ABSTRACT
Intestinal dysbiosis frequently occurs in abdominal radiotherapy and contributes to irradiation (IR)-induced intestinal damage and inflammation. Akkermansia muciniphila (A. muciniphila) is a recently characterized probiotic, which is critical for maintaining the dynamics of the intestinal mucus layer and preserving intestinal microbiota homeostasis. However, the role of A. muciniphila in the alleviation of radiation enteritis remains unknown. In this study, we reported that the abundance of A. muciniphila was markedly reduced in the intestines of mice exposed to abdominal IR and in the feces of patients who received abdominal radiotherapy. Abundance of A. muciniphila in feces of radiotherapy patients was negatively correlated with the duration of diarrhea in patients. Administration of A. muciniphila substantially mitigated IR-induced intestinal damage and prevented mouse death. Analyzing the metabolic products of A. muciniphila revealed that propionic acid, a short-chain fatty acid secreted by the microbe, mediated the radioprotective effect. We further demonstrated that propionic acid bound to G-protein coupled receptor 43 (GRP43) on the surface of intestinal epithelia and increased histone acetylation and hence enhanced the expression of tight junction proteins occludin and ZO-1 and elevated the level of mucins, leading to enhanced integrity of intestinal epithelial barrier and reduced radiation-induced intestinal damage. Metformin, a first-line agent for the treatment of type II diabetes, promoted intestinal epithelial barrier integrity and reduced radiation intestinal damage through increasing the abundance of A. muciniphila. Together, our results demonstrated that A. muciniphila plays a critical role in the reduction of abdominal IR-induced intestinal damage. Application of probiotics or their regulators, such as metformin, could be an effective treatment for the protection of radiation exposure-damaged intestine.
Introduction
Radiotherapy is indispensable for a comprehensive treatment of cancer, and enteritis is the most common complication of abdominal radiotherapyCitation1. Acute enteritis occurs in 50% to 70% of the patients, who received abdominal or pelvic radiotherapy. Abdominal irradiation (IR) destroys the intestinal epithelial cells, leading to elevated permeabilization of the intestinal epithelial barrier, intestinal infection, and multiple-organ dysfunction syndromeCitation2.
Gut microorganisms, mainly composed of bacteria, fungi, viruses, and protists, co-exist and co-evolve with the hostCitation3. Gut microbiota, which is normally between 1010 and 1011 microbial cells per wet-weight gram of feces in the intestine, weights less than 500 g in an adultCitation4,Citation5. The number of intestinal flora is close to a ratio of 1:1 with that of human cellsCitation6. Based on the spatial distribution, gut microbiota is divided into mucus layer flora and intestinal cavity flora. Both play an important role in nutrient exchange, immune system development, signal transduction, and defenses against pathogen invasionCitation7,Citation8. Lactobacillus dominates in intestinal cavity bacteria, whereas Bacteroidia, Clostridia, and Akkermansia muciniphila (A. muciniphila) mainly colonize in the mucus layerCitation9.
By transplanting microbiota from mice with IR-induced intestine injury to germ-free and dextran sodium sulfate (DSS)-induced colitis mice, Gerassy-Vainberg et al. demonstrated that radiotherapy-induced dysbiosis contributes to the development of IR-induced intestinal damage and susceptibility of mice to intestinal inflammationCitation10. Cui et al. also reported that fecal microbiota transplantation (FMT) reduces the gastrointestinal toxicity caused by radiotherapy and protects the intestinal epithelia from radiotherapy-induced damageCitation11. Uribe et al. reported that Lactobacillus rhamnosus GG maintains intestinal homeostasis by increasing the expression of cyclooxygenase-2 (COX-2) and secretion of prostaglandin E2 in colonic myofibroblasts in a MyD88-dependent mannerCitation4. In addition, Lactobacillus was found to protect the intestinal epithelium from IR-induced injury by promoting the activation of toll like receptor 2 (TLR-2)/COX-2, release of lipoteichoic acid, activation of macrophage, and migration of mesenchymal stem cellsCitation5,Citation12. Together, current studies suggest that IR leads to intestinal dysbiosis, which possibly contributes to IR-induced intestinal epithelial damage. Correction of the intestinal dysbiosis could be an effective strategy for the treatment of IR-induced intestinal injuryCitation11.
A. muciniphila, a member of the Verrucomicrobia phylum, is a gram-negative anaerobic bacterium. It preferentially colonizes in mucins-rich intestinal mucus layers, in which it specifically degrades mucins as carbon, nitrogen and energy sources for itself and for the hostCitation13. A. muciniphila also degrades oligosaccharides in the milk to generate propionic acid to promote self-colonization. A. muciniphila-produced short-chain fatty acids (SCFAs) stimulate the growth and metabolism of bacteria that colonize in the mucus layers and prevent pathogenic bacteria passing through the mucus layers to destroy intestinal epithelial cells (IECs)Citation14. Thus, it plays a protective role in the maintenance of intestinal barrier integrity. However, it has not been explored whether A. muciniphila is altered in abdominal IR-induced intestinal damage and plays a role in the alleviation of the damage.
Metformin, a first line drug for the treatment of type II diabetes, was previously found to reduce the blood glucose of diabetic patients by maintaining a balance of gut commensal microorganismsCitation15,Citation16. We recently reported that metformin improved the composition of gut microbiota in patients receiving abdominal IRCitation17. Abundance of A. muciniphila was markedly increased in radiotherapy patients and in abdominal IR mice treated with metforminCitation17, indicating the potential application of metformin in abdominal IR subjects.
Although A. muciniphila, propionic acid, and metformin have individually been reported to decrease intestinal inflammation, it remains unknown whether these agents could alleviate radiation enteritis and if propionic acid plays a role in the mediation of the probiotic function of A. muciniphila. Additionally, the functional outcomes of metformin with the increase of A. muciniphila abundance and alleviation of radiation enteritis are undefined. In the current study, we identified that A. muciniphila was substantially decreased in the intestine of mice and in patients received abdominal IR. Administration of A. muciniphila alone was sufficient to lessen IR-induced intestinal injury in mice and prevented mice from IR enteritis-associated death. We presented evidence showing that propionic acid secreted by A. muciniphila mediated the protective effect. Metformin promoted the integrity of intestinal epithelial barrier and reduced radiation intestinal damage by elevating the abundance of A. muciniphila in intestine of abdominal IR mice. Together, our findings provide new insights for the prevention and treatment of IR-induced intestinal injury.
Results
Gut dysbiosis in patients with abdominal radiotherapy
To determine the impact of IR on intestinal flora, we collected fecal samples from cervical cancer patients with (IR-CC) or without (CC) abdominal radiotherapy and from age-matched healthy subjects (HC) for 16S rRNA sequencing. The diversity of the gut microbiota was markedly decreased in IR-CC based on the Shannon index analysis (). The abundance of intestinal flora was also reduced in IR-CC based on the Chao index analysis (). A relatively close distance between HC and CC was observed, suggesting that the composition of microbiota in the two groups bore a similar pattern, whereas the distance between IR-CC and CC was relatively farther, suggesting a less similarity in the composition of flora in the two groups (). Together, these results indicate that IR leads to the gut dysbiosis in cervical cancer patients.
Figure 1. Abundance of A. muciniphila is reduced in stool from cervical cancer patients with radiotherapy.
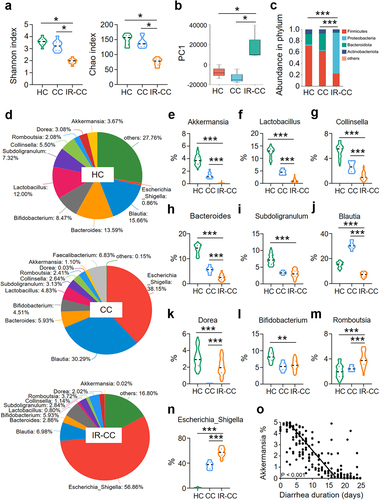
The composition of gut microbiota in IR-CC, CC, and HC was quite different (). Abundance of Proteobacteria was augmented, whereas that of Firmicutes and Bacteroidota were reduced in IR-CC, as compared with those in CC or HC (). At the genus level, the abundance of Romboutsia and Escherichia-Shigella was increased (), whereas the abundance of A. muciniphila, Lactobacillus, Collinsella, Bacteroides, and Blautia was decreased in IR-CC (). Among them, reduction of A. muciniphila was mostly substantial in IR-CC, as compared with that in CC or HC (). Similar results were obtained when we monitored the gut flora of patients with hepatocellular carcinoma and renal clear cell carcinoma with or without abdominal radiotherapy (Figure S1). We analyzed the correlation between the abundance of A. muciniphila and the duration of diarrhea in patients during a 30-day observation period after the end of abdominal radiation therapy, and found that the abundance of A. muciniphila was negatively correlated with the duration of diarrhea in IR patients (). Consistent with the alteration of microbiota in feces from radiotherapy patients, IR also led to changes in the composition of intestinal flora in mice, with a significant reduction in the abundance of A. muciniphila (Figure S2).
