ABSTRACT
Autism spectrum disorder (ASD) is a neurodevelopmental disorder affecting over 1% of the global population. Individuals with ASD often exhibit complex behavioral conditions, including significant social difficulties and repetitive behaviors. Moreover, ASD often co-occurs with several other conditions, including intellectual disabilities and anxiety disorders. The etiology of ASD remains largely unknown owing to its complex genetic variations and associated environmental risks. Ultimately, this poses a fundamental challenge for the development of effective ASD treatment strategies. Previously, we demonstrated that daily supplementation with the probiotic Lactiplantibacillus plantarum PS128 (PS128) alleviates ASD symptoms in children. However, the mechanism underlying this improvement in ASD-associated behaviors remains unclear. Here, we used a well-established ASD mouse model, induced by prenatal exposure to valproic acid (VPA), to study the physiological roles of PS128 in vivo. Overall, we showed that PS128 selectively ameliorates behavioral abnormalities in social and spatial memory in VPA-induced ASD mice. Morphological examination of dendritic architecture further revealed that PS128 facilitated the restoration of dendritic arborization and spine density in the hippocampus and prefrontal cortex of ASD mice. Notably, PS128 was crucial for restoring oxytocin levels in the paraventricular nucleus and oxytocin receptor signaling in the hippocampus. Moreover, PS128 alters the gut microbiota composition and increases the abundance of Bifidobacterium spp. and PS128-induced changes in Bifidobacterium abundance positively correlated with PS128-induced behavioral improvements. Together, our results show that PS128 treatment can effectively ameliorate ASD-associated behaviors and reinstate oxytocin levels in VPA-induced mice, thereby providing a promising strategy for the future development of ASD therapeutics.
Introduction
Autism spectrum disorder (ASD) is a prevalent neurodevelopmental disorder characterized by complex behavioral traits, including social difficulties and repetitive behaviors, alongside frequent comorbidity with intellectual disability and anxiety disorders.Citation1,Citation2 ASD is caused by various genetic variations and environmental risks.Citation3,Citation4 Interestingly, individuals with ASD often experience gastrointestinal problems such as diarrhea, gastric reflux, and inflammatory bowel disease.Citation5,Citation6 Moreover, meta-analyses of the relative abundance of bacterial genera within the gut microbiota of patients with ASD have indicated a link between ASD and alterations in gut microbiota composition.Citation7 Several studies in humans and mice have also demonstrated that fecal transplantation of the gut microbiome from ASD donors into germ-free mice can induce ASD-like behaviors.Citation8–10 Together, these findings suggest a direct link between gut microbiota composition and ASD development.
The gut microbiome has been shown to modulate the central nervous system through the gut-brain axis.Citation11–13 Gut microbes have been reported to influence various neural processes, including neurogenesis, axonal myelination, dendritic arborization, synaptic transmission, synaptic plasticity, and blood – brain barrier permeability.Citation14,Citation15 However, due to the complexity of the gut-brain system, the precise mechanism by which gut microbes regulate brain function remains largely unknown. Several hypotheses and initial findings suggest various pathways, which can generally be divided into two classes that are not necessarily mutually exclusive. The indirect pathway involves the production of some microbial molecular byproducts, such as metabolites, neurotransmitters, and inflammatory mediators, that affect the host’s hormone regulation, immune system, and brain function.Citation8,Citation16 Additionally, the direct pathway involves the vagus nerve, which serves as the principal nerve of the parasympathetic system connecting visceral organs to brain regions such as the hypothalamus, amygdala, brainstem, and insular cortex, and is known to provide a direct transmission link in the gut-brain axis to facilitate bidirectional gut-brain communication.Citation17
Probiotics are microorganisms that offer health benefits by modulating the homeostasis of the gut – brain axis, thereby improving the physical and mental health of the host.Citation18,Citation19 Previously, we demonstrated that Lactiplantibacillus plantarum PS128 (PS128) increases dopamine and serotonin levels in the striatum, reducing anxiety- and depression-like behaviors in mice.Citation20 Clinical studies have further demonstrated that PS128 supplementation reduces oppositional/defiant behavior, improves attention and communication skills, and increases personal autonomy in patients with ASD.Citation21,Citation22 Recently, the administration of probiotics to improve ASD-associated behaviors has become a key focus of clinical research. However, there remains a significant gap in our understanding regarding the mechanisms responsible for these beneficial effects of microbial administration.
Oxytocin is a neuropeptide primarily synthesized in the hypothalamus of the brain. In addition to its well-known roles in the periphery reproductive organs, oxytocin also has important functions in the central nervous system that have received much attention due to its strong association with ASD. In the brain, oxytocin is released from the axon terminals of oxytocin-producing neurons, and acts on the oxytocin receptors, a type of G protein-coupled receptors, to initiate intracellular signaling. Interestingly, oxytocin receptors are expressed in key regions, including the hypothalamus, amygdala, hippocampus, and prefrontal cortex, which are known for their importance in social recognition and memory, as well as stress and emotional behaviors.Citation23–28 Moreover, autistic children generally have lower levels of oxytocinCitation29 and mice lacking oxytocin receptors show impaired social behavior.Citation30 Together, these findings suggest that dysfunction of the oxytocin circuitry or disruption of oxytocin receptor-mediated signaling may contribute to the pathophysiology underlying core symptoms of ASD.
Notably, antibiotic depletion of the gut microbiota alters oxytocin and Oxtr expression, suggesting that the gut microbiota is somehow related to the oxytocin system.Citation31,Citation32 Moreover, oxytocin treatment has been shown to reverse social deficits in several mouse models of ASD.Citation33–35 Therefore, whether probiotics can be used as an ASD intervention is an emerging area of research. Indeed, some probiotics, particularly the Bifidobacterium and Lactobacillus, have been shown to be beneficial in improving ASD-associated behaviors.Citation13,Citation36 While the intact vagus nerve and oxytocin receptors appear to be critical for the effect of probiotics such as Lactobacillus reuteri on social behavior,Citation13 how oxytocin or oxytocin receptor-mediated signaling and neural function are regulated by the probiotics remains largely unexplored.
In the present study, we aimed to characterize the effects of PS128 on ASD-associated behavioral abnormalities in a mouse model of ASD induced by prenatal exposure to valproic acid (VPA). Using multidisciplinary approaches including immunohistochemistry, biochemistry, mouse genetics, metagenomic sequencing, and behavioral assays, we provide mechanistic evidence that PS128 supplementation significantly alleviates ASD dysfunction in the brain circuitry and improves animal behavior by altering the gut microbiota composition and restoring oxytocin levels in the brain.
Results
PS128 reduces ASD-associated behaviors in a VPA-induced ASD mouse model
Epidemiological studies have revealed an increased risk of fetal ASD development in mothers exposed to VPA during the first trimester of pregnancy.Citation4 Additionally, prenatal exposure to VPA has been shown to induce ASD-like behaviors in animal models.Citation37,Citation38 Therefore, we established an ASD mouse model by injecting VPA into pregnant mothers at embryonic day 12.5 (E12.5). The prenatally VPA-exposed offspring (VPA mice) were then given access to either pure water or PS128 mixed with their daily drinking water for a period of 4 weeks; this supplementation began at the age of one month, immediately after weaning ().
Figure 1. PS128 rescues behavioral abnormalities of the VPA mice.
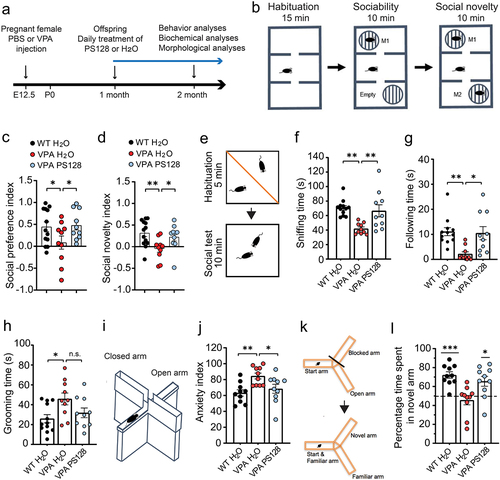
Subsequently, the effects of PS128 treatment on social behavior were evaluated using a well-established three-chamber social test () and Figure S1a–b). In the sociability test ( and Figure S1a), wild-type (WT) mice spent significantly more time interacting with the mouse than with the empty cup as expected, reflecting the natural preference of mice to socialize. In contrast, the VPA mice showed comparable interacting time and no preference to the mouse and the empty cup, indicating and impairment in sociability. Remarkably, the VPA mice treated with PS128 showed significant recovery in their sociability (WT H2O vs. VPA H2O, p = .0446; VPA H2O vs. VPA PS128, p = .0391; WT H2O vs. VPA PS128, p = .8451). In the social novelty test ( and Figure S1b), WT mice showed higher preference to the novel mouse than the familiar one, but the VPA mice did not. Similar to the sociability test, we also observed that the social novelty preference can be fully restored by PS128 supplement (WT H2O vs. VPA H2O, p = .0048; VPA H2O vs. VPA PS128, p = .0389; WT H2O vs. VPA PS128, p = .4587).
To validate these findings, we used another independent test of social behavior: a reciprocal social interaction test (). We closely monitored how the test mouse interacted with a new, unfamiliar, and freely moving reference mouse and measured the interaction time the test mouse spent in different types of social behaviors. Similar to the three-chamber social test, VPA mice spent less time interacting with the reference mouse in sniffing, and following the reference mouse (WT H2O vs. VPA H2O: sniffing, p = .0011, and following: p = .0041). However, PS128 was found to significantly reverse deficits in these social behaviors (VPA PS128 vs. VPA H2O: sniffing, p = .0071, and following, p = .0106; VPA PS128 vs. WT H2O: p > .05 for both sniffing and following behaviors). Notably, PS128 treatment had no apparent improvement effect on self-grooming behavior of VPA-treated mice, an indicator of repetitive behaviorCitation39 in VPA mice ().
Anxiety is one of the most common comorbidities in patients with ASD.Citation40 Therefore, we performed the elevated plus maze test to evaluate the anxiety levels in our mouse model. The elevated plus maze () was constructed according to the understanding that mice possess a natural aversion to staying in open and elevated locations, while also exhibiting exploratory behavior when placed in a novel environment.Citation41 We found that VPA mice spent significantly more time in the closed arms than WT mice (VPA H2O vs. WT H2O: p = .0069), indicating that VPA exposure induced heightened anxiety behaviors. In contrast, PS128-treated VPA mice spent a similar amount of exploratory time as the WT mice (VPA PS128 vs. WT H2O: p = .6942; VPA PS128 vs. VPA H2O, p = .0462) demonstrating the beneficial effects of PS128 in alleviating the elevated anxiety levels observed in VPA-induced ASD mice.
Alongside social deficits, children with prenatal VPA exposure have been reported to exhibit cognitive behavioral abnormalities.Citation42,Citation43 To determine whether PS128 regulates cognitive function in VPA mice, we assessed the spatial and reference memories of these mice using a Y maze testCitation44 and analyzed their time spent in the novel arm or previously visited familiar arm (). As expected, WT mice displayed a natural preference for the novel arm, spending approximately 72% of their time exploring this arm (p = .0001). Conversely, VPA mice showed almost no preference (46%, p = .389). Nonetheless, PS128-treated VPA mice exhibited significant improvements, dedicating approximately 65% of their time to exploring the novel arm (p = .0156). Taken together, our findings demonstrated that mice prenatally exposed to VPA exhibited ASD-like behavioral abnormalities in social communication, anxiety, and animal cognition. Nevertheless, PS128 supplementation significantly ameliorated these behavioral abnormalities in VPA mice.
