterium pseudolongum ATCC25526 gavage along with daily tacrolimus immunosuppression display improved long-term allograft survival and decreased graft inflammation.Citation36 Furthermore, treatment with B. pseudolongum ATCC25526 results in lymph node (LN) architectural changes, increasing the ratio between extracellular matrix glycoproteins laminin α4 and laminin α5, which is associated with tolerance.Citation37 Treatment with Bifidobacteria also promotes intestinal homeostasis following transplantation. In a rat model of liver transplant, prolonged antibiotic use and semi-starvation for 4–5 weeks (associated with decreased ileocecal Bifidobacterium), and supplementation with Bifidobacterium and Lactobacillus-containing probiotic promotes partial restoration of intestinal microflora and improved intestinal barrier function.Citation38 In a separate rat model of liver ischemia reperfusion injury, treatment with Bifidobacterium catenulatum ZYB0401 decreases serum TNFα and liver malondialdehyde and increases liver superoxide dismutase, which is associated with reduced liver injury.Citation39 Rats administered B. longum-containing probiotic cocktail followed by liver transplant without immunosuppression display increased intestinal Treg cells and TGFβ in the serum and liver, with concomitant decreases in CD4/CD8 T cell ratios and serum IL-2.Citation40
Exemplifying the dysbiotic state associated with immunosuppressant treatment, metagenomic analyses of human liver transplant recipients reveal deficits in beneficial Bifidobacteriaceae compared to healthy controls.Citation41,Citation42 Similarly, in renal transplant recipients, the gut microbiome displays a decreased abundance of multiple Bifidobacterium speciesCitation43. However, the presence of the family Bifidobacteriaceae in the gut microbiomes of liver transplant recipients is associated with acute cellular rejection.Citation44 As the family includes a large variety of species and strains that can have distinct immune modulatory properties, it is important to have species- and even strain-level taxonomic resolution to assist in characterizing the immunomodulatory effects of specific microbiota species and strains ().
Table 2. Bifidobacterium association with disease states and models.
DSS colitis
Various species and strains of Bifidobacterium are linked to the downmodulation of dextran sodium sulfate (DSS)-induced colitis in mice, including B. pseudocatenulatum MY40C and CCFM680,Citation45 B. infantis CGMCC0460.1,Citation46 B. animalis subsp. lactis XLTG11,Citation47 and B. breve M1, M2, M3, and M4.Citation48 DSS is a water-soluble, negatively charged polysaccharide that causes intestinal damage and inflammation, closely resembling UC in humans. Colitic mice treated with B. infantis CGMCC0460.1 or Bifidobacterium animalis subsp. lactis XLTG11 show a reduction in disease as assessed by weight loss, colon length, histologic tissue damage, myeloperoxidase activity, gut permeability, spleen weight, and disease activity index.Citation46,Citation47 Mechanistically, B. pseudocatenulatum MY40C and CCFM680, B. infantis CGMCC0460.1, B. animalis subsp. lactis XLTG11, and B. breve M1 and M2 but not M3 and M4 treatment results in upregulation of tight junction and adherens junction proteins (β-catenin, claudin-3, occludin, and ZO-1) along with increased barrier modifying mucin 2, IL-10, and PPARγ, while downregulating pro-inflammatory TNFα and IL-6.Citation45–48 B. pseudocatenulatum MY40C and CCFM680 and B. animalis subsp. lactis XLTG11 also downregulate the TLR4/NFκB pathway in colitic animals, reducing immune responsiveness to gut microbes and shifting the host immune state from inflammation to homeostasis.Citation45,Citation47 Although the mechanism underlying these effects remains poorly understood, production of colonic conjugated linoleic acid, an anti-inflammatory polyunsaturated fatty acid, also increased in mice treated with B. pseudocatenulatum MY40C and CCFM680 or B. breve M1, M2, M3, and M4.Citation45,Citation48 Additionally, treatment of DSS-induced colitic mice with a mixture of Bifidobacterium species (B. bifidum, B. longum, B. lactis, and B. breve) results in an altered host gut microbiome in a Treg dependent fashion.Citation49 Bifidobacterium treatment also resulted in enhanced Treg function via promotion of a self-stimulatory IL-10/IL-10 Rα loop and upregulation of mitochondrial activity. Of the Bifidobacterium mixture, B. breve specifically ameliorates CTLA-4 blockade-induced colitis, also via enhanced Treg function ().
Gliadin-induced enteropathy
Bifidobacteria protect intestinal epithelial cells from damage in a gliadin-induced enteropathy (GIE) model of celiac disease, in which incomplete hydrolysis of dietary proteins leads to small intestinal inflammation, lymphocyte infiltration, villous atrophy, and crypt hyperplasia.Citation50 Specifically, treatment of mice with B. longum CECT 7347 partially suppresses disease by inhibiting the production of inflammatory cytokines and CD4 T cell mediated immune responses. Similar anti-inflammatory effects are observed with B. longum ES1 and B. bifidum ES2 in vitro.Citation53 While B. longum CECT 7347 restores intestinal structure without reversing cellular infiltration,Citation50 B. longum NCC2705 treatment prevents intraepithelial infiltration of lymphocytes in mice sensitized with gliadin.Citation52 Further, in culture with epithelial cells, B. lactis counteracts the gliadin-induced permeability of intestinal epithelium, inhibited membrane ruffle formation, and protected tight junctions.Citation54 While GIE leads to decreased NFκB and increased TNFα and IL-10 expression, B. longum CECT 7347 treatment restores baseline NFκB and IL-10 levels, but further increases TNFα.Citation50 B. longum CECT 7347 treatment also modulates T cell differentiation by reducing overall CD4 and Treg populations while increasing CD8 T cells.Citation50 In another study, B. longum CECT 7347 co-administration with gliadin to IFNγ-sensitized mice results in upregulated stress and intestinal absorption proteins along with downregulated cellular homeostasis proteins involved in cytoskeletal organization, protein transport, gene transcription, retinoic acid binding, and cell starvation.Citation51 These observations reinforce the notion that the effects of Bifidobacterium treatment are pleiotropic and context dependent ().
Experimental autoimmune myasthenia gravis
Administration of Bifidobacterium, alone or in combination with other probiotics, ameliorates symptoms (clinical score, weight loss, and body trembling) of experimental autoimmune myasthenia gravis (EAMG) in rats.Citation55,Citation56,Citation66 Serum nicotinic acetylcholine receptor autoantibodies, elevated in EAMG models, are decreased in Bifidobacterium-treated rats.Citation55,Citation56,Citation66 Pro-inflammatory IFNγ, TNFα, IL-6, and IL-17 are downregulated in the thymus of Bifidobacterium-treated EAMG rats.Citation55 Furthermore, naïve rats treated with B. animalis subsp. Lactis BB12 and LMG S-28195 have increased Tregs in Peyer’s patches, mesenteric LNs (MLN), and in peripheral blood leukocytes. B bifidum, as part of a probiotic cocktail consisting of Lactobacillus casei, Lactobacillus acidophilus, Lactobacillus reuteni, and Streptococcus thermophilus, also skews in vitro DCs toward a regulatory phenotype with increased expression of IL-10, TGFβ, arginase 1, and aldh1a2.Citation56 Treatment with a mixture of B. animalis subsp. lactis BB12 and B. animalis subsp. lactis LMG S-28195 modifies the diversity of EAMG animal microbiomes, reducing disease-associated dysbiosis.Citation66 While largely associative, these studies point to Bifidobacterium as a critical mediator of neuroimmune homeostasis ().
Experimental autoimmune encephalomyelitis
Similar to EAMG, Bifidobacterium also ameliorates experimental autoimmune encephalomyelitis (EAE) in rats.Citation55 Myelin basic protein (MBP)-immunized rats, after injection with MBP-specific T cell blasts, have fewer of these cells localized to spinal cord tissue after treatment with B. animalis subsp. Lactis BB12 and LMG S-28195. Bifidobacterium in combination with Lactobacillus or as part of a larger cocktail (B. bifidum, Lactobacillus casei, Lactobacillus acidophilus, Lactobacillus reuteni, and Streptococcus thermophilus) prevents the progression of EAE in mice.Citation57,Citation58 EAE and multiple sclerosis share many clinical and pathological features, with pathogenesis dependent on IL-17-producing T cells.Citation58 Probiotic-treated EAE mice show reduced central nervous system inflammation with limited neuronal demyelination. Treatment also increases Tregs in peripheral LN (PLN) and spleen while inhibiting Th1 and Th17 polarization. IL-17, IL-6, and IFNγ are downregulated, while TGFβ, IL-4, and IL-10 are upregulated.Citation57,Citation58 In contrast, Bifidobacterium is enriched in patients with multiple sclerosis in both pediatric and adult human case–control seriesCitation59,Citation60 ().
Food allergies
Bifidobacteria are more commonly found in the gut microbiomes of individuals who do not suffer from food allergies, suggesting the importance of Bifidobacteria in regulating allergic responses. In a mouse model of shrimp tropomyosin-induced allergy, treatment with either B. lactis or B. infantis 14.518 reduces allergic symptoms (including decreased serum IgE in both children with food allergies and mouse allergy models).Citation61,Citation62 Mice treated with B. lactis exhibit increased Treg/Th17 ratiosCitation61. Increased microbiome Dorea and decreased Ralstonia in treated animals correlates with elevated Treg/Th17 ratios, suggesting their involvement in the immunomodulatory response induced by Bifidobacterium administration. B. infantis 14.518 increases DC maturation and CD103+ tolerogenic DC accumulation in Peyer’s patches and MLN. This leads to a similar increase in Tregs and suppression of Th2 responses.Citation62 B. infantis 14.518 partially restores gut microbiome richness in tropomyosin-sensitized animals.Citation62 In mice with ovalbumin (Ova)-induced food allergy, gut dysbiosis, inflammation, and inflammatory cell infiltration are reduced or inhibited by concurrent treatment with B. breve M-16 V.Citation63 The probiotic modulates immune responses by inhibiting Th2 responses via ST2 blockade.Citation63 A similar study on the effects of B. breve administration on Ova-induced rhinitis shows increased levels of splenic Treg and decreased Th2 responses, including serum IgE, IL-4, and IL-10.Citation65 B. longum KACC 91,563 also synergizes with IgETRAP, a fusion protein of human high-affinity IgE receptor extracellular domain, hFcεRI, and an IgD/IgG4 hybrid Fc domain, to neutralize IgE and alleviate allergic responses in an Ova-induced food allergy model, including hypothermia, anaphylaxis score, serum IgE and MCPT-1, mast cell numbers, and goblet cell hyperplasia.Citation64 This combined therapy does not alter the host microbiome, suggesting that B. longum KACC 91,563 does this without host colonizationCitation64 ().