Supplement of A. muciniphila mitigates abdominal IR-induced intestinal damage
To determine whether the reduction of A. muciniphila plays a role in IR-induced intestinal damage, we pretreated the mice with 1 × 108 CFU of A. muciniphila gavage for 28 consecutive days before and 3 days after abdominal IR at 8 Gy. Five mice in each group were euthanized on day 3 post IR for the observation of intestinal damage. Ten mice in each group were used for the observation of murine survival until day 30 post IR (). As compared with the application of PBS (control), intragastric administration of A. muciniphila markedly increased the abundance of A. muciniphila in the intestine (Figure S3a). No changes in the morphology and weight of spleen and the number of peripheral blood cells were observed in abdominal IR mice regardless of the administration of A. muciniphila, indicating that abdominal IR did not affect the hematopoiesis of mice (Figure S3b-f). Supplementation of A. muciniphila improved the body weight and survival of IR mice as compared with those in PBS-treated IR mice (). Fecal weight and ileal length of IR mice remained stable in the exposure to A. muciniphila (), whereas the intake of food and water in IR mice was not affected ().
Figure 2. Supplement of A. muciniphila mitigates IR-induced intestinal damage.
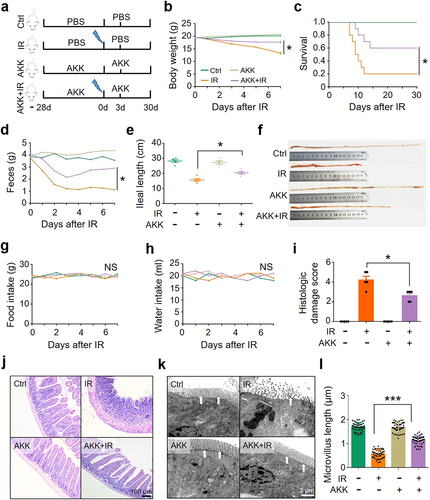
In histology examination and electron microscopy, intestinal epithelial cells (IECs) of IR mice were degenerated, necrotic, and shed. Inflammatory cell infiltration and ileal tissue damage were substantially increased (). Congested and swollen crypts and expanded lamina propria were observed. Relative to the treatment of PBS, administration of A. muciniphila substantially alleviated the ileal damage (), reduced tissue damage score of the intestine in IR mice (). Treatment of A. muciniphila maintained the microvillus length and reduced intestinal cell death (). Thus, we conclude that A. muciniphila possesses a radiation-protective effect on the intestine of IR mice.
We previously reported that Lactobacillus reduces IR-induced intestinal injuryCitation17,Citation18. Escherichia coli (E. coli), known as conditional pathogens, were found to negatively regulate IR-induced intestinal injuryCitation19. We found that, as compared with E. coli, A. muciniphila and Lactobacillus improved the body weight, survival, fecal weight, and ileal length, and reduced intestinal tissue damage of IR mice (Figure S3g-l). In addition, we found that A. muciniphila bore a better radioprotective effect against IR-induced intestinal damage in mice than Lactobacillus did (Figure S3g-l). To substantiate the radiation-protective effect of A. muciniphila, we established an intestinal flora-depleted model of mice by adding 4 antibiotics (ampicillin, metronidazole, neomycin, and vancomycin) in drinking water for 4 weeksCitation20,Citation21. Application of the 16S rRNA sequencing, we demonstrated that combination of the 4 antibiotics could effectively deplete intestinal microbiomeCitation18. We then administered A. muciniphila intragastrically to the mice for 4 weeks to reconstitute the microorganisms in the intestinal microenvironment (Figure S4a). The introduction of A. muciniphila slowed down the weight loss of mice (Figure S4b) and improved the survival of intestinal flora-depleted mice exposed to abdominal IR (Figure S4c). Consistently, the administration of A. muciniphila abrogated the decrease in fecal weight, alleviated the shortening of ileum (Figure S4d-f) and lessened ileal injury in IR mice with intestinal flora-depleted (Figure S4g, h). Collectively, these results support our notion that A. muciniphila is critical and sufficient to improve IR-induced intestinal injury and that the effect is A. muciniphila specific.
To further validate the finding that A. muciniphila alleviated IR-induced intestinal damage and promoted the recovery of mice, we compared the outcome of abdominal IR mice treated with live or pasteurization-inactivated A. muciniphila. It showed that viable, but not pasteurization-inactivated, A. muciniphila ameliorated abdominal IR-induced reduction in murine body weight, fecal volume, and ileal length (), alleviated intestinal tissue damage (), and promoted murine survival (). Our results indicate that viable A. muciniphila mitigates IR-induced intestinal damage, possibly through active substance(s) secreted by the microorganism, rather than the abundance of this bacterium.
Figure 3. Viable A. muciniphila alleviates IR-induced intestinal damage.
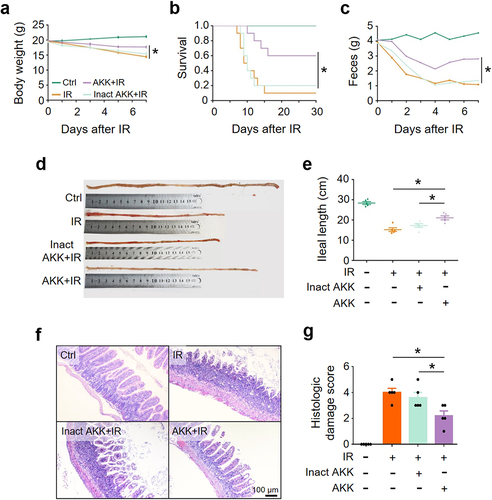
A. muciniphila reduces abdominal IR-induced intestinal damage through its secretion
To assess the possibility that active substances secreted by A. muciniphila mediate the radioprotective effect on intestine, we applied the supernatant of A. muciniphila culture or the control media to mice by gavage for 28 days followed by abdominal IR (Figure S5a). We found that the body weight, survival, fecal weight, ileal length, and ileal damage score were significantly improved in IR mice treated with supernatant of A. muciniphila culture, whereas no protective effects were observed in control media-treated IR mice (Figure S5b-h). In addition, we collected the intestine of mice for organoid culture and treated the intestinal organoids with supernatant of A. muciniphila culture. IR treatment markedly reduced the formation of intestinal organoids. The buds and sizes of intestinal organoids were substantially reduced due to the IR. Compared with treatment of control media, pretreatment with the supernatant of A. muciniphila culture markedly improved the survival of intestinal organoids exposed to IR. Reductions in the number and size of buds in the intestinal organoids resulted from IR exposure were revoked following the pretreatment of supernatant from A. muciniphila culture (Figure S5i, j). Taken together, these results indicate that supernatant from A. muciniphila culture promotes the survival of irradiated mice and protects intestinal organoids from IR-induced damage, supporting the notion that A. muciniphila plays a role in reducing IR-induced intestinal damage through active substance(s) it secreted.
A. muciniphila alleviates IR-induced intestinal damage by secreting propionic acid
A muciniphila degrades intestinal mucins to generate SCFAs as bioenergetics sources for itself and for the host, stimulates the colonization of mucus layer flora, and prevents pathogenic bacteria from entering into the mucus layer to destroy IECsCitation22,Citation23. To determine whether SCFAs mediate the protective effect of A. muciniphila against IR-induced intestinal damage, we analyzed SCFA level in the supernatant of A. muciniphila culture and in the sera and intestinal content of mice treated with or without A. muciniphila by gas chromatography-mass spectrometry. We found that levels of acetic acid, propionic acid, and isobutyric acid in the supernatant of A. muciniphila culture and in the serum and intestinal content of A. muciniphila-treated mice were substantially increased, whereas no difference was identified in the levels of butyric acid, isovaleric acid, valeric acid, and caproic acid ( and Figure S6).
Figure 4. A. muciniphila alleviates IR-induced intestinal damage by secreting propionic acid.
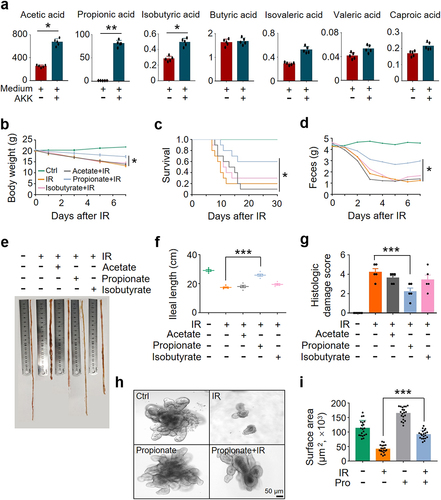
To identify the SCFA(s) secreted by A. muciniphila in preventing IR-induced intestinal damage, we evaluated the outcome of acetic acid, propionic acid, and isobutyric acid in the protection of IR-induced intestinal damage in mice. Two hundred µl of 60 mM acetate, propionate, or isobutyrateCitation24 were administered to mice by gavage for 28 days before IR. Application of propionate markedly slowed down the weight loss and promoted the survival of IR mice. In contrast, no radioprotective effect was found in mice treated with acetate, isobutyrate, or PBS (). Treatment with propionate, but not acetate, isobutyrate, or PBS, led to a substantial increase in fecal weight and ileal length and a reduction in intestinal damage score (). Administration of propionate generated a similar radioprotective effect on murine intestine as the gavage of A. muciniphila and its culture supernatant did (Figure S7), showing a markedly improved body weight, survival, ileal length, and intestinal damage score (Figure S7). In addition, we collected the intestine of mice for organoid culture. Compared with PBS treatment, pretreatment with propionate markedly improved the number and size of buds in intestinal organoids exposed to IR (), similar to those observed in irradiated organoids pretreated with the supernatant of A. muciniphila culture (Figure S5i, j). Collectively, our results support the notion that propionic acid is the mediator of A. muciniphila in mitigating IR-induced intestinal injury.