PS128 restores morphological neuronal deficits in VPA mice
Behavioral deficits have been postulated to result from abnormalities in dendritic structure and spine density, which consequently disrupt neuronal connectivity and circuit function. Moreover, pyramidal neurons in the hippocampal CA1 region of postmortem human brains and animal models of ASD have been reported to exhibit reduced dendritic arborization and spine number.Citation45–49 We therefore used Thy1-green fluorescent protein (GFP) mice, which exhibit GFP expression in a limited number of pyramidal neurons in the brain, including hippocampus and cortex, to visualize and analyze individual neuron morphology in vivo. Citation50 Notably, this Thy1-GFP mouse line has undergone more than 10 rounds of backcrossing and has been consistently maintained on the same genetic background as all other mice used in the study. We have also confirmed Thy1-GFP mice showed comparable social behaviors, anxiety levels, and spatial memory to the WT, Thy1-GFP negative, littermates (Figure S1c-h).
To ascertain whether VPA alters neuron morphology in the brain, we injected pregnant Thy1-GFP transgenic mice with VPA at E12.5. Immediately after weaning at one month of age, we provided the Thy1-GFP offspring with pure or PS128-containing drinking water for 4 weeks, and collected brain samples at two months of age (). Subsequently, we conducted confocal z-stack imaging of GFP-expressing pyramidal neurons in the hippocampal CA1 & CA2 regions and prefrontal cortex (). This enabled the three-dimensional reconstruction of dendritic arbors for morphological analyses.
Figure 2. PS128 restores dendritic morphology of VPA mice.
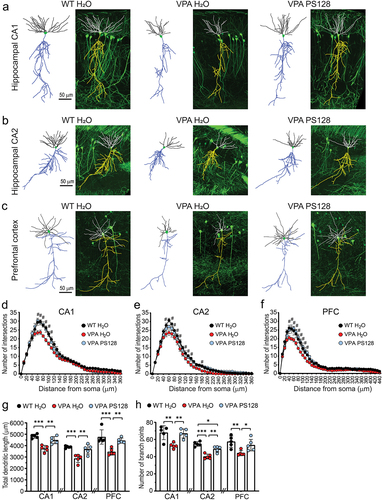
Overall, we found that neurons of VPA mice exhibited decreased dendritic arborizations, particularly at a distance of 60–100 μm from the soma in all three brain regions we examined (). Moreover, we detected significant reductions in total dendritic length and the number of branch points in the hippocampal CA1 and CA2 regions and prefrontal cortex (, dendritic length: VPA H2O vs. WT H2O: CA1, p < .0001, CA2 p = .0009, prefrontal cortex, p = .001; , branch point: VPA H2O vs. WT H2O: CA1, p = .0041, CA2 p < .0001, prefrontal cortex, p = .0085). Notably, PS128 treatment restored these disruptions in dendritic morphology in the VPA mice (, dendritic length: VPA PS128 vs. VPA H2O: CA1, p = .002, CA2 p = .0046, prefrontal cortex, p = .0073; , branch point: VPA PS128 vs. VPA H2O: CA1, p = .0063, CA2 p = .0015, prefrontal cortex, p = .0442). These PS128-mediated improvements were evident in both the basal and apical dendrites of pyramidal neurons, despite the understanding that different parts of dendrites receive input from different afferent neurons and brain regions (Figure S2).
PS128 restores neuronal connectivity of VPA mice
Dendritic spines are small protrusions stemming from the dendritic shafts. Excitatory synapses are localized within these specialized structures, facilitating the detection and transmission of chemical and electrical signals between neurons within the brain circuit.Citation51,Citation52 Notably, spine density is commonly used as an indicator for this neuronal connectivity. To investigate the impact of PS128 on neuronal connectivity, we measured the number of spines in the secondary dendrites of pyramidal neurons within the hippocampus and prefrontal cortex (). Through this, we revealed that VPA mice exhibited an overall reduction of approximately 25% in spine density in both basal and apical dendrites across the hippocampal CA1 (, VPA H2O vs. WT H2O: basal, p = .0137, and apical, p = .01), CA2 (, VPA H2O vs. WT H2O: basal, p = .0002, and apical, p = .0011), and prefrontal cortical (, VPA H2O vs. WT H2O: basal, p = .0008, and apical, p = .0002) regions. Nonetheless, PS128 supplementation of VPA mice effectively restored spine density in all examined dendritic areas and brain regions (, VPA H2O vs. VPA PS128: CA1 basal, p = .0484, and apical, p = .0141; , CA2 basal, p = .002, and apical, p = .0252; , PFC basal, p = .0107, and apical, p = .0033).
Figure 3. PS128 rescues spine loss in VPA mice.
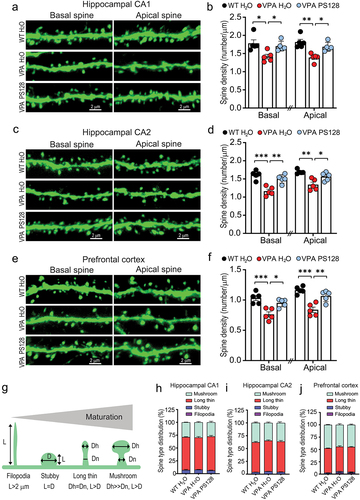
Spines can be morphologically classified into several types based on their maturity, including filopodia, stubby, long-thin, and mushroom-shaped spines ().Citation52 Therefore, we analyzed these four spine types within the pyramidal neurons of the hippocampus and prefrontal cortex. We found that the distribution of spine types in pyramidal neurons of hippocampus and prefrontal cortex were comparable among all experimental groups (). However, detailed analyses of each spine type revealed that the main spine types of pyramidal neurons in two-month-old mice were long-thin and mushroom-shaped; and the density of these two more mature spine types were much reduced in almost all dendritic areas and brain regions examined in VPA mice (Figure S3c–d, S3g–h and S3k–l). In summary, VPA significantly impaired dendritic arborization and spine density in the hippocampus and prefrontal cortex of mice. These disruptions were postulated to affect the neuronal connectivity and synaptic transmission required for effective neuronal circuit function. Therefore, the ability of PS128 to alleviated VPA-induced behavioral abnormalities appears to be linked to its capacity to restore dendritic morphology and promote neuronal connectivity.
PS128 restores dysregulated signaling and glutamate receptor expression in VPA mice
To investigate the molecular mechanism through which PS128 restores neuronal morphology in VPA mice, we performed biochemical analyses on key proteins present in hippocampal homogenates (). The proteins we analyzed in this study have been recognized to play a pivotal role in the regulation of excitatory synaptic structure and plasticity. Specifically, these proteins encompassed signaling molecules, such as Erk, CaMKII, and PKA,Citation53–57 as well as AMPA and NMDA-type of glutamate receptors.Citation58,Citation59 Since the density of dendritic spines was significantly reduced in VPA mice, we also performed subcellular fractionation, enabling analysis of these proteins in the post-synaptic density (PSD) fractions ().
Figure 4. PS128 restores reduced kinase activities and synaptic glutamate receptors in the hippocampus of VPA mice.
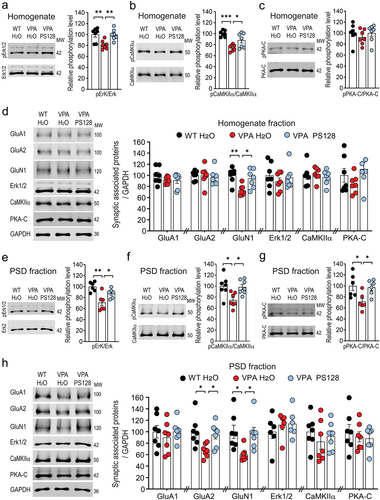
Our biochemical analysis revealed that the levels of phosphorylated or active Erk1/2 and CaMKIIα were diminished in both the homogenate and PSD fractions of VPA mice; nonetheless, PS128 supplementation significantly restored the activities of these kinases (, Homogenate: Erk1/2: VPA H2O vs. WT H2O, p = .0071; VPA H2O vs. VPA PS128, p = .0084. CaMKIIα: VPA H2O vs. WT H2O, p = .0001; VPA H2O vs. VPA PS128, p = .0272; , PSD fraction: Erk1/2: VPA H2O vs. WT H2O, p = .0012; VPA H2O vs. VPA PS128, p = .0497. CaMKIIα: VPA H2O vs. WT H2O, p = .0144; VPA H2O vs. VPA PS128, p = .0244.) Moreover, a reduction in phosphorylated Thr197 on the catalytic subunit of PKA (PKA-C) was observed in the PSD fraction of VPA mice (, VPA H2O vs. WT H2O, p = .0256; VPA H2O vs. VPA PS128, p = .0432;) but remained consistent with the control in the homogenate (, VPA H2O vs. WT H2O, p = .6476; VPA H2O vs. VPA PS128, p = .6433).
VPA mice exhibit significant impairments in social behavior () and spatial memory (), suggesting a disruption of neurotransmission in the hippocampus. Therefore, we examined glutamate receptor levels in the hippocampal homogenate and PSD fractions. Expression of GluN1, the obligatory NMDA receptor subunit, was reduced in both the homogenate and PSD fractions of VPA mice (, Homogenate: VPA H2O vs. WT H2O, p = .0039; VPA H2O vs. VPA PS128, p = .0213; , PSD fraction: VPA H2O vs. WT H2O, p = .0205; VPA H2O vs. VPA PS128, p = .0317). Expression of the GluA2 subunit of the AMPA receptor was also reduced in the PSD fraction of VPA mice (, VPA H2O vs. WT H2O, p = .0137; VPA H2O vs. VPA PS128, p = .0496). Based on these biochemical results, we postulated that the reductions in Erk1/2, CaMKIIα, and PKA kinases activities at the synapses may affect signaling cascades, gene transcription, and disruption of glutamate receptor signaling. Moreover, the observed alterations in GluN1 and GluA2 expression may impair excitatory synaptic transmission and plasticity, and resultant animal behavior.
PS128 restores oxytocin levels in the paraventricular nucleus of VPA mice
The microbiota gut-brain axis has been shown to influence host neuronal activity by modulating neuropeptide expression.Citation13,Citation60,Citation61 Notably, VPA has been reported to alter transcriptional profiles in rodent brains and human forebrain organoids, and these affected genes are enriched in neuronal development, synaptic function, calcium signaling, and oxytocin signaling pathways.Citation62,Citation63 Oxytocin, known for its potent role in regulating of social circuit function, is found at lower levels in children with ASD,Citation29 and oxytocin treatment has been shown to reverse social deficits in several mouse models of ASD.Citation33–35 In addition, oxytocin regulates Erk, CaMKII, PKA activities, as they are known downstream targets of oxytocin receptors. The deficient kinase activities observed in the VPA mice, along with the restoration of kinase activities by PS128 (), suggest that PS128 may potentially restore oxytocin signaling through the regulation of oxytocin expression.
To test this hypothesis, we performed immunostaining for oxytocin in brain sections containing the paraventricular nucleus (PVN) of the hypothalamus, where oxytocin-producing neurons are enriched (). Subsequent quantification of oxytocin florescent signals revealed an apparent reduction in oxytocin fluorescence intensity within the PVN of VPA mice (, VPA H2O vs. WT H2O, p < .0001). Moreover, the number of detectable oxytocin positive cells was significantly decreased (, VPA H2O vs. WT H2O, p = .0223). Nonetheless, these reductions in PVN oxytocin levels and oxytocin-positive cells were restored by PS128 treatment (, VPA H2O vs. VPA PS128, p = .0059; , VPA H2O vs. VPA PS128, p = .0357). Collectively, these findings demonstrate that prenatal exposure to VPA reduces oxytocin levels in the PVN, while PS128 supplementation can be used to restore oxytocin expression to WT levels. Notably, the vagal nerve originating from the gut is known to project to and innervate the PVN, forming a hypothalamic – vagal oxytocinergic neural circuit.Citation64,Citation65 This implies that PS128 may elevate oxytocin expression in the PVN through the vagal nerve-dependent microbiota – brain axis.
Figure 5. PS128 restores oxytocin expression in the PVN of VPA mice.
