ABSTRACT
Repeated exposure to antibiotics and changes in the diet and environment shift the gut microbial diversity and composition, making the host susceptible to pathogenic infection. The emergence and ongoing spread of AMR pathogens is a challenging public health issue. Recent evidence showed that probiotics and prebiotics may play a role in decolonizing drug-resistant pathogens by enhancing the colonization resistance in the gut. This review aims to analyze available evidence from human-controlled trials to determine the effect size of probiotic interventions in decolonizing AMR pathogenic bacteria from the gut. We further studied the effects of prebiotics in human and animal studies. PubMed, Embase, Web of Science, Scopus, and CINAHL were used to collect articles. The random-effects model meta-analysis was used to pool the data. GRADE Pro and Cochrane collaboration tools were used to assess the bias and quality of evidence. Out of 1395 citations, 29 RCTs were eligible, involving 2871 subjects who underwent either probiotics or placebo treatment to decolonize AMR pathogens. The persistence of pathogenic bacteria after treatment was 22%(probiotics) and 30.8%(placebo). The pooled odds ratio was 0.59(95% CI:0.43–0.81), favoring probiotics with moderate certainty (p = 0.0001) and low heterogeneity (I2 = 49.2%, p = 0.0001). The funnel plot showed no asymmetry in the study distribution (Kendall’sTau = −1.06, p = 0.445). In subgroup, C. difficile showed the highest decolonization (82.4%) in probiotics group. Lactobacillus-based probiotics and Saccharomyces boulardii decolonize 71% and 77% of pathogens effectively. The types of probiotics (p < 0.018) and pathogens (p < 0.02) significantly moderate the outcome of decolonization, whereas the dosages and regions of the studies were insignificant (p < 0.05). Prebiotics reduced the pathogens from 30% to 80% of initial challenges. Moderate certainty of evidence suggests that probiotics and prebiotics may decolonize pathogens through modulation of gut diversity. However, more clinical outcomes are required on particular strains to confirm the decolonization of the pathogens. Protocol registration: PROSPERO (ID = CRD42021276045).
Introduction
The human intestinal microbiota is a complex microbial ecosystem that colonizes the gastrointestinal system and comprises approximately 500 to 1000 bacterial species,Citation1 besides viruses, archaea, protozoa, and other eukaryotes. It is a dynamic ecosystem affected by diet, lifestyle and stress.Citation2 This microbial community modulates human health, including host metabolism, physiology, nutrition, and immune response.Citation2 Besides, the host microbiota is essential for fostering resistance to pathogens.Citation3,Citation4 Repeated exposure to antibiotics and changes in diet and environment can cause an imbalance in gut microbial diversity and composition, termed gut dysbiosis.
Gut dysbiosis makes the host susceptible to pathogenic colonization, as the compromised microbiota cannot offer resistance against colonization by exogenous antibiotic-resistant or pathogenic bacteria.Citation3 The gastrointestinal tract can, therefore, function as a reservoir to harbor a range of bacterial pathogens as they colonize and multiply to high densities. This gives rise to two important concerns. Firstly, individuals heavily colonized with these pathogens act as reservoirs, contributing to the ongoing persistence of pathogens and facilitating person-to-person transmission. Secondly, colonization can lead to potentially fatal infections, particularly in individuals with impaired immune systems.Citation5
Modulating the gut microbiota can be an innovative and effective strategy to defend against colonization of antimicrobial-resistant or infectious enteric pathogens.Citation6 However, conventional therapies do not provide reliable decolonization outcomes.Citation7 Research has been conducted using prebiotics and probiotics to decolonize AMR pathogens as a public health demand by increasing bacterial diversity in the gut.
Probiotics are defined as live nonpathogenic microorganisms administered to improve microbial diversity in the gut. Prebiotics are compounds that do not contain microorganisms but provide health benefits by stimulating the growth of beneficial microorganisms in the gut.Citation8 Probiotics have the potential to eliminate or reduce pathogens in the gut through various mechanisms, including competitive exclusion, enhancing intestinal colonization, improving intestinal barrier function, and regulating immune properties.Citation9 Prebiotics help to balance the gut flora by promoting the growth of beneficial microbes. Digestion of prebiotics produces SCFAs and decreases the gut lumen’s pH, which helps avoid pathogen colonization.Citation10 In addition, the anti-adherence properties of prebiotics can help reduce pathogenic microorganisms in the gut.Citation11
While alternative approaches like antibiotics, fecal microbiota transplantation (FMT), phage therapy, and CRISPR-Cas9 systems have also been used to decolonize pathogens, they all have inherent limitations.Citation12 Although evidence suggests that probiotics and prebiotics can positively impact pathogenic decolonization,Citation9,Citation11-14 it would be necessary to understand the magnitude of these effects and their generalizability since some studies failed to demonstrate successful decolonization or reduction of the carriage of drug-resistant pathogens.Citation15,Citation16 To address this variability in clinical efficacy, we have conducted a systematic review and meta-analysis of randomized clinical trials (RCTs) on probiotics and prebiotics to decolonize or eradicate pathogenic bacteria from the human gut microbiota.
Methodology
Protocol development and registration
The systematic review protocol was developed following the recommendations of the PRISMA-P 2015 guidelinesCitation17 (Supplement Table S1). The review protocol was registered in the International Prospective Register of Systematic Reviews (PROSPERO) and is available at https://www.crd.york.ac.uk/prospero/display_record.php?ID=CRD42021276045.
Systematic search strategy
A comprehensive search was conducted using databases including PubMed, SCOPUS, Web of Science (SCI), CINAHL (EBSCO), Embase (via Ovid), and MEDLINE (via Ovid), and Google Scholar to extract data for this review and meta-analysis. The details of search strategies used in different databases can be found in Supplement Table S2a-f. There was no time limit for searching the data, which was completed to that available in February 2023. Additional articles from the reference lists of identified publications were also added to the COVIDENCE platform for screening when appropriate.
Eligibility criteria
The PICOS (Population, Intervention, Comparator, Outcomes, and Study Design) approach was used to guide the determination of the eligibility criteria.Citation18 This systematic review analyses the effect of two interventions, probiotics and prebiotics, in decolonizing pathogenic bacteria from the gut. We considered probiotics intervention studies with or without concomitant conventional treatment (as an experimental group) and compared them with the placebo to evaluate the outcomes. Similarly, in the case of the efficacy of prebiotics to decolonize or reduce the pathogen’s load, we considered the studies that used prebiotics (treatment group) compared with the placebo, antimicrobial agent, or control group (no treatment). The primary outcome evaluated for the probiotic-treated cohorts was the decolonization of pathogens from the gut within one month (30 days) or the end of the study, whichever is earliest, confirmed by PCR or plate culture method. However, complete eradication and CFU reduction were also considered outcomes of interest for the prebiotics.
For the effect of the probiotics cohort, we included randomized control trials (RCTs) and other clinical studies with a distinct control arm compared with a placebo administered for any length of time among people to eradicate pathogens from the gut. show the details of the inclusion and exclusion criteria.
Table 1. Inclusion and exclusion criteria for the Probiotics study.
Table 2. Inclusion and exclusion criteria for the Prebiotics study.
Quality assessment and reduction of risk of bias to assess the eligibility of the articles
The web platform https://www.covidence.org was used to conduct this assessment. All citations from the databases were imported into the software. All authors reached a consensus to include articles from Google Scholar. NR and NB conducted preliminary abstract screening and inclusion of articles for this review. Then NR, NB, MT, and PD conducted a full-text review of the chosen studies to reduce bias. Any discrepancies were reviewed with the senior author MI.
We applied the Cochrane Collaboration tool to assess the risk of bias of the included RCTs (https://www.bmj.com/content/343/bmj.d5928##) via the RevMan 5.4 version. The risk of bias parameters encompassed randomization (selection bias), allocation concealment (selection bias), blinding (performance bias), blinding of outcome assessment (detection bias), incomplete outcome data (attrition bias), selective reporting (reporting bias), group similarity baseline (selecting bias), compliance (performance bias), co-intervention (performance bias) and others. Each potential bias was rated by assigning uncertain, high, or low risk.
Data extraction
We created a spreadsheet for data abstraction using Excel 2022 (Microsoft Office 365, Microsoft Corporation, Redmond, Washington, U.S.A.) The details are found in Supplement Tables S3 and S4. A final column was added for the reviewer’s judgment of whether the study was accepted or rejected.
Data synthesis and analysis
All RCTs and clinical trials with a distinct control arm for the efficacy of probiotic(s) underwent a meta-analysis for the primary outcome (Decolonization of pathogenic bacteria at the end of the intervention or 1-month time point). Meta-analysis was conducted using RevMan 5.4, Statistical Packages for Social Science (SPSS) 28, USA, and JamoviCitation22 (version 2.3, Sydney, Australia) following the generic inverse-variance approach of the random-effects model. The combined measures of the estimate were illustrated by creating a forest plot. Subgroup analyses were conducted for different pathogenic species, probiotic(s) groups, and regions to understand treatment efficacy and study heterogeneity. Any moderators affecting the outcome were evaluated by Jamovi.Citation22 The meta-regression function of the Open meta-analyst was used to assess the effect of probiotics dose on the outcome.Citation23 Statistical heterogeneity between the studies included in the meta-analysis was described using I2 statistic. A funnel plot was generated to determine the publication bias and asymmetry of the studies. Since meta-analysis should not rely on a single test to quantify publication bias, we analyzed bias using rank correlation and regression of funnel plot asymmetry and Eggar’s and Begg’s tests. Eggar’s and Begg’s regression tests detect publication bias more frequently than others.Citation24,Citation25 Prebiotic studies did not have a similar control arm and decolonization objectives. Thus, a narrative synthesis of data from the articles involved in decolonizing or reducing pathogenic burdens by prebiotic interventions was performed. We also conducted a narrative synthesis of data from the study with metagenomic analysis.