PART IV. Bifidobacterium cell surface components serve as immunomodulators
Exopolysaccharides
Exopolysaccharides (EPS) are carbohydrate polymers expressed on the cell surface or secreted by bacteria for both protection and interaction with the surrounding environment.Citation67,Citation68 Reflected by the large inter- and intra-species variability in gene clusters responsible for EPS biosynthesis, structure, and composition, these molecules play numerous roles in host–microbe interactions, including adhesion to the intestinal epithelium and protection from adverse environmental conditions.Citation69,Citation70 In Bifidobacterium, EPS are also implicated in the modulation of host immune responses ().Citation67,Citation70–72 Knocking out EPS expression in Bifidobacterium breve enhances the DC inflammatory phenotype by increasing the expression of co-stimulatory molecule genes Cd80 and Cd83.Citation18 Cell surface β-glucan/galactan polysaccharides of B. bifidum PRI1 induce immunosuppressive Tregs via Toll-like receptor (TLR) 2 signaling on regulatory DCs.Citation6 Even between strains of the same Bifidobacterium species, EPS structure and immune regulation differ. For example, B. breve UCC2003 has a thicker EPS layer and more anti-inflammatory phenotype than B. breve JCM7017 through modulation of macrophage IL-10 and TNFα and DC Tnfa, Il6, and Il23a expression.Citation18 Murine B. pseudolongum UMB287 MBP-01 EPS increases intestinal Tregs compared to control, but EPS from porcine-derived B. pseudolongum ATCC25526 did not. EPS from both strains results in increased intestinal DC, MLN DC, and MLN macrophages.Citation73 The cell surface components of B. longum strains NCC 2705, ATCC 15,707, and BIF53, but not BB536 or NCIMB 8809, stimulate the production of IL-10 and TNFα in isolated peripheral blood mononuclear cells.Citation74 Given the context-dependence of bacterial EPS expression, its effects are likely only a portion of the Bifidobacterial immune modulatory mechanism ().
Figure 1. Bifidobacterial cell surface components, present in both attached and secreted forms, within gut lumen. These components act on both gut epithelium (increasing proliferation, enterocyte adhesion, and modulating cytokine production) and host immune cells (DC, macrophages, and Tregs). DC = dendritic cell; EPS = exopolysaccharide; Mac = macrophage; Treg = Foxp3+ regulatory T cells.
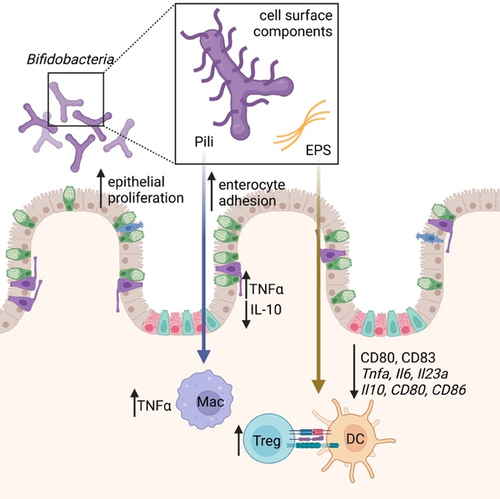
Table 3. Bifidobacterium cell surface components and their immunomodulatory properties.
Pili
Pili are surface appendages that mediate adhesion to the host intestinal epithelium, bacterial cell aggregation, motility, electron transfer, biofilm formation, and immunomodulation.Citation77 These structures display bacterial species-specific immunomodulatory properties.Citation69 Bifidobacteria utilize sortase-dependent (SD) pili, consisting of covalently cross-linked pilin monomers anchored to the cell wall.Citation69,Citation76 These SD pili have roles in virulence, nutrient acquisition, mucin production, host adhesion, and immune signaling ().Citation78 Bifidobacteria also express type IVb tight adherence (Tad) pili, which mediate host surface adhesion.Citation76 Expression of the B. bifidum PRL2010 SD pil3PRL2010 gene cluster in the normally non-piliated Lactococcus lactis NZ9000 enhances adhesion to human intestinal enterocytes and evokes increased TNFα in a human macrophage-like cell line and mouse model compared to non-piliated control bacteria.Citation75 Oral administration of piliated L. lactis-pil3PRL2010 results in decreased IL-10 compared with non-piliated controls in mice.Citation75 Although B. bifidum PRL2010 pili induce higher TNFα expression, they act as weak inducers of other systemic pro-inflammatory cytokines such as IL-12,Citation69,Citation75 suggesting that the immunomodulatory effects of SD pili may be limited to local mucosal immune responses.Citation75 Given the presence of B. bifidum PRL2010 in the infant gut microbiome, the local pro-inflammatory effects of B. bifidum PRL2010 SD pili may prime the neonatal immune systemCitation69,Citation75 ().
PART V. Metabolites directly produced by Bifidobacterium act as immune mediators
Acetate
Metabolites from Bifidobacterium carbohydrate fermentation directly and indirectly influence host immune responses.Citation69 Acetate is one of the most abundant metabolites produced by Bifidobacterium longum 51A reaching the systemic circulation.Citation79 Acetate modulates host defenses and provide protection against various diseases. Mice pre-treated with live B. longum 51A or with acetate have elevated levels of IL-10 in lung tissue following Klebsiella pneumoniae infection compared to untreated infected mice, thus protecting the lungs from injury.Citation79 The ability of Bifidobacterium species and strains to produce acetate varies considerably.Citation80 This variability is influenced not only by the specific species or strain of Bifidobacterium, but also by the nutrient environment in which these bacteria reside. The specific genetic composition of each Bifidobacterium strain confers it with the capability to metabolize a different range of carbohydrate types, directly shaping its metabolic potential. For example, colonization of germ-free mice with B. longum subsp. longum JCM 1217 or B. longum subsp. infantis 157F results in significantly higher concentrations of fecal acetate than colonization with B. longum subsp. infantis JCM 1222T or B. adolescentis JCM 1275T81. Colonization with the former two strains conferred increased survival in mice inoculated with Shiga toxin-producing enteropathogenic E. coli O157.Citation81 These B. longum strains increase acetate production via expression of ATP-binding cassette (ABC)-type carbohydrate transporters that increase sugar consumption for catabolism and acetate production, explaining how these bacteria can still produce acetate even in the fructose-limited distal colon.Citation81 Protection against lethal enteropathogenic E. coli O157:H7 infection in the colon by Bifidobacterium longum subsp. longum JCM 1217T is dependent on acetate production in the distal colon. This is accompanied by upregulation of host immune modulating genes (Apoe, C3, and Pla2g2a) and prevention of Shiga toxin translocation from the gut to the circulation.Citation81,Citation82 This also demonstrates how different strains of B. longum subsp. infantis, in addition to different Bifidobacterium species, can induce differential gene expression related to acetate production, leading to a spectrum of downstream immune effects ().
Table 4. Bifidobacteria-produced metabolites and immunomodulatory properties.
Lactate
Unlike acetate, lactate production is independent of the nutrient environment and relies only on the presence of bacterial strains.Citation80 B. kashiwanohense DSM21854, B. gallicum DSM 20,093, and B. longum infantis 157 F NC produce acetate and lactate. Polysaccharides including starch, inulin, and arabinoxylab via the DC and macrophage receptor GPR81, blunting the inflammatory responses of cells to TLR4 and TLR9.Citation83 Treatment with lactate results in decreased LPS- and galactosamine-induced hepatitis and LPS-induced pancreatitis. Furthermore, in a murine pancreatitis model using intraperitoneal caerulein or retrograde sodium taurocholate injection into the pancreatic duct, B. animalis ATCC25527 and its metabolite lactate, exert a protective effect.Citation84 This is demonstrated through serum amylase reduction, reduction of pancreatic lesions, and improved survival ().
Conjugated linoleic acid
Bifidobacterium-mediated conversion of linoleic acid (LA) into conjugated linoleic acid (CLA) is critical for the maintenance of intestinal homeostasis.Citation85 LA induces redox stress and reduces growth of many different bacterial species, resulting in widespread metabolic reprogramming and dysbiosis.Citation86 However, several Bifidobacterial strains are capable of converting free LA into CLA isomers.Citation85,Citation86 While the exact mechanism behind this bioconversion is unknown, production of CLA promotes gut homeostasis by protecting the microbiome from LA accumulation, which has been shown to confer protective effects in a number of disease models as well as to promote an anti-inflammatory environmentCitation85,Citation86,Citation95 ().
Inosine
The purine nucleoside inosine has best been characterized for its role as an anti-tumor immunomodulator.Citation93 Inosine is produced by B. pseudolongum and enhances anti-tumor immunity via modulation of immune checkpoint blockade by anti-CTLA4 treatment. Inosine mediates CD4 Th1 differentiation via IFN-γ signaling through A2AR on T cells. Immune checkpoint blockage (ICB) therapy is associated with elevated intestinal barrier permeability, which potentially facilitates translocation of inosine and other metabolites from the gut lumen into the systemic circulation. This augmented systemic translocation of metabolites may underpin the observed systemic effects of B. pseudolongum in the context of ICB therapy. Inosine also upregulates the IL-12 receptor on CD4 T cells, which is engaged by conventional DC-produced IL-12Citation89 ().
Tryptophan
Tryptophan metabolism is an important mediator of Bifidobacterial immune modulation. Bifidobacterium longum subsp. infantis ATCC 15,697, a “star colonizer” in breastmilk fed infant gut, produces aromatic lactic acids such as indole-3-lactic acid (ILA) from metabolizing tryptophan, which reduces inflammatory IL-8 production in the human H4 immature primary small intestinal epithelial cell line after IL-1β stimulation.Citation89,Citation96 Similarly, ILA pre-treatment significantly decreases LPS-induced IL-8 production in the Caco-2 intestinal epithelial cell line.Citation90 ILA production is enriched in B. infantis ATCC 15,697 grown on HMO-supplemented media compared with lactose-supplemented media, suggesting that ILA production is driven by the metabolism of milk glycans.Citation90 ILA pre-treatment inhibits LPS-induced NFκB activation in a dose-dependent manner in the murine RAW-blue macrophage reporter cell line, engineered with secreted embryonic alkaline phosphatase.Citation90 ILA signals through the aryl hydrocarbon receptor (AhR), which plays a role in regulating intestinal homeostasis and crosstalk with the cytoprotective Nrf2 pathway that reduces oxidative stressCitation90 ().
