ABSTRACT
The intestinal microbiota, consisting of an estimated 10^10–10^11 organisms, regulate physiological processes involved in digestion, metabolism, and immunity. Surprisingly, these intestinal microorganisms have been found to influence tissues that are not directly in contact with the gut, such as adipose tissue, the liver, skeletal muscle, and the brain. This interaction takes place even when intestinal barrier function is uncompromised. An increasing body of evidence suggests that bacterial membrane vesicles (bMVs), in addition to bacterial metabolites such as short-chain fatty acids, are able to mediate effects of the microbiota on these host tissues. The ability of bMVs to dissipate from the intestinal lumen into systemic circulation hereby facilitates the transport and presentation of bacterial components and metabolites to host organs. Importantly, there are indications that the interaction between bMVs and tissues or immune cells may play a role in the etiology of (chronic metabolic) disease. For example, the gut-derived bMV-mediated induction of insulin resistance in skeletal muscle cells and pro-inflammatory signaling by adipocytes possibly underlies diseases such as type 2 diabetes and obesity. Here, we review the current knowledge on bMVs in the microbiota’s effects on host energy/substrate metabolism with a focus on etiological roles in the onset and progression of metabolic disease. We furthermore illustrate that vesicle production by bacterial microbiota could potentially be modulated through lifestyle intervention to improve host metabolism.
Introduction
One of the greatest health challenges of the 21st century is the increase in occurrence of obesity and obesity-related metabolic disorders including metabolic syndrome (MetS) and type 2 diabetes (T2D).Citation1 As of 2019, more than 2 billion people globally are overweight (Body Mass Index (BMI) ≥ 25 kg/m2), and approximately 800 million of these are obese (BMI ≥ 30 kg/m2).Citation2 In MetS and obesity, whole-body energy and substrate metabolism is altered, thereby increasing the risk of cardiovascular disease, diabetes, cancer, and all-cause mortality.Citation3 Obesity is often associated with insulin resistance and low-grade inflammation of insulin-sensitive tissues including adipose tissue (AT), skeletal muscle, and the liver.Citation4,Citation5 A concomitant lipid overflow into the circulation and consequently, lipid storage in non-ATs contributes to the onset and progression of insulin resistance and aberrant glucose metabolism in skeletal muscle and the liver.Citation6
Importantly, the onset and progression of obesity and MetS has often been found concerted with gut microbial dysbiosis, in which the composition and/or functioning of the microbes inhabiting the gastrointestinal tract is imbalanced.Citation7,Citation8 Functionalities of the gut microbiota include modulation of the gut barrier integrity and digestion of indigestible fibers, thereby allowing energy harvest from otherwise indigestible food sources.Citation9 Bacterial metabolites herein produced, such as short-chain fatty acids (SCFAs), have been found to regulate host energy and substrate metabolism and are a focal point in research investigating microbiome–host interactions.Citation9 Bacterial membrane vesicles (bMVs) are increasingly recognized as important players in microbiome-host interactions, in addition to SCFA’s and other gut-derived metabolites such as bile acids, branched-chain amino acids, tryptophan, and indole derivatives.Citation10,Citation11 These bMVs are defined as non-reproducing, lipid-bilayer delimited particles released from bacterial cell membranes that can be generated by both Gram-negative and Gram-positive bacteria.Citation12 Characterization studies have found that bMVs are thermostable and readily contain bacterial nucleic acids, metabolites, proteins, and endotoxins.Citation10–13–Citation16 They typically range between 20 and 400 nm in diameterCitation12 and, conceivably, are able to pass the intestinal barrier in healthCitation17 and diseaseCitation18 conditions. In contrast to secreted bacterial proteins and nucleic acids that are not encapsulated, vesiculated bacterial factors are protected from proteolytic degradation and nuclease activity.Citation13–15 The systemic dissemination and concentrated delivery of these bacterial factors to host cells is facilitated by molecules on the vesicle membrane that interact with the host cell membrane and its receptors, to enable delivery of bMV cargo.Citation19–22 In the context of MetS and obesity, researchers have reported an interaction between gut-derived bMVs and insulin sensitive tissues (such as AT, the liver, and skeletal muscle) in rodent studies, with consequential changes in signaling from adipocytes to liver and skeletal muscle ().Citation23–25
Figure 1. A central role for bacterial membrane vesicles in glucose metabolism and metabolic disease. Illustrating reported effects of gut-derived bMVs on adipose tissue (AT), skeletal muscle tissue and the liver in metabolic disease, with a secondary focus on the consequential signalling effects of AT on skeletal muscle cells and the liver. In mice, gut-derived Pseudomonas panacis bMVs decreased glucose uptake in AT and skeletal