Results
Study selection
The overall process of study selection is summarized in a PRISMA flow diagram (). Forty articles were eligible for detailed data abstraction and discussion. Twenty-nine RCTs focused on probiotic treatments were selected for quantitative meta-analysis, whereas 15 articles were chosen for qualitative data analysis (10 prebiotics and 5 probiotics).
Characteristics of probiotic studies
A total of 29 RCTs with probiotic(s) treatment were included for quantitative meta-analysis. A detailed summary of these studies is listed in Supplement Table S5. A total of 6 RCTs on probiotics intervention with metagenomic analysis were chosen for qualitative analysis (Supplement Table S6). The probiotics and their dosages to decolonize pathogens varied among the studies. The maximum dose of probiotics was 2 × 1011 CFU/day (E. coli nissle), whereas the minimum dose was 1 × 107 CFU/day (non-toxigenic C. difficile). Most studies decolonized bacteria using 1010 CFU/day ().
Table 3. Types and dosages of probiotics.
Efficacy of the probiotics on the primary outcome (decolonization of pathogenic bacteria)
All 29 studies (2871 patients) included in the meta-analysis evaluated the efficacy of probiotics with or without traditional treatment over traditional treatment alone (or placebo) for decolonizing pathogenic bacteria at the end of the trial or at a 1-month time-point. The persistence of pathogenic bacteria after treatment was 361 (23.24%) of the 1353 patients in the probiotic(s)-treated group (decolonization rate 77%) and 498 (32.8%) of the 1518 patients in the placebo group (decolonization rate 67.2%) respectively. The pooled odds ratio (OR) for total events (persistence of bacteria upon treatment) was 0.59, indicating that pathogenic bacteria persistence was significantly lower in the probiotic(s) group than placebo (p = 0.0001, CI:0.43–0.81). Acceptable heterogeneity was observed in the 29 clinical trials (I2 = 49%, ).
Figure 2. Forest plot for the intervention group (probiotics) vs. placebo for the decolonization success at the end of the study or at a 1-month time point. The I2 values are interpreted as low, moderate, and high levels of heterogeneity, where I2 = 0–49%, I2= 50–75%, and I2 > 75%.Citation19,Citation53 A 5% significance level (p<0.05) was used to determine the significant difference between the treatment efficacies. The events consist of the persistence of bacteria.
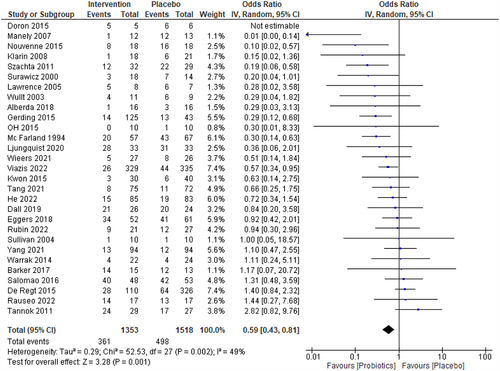
Subgroup analysis for the effect of probiotics with or without conventional medicine
The effects of probiotics concurrent with or without conventional medicine in decolonizing pathogens have been depicted in . The probiotics with traditional medicine or antibiotics (20 assigned by hospital depending on diseases, total 21 studies) decolonized pathogens from 818 (78.65%) of 1040 subjects in the treatment group vs. 919 (72.19%) of 1273 subjects in the placebo group (OR = 0.51, p = 0.001). The observed statistical heterogeneity is low (I2 = 52%, p = 0.002). Besides, the probiotics without antibiotics or traditional treatment (8 studies), decolonized pathogens from 174 (56%) of 313 intervene subjects vs. 101 (41%) of 245 placebo subjects (OR = 0.79, p = 0.44) with the statistical heterogeneity I2 = 43%, p = 0.11. However, the observed subgroup difference estimate (chi2 = 1.46, p = 0.23) is not significant.
Figure 3. Forest plot of the sub-group analysis for the efficacy of probiotics concomitant with or without conventional medicine. The I2 values are interpreted as low, moderate, and high levels of heterogeneity, where I2 = 0–49%, I2= 50–75%, and I2> 75%, respectively.Citation19,Citation53 A 5% significance level (p<0.05) was used to determine the significant difference between the treatment efficacies. The events consist of the persistence of bacteria.
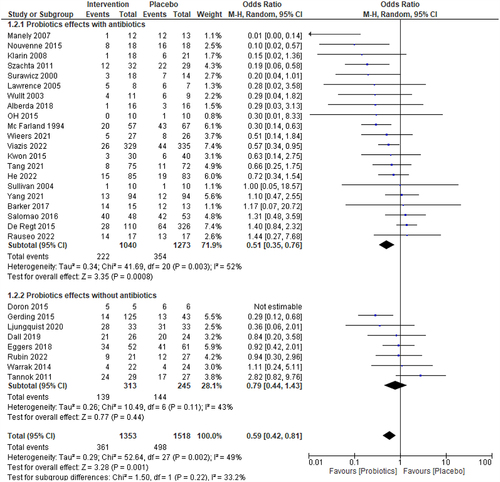
Subgroup analysis for the types of probiotics
depicts the probiotics group-wise effects on the outcome of decolonizing pathogens from the intestine and statistical heterogeneity among probiotics studies. Lactobacillus-based probiotics (16 studies) decolonized pathogens from 168 (62%) of 442 patients vs. 212 (53%) of 451 patients in the placebo group (OR = 0.70, p = 0.04) with statistical heterogeneity I2 = 39%, p = 0.06. In five studies using Bifidobacterium-based mixed probiotics, 413 (80%) of 514 subjects on probiotics vs. 574 (78%) of 733 subjects on placebo decolonized pathogens, and no significant differences were demonstrated between the two groups (OR:0.83, p = 0.23, I2 = 48%). S. boulardii significantly decolonized pathogens (76.3%) compared to placebo (57.9%) (OR = 0.43, p = 0.0009) with minimal statistical heterogeneity (I2 = 43%, p = 0.05).
Figure 4. Forest plot of the sub-group analysis for the efficacy of specific probiotics Lactobacillus, Mixed probiotics, S. boulardii, and other probiotics vs placebo. The I2 values are interpreted as low, moderate, and high levels of heterogeneity, where I2 = 0–49%, I2= 50–75%, and I2> 75%, respectively.Citation19,Citation53 A 5% significance level (p<0.05) was used to determine the significant difference between the treatment efficacies. The events consist of the persistence of bacteria.
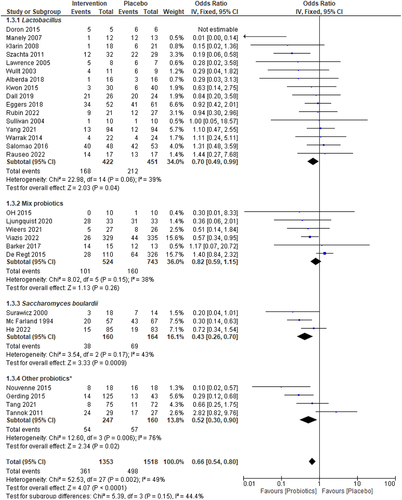
Subgroup analysis for the types of bacterial pathogen
shows the statistical heterogeneity and treatment efficacy of the studies of decolonized primary gut colonizers. C. difficile decolonization without recurrent infection was achieved in 206 (76.9%) of 268 patients who received combined treatment (Probiotic + conventional treatment) and in 94 (49.5%) of the 190 in placebo (conventional treatment alone) (OR = 0.29, p < 0.00001). Importantly, no heterogeneity was observed between the 8 studies on C. difficile (I2 = 0, p = 0.98). Probiotics intervention showed no advantage in treatment efficacy over placebo for MDR-Enterobacteriaceae in 5 RCTs involving 228 subjects. The pooled odds ratio was 0.64 (95% CI: 0.19–2.16) with moderate heterogeneity (I2 = 61). The probiotics intervention shows a statistically significant benefit on decolonizing Vancomycin-resistant Enterococcus (VRE) (4-RCTs, n = 145, pooled OR 0.17, p = 0.08, 95% CI:0.02–1.25, I2 = 81) and Helicobacter pylori (5-RCTs, n = 1167, pooled OR:0.68, p = 0.04, 95% CI:0.48–0.97, low or no heterogeneity I2=0).
Figure 5. Forest plots for sub-group analysis of the efficacy of the intervention (Probiotics) vs. Placebo on C. difficile, MDR-Enterobacteriaceae, VRE, and H. pylori. The I2 values are interpreted as low, moderate, and high levels of heterogeneity, where I2 = 0–49%, I2= 50–75%, and I2> 75%, respectively.Citation19,Citation53 A 5% significance level (p<0.05) was used to determine the significant difference between the treatment efficacies. The events consist of the persistence of bacteria.