Propionic acid protects intestinal epithelial barrier from IR-induced damage by elevating tight junction proteins and mucin-2
To determine the mechanisms by which propionic acid alleviates IR-induced intestinal injury, we performed an RNA transcriptome sequencing of the intestine from IR mice after propionate treatment. We found that the mRNA levels of occludin (Ocln), ZO-1 (Tjp1), and mucin-2 (Muc2) were increased in the intestine of mice treated with propionate and IR as compared with those in IR mice (). Propionic acid binds to its receptor GRP43/41 on the surface of IECs and hence activates the transcription of tight junction genesCitation25,Citation26. Interestingly, transcriptome results showed that the mRNA level of GRP43 (Ffar2) was not altered in the intestine of mice treated with or without propionate in the presence or absence of abdominal IR (), which is consistent with previous reports demonstrating that expression of GRP43 is not affected by its ligandsCitation14,Citation27,Citation28.
Figure 5. Propionic acid promotes the expression of occludin, ZO-1, and MUC2 through enhancing histone acetylation.
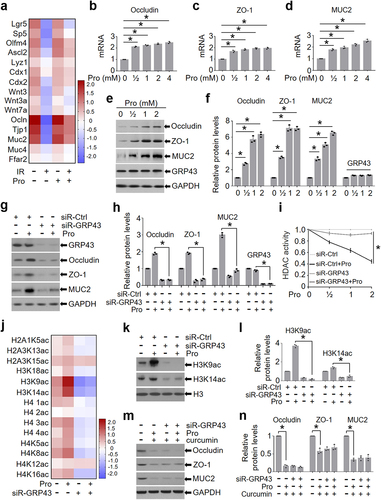
To validate the finding that propionate upregulated tight junction molecules and MUC2 in the intestine of IR mice (), we detected the expression of occludin, ZO-1, and MUC2 in intestinal organoids treated with propionate. Application of propionate increased the mRNA and protein levels of occludin, ZO-1, and MUC2 in a dosage-dependent manner (). Consistently, the expression of GRP43 (Ffar2) was not affected by propionate ().
To assess the necessity of GRP43/41 in propionate-induced upregulation of occludin, ZO-1, and MUC2, we knocked down GRP43 and GRP41 in the intestinal organoids and exposed the organoids to propionate. Depletion of GRP43, rather than GRP41 (Figure S8), markedly reduced the elevation of occludin, ZO-1, and MUC2 in propionate-treated organoids (). Taken together, these results suggest that propionic acid increases the expression of tight junction proteins and MUC2 in intestinal epithelia by upregulating their transcription, which is dependent on an intact GRP43.
Next, we tested the impact of propionate on the expression of occludin, ZO-1, and MUC2 in the murine intestine. Abdominal IR led to the destruction of tight junctions in the murine intestine (Figure S9a) and a substantial reduction of occludin, ZO-1, and MUC2 (Figure S9b-h). Application of propionate promoted the maintenance of tight junctions in the intestine of IR mice (Figure S9a) and increased the expression of occludin, ZO-1, and MUC2 (Figure S9b-h). Similarly, administration of A. muciniphila elevated the expression of occludin, ZO-1, and MUC2 in the intestine of mice (Figure S10).
Stable expression of occludin, ZO-1, and mucins facilitates intestinal barrier function and promotes the integrity of the intestinal epithelia. Thus, we further assessed the gut barrier function by administering FITC-dextran via gavage to mice 4 h prior to euthanize and measuring the concentration of FITC-dextran in the serum. As depicted in Figure S9i, administration of propionate resulted in a significant reduction of FITC-dextran in the serum of IR mice as compared with that in IR mice without propionate treatment, suggesting an improvement in the gut barrier integrity. Consistently, Periodic Acid-Schiff (PAS) staining, a method used to detect polysaccharides, glycoproteins, glycolipids, and mucins in tissues, showed that treatment with propionate restored the goblet cells in intestine, which was depleted by IR (Figure S9j, k), and maintained the mucus layer on the surface of intestinal villi (Figure S10j, l).
To validate the role of GRP43 in the mediation of propionate-promoted the integrity of the intestinal epithelial barrier, we detected the expression of tight junction proteins and MUC2 in the intestine of IR mice under the treatment with GRP43 regulators. IR mice pretreated with 4-CMTB (10 mg/kg/d, ip), a GRP43 agonist, bore a higher level of occludin, ZO-1, and MUC2 in the intestine of mice in the presence of propionate as compared with those in mice treated with propionate alone (Figure S11a, b). In contrast, pretreatment of GLPG0974 (1 mg/kg/d, oral gavage), a GRP43 inhibitor, abrogated propionate-promoted upregulation of occludin, ZO-1, and MUC2 in the intestine of IR mice (Figure S11c, d), reassuring the necessarity of GRP43 in the mediation of propionic acid-upregulated integrity of intestinal epithelial barrier in IR mice.
Propionic acid promotes the expression of occludin, ZO-1, and MUC2 by enhancing histone acetylation
One mechanism by which propionic acid regulates downstream targets is to enhance histone acetylation by activation of histone acetyltransferase (HAT) or inhibition of histone deacetylase (HDAC)Citation29,Citation30. Given that propionate promoted the expression of tight junction genes and MUC2 in the study (), we asked whether propionate upregulates these genes by promoting histone acetylation. To address the question, we treated siR-Ctrl- and siR-GRP43-transduced intestinal organoids with propionate and measured the activity of HDAC. As shown in , propionate treatment significantly reduced the activity of HDAC in siR-Ctrl-transduced organoids, but not in siR-GRP43-transduced organoids (). Histone proteomics analysis showed that propionate treatment enhanced histone acetylation of intestinal organoids, and the most significant changes were observed in H3K9ac and H3K14ac (). Western blot analysis confirmed that propionate treatment elevated the level of H3K9ac and H3K14ac in intestinal organoids, both of which were dependent on GRP43 expression (). In addition, we detected the level of histone acetylation in IECs of mice treated with the GRP43 agonist (4-CMTB) or the GRP43 inhibitor (GLPG0974) in the presence or absence of propionate and found that propionate combined with 4-CMTB increased, whereas combination of propionate with GLPG0974 reduced, the level of H3K9ac and H3K14ac in the intestine (Figure S12).
To further validate histone acetylation in the mediation of propionate-induced expression of tight junction proteins and MUC2, we exposed intestinal organoids to 20 μmol/L curcumin, a histone acetyltransferase inhibitorCitation31, to reduce histone acetylation. We found that propionate no longer impacted the levels of occludin, ZO-1, and MUC2 in intestinal organoids in the presence of curcumin (), emphasizing the importance of histone acetylation in propionate-induced expression of tight junction proteins and mucin. Taken together, our results suggest that enhanced histone acetylation by propionate treatment upregulates the transcription of tight junction proteins and mucins in intestinal epithelia through GRP43, which may eventually be beneficial to the integrity of intestinal epithelia.
Metformin increases the abundance of A. muciniphila in abdominal IR mice
Metformin is the most widely used drug for the treatment of type 2 diabetesCitation32,Citation33. It was reported that metformin promotes glucose sensing in small intestine by regulating gut microbiotaCitation34. To determine whether metformin plays a role in the maintenance of gut microbiota balance in IR mice, we performed a 16S rRNA sequencing on intestinal flora of abdominal IR mice treated with metformin. We found that metformin improves the composition of gut microbiota in abdominal IR mice and Lactobacillus is the most abundant bacteria in the intestine of mice treated with metformin, whereas A. muciniphila is the bacterium with the highest fold increase after metformin treatment (Figure S13a-i). In addition, application of metformin mitigated IR-induced intestinal injury (Figure S13j-m) and reduced intestinal damage score in abdominal IR mice (Figure S13n).
Since A. muciniphila was increased with highest fold in metformin-treated mice, we determined the role of A. muciniphila in the mediation of metformin-alleviated intestinal damage in IR mice. We reconstituted the intestinal microbiota of microbiota-depleted (Abx) mice and germ-free (GF) mice with A. muciniphila and treated the mice with metformin. After reorganization of A. muciniphila, administration of metformin increased villus height, crypts depth and mucus depth () and reduced intestinal damage score in abdominal IR mice (), compared with Abx+IR mice and GF+IR mice treated with metformin. Thus, our data demonstrated that A. muciniphila was necessary and sufficient for metformin-induced radioprotection in intestine ().
Figure 6. A. muciniphila is necessary and sufficient for metformin-induced radioprotection in intestine.