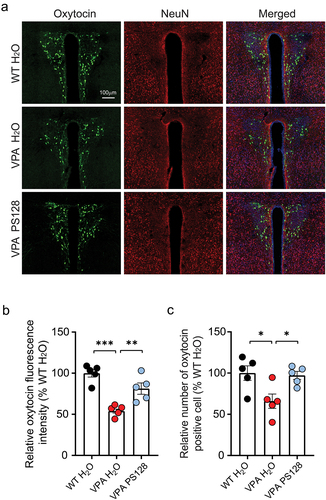
PS128 restores behavioral deficits in VPA mice via oxytocin receptor signaling
Oxytocin is a ligand for oxytocin receptors, which are G protein-coupled receptors responsible for activating the Gq protein subunit and initiating downstream intracellular signaling pathways. Brain mapping studies have identified the presence of oxytocin receptors in several brain regions including the olfactory system, basal ganglia, hippocampus, central amygdala, prefrontal cortex, and hypothalamus.Citation23–25 Notably, oxytocin receptor signaling plays a crucial role in hippocampus-dependent social memory and prefrontal cortex – dependent social cognition.Citation26,Citation27,Citation66 Therefore, PS128 may enhance oxytocin signaling along the gut-brain axis, facilitating communication between the PVN, hippocampus, and prefrontal cortex, which could potentially ameliorate social and memory deficits in VPA mice.
To determine whether PS128 improves ASD-associated behavior by modulating oxytocin signaling, we conducted behavioral experiments using oxytocin receptor knockout (Oxtr-KO) mice.Citation30,Citation67 After confirming the complete loss of oxytocin receptor transcripts in hippocampal tissues (, p = .0022), we injected VPA into pregnant Oxtr-KO females at E12.5; then, the Oxtr-KO VPA offspring were provided with pure water or water mixed with PS128 for 4 weeks as our previous experimental design ().
Figure 6. PS128 loses ability to rescue VPA-induced behaviors in mice lacking oxytocin receptors.
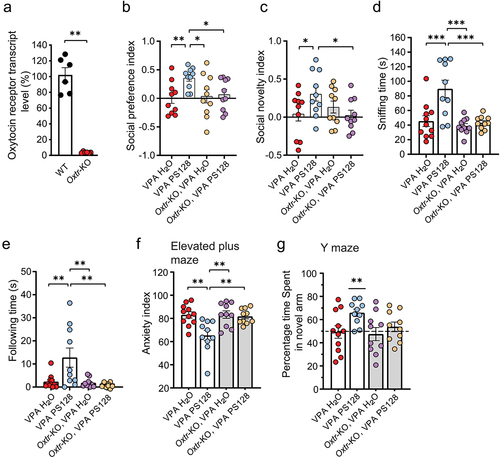
We first compared the social behavior of VPA mice supplemented with H2O or PS128, and Oxtr-KO VPA mice supplement with H2O and PS128 using the three-chamber test to determine whether PS128 acts through oxytocin receptors to improve the behavioral performance of VPA mice. Similar to the VPA mice, Oxtr-KO VPA mice that were given regular drinking water showed neither preference in sociability nor social novelty. Notably, while daily supplementation of PS128 recovers these social preferences in the VPA mice, it exhibited no improvements in the Oxtr-KO VPA mice (, social preference: VPA PS128 vs. VPA H2O, p = .0088; VPA PS128 vs. Oxtr-KO VPA H2O, p = .0179; VPA PS128 vs. Oxtr-KO VPA PS128, p = .0308; , social novelty: VPA PS128 vs. VPA H2O, p = .0347; VPA PS128 vs. Oxtr-KO VPA H2O, p = .1564; VPA PS128 vs. Oxtr-KO VPA PS128, p = .0241).
Likewise, PS128 also failed to restore the social behaviors of sniffing and following unfamiliar mice in the VPA-treated Oxtr-KO mice in the reciprocal social test (, sniffing: VPA PS128 vs. VPA H2O, p = .0008; VPA PS128 vs. Oxtr-KO VPA H2O, p = .0001; VPA PS128 vs. Oxtr-KO VPA PS128, p = .0005; , following: VPA PS128 vs. VPA H2O, p = .0056; VPA PS128 vs. Oxtr-KO VPA H2O, p = .0045; VPA PS128 vs. Oxtr-KO VPA PS128, p = .0034). These results demonstrate that oxytocin receptors are required for the PS128-mediated restoration of social behaviors.
Since VPA increased anxiety levels and impaired spatial memory (), we also tested these ASD-associated behaviors in Oxtr-KO mice. VPA mice treated with PS128 exhibited a restoration in anxiety in the elevated plus maze. However, all Oxtr-KO VPA mice, regardless of treatment with pure or PS128-containing water, retained high anxiety levels (, VPA PS128 vs. VPA H2O, p = .0018; VPA PS128 vs. Oxtr-KO VPA H2O, p = .002; VPA PS128 vs. Oxtr-KO VPA PS128, p = .0052). These results indicate that PS128 requires oxytocin receptors to ameliorate anxiety-associated behavior. In the spatial memory test, VPA PS128 mice spent more time exploring the novel arm (>65%), consistent with our previous results (). However, PS128 failed to restore this preference for exploring the novel arm in the Oxtr-KO VPA group (, VPA H2O, p = .9017; VPA PS128, p = .0008; Oxtr-KO VPA H2O, p = .6633; Oxtr-KO VPA PS128, p = .3823), indicating that PS128 affects hippocampus-dependent memory via the oxytocin receptor signaling pathway.
Concurrently with this set of experiments, we also established alongside a set of pregnant WT females with vehicle or VPA injections to produce WT and VPA-treated offspring, which were also provided with pure water or water supplemented water with PS128 for control comparisons (Figure S4). Notably, we did not observe any enhancement in social behavior, anxiety, and spatial memory in WT mice treated with PS128, suggesting that PS128 does not affect basal behavior traits in WT mice. Taken together, our results demonstrate that PS128 restores social and memory deficits in VPA mice but not in Oxtr-KO VPA mice, supporting a critical role for oxytocin receptor signaling in the reduction of ASD-associated behaviors via the PS128-mediated gut-brain axis.
PS128 alters gut microbiota composition and increases Bifidobacterium abundance
Our findings demonstrate that PS128 supplementation alleviates ASD-associated behaviors and concurrently restores neuronal morphology and oxytocin expression in the VPA-induced ASD mouse model. Motivated by these positive effects, we investigated the impact of PS128 on gut microbiota composition, and explored potential correlations between microbiota changes and behavioral performance. We first collected a total of 61 fecal samples, with 10–11 samples from each group, to examine microbiota composition through full-length 16S rRNA bacterial sequencing. The average number of reads per sample was 18,123, ranging from 8,329 to 48,209 reads, and only sequences that passed quality control were used for subsequent analyses (Figure S5a). The rarefaction curves (Figure S5b) reach a plateau, indicating that the sampling effort has effectively captured the majority of microbial diversity.
Next, we used an analytical approach based on amplicon sequence variants (ASVs) to calculate alpha diversity to gain insights into the diversity of the gut microbial community in the fecal samples. The Chao1 index showed comparable community richness across all groups, indicating a similar number of bacterial species in these mice (). However, the Shannon index showed a decrease in species richness and evenness in VPA PS128 mice, indicating a biased community structure with imbalanced bacterial species in these mice (, VPA PS128 vs. WT H2O, p = .0017; VPA PS128 vs. Oxtr-KO VPA H2O, p = .011). Beta diversity was also analyzed to assess differences in microbiota composition among groups. Principal coordinates analysis-weighted UniFrac (PCoA-wUniFrac) distance, utilizing species abundance to weight branch length based on abundance differences, revealed distinctive diversity patterns depending on whether mice were provided with pure or PS128-supplemented water ().
Figure 7. PS128 increases Bifidobacterium abundance in the gut, which correlates with improvements in ASD-associated behaviors in VPA mice.
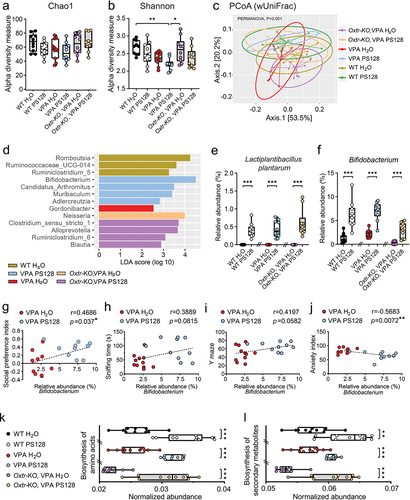
To determine whether PS128 supplementation affected the abundance of other microorganisms, we used the Linear Discriminant Analysis (LDA) Effect Size (LEfSe) algorithm to calculate differential abundance in each group. The resulting cladogram generated by LEfSe revealed a significant branching pattern of phylogenetic distribution in VPA and Oxtr-KO VPA mice treated with PS128 (Figure S5c). Utilizing LEfSe, dominant genera of the microbiota in each group were identified, and the differential abundance of each taxon was compared. Notable, Bifidobacterium, Candidatus Arthromitus, Muribaculum, and Adlercreutzia were the dominant taxonomic groups in VPA PS128 mice (). Conversely, Gordonibacter were enriched only in VPA mice provided with regular water ( and Figure S5l).
To determine whether the abundance of specific microbes could influence animal behavior, we analyzed the correlation between the relative abundance of individual microorganisms found in mouse fecal samples and corresponding behavioral traits, including sociability, anxiety, and spatial memory. The relative abundances of L. plantarum, Bifidobacterium, Candidatus Arthromitus, Muribaculum, and Adlercreutzia in the mouse fecal samples exhibited different patterns among the groups ( and Figure S5i–k). Notably, Bifidobacterium was the only one that showed significant differences between the PS128-supplemented and water-treated groups, suggesting that PS128 may facilitate the colonization of Bifidobacterium in the gut (, p < .0001 for comparisons between WT H2O vs. WT PS128, VPA H2O vs. VPA PS128, and Oxtr-KO VPA H2O vs. Oxtr-KO VPA PS128). Moreover, L. plantarum was not identified in any of the groups provided with regular drinking water, indicating that PS128 is an exogenous species in the mouse gut microbiota (, p < .0001 for comparisons between WT H2O vs. WT PS128, VPA H2O vs. VPA PS128, and Oxtr-KO VPA H2O vs. Oxtr-KO VPA PS128). Candidatus Arthromitus was enriched only in the VPA PS128 group (Figure S5i). Further analyzing the correlation of relative abundance between L. plantarum and other species showed that only the abundance of Bifidobacterium exhibited the positive correlation with L. plantarum (Figure S5d–h).
Interestingly, the relative abundance of Bifidobacterium in fecal samples from VPA H2O and VPA PS128 groups demonstrated a significant positive correlation with the social preference index (, r = 0.4686, p = .037), suggesting a beneficial effect of Bifidobacterium on social behavior. Moreover, the relative abundance of Bifidobacterium in the same groups of mice showed a negative correlation with the anxiety index (, r = 0.5683, p = .0072), indicating a reduction with the animal’s anxiety behavior. Bifidobacterium abundance also showed a tendency to correlate positively with sniffing time in the reciprocal social test (, r = 0.3889, p = .0815) and memory performance in the Y maze test (, r = 0.4197, p = .0582), although these associations did not reach statistical significance.
For functional analysis of the microbiota, we used the Tax4Fun2 algorithm to compare predicted functional pathways using 16s RNA sequence data from the gut microbiota.Citation68 Overall, we found that the pathways associated with the biosynthesis of amino acids and secondary metabolites were significantly upregulated in all groups that received PS128 supplementation (, p < .0001 for comparisons between WT H2O vs. WT PS128, VPA H2O vs. VPA PS128, Oxtr-KO VPA H2O vs. Oxtr-KO VPA PS128; , WT H2O vs. WT PS128, p = .0015; VPA H2O vs. VPA PS128, p < .0001; Oxtr-KO VPA H2O vs. Oxtr-KO VPA PS128, p < .0001).