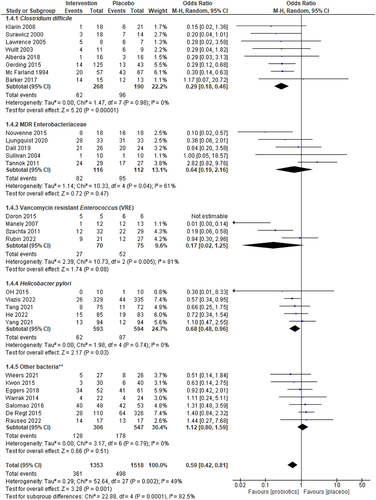
Subgroup analysis based on region
We further clustered the studies by continents where they were studied, according to North America (n = 9), Europe (n = 12), Asia (n = 3), and others* (n = 4), to observe differences in the treatment efficacy (Supplement Figure S1 and S2). No significant differences (p = 0.43) were found among regions in decolonizing pathogenic bacteria. Studies conducted in the USA effectively (p=0.008) eradicated pathogenic colonizers compared to placebo (pooled OR: 0.56, 95% CI:0.36–0.85, low heterogeneity, I2 = 16%), and that conducted in Europe (OR: 0.71, = 0.02, 95% CI:0.53–0.94, moderate heterogeneity, I2 = 53%). The Others* cluster showed excellent effectiveness in decolonizing bacteria from the gut, where pooled effect size, OR was 0.27, CI:0.14–0.53, and p = 0.0002. Though the study in other clusters was conducted in different countries, the observed heterogeneity, I2 was 0%.
Moderation effects of the different covariates of probiotics intervention
shows the moderation effects of probiotics types, pathogens, clusters, and the dose of probiotics (CFU/day) on the primary outcome. The types of probiotics (p = 0.018) and pathogenic gut colonizers (p=0.003) significantly predict the effect size of decolonization. However, other covariates, regions (p=0.842), and the dose of probiotics (p = 0.737) could not control the outcome of pathogen decolonization. The regression models for probiotics in decolonizing pathogens are shown in . The QQ plot was used to examine the RCT distribution before running the regression model (Supplement Figure S3). The distribution of studies met the assumption for meta-regressions conducted in open-meta analystCitation17 and Jamovi.Citation16
Figure 6. Results of the meta-regression of the covariates, dosages of probiotics, and types of probiotics and types of bacteria on the decolonization of pathogens.
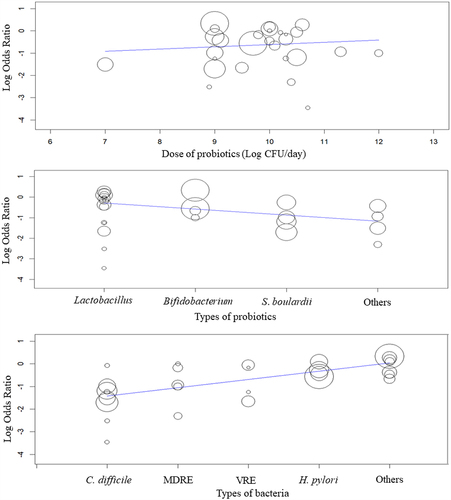
Table 4. Moderation effect of the covariates of probiotic intervention, types of probiotics, pathogens, regions, and dosages of probiotics on the effect size of pathogenic decolonization.
Risk of bias assessment
Eighteen of 25 RCTs possess minimal or no elements of biasCitation13,Citation16,Citation27,Citation30-32,Citation34,Citation36,Citation37,Citation40-42,Citation44,Citation46,Citation48-51 (Supplement Figure S4). We selected 3 prospective cohort studies, twoCitation39,Citation47 of which retain outstanding quality concerning predilection, whereas 1 studyCitation42 owns moderate features. A case-control studyCitation26 also confirmed its strength concerning bias reduction. There are 11 studiesCitation13,Citation30,Citation34,Citation36,Citation37,Citation39,Citation41,Citation46,Citation47,Citation49,Citation51 that thoroughly addressed all 10 possible risks of bias. At the same time, between two to seven studies pose a high risk of bias random sequence generation,Citation29,Citation45 allocation concealment,Citation35 blinding of participants,Citation26,Citation28,Citation33,Citation35,Citation45 blinding of outcome assessment,Citation28 incomplete outcome data,Citation35 and selective reportingCitation31,Citation43 respectively.
All elements of bias were less than 5% except blinding of participants and personnel (performance bias) and blinding of outcomes (detection bias). However, the performance and detection biases did not exceed 25%, as shown in the summary graph (Supplement Figure S5).
Publication bias
There was no indication of potential publication bias influencing observed aggregated results. A funnel plot was created by RevMan 5.4 to determine the asymmetry of the 29 RCTs (). The funnel plot shows a symmetrical distribution. According to the Egger test, the publication bias and asymmetry between the studies were insignificant (). The Begg’s rank correlation (p=0.445) and regression tests (0.272) for funnel plot asymmetry were also not statistically significant at a 5% significance level. Cook’s distances were examined to determine any outliers or influential studies that could affect the outcomes (Supplement Figure S6). Though there are flagged studies among covariates, no studies were found to be extreme outliers or influencers of outcomes (Cook’s distance < 1).
Table 5. Publication bias summary.
Quality of evidence through GRADEPro analysis
The quality of evidence of the studies was analyzed using the Cochrane review tool GRADEpro with the relative and absolute effects of probiotics intervention to decolonize pathogens. shows that the certainty of overall probiotics (n = 29) effects was moderate (⨁⨁⨁◯), which reveals the effectiveness of probiotics in decolonizing pathogens. However, certainty was increased from moderate to high in the subgroup analysis for the probiotics and types of pathogens. The certainty of the evidence for prebiotics trials (n = 10) was low due to not having a control arm and randomization, as GRADEpro considers these criteria to judge the certainty of evidence. GRADEpro analysis of evidence for all subgroups is presented in Supplement Table S7.
Table 6. GRADEpro summary of findings for assessing the quality of evidence as assessed separately by two independent reviewers.
Sensitivity analysis
Post-hoc sensitivity analyses were conducted, keeping aside the high risk of bias studies according to JBI guidelines (Supplement Figure S7). We further analyze post-hoc sensitivity by excluding the most weighted (influential) studies (Supplement Figure S8). The intervention effect size for all included studies was 0.59 (favoring probiotics). However, the pooled intervention effect sizes (OR) of the probiotics intervention were 0.63 (p = 0.01) and 0.54 (p = 0.0001) after eliminating the potential high risk of biased and comparatively larger weighted studies, respectively. No significant differences were observed between the adjusted OR and overall OR (0.59) (p > 0.05, t-test of SPSS). Besides, heterogeneity (I2 = 43%) was noticeably changed after eliminating biased and weighted studies from the analysis. A Trial Sequence Analysis (TSA) graph of 29 RCTs was constructed to confirm the effectiveness of probiotics intervention, which also shows that RCTs favor the probiotics intervention for decolonizing pathogens (Supplement Figure S9).
Characteristics of the selected prebiotic studies
A total of ten studies investigating prebiotic treatments for the decolonization or reduction of pathogenic bacterial burden from the intestine were included and are summarized in Supplement Table S8. The prebiotics used to reduce the burden of pathogens by the selected studies are Fructooligosaccharide (FOS), Xylooligosaccharide (XOS), Galactooligosaccharide (GOS), Xylooligosaccharide and mannose-oligosaccharide (MOS), Mannan-oligosaccharide, Beta-glucan and galactoglucomannan oligosaccharide (GGM). The dosages of the prebiotics varied with the types of prebiotics and the challenged microorganisms. The dosage of fructooligosaccharides ranged from 5% for low doses and 25% for high doses.Citation54–56 Mannan-oligosaccharide was used as a 2 g/kg basal diet for chickens.Citation57 In comparison, a dose of Galacto-oligosaccharides (GOS) 0.2 g/kg body weight was used to reduce the burden of Helicobacter and Clostridium.Citation58 However, the xylooligosaccharide dose was 1.2 gm mixed with 150 gm rice for the human trial.Citation59 Besides, Rajani et al.Citation60 mentioned that the galactoglucomannan oligosaccharides (GGMs) dose in their study was up to 0.3%. The prebiotics were mixed with drinking water or rice porridge for consumption ().
Table 7. Types and dosages of prebiotics.