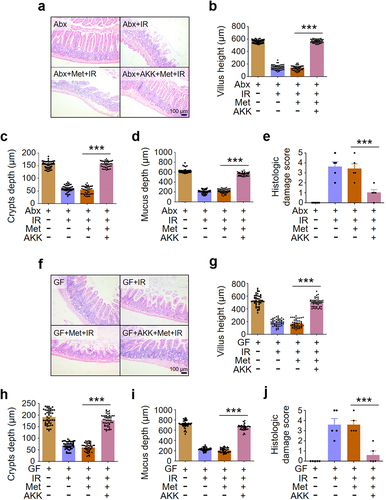
Metformin enhances the integrity of intestinal epithelial barrier
To determine whether metformin-reduced intestinal damage in abdominal IR mice is mediated by the maintenance of intestinal epithelial barrier integrity, we detected the level of occludin, ZO-1, and MUC2 in intestinal epithelia in mice treated with metformin. Expressions of occludin, ZO-1, and MUC2 in the intestine of abdominal IR mice treated with metformin were increased as compared with those in IR mice treated with PBS (). Consistent with these results, metformin+IR mice exhibited a decrease in serum FITC-dextran levels, a biomarker for intestinal permeability, as compared with IR mice (). The number of goblet cells in the ileum of IR mice was upregulated after metformin treatment (). The mucus layer area on the surface of the ileum was also increased (). These results suggest that metformin not only increases the abundance of A. muciniphila in the intestine, but also enhances the intestinal barrier function and minimizes IR-induced intestinal injury.
Figure 7. Metformin enhances intestinal barrier function.
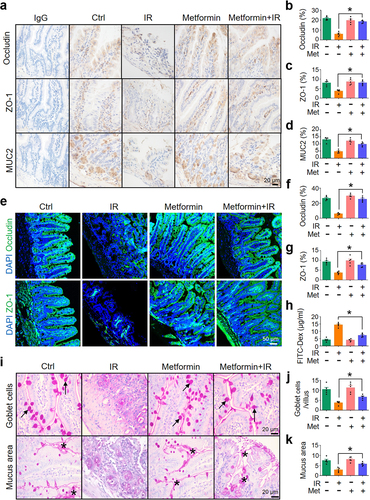
To assess the role of GRP43 in metformin-maintained the integrity of the intestinal epithelial barrier, we treated the mice with metformin in the presence or absence of GRP43 inhibitor (GLPG0974). Inhibition of GRP43 markedly abrogated the upregulation of occludin, ZO-1, and MUC2 in the intestine of metformin-treated mice (Figure S14a, b). In addition, administration of GLPG0974 also reduced the level of H3K9ac and H3K14ac in the intestine of metformin-treated mice (Figure S14c, d). To further validate histone acetylation in the mediation of metformin-induced expression of tight junction proteins and MUC2, mice were treated with metformin and 20 mg/kg/d curcumin (a histone acetyltransferase inhibitor) by gastric gavages for 7 days before and an additional 3 days after IR for a total of 10 days to suppress histone acetylation. In this case, metformin-induced upregulation of occludin, ZO-1, and MUC2 in the intestine of mice were substantially mitigated due to the co-treatment with curcumin (Figure S14e, f), supporting our notion that histone acetylation mediated metformin-upregulated expression of tight junction proteins and MUC2. Taken together, our results suggest that metformin (and propionate) treatment enhances histone acetylation through GRP43, and hence upregulates the transcription of tight junction proteins and MUC2 in intestinal epithelia, which eventually contributes to the maintenance of integrity of intestinal epithelial barrier.
Discussion
A muciniphila is relatively insensitive to oxygen, and survives under microaerobic conditions to generate additional energyCitation35. This feature makes the species in a more advantageous position in the competition with other strict anaerobic bacteria in the intestinal tract. There are at least 12 subspecies of Akkermansia colonized in human intestineCitation22, among which A. muciniphila is the most common and abundant AkkermansiaCitation36. We showed that the diversity and abundance of intestinal flora were decreased in IR-CC patients compared with CC or HC, indicating that IR led to intestinal dysbiosis in patients. The abundance of A. muciniphila was negatively correlated with the diarrhea duration of patients. Moreover, in the current study, we successfully established abdominal IR-induced murine intestinal damage model and analyzed microbial diversity of ileal contents using 16S rRNA sequencing. We demonstrated that abdominal IR led to the imbalance of ileal flora toward reduced diversity. A. muciniphila was the most severely decreased genus in mice exposed to abdominal IR (Figure S2b). Our investigation showed that the imbalance of intestinal florain particular, the reduction of A. muciniphila mediated IR-induced intestinal damage. Administration of A. muciniphila alleviated IR-induced intestinal injury and improved the survival of irradiated mice (), validating its protective role in mice.
A muciniphila prefers to colonize in the mucin-rich intestinal mucus layer, where it specifically degrades mucins to produce SCFAs as carbon, nitrogen, and bioenergetic sources for flora in the intestinal mucus layer and for the host. The whole mucus layer is in a dynamic homeostasisCitation14. The outer loose mucus layer in the intestinal cavity is derived from the inner mucus layer and prone to falling off and degradation. The inner mucus layer is replenished by mucins secreted by goblet cells. A. muciniphila participates in this dynamic process and plays a critical role in the maintenance of the integrity of the mucus layer (Figure S15). On the one hand, A. muciniphila uses mucins as a nutrient source and increased colonization of the bacterium in mucus layer accelerates the mucin degradationCitation37. On the other hand, A. muciniphila is able to positively regulate the thickness of mucus. It is possible that, it degrades mucins to produce SCFAs, which act as an energy source for host epithelial cells to synthesize and secrete mucus proteins. Furthermore, mucin degradation could stimulate goblet cells to produce more new mucins, which promote the growth and colonization of A. muciniphila. Thus, a positive feedback loop maintains continuous renewal of the intestinal mucus layer, rendering a protective role in intestinal epithelial injury (Figure S15). Indeed, our findings support the hypothesis and demonstrate that application of A. muciniphila led to increased numbers of goblet cells, promoted the expression of MUC2 in the intestine of IR mice, and extended the mucus layer thickness (Figure S9j-l), which eventually strengthened intestinal epithelial barrier and reduced IR-induced intestinal damage. In short, our data provide strong evidence demonstrating that A. muciniphila promotes the integrity of intestinal barrier in humans and animals.
Microbial-generated SCFAs are readily absorbed in the colon, participate in the repair of the wounded epithelium, and regulate glucose and lipid metabolism of the hostCitation14. Thus, SCFAs exert a protective effect against bacterial pathogens by maintaining the integrity of the epithelial barrierCitation38. In the current study, we identified that the function of A. muciniphila in the protection of IR-induced intestinal damage is to facilitate the generation of high levels of SCFAs ( and S6). We further demonstrated that propionic acid but not acetic acid, butyric acid, or other SCFAs, secreted by A. muciniphila, mediated the function of the probiotic. Therefore, A. muciniphila may not only play a protective role against bacterial pathogens in physiology but also bear a pathophysiological function in the pathogenesis of certain diseases, such as irradiation-induced intestinal damage (this study) and inflammatory bowel diseases (IBD)Citation38.
Recent evidence points to a direct role for the GRP43, GRP41, and GRP109A receptors in mediating the protective roles of SCFAs in IBDCitation38,Citation39, as well as in controlling the production of cytokines and chemokines by IECsCitation38. GRP43, a G-protein-coupled receptor, is mainly activated by acetic acid and propionic acidCitation40. We found that propionate promoted the expression of tight junction proteins occludin and ZO-1 and that GRP43 was necessary for conveying the propionate signal (). These tight junction proteins are located in the most apical side of the basolateral membrane, forming the paracellular pathways that regulate the passage of ions, solutes, bacteria, and toxins across the epithelial monolayer and are also responsible for maintaining/imparting cell polarityCitation41. The integrity of the tight junction structure largely depends on the expression levels of tight junction proteinsCitation41. Thus, findings in the current study that A. muciniphila upregulates tight junction proteins occludin and ZO-1 through the secretion of propionic acid provide another layer of evidence demonstrating that A. muciniphila is critical in the maintenance of the integrity of intestinal epithelial barrier and in the prevention of IR-induced intestinal injury.