Changes in the gut microbiome composition have been shown to alter the levels of gut metabolites; these changes can, in turn, affect host health and behavior through the gut – brain axis.Citation69,Citation70 Therefore, we further investigated whether PS128 functions independently or induces the production and release of metabolites, such as short-chain fatty acids, into the microenvironment, which subsequently activate gut signaling. In this study, we adopted a candidate-based approach to identify short-chain fatty acids linked to amino acid metabolic pathways or those with established effects on host health. For this analysis, we collected and quantified the short-chain fatty acids in mouse fecal samples using mass spectrometry. The concentrations of most short-chain fatty acids, including acetic, propionic, isobutyric, valeric, and isovaleric acid, were not significantly different among all groups (Figure S6). Nonetheless, butyric acid showed an approximately 2.2-fold increase in Oxtr-KO VPA mice treated with PS128 compared to those treated with water (Figure S6C). However, no behavioral improvements were observed in Oxtr-KO VPA mice treated with PS128 (); this indicated that the increased butyric acid had no detectable effect on the behavior of these mice. Taken together, these findings suggest that short fatty acids are not responsible for the PS128-associated restoration of behavioral performance in this VPA-induced ASD model.
Discussion
Emerging evidence from preclinical studies and animal research has highlighted the impact of gut microbiota dysbiosis on host brain function and social behavior, demonstrating the important role of the microbiota – brain axis as a bidirectional communication system linking the gut and the brain.Citation11,Citation12,Citation71 While the pathophysiology of ASD is multifaceted, involving genetic factors, environmental factors, and their interplay, the microbiota gut – brain axis offers an explanation for how these microorganisms can influence brain function and behavior. Several probiotics have been reported to have beneficial effects on a range of neurological disorders such as ASD, anxiety, depression, and Parkinson’s disease.Citation72,Citation73 We have demonstrated that PS128 treatment improves attention, communication skills, anxiety, and personal autonomy among individuals with ASD; these benefits appear to be consistent regardless of the initial genetic or environmental factors that contributed to the development of ASD and regardless of the extent of gut microbial community alterations.Citation21,Citation74,Citation75 To gain insight into the mechanistic effect of PS128 on ASD-associated behaviors, we treated VPA mice with PS128. Notably, PS128 not only ameliorated the core behavioral abnormalities associated with ASD in terms of social interactions (), but also demonstrated efficacy in addressing the key comorbidities of ASD in anxiety and cognitive deficits (). However, PS128 did not affect repetitive behavior (). Given that various neural circuits in the brain control behavior in distinct domains, the observations in this study suggest that PS128 selectively targets neural circuits that are crucial for social interactions, anxiety management, and learning behaviors, but does not target neural circuits associated with repetitive behavior.
Many neurological disorders are characterized by abnormalities in dendritic structures and synaptic pathologies, encompassing abnormal dendritic arborization, spine density, spine maturation, synaptic signaling, and synaptic plasticity. In postmortem hippocampal tissues, children with ASD display reduced dendritic complexity in pyramidal neurons compared with age-matched healthy children.Citation48 Abnormal dendritic outgrowth of hippocampal CA3 neurons has also been reported in a rat model of ASD induced by prenatal VPA exposure.Citation45 Moreover, genetic inactivation of CA2 pyramidal neurons has been linked to a significant loss of social memory.Citation76 Apart from the hippocampus, the prefrontal cortex is considered a key social hub involved in anxiety regulation and social preference.Citation77,Citation78 In the present study, we showed that VPA mice exhibited reduced dendritic complexity, dendritic length, and branch point number in both the hippocampus and prefrontal cortex (). Moreover, there was a notable decrease in the number of synaptic spines in the VPA mice (). These findings demonstrated that when female mice are exposed to VPA during pregnancy, their offspring exhibit deficits in dendritic arborization and spine density. These impairments in neuronal morphology and connectivity in prenatally VPA-exposed mice may contribute to altered circuit functions and the manifestation of ASD-associated behaviors. Overall, our findings are consistent with the established FDA warnings regarding VPA exposure. VPA and related products have been approved by the FDA for the treatment of seizures. However, these anti-seizure medications have been associated with adverse side effects in pregnant mothers, with their children being at a heightened risk of impaired cognitive development.Citation43
Ultimately, it is important to understand the pathophysiological mechanism responsible for the behavioral abnormalities observed in VPA mice and to determine how PS128 supplementation can reverse these deficits. In the present study, we showed that key synaptic signaling pathways, including Erk1/2, CaMKIIα, and PKA, were impaired in VPA mice (). Attenuated Erk signaling has been reported to reduce spine formation,Citation53 whereas increased Erk activity is associated with increased spine morphogenesis.Citation79 CaMKII is the most abundant kinase enriched in post-synaptic density, which modulates diverse neuronal functions, including glutamate receptor trafficking, actin polymerization, synaptic plasticity, mRNA translation, and gene transcription, through the activation of CREB.Citation56,Citation57 Additionally, CaMKII is essential for promoting dendritic spine growth and maintaining dendritic structures in the adult hippocampus.Citation80,Citation81 PKA, another key kinase that exhibits reduced activity in the VPA hippocampus, plays an important role in the cAMP-PAK pathway, which regulates various aspects of neuronal function, including synaptic remodeling, glutamate receptor insertion, synaptic plasticity, and dendrite outgrowth.Citation54,Citation55,Citation58
In addition to changes in kinase activity, reductions in the levels of glutamate receptor subunits GluN1 and GluA2 were also observed in the hippocampi of VPA mice (). GluN1, a major subunit of the NMDA receptor, plays a crucial role in excitatory synaptic transmission and plasticity. Interestingly, GluN1 expression can also be regulated by CaMKII-CREB signaling through transcriptional activation.Citation81,Citation82 Therefore, the reduced CaMKII activity observed in VPA mice may have resulted in the corresponding decrease in GluN1 protein expression. In addition, local GluA2 protein synthesis has been reported in dendrites, and it is tightly regulated by synaptic activity.Citation83–86 Overall, these findings suggest that prenatal exposure to VPA disrupts the kinase activity of Erk1/2, CaMKII, and PKA, thereby affecting the synaptic expression of glutamate receptors through transcriptional or translational regulation.
How does oral supplementation with PS128 probiotics influence kinase activities, thereby impacting glutamate receptors, circuit function and animal behavior in VPA mice? A key finding of this study is the restoration of oxytocin expression in the PVN (). Moreover, ErK1/2, CaMKIIα, and PKA, the kinases that exhibited reduced activity in VPA mice (), are downstream targets of the oxytocin receptors.Citation87 Given the critical role of oxytocin as a regulator of social circuit function, the restoration of oxytocin expression in the PVN is expected to restore oxytocin receptor activity and its subsequent signaling in the brain regions influenced by oxytocin. Thus, as part of the oxytocin-targeted social circuit, correction of deficient downstream kinase activities in the hippocampus () is likely to contribute to the beneficial effects of PS128 on synaptic glutamate receptor expression, neuronal connectivity, circuit function, and ASD-like behaviors in VPA mice.
Previous studies have highlighted the role of the microbiota in modulating behavior act via the vagal nerve, which innervates PVN neurons in the hypothalamus, forming a hypothalamic – vagal oxytocinergic neural circuit.Citation64,Citation65 Upon stimulation, PVN neurons release oxytocin, which then stimulates downstream circuits, including the hippocampus, amygdala, and prefrontal cortex.Citation23,Citation28,Citation64 Collectively, these results suggest that PS128 activates the hypothalamic – vagal oxytocinergic neural circuit, thereby restoring synaptic function and neuronal connectivity in the hippocampus and prefrontal cortex, ultimately ameliorating ASD-associated behaviors.
To further confirm that the rescue effect of PS128 in the VPA-induced ASD mouse model is mediated by restoring oxytocin signaling, we conducted behavioral experiments in VPA-treated Oxtr-KO mice. Oxtr-KO mice have been well documented to exhibit impaired social behavior in both the three-chamber assay and reciprocal social interaction test.Citation88–90 Interestingly, as the PS128 successfully reversed social behavior impairments in sociability, social novelty and reciprocal social interaction in VPA mice, it failed to do so in Oxtr-KO VPA mice (). This suggests that oxytocin receptor signaling plays a critical role in mediating the rescue effect of PS128’s on the core symptoms of ASD in social behavior. Additionally, it supports the understanding that PS128 enhances oxytocin receptor signaling in brain regions that are innervated by oxytocin-expressing neurons in the PVN. Notably, we did not observe an exacerbation of behavioral deficits in Oxtr-KO VPA mice when compared to VPA mice provided with water (). This could be attributed to the fact that prenatal exposure to VPA attenuates oxytocin signaling that shares the same signaling pathways as the Oxtr-KO. However, further research is required to validate this hypothesis.
In this study, PS128 treatment resulted in an increased abundance of specific bacterial genera in VPA mice, including Bifidobacterium and Candidatus Arthromitus. ( and Figure S5i). In particular, we found significant correlations between the abundance of Bifidobacterium in the VPA H2O vs. VPA PS128 groups and improvements in three-chamber sociability () and elevated plus maze performance in anxiety (). Additionally, correlations between Bifidobacterium abundance and reciprocal social behavior (), as well as memory performance (), approached borderline significance. These results suggest that the increased Bifidobacterium colonization facilitated by PS128 contributes to improvements in ASD-associated behaviors, at least in animal social and anxiety behaviors.
Interestingly, L. plantarum was exclusively detected in PS128-treated mice (), and its abundance exhibited a positive correlation with that of Bifidobacterium (Figure S5d). Notably, a lower relative abundance of Bifidobacterium spp. was found in the metagenomic analysis of the gut microbiota from fecal samples of children with ASD.Citation91 Furthermore, Bifidobacterium treatment has been reported to ameliorate social impairments in a rat model of ASD,Citation36 and consumption of Bifidobacterium longum 1714 has also been shown to reduce stress and improve cognitive function in healthy human volunteers.Citation92 These findings further support the role of PS128 supplementation in alleviating ASD-associated behaviors by promoting Bifidobacterium colonization in the gut of VPA mice. Therefore, coexistence of L. plantarum and Bifidobacterium in the host gut may be an effective strategy for maintaining gut microbiota homeostasis and improving mental health.
In our study, functional prediction analyses of the metagenomic sequence revealed that amino acid biosynthesis and secondary metabolite pathways were enriched in the PS128 groups (). Moreover, we observed an increase in the concentration of butyric acid in Oxtr-KO VPA PS128 mice (Figure S6c). However, no significant improvements in the behavioral performance in social, anxiety, and spatial memory tests were observed in these mice. These results suggest that there is no direct correlation between the increase in butyric acid levels and the observed behavioral outcomes. Nevertheless, butyric acid, a major product of intestinal microbial fermentation, has been reported to have beneficial effects on host inflammation, thermogenesis, and lipid and glucose metabolism.Citation93 Therefore, further studies are warranted to understand how PS128 increases butyric acid levels in the gut and to gain a deeper understanding of its potential effects on other aspects of host physiological functions.
PS128, an oral probiotic, was first isolated from the traditional fermented food Fu-tsai.Citation94 Our present study demonstrated that the ability of PS128 to restore oxytocin expression for proper oxytocin signaling, which in turn can restore dendritic impairments and ameliorate behavioral deficits in the VPA-induced ASD mouse model. Notably, PS128, an exogenous gut microbiota species, induces changes in the composition of the gut microbiota and promotes the abundance of the beneficial genus Bifidobacterium. In addition, independent clinical studies have reported beneficial effects of PS128 on various behavioral symptoms in children with ASD. In summary, our study represents the first attempt to elucidate the underlying mechanisms by which PS128 alleviates ASD-associated behavioral abnormalities in mice, and supports that PS128 may be a promising therapeutic candidate to alleviate ASD-associated behavioral symptoms.