Decolonisation potential of prebiotics
The prebiotic studies showed the potential to eradicate pathogens from the gut. All studies used oligosaccharides to reduce the colonization of pathogens, though they have high heterogeneity in types and dosages of prebiotics. One study was conducted on humans to evaluate the efficacy of prebiotics on gut microbiota. The study showed that xylooligosaccharides reduced the Clostridium perfringens content from 4log to 2log CFU/g stool. The probiotics also reduced Coliform content, but the difference in CFU content is insignificant compared to the control group.Citation59 The frequently used prebiotic was fructooligosaccharide (FOS), which reduced the fecal shedding of Salmonella enterica significantly in the treatment group (102 CFU/g) compared to the control (105 CFU/g) (initial challenge 108 CFU).Citation54 FOS reduced Clostridium subcluster XIVa from 6% to 2.7% in the treatment group, whereas it increased from 5.9% to 26.3% in the no-treatment group.Citation55
Mao et al.Citation56 mentioned that FOS decreased Actinobacteria from 0.3% to 4.2% at a 95% confidence level (p < 0.05) in the treatment group. It also reduces Proteobacteria and Verrucomicrobia at different levels. Monteagudo-Mera et al.Citation58 showed that galacto-oligosaccharide (GOS) effectively reduced the abundance of Bacteroidales, Helicobacter, and Clostridium. According to Pourabedin et al.,Citation61 mannose-oligosaccharide (MOS) was more effective than xylooligosaccharide (XOS) in reducing pathogens. MOS reduced the burden of Salmonella enteritidis by 1.6logs, whereas XOS reduced only 1log compared to the placebo group.Citation61 Beta-glucan also reduced the burden of Salmonella enterica from 50% of subjects in the treatment group to 6% in the control group.Citation63 Spring et al.Citation62 showed that mannan-oligosaccharide had reduced cecal S. typhimurium concentrations on Day 10 in the treatment group, which significantly differed from the control group (5.40 vs. 4.01 log CFU/g; p < 0.05). The highest rate of colonization reduction of S. typhimurium was observed in the chicks that received 0.2% GGM consisting of 2logs reductionCitation60 (Supplement Table S8).
Changes in microbial diversity in the gut
We narratively synthesize the metagenomic data of included studies to assess alpha diversity’s shifting in gut microbiota after having probiotics intervention.Citation46,Citation51,Citation64 According to the Shannon and Chao indexes, the bacterial diversity of the studies with metagenomic analysis had shifted after probiotic treatment (). Oh et al.Citation52 and Yang et al.Citation51 showed that probiotic intervention significantly increased the alpha diversity of bacteria in the gut compared with placebo. Conversely, the alpha diversity in the probiotics group was decreased compared to placebo in the study conducted by Chen et al.Citation65 However, the change in diversity was insignificant. Tang et al.Citation46 and Yang et al.Citation51 reported a statistically significant shift in beta diversity in the probiotics group compared to the placebo. Piewngam et al.Citation64 also altered but did not reach statistical significance.
Table 8. Metagenomic analysis outcome on alpha diversity for probiotic intervention.
Microbiome composition changes after probiotic treatments
The qualitative synthesis of the available data from the included studies revealed that the probiotics changed the gut microbiome compositions (). OH et al.Citation52 revealed that after 14 days of probiotics treatment, the drug-resistant Klebsiella, Citrobacter, Pseudomonas, and Escherichia were reduced significantly in the probiotics group. In contrast, Klebsiella and Citrobacter were increased in the placebo group. As both arms received the combination therapy with antibiotics and proton pump inhibitor for treating H. pylori infection, the total Operational Taxonomic Units (OTUs) were reduced in both groups. However, the reduction was significantly higher in the placebo group.Citation52 Chen et al.Citation65 mentioned that the Lactobacillus intervention changed the phyla and genera of bacteria in the gut significantly. After 28 days of treatment, Firmicutes and Actinobacteria were reduced in the probiotics group and increased in the placebo group. He et al.Citation33 showed that probiotics increased beneficial bacteria, including Bacteroides, Bifidobacterium, and Lactobacillus, but decreased Enterococcus and Enterobacter in the gut, and the reverse occurred in the gut of the placebo group. Yang et al.Citation51 mentioned no change in the gut microbiota composition in the probiotics and placebo groups. However, some changes were observed in the phyla, as Bacteroides, Firmicutes, and Actinobacteria were increased in the probiotics group, while only Proteobacteria was increased in the placebo group.Citation51 According to Tang et al.,Citation46 Fecalibacterium and Bacteroides were increased in the probiotics group but decreased in the placebo group. However, the changes observed in Shigella, Klebsiella, Streptococcus, Veillonella, Roseburia, Phascolaretobacterium, and Blautia were the same for the probiotic and placebo groups.Citation46 Piewngam et al.Citation64 also presented the same scenario as He et al.,Citation33 stating that after the probiotic treatment, the Firmicutes and Actinobacteria were increased, and Bacteroidetes and Proteobacteria were decreased in the probiotics group. In contrast, the reverse was noted in the placebo group.
Table 9. Metagenomic analysis outcome on the microbiome composition for probiotic intervention.
Discussion
The influence of the gut microbiota on the host physiology is well recognized. The increasing prevalence of multiple drug-resistant pathogens mandates the need to explore alternatives to antibiotics, particularly as antibiotics have long-term effects on gut microbiota and human health,Citation66–68 and may contribute to increasing undesirable microbial communities and resistant pathogens.Citation14,Citation69,Citation70 Besides, antibiotics do not consistently decolonize multi-drug-resistant bacteria. It may reduce healthy bacteria together with the pathogens from the gut.Citation69,Citation71 Probiotics or prebiotics with antibiotics treatment may improve long-term effects on health and microbial diversity.Citation9,Citation12,Citation72 However, probiotics and prebiotics have received little attention as therapies for eliminating or decolonizing harmful bacteria. Results from this meta-analysis suggest that probiotics may decolonize pathogens from the intestinal tract irrespective of the types of bacteria. The results also revealed that all types of probiotics used in these studies could be effective against pathogens.
The use of antibiotics to decolonize pathogens is a common practice worldwide. Recent studies have established that antibiotics disturb the gut and alter the microbial composition, affecting normal gut homeostasis.Citation14,Citation68,Citation70,Citation71,Citation73-76 Therefore, probiotics are used concomitantly with antibiotics to reduce long-term antibiotic effects on the gut microbiome ,Citation77-79 On the other hand, without antibiotics, probiotics showed successful decolonization of pathogens, which agrees with the results of recent studies.Citation13,Citation28,Citation32 Thus, considering the shortcomings of antibiotics, probiotics could be an alternative way of decolonizing potential pathogens.
However, differences in effect size are observed in the subgroup analysis (). The highest decolonization rate was observed with S. boulardii, followed by other* probiotics (including E. coli Nissle 1971, E. faecium, and nontoxigenic C. difficile). S. boulardii was especially effective on CDI during or following antibiotics.Citation80,Citation81 In fact, probiotic use was most effective in accelerating the decolonization rate of C. difficile, followed by MDR Enterobacteriaceae and vancomycin-resistant Enterococci (VRE) (). This could be due to the varying efficacy of specific probiotics against particular pathogenic colonizers. The overall heterogeneity among the RCTs is minimal. These results are consistent with previous systematic reviews and meta-analyses conducted by Penumetcha et al.Citation20 and Wang et al.Citation19 However, it was significantly reduced when we divided them into subgroups according to types of bacteria, probiotics or regions as these grouping collated the RCTs with the same characteristics.
We observed that the types of probiotics and pathogens significantly affect the results. Decolonization by probiotics depends on many factors, which may include competitive inhibition and exclusion of pathogens for adhesion sites, production of antimicrobial agents, improvement of immune response, and strengthening of the intestinal barrier.Citation82,Citation83 However, other covariates, including probiotics doses and region of studies, did not moderate effect size (p > 0.05). There were no substantial differences in doses among most probiotics (from 109 to 1010 CFU/day), so suggesting that the minimum quantities of probiotics should be given as 109 CFU/day to have positive effects. These results are consistent with the study conducted by Christensen et al.Citation84 However, despite having proven benefits, certain issues such as the probiotics quality, supply chain, single or multiple-strains formula, and CFU quantitation required for decolonization should be further resolved through clinical research.
Although the changes in gut microbial diversity due to probiotic intervention are under dispute, some studies established that probiotics could shift the alpha or beta diversity in the gut.Citation46,Citation65 Éliás et al.Citation85 stated that probiotics are ineffective at preventing antibiotic-induced low-diversity dysbiosis in the gut. Washburn et al.Citation86 also studied the effect of Bifidobacterium infantis commercial probiotics on gut microbiota diversity. They concluded that using a single-species probiotic has no appreciable impact on gut bacteria diversity in healthy individuals. Conversely, Muwonge et al.showed that probiotics could significantly change the diversity of the microbiome of an individual recovering from enteric pathogens.Citation87
Antibiotics are well known to disrupt the microbial composition and negatively impact the host’s health. Probiotic intervention modulates through minimizing the gut microbiota alteration and increasing the gut microbiome diversity. Even though some studies gave variable results on bacterial diversities,Citation46,Citation51,Citation52,Citation65 in cases of antibiotics as eradication therapy, probiotics helped to establish a beneficial bacterial profile.Citation46 Grazul et al. stated that probiotics did not successfully colonize the intestine after antibiotic treatments and did not seem to alter the overall diversity of the gut microbiota.Citation88 However, probiotics effectively suppress the growth of Enterobacteriaceae, including harmful bacteria like Shigella and Escherichia, while promoting the growth of beneficial bacteria from the Anaerotruncus genus.Citation88 Therefore, supplementation of probiotics is a safe and effective way for the recovery of healthy gut microbiota.
Our analysis showed that probiotics might help shift the diminished alpha and beta diversity caused by antibiotic exposure. Although the RCTs with metagenomics data were limited, some studies showed reverse results, consistent with the meta-analysis, which demonstrated the effect of probiotics supplementation during antibiotics treatment.Citation85 Probiotics can alter the microbiome composition and increase the beneficial bacteria, increasing their functional activity and helping remove pathogens or harmful bacteria.Citation89 Our study also shows that probiotics changed the gut compositions, increasing beneficial bacteria and decreasing antibiotic-resistant bacteria, including Klebsiella, Citrobacter, Enterococcus, Enterobacter etc. Antibiotics-induced decrease in Bacteroidetes: Firmicutes (B: F) ratio is associated with several metabolic disorders.Citation90,Citation91 Probiotics can balance the ratio and normalize the dysbiotic gut,Citation92 as our results show Proteobacteria may be a microbiological hallmark of several illnesses, including inflammatory bowel disease and metabolic problems, which may make the host susceptible to infection by pathogens.Citation93 The included studies in our analysis showed that the Proteobacteria was decreased in the probiotics group and increased in the placebo group.