Guo et al. identified a population of mice that recovered from high-dose radiation to live normal life spans harbor distinct gut microbiota and metabolites. Commensal-associated propionic acid suppresses radiation-induced death and intestinal and hematopoietic damage of miceCitation42. Although propionic acid has individually been reported to decrease intestinal inflammation, it remains unknown how propionic acid alleviates radiation enteritis and if propionic acid plays a role in A. muciniphila-mitigated intestinal damage. In this study, we reported that abundance of A. muciniphila was markedly reduced in the intestine of mice exposed to abdominal IR and in feces of patients who received abdominal radiotherapy. Analyzing the metabolic products of A. muciniphila revealed that propionic acid mediated the radioprotective effect. It is generally believed that SCFAs induce physiological effects by altering the epigenetic state of cellsCitation30. With a histone proteomics analysis and a following-up validation assay, we found that propionate treatment substantially enhanced histone acetylationin particular, H3K9ac and H3K14ac, which is dependent on an intact expression of GRP43 (). Furthermore, we found that propionate no longer upregulated the levels of occludin, ZO-1, and MUC2 in intestinal organoids in the presence of curcumin, a histone acetyltransferase inhibitor, which reduces histone acetylation (), implying the importance of histone acetylation in propionic acid-induced expression of tight junction proteins and MUC2. Thus, we propose that A. muciniphila-secreted propionic acid acts on its receptor GRP43 and enhances histone acetylation, which leads to the upregulation of tight junction proteins occludin and ZO-1 and eventually contributes to the maintenance of intestinal epithelial barrier integrity. The production of adequate and balanced SCFAs by healthy gut microbiota, such as A. muciniphila, is an important factor for the prevention of IR-induced intestinal epithelial barrier damage and avoidance of its resultant damages to the intestine.
A. muciniphila is a novel probiotic, which has not been characterized extensively. Interactive microbe in the gut and agents that regulate the abundance of A. muciniphila remain largely undefined. Metformin is a first line drug for the treatment of type II diabetes. Reports showed that metformin increases the abundance of gut microbiota in diabetes patientsCitation43. Metformin was previously reported to reduce radiation-induced lung injury, alleviate pulmonary fibrosis and inflammatory infiltration, and hence bear a potential for the application in radioprotectionCitation44. Chen et al. reported metformin alleviated radiation intestinal injury by optimizing mitophagy in an AMPK-dependent mannerCitation45. In the present study, we found that metformin modulated the microbiota composition in the ileum of abdominal IR mice. In particular, A. muciniphila in the intestine was markedly elevated by metformin (Figure S13). We reconstituted the intestinal microbiota of microbiota-depleted (Abx) and GF mice with A. muciniphila and demonstrated that A. muciniphila was necessary and sufficient for metformin-induced radioprotection in the intestine. We further exhibited that application of metformin prior to and post IR reduced the death of IECs and increased intestinal villus height, crypts depth, and mucus layer thickness. In addition, treatment of metformin enhanced the level of tight junction proteins occludin and ZO-1, elevated the number of goblet cells, increased MUC2, and enlarged the mucus layer (Figure S15). Thus, our data endorse a robust role of metformin in the protection of the intestine from IR-induced damage by increasing the abundance of A. muciniphila and by enhancing the intestinal barrier integrity.
In summary, in the current study, we identified that A. muciniphila was the most severely decreased microorganism in the intestine upon abdominal IR. Administration of A. muciniphila to abdominal IR mice substantially mitigated intestinal damage and prevented mice from IR-induced death. We further demonstrated that metformin increased the abundance of A. muciniphila, which in turn upregulated the levels of SCFAs in the intestine and in the serum, protected the integrity of intestinal epithelial barrier, and hence mitigated abdominal IR damage. These studies shed new light on the application of probiotics or their regulators, such as metformin, in the protection of radiation exposure-damaged intestine.
Materials and Methods
Collection of stool from patients with cervical cancer
We collected feces from cervical cancer patients registered in the Department of Gynecology of Jiangsu Cancer Hospital and underwent abdominal radiotherapy. The irradiation dose was 2 Gy/time, 5 times/week, for 5 consecutive weeks. Inclusion criteria: (1) 30 ~ 60 years old; (2) receiving abdominal radiation therapy; (3) with or without clinical manifestations of radiation-induced intestine injury (tenesmus, diarrhea, rectal bleeding, mucus and fecal incontinence, etc.). Exclusion criteria: radiotherapy was interrupted; inflammatory bowel diseases; tumor involving the bowel; antibiotic administration during radiation therapy; diabetes. Fecal samples from 87 female patients with abdominal IR were collected for 16S rRNA sequencing. In addition, 83 healthy female subjects and 78 female cervical cancer patients without abdominal IR, aged between 30 and 60, were recruited locally, and their stool samples were collected to serve as controls. In addition, we also collected stool samples from hepatocellular carcinoma (n = 26 in LIHC and n = 27 in IR-LIHC) and renal clear cell carcinoma (n = 29 in KIRC and n = 23 in IR-KIRC) patients with or without abdominal IR. Informed consent was obtained from the participants for collecting the stool from patients. Patients’ sample collection and use were approved by the ethical committee of Jiangsu Cancer Hospital and Henan University.
Establishment of radiation injury model in mice
Experiments involving mice were under the regulation of ethical requirements and approved by the institutional animal care and use committee of Henan University in China.
Consistent with the gender of cervical cancer patients, we selected healthy BALB/c female mice for the study. The mice were housed in animal barrier facility in Henan University on a 12 h light-dark cycle with food and water available ad libitum. Mice were randomly assigned into cages for control or IR treatment in the presence or absence of metformin, or for the collection of feces. Eight Gy one-time abdominal IR with a dose rate of 1.0 Gy/min by X-Ray was appliedCitation46. Five mice in each group were euthanized on day 3 post IR for the observation of intestinal damage. Ten mice in each group were used for monitoring mouse survival until day 30 post IR. Each mouse was fed and housed separately and data were generalized from single cage. Each experiment was repeated at least 3 times to ensure the stability and repeatability of the experiment.
Metformin was intragastrically administered at a dose of 250 mg/kg/dCitation47. Mice were treated with metformin continuously for 7 days before and additional 3 days after IR for a total of 10 days.
Collection of feces and ileal contents from mice
Fresh feces from mice were collected after IR. Thirty mg of feces were kept in sterilized Eppendorf tubes and maintained in −80°C. After the mice were euthanized, ileocecal area in abdominal cavity was located. Sixty mg of mucosa and intestinal content within 5 cm-distal ileum were collected by scrapingCitation18.
16S rRNA sequencing
The sequencing was performed by using the Illumina Hiseq platform (Meiji Biology Company, Shanghai, China). DNAs in the intestinal contents of mice were extracted with a DNA extraction kit (GenElute™ Stool DNA Isolation Kit, Sigma-Aldrich), and the purity and concentration of DNA extracted were detected by a ultraviolet spectrophotometer and subjected to 1% agarose gel electrophoresis to determine the integrity of DNA. After DNA was qualified, PCR amplification was carried out, and 16S rRNA gene V3-V4 region was amplified. PCR amplification primers for V3-V4 region were 338 F (ACTCCTACGGGAGGCAGCAG) and 806 R (GGACTACHVGGGTWTCTAAT)Citation48. The amplified sample was visualized by 2% agarose gel electrophoresis. PCR products at target band size were subject to high-throughput sequencing using the Illumina Hiseq platform (Meiji Biology Company, China). Sequences analysis was performed through Uparse software. ≥97% similarity of sequences were classified to the same OTUs (operational taxonomic units). A representative sequence for each OTU was selected for annotation. For each representative sequence, the Silva123 database was applied to annotate taxonomic information according to the RDP classifier algorithm.
Quantitative detection of short chain fatty acids (SCFAs) by gas chromatography – mass spectrometry (GC-MS)
Both the qualitation and quantitation of SCFAs were processed by Metware Biotechnology Co., Ltd (Wuhan, China). The measurement was performed following the protocol previously reportedCitation49. Briefly, Agilent 7890B gas chromatograph coupled to a 7000D mass spectrometer was applied for the detection. A DB-5 MS column was used for the detection. Helium was used as carrier gas, at a flow rate of 1.2 mL/min. Injections were made in the splitless mode and the injection volume was 2 μL. Samples were analyzed in multiple reaction monitoring mode. SCFAs mixtures were prepared to construct the calibration curves.
Intestinal organoid culture
6–8 week-old BALB/C mice were euthanized by decapitation. 10 cm of terminal ileum was taken immediately and washed with pre-cooled (4°C) PBS. Adipose tissue and mesentery in the intestine were removed. Intestinal contents were washed away with 4°C pre-chilled PBS. After the ileum was cut into 2 mm pieces, pipetting was repeated 6 times with PBS. The supernatant was removed after the tissue was precipitated. EDTA-containing PBS was added to precipitates and incubated for 30 min at 4°C on a shaker. After incubation, pipetting was repeated 6 times with PBS until the crypts are dispersed. 200–500 crypts per well were suspended in Matrigel (Corning). Complete ENR medium (all components from Thermo Fisher Scientific) consisted of advanced DMEM/F12 (Gibco), antibiotic and antifungal (×100), 1 mM N-acetylcysteine (Sigma-Aldrich), B27 Supplement, N2 Supplement, EGF, Noggin (R&D Systems), R-spondin-1-conditioned medium was added for the culture. The ENR medium was replaced every 2 to 3 days. The surface area of intestinal organoids was measured from non-overlapping photographs of the organoids randomly taken in a well using an inverted microscope (Carl Zeiss). Each photo was analyzed using ImageJ software (NIH) and the Zen image program (Carl Zeiss)Citation50.