Materials and methods
Animals
All animal care and treatment procedures were in accordance with institutional and NIH guidelines. Animals were group housed in cages with corn stalk crumbs for bedding and wooden sticks for enrichment at the institutional mouse facility maintained under standard conditions (room temperature ~ 22°C; relative humidity ~ 50%; light-dark cycle: 12-12 hrs). C57BL/6J wild-type (WT) mice were obtained from the National Laboratory Animal Center, Taiwan; EIIαCre mice were obtained from the IMB transgenic core at Academia Sinica; Thy1-GFP-M (Thy1-GFP) transgenic and oxytocin receptor conditional knockout (Oxtrfl/fl) mice were obtained from the Jackson Laboratory and all mice are maintained on the C57BL/6J background. All experiments were conducted on both sexes, except for the behavioral assays where only adult male mice were used to avoid the potential issue of the estrous cycle in females.
VPA-induced ASD mouse model
A single dose of 600 mg/kg VPA (Sigma Aldrich, P4543) injected intraperitoneally (IP) injected into pregnant WT females at E12.5 was used to induce ASD-like behaviors in the offering.Citation95 VPA injection caused a marked sedation of the dams for a few hours, with full recovery on the next day. For behavior and biochemical experiments, a total of 12 timed pregnant dams were injected with saline, and 24 timed pregnant dams were injected with VPA. Two pregnant dams receiving the same treatment were housed in the same cage. At one month of age, the offspring of saline-injected dams were weaned and provided with pure water as a WT control group. The offspring of VPA-injected dams were evenly divided into two groups and received either pure water or PS128-mixed water as VPA experimental groups.
Oxytocin receptor knockout (Oxtr-KO) mice
Oxtrfl/fl mice were crossed with EIIα-cre mice to generate whole body Oxtr-KO mice. The EIIα-Cre mouse line carries a cre transgene under the control of the adenovirus EIIα promoter, allowing direct germline deletion of the loxP-flanked oxytocin receptor gene at the early mouse embryonic stage. A total of 16 timed pregnant Oxtr-KO pregnant females were IP injected with VPA at E12.5. Since Oxtr-KO mice have problems with lactation, the newborn pups were fostered by nursing WT females to increase their survival rate and proper growth. Meanwhile, we also established a set of pregnant WT females with vehicle or VPA injections to produce WT and VPA-treated offspring for comparisons At one month of age, littermates of Oxtr-KO, WT or VPA dams were weaned and evenly divided into two groups to receive pure water or PS128-mixed water.
PS128 preparation and administration
L. plantarum PS128 (PS128) was cultured and prepared according to a previously published method.Citation20 Briefly, cryopreserved PS128 was awakened by anaerobic culture in De Man-Rogosa-Sharpe broth (BD Difco, Becton-Dickinson) at 37°C for 18 h, and then centrifuged at 6000×g for 10 min. The supernatants were removed, and the bacterial pellets were washed with saline and then centrifuged at 6000×g for 10 min. The PS128 were resuspended in pure water at a concentration of 10Citation9 CFU/ml. Mice from the same litter were evenly divided into two groups at their weaning age to receive either pure water or water mixed with PS128 as their sole source of drinking water throughout the period prior to experiments performed at 2 months of age. Water bottles were changed twice a week. The average water consumption is ~4 ml per day for each mouse, and no apparent difference in the consumption of water or water mixed with PS128 was observed.
Behavioral tests
The mouse cages were transferred to the test room for a one-hour acclimation prior to conducting any behavioral tests. All experiments were video recorded, and the movements and positions of the mice were automatically tracked using the ANY-maze tracking system. Human observers were blinded to the mouse groups.
Y maze test
Individual test mice were placed in the start arm of a Y maze arena, with either the left or right arm blocked by a sliding gate. After a 5 min of free access to the start and open arms, the mice were returned to their home cage for a 2 min rest period. The gate was removed from the blocked arm before the test mice were placed back into the start arm for a 3 min of free exploration of all arms. Memory performance was calculated as the time mice spent in the novel arm, divided by the total time spent in both the novel and familiar arms. If the mice did not member the previously visited arm, they were expected to have an equal probability of exploring the novel and familiar arms. A one-sample t-test was conducted to determine whether the memory performance was significantly greater than 50%.
Three-chamber social test
Individual test mice were first habituated and allowed free access for 10 min to an empty Plexiglas arena (60×40x25 cm, LxWxH) divided into three interconnected chambers with both sliding gates removed. The sociability test was performed in a second 10 min period during which individual test mice were allowed to interact with either an empty wire cup (Empty) or a wire cup containing an unfamiliar conspecific (Mouse1). The position of the empty cup or the cup containing Mouse1 in the left or right side chamber during the sociability test period was counterbalanced across trials to avoid chamber preference bias. During the third 10 min, social novelty was accessed by introducing another unfamiliar mouse (Mouse2) into the previously empty wire cup. Social interaction time to the empty cup, Mouse1 or Mouse2 was measured as the amount of time the test animal spent in close proximity to the cup (<2 cm of the test mouse’s head to the edge of the cup) to avoid scoring bias. Manual scoring was performed in a separate pilot experiment on wild-type mice to validate the proper parameter settings in the Any-maze. The preference index of each individual animals is defined as (Time Mouse1 - Time empty cup)/(Time Mouse1 + Time empty cup) for the sociability test, and (Time Mouse2 – Time Mouse1)/(Time Mouse2 + Time Mouse1) for the social novelty test. Mouse1 and Mouse2 were WT males of similar age or 1–2 weeks younger, no heavier than the test mice, and group housed in separate cages.Citation96 They were habituated to the wire cup restraint for 20 min per day for at least 3 days prior to the tests. Only mice without obvious aggregation and anxiety behaviors, such as biting the wire cup or excessive urination and defecation, were used in the tests.
Reciprocal social interaction
Individual test mice were placed in one side of a Plexiglas neutral arena (25×25x25 cm, LxWxH) with a diagonal divider, and an unfamiliar mouse (male of similar age or 1–2 weeks younger, not heavier than the test mouse) was placed in the other side of the arena. After 5 min of habituation, the divider was removed to allow the test mice to freely interact with the unfamiliar mice. Trained, independent observers monitored and manually scored social behaviors in following and sniffing (nose-to-nose or nose-to-anus) and self-grooming.
Elevated plus maze test
The apparatus consists of two open (stressful) arms and two closed (protective) arms (48×10x38 cm, LxWxH) and a center platform (10 × 10 cm) forming a cross, raised 40 cm above the ground. Individual test mice were placed in an open arm facing the center and allowed to freely explore the maze for 5 min. The anxiety index was defined as the percentage of time spent in the closed arms (more anxious) divided by the total time the mice spent in both the closed and open arms.
Dendrite reconstructions and analyses
After transcardiac perfusion with PBS followed by 4% paraformaldehyde (PFA), brains of Thy1-GFP mice were dissected, post-fixed in 4% PFA at 4°C overnight, and cut into serial coronal sections (300 μm) using a vibratome (Leica VT1200S). Sections were mounted in PermaFluor mounting medium (Epredia TA-030-FM), and GFP-filled pyramidal neurons were imaged using a 40X objective to visualize whole dendritic fields (512 × 512 pixels; 1-μm optical scan), followed by a 3 × 4 tile scan using a Zeiss LSM800 confocal microscope (Carl Zeiss). Z-stacks were stitched together using Zen software. All neurons analyzed were sufficiently bright to allow complete tracing of dendritic arbors. Neuronal somas and dendrites were manually traced using Imaris 9.5.1 software (Bitplane) to generate 3D reconstructions of dendritic structures. Dendritic complexity was analyzed by 3D Sholl analysis, and calculating the number of dendritic intersections with concentric spheres that radiating from the soma in 10-μm radius increments. Total dendritic length and branch point were also quantified using Imaris. Five neurons were traced per animal, and the average was used as the value for each mouse for statistical analysis.
Neuronal connectivity and morphology analyses
Neuronal connectivity, or the number of synaptic contacts, of hippocampal neurons is estimated as the number of spines per micrometer of dendrites in Thy1-GFP mice. Density Images of the first few splits of basal and apical secondary dendrites (around 50–150 μm away from the soma) of the hippocampal CA1 neurons were taken at resolution using a 63X objective to visualize individual spines. All apparent protrusions on dendrites, regardless of the shape, were counted as spines in the z-series images, and the total number of spines was divided by the dendritic length measured in Imaris. Spine morphology was classified as filopodia-, stubby-, long thin- and mushroom-shaped spines according to the criteria illustrated in . At least 10 dendrites with a total length of 2,000∼3,000 μm from 5 different neurons per mouse were collected for statistical analysis.
Hippocampal subcellular fractionation
Immediately after cervical dislocation, mouse hippocampi were rapidly isolated and homogenized in ice cold homogenization buffer (320 mM sucrose, 5 mM sodium pyrophosphate, 1 mM EDTA, 10 mM HEPES (pH 7.4), protease and phosphatase inhibitor cocktails (cOmplete, Mini, EDTA-free and PhosSTOP, Roche) by passage through a 26-gauge needle for 12 times. The homogenate was then centrifuged at 800×g for 10 min at 4°C to obtain pelleted fraction 1 (P1, nuclear fraction) and supernatant fraction 1 (S1, post-nuclear fraction). S1 was further centrifuged at 15,000×g for 20 min at 4°C to give P2 (membrane fraction) and S2 (cytosol fraction). P2 was resuspended in Milli-Q water, adjusted to 4 mM HEPES (pH 7.4), and incubated for 30 min at 4°C with agitation. The suspended P2 was centrifuged at 25,000×g for 20 min at 4°C to pellet LP1 (synaptosomal membrane fraction). LP1 was resuspended in 50 mM HEPES (pH 7.4), mixed with an equal volume of 1% Triton X-100, and incubated at 4°C with agitation for 15 min. The final PSD pellet, obtained by centrifugation at 32,000×g for 20 min at 4°C, was resuspended in 50 mM HEPES for protein quantification for standard Western blot analysis. The antibodies used were: GluA1and GluA2 (JHMI monoclonal antibody core), GluN1 (Millipore; MAB363), CaMKII (Cell Signaling; 4436), p-CaMKII (Thr286) (Cell Signaling; 12716), p-Erk1/2 (Cell Signaling; 9106), Erk1/2 (Santa Cruz; sc -135,900), p-PKA-C (Thr197) (Cell Signaling; 4781), PKA-C (Cell Signaling; 4782), and GAPDH (BioLenend; 649202).
Immunofluorescence analyses
After transcardiac perfusion, mouse brains were dissected and post-fixed in 4% PFA at 4°C overnight, cryoprotected in 30% sucrose in PBS for 48 hr, frozen in OCT (Tissue-Tek) on dry ice, and stored at −80°C. Prior to staining, sections were serially cut at 30 μm, washed in PBS, permeabilized in 0.25% Triton X-100 in PBS, and blocked with 10% normal goat serum + 3% BSA for 2 h. Sections were then incubated overnight with primary antibodies against oxytocin (1:200, Sigma-Aldrich; ab911) and NeuN (1:500, EMD Millipore; MAB377/A60). After three PBS washes, sections were incubated with goat anti-rabbit and mouse Alexa-Fluor-conjugated secondary antibodies (Invitrogen A-11008 and A-11004; 1:500) for 1 h. In some cases, nuclear counterstaining was performed with DAPI (Sigma-Aldrich, D9542) for 20 min before the final PBS wash. Sections were mounted in PermaFluor followed by confocal imaging with a 20X objective. Images were stitched together using Zen software to cover the entire PVN region. Oxytocin fluorescence intensity was measured in ImageJ by selecting regions of interest using the ROI manager and the measure function to store individual ROI coordinates and measurement results. The number of oxytocin positive cells in the PVN regions obtained were 2379, 1753, 2314 for the WT group, VPA H2O group, and VPA PS128 group, respectively.