We did not assess bias for the prebiotics study selected for narrative reviews since these studies did not have any control arm. There is a scarcity of prebiotics intervention studies conducted on human subjects. The dosages of prebiotics were not consistent among the studies. About 1.2 to 2.5 g of oligosaccharides was used to decolonize the pathogens. However, a beta-glucan-supplemented diet of up to 40 mg/kg of chicken significantly up-regulated innate immunity and significantly reduced the S. enterica organ invasion.Citation63 Though the exact mechanisms are yet to be elucidated, the authors speculated that the purified beta-glucan helps to prime the heterophils to phagocytize and kill invasive S. enterica by releasing oxygen-free radicals.Citation63 Another possible mechanism could be the dectin-1 receptor-mediated event against the invading enteropathogens.Citation94,Citation95 The dectin-1 receptor on the surface of mammalian macrophages and neutrophils is specific to beta-glucan and activated once it binds with beta-glucan.Citation96
The impact of prebiotics on the gut microbiome and their mechanism of action are subjects of increasing interest and have become a wider topic of debate. Prebiotics selectively influence the growth and activity of probiotics or friendly bacteria and help in -homeostasis in the gut.Citation10,Citation97,Citation98 In =homeostatic conditions, certain Gram-negative commensal bacteria stimulate antibody production, such as IgA and IgG, identifying Gram-negative bacteria surface antigens and contributing to host defense against symbionts and pathogens.Citation99 Besides, the intestinal flora breaks them down and selectively ferments them to create specific secondary metabolites.Citation98 These metabolites are then absorbed by the intestinal epithelium or go to the liver via the portal vein, where they can improve the function of the intestinal barrier, regulate immunity, and resist pathogens.Citation98,Citation99
It has already been established that probiotics use prebiotics as their food source. However, it raised a burning question of whether pathogenic bacteria use prebiotics as their food to grow. Very few studies have explored this issue. According to recent research, inulin and FOS promote the growth of Klebsiella, a bacterium linked to increased intestinal permeability.Citation100 Valyshev (2000)Citation101 stated that inulin increased the growth of Klebsiella, whereas it restricted E. coli and Salmonella. According to the literature review, prebiotics may enhance the growth of certain pathogens, but this is far exceeded by the rate at which they increase friendly bacteria.Citation9,Citation102
Research on the impact of prebiotics on the fecal microbiota of healthy human volunteers revealed that Lactobacilli and Bifidobacterium proliferated, with a slight increase of Enterobacteriaceae (mainly Klebsiella),Citation103-104,Citation105 Recent research also showed that prebiotics appear to primarily increase the amount of friendly bacteria in the gut, especially Lactobacillus and Bifidobacteria.Citation97,Citation106,Citation107 It should be noted that although this study was conducted in vitro, it is always preferable to have results verified by human clinical trials. Prebiotics increase the ratio of “good” to “bad” bacteria. Still, the precise rate at which various genus and species in the gut alter in response to varying kinds and dosages of prebiotics is unknown. Moreover, more research is needed to confirm that the pathogenic load does not reach an unhealthy level due to prebiotic application.
Conclusions
Probiotics and prebiotics may help to decolonize pathogens from the gut or increase the effectiveness of conventional medicine to decolonize multidrug-resistant pathogens. The certainty of the evidence of probiotic intervention in the subgroup was moderate to high. Though the overall effect size favored probiotic intervention, more clinical trials are warranted to make a robust conclusion. Prebiotics help maintain a healthy gut by influencing the growth of friendly bacteria, but human clinical trials are required to determine whether they enhance the growth of pathogens. Dosage, type of probiotic(s), and pathogens for decolonization are important factors influencing the effect size, and thus, maintaining uniformity in the properties or composition of probiotics and prebiotics, dosages, and categories of patient inclusion for conducting RCTs is necessary.
Author Contributions
Conceptualization, MI; methodology, NR and NB; writing – original draft preparation, NR, NB, MT, PD; writing – Review and editing, MI, MCFT, SHW; supervision, MI; project administration. All authors have read and agreed to the published version of the manuscript.
KGMI_20231512_Cleaned copy_Final Supplement file.docx
Download MS Word (760.5 KB)Disclosure statement
No potential conflict of interest was reported by the author(s).
Supplementary Material
Supplemental data for this article can be accessed online at https://doi.org/10.1080/19490976.2024.2356279
Additional information
Funding
References
- Jandhyala SM, Talukdar R, Subramanyam C, Vuyyuru H, Sasikala M, Reddy DN. Role of the normal gut microbiota. World J Gastroenterol. 2015;21(29):8836–25. doi:10.3748/wjg.v21.i29.8787.
- Ley RE, Lozupone CA, Hamady M, Knight R, Gordon JI. Worlds within worlds: evolution of the vertebrate gut microbiota. Nat Rev Microbiol. 2008;6(10):776–788.
- Ducarmon QR, Zwittink RD, Hornung BVH, van Schaik W, Young VB, Kuijper EJ. Gut microbiota and colonization resistance against bacterial enteric infection. Microbiol Mol Biol Rev. 2019;83(3):1–29. doi:10.1128/MMBR.00007-19.
- Yamamoto S, Saito M, Tamura A, Prawisuda D, Mizutani T, Yotsuyanagi H, Blachier F. The human microbiome and COVID-19: A systematic review. PLoS One [Internet]. 2021;16(6):1–13. Available from: 10.1371/journal.pone.0253293.
- Anthony WE, Burnham CAD, Dantas G, Kwon JH. The gut microbiome as a reservoir for antimicrobial resistance. J Infect Dis. 2021;223(Supplement_3):S209–13. doi:10.1093/infdis/jiaa497.
- Kim S, Covington A, Pamer EG. The intestinal microbiota: Antibiotics, colonisation resistance, and enteric pathogens. Immunol Rev. 2017;279(1):90–105. doi:10.1111/imr.12563.
- Dharmaratne P, Rahman N, Leung A, Ip M. Is there a role of faecal microbiota transplantation in reducing antibiotic resistance burden in gut? A systematic review and meta-analysis. Ann Med [Internet]. 2021;53(1):662–681. Available from: 10.1080/07853890.2021.1927170.
- Roberfroid MB. Roberfroid_2018. Prebiotics and probiotics: are they functional foods? Am J Clin Nutr. 2000;71(6):1682S–1687S. doi:10.1093/ajcn/71.6.1682S.
- Karbalaei M, Keikha M. Probiotics and intestinal decolonization of antibiotic-resistant microorganisms; a reality or fantasy? Ann Med Surg [Internet]. 2022;80:104269. Available from: 10.1016/j.amsu.2022.104269.
- Amorim C, Silvério SC, Cardoso BB, Alves JI, Pereira MA, Rodrigues LR. In vitro assessment of prebiotic properties of xylooligosaccharides produced by Bacillus subtilis 3610. Carbohydr Polym [Internet]. 2020;229:115460. Available from: 10.1016/j.carbpol.2019.115460.
- Janapatla RP, Dudek A, Chen CL, Chuang CH, Chien KY, Feng Y, Yeh YM, Wang YH, Chang HJ, Lee YC. et al. Marine prebiotics mediate decolonization of Pseudomonas aeruginosa from gut by inhibiting secreted virulence factor interactions with mucins and enriching bacteroides population. J Biomed Sci [Internet]. 2023;30(1):1–20. Available from: 10.1186/s12929-023-00902-w.
- Roson-Calero N, Ballesté-Delpierre C, Fernández J, Vila J. Insights on current strategies to decolonize the gut from multidrug-resistant bacteria: pros and cons. Antibiotics. 2023;12(6):1–13. doi:10.3390/antibiotics12061074.
- Ljungquist O, Kampmann C, Resman F, Riesbeck K, Tham J. Probiotics for intestinal decolonization of ESBL-producing Enterobacteriaceae: a randomised, placebo-controlled clinical trial. Clin Microbiol Infect [Internet]. 2020;26(4):456–462. Available from: 10.1016/j.cmi.2019.08.019.
- Matzaras R, Nikopoulou A, Protonotariou E, Christaki E. Gut microbiota modulation and prevention of dysbiosis as an alternative approach to antimicrobial resistance: a narrative review. Yale J Biol Med. 2022;95(4):479–494. doi:10.1101/gr.096651.109.
- Mourand G, Paboeuf F, Fleury MA, Jouy E, Bougeard S, Denamur E, Kempf I. Escherichia coli probiotic strain ed1a in pigs has a limited impact on the gut carriage of extended-spectrum-β-lactamase-producing E. coli. Cross Escherichia coli Probiotic Strain ED1a In. 2017;61(1):1–16. doi:10.1128/AAC.01293-16.