Hematoxylin-eosin (HE) staining and immunohistochemistry (IHC)
The paraffin sections were placed in xylene for overnight. Complete dewaxing with fresh xylene and graded alcohol hydration were carried out on the following day. The slides were then stained with HECitation51. Additional sections were used to probe the expression of occludin, ZO-1, and MUC2 in ilea by IHC using antibodies from Abcam (USA) following thermal antigen retrieval. Endogenous peroxidase blocking, antibody action, and development were performed as reportedCitation51. Quantification of positive areas in intestinal epithelial cells was completed using IHC plugin of ImageJ softwareCitation52,Citation53.
Immunofluorescence
Ileal tissues from mice were snap-frozen for immunostaining. Tissue sections were fixed with 4% paraformaldehyde in PBS for 30 minutes, followed by blocking with 5% normal goat serum (NGS) for 2 h. Antibodies against ZO-1 (Abcam, USA) and occludin (Abcam, USA) were incubated at 1:100 dilution in PBS with 1% NGS and incubated overnight at 4°C. The sections were mounted using Slowfade Gold antifade with DAPI reagent (Invitrogen), followed by imaging with LSM 8000 laser confocal microscope (Carl Zeiss AG).
Histone proteomics
Cells were trypsinized, washed once with PBS, and then histones were extracted and derivatized. Derivatized histone peptides were injected into a Dionex Ultimate 3000 nanoflow HPLC using a Waters nanoAcquity UPLC C18 column (100 m × 150 mm, 3 m) and connected to a Thermo Fisher Q-Exactive mass spectrometer at 700 nl/min. UPLC MS analysis was then performed as described by Thomas et al.Citation30. Data were collected using data independent acquisition (DIA) mode.
Electron microscopy
The mouse ilea were rinsed with physiological saline, rapidly immersed in glutaraldehyde solution (4%) for pre-fixation. The tissues were then subjected to TEM biological sample preparation to complete the sample rinsing, post-fixation, rinsing, block dyeing, gradient dehydration, infiltration, embedding, ultra-thin slicer sectioning, positive staining, and electron microscopy observationCitation54.
Periodic acid-schiff (PAS) staining
The paraffin sections were dewaxed and hydrated as described above. The slides were stained with 1% periodic acid for 10 minutes. After washing thoroughly with distilled water, the slides were stained with Schiff reagent for 30 minutes. Slides were then rinsed in lukewarm tap water, counterstained with HE, dehydrated, and mounted with a synthetic mount medium. The area of mucus in the intestine was measured by taking 5 random non-overlapping photographs of the intestine using a light microscope (n = 5 per group)Citation55. Each photo was analyzed using ImageJ software (NIH) and the Zen image program (Carl Zeiss) for calculating the area of mucus.
Histological injury score
Intestinal epithelial injury was classified using the Chiu’s methodCitation56: 0, normal intestinal mucosal villi; 1, capillary hyperemia and cystic gaps under the epithelium at the villus apex; 2, cystic gaps enlarged under the epithelium, edema extended in the lamina propria, and central cheliferous vessels dilated; 3, degeneration and necrosis of IECs, severe edema in the lamina propria, and rarely seen abscission in villus apexes; 4, degeneration, necrosis, and exfoliation of IECs, hyperemia, dilation of capillary, uncovering of lamina propria, abscission in some villi, and 5, bleeding, ulceration, disintegration of the lamina propria, and abscission of villiCitation57.
FITC dextran assay
Fluorescein isothiocyanate (FITC)-labeled dextran (FD4, Sigma-Aldrich, USA) was used for the measurement of gut mucosal permeability as described previouslyCitation58. PBS-diluted FITC-dextran (500 mg/kg) was administered to mice via oral gavage. Mice were euthanized after 4 h, and serum was collected by cardiac puncture, centrifuging at 10,000 g for 10 min. The FITC-dextran concentration in the serum was determined based on a standard calibration curve.
Establishment of intestinal microbiota-depleted mice
Mice were treated with a mixture of four antibiotics in drinking water for 4 weeksCitation59. The antibiotic mixture consists of ampicillin (200 mg/L), metronidazole (200 mg/L), neomycin (200 mg/L), and vancomycin (100 mg/L), which were purchased from MedChemExpress, China. The presence of microbes in the feces of mice was determined by 16S rRNA.
Culture and pasteurization of A. muciniphila
A. muciniphila was purchased from the American Type Culture Collection (BAA-835) and cultured in brain heart infusion media (BD Bioscience) supplemented with 0.4% mucin (Sigma) under strict anaerobic conditions at 37°CCitation60. Cultures were centrifuged and the culture pellet was suspended in PBS. 1 × 108 CFU of A. muciniphila in 0.2 mL PBS or PBS alone was intragastrically administered into mice for 4 weeks continuously to re-organize the intestinal microenvironment before IR. In separate experiments, aliquots of A. muciniphila culture were inactivated by pasteurization for 30 min at 70°CCitation60. No viable A. muciniphila could be recovered. Administration of active or inactivated A. muciniphila was conducted as described.
Statistical analysis
SPSS 17.0 was used for statistical analysis of experimental data. One-way ANOVA and t-test were used for the significant test. P < 0.05 (* P < 0.05, ** P < 0.01, *** P < 0.001) was defined as statistically significant, whereas P > 0.05, the experimental results were set to be statistically insignificant.
Authors’ contributions
Yong-Ping Jian, Kai-Yue He, Xin-Yuan Lei, and Dan-Hui Wu designed the study, performed the experiments, and wrote the manuscript. Lei Zhang, Jun-Qi Li, Qiu-Tong Li, Wei-Tao Yin, Zi-Long Zhao, Huai Liu, Xiong-Yan Xiang, Ling-Jun Zhu, Cui-Yun Cui, Qian-Hui Sun, and Ke-Ke Wang performed the experiments. Zhi-Xiang Xu, Jin-Hua Wang, Lin Lv, and Guo-Long Liu contributed to the conception and writing. All authors read and approved the final manuscript.
Availability of data and materials
The authors declare that all the data supporting our findings in the study are available within the paper and its supplementary information files.
Abbreviations
A. muciniphila | = | Akkermansia muciniphila |
Abx | = | microbiota-depleted |
COX-2 | = | cyclooxygenase-2 |
GRP | = | G-protein coupled receptor |
IBD | = | inflammatory bowel disease |
IHC | = | immunohistochemistry |
IR | = | irradiation |
MUC2 | = | mucin 2 |
PAS | = | Periodic Acid-Schiff |
SCFAs | = | short-chain fatty acids |
TLR-2 | = | toll like receptor 2 |
ZO-1 | = | zonula occludens-1 |
Ethical approval and consent to participate
Protocols for animal usage were approved by the institutional animal care and use committee (IACUC) at Henan University, China. All animal experiments were conducted on the basis of the institutional guidelines, and were approved by the Laboratory Animal Center of Henan University. Collection and usage of patients’ feces were approved by the ethical committee of Jiangsu Cancer Institute and Henan University.
Consent for publication
All authors consent to submit and publish this article.
Supplemental Material
Download Zip (12.6 MB)Acknowledgments
This work was supported by the National Natural Science Foundation of China (Nos. 82020108024 and 82200596), the National Key Research and Development Program of China (2023YFE0109800), the China Postdoctoral Science Foundation (No. 2022M721014) and the Scientific and Technological Research Project of Henan Province (No. 232102311034).
Disclosure statement
No potential conflict of interest was reported by the author(s).
Supplementary material
Supplemental data for this article can be accessed online at https://doi.org/10.1080/19490976.2023.2293312.
Additional information
Funding
References
- Jian YP Y, Zhang MD, Liu M, Wang Y, Xu Z-X The impact of gut microbiota on radiation-induced enteritis. Front Cell Infect Microbiol. 2021;11:586392. doi:10.3389/fcimb.2021.586392.
- Andreyev HJ, Wotherspoon A, Denham JW, Hauer-Jensen M. Defining pelvic-radiation disease for the survivorship era. Lancet Oncol. 2010;11(4):310–23. doi:10.1016/S1470-2045(10)70026-7.
- Sender R, Fuchs S, Milo R. Are we really vastly outnumbered? Revisiting the ratio of bacterial to host cells in humans. Cell. 2016;164(3):337–340. doi:10.1016/j.cell.2016.01.013.
- Uribe G, Villéger R, Bressollier P, Dillard RN, Worthley DL, Wang TC, Powell DW, Urdaci MC, Pinchuk IV. Lactobacillus rhamnosus GG increases cyclooxygenase-2 expression and prostaglandin E2 secretion in colonic myofibroblasts via a MyD88-dependent mechanism during homeostasis. Cellular Microbiology. 2018;20(11):12871. doi:10.1111/cmi.12871.
- Ciorba MA, Riehl TE, Rao MS, Moon C, Ee X, Nava GM, Walker MR, Marinshaw JM, Stappenbeck TS, Stenson WF, et al. Lactobacillus probiotic protects intestinal epithelium from radiation injury in a TLR-2/cyclo-oxygenase-2-dependent manner. Gut. 2012;61(6):829–838. doi:10.1136/gutjnl-2011-300367.
- Walker AW, Hoyles L. Human microbiome myths and misconceptions. Nat Microbiol. 2023;8(8):1392–1396. doi:10.1038/s41564-023-01426-7.