Real-time qPCR analyses
Total RNA was prepared from dissected hippocampi using TRIzol-chloroform extraction (ThermoFisher; 10296–010). RNA was then reverse transcribed using a RETROscript Reverse Transcription Kit (ThermoFisher; AM1710). Real-time qPCR was performed using a Maxima SYBR Green/Rox Q-PCR Master Mix (ThermoFisher; K0221) in a 7300 Real-time PCR System (Applied Biosystems). Primers used were: Oxtr-F: 5’-GCACGGGTCAGTAGTGTCAAGC-3’, Oxtr-R: 5’- GGTTGCAGCAGCTGTTGAGG-3’; GAPDH-F: 5’-GGTGAAGCAGGCATCTGAGG-3’; and GAPDH-R: 5’- CACCACCCTGTTGCTGTAGC-3’. The PCR amplified products for Oxtr and GAPDH are 205 and 192 bp, respectively. The relative ΔCT (cycle threshold) was calculated as CTOxr – CTGAPDH. The ΔΔCT values of Oxtr-KO samples are normalized to WT samples and the resulting average CT values were obtained from three independent biological triplicates.
DNA extraction from fecal samples
Bacterial DNA was extracted and purified according to previously published methods with minor modifications.Citation97 Briefly, 100 mg of fecal sample was washed three times with PBS and resuspended in extraction buffer (100 mM Tris-HCl, 40 mM EDTA, 1% SDS; pH 9.0) containing 0.3 g of glass beads (0.1 mm in diameter) and 500 μL of buffer-saturated phenol. The mixture was shaken vigorously for 30s in the FastPrep FP120 homogenizer (Q-Biogene, CA, USA) at a speed setting of 5 m/s. The fecal sample was then centrifuged at 12,000×g for 5 min. 400 μl of the supernatant was added to an equal volume of phenol-chloroform-isoamyl alcohol (25:24:1, v/v). The mixture was again shaken in the FastPrep FP120 at a speed of 4 m/s for 45 s and then centrifuged at 12,000×g for 5 min. The DNA was precipitated with 3 M sodium acetate (pH 5.4) and isopropanol. The DNA was then air dried and dissolved in TE buffer (10 mM Tris-HCl, 1 mM EDTA, pH 8.0). DNA concentration was adjusted to 10 ng/μl and the samples were stored at − 20°C.
Amplicon sequencing
The universal primer set 27F (AGRGTTYGATYMTGGCTCAG) and 1492 R (RGYTACCTTGTTACGACTT) was used to amplify the full-length 16S rRNA gene from genomic DNA. For each sample’s amplified DNA product, sample-specific PacBio barcode sequences were added to both the forward and reverse 16S primers to allow multiplexed sequencing. PCR amplification was then performed using KAPA HiFi Hot Start DNA Polymerase (Kapa Biosystems) for 25 cycles with denaturation at 95°C for 30 seconds, annealing at 57°C for 30 seconds and extension at 72°C for 60 seconds. Quality control after amplification was conducted using a bioanalyzer (Agilent Technologies, Santa Clara, CA, USA) to assure sample accuracy. The amplified DNA from each sample was then pooled at equal concentrations before the start of library preparation. The amplified DNA pool was processed using the SMRTbell Express Template Prep Kit 2.0 (PacBio, USA) according to the Procedure & Checklist – Amplification of Full-Length 16S Gene with Barcoded Primers for Multiplexed SMRTbell® Library Preparation and Sequencing protocol (part number 101-599-700 Version 04, PACBIO). The SMRTbell libraries were prepared from pooled and barcoded fecal samples and sequenced on the Sequel II PacBio system using the Sequel II Sequencing Kit 2.0 (PacBio, USA) by Taiwan Genomic Industry Alliance Inc.
Sequence data analysis
A total of 61 fecal samples were collected for full-length 16S rRNA sequencing for gut microbiota characterization using the PacBio Sequel lle SMRT platform. The average number of reads per sample was 18,123, ranging from 8,329 to 48,209 reads. Following quality filtering, reads were processed using the R package DADA2.Citation98 Read orientation was synchronized during primer sequence trimming, and reads shorter than 1200 bp or longer than 1600 bp were removed, along with reads with an expected error greater than 5 or containing any ambiguous base. The DADA2 workflow included read de-replication, error model learning, amplicon sequence variant (ASV) inference, chimera removal and taxonomic assignment using the SILVA database v132.Citation99 All statistical analyses were performed in R (http://www.r-project.org/) unless otherwise specified. Chao1 and Shannon diversity indices were estimated using the phyloseq R package.Citation100 Hierarchical clustering based on the weighted UniFrac distance, using the complete linkage algorithm, was performed using Principal Coordinate Analysis (PCoA) using the ade4 R package.Citation101 The LEfSe method was used to identify significantly different bacterial taxa (LDA threshold >2) among samples from six groups. Relative bacterial abundance was calculated as the percentage of identified bacterial sequence reads out of the total reads for each sample. Functional prediction analysis was performed using the Tax4Fun2 R package, which predicts functional profiles and gene redundancies of prokaryotic communities from 16S rRNA gene sequences. The abundance of the sequences associated with different functional pathways was determined for each sample. Sequencing and bioinformatics analyses here were performed by Germark Biotechnology.
Statistical analysis
Statistical analyses for behavioral, morphological, biochemical experiments were performed using GraphPad Prism9 software. No sample was excluded from the analyses. When the data follows a normal distribution, we use the t-test to compare data between two groups. If there are three or more groups, we employ One-way ANOVA with Tukey or Fisher LSD post hoc test for analysis. If the data does not follow a normal distribution, the Mann-Whitney test is utilized to compare data between two groups. Regardless of the data type, the Bonferroni correction is applied to control the Type I error for multiple comparisons. The detailed analysis methods are annotated in each figure caption and the values are listed in the supplementary Excel file.
Supplemental Material
Download Zip (120.1 MB)Acknowledgments
We thank the Laboratory Animal Facility and Imaging Core facility of ICOB, Academia Sinica for the animal care and imaging assistance. We also thank Taiwan Genomic Industry Alliance Inc. for sequencing and bioinformatics services, Leeuwenhoek Laboratories for metabolite analysis, the Transgenic Core Facility of IMB, Academia Sinica (AS-CFII-113-A1) for the EIIa-Cre mice and the Data Science Statistical Cooperation Center of Academia Sinica (AS-CFII-111-215) for statistical support. Finally, we would like to thank Drs. Li-Hao Cheng, Chih-Chieh Hsu and Shih-Hsuan Cheng for their helpful discussions and comments.
Disclosure statement
C.-M.C., and C.-C.W. were employed by Bened Biomedical Co., Ltd. at the time of the study. Y.-C.T. serves as a consultant and owns stock in Bened Biomedical Co., Ltd. None of the other authors had any personal or financial conflict of interest. The funder had no role in the design of the study; in the collection, analysis, or interpretation of the data; in the writing of the manuscript; or in the decision to publish the results.
Data availability statement
The data supporting the findings of this study are available within the article and its supplementary materials. The raw sequencing data for all 61 samples are deposited on Figshare https://figshare.com/s/6e1b71b946d8f74a7505.
Supplementary material
Supplemental data for this article can be accessed online at https://doi.org/10.1080/19490976.2024.2359501
Additional information
Funding
References
- Sharma SR, Gonda X, Tarazi FI. Autism spectrum disorder: classification, diagnosis and therapy. Pharmacology & Therapeutics. 2018;190:91–28. doi:10.1016/j.pharmthera.2018.05.007.
- Lai MC, Lombardo MV, Baron-Cohen S. Autism. Lancet. 2014;383(9920):896–910. doi:10.1016/S0140-6736(13)61539-1.
- Lord C, Brugha TS, Charman T, Cusack J, Dumas G, Frazier T, Jones EJH, Jones RM, Pickles A, State MW. et al. Autism spectrum disorder. Nat Rev Dis Primers. 2020;6(1):5. doi:10.1038/s41572-019-0138-4.
- Kim YS, Leventhal BL. Genetic epidemiology and insights into interactive genetic and environmental effects in autism spectrum disorders. Biol Psychiatry. 2015;77(1):66–74. doi:10.1016/j.biopsych.2014.11.001.
- Madra M, Ringel R, Margolis KG. Gastrointestinal issues and autism spectrum disorder. Child Adolesc Psychiatr Clin N Am. 2020;29(3):501–513. doi:10.1016/j.chc.2020.02.005.
- Holingue C, Newill C, Lee LC, Pasricha PJ, Daniele Fallin M. Gastrointestinal symptoms in autism spectrum disorder: a review of the literature on ascertainment and prevalence. Autism Res. 2018;11(1):24–36. doi:10.1002/aur.1854.
- Xu M, Xu X, Li J, Li F. Association between gut microbiota and autism spectrum disorder: a systematic review and meta-analysis. Front Psychiatry. 2019;10:473. doi:10.3389/fpsyt.2019.00473.
- Chernikova MA, Flores GD, Kilroy E, Labus JS, Mayer EA, Aziz-Zadeh L. The brain-gut-microbiome system: pathways and implications for autism spectrum disorder. Nutrients. 2021;13(12):4497. doi:10.3390/nu13124497.
- Ding HT, Taur Y, Walkup JT. Gut microbiota and autism: key concepts and findings. J Autism Dev Disord. 2017;47(2):480–489. doi:10.1007/s10803-016-2960-9.
- Sharon G, Cruz NJ, Kang D-W, Gandal MJ, Wang B, Kim Y-M, Zink EM, Casey CP, Taylor BC, Lane CJ. et al. Human gut microbiota from autism spectrum disorder promote behavioral symptoms in mice. Cell. 2019;177(6):1600–1618 e1617. doi:10.1016/j.cell.2019.05.004.
- Borre YE, O’Keeffe GW, Clarke G, Stanton C, Dinan TG, Cryan JF. Microbiota and neurodevelopmental windows: implications for brain disorders. Trends Mol Med. 2014;20(9):509–518. doi:10.1016/j.molmed.2014.05.002.
- Vuong HE, Hsiao EY. Emerging roles for the gut microbiome in autism spectrum disorder. Biol Psychiatry. 2017;81(5):411–423. doi:10.1016/j.biopsych.2016.08.024.
- Sgritta M, Dooling SW, Buffington SA, Momin EN, Francis MB, Britton RA, Costa-Mattioli M. Mechanisms underlying microbial-mediated changes in social behavior in mouse models of autism spectrum disorder. Neuron. 2019;101(2):246–259 e246. doi:10.1016/j.neuron.2018.11.018.
- Garcia-Gutierrez E, Narbad A, Rodriguez JM. Autism spectrum disorder associated with gut microbiota at immune, metabolomic, and neuroactive level. Front Neurosci. 2020;14:578666. doi:10.3389/fnins.2020.578666.
- Cryan JF, O’Riordan KJ, Cowan CSM, Sandhu KV, Bastiaanssen TFS, Boehme M, Codagnone MG, Cussotto S, Fulling C, Golubeva AV. et al. The microbiota-gut-brain axis. Physiol Rev. 2019;99(4):1877–2013. doi:10.1152/physrev.00018.2018.
- Fung TC, Olson CA, Hsiao EY. Interactions between the microbiota, immune and nervous systems in health and disease. Nat Neurosci. 2017;20(2):145–155. doi:10.1038/nn.4476.
- Bonaz B, Bazin T, Pellissier S. The vagus nerve at the interface of the microbiota-gut-brain axis. Front Neurosci. 2018;12:49. doi:10.3389/fnins.2018.00049.
- Shabbir U, Arshad MS, Sameen A, Oh DH. Crosstalk between gut and brain in alzheimer’s disease: the role of gut microbiota modulation strategies. Nutrients. 2021;13(2):690. doi:10.3390/nu13020690.
- Butel MJ. Probiotics, gut microbiota and health. Méd et Mal Infectieuses. 2014;44(1):1–8. doi:10.1016/j.medmal.2013.10.002.