- Tannock GW, Tiong IS, Priest P, Munro K, Taylor C, Richardson A, Schultz M. Testing probiotic strain Escherichia coli Nissle 1917 (Mutaflor) for its ability to reduce carriage of multidrug-resistant E. coli by elderly residents in long-term care facilities. J Med Microbiol. 2011;60(3):366–370. doi:10.1099/jmm.0.025874-0.
- Moher D, Shamseer L, Clarke M, Ghersi D, Liberati A, Petticrew M, Shekelle P, Stewart LA. Preferred reporting items for systematic review and meta-analysis protocols (prisma-p) 2015 statement. Syst Rev. 2015;4(1):1–9. doi:10.1186/2046-4053-4-1.
- Amir-Behghadami M, Janati A. Population, Intervention, Comparison, Outcomes and Study (PICOS) design as a framework to formulate eligibility criteria in systematic reviews. Emerg Med J. 2020;37(6):387. doi:10.1136/emermed-2020-209567.
- Wang F, Feng J, Chen P, Liu X, Ma M, Zhou R, Chang Y, Liu J, Li J, Zhao Q. Probiotics in Helicobacter pylori eradication therapy: Systematic review and network meta-analysis. Clin Res Hepatol Gastroenterol [Internet]. 2017;41(4):466–475. Available from: 10.1016/j.clinre.2017.04.004.
- Penumetcha SS, Ahluwalia S, Irfan R, Khan SA, Rohit Reddy S, Vasquez Lopez ME, Zahid M, Busmail A, Mohammed L. The efficacy of probiotics in the management of Helicobacter Pylori: A systematic review. Cureus. 2021;13. doi:10.7759/cureus.20483.
- Richard A, Laura R, Harshavardhan K, Sonia M, Khamis HJ, Paliy O. Distal Gut Microbiota of Adolescent Children is Different from That of Adults. Fems Microbiology Ecology. 2011;77(2):404–412. doi:10.1111/j.1574-6941.2011.01120.x.
- The Jamovi Project [Internet]. 2020; Available from: https://www.jamovi.org.
- Wallace BC, Dahabreh IJ, Trikalinos TA, Lau J, Trow P, Schmid CH. Closing the gap between methodologists and end-users: R as a computational back-end. J Stat Softw. 2012;49(5):1–15. doi:10.18637/jss.v049.i05.
- Lin L, Chu H, Murad MH, Hong C, Qu Z, Cole SR, Chen Y. Empirical comparison of publication bias tests in meta-analysis. Journal Of General Internal Medicine. 2018;33(8):1260–1267. doi:10.1007/s11606-018-4425-7.
- Van Enst WA, Ochodo E, Scholten RJ, Hooft L, Leeflang MM. Investigation of publication bias in meta-analyses of diagnostic test accuracy: A meta-epidemiological study. BMC Med Res Methodol. 2014;14(1):1–11. doi:10.1186/1471-2288-14-70.
- Alberda C, Marcushamer S, Hewer T, Journault N, Kutsogiannis D. Feasibility of a lactobacillus casei drink in the intensive care unit for prevention of antibiotic associated diarrhea and clostridium difficile. Nutrients. 2018;10(5):10. doi:10.3390/nu10050539.
- Barker AK, Duster M, Valentine S, Hess T, Archbald-Pannone L, Guerrant R, Safdar N. A randomised controlled trial of probiotics for Clostridium difficile infection in adults (PICO). J Antimicrob Chemother. 2017;72(11):3177–3180. doi:10.1093/jac/dkx254.
- Dall LB, Lausch KR, Gedebjerg A, Fuursted K, Storgaard M, Larsen CS. Do probiotics prevent colonisation with multi-resistant Enterobacteriaceae during travel? A randomised controlled trial. Travel Med Infect Dis [Internet]. 2019;27:81–86. Available from: 10.1016/j.tmaid.2018.11.013.
- De Regt MJA, Willems RJL, Hené RJ, Siersema PD, Verhaar HJJ, Hopmans TEM, Bonten MJM. Effects of probiotics on acquisition and spread of multiresistant enterococci. Antimicrob Agents Chemother. 2010;54(7):2801–2805. doi:10.1128/AAC.01765-09.
- Doron S, Hibberd PL, Goldin B, Thorpe C, McDermott L, Snydman DR. Effect of Lactobacillus rhamnosus GG administration on vancomycin-resistant Enterococcus colonisation in adults with comorbidities. Antimicrob Agents Chemother. 2015;59(8):4593–4599. doi:10.1128/AAC.00300-15.
- Eggers S, Barker AK, Valentine S, Hess T, Duster M, Safdar N. Effect of Lactobacillus rhamnosus HN001 on carriage of Staphylococcus aureus: Results of the impact of probiotics for reducing infections in veterans (IMPROVE) study. BMC Infect Dis. 2018;18(1):1–8. doi:10.1186/s12879-018-3028-6.
- Gerding DN, Meyer T, Lee C, Cohen SH, Murthy UK, Poirier A, Van Schooneveld TC, Pardi DS, Ramos A, Barron MA. et al. Administration of spores of nontoxigenic clostridium difficile strain M3 for prevention of recurrent C difficile infection: A randomised clinical trial. JAMA - J Am Med Assoc. 2015;313(17):1719–1727. doi:10.1001/jama.2015.3725.
- He C, Kong F, Chai X, Zou C, Zhu X, Zhao D, Tan LTH. Effect of probiotic-assisted eradication of caga +/vaca s1m1 helicobacter pylori on intestinal flora. Biomed Res Int. 2022;2022:1–12. doi:10.1155/2022/8607671.
- Klarin B, Wullt M, Palmquist I, Molin G, Larsson A, Jeppsson B. Lactobacillus plantarum 299v reduces colonisation of Clostridium difficile in critically ill patients treated with antibiotics. Acta Anaesthesiol Scand. 2008;52(8):1096–1102. doi:10.1111/j.1399-6576.2008.01748.x.
- Kwon JH, Bommarito KM, Reske KA, Seiler SM, Hink T, Babcock HM, Kollef MH, Fraser VJ, Burnham CAD, Dubberke ER. Randomised controlled trial to determine the impact of probiotic administration on colonisation with multidrug-resistant organisms in critically ill patients. Infect Control Hosp Epidemiol. 2015;36(12):1451–1454. doi:10.1017/ice.2015.195.
- Magee HR. Probiotic treatment of vancomycin-resistant enterococci: A randomised controlled trial. Med J Aust. 2007;187(5):320. doi:10.5694/j.1326-5377.2007.tb01267.x.
- Mcfarland LV, Surawicz CM, Elmer GW, Moyer KA, Melcher SA, Fekety R, Bowen KE, Cox JL, Noorani Z, Harrington G. et al. A randomised placebo-controlled trial of saccharomyces boulardii in combination with standard antibiotics for clostridium difficile disease. JAMA: J Am Med Assoc. 1994;271(24):1913–1918. doi:10.1001/jama.1994.03510480037031.
- Nouvenne A, Ticinesi A, Meschi T. Carbapenemase-producing Klebsiella pneumoniae in elderly frail patients admitted to medical wards. Ital J Med. 2015;9:116–119. doi:10.4081/itjm.2014.476.
- Rauseo AM, Hink T, Reske KA, Seiler SM, Bommarito KM, Fraser VJ, Burnham CAD, Dubberke ER. A randomized controlled trial of Lactobacillus rhamnosus GG on antimicrobial-resistant organism colonization. Infect Control Hosp Epidemiol. 2022;43(2):167–173. doi:10.1017/ice.2021.94.
- Rubin IMC, Mollerup S, Broholm C, Knudsen SB, Baker A, Helms M, Holm MKA, Kallemose T, Westh H, Dahl Knudsen J. et al. No effect of lactobacillus rhamnosus gg on eradication of colonization by vancomycin-resistant enterococcus faecium or microbiome diversity in hospitalized adult patients. Microbiol Spectr. 2022;10(3):1–12. doi:10.1128/spectrum.02348-21.
- Salomão MCC, Heluany-Filho MA, Menegueti MG, de Kraker MEA, Martinez R, Bellissimo-Rodrigues F. A randomised clinical trial on the effectiveness of a symbiotic product to decolonise patients harboring multidrug-resistant gram-negative bacilli. Rev Soc Bras Med Trop. 2016;49(5):559–566. doi:10.1590/0037-8682-0233-2016.
- Sullivan Å, Johansson A, Svenungsson B, Nord CE. Effect of lactobacillus F19 on the emergence of antibiotic-resistant microorganisms in the intestinal microflora. J Antimicrob Chemother. 2004;54(4):791–797. doi:10.1093/jac/dkh406.
- Lawrence SJ, Korzenik JR, Mundy LM. Probiotics for recurrent Clostridium difficile disease [1]. J Med Microbiol. 2005;54(9):905–906. doi:10.1099/jmm.0.46096-0.
- Surawicz CM, McFarland LV, McFarland LV, Greenberg RN, Rubin M, Fekety R, Mulligan ME, Garcia RJ, Brandmarker S, Bowen K. et al. The search for a better treatment for recurrent clostridium difficile disease: Use of high-dose vancomycin combined with saccharomyces boulardii. Clin Infect Dis. 2000;31(4):1012–1017. doi:10.1086/318130.
- Szachta P, Ignyś I, Cichy W. An evaluation of the ability of the probiotic strain lactobacillus rhamnosus GG to eliminate the gastrointestinal carrier state of vancomycin-resistant enterococci in colonised children. J Clin Gastroenterol. 2011;45(10):872–877. doi:10.1097/MCG.0b013e318227439f.