- Pryor R, Norvaisas P, Marinos G, Best L, Thingholm LB, Quintaneiro LM, De Haes W, Esser D, Waschina S, Lujan C, et al. Host-Microbe-Drug-Nutrient Screen Identifies Bacterial Effectors of Metformin Therapy. Cell. 2019;178(6):1299–312.e29. doi:10.1016/j.cell.2019.08.003.
- Lee Y, Sugihara K, MG G, Jon S, Kamada N, Moon JJ. Hyaluronic acid–bilirubin nanomedicine for targeted modulation of dysregulated intestinal barrier, microbiome and immune responses in colitis. Nat Mater. 2020;19(1):118–26. doi:10.1038/s41563-019-0462-9.
- Duncan K, Carey-Ewend K, Vaishnava S. Spatial analysis of gut microbiome reveals a distinct ecological niche associated with the mucus layer. Gut Microbes. 2021;13(1):1–21. doi:10.1080/19490976.2021.1874815.
- Gerassy-Vainberg S, Blatt A, Danin-Poleg Y, Gershovich K, Sabo E, Nevelsky A, Daniel S, Dahan A, Ziv O, Dheer R, et al. Radiation induces proinflammatory dysbiosis: transmission of inflammatory susceptibility by host cytokine induction. Gut. 2018;67(1):97–107. doi:10.1136/gutjnl-2017-313789.
- Cui M, Xiao H, Li Y, Zhou L, Zhao S, Luo D, Zheng Q, Dong J, Zhao Y, Zhang X, et al. Faecal microbiota transplantation protects against radiation-induced toxicity. EMBO Mol Med. 2017;9(4):448–461. doi:10.15252/emmm.201606932.
- Riehl TE, Alvarado D, Ee X, Zuckerman A, Foster L, Kapoor V, Thotala D, Ciorba MA, Stenson WF. Lactobacillus rhamnosus GG protects the intestinal epithelium from radiation injury through release of lipoteichoic acid, macrophage activation and the migration of mesenchymal stem cells. Gut. 2019;68(6):1003–13. doi:10.1136/gutjnl-2018-316226.
- Bae M, Cassilly CD, Liu X, Park SM, Tusi BK, Chen X, Kwon J, Filipčík P, Bolze AS, Liu Z, et al. Akkermansia muciniphila phospholipid induces homeostatic immune responses. Nature. 2022;608(7921):168–173. doi:10.1038/s41586-022-04985-7.
- Liu MJ, Yang JY, Yan ZH, Hu S, Li JQ, Xu ZX, Jian Y-P. Recent findings in Akkermansia muciniphila-regulated metabolism and its role in intestinal diseases. Clin Nutr. 2022;41(10):2333–44. doi:10.1016/j.clnu.2022.08.029.
- Wu H, Esteve E, Tremaroli V, Khan MT, Caesar R, Mannerås-Holm L, Ståhlman M, Olsson LM, Serino M, Planas-Fèlix M, et al. Metformin alters the gut microbiome of individuals with treatment-naive type 2 diabetes, contributing to the therapeutic effects of the drug. Nat Med. 2017;23(7):850–858. doi:10.1038/nm.4345.
- Sun L, Xie C, Wang G, Wu Y, Wu Q, Wang X, Liu J, Deng Y, Xia J, Chen B, et al. Gut microbiota and intestinal FXR mediate the clinical benefits of metformin. Nat Med. 2018;24(12):1919–1929. doi:10.1038/s41591-018-0222-4.
- Yang JY, Liu MJ, Lv L, Guo JR, He KY, Zhang H, Wang K-K, Cui C-Y, Yan B-Z, Du D-D, et al. Metformin alleviates irradiation-induced intestinal injury by activation of FXR in intestinal epithelia. Front Microbiol. 2022;13:932294. doi:10.3389/fmicb.2022.932294.
- Jian YP, Yang G, Zhang LH, Liang JY, Zhou HL, Wang YS, Xu Z-X. Lactobacillus plantarum alleviates irradiation-induced intestinal injury by activation of FXR-FGF15 signaling in intestinal epithelia. J Cell Physiol. 2022;237(3):1845–56. doi:10.1002/jcp.30651.
- Laganenka L, López ME, Colin R, Sourjik V, Groisman EA. Flagellum-mediated mechanosensing and RflP control motility state of pathogenic Escherichia coli. mBio. 2020;11(2):e02269–19. doi:10.1128/mBio.02269-19.
- Yang D, Chen X, Wang J, Lou Q, Lou Y, Li L, Wang H, Chen J, Wu M, Song X, et al. Dysregulated lung commensal bacteria drive interleukin-17B production to promote pulmonary fibrosis through their outer membrane vesicles. Immunity. 2019;50(3):692–706.e7. doi:10.1016/j.immuni.2019.02.001.
- Castellanos JG, Woo V, Viladomiu M, Putzel G, Lima S, Diehl GE, Marderstein AR, Gandara J, Perez AR, Withers DR, et al. Microbiota-induced TNF-like ligand 1A drives group 3 innate lymphoid cell-mediated barrier protection and intestinal T cell activation during colitis. Immunity. 2018;49(6):1077–89.e5. doi:10.1016/j.immuni.2018.10.014.
- Derrien M, Vaughan EE, Plugge CM, de Vos WM. Akkermansia muciniphila gen. nov., sp. nov., a human intestinal mucin-degrading bacterium. Int J Syst Evol Microbiol. 2004;54(5):1469–1476. doi:10.1099/ijs.0.02873-0.
- Derrien M, Collado MC, Ben-Amor K, Salminen S, de Vos WM. The mucin degrader Akkermansia muciniphila is an abundant resident of the human intestinal tract. Appl Environ Microb. 2008;74(5):1646–1648. doi:10.1128/AEM.01226-07.
- He Y, Fu L, Li Y, Wang W, Gong M, Zhang J, Dong X, Huang J, Wang Q, Mackay CR, et al. Gut microbial metabolites facilitate anticancer therapy efficacy by modulating cytotoxic CD8+ T cell immunity. Cell Metab. 2021;33(5):988–1000.e7. doi:10.1016/j.cmet.2021.03.002.
- Plaza-Diaz J, Ruiz-Ojeda FJ, Gil-Campos M, Gil A Mechanisms of action of probiotics. Adv Nutr (Bethesda, Md). 2019;10:S49–s66. doi:10.1093/advances/nmy063.
- Canfora EE, Jocken JW, Blaak EE. Short-chain fatty acids in control of body weight and insulin sensitivity. Nat Rev Endocrinol. 2015;11(10):577–591. doi:10.1038/nrendo.2015.128.
- Yoshida H, Ishii M, Akagawa M Propionate suppresses hepatic gluconeogenesis via GPR43/AMPK signaling pathway. Arch Biochem Biophys. 2019;672:108057. doi:10.1016/j.abb.2019.07.022.
- Bilotta AJ, Ma C, Yang W, Yu Y, Yu Y, Zhao X, Zhou Z, Yao S, Dann SM, Cong Y, et al. Propionate enhances cell speed and persistence to promote intestinal epithelial turnover and repair. Cell Mol Gastroenterol Hepatol. 2021;11(4):1023–44. doi:10.1016/j.jcmgh.2020.11.011.
- Smith PM, Howitt MR, Panikov N, Michaud M, Gallini CA, Bohlooly YM, Glickman JN, Garrett WS. The microbial metabolites, short-chain fatty acids, regulate colonic treg cell homeostasis. Sci. 2013;341(6145):569–73. doi:10.1126/science.1241165.
- Thomas SP, Denu JM Short-chain fatty acids activate acetyltransferase p300. Elife. 2021;10:e72171. doi:10.7554/eLife.72171.
- Sun HJ, Ren XS, Xiong XQ, Chen YZ, Zhao MX, Wang JJ, Zhou Y-B, Han Y, Chen Q, Li Y-H, et al. NLRP3 inflammasome activation contributes to VSMC phenotypic transformation and proliferation in hypertension. Cell Death Disease. 2017;8(10):e3074. doi:10.1038/cddis.2017.470.
- García-Calzón S, Perfilyev A, Martinell M, Ustinova M, Kalamajski S, Franks PW, Bacos K, Elbere I, Pihlajamäki J, Volkov P, et al. Epigenetic markers associated with metformin response and intolerance in drug-naïve patients with type 2 diabetes. Sci Transl Med. 2020;12(561):eaaz1803. doi:10.1126/scitranslmed.aaz1803.
- Koh A, Mannerås-Holm L, Yunn NO, Nilsson PM, Ryu SH, Molinaro A, Perkins R, Smith JG, Bäckhed F. Microbial imidazole propionate affects responses to metformin through p38γ-dependent inhibitory AMPK phosphorylation. Cell Metab. 2020;32(4):643–53.e4. doi:10.1016/j.cmet.2020.07.012.
- Bauer PV, Duca FA, Waise TMZ, Rasmussen BA, Abraham MA, Dranse HJ, Puri A, O’Brien CA, Lam TKT. Metformin Alters Upper Small Intestinal Microbiota that Impact a Glucose-SGLT1-Sensing Glucoregulatory Pathway. Cell Metabolism. 2018;27(1):101–17.e5. doi:10.1016/j.cmet.2017.09.019.