- Liu YW, Liu W-H, Wu C-C, Juan Y-C, Wu Y-C, Tsai H-P, Wang S, Tsai Y-C. Psychotropic effects of Lactobacillus plantarum PS128 in early life-stressed and naïve adult mice. Brain Res. 2016;1631:1–12. doi:10.1016/j.brainres.2015.11.018.
- Mensi MM, Rogantini C, Marchesi M, Borgatti R, Chiappedi M. Lactobacillus plantarum PS128 and other probiotics in children and adolescents with autism spectrum disorder: a real-world experience. Nutrients. 2021;13(6):2036. doi:10.3390/nu13062036.
- Liu YW, Liong, MT, Chung, YC, Huang, HY, Peng, WS, Cheng, YF, Lin, YS, Wu, YY, Tsai, YC. Effects of Lactobacillus plantarum PS128 on children with autism spectrum disorder in Taiwan: a randomized, double-blind, placebo-controlled trial. Nutrients. 2019;11(4):820. doi:10.3390/nu11040820.
- Acevedo-Rodriguez A, Mani SK, Handa RJ. Oxytocin and estrogen receptor beta in the brain: an overview. Front Endocrinol (Lausanne). 2015;6:160. doi:10.3389/fendo.2015.00160.
- Gimpl G, Fahrenholz F. The oxytocin receptor system: structure, function, and regulation. Physiol Rev. 2001;81(2):629–683. doi:10.1152/physrev.2001.81.2.629.
- Liao PY, Chiu YM, Yu JH, Chen SK. Mapping central projection of oxytocin neurons in unmated mice using cre and alkaline phosphatase reporter. Front Neuroanat. 2020;14:559402. doi:10.3389/fnana.2020.559402.
- Shi DD, Zhang Y-D, Ren Y-Y, Peng S-Y, Yuan T-F, Wang Z. Predictable maternal separation confers adult stress resilience via the medial prefrontal cortex oxytocin signaling pathway in rats. Mol Psychiatry. 2021;26(12):7296–7307. doi:10.1038/s41380-021-01293-w.
- Raam T, McAvoy KM, Besnard A, Veenema AH, Sahay A. Hippocampal oxytocin receptors are necessary for discrimination of social stimuli. Nat Commun. 2017;8(1):2001. doi:10.1038/s41467-017-02173-0.
- Knobloch HS, Charlet A, Hoffmann L, Eliava M, Khrulev S, Cetin A, Osten P, Schwarz M, Seeburg P, Stoop R. et al. Evoked axonal oxytocin release in the central amygdala attenuates fear response. Neuron. 2012;73(3):553–566. doi:10.1016/j.neuron.2011.11.030.
- John S, Jaeggi AV. Oxytocin levels tend to be lower in autistic children: a meta-analysis of 31 studies. Autism. 2021;25(8):2152–2161. doi:10.1177/13623613211034375.
- Takayanagi Y, Yoshida M, Bielsky IF, Ross HE, Kawamata M, Onaka T, Yanagisawa T, Kimura T, Matzuk MM, Young LJ. et al. Pervasive social deficits, but normal parturition, in oxytocin receptor-deficient mice. Proc Natl Acad Sci USA. 2005;102(44):16096–16101. doi:10.1073/pnas.0505312102.
- Desbonnet L, Clarke G, Traplin A, O’Sullivan O, Crispie F, Moloney RD, Cotter PD, Dinan TG, Cryan JF. Gut microbiota depletion from early adolescence in mice: implications for brain and behaviour. Brain Behav Immun. 2015;48:165–173. doi:10.1016/j.bbi.2015.04.004.
- Johnson KVA & Burnet PWJ. Opposing effects of antibiotics and germ-free status on neuropeptide systems involved in social behaviour and pain regulation. BMC Neurosci. 2020;21(1):32. doi:10.1186/s12868-020-00583-3.
- Bertoni A, Schaller F, Tyzio R, Gaillard S, Santini F, Xolin M, Diabira D, Vaidyanathan R, Matarazzo V, Medina I. et al. Oxytocin administration in neonates shapes hippocampal circuitry and restores social behavior in a mouse model of autism. Mol Psychiatry. 2021;26(12):7582–7595. doi:10.1038/s41380-021-01227-6.
- Buffington SA, Di Prisco GV, Auchtung TA, Ajami NJ, Petrosino JF, Costa-Mattioli M. Microbial reconstitution reverses maternal diet-induced social and synaptic deficits in offspring. Cell. 2016;165(7):1762–1775. doi:10.1016/j.cell.2016.06.001.
- Harony-Nicolas H, Kay M, Hoffmann JD, Klein ME, Bozdagi-Gunal O, Riad M, Daskalakis NP, Sonar S, Castillo PE, Hof PR. et al. Oxytocin improves behavioral and electrophysiological deficits in a novel Shank3-deficient rat. Elife. 2017;6. doi:10.7554/eLife.18904.
- Abuaish S, Al-Otaibi NM, Abujamel TS, Alzahrani SA, Alotaibi SM, AlShawakir YA, Aabed K, El-Ansary A. Fecal transplant and Bifidobacterium treatments modulate gut Clostridium bacteria and rescue social impairment and hippocampal BDNF expression in a rodent model of autism. Brain Sci. 2021;11(8):1038. doi:10.3390/brainsci11081038.
- Ornoy A, Weinstein-Fudim L, Ergaz Z. Prenatal factors associated with autism spectrum disorder (ASD). Reprod Toxicol. 2015;56:155–169. doi:10.1016/j.reprotox.2015.05.007.
- Roullet FI, Lai JK, Foster JA. In utero exposure to valproic acid and autism–a current review of clinical and animal studies. Neurotoxicol Teratol. 2013;36:47–56. doi:10.1016/j.ntt.2013.01.004.
- Kalueff AV, Stewart AM, Song C, Berridge KC, Graybiel AM, Fentress JC. Neurobiology of rodent self-grooming and its value for translational neuroscience. Nat Rev Neurosci. 2016;17(1):45–59. doi:10.1038/nrn.2015.8.
- Leyfer OT, Folstein SE, Bacalman S, Davis NO, Dinh E, Morgan J, Tager-Flusberg H, Lainhart JE. Comorbid psychiatric disorders in children with autism: interview development and rates of disorders. J Autism Dev Disord. 2006;36(7):849–861. doi:10.1007/s10803-006-0123-0.
- Walf AA, Frye CA. The use of the elevated plus maze as an assay of anxiety-related behavior in rodents. Nat Protoc. 2007;2(2):322–328. doi:10.1038/nprot.2007.44.
- Meador KJ, Baker GA, Browning N, Clayton-Smith J, Combs-Cantrell DT, Cohen M, Kalayjian LA, Kanner A, Liporace JD, Pennell PB. et al. Cognitive function at 3 years of age after fetal exposure to antiepileptic drugs. N Engl J Med. 2009;360(16):1597–1605. doi:10.1056/NEJMoa0803531.
- Strzelczyk A, Schubert-Bast S. Psychobehavioural and cognitive adverse events of anti-seizure medications for the treatment of developmental and epileptic encephalopathies. CNS Drugs. 2022;36(10):1079–1111. doi:10.1007/s40263-022-00955-9.
- Kraeuter AK, Guest PC, Sarnyai Z. The Y-Maze for assessment of spatial working and reference memory in mice. Methods Mol Biol. 2019;1916:105–111. doi:10.1007/978-1-4939-8994-2_10.
- Cloarec R, Riffault B, Dufour A, Rabiei H, Gouty-Colomer L-A, Dumon C, Guimond D, Bonifazi P, Eftekhari S, Lozovaya N. et al. Pyramidal neuron growth and increased hippocampal volume during labor and birth in autism. Sci Adv. 2019;5(1):eaav0394. doi:10.1126/sciadv.aav0394.
- Molumby MJ, Anderson RM, Newbold DJ, Koblesky NK, Garrett AM, Schreiner D, Radley JJ, Weiner JA. γ-protocadherins interact with neuroligin-1 and negatively regulate dendritic spine morphogenesis. Cell Rep. 2017;18(11):2702–2714. doi:10.1016/j.celrep.2017.02.060.
- Reichova A, Bacova Z, Bukatova S, Kokavcova M, Meliskova V, Frimmel K, Ostatnikova D, Bakos J. Abnormal neuronal morphology and altered synaptic proteins are restored by oxytocin in autism-related SHANK3 deficient model. Mol Cell Endocrinol. 2020;518:110924. doi:10.1016/j.mce.2020.110924.
- Raymond GV, Bauman ML, Kemper TL. Hippocampus in autism: a golgi analysis. Acta Neuropathol. 1996;91(1):117–119. doi:10.1007/s004010050401.
- Sgobio C, Ghiglieri V, Costa C, Bagetta V, Siliquini S, Barone I, Di Filippo M, Gardoni F, Gundelfinger ED, Di Luca M. et al. Hippocampal synaptic plasticity, memory, and epilepsy: effects of long-term valproic acid treatment. Biol Psychiatry. 2010;67(6):567–574. doi:10.1016/j.biopsych.2009.11.008.
- Feng G, Mellor RH, Bernstein M, Keller-Peck C, Nguyen QT, Wallace M, Nerbonne JM, Lichtman JW, Sanes JR. Imaging neuronal subsets in transgenic mice expressing multiple spectral variants of GFP. Neuron. 2000;28(1):41–51. doi:10.1016/s0896-6273(00)00084-2.
- Bourne JN, Harris KM. Balancing structure and function at hippocampal dendritic spines. Annu Rev Neurosci. 2008;31(1):47–67. doi:10.1146/annurev.neuro.31.060407.125646.
- Qiao H, Li M-X, Xu C, Chen H-B, An S-C, Ma X-M. Dendritic spines in depression: what we learned from animal models. Neural Plast. 2016;2016:1–26. doi:10.1155/2016/8056370.
- Ohta KI, Suzuki S, Warita K, Kaji T, Kusaka T, Miki T. Prolonged maternal separation attenuates BDNF-ERK signaling correlated with spine formation in the hippocampus during early brain development. J Neurochem. 2017;141(2):179–194. doi:10.1111/jnc.13977.
- Aglah C, Gordon T, Posse de Chaves EI. cAMP promotes neurite outgrowth and extension through protein kinase a but independently of Erk activation in cultured rat motoneurons. Neuropharmacology. 2008;55(1):8–17. doi:10.1016/j.neuropharm.2008.04.005.
- Copf T. Developmental shaping of dendritic arbors in Drosophila relies on tightly regulated intra-neuronal activity of protein kinase a (PKA). Dev Biol. 2014;393(2):282–297. doi:10.1016/j.ydbio.2014.07.002.
- Okamoto K, Narayanan R, Lee SH, Murata K, Hayashi Y. The role of CaMKII as an F-actin-bundling protein crucial for maintenance of dendritic spine structure. Proc Natl Acad Sci USA. 2007;104(15):6418–6423. doi:10.1073/pnas.0701656104.
- Yasuda R, Hayashi Y, Hell JW. CaMKII: a central molecular organizer of synaptic plasticity, learning and memory. Nat Rev Neurosci. 2022;23(11):666–682. doi:10.1038/s41583-022-00624-2.
- Waltereit R, Weller M. Signaling from cAMP/PKA to MAPK and synaptic plasticity. Mol Neurobiol. 2003;27(1):99–106. doi:10.1385/MN:27:1:99.
- Sin WC, Haas K, Ruthazer ES, Cline HT. Dendrite growth increased by visual activity requires NMDA receptor and Rho GTPases. Nature. 2002;419(6906):475–480. doi:10.1038/nature00987.
- Varian BJ, Poutahidis T, DiBenedictis BT, Levkovich T, Ibrahim Y, Didyk E, Shikhman L, Cheung HK, Hardas A, Ricciardi CE. et al. Microbial lysate upregulates host oxytocin. Brain Behav Immun. 2017;61:36–49. doi:10.1016/j.bbi.2016.11.002.