- Tang B, Tang L, Huang C, Tian C, Chen L, He Z, Yang G, Zuo L, Zhao G, Liu E. et al. The effect of probiotics supplementation on gut microbiota after helicobacter pylori eradication: A multicenter randomized controlled trial. Infect Dis Ther [Internet]. 2021;10(1):317–333. Available from: 10.1007/s40121-020-00372-9.
- Viazis N, Argyriou K, Kotzampassi K, Christodoulou DK, Apostolopoulos P, Georgopoulos SD, Liatsos C, Giouleme O, Koustenis K, Veretanos C. et al. A four-probiotics regimen combined with a standard helicobacter pylori-eradication treatment reduces side effects and increases eradication rates. Nutrients. 2022;14(3):1–10. doi:10.3390/nu14030632.
- Warrack S, Panjikar P, Duster M, Safdar N. Tolerability of a probiotic in subjects with a history of methicillin-resistant Staphylococcus aureus colonisation. Benef Microbes. 2014;5(4):389–396. doi:10.3920/BM2013.0062.
- Wieërs G, Verbelen V, Van Den Driessche M, Melnik E, Vanheule G, Marot JC, Cani PD. Do probiotics during in-hospital antibiotic treatment prevent colonisation of gut microbiota with multi-drug-resistant bacteria? a randomized placebo-controlled trial comparing saccharomyces to a mixture of lactobacillus, bifidobacterium, and saccharomyce. Front Public Heal. 2021;8:1–10. doi:10.3389/fpubh.2020.578089.
- Wullt M, Hagslätt MLJ, Odenholt I. Lactobacillus plantarum 299v for the treatment of recurrent clostridium difficile-associated diarrhoea: A double-blind, placebo-controlled trial. Scand J Infect Dis. 2003;35(6–7):365–367. doi:10.1080/00365540310010985.
- Yang C, Liang L, Lv P, Liu L, Wang S, And WZ, Chen Y. Effects of non-viable Lactobacillus reuteri combining with 14-day standard triple therapy on Helicobacter pylori eradication: A randomized double-blind placebo-controlled trial. Helicobacter. 2021;26(6):e12856. doi:10.1111/hel.12856.
- Oh B, Kim BS, Kim JW, Kim JS, Koh SJ, Kim BG, Lee KL, Chun J. The effect of probiotics on gut microbiota during the helicobacter pylori eradication: randomized controlled trial. Helicobacter. 2016;21(3):165–174. doi:10.1111/hel.12270.
- Rajani J, Dastar B, Samadi F, Karimi Torshizi MA, Abdulkhani A, Esfandyarpour S. Effect of extracted galactoglucomannan oligosaccharides from pine wood (Pinus brutia) on Salmonella typhimurium colonisation, growth performance and intestinal morphology in broiler chicks. Br Poult Sci. 2016;57(5):682–692. doi:10.1080/00071668.2016.1200013.
- Adhikari P, Cosby DE, Cox NA, Franca MS, Williams SM, Gogal RM, Ritz CW, Kim WK. Effect of dietary fructooligosaccharide supplementation on internal organs Salmonella colonisation, immune response, ileal morphology, and ileal immunohistochemistry in laying hens challenged with Salmonella enteritidis. Poult Sci [Internet]. 2018;97(7):2525–2533. Available from: 10.3382/ps/pey101.
- Matsumoto K, Ichimura M, Tsuneyama K, Moritoki Y, Tsunashima H, Omagari K, Hara M, Yasuda I, Miyakawa H, Kikuchi K. et al. Fructo-oligosaccharides and intestinal barrier function in a methionine–choline-deficient mouse model of nonalcoholic steatohepatitis. PLoS One. 2017;12(6):1–14. doi:10.1371/journal.pone.0175406.
- Mao B, Gu J, Li D, Cui S, Zhao J, Zhang H, Chen W. Effects of different doses of fructooligosaccharides (FOS) on the composition of mice fecal microbiota, especially the bifidobacterium composition. Nutrients. 2018;10(8):10. doi:10.3390/nu10081105.
- Ribeiro AML, Vogt LK, Canal CW, Cardoso MRDI, Labres RV, Streck AF and Bessa MC. Effects of Prebiotics and probiotics on the colonisation and immune response of broiler chickens challenged with Salmonella Enteritidis. Brazilian J Poult Sci [Internet]. 2007;9(3):193–200. Available from: http://www.sensores-de-medida.es/sensing_sl/SENSORES-Y-TRANSDUCTORES_35/Células-de-carga—Sensores-de-fuerza_69/Sensores-de-fuerza-tracción-compresión_239/Célula-de-carga-patrón-KAL_230.html.
- Monteagudo-Mera A, Arthur JC, Jobin C, Keku T, Bruno-Barcena AJ, Azcarate-Peril MA. High purity galacto-oligosaccharides (GOS) enhance specific Bifidobacterium species and their metabolic activity in the mouse gut microbiome. BM. 2016;7(2):247–264. doi:10.3920/BM2015.0114.
- Lin SH, Chou LM, Chien YW, Chang JS, Lin CI. Prebiotic Effects of Xylooligosaccharides on the Improvement of Microbiota Balance in Human Subjects. Gastroenterol Res Pract. 2016;2016:1–6. doi:10.1155/2016/5789232.
- Rajani J, Dastar B, Samadi F, Karimi Torshizi MA, Abdulkhani A, Esfandyarpour S. Effect of extracted galactoglucomannan oligosaccharides from pine wood (Pinus brutia) on Salmonella typhimurium colonisation, growth performance and intestinal morphology in broiler chicks. Br Poult Sci. 2016;57:682–692. doi:10.1080/00071668.2016.1200013.
- Pourabedin M, Chen Q, Yang MM, Zhao X-M, Nakatsu C. Mannan- and xylooligosaccharides modulate caecal microbiota and expression of inflammatory-related cytokines and reduce caecal Salmonella Enteritidis colonisation in young chickens. FEMS Microbiol Ecol. 2017;93(1):1–11. doi:10.1093/femsec/fiw226.
- Spring P, Wenk C, Dawson KA, Newman KE. The effects of dietary mannanoligosaccharides on cecal parameters and the concentrations of enteric bacteria in the ceca of salmonella-challenged broiler chicks. Poult Sci [Internet]. 2000;79(2):205–211. Available from: 10.1093/ps/79.2.205.
- Lowry VK, Farnell MB, Ferro PJ, Swaggerty CL, Bahl A, Kogut MH. Purified β-glucan as an abiotic feed additive up-regulates the innate immune response in immature chickens against Salmonella enterica serovar Enteritidis. Int J Food Microbiol. 2005;98(3):309–318. doi:10.1016/j.ijfoodmicro.2004.06.008.
- Piewngam P, Zheng Y, Nguyen TH, Dickey SW, Joo HS, Villaruz AE, Glose KA, Fisher EL, Hunt RL, Li B. et al. Pathogen elimination by probiotic Bacillus via signalling interference. Nature [Internet]. 2018;562(7728):532–537. Available from: 10.1038/s41586-018-0616-y.
- Chen MJ, Chen CC, Huang YC, Tseng CC, Hsu JT, Lin YF, Fang YJ, Wu MS, Liou JM. The efficacy of Lactobacillus acidophilus and rhamnosus in the reduction of bacterial load of Helicobacter pylori and modification of gut microbiota—a double-blind, placebo-controlled, randomized trial. Helicobacter. 2021;26(6):1–14. doi:10.1111/hel.12857.
- Fishbein SRS, Mahmud B, Dantas G. Antibiotic perturbations to the gut microbiome. Nat Rev Microbiol. 2023;21(12):772–788. doi:10.1038/s41579-023-00933-y.
- Schwartz DJ, Langdon AE, Dantas G. Understanding the impact of antibiotic perturbation on the human microbiome. Genome Med. 2020;12(1):1–12. doi:10.1186/s13073-020-00782-x.
- Ramirez J, Guarner F, Bustos Fernandez L, Maruy A, Sdepanian VL, Cohen H. Antibiotics as major disruptors of gut Microbiota. Front Cell Infect Microbiol. 2020;10:1–10. doi:10.3389/fcimb.2020.572912.
- Shah T, Baloch Z, Shah Z, Cui X, Xia X. The intestinal microbiota: Impacts of antibiotics therapy, colonisation resistance, and diseases. Int J Mol Sci. 2021;22(12):22. doi:10.3390/ijms22126597.
- McFarland LV, Goh S. Are probiotics and prebiotics effective in the prevention of travellers’ diarrhea: A systematic review and meta-analysis. Travel Med Infect Dis. 2019;27:11–19. doi:10.1016/j.tmaid.2018.09.007.
- Titelman E, Hasan CM, Iversen A, Nauclér P, Kais M, Kalin M, Giske CG. Faecal carriage of extended-spectrum β-lactamase-producing Enterobacteriaceae is common 12 months after infection and is related to strain factors. Clin Microbiol Infect. 2014;20(8):O508–O515. doi:10.1111/1469-0691.12559.
- Galina D, Valdovska A. Effect of probiotics and herbals on health and shedding of resistant Escherichia coli in piglets. Res Rural Dev. 2017;1:251–258.