Aromatic amino acid-derived aromatic lactic acids are ligands for AhR that influence gut homeostasis through enhanced mucosal barrier function, protection from pathogens,Citation97 and host metabolism.Citation98 Bifidobacterial species convert aromatic amino acids (tryptophan, phenylalanine, and tyrosine) into their respective aromatic lactic acids (ILA, phenyllactic acid, and 4-hydroxyphenyllactic acid), which in turn activates AhR.Citation96 Many Bifidobacterium-derived aromatic lactic acids are found in the infant gut from B. longum, B. bifidum, and B. breve.Citation96 The ability of each species to produce aromatic lactic acids is tied to its ability to use HMOs as a carbohydrate source. In particular, ILA is enriched in the gut of breastfed infants, which modulates IL-22 production in CD4 T cells in vitro via AhR. B. longum CCFM1029 administration increases levels of the tryptophan metabolite indole-3-carbaldehyde (I3C) and improves atopic dermatitis symptoms in mice and humans by suppression of Th2 type immune responses via AhR activation.Citation91 Indole-3-acetic acid (IAA) is another tryptophan metabolite and AhR ligand produced by Bifidobacterial species.Citation99 In ankylosing spondylitis mice, IAA restores the ileal lamina propria Th17/Treg balance via AhR, resulting in increased levels of Foxp3 and downregulation of RORγt and STAT3 ().Citation92 However, there is a broad array of microbiota-derived molecules that act as AhR modulators apart from aromatic lactic acids. For example, the production of butyrate, which can be stimulated by Bifidobacterium as mentioned above,Citation19 acts as an HDAC inhibitor in human intestinal epithelial cell lines and colonic biopsies, increasing the recruitment of AhR to the target gene promoter in the presence of tryptophan-derived AhR agonists,Citation100 Furthermore, tryptophan metabolite indole-3-aldehyde (I3A), catabolized by Lactobacillus reuteri, found within the melanoma tumor microenvironment, facilitates immune checkpoint inhibition via AhR.Citation101
Figure 2. Bifidobacterium-derived metabolites modulate transcription factors and cytokine production in immune cells as well as in gut epithelium.
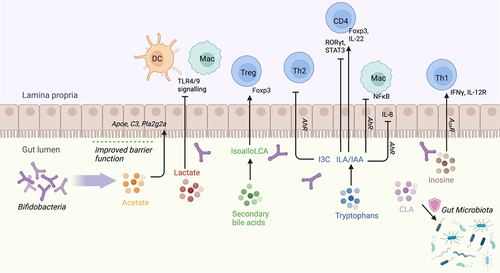
Vitamins
Bifidobacterium, along with lactic acid fermenting bacteria, have been reported to synthesize B-group and K-group vitamins de novo, providing an estimated 30% of the host’s daily intake.Citation102 Notably, several Bifidobacterium species are known to produce B vitamins, including B2 (riboflavin), B6, B9 (folate), and B12 (cobalamin). These vitamins are vital cofactors in numerous metabolic processes, including those integral to host immune function.Citation103 The impact of these vitamins on the immune system is multifaceted: they aid in the development and function of lymphocytes, modulate cytokine production, and support the integrity of the mucosal barriers, forming the first line of defense against pathogens. Furthermore, Bifidobacterium‘s role in vitamin synthesis extends beyond B and K vitamins. A recent study showed commonly used human probiotic strain Bifidobacterium bifidum, along with other bacteria from Bacilli and Clostridia, converts dietary vitamin A to retinoic acid (RA) via aldehyde dehydrogenases.Citation104,Citation105 The upregulated RA-dependent responses in intestinal epithelial cells can provide protection against pathogen colonization, and crucially, contribute to both immunological tolerance and the elicitation of adaptive immune responses.Citation106 For example, RA generation from vitamin A occurs in intestinal epithelial cells and a subset of DCs, resulting in the conversion of naïve T cells into Tregs.Citation107 The vitamin-producing capability of Bifidobacterium thus not only underscores its importance in maintaining gut health, but also highlights its broader influence in immune regulation.
PART VI. Bifidobacterium-influenced metabolites as immunomodulators
While Bifidobacterium does not possess all the metabolic machinery to produce all known immunomodulatory metabolites, it does contribute to an environment permissive toward production of these metabolites by other pro-homeostatic bacteria through mechanisms such as cross-feeding ().
Table 5. Bifidobacteria-influenced metabolites and immunomodulatory properties.
Butyrate
Butyrate regulates the expression of anti-inflammatory cytokine genes, such as TGFβ and IL-10, in antigen presenting cells (APCs) and intestinal epithelial cells (IECs) and stimulates Treg development.Citation108,Citation109 Butyrate has anti-oncogenic properties through induction of tumor cell apoptosis in colorectal cancer.Citation108 Similarly, acetate induces genes involved in anti-inflammatory responsesCitation81 and acts on the colonic epithelium to enhance barrier function, thereby blocking the translocation of pathogens and toxins ().Citation81 While Bifidobacteria are unable to directly synthesize butyrate, Bifidobacterium and the acetate they produce may influence the activity and composition of other members of the gut microbiota that produce butyrate, thus stimulating a secondary butyrogenic effect.Citation19,Citation69,Citation115 Co-culture of B. longum subsp. longum NCC2705, an arabinoxylan oligosaccharide (AXOS)-converting acetate producer, and Eubacterium rectale ATCC 33,656, an acetate-converting butyrate producer, in a growth medium with AXOS yields Bifidobacterium proliferation and butyrate production.Citation19 This occurs due to butyryl-CoA:acetate CoA-transferase upregulation in E. rectale ATCC 33,656, which utilizes acetate as a co-substrate in the final step of butyrate biosynthesis ().
Secondary bile acids (BAs)
Secondary BAs also contribute to the regulation of immune homeostasis that are mostly known to be indirectly influenced by Bifidobacterium via modulating the gut environment and the microbial community structureCitation116. Certain Bifidobacterium species can deconjugate bile salts, which can then influence the ability of other bacteria such as Bacteroides, Eubacterium, Ruminococcus, Clostridium, and Escherichia to further metabolize these bile components into secondary bile acids. The conversion of primary to secondary bile acids is a key process in the gut, which plays a significant role in maintaining gut homeostasis in addition to influencing metabolic and immune functions. The interplay between Bifidobacterium and these other bacterial species in the metabolism of bile components highlights the intricate and collaborative nature of the gut microbiome. Derived from cholesterol catabolism in the liver, primary BAs are secreted postprandially into the duodenum to aid the uptake of dietary fatty acids and fat-soluble vitamins.Citation87,Citation117 Most BAs return to the liver from the gut via the enterohepatic circulation. However, approximately 5% of BAs escape reabsorption in the ileum and are modified by resident enteric bacteria into secondary BAs via dehydroxylation, dehydrogenation, and deconjugation.Citation87,Citation110,Citation117 Secondary BAs can signal Tregs, Th17, or DCs in immune-mediated disorders.Citation87,Citation118,Citation119
Bifidobacterium have been identified in a high-throughput screen of human stool as a converter of 3-oxolithocholic acid (3-oxoLCA), a BA found in the human gut, to isoallolithocholic acid (isoalloLCA), a known immunomodulatory secondary BA.Citation87 IsoalloLCA increases the rate of cellular oxygen consumption, inducing mitochondrial reactive oxygen species that increases the expression of FOXP3, thereby increasing Treg differentiation.Citation88 Despite these connections, few studies have directly investigated the production of secondary BAs by Bifidobacterial species or determined their effects on immune regulation ().
Anti-inflammatory secondary BA 3β-hydroxydeoxycholic acid (isoDCA) decreases DC TNFα and IL-6 production.Citation110 IsoDCA also promotes Foxp3 induction, increasing the number of peripheral Tregs (). Although Bifidobacterium lack 7α-dehydroxylation activity to produce isoDCA from cholic acid.Citation120 Bifidobacterium encode bile salt hydrolases that deconjugate BAs into other secondary BAs that may have similar immunomodulatory roles.Citation111,Citation112
Polyamines
Polyamines (i.e., putrescine, spermidine, and spermine) play a critical role in regulating immunity and inflammation.Citation121,Citation122 While Bifidobacterium does not possess polyamine biosynthetic machinery, its presence does enhance the production of polyamines from other bacterial sources. Administration of several different strains of Bifidobacterium can increase intralumenal putrescene via acidification, likely through acetate and lactate production, which augments putrescine production by E. coli and Enterococcus faecalis Citation33. Spermine restrains innate immune responses by inhibiting M1 macrophage activation via suppression of ornithine decarboxylase and pro-inflammatory cytokine synthesis without perturbing anti-inflammatory TGFβ and IL-10 ().Citation113,Citation114 Spermidine also modulates systemic and mucosal adaptive immunity by modulating T cell differentiation.Citation122,Citation123 Clinically, N-acetyl putrescine and N-acetyl spermidine are enriched in allo-hematopoietic stem cell transplantation (HSCT) recipients free from graft versus host disease (GvHD) compared to those with GvHDCitation124 ().
PART VII. Future directions: therapeutics and technological pipeline
Initial investigations into the role of Bifidobacterium in human disease has been predominantly associative, yet recent studies have begun to delve into the immunomodulatory mechanisms and causal role Bifidobacterium plays in host immune responses. Recognizing a causal relationship not only lays the groundwork for the formulation of targeted therapeutic strategies but also illuminates the underpinnings of disease pathogenesis. This enhanced understanding paves the way for novel drug development, refined disease progression predictions, and innovative preventative strategies.
Though Bifidobacterium displays immunologic pleiotropy, varying across host species and specific strains, there is an increasing interest in its surface components and derived metabolites. These components present promising targets for host immune modulation. A better understanding of the metabolic perturbations due to Bifidobacterium and the influence of their metabolic products on pro-tolerant and pro-homeostatic immunity are critical, especially when considering clinical scenarios such as solid organ transplantation, autoimmunity, and anti-tumor responses. Furthermore, a deeper understanding of the role Bifidobacteria within the broader commensal gut microbiota communities is pivotal. Such knowledge will be instrumental in optimizing live biotherapeutics for the prophylaxis or treatment of disease conditions.
Live biotherapeutics
The absence of HMO-metabolizing Bifidobacterium in the infant gut correlates with Th2- and Th17-driven inflammation in the intestine, as well as both acute and chronic systemic immune disorders.Citation8,Citation125 Investigators have begun to test the impact of exogenous Bifidobacteria on improving overall immune health. Infants fed a diet supplemented with B. longum subsp. infantis EVC001, an optimized strain containing all HMO-utilization genes, display a higher abundance of Bifidobacterium within two months of administration.Citation125,Citation126 These infants have decreased intestinal inflammation, as evidenced by decreased fecal inflammatory cytokine and calprotectin levelsCitation125. Fecal water from EVC001-treated infants also skews the polarization of naïve T cells cultured under Th0 conditions toward a Th1-like state, whereas fecal water from infants lacking B. infantis induces a Th2-like state. When cultured under Th17 polarizing conditions and exposed to EVC001 fecal water, T cells show reduced levels of activation and proliferation markers compared to controls. This effect is recapitulated by B. infantis-derived metabolite ILA, which in addition to the effects mentioned above, upregulates CXCR3, granzyme B, and galectin-1 in cultured cells.Citation8 Administration of EVC001 is also associated with an increase in intestinal IFNβ, a known inducer of Tregs.Citation8,Citation127 Along with changes in cytokine and immune responses, EVC001 supplementation contributes to reducing the amount of virulence factors in the infant gut, restricting the establishment of pathogenic bacterial communities.Citation126 This reinforces the importance of Bifidobacterium-derived metabolites as a mechanism of immunomodulation and as potential therapeutic avenues.