- Lach G, Schellekens H, Dinan TG, Cryan JF. Anxiety, depression, and the microbiome: a role for gut peptides. Neurotherapeutics. 2018;15(1):36–59. doi:10.1007/s13311-017-0585-0.
- Feleke R, Jazayeri D, Abouzeid M, Powell KL, Srivastava PK, O’Brien TJ, Jones NC, Johnson MR. Integrative genomics reveals pathogenic mediator of valproate-induced neurodevelopmental disability. Brain. 2022;145(11):3832–3842. doi:10.1093/brain/awac296.
- Meng Q, Zhang W, Wang X, Jiao C, Xu S, Liu C, Tang B, Chen C. Human forebrain organoids reveal connections between valproic acid exposure and autism risk. Transl Psychiatry. 2022;12(1):130. doi:10.1038/s41398-022-01898-x.
- Jiang Y, Travagli RA. Hypothalamic–vagal oxytocinergic neurocircuitry modulates gastric emptying and motility following stress. J Physiol. 2020;598(21):4941–4955. doi:10.1113/JP280023.
- Sabatier N, Leng G, Menzies J. Oxytocin, feeding, and satiety. Front Endocrinol (Lausanne). 2013;4:35. doi:10.3389/fendo.2013.00035.
- Lee MR, Sheskier MB, Farokhnia M, Feng N, Marenco S, Lipska BK, Leggio L. Oxytocin receptor mRNA expression in dorsolateral prefrontal cortex in major psychiatric disorders: a human post-mortem study. Psychoneuroendocrinology. 2018;96:143–147. doi:10.1016/j.psyneuen.2018.05.039.
- Lee HJ, Caldwell HK, Macbeth AH, Young WS 3rd. Behavioural studies using temporal and spatial inactivation of the oxytocin receptor. Prog Brain Res. 2008;170:73–77. doi:10.1016/S0079-6123(08)00407-X.
- Wemheuer F, Taylor JA, Daniel R, Johnston E, Meinicke P, Thomas T, Wemheuer B. Tax4Fun2: prediction of habitat-specific functional profiles and functional redundancy based on 16S rRNA gene sequences. Environ Microbiome. 2020;15(1):11. doi:10.1186/s40793-020-00358-7.
- van de Wouw M, Boehme M, Lyte JM, Wiley N, Strain C, O’Sullivan O, Clarke G, Stanton C, Dinan TG, Cryan JF. et al. Short-chain fatty acids: microbial metabolites that alleviate stress-induced brain–gut axis alterations. J Physiol. 2018;596(20):4923–4944. doi:10.1113/JP276431.
- Koh A, De Vadder F, Kovatcheva-Datchary P, Backhed F. From dietary fiber to host physiology: short-chain fatty acids as key bacterial metabolites. Cell. 2016;165(6):1332–1345. doi:10.1016/j.cell.2016.05.041.
- Sharon G, Sampson TR, Geschwind DH, Mazmanian SK. The central nervous system and the gut microbiome. Cell. 2016;167(4):915–932. doi:10.1016/j.cell.2016.10.027.
- Snigdha S, Ha K, Tsai P, Dinan TG, Bartos JD, Shahid M. Probiotics: potential novel therapeutics for microbiota-gut-brain axis dysfunction across gender and lifespan. Pharmacology & Therapeutics. 2022;231:107978. doi:10.1016/j.pharmthera.2021.107978.
- Cheng LH, Liu YW, Wu CC, Wang S, Tsai YC. Psychobiotics in mental health, neurodegenerative and neurodevelopmental disorders. J Food Drug Anal. 2019;27(3):632–648. doi:10.1016/j.jfda.2019.01.002.
- Kong XJ, Liu J, Liu K, Koh M, Sherman H, Liu S, Tian R, Sukijthamapan P, Wang J, Fong M. et al. Probiotic and oxytocin combination therapy in patients with autism spectrum disorder: a randomized, double-blinded, placebo-controlled pilot trial. Nutrients. 2021;13(5):1552. doi:10.3390/nu13051552.
- Meng X, Gao Y, Qi H, Ding Y, Sun Y. Clinical application value of Lactobacillus plantarum PS128 in patients with anxiety disorders. Clin Psychopharmacol Neurosci. 2022;20(3):560–566. doi:10.9758/cpn.2022.20.3.560.
- Hitti FL, Siegelbaum SA. The hippocampal CA2 region is essential for social memory. Nature. 2014;508(7494):88–92. doi:10.1038/nature13028.
- Kelly E, Meng F, Fujita H, Morgado F, Kazemi Y, Rice LC, Ren C, Escamilla CO, Gibson JM, Sajadi S. et al. Regulation of autism-relevant behaviors by cerebellar–prefrontal cortical circuits. Nat Neurosci. 2020;23(9):1102–1110. doi:10.1038/s41593-020-0665-z.
- Fortier AV, Meisner OC, Nair AR, Chang SWC. Prefrontal circuits guiding social preference: implications in autism spectrum disorder. Neurosci Biobehav Rev. 2022;141:104803. doi:10.1016/j.neubiorev.2022.104803.
- Shioda N, Yabuki Y, Wang Y, Uchigashima M, Hikida T, Sasaoka T, Mori H, Watanabe M, Sasahara M, Fukunaga K. et al. Endocytosis following dopamine D2 receptor activation is critical for neuronal activity and dendritic spine formation via Rabex-5/PDGFRβ signaling in striatopallidal medium spiny neurons. Mol Psychiatry. 2017;22(8):1205–1222. doi:10.1038/mp.2016.200.
- Ciani L, Boyle KA, Dickins E, Sahores M, Anane D, Lopes DM, Gibb AJ, Salinas PC. Wnt7a signaling promotes dendritic spine growth and synaptic strength through Ca 2+ /Calmodulin-dependent protein kinase II. Proc Natl Acad Sci USA. 2011;108(26):10732–10737. doi:10.1073/pnas.1018132108.
- Chen CM, Orefice LL, Chiu S-L, LeGates TA, Hattar S, Huganir RL, Zhao H, Xu B, Kuruvilla R. Wnt5a is essential for hippocampal dendritic maintenance and spatial learning and memory in adult mice. Proc Natl Acad Sci USA. 2017;114(4):E619–E628. doi:10.1073/pnas.1615792114.
- Bard L, Groc L. Glutamate receptor dynamics and protein interaction: lessons from the NMDA receptor. Mol Cell Neurosci. 2011;48(4):298–307. doi:10.1016/j.mcn.2011.05.009.
- Ju W, Morishita W, Tsui J, Gaietta G, Deerinck TJ, Adams SR, Garner CC, Tsien RY, Ellisman MH, Malenka RC. et al. Activity-dependent regulation of dendritic synthesis and trafficking of AMPA receptors. Nat Neurosci. 2004;7(3):244–253. doi:10.1038/nn1189.
- Hwang JY, Monday HR, Yan J, Gompers A, Buxbaum AR, Sawicka KJ, Singer RH, Castillo PE, Zukin RS. CPEB3-dependent increase in GluA2 subunits impairs excitatory transmission onto inhibitory interneurons in a mouse model of fragile X. Cell Rep. 2022;39(10):110853. doi:10.1016/j.celrep.2022.110853.
- Grooms SY, Noh K-M, Regis R, Bassell GJ, Bryan MK, Carroll RC, Zukin RS. Activity bidirectionally regulates AMPA receptor mRNA abundance in dendrites of hippocampal neurons. J Neurosci. 2006;26(32):8339–8351. doi:10.1523/JNEUROSCI.0472-06.2006.
- Rajgor D, Welle TM, Smith KR. The coordination of local translation, membranous organelle trafficking, and synaptic plasticity in neurons. Front Cell Dev Biol. 2021;9:711446. doi:10.3389/fcell.2021.711446.
- Jurek B, Neumann ID. The oxytocin receptor: from intracellular signaling to behavior. Physiol Rev. 2018;98(3):1805–1908. doi:10.1152/physrev.00031.2017.
- Pobbe RL, Pearson BL, Defensor EB, Bolivar VJ, Young WS, Lee H-J, Blanchard DC, Blanchard RJ. Oxytocin receptor knockout mice display deficits in the expression of autism-related behaviors. Horm Behav. 2012;61(3):436–444. doi:10.1016/j.yhbeh.2011.10.010.
- Pobbe RL, Pearson BL, Blanchard DC, Blanchard RJ. Oxytocin receptor and Mecp2 308/Y knockout mice exhibit altered expression of autism-related social behaviors. Physiol Behav. 2012;107(5):641–648. doi:10.1016/j.physbeh.2012.02.024.
- Sala M, Braida D, Lentini D, Busnelli M, Bulgheroni E, Capurro V, Finardi A, Donzelli A, Pattini L, Rubino T. et al. Pharmacologic rescue of impaired cognitive flexibility, social deficits, increased aggression, and seizure susceptibility in oxytocin receptor null mice: a neurobehavioral model of autism. Biol Psychiatry. 2011;69(9):875–882. doi:10.1016/j.biopsych.2010.12.022.
- Andreo-Martinez P, Rubio-Aparicio M, Sanchez-Meca J, Veas A, Martinez-Gonzalez AE. A meta-analysis of gut microbiota in children with autism. J Autism Dev Disord. 2022;52(3):1374–1387. doi:10.1007/s10803-021-05002-y.
- Allen AP, Hutch W, Borre YE, Kennedy PJ, Temko A, Boylan G, Murphy E, Cryan JF, Dinan TG, Clarke G. et al. Bifidobacterium longum 1714 as a translational psychobiotic: modulation of stress, electrophysiology and neurocognition in healthy volunteers. Transl Psychiatry. 2016;6(11):e939. doi:10.1038/tp.2016.191.
- Zhang L, Liu C, Jiang Q, Yin Y. Butyrate in energy metabolism: there is still more to learn. Trends Endocrinol Metab. 2021;32(3):159–169. doi:10.1016/j.tem.2020.12.003.
- Chao SH, Wu RJ, Watanabe K, Tsai YC. Diversity of lactic acid bacteria in suan-tsai and fu-tsai, traditional fermented mustard products of Taiwan. Int J Food Microbiol. 2009;135(3):203–210. doi:10.1016/j.ijfoodmicro.2009.07.032.
- Nicolini C, Fahnestock M. The valproic acid-induced rodent model of autism. Exp Neurol. 2018;299:217–227. doi:10.1016/j.expneurol.2017.04.017.
- Rein B, Ma K, Yan Z. A standardized social preference protocol for measuring social deficits in mouse models of autism. Nat Protoc. 2020;15(10):3464–3477. doi:10.1038/s41596-020-0382-9.
- Nakayama J, Watanabe K, Jiang J, Matsuda K, Chao S-H, Haryono P, La-Ongkham O, Sarwoko M-A, Sujaya IN, Zhao L. et al. Diversity in gut bacterial community of school-age children in Asia. Sci Rep. 2015;5(1):8397. doi:10.1038/srep08397.
- Callahan BJ, McMurdie PJ, Rosen MJ, Han AW, Johnson AJA, Holmes SP. DADA2: High-resolution sample inference from illumina amplicon data. Nat Methods. 2016;13(7):581–583. doi:10.1038/nmeth.3869.
- Quast C, Pruesse E, Yilmaz P, Gerken J, Schweer T, Yarza P, Peplies J, Glöckner FO. The SILVA ribosomal RNA gene database project: improved data processing and web-based tools. Nucleic Acids Res. 2013;41(D1):D590–596. doi:10.1093/nar/gks1219.
- McMurdie PJ, Holmes S, Watson M. phyloseq: an R package for reproducible interactive analysis and graphics of microbiome census data. PLOS ONE. 2013;8(4):e61217. doi:10.1371/journal.pone.0061217.
- Dray S, Dufour AB. The ade4 package: Implementing the duality diagram for ecologists. J Stat Softw. 2007;22(4):1–20. doi:10.18637/jss.v022.i04.