- Ouwerkerk JP, van der Ark KCH, Davids M, Claassens NJ, Finestra TR, de Vos WM, Belzer C. Adaptation of Akkermansia muciniphila to the oxic-anoxic interface of the mucus layer. Appl Environ Microbiol. 2016;82(23):6983–93. doi:10.1128/AEM.01641-16.
- Belzer C, de Vos WM. Microbes inside—from diversity to function: the case of Akkermansia. ISME J. 2012;6(8):1449–58. doi:10.1038/ismej.2012.6.
- Everard A, Belzer C, Geurts L, Ouwerkerk JP, Druart C, Bindels LB, Guiot Y, Derrien M, Muccioli GG, Delzenne NM, et al. Cross-talk between Akkermansia muciniphila and intestinal epithelium controls diet-induced obesity. Proc Natl Acad Sci USA. 2013;110(22):9066–71. doi:10.1073/pnas.1219451110.
- D’Souza WN, Douangpanya J, Mu S, Jaeckel P, Zhang M, Maxwell JR, Rottman JB, Labitzke K, Willee A, Beckmann H, et al. Differing roles for short chain fatty acids and GPR43 agonism in the regulation of intestinal barrier function and immune responses. PLoS ONE. 2017;12(7):e0180190. doi:10.1371/journal.pone.0180190.
- Pan P, Oshima K, Huang YW, Agle KA, Drobyski WR, Chen X, Zhang J, Yearsley MM, Yu J, Wang L-S. Loss of FFAR2 promotes colon cancer by epigenetic dysregulation of inflammation suppressors. Intl Journal of Cancer. 2018;143(4):886–96. doi:10.1002/ijc.31366.
- Parada Venegas D, De la Fuente MK, Landskron G, González MJ, Quera R, Dijkstra G, Harmsen HJM, Faber KN, Hermoso MA Short chain fatty acids (SCFAs)-mediated gut epithelial and immune regulation and its relevance for inflammatory bowel diseases. Front Immunol. 2019;10:277. doi:10.3389/fimmu.2019.00277.
- Pérez-Reytor D, Puebla C, Karahanian E, García K Use of short-chain fatty acids for the recovery of the intestinal epithelial barrier affected by bacterial toxins. Front Physiol. 2021;12:650313. doi:10.3389/fphys.2021.650313.
- Guo H, Chou WC, Lai Y, Liang K, Tam JW, Brickey WJ, Chen L, Montgomery ND, Li X, Bohannon LM, et al. Multi-omics analyses of radiation survivors identify radioprotective microbes and metabolites. Sci. 2020;370(6516):370. doi:10.1126/science.aay9097.
- Forslund K, Hildebrand F, Nielsen T, Falony G, Le Chatelier E, Sunagawa S, Prifti E, Vieira-Silva S, Gudmundsdottir V, Krogh Pedersen H, et al. Disentangling type 2 diabetes and metformin treatment signatures in the human gut microbiota. Nature. 2015;528(7581):262–266. doi:10.1038/nature15766.
- Wang J, Wang Y, Han J, Mei H, Yu D, Ding Q, Zhang T, Wu G, Peng G, Lin Z, et al. Metformin attenuates radiation-induced pulmonary fibrosis in a murine model. Radiat Res. 2017;188(1):105–113. doi:10.1667/RR14708.1.
- Chen L, Liao F, Jiang Z, Zhang C, Wang Z, Luo P, Jiang Q, Wu J, Wang Q, Luo M, et al. Metformin mitigates gastrointestinal radiotoxicity and radiosensitises P53 mutation colorectal tumours via optimising autophagy. Br J Pharmacol. 2020;177(17):3991–4006. doi:10.1111/bph.15149.
- Ottewell PD, Watson AJ, Wang TC, Varro A, Dockray GJ, Pritchard DM. Progastrin stimulates murine colonic epithelial mitosis after DNA damage. Gastroenterology. 2003;124(5):1348–1357. doi:10.1016/S0016-5085(03)00288-9.
- Higurashi T, Hosono K, Takahashi H, Komiya Y, Umezawa S, Sakai E, Uchiyama T, Taniguchi L, Hata Y, Uchiyama S, et al. Metformin for chemoprevention of metachronous colorectal adenoma or polyps in post-polypectomy patients without diabetes: a multicentre double-blind, placebo-controlled, randomised phase 3 trial. Lancet Oncol. 2016;17(4):475–483. doi:10.1016/S1470-2045(15)00565-3.
- Wang Y, Wang M, Chen J, Li Y, Kuang Z, Dende C, Raj P, Quinn G, Hu Z, Srinivasan T, et al. The gut microbiota reprograms intestinal lipid metabolism through long noncoding RNA Snhg9. Sci. 2023;381(6660):851–857. doi:10.1126/science.ade0522.
- Zhang F, Wan Y, Zuo T, Yeoh YK, Liu Q, Zhang L, Zhan H, Lu W, Xu W, Lui GCY, et al. Prolonged impairment of short-chain fatty acid and L-Isoleucine biosynthesis in gut microbiome in patients with COVID-19. Gastroenterology. 2022;162(2):548–61.e4. doi:10.1053/j.gastro.2021.10.013.
- Kim S, Shin YC, Kim TY, Kim Y, Lee YS, Lee SH, Kim M-N, O E, Kim KS, Kweon M-N, et al. Mucin degrader Akkermansia muciniphila accelerates intestinal stem cell-mediated epithelial development. Gut Microbes. 2021;13(1):1–20. doi:10.1080/19490976.2021.1892441.
- Yan F, Liu L, Cao H, Moore DJ, Washington MK, Wang B, Peek RM, Acra SA, Polk DB. Neonatal colonization of mice with LGG promotes intestinal development and decreases susceptibility to colitis in adulthood. Mucosal Immunology. 2017;10(1):117–27. doi:10.1038/mi.2016.43.
- Wang KK, He KY, Yang JY, Liu MJ, Guo JR, Liang JY, Wang J-H, Xu Z-X, Jian Y-P Lactobacillus suppresses tumorigenesis of oropharyngeal cancer via enhancing anti-tumor immune response. Front Cell Dev Biol. 2022;10:842153. doi:10.3389/fcell.2022.842153.
- Lu C, Chen J, Xu HG, Zhou X, He Q, Li YL, Jiang G, Shan Y, Xue B, Zhao R, et al. MIR106B and MIR93 prevent removal of bacteria from epithelial cells by disrupting ATG16L1-mediated autophagy. Gastroenterology. 2014;146(1):188–199. doi:10.1053/j.gastro.2013.09.006.
- Ding S, Song Y, Brulois KF, Pan J, Co JY, Ren L, Feng N, Yasukawa LL, Sánchez-Tacuba L, Wosen JE, et al. Retinoic acid and lymphotoxin signaling promote differentiation of human intestinal M cells. Gastroenterology. 2020;159(1):214–26.e1. doi:10.1053/j.gastro.2020.03.053.
- Hänninen A, Toivonen R, Pöysti S, Belzer C, Plovier H, Ouwerkerk JP, Emani R, Cani PD, De Vos WM. Akkermansia muciniphila induces gut microbiota remodelling and controls islet autoimmunity in NOD mice. Gut. 2018;67(8):1445–53. doi:10.1136/gutjnl-2017-314508.
- Chiu CJ, McArdle AH, Brown R, Scott HJ, Gurd FN. Intestinal mucosal lesion in low-flow states. I. A morphological, hemodynamic, and metabolic reappraisal. Archives of surgery (Chicago, Ill: 1960). Arch Surg. 1970;101(4):478–83. doi:10.1001/archsurg.1970.01340280030009.
- Mori K, Morisaki H, Yajima S, Suzuki T, Ishikawa A, Nakamura N, Innami Y, Takeda J. Beta-1 blocker improves survival of septic rats through preservation of gut barrier function. Intensive Care Med. 2011;37(11):1849–56. doi:10.1007/s00134-011-2326-x.
- Ma F, Hao H, Gao X, Cai Y, Zhou J, Liang P, Lv J, He Q, Shi C, Hu D, et al. Melatonin ameliorates necrotizing enterocolitis by preventing Th17/Treg imbalance through activation of the AMPK/SIRT1 pathway. Theranostics. 2020;10(17):7730–7746. doi:10.7150/thno.45862.
- Scott NA, Andrusaite A, Andersen P, Lawson M, Alcon-Giner C, Leclaire C, Caim S, Le Gall G, Shaw T, Connolly JPR, et al. Antibiotics induce sustained dysregulation of intestinal T cell immunity by perturbing macrophage homeostasis. Sci Transl Med. 2018;10(464):eaao4755. doi:10.1126/scitranslmed.aao4755.
- Wang L, Tang L, Feng Y, Zhao S, Han M, Zhang C, Yuan G, Zhu J, Cao S, Wu Q, et al. A purified membrane protein from Akkermansia muciniphila or the pasteurised bacterium blunts colitis associated tumourigenesis by modulation of CD8 + T cells in mice. Gut. 2020;69(11):1988–97. doi:10.1136/gutjnl-2019-320105.