In adults with active UC, one month of treatment with B. longum and prebiotic (preferential inulin-oligofructose growth substrate) reduces endoscopic and histologic inflammation in the colon and reduces mucosal TNFα and IL1 mRNA levels.Citation128 Ex vivo, heat-killed B. breve strain Yakult and B. bifidum strain Yakult both induce increased IL-10 levels in peripheral blood mononuclear cells from UC patients.Citation129 Similarly, in CD patients, B. longum and prebiotic reduces disease activity indices, histologic scores, and mucosal TNFα levels.Citation130 B. infantis 35624 demonstrates a clinical anti-inflammatory effect in UC, chronic fatigue syndrome, and psoriasis. After 6–8 weeks of administration, this probiotic strain reduces plasma CRP levels in all three conditions, reduces TNFα in chronic fatigue syndrome and psoriasis, and reduces IL-6 in UC and chronic fatigue syndrome.Citation131
Although there has been much interest and studies of the benefits of Bifidobacteria-based probiotics, colonization of strains and the direct mechanisms underlying these effects have been challenging to elucidate. Given the complex interactions that Bifidobacteria has with other members of the gut microbiome, off target effects of ongoing probiotic treatment are likely. However, small molecule mediators of the immune modulatory effects of Bifidobacterial strains have a demonstrated potential as therapeutics that have higher purity with more direct and consistent effects than probiotics. Furthermore, metabolites may be able to be administered in a more targeted, tissue-specific manner, compared to probiotics, which must be administered enterally. Future studies will necessarily need to focus on the tissue-specific effects of probiotics, but more specifically of individual metabolic alterations in response to probiotics.
Anti-tumor immunotherapy enhancement
In addition to its direct role in the treatment of autoimmune and inflammatory diseases, Bifidobacterium has also been implicated in modulating the effects of anti-cancer regimens and enhancing anti-tumor immunity. From a mechanistic standpoint, some of these effects may be mediated by Bifidobacterium-derived metabolites. B. pseudolongum promotes immune checkpoint blockade efficacy in mouse models of melanoma, bladder cancer, and colorectal cancer through its production of inosine, which acts on T cells via A2AR.Citation94 In humans, stool samples from patients with improved clinical responses to anti-programmed cell death protein 1 (αPD-1)-based immunotherapy for metastatic melanoma show Bifidobacterium longum enrichment.Citation132 Administration of a Bifidobacterial cocktail containing B. breve and B. longum, both alone and in combination with anti-programmed cell death protein 1 ligand 1 (αPD-L1) to mice with B16.SIY melanoma results in increased gut Bifidobacterium and decreased tumor growth.Citation133 The reduction in tumor growth is dependent on Bifidobacterial-stimulated DC maturation, which enhances CD8 T cell priming and accumulation in the tumor microenvironment.Citation133 B. breve strain JCM92 also augments oxaliplatin’s anti-tumor efficacy in MC38 colon carcinoma-bearing mice compared with oxaliplatin alone, with increased intra-tumor CD8 T cells as well as increased CD4/Treg and CD8/Treg ratios.Citation134 This effect is also true with B. breve JCM92 PD-1 blockade, with increased intra-tumor CD8 T cells and CD8/Treg ratios compared to PD-1 blockade alone. At the transcriptional level, B. breve JCM92 results in higher IL-2, STAT5 signaling, and IFN-γ responses compared to mice treated with oxaliplatin or PD-1 blockade and control bacteria. Addition of B. infantis to monoclonal antibody and radiotherapy treatment of Lewis lung carcinoma in mice slows tumor growth and prolongs animal survival.Citation135 In the same model, B. pseudolongum is significantly elevated in the gut of mice with delayed or absent tumorigenesis.Citation136
In addition to augmenting anti-cancer therapies, Bifidobacteria has also been shown to have independent anti-tumor activity. B. breve lw01 administration suppresses head and neck squamous cell carcinoma growth in mice, mediated by the upregulation of CCL20, which is associated with increased migration of CD11c DCs to ileal villi and tumor microenvironment via upregulation of IL-12Citation137. Furthermore, Bifidobacteria ameliorate checkpoint inhibitor-associated autoimmunity. For examples, mice receiving anti-CTLA-4 with superimposed DSS colitis also receiving a cocktail of Bifidobacterium bifidum, Bifidobacterium longum, Bifidobacterium lactis, and Bifidobacterium breve show less colitis compared to no bacteria controls without impacting anti-tumor efficacy against B16F10 melanoma.Citation138 Tregs are required for this protective effect as it is abrogated in Treg-depleted mice. Despite their broad tolerogenic immune function, Bifidobacteria are also capable of contributing alone or in concert with immunotherapies and chemotherapies to increase host anti-tumor activity.
Pipeline for discovery
Over the past several years, the use of untargeted metabolomics to detect alterations in systemic metabolism has greatly expanded. This technology has provided a hypothesis generating engine for labs studying the metabolite mediators generated or induced by members of the gut microbiota. Other groups have begun to employ spatial transcriptomics to compare local transcriptional changes in cells at the host microbiota interface.Citation139 Application of a spatial omics approaches have proven critical to studying microbiota–gut interface given the significant artifacts that accompany cellular disaggregation protocols used to isolate mucosal cell populations for flow cytometry or single-cell RNA sequencing (scRNAseq). Depending on the protocol used to produce single-cell suspensions for assays, the composition of immune cells from similar starting samples will differ.Citation140 However, both tools only demonstrate association rather than causation. More recently, teams have begun to adopt a hybrid spatial metabolomics approach, which permits spatial resolution of metabolic changes in the host.Citation136 Authors localized metabolic changes, particularly in glycolysis and amino acid catabolism, in tumor tissue in mice administered Lewis Cancer cells and subsequent Akkermansia muciniphila treatment. For example, lactic acid (highly expressed in tumor tissue) was downregulated in the tumors of mice treated with Akkermansia, with confirmed downregulation of lactate dehydrogenase-A enzyme via immunofluorescence. Again, as hypothesis generators, this omics pipeline identifies small molecules of interest both for mechanic studies in vitro and in vivo, but also for development of potential diagnostic biomarkers and therapeutic interventions. While these techniques have not been applied directly to the study of Bifidobacteria, these early studies provide a blueprint.
Disclaimer
Dr. Mongodin contributed to this article as an employee of the University of Maryland School of Medicine. The views expressed are his own and do not necessarily represent the views of the National Institutes of Health or the US government.
Disclosure statement
No potential conflict of interest was reported by the authors.
Data availability statement
Data sharing is not applicable to this article as no new data were created or analyzed in this work.
Additional information
Funding
References
- Ventura M, Canchaya C, Tauch A, Chandra G, Fitzgerald GF, Chater KF, van Sinderen D. Genomics of Actinobacteria: tracing the evolutionary history of an ancient phylum. Microbiol Mol Biol Rev. 2007;71(3):495–23. doi:10.1128/MMBR.00005-07.
- Lugli GA, Milani C, Duranti S, Alessandri G, Turroni F, Mancabelli L, Tatoni D, Ossiprandi MC, van Sinderen D, Ventura M, et al. Isolation of novel gut bifidobacteria using a combination of metagenomic and cultivation approaches. Genome Biol. 2019;20(1):96. doi:10.1186/s13059-019-1711-6.
- Roy D. Media for the isolation and enumeration of bifidobacteria in dairy products. Int J Food Microbiol. 2001;69(3):167–182. doi:10.1016/S0168-1605(01)00496-2.
- Sghir A, Chow JM, Mackie RI. Continuous culture selection of bifidobacteria and lactobacilli from human faecal samples using fructooligosaccharide as selective substrate. J Appl Microbiol. 1998;85(4):769–777. doi:10.1111/j.1365-2672.1998.00590.x.
- Sanders ME, Merenstein DJ, Reid G, Gibson GR, Rastall RA. Probiotics and prebiotics in intestinal health and disease: from biology to the clinic. Nat Rev Gastroenterol Hepatol. 2019;16(10):605–616. doi:10.1038/s41575-019-0173-3.
- Verma R, Lee C, Jeun EJ, Yi J, Kim KS, Ghosh A, Byun S, Lee, CG, Kang, HJ, Kim, GC et al. Cell surface polysaccharides of Bifidobacterium bifidum induce the generation of Foxp3+regulatory T cells. Sci Immunol. 2018;3(28):3. doi:10.1126/sciimmunol.aat6975.
- Guo S, Gillingham T, Guo Y, Meng D, Zhu W, Walker WA, Ganguli K. Secretions of Bifidobacterium infantis and Lactobacillus acidophilus protect intestinal epithelial barrier function. J Pediatr Gastroenterol Nutr. 2017;64(3):404–12. doi:10.1097/MPG.0000000000001310.
- Henrick BM, Rodriguez L, Lakshmikanth T, Pou C, Henckel E, Arzoomand A, Olin A, Wang J, Mikes J, Tan Z, et al. Bifidobacteria-mediated immune system imprinting early in life. Cell. 2021;184(15):3884–98 e11. doi:10.1016/j.cell.2021.05.030.
- Gevers D, Kugathasan S, Denson LA, Vazquez-Baeza Y, Van Treuren W, Ren B, Schwager E, Knights D, Song S, Yassour M, et al. The treatment-naive microbiome in new-onset Crohn’s disease. Cell Host & Microbe. 2014;15(3):382–392. doi:10.1016/j.chom.2014.02.005.
- Shi P, Qu H, Nian D, Chen Y, Liu X, Li Q, Li Q, Wang C, Ye M, Ma B, et al. Treatment of guillain-barré syndrome with Bifidobacterium infantis through regulation of T helper cells subsets. Int Immunopharmacol. 2018;61:290–6. doi:10.1016/j.intimp.2018.06.015.
- Bromberg JS, Hittle LE, Xiong Y, Saxena V, Smyth EM, Li L, Zhang T, Wagner C, Fricke WF, Simon T, et al. Gut microbiota–dependent modulation of innate immunity and lymph node remodeling affects cardiac allograft outcomes. JCI Insight. 2018;3(19):3. doi:10.1172/jci.insight.121045.
- Romano S, Savva GM, Bedarf JR, Charles IG, Hildebrand F, Narbad A. Meta-analysis of the Parkinson’s disease gut microbiome suggests alterations linked to intestinal inflammation. NPJ Parkinsons Dis. 2021;7(1):27. doi:10.1038/s41531-021-00156-z.