- Deep S. Probiotics may offset gut damage caused by antibiotics. Med News Today [Internet]. 2023; Available from: https://www.medicalnewstoday.com/articles/probiotics-may-offset-gut-damage-caused-by-antibiotics.
- Luchen CC, Chibuye M, Spijker R, Simuyandi M, Chisenga C, Bosomprah S, Chilengi R, Schultsz C, Mende DR, Harris VC. Impact of antibiotics on gut microbiome composition and resistome in the first years of life in low- to middle-income countries: A systematic review. PLoS Med [Internet]. 2023;20(6):1–20. Available from: 10.1371/journal.pmed.1004235.
- Patangia DV, Anthony Ryan C, Dempsey E, Paul Ross R, Stanton C. Impact of antibiotics on the human microbiome and consequences for host health. Microbiologyopen. 2022;11(1):1–23. doi:10.1002/mbo3.1260.
- McDonnell L, Gilkes A, Ashworth M, Rowland V, Harries TH, Armstrong D, White P. Association between antibiotics and gut microbiome dysbiosis in children: systematic review and meta-analysis. Gut Microbes [Internet]. 2021;13(1):1–18. Available from: 10.1080/19490976.2020.1870402.
- Hemarajata P, Versalovic J. Effects of probiotics on gut microbiota: Mechanisms of intestinal immunomodulation and neuromodulation. Therap Adv Gastroenterol. 2013;6(1):39–51. doi:10.1177/1756283X12459294.
- Fernández-Alonso M, Aguirre Camorlinga A, Messiah SE, Marroquin E. Effect of adding probiotics to an antibiotic intervention on the human gut microbial diversity and composition: A systematic review. J Med Microbiol. 2022;71:001625. doi:10.1099/jmm.0.001625.
- Kesavelu D, Jog P. Current understanding of antibiotic associated dysbiosis and approaches for its management. Ther Adv Infect Dis. 2023;10:1–18. doi:10.1177/20499361231154443.
- Ooi CY, Dilley AV, Day AS. Saccharomyces boulardii in a child with recurrent Clostridium difficile. Pediatr Int. 2009;51(1):156–158. doi:10.1111/j.1442-200X.2008.02782.x.
- Wombwell E, Patterson ME, Bransteitter B, Gillen L. The effect of saccharomyces boulardii primary prevention on risk of hospital-onset clostridioides difficile infection in hospitalized patients administered antibiotics frequently associated with c. difficile infection. Clinical Infectious Diseases. 2021;73(9):e2512–e2518. doi:10.1093/cid/ciaa808.
- Latif A, Shehzad A, Niazi S, Zahid A, Ashraf W, Iqbal MW, Rehman A, Riaz T, Aadil RM, Khan IM. et al. Probiotics: mechanism of action, health benefits and their application in food industries. Front Microbiol. 2023;14:14. doi:10.3389/fmicb.2023.1216674.
- Plaza-Diaz J, Ruiz-Ojeda FJ, Gil-Campos M, Gil A. Mechanisms of Action of Probiotics. Adv Nutr. 2019;10:S49–66. doi:10.1093/advances/nmy063.
- Christensen HR, Larsen CN, Kæstel P, Rosholm LB, Sternberg C, Michaelsen KF, Frøkiær H. Immunomodulating potential of supplementation with probiotics: a doseâ??response study in healthy young adults. FEMS Immunol Med Microbiol. 2006;47(3):380–390. doi:10.1111/j.1574-695X.2006.00109.x.
- Éliás AJ, Barna V, Patoni C, Demeter D, Veres DS, Bunduc S, Erőss B, Hegyi P, Földvári-Nagy L, Lenti K. Probiotic supplementation during antibiotic treatment is unjustified in maintaining the gut microbiome diversity: a systematic review and meta-analysis. BMC Med [Internet]. 2023;21(1):1–18. Available from: 10.1186/s12916-023-02961-0.
- Washburn RL, Sandberg D, Gazdik Stofer MA. Supplementation of a single species probiotic does not affect diversity and composition of the healthy adult gastrointestinal microbiome. Hum Nutr Metab [Internet]. 2022;28:200148. Available from: 10.1016/j.hnm.2022.200148.
- Muwonge A, Karuppannan AK, Opriessnig T. Probiotics mediated gut microbiota diversity shifts are associated with reduction in histopathology and shedding of Lawsonia intracellularis. Anim Microbiome. 2021;3(1):3. doi:10.1186/s42523-021-00084-6.
- Grazul H, Kanda LL, Gondek D. Impact of probiotic supplements on microbiome diversity following antibiotic treatment of mice. Gut Microbes [Internet]. 2016;7(2):101–114. Available from: 10.1080/19490976.2016.1138197.
- Wang Z, Li L, Wang S, Wei J, Qu L, Pan L, Xu K. The role of the gut microbiota and probiotics associated with microbial metabolisms in cancer prevention and therapy. Front Pharmacol. 2022;13(1):e1–e8. doi:10.1055/s-0041-1742095.
- Indiani CMDSP, Rizzardi KF, Castelo PM, Ferraz LFC, Darrieux M, Parisotto TM. Child obes and Firmicutes/Bacteroidetes ratio in the gut Microbiota: A systematic review. Childhood Obesity. 2018;14(8):501–509. doi:10.1089/chi.2018.0040.
- Magne F, Gotteland M, Gauthier L, Zazueta A, Pesoa S, Navarrete P, Balamurugan R. The Firmicutes/Bacteroidetes ratio: A relevant marker of gut dysbiosis in obese patients? Nutrients. 2020;12(5):12. doi:10.3390/nu12051474.
- Stojanov S, Berlec A, Štrukelj B. The influence of probiotics on the Firmicutes/Bacteroidetes ratio in the treatment of obesity and inflammatory bowel disease. Microorganisms. 2020;8(11):1715–1716. doi:10.3390/microorganisms8111715.
- Rizzatti G, Lopetuso LR, Gibiino G, Binda C, Gasbarrini A. Proteobacteria: A common factor in human diseases. Biomed Res Int. 2017;2017:1–7. doi:10.1155/2017/9351507.
- Brown GD, Taylor PR, Reid DM, Willment JA, Williams DL, Martinez-Pomares L, Wong SYC, Gordon S. Dectin-1 is a major β-glucan receptor on macrophages. J Exp Med. 2002;196(3):407–412. doi:10.1084/jem.20020470.
- Brown GD, Gordon S. A new receptor for β-glucans. Nature. 2001;413(6851):36–37. doi:10.1038/35092620.
- Jin Y, Li P, Wang F. β-glucans as potential immunoadjuvants: A review on the adjuvanticity, structure-activity relationship and receptor recognition properties. Vaccine. 2018;36(35):5235–5244. Available from: 10.1016/j.vaccine.2018.07.038.
- Macfarlane GT, Cummings JH. Probiotics and prebiotics: Can regulating the activities of intestinal bacteria benefit health? West J Med. 1999;171(3):187–191.
- Gibson GR, Probert HM, Van LJ, Rastall RA, Roberfroid MB. Dietary modulation of the human colonic microbiota: updating the concept of prebiotics. Nutr Res Rev. 2004;17(2):259–275. doi:10.1079/NRR200479.
- Ji J, Jin W, Liu SJ, Jiao Z, Li X. Probiotics, prebiotics, and postbiotics in health and disease. MedComm. 2023;4(6):1–23. doi:10.1002/mco2.420.
- Hartemink R, Van Laere KMJ, Rombouts FM. Growth of enterobacteria on fructo-oligosaccharides. J Appl Microbiol. 1997;83(3):367–374. doi:10.1046/j.1365-2672.1997.00239.x.
- Valyshev AV, Kirillov VA, Kirillov DA, Bukharin OV. The effect of inulin on the biological properties of enterobacteria. Zh Mikrobiol Epidemiol i Immunobiol. 2000;1:79–80.
- Gibson GR. Dietary modulation of the human gut microflora using the prebiotics oligofructose and inulin. J Nutr [Internet]. 1999;129(7):1438S–1441S. Available from: 10.1093/jn/129.7.1438S.
- Jung TH, Jeon WM, Han KS. In vitro effects of dietary inulin on human fecal microbiota and butyrate production. J Microbiol Biotechnol. 2015;25(9):1555–1558. doi:10.4014/jmb.1505.05078.
- Langlands SJ, Hopkins MJ, Coleman N, Cummings JH. Prebiotic carbohydrates modify the mucosa associated microflora of the human large bowel. Gut. 2004;53(11):1610–1616. doi:10.1136/gut.2003.037580.
- Kolida S, Tuohy K, Gibson GR. Prebiotic effects of inulin and oligofructose. Br J Nutr. 2002;87(S2):S193–7. doi:10.1079/BJN/2002537.
- Delgado-Fernández P, Corzo N, Olano A, Hernández-Hernández O, Moreno FJ. Effect of selected prebiotics on the growth of lactic acid bacteria and physicochemical properties of yoghurts. Int Dairy J. 2019;89:77–85. doi:10.1016/j.idairyj.2018.09.003.
- Akhavan N, Hrynkiewicz K, Thiem D, Randazzo C, Walsh AM, Guinan KJ, O’Sullivan JT, Stadnicka K. Evaluation of probiotic growth stimulation using prebiotic ingredients to optimise compounds for in ovo delivery. Front Microbiol. 2023;14:1–12. doi:10.3389/fmicb.2023.1242027.