- Forbes JD, Chen CY, Knox NC, Marrie RA, El-Gabalawy H, de Kievit T, Alfa M, Bernstein CN, Van Domselaar G. A comparative study of the gut microbiota in immune-mediated inflammatory diseases—does a common dysbiosis exist? Microbiome. 2018;6(1):221. doi:10.1186/s40168-018-0603-4.
- Fan L, Qi Y, Qu S, Chen X, Li A, Hendi M, Xu C, Wang L, Hou T, Si J, et al. B. adolescentis ameliorates chronic colitis by regulating Treg/Th2 response and gut microbiota remodeling. Gut Microbes. 2021;13(1):1–17. doi:10.1080/19490976.2020.1826746.
- Nicola S, Amoruso A, Deidda F, Pane M, Allesina S, Mogna L, Del Piano M, Mogna G. Searching for the perfect homeostasis: five strains of Bifidobacterium longum from centenarians have a similar Behavior in the production of cytokines. J Clin Gastroenterol. 2016;50(Suppl 2):S126–S30. Proceedings from the 8th Probiotics, Prebiotics & New Foods for Microbiota and Human Health meeting held in Rome, Italy on September 13-15, 2015. doi:10.1097/MCG.0000000000000678.
- Geva-Zatorsky N, Sefik E, Kua L, Pasman L, Tan TG, Ortiz-Lopez A, Yanortsang TB, Yang L, Jupp R, Mathis D, et al. Mining the Human Gut Microbiota for Immunomodulatory Organisms. Cell. 2017;168(5):928–943.e11. doi:10.1016/j.cell.2017.01.022.
- Hrdy J, Alard J, Couturier-Maillard A, Boulard O, Boutillier D, Delacre M, Lapadatescu C, Cesaro A, Blanc P, Pot B, et al. Lactobacillus reuteri 5454 and Bifidobacterium animalis ssp. lactis 5764 improve colitis while differentially impacting dendritic cells maturation and antimicrobial responses. Sci Rep. 2020;10(1):5345. doi:10.1038/s41598-020-62161-1.
- Hickey A, Stamou P, Udayan S, Ramon-Vazquez A, Esteban-Torres M, Bottacini F, Woznicki JA, Hughes O, Melgar S, Ventura M, et al. Bifidobacterium breve exopolysaccharide blocks dendritic cell maturation and activation of CD4+ T cells. Front Microbiol. 2021;12:653587. doi:10.3389/fmicb.2021.653587.
- Riviere A, Gagnon M, Weckx S, Roy D, De Vuyst L, Schloss PD. Mutual cross-feeding interactions between Bifidobacterium longum subsp. longum NCC2705 and Eubacterium rectale ATCC 33656 explain the Bifidogenic and butyrogenic effects of Arabinoxylan oligosaccharides. Appl Environ Microbiol. 2015;81(22):7767–7781. doi:10.1128/AEM.02089-15.
- Chia LW, Mank M, Blijenberg B, Bongers RS, van Limpt K, Wopereis H, Tims S, Stahl B, Belzer C, Knol J, et al. Cross-feeding between Bifidobacterium infantis and Anaerostipes caccae on lactose and human milk oligosaccharides. Benef Microbes. 2021;12(1):69–83. doi:10.3920/BM2020.0005.
- Rios-Covian D, Gueimonde M, Duncan SH, Flint HJ, de Los Reyes-Gavilan CG, Cox M. Enhanced butyrate formation by cross-feeding between Faecalibacterium prausnitzii and Bifidobacterium adolescentis. FEMS Microbiol Lett. 2015;362(21):fnv176. doi:10.1093/femsle/fnv176.
- Kim H, Jeong Y, Kang S, You HJ, Ji GE. Co-Culture with Bifidobacterium catenulatum Improves the Growth, Gut Colonization and Butyrate Production. Of Faecalibacterium Prausnitzii: In Vitro And In Vivo Studies. Microorganisms. 2020;8(5):8. doi:10.3390/microorganisms8050788.
- Egan M, Motherway MO, Kilcoyne M, Kane M, Joshi L, Ventura M, van Sinderen D. Cross-feeding by Bifidobacterium breve UCC2003 during co-cultivation with Bifidobacterium bifidum PRL2010 in a mucin-based medium. BMC Microbiol. 2014;14(1):282. doi:10.1186/s12866-014-0282-7.
- Lawson MAE, O’Neill IJ, Kujawska M, Gowrinadh Javvadi S, Wijeyesekera A, Flegg Z, Chalklen L, Hall LJ. Breast milk-derived human milk oligosaccharides promote Bifidobacterium interactions within a single ecosystem. ISME J. 2020;14(2):635–48. doi:10.1038/s41396-019-0553-2.
- Turroni F, Ozcan E, Milani C, Mancabelli L, Viappiani A, van Sinderen D, Sela DA, Ventura M. Glycan cross-feeding activities between bifidobacteria under in vitro conditions. Front Microbiol. 2015;6:1030. doi:10.3389/fmicb.2015.01030.
- Milani C, Lugli GA, Duranti S, Turroni F, Mancabelli L, Ferrario C, Mangifesta M, Hevia A, Viappiani A, Scholz M, et al. Bifidobacteria exhibit social behavior through carbohydrate resource sharing in the gut. Sci Rep. 2015;5(1):15782. doi:10.1038/srep15782.
- Riviere A, Selak M, Geirnaert A, Van den Abbeele P, De Vuyst L, Björkroth J. Complementary mechanisms for degradation of inulin-type fructans and arabinoxylan oligosaccharides among Bifidobacterial strains suggest bacterial cooperation. Appl Environ Microbiol. 2018;84(9):84. doi:10.1128/AEM.02893-17.
- Falony G, Vlachou A, Verbrugghe K, De Vuyst L. Cross-feeding between Bifidobacterium longum BB536 and acetate-converting, butyrate-producing colon bacteria during growth on oligofructose. Appl Environ Microbiol. 2006;72(12):7835–7841. doi:10.1128/AEM.01296-06.
- Moens F, Weckx S, De Vuyst L. Bifidobacterial inulin-type fructan degradation capacity determines cross-feeding interactions between bifidobacteria and Faecalibacterium prausnitzii. Int J Food Microbiol. 2016;231:76–85. doi:10.1016/j.ijfoodmicro.2016.05.015.
- Arpaia N, Campbell C, Fan X, Dikiy S, van der Veeken J, deRoos P, Liu H, Cross JR, Pfeffer K, Coffer PJ, et al. Metabolites produced by commensal bacteria promote peripheral regulatory T-cell generation. Nature. 2013;504(7480):451–5. doi:10.1038/nature12726.
- Smith PM, Howitt MR, Panikov N, Michaud M, Gallini CA, Bohlooly YM, Glickman JN, Garrett WS. The microbial metabolites, short-chain fatty acids, regulate colonic Treg cell homeostasis. Sci. 2013;341(6145):569–73. doi:10.1126/science.1241165.
- Singh N, Gurav A, Sivaprakasam S, Brady E, Padia R, Shi H, Thangaraju M, Prasad P, Manicassamy S, Munn D, et al. Activation of Gpr109a, receptor for niacin and the commensal metabolite butyrate, suppresses colonic inflammation and carcinogenesis. Immunity. 2014;40(1):128–139. doi:10.1016/j.immuni.2013.12.007.
- Kitada Y, Muramatsu K, Toju H, Kibe R, Benno Y, Kurihara S, Matsumoto M. Bioactive polyamine production by a novel hybrid system comprising multiple indigenous gut bacterial strategies. Sci Adv. 2018;4(6):eaat0062. doi:10.1126/sciadv.aat0062.
- Chung SW, Chan BT. Trimethylamine oxide, dimethylamine, trimethylamine and formaldehyde levels in main traded fish species in Hong Kong. Food Addit Contam Part B Surveill. 2009;2(1):44–51. doi:10.1080/02652030902858921.
- Wang Q, Guo M, Liu Y, Xu M, Shi L, Li X, Zhao J, Zhang H, Wang G, Chen W, et al. Bifidobacterium breve and Bifidobacterium longum Attenuate Choline-Induced Plasma Trimethylamine N-Oxide Production by Modulating Gut Microbiota in Mice. Nutrients. 2022;14(6):14. doi:10.3390/nu14061222.
- Bromberg JS, Hittle L, Xiong Y, Saxena V, Smyth EM, Li L, Zhang T, Wagner C, Fricke WF, Simon T, et al. Gut microbiota–dependent modulation of innate immunity and lymph node remodeling affects cardiac allograft outcomes. JCI Insight. 2018;3(19):3. doi:10.1172/jci.insight.121045.
- Li L, Shirkey MW, Zhang T, Piao W, Li X, Zhao J, Mei Z, Guo Y, Saxena V, Kensiski A, et al. Lymph node fibroblastic reticular cells preserve a tolerogenic niche in allograft transplantation through laminin α4. J Clin Invest. 2022;132(13):132. doi:10.1172/JCI156994.
- Ren Z-G, Liu H, Jiang J-W, Jiang L, Chen H, Xie H-Y, Zhou, L., Zheng, S-S. Protective effect of probiotics on intestinal barrier function in malnourished rats after liver transplantation. Hepatobiliary & Pancreatic Diseases International. 2011;10(5):489–496. doi:10.1016/S1499-3872(11)60083-0.
- Xing HC, Li LJ, Xu KJ, Shen T, Chen YB, Sheng JF, Chen Y, Fu S-Z, Chen C-L, Wang J-G, et al. Protective role of supplement with foreign Bifidobacterium and Lactobacillus in experimental hepatic ischemia-reperfusion injury. J Gastroenterol Hepatol. 2006;21(4):647–56. doi:10.1111/j.1440-1746.2006.04306.x.
- Xie Y, Chen H, Zhu B, Qin N, Chen Y, Li Z, Deng M, Jiang H, Xu X, Yang J, et al. Effect of intestinal microbiota alteration on hepatic damage in rats with acute rejection after liver transplantation. Microb Ecol. 2014;68(4):871–880. doi:10.1007/s00248-014-0452-z.
- Bajaj JS, Fagan A, Sikaroodi M, White MB, Sterling RK, Gilles H, Heuman D, Stravitz RT, Matherly SC, Siddiqui MS, et al. Liver transplant modulates gut microbial dysbiosis and cognitive function in cirrhosis. Liver Transpl. 2017;23(7):907–914. doi:10.1002/lt.24754.
- Wu Z-W, Ling Z-X, Lu H-F, Zuo J, Sheng J-F, Zheng S-S, Li L-J. Changes of gut bacteria and immune parameters in liver transplant recipients. Hepatob Pancreat Dis. 2012;11(1):40–50. doi:10.1016/S1499-3872(11)60124-0.
- Swarte JC, Douwes RM, Hu S, Vich Vila A, Eisenga MF, van Londen M, Gomes-Neto AW, Weersma RK, Harmsen HJM, Bakker SJL, et al. Characteristics and dysbiosis of the gut microbiome in renal transplant recipients. J Clin Med. 2020;9(2):386. doi:10.3390/jcm9020386.
- Kato K, Nagao M, Miyamoto K, Oka K, Takahashi M, Yamamoto M, Matsumura Y, Kaido T, Uemoto S, Ichiyama S, et al. Longitudinal analysis of the intestinal microbiota in liver transplantation. Transplant Direct. 2017;3(4):e144. doi:10.1097/TXD.0000000000000661.
- Chen Y, Yang B, Stanton C, Ross RP, Zhao J, Zhang H, Chen W. Bifidobacterium pseudocatenulatum Ameliorates DSS-Induced Colitis by Maintaining Intestinal Mechanical Barrier, Blocking Proinflammatory Cytokines, Inhibiting TLR4/NF-κB Signaling, and Altering Gut Microbiota. J Agric Food Chem. 2021;69(5):1496–512. doi:10.1021/acs.jafc.0c06329.
- Han T, Hu X, Li K, Zhang D, Zhang Y, Li J. Bifidobacterium infantis maintains genome stability in ulcerative colitis via regulating anaphase-promoting complex subunit 7. Front Microbiol. 2021;12:761113. doi:10.3389/fmicb.2021.761113.
- Wang N, Wang S, Xu B, Liu F, Huo G, Li B. Alleviation effects of Bifidobacterium animalis subsp. lactis XLTG11 on Dextran Sulfate sodium-induced colitis in mice. Microorganisms. 2021;9(10):2093. doi:10.3390/microorganisms9102093.
- Chen Y, Jin Y, Stanton C, Paul Ross R, Zhao J, Zhang H, Yang B, Chen W. Alleviation effects of Bifidobacterium breve on DSS-induced colitis depends on intestinal tract barrier maintenance and gut microbiota modulation. Eur J Nutr. 2021;60(1):369–87. doi:10.1007/s00394-020-02252-x.
- Sun S, Luo L, Liang W, Yin Q, Guo J, Rush AM, Lv Z, Liang Q, Fischbach, MA, Sonnenburg, JL et al. Bifidobacterium alters the gut microbiota and modulates the functional metabolism of T regulatory cells in the context of immune checkpoint blockade. Proceedings Of The National Academy Of Sciences Of The United States Of America 2020; 117:27509–15.
- Laparra JM, Olivares M, Gallina O, Sanz Y, Leulier F. Bifidobacterium longum CECT 7347 modulates immune responses in a gliadin-induced enteropathy animal model. PLoS ONE. 2012;7(2):e30744. doi:10.1371/journal.pone.0030744.
- Olivares M, Laparra M, Sanz Y. Oral administration of Bifidobacterium longum CECT 7347 modulates jejunal proteome in an in vivo gliadin-induced enteropathy animal model. J Proteomics. 2012;77:310–320. doi:10.1016/j.jprot.2012.09.005.
- McCarville JL, Dong J, Caminero A, Bermudez-Brito M, Jury J, Murray JA, Duboux S, Steinmann M, Delley M, Tangyu M, et al. A commensal Bifidobacterium longum strain prevents gluten-related immunopathology in mice through expression of a serine protease inhibitor. Appl Environ Microbiol. 2017;83(19). doi:10.1128/AEM.01323-17.
- Medina M, De Palma G, Ribes-Koninckx C, Calabuig M, Sanz Y. Bifidobacterium strains suppress in vitro the pro-inflammatory milieu triggered by the large intestinal microbiota of coeliac patients. J Inflamm (Lond). 2008;5(1):19. doi:10.1186/1476-9255-5-19.
- Lindfors K, Blomqvist T, Juuti-Uusitalo K, Stenman S, Venalainen J, Maki M, Kaukinen K. Live probiotic Bifidobacterium lactis bacteria inhibit the toxic effects induced by wheat gliadin in epithelial cell culture. Clin Exp Immunol. 2008;152(3):552–558. doi:10.1111/j.1365-2249.2008.03635.x.
- Consonni A, Cordiglieri C, Rinaldi E, Marolda R, Ravanelli I, Guidesi E, Elli M, Mantegazza R, Baggi F. Administration of bifidobacterium and lactobacillus strains modulates experimental myasthenia gravis and experimental encephalomyelitis in Lewis rats. Oncotarget. 2018;9(32):22269–87. doi:10.18632/oncotarget.25170.
- Chae CS, Kwon HK, Hwang JS, Kim JE, Im SH, Platten M. Prophylactic effect of probiotics on the development of experimental autoimmune myasthenia gravis. PLoS ONE. 2012;7(12):e52119. doi:10.1371/journal.pone.0052119.
- Salehipour Z, Haghmorad D, Sankian M, Rastin M, Nosratabadi R, Soltan Dallal MM, Tabasi N, Khazaee M, Nasiraii LR, Mahmoudi M, et al. Bifidobacterium animalis in combination with human origin of Lactobacillus plantarum ameliorate neuroinflammation in experimental model of multiple sclerosis by altering CD4+ T cell subset balance. Biomed Pharmacother. 2017;95:1535–1548. doi:10.1016/j.biopha.2017.08.117.
- Kwon HK, Kim GC, Kim Y, Hwang W, Jash A, Sahoo A, Kim J-E, Nam JH, Im S-H. Amelioration of experimental autoimmune encephalomyelitis by probiotic mixture is mediated by a shift in T helper cell immune response. Clin Immunol. 2013;146(3):217–27. doi:10.1016/j.clim.2013.01.001.
- Tremlett H, Fadrosh DW, Faruqi AA, Zhu F, Hart J, Roalstad S, Graves J, Lynch S, Waubant E. Gut microbiota in early pediatric multiple sclerosis: a case−control study. Eur J Neurol. 2016;23(8):1308–21. doi:10.1111/ene.13026.
- Baum K, Rejmus R, Dorffel Y. Commensal gut flora in MS: Spatial organization and composition. Mult Scler. 2015;21:458–459.
- Liu Q, Jing W, Wang W. Bifidobacterium lactis ameliorates the risk of food allergy in Chinese children by affecting relative percentage of Treg and Th17 cells. Can J Infect Dis Med Microbiol. 2018;2018:1–12. doi:10.1155/2018/4561038.
- Fu L, Song J, Wang C, Fu S, Wang Y. Bifidobacterium infantis potentially alleviates Shrimp tropomyosin-induced allergy by tolerogenic dendritic cell-dependent induction of regulatory T cells and alterations in gut microbiota. Front Immunol. 2017;8:1536. doi:10.3389/fimmu.2017.01536.
- Li N, Yu Y, Chen X, Gao S, Zhang Q, Xu C. Bifidobacterium breve M-16V alters the gut microbiota to alleviate OVA-induced food allergy through IL-33/ST2 signal pathway. J Cell Physiol. 2020;235(12):9464–9473. doi:10.1002/jcp.29751.
- An SB, Yang BG, Jang G, Kim DY, Kim J, Oh SM, Oh N, Lee S, Moon J-Y, Kim J-A, et al. Combined IgE neutralization and Bifidobacterium longum supplementation reduces the allergic response in models of food allergy. Nat Commun. 2022;13(1):5669. doi:10.1038/s41467-022-33176-1.
- Ren J, Zhao Y, Huang S, Lv D, Yang F, Lou L, Zheng Y, Zhang J, Liu S, Zhang N, et al. Immunomodulatory effect of Bifidobacterium breve on experimental allergic rhinitis in BALB/c mice. Exp Ther Med. 2018;16:3996–4004. doi:10.3892/etm.2018.6704.
- Rinaldi E, Consonni A, Cordiglieri C, Sacco G, Crasa C, Fontana A, Morelli L, Elli M, Mantegazza R, Baggi F, et al. Therapeutic Effect of Bifidobacterium Administration on Experimental Autoimmune Myasthenia Gravis in Lewis Rats. Front Immunol. 2019;10:2949. doi:10.3389/fimmu.2019.02949.
- Castro-Bravo N, Wells JM, Margolles A, Ruas-Madiedo P. Interactions of surface Exopolysaccharides from Bifidobacterium and Lactobacillus within the intestinal environment. Front Microbiol. 2018;9:2426. doi:10.3389/fmicb.2018.02426.
- Costa OYA, Raaijmakers JM, Kuramae EE. Microbial Extracellular Polymeric Substances: Ecological Function and Impact on Soil Aggregation. Front Microbiol. 2018;9:1636. doi:10.3389/fmicb.2018.01636.
- Alessandri G, Ossiprandi MC, MacSharry J, van Sinderen D, Ventura M. Bifidobacterial dialogue with its human host and consequent modulation of the immune system. Front Immunol. 2019;10:2348. doi:10.3389/fimmu.2019.02348.
- López P, Monteserín DC, Gueimonde M, de Los Reyes-Gavilán CG, Margolles A, Suárez A, Ruas-Madiedo P. Exopolysaccharide-producing Bifidobacterium strains elicit different in vitro responses upon interaction with human cells. Food Res Int. 2012;46(1):99–107. doi:10.1016/j.foodres.2011.11.020.
- Fanning S, Hall LJ, Cronin M, Zomer A, MacSharry J, Goulding D, O’Connell Motherway M, Shanahan F, Nally K, Dougan G, et al. Bifidobacterial surface-exopolysaccharide facilitates commensal-host interaction through immune modulation and pathogen protection. Proc Natl Acad Sci U S A. 2012;109(6):2108–2113. doi:10.1073/pnas.1115621109.
- Hidalgo-Cantabrana C, Lopez P, Gueimonde M, de Los Reyes-Gavilan CG, Suarez A, Margolles A, Ruas-Madiedo P. Immune modulation capability of Exopolysaccharides synthesised by lactic acid bacteria and Bifidobacteria. Probiotics Antimicrob Proteins. 2012;4(4):227–37. doi:10.1007/s12602-012-9110-2.
- Ma B, Gavzy SJ, Saxena V, Song Y, Piao W, Lwin HW, Lakhan R, Iyyathurai J, Li L, France M, et al. Strain-specific alterations in gut microbiome and host immune responses elicited by tolerogenic Bifidobacterium pseudolongum. Sci Rep. 2023;13(1):1023. doi:10.1038/s41598-023-27706-0.
- Medina M, Izquierdo E, Ennahar S, Sanz Y. Differential immunomodulatory properties of Bifidobacterium logum strains: relevance to probiotic selection and clinical applications. Clin Exp Immunol. 2007;150(3):531–538. doi:10.1111/j.1365-2249.2007.03522.x.
- Turroni F, Serafini F, Foroni E, Duranti S, O’Connell Motherway M, Taverniti V, Mangifesta M, Milani C, Viappiani A, Roversi T, et al. Role of sortase-dependent pili of Bifidobacterium bifidum PRL2010 in modulating bacterium–host interactions. Proc Natl Acad Sci U S A. 2013;110(27):11151–6. doi:10.1073/pnas.1303897110.
- O’Connell Motherway M, Zomer A, Leahy SC, Reunanen J, Bottacini F, Claesson MJ, O’Brien F, Flynn K, Casey PG, Moreno Munoz JA, et al. Functional genome analysis of Bifidobacterium breve UCC2003 reveals type IVb tight adherence (Tad) pili as an essential and conserved host-colonization factor. Proc Natl Acad Sci USA. 2011;108(27):11217–22. doi:10.1073/pnas.1105380108.
- Milani C, Mangifesta M, Mancabelli L, Lugli GA, Mancino W, Viappiani A, Faccini A, van Sinderen D, Ventura M, Turroni F, et al. The sortase-dependent fimbriome of the genus Bifidobacterium: extracellular structures with potential to modulate Microbe-host dialogue. Appl Environ Microbiol. 2017;83(19). doi:10.1128/AEM.01295-17.
- Call EK, Klaenhammer TR. Relevance and application of sortase and sortase-dependent proteins in lactic acid bacteria. Front Microbiol. 2013;4:73. doi:10.3389/fmicb.2013.00073.
- Vieira AT, Rocha VM, Tavares L, Garcia CC, Teixeira MM, Oliveira SC, Cassali GD, Gamba C, Martins FS, Nicoli JR. Control of Klebsiella pneumoniae pulmonary infection and immunomodulation by oral treatment with the commensal probiotic Bifidobacterium longum 51A. Microbes Infect. 2016;18(3):180–9. doi:10.1016/j.micinf.2015.10.008.
- Devika NT, Raman K. Deciphering the metabolic capabilities of Bifidobacteria using genome-scale metabolic models. Sci Rep. 2019;9(1):18222. doi:10.1038/s41598-019-54696-9.
- Fukuda S, Toh H, Hase K, Oshima K, Nakanishi Y, Yoshimura K, Tobe T, Clarke JM, Topping DL, Suzuki T, et al. Bifidobacteria can protect from enteropathogenic infection through production of acetate. Nature. 2011;469(7331):543–547. doi:10.1038/nature09646.
- Fukuda S, Toh H, Taylor TD, Ohno H, Hattori M. Acetate-producing bifidobacteria protect the host from enteropathogenic infection via carbohydrate transporters. Gut Microbes. 2012;3(5):449–454. doi:10.4161/gmic.21214.
- Hoque R, Farooq A, Ghani A, Gorelick F, Mehal WZ. Lactate reduces liver and pancreatic injury in Toll-like receptor– and inflammasome-mediated inflammation via GPR81-mediated suppression of innate immunity. Gastroenterology. 2014;146(7):1763–74. doi:10.1053/j.gastro.2014.03.014.
- Li H, Xie J, Guo X, Yang G, Cai B, Liu J, Yue M, Tang Y, Wang G, Chen S, et al. Bifidobacterium spp. and their metabolite lactate protect against acute pancreatitis via inhibition of pancreatic and systemic inflammatory responses. Gut Microbes. 2022;14(1):2127456. doi:10.1080/19490976.2022.2127456.
- Mei Y, Chen H, Yang B, Zhao J, Zhang H, Chen W. Computational analysis and Heterologous expression of BBI-like proteins from food-grade Bifidobacterium species reveal possibly a key factor in conjugated linoleic acid bioconversion. J Agric Food Chem. 2023;71(21):8093–103. doi:10.1021/acs.jafc.3c00857.
- Senizza A, Rocchetti G, Callegari ML, Lucini L, Morelli L. Linoleic acid induces metabolic stress in the intestinal microorganism Bifidobacterium breve DSM 20213. Sci Rep. 2020;10(1):5997. doi:10.1038/s41598-020-62897-w.
- Li W, Hang S, Fang Y, Bae S, Zhang Y, Zhang M, Wang G, McCurry MD, Bae M, Paik D, et al. A bacterial bile acid metabolite modulates T(reg) activity through the nuclear hormone receptor NR4A1. Cell Host & Microbe. 2021;29(9):1366–1377.e9. doi:10.1016/j.chom.2021.07.013.
- Hang S, Paik D, Yao L, Kim E, Trinath J, Lu J, Ha S, Nelson BN, Kelly SP, Wu L, et al. Bile acid metabolites control TH17 and Treg cell differentiation. Nature. 2019;576(7785):143–148. doi:10.1038/s41586-019-1785-z.
- Meng D, Sommella E, Salviati E, Campiglia P, Ganguli K, Djebali K, Zhu W, Walker WA. Indole-3-lactic acid, a metabolite of tryptophan, secreted by Bifidobacterium longum subspecies infantis is anti-inflammatory in the immature intestine. Pediatr Res. 2020;88(2):209–17. doi:10.1038/s41390-019-0740-x.
- Ehrlich AM, Pacheco AR, Henrick BM, Taft D, Xu G, Huda MN, Mishchuk D, Goodson ML, Slupsky C, Barile D, et al. Indole-3-lactic acid associated with Bifidobacterium-dominated microbiota significantly decreases inflammation in intestinal epithelial cells. BMC Microbiol. 2020;20(1):357. doi:10.1186/s12866-020-02023-y.
- Fang Z, Pan T, Li L, Wang H, Zhu J, Zhang H, Zhao J, Chen W, Lu W. Bifidobacterium longum mediated tryptophan metabolism to improve atopic dermatitis via the gut-skin axis. Gut Microbes. 2022;14(1):14. doi:10.1080/19490976.2022.2044723.
- Shen J, Yang L, You K, Chen T, Su Z, Cui Z, Wang M, Zhang W, Liu B, Zhou K, et al. Indole-3-acetic acid alters intestinal microbiota and alleviates ankylosing spondylitis in mice. Front Immunol. 2022;13:762580. doi:10.3389/fimmu.2022.762580.
- Wang T, Gnanaprakasam JNR, Chen X, Kang S, Xu X, Sun H, Liu L, Rodgers H, Miller E, Cassel TA, et al. Inosine is an alternative carbon source for CD8(+)-T-cell function under glucose restriction. Nat Metab. 2020;2(7):635–47. doi:10.1038/s42255-020-0219-4.
- Mager LF, Burkhard R, Pett N, Cooke NCA, Brown K, Ramay H, Paik S, Stagg J, Groves RA, Gallo M, et al. Microbiome-derived inosine modulates response to checkpoint inhibitor immunotherapy. Sci. 2020;369(6510):1481–1489. doi:10.1126/science.abc3421.
- Carafa I, Masuero D, Vrhovsek U, Bittante G, Franciosi E, Tuohy KM Production of conjugated linoleic acid (CLA): effect of inulin on microbial composition and CLA concentration in a human intestinal model. Proceedings of the Nutrition Society 2020; 79.
- Laursen MF, Sakanaka M, von Burg N, Morbe U, Andersen D, Moll JM, Pekmez CT, Rivollier A, Michaelsen KF, Mølgaard C, et al. Bifidobacterium species associated with breastfeeding produce aromatic lactic acids in the infant gut. Nat microbiol. 2021;6(11):1367–1382. doi:10.1038/s41564-021-00970-4.
- Zelante T, Iannitti RG, Cunha C, De Luca A, Giovannini G, Pieraccini G, Zecchi R, D’Angelo C, Massi-Benedetti C, Fallarino F, et al. Tryptophan catabolites from microbiota engage aryl hydrocarbon receptor and balance mucosal reactivity via interleukin-22. Immunity. 2013;39(2):372–385. doi:10.1016/j.immuni.2013.08.003.
- Natividad JM, Agus A, Planchais J, Lamas B, Jarry AC, Martin R, Michel M-L, Chong-Nguyen C, Roussel R, Straube M, et al. Impaired aryl hydrocarbon receptor ligand production by the gut microbiota is a key factor in metabolic syndrome. Cell Metab. 2018;28(5):737–749.e4. doi:10.1016/j.cmet.2018.07.001.
- Gao K, Mu CL, Farzi A, Zhu WY. Tryptophan metabolism: a link between the gut microbiota and brain. Adv Nutr. 2020;11(3):709–23. doi:10.1093/advances/nmz127.
- Modoux M, Rolhion N, Lefevre JH, Oeuvray C, Nadvornik P, Illes P, Emond P, Parc Y, Mani S, Dvorak Z, et al. Butyrate acts through HDAC inhibition to enhance aryl hydrocarbon receptor activation by gut microbiota-derived ligands. Gut Microbes. 2022;14(1):2105637. doi:10.1080/19490976.2022.2105637.
- Bender MJ, McPherson AC, Phelps CM, Pandey SP, Laughlin CR, Shapira JH, Medina Sanchez L, Rana M, Richie TG, Mims TS, et al. Dietary tryptophan metabolite released by intratumoral Lactobacillus reuteri facilitates immune checkpoint inhibitor treatment. Cell. 2023;186(9):1846–1862.e26. doi:10.1016/j.cell.2023.03.011.
- LeBlanc JG, Milani C, de Giori GS, Sesma F, van Sinderen D, Ventura M. Bacteria as vitamin suppliers to their host: a gut microbiota perspective. Curr Opin Biotechnol. 2013;24(2):160–168. doi:10.1016/j.copbio.2012.08.005.
- Yoshii K, Hosomi K, Sawane K, Kunisawa J. Metabolism of dietary and microbial vitamin B family in the regulation of host immunity. Front Nutr. 2019;6:48. doi:10.3389/fnut.2019.00048.
- Woo V, Eshleman EM, Hashimoto-Hill S, Whitt J, Wu SE, Engleman L, Rice T, Karns R, Qualls JE, Haslam DB, et al. Commensal segmented filamentous bacteria-derived retinoic acid primes host defense to intestinal infection. Cell Host & Microbe. 2021;29(12):1744–1756.e5. doi:10.1016/j.chom.2021.09.010.
- Bonakdar M, Czuba LC, Han G, Zhong G, Luong H, Isoherranen N, Vaishnava S. Gut commensals expand vitamin a metabolic capacity of the mammalian host. Cell Host & Microbe. 2022;30(8):1084–1092.e5. doi:10.1016/j.chom.2022.06.011.
- Hall JA, Grainger JR, Spencer SP, Belkaid Y. The role of retinoic acid in tolerance and immunity. Immunity. 2011;35(1):13–22. doi:10.1016/j.immuni.2011.07.002.
- Duriancik DM, Lackey DE, Hoag KA. Vitamin A as a regulator of antigen presenting cells. J Nutr. 2010;140(8):1395–1399. doi:10.3945/jn.110.124461.
- Canani RB, Costanzo MD, Leone L, Pedata M, Meli R, Calignano A. Potential beneficial effects of butyrate in intestinal and extraintestinal diseases. World J Gastroenterol. 2011;17(12):1519–28. doi:10.3748/wjg.v17.i12.1519.
- Furusawa Y, Obata Y, Fukuda S, Endo TA, Nakato G, Takahashi D, Nakanishi Y, Uetake C, Kato K, Kato T, et al. Commensal microbe-derived butyrate induces the differentiation of colonic regulatory T cells. Nature. 2013;504(7480):446–450. doi:10.1038/nature12721.
- Campbell C, McKenney PT, Konstantinovsky D, Isaeva OI, Schizas M, Verter J, Mai C, Jin W-B, Guo C-J, Violante S, et al. Bacterial metabolism of bile acids promotes generation of peripheral regulatory T cells. Nature. 2020;581(7809):475–479. doi:10.1038/s41586-020-2193-0.
- Kriaa A, Bourgin M, Potiron A, Mkaouar H, Jablaoui A, Gerard P, Maguin E, Rhimi M. Microbial impact on cholesterol and bile acid metabolism: current status and future prospects. J Lipid Res. 2019;60(2):323–32. doi:10.1194/jlr.R088989.
- Begley M, Hill C, Gahan CG. Bile salt hydrolase activity in probiotics. Appl Environ Microbiol. 2006;72(3):1729–1738. doi:10.1128/AEM.72.3.1729-1738.2006.
- Zhang M, Wang H, Tracey KJ. Regulation of macrophage activation and inflammation by spermine: a new chapter in an old story. Crit Care Med. 2000;28(Supplement):N60–6. doi:10.1097/00003246-200004001-00007.
- Zhang M, Caragine T, Wang H, Cohen PS, Botchkina G, Soda K, Bianchi M, Ulrich P, Cerami A, Sherry B, et al. Spermine inhibits proinflammatory cytokine synthesis in human mononuclear cells: a counterregulatory mechanism that restrains the immune response. J Exp Med. 1997;185(10):1759–1768. doi:10.1084/jem.185.10.1759.
- Riviere A, Selak M, Lantin D, Leroy F, De Vuyst L. Bifidobacteria and butyrate-producing colon bacteria: importance and strategies for their stimulation in the human gut. Front Microbiol. 2016;7:979. doi:10.3389/fmicb.2016.00979.
- Staley C, Weingarden AR, Khoruts A, Sadowsky MJ. Interaction of gut microbiota with bile acid metabolism and its influence on disease states. Appl Microbiol Biotechnol. 2017;101(1):47–64. doi:10.1007/s00253-016-8006-6.
- Shapiro H, Thaiss CA, Levy M, Elinav E. The cross talk between microbiota and the immune system: metabolites take center stage. Curr Opin Immunol. 2014;30:54–62. doi:10.1016/j.coi.2014.07.003.
- Paik D, Yao L, Zhang Y, Bae S, D’Agostino GD, Zhang M, Kim E, Franzosa EA, Avila-Pacheco J, Bisanz JE, et al. Human gut bacteria produce ΤΗ17-modulating bile acid metabolites. Nature. 2022;603(7903):907–12. doi:10.1038/s41586-022-04480-z.
- Hu J, Wang C, Huang X, Yi S, Pan S, Zhang Y, Yuan G, Cao Q, Ye X, Li H, et al. Gut microbiota-mediated secondary bile acids regulate dendritic cells to attenuate autoimmune uveitis through TGR5 signaling. Cell Rep. 2021;36(12):109726. doi:10.1016/j.celrep.2021.109726.
- Takahashi T, Morotomi M. Absence of cholic acid 7α-dehydroxylase activity in the strains of Lactobacillus and Bifidobacterium. J Dairy Sci. 1994;77(11):3275–3286. doi:10.3168/jds.S0022-0302(94)77268-4.
- Proietti E, Rossini S, Grohmann U, Mondanelli G. Polyamines and Kynurenines at the intersection of immune modulation. Trends Immunol. 2020;41(11):1037–1050. doi:10.1016/j.it.2020.09.007.
- Carriche GM, Almeida L, Stuve P, Velasquez L, Dhillon-LaBrooy A, Roy U, Lindenberg M, Strowig T, Plaza-Sirvent C, Schmitz I, et al. Regulating T-cell differentiation through the polyamine spermidine. J Allergy Clin Immunol. 2021;147(1):335–348.e11. doi:10.1016/j.jaci.2020.04.037.
- Puleston DJ, Baixauli F, Sanin DE, Edwards-Hicks J, Villa M, Kabat AM, Kamiński MM, Stanckzak M, Weiss HJ, Grzes KM, et al. Polyamine metabolism is a central determinant of helper T cell lineage fidelity. Cell. 2021;184(16):4186–4202.e20. doi:10.1016/j.cell.2021.06.007.
- Michonneau D, Latis E, Curis E, Dubouchet L, Ramamoorthy S, Ingram B, de Latour RP, Robin M, de Fontbrune FS, Chevret S, et al. Metabolomics analysis of human acute graft-versus-host disease reveals changes in host and microbiota-derived metabolites. Nat Commun. 2019;10(1):5695. doi:10.1038/s41467-019-13498-3.
- Henrick BM, Chew S, Casaburi G, Brown HK, Frese SA, Zhou Y, Underwood MA, Smilowitz JT. Colonization by B. infantis EVC001 modulates enteric inflammation in exclusively breastfed infants. Pediatr Res. 2019;86(6):749–57. doi:10.1038/s41390-019-0533-2.
- Casaburi G, Frese SA. Colonization of breastfed infants by Bifidobacterium longum subsp. infantis EVC001 reduces virulence gene abundance. Human Microbiome J. 2018;9:7–10. doi:10.1016/j.humic.2018.05.001.
- Dikopoulos N, Bertoletti A, Kroger A, Hauser H, Schirmbeck R, Reimann J. Type I IFN negatively regulates CD8+ T cell responses through IL-10-producing CD4+ T regulatory 1 cells. J Immunol. 2005;174(1):99–109. doi:10.4049/jimmunol.174.1.99.
- Furrie E, Macfarlane S, Kennedy A, Cummings JH, Walsh SV, O’Neil DA, Macfarlane GT. Synbiotic therapy (Bifidobacterium longum/Synergy 1) initiates resolution of inflammation in patients with active ulcerative colitis: a randomised controlled pilot trial. Gut. 2005;54(2):242–9. doi:10.1136/gut.2004.044834.
- Imaoka A, Shima T, Kato K, Mizuno S, Uehara T, Matsumoto S, Setoyama H, Hara T, Umesaki Y. Anti-inflammatory activity of probiotic Bifidobacterium: enhancement of IL-10 production in peripheral blood mononuclear cells from ulcerative colitis patients and inhibition of IL-8 secretion in HT-29 cells. World J Gastroenterol. 2008;14(16):2511–6. doi:10.3748/wjg.14.2511.
- Steed H, Macfarlane GT, Blackett KL, Bahrami B, Reynolds N, Walsh SV, Cummings JH, Macfarlane S. Clinical trial: the microbiological and immunological effects of synbiotic consumption – a randomized double-blind placebo-controlled study in active Crohn’s disease. Aliment Pharmacol Ther. 2010;32(7):872–83. doi:10.1111/j.1365-2036.2010.04417.x.
- Groeger D, O’Mahony L, Murphy EF, Bourke JF, Dinan TG, Kiely B, Shanahan F, Quigley EMM. Bifidobacterium infantis 35624 modulates host inflammatory processes beyond the gut. Gut Microbes. 2013;4(4):325–39. doi:10.4161/gmic.25487.
- Matson V, Fessler J, Bao R, Chongsuwat T, Zha Y, Alegre ML, Luke JJ, Gajewski TF. The commensal microbiome is associated with anti–PD-1 efficacy in metastatic melanoma patients. Sci. 2018;359(6371):104–8. doi:10.1126/science.aao3290.
- Sivan A, Corrales L, Hubert N, Williams JB, Aquino-Michaels K, Earley ZM, Benyamin FW, Man Lei Y, Jabri B, Alegre M-L, et al. Commensal Bifidobacterium promotes antitumor immunity and facilitates anti–PD-L1 efficacy. Sci. 2015;350(6264):1084–9. doi:10.1126/science.aac4255.
- Yoon Y, Kim G, Jeon BN, Fang S, Park H. Bifidobacterium strain-specific enhances the efficacy of cancer therapeutics in tumor-bearing mice. Cancers Basel. 2021;13(5):957. doi:10.3390/cancers13050957.
- Yang J, Wu Z, Chen Y, Hu C, Li D, Chen Y, Imani S, Wen Q, Fu S, Wu J, et al. Pre-treatment with Bifidobacterium infantis and its specific antibodies enhance targeted radiosensitization in a murine model for lung cancer. J Cancer Res Clin Oncol. 2021;147(2):411–422. doi:10.1007/s00432-020-03434-0.
- Zhu Z, Cai J, Hou W, Xu K, Wu X, Song Y, Bai C, Mo Y-Y, Zhang Z. Microbiome and spatially resolved metabolomics analysis reveal the anticancer role of gut Akkermansia muciniphila by crosstalk with intratumoral microbiota and reprogramming tumoral metabolism in mice. Gut Microbes. 2023;15(1):2166700. doi:10.1080/19490976.2023.2166700.
- Li Q, Li Y, Wang Y, Xu L, Guo Y, Wang Y, Wang L, Guo C. Oral administration of Bifidobacterium breve promotes antitumor efficacy via dendritic cells-derived interleukin 12. Oncoimmunology. 2021;10(1):1868122. doi:10.1080/2162402X.2020.1868122.
- Wang F, Yin Q, Chen L, Davis MM Bifidobacterium can mitigate intestinal immunopathology in the context of CTLA-4 blockade. Proceedings of the National Academy of Sciences of the United States of America 2018; 115:157–61.
- Galeano Nino JL, Wu H, LaCourse KD, Kempchinsky AG, Baryiames A, Barber B, Futran N, Houlton J, Sather C, Sicinska E, et al. Effect of the intratumoral microbiota on spatial and cellular heterogeneity in cancer. Nature. 2022;611(7937):810–817. doi:10.1038/s41586-022-05435-0.
- Uniken Venema WTC, Ramirez-Sanchez AD, Bigaeva E, Withoff S, Jonkers I, McIntyre RE, Ghouraba M, Raine T, Weersma RK, Franke L, et al. Gut mucosa dissociation protocols influence cell type proportions and single-cell gene expression levels. Sci Rep. 2022;12(1):9897. doi:10.1038/s41598-022-13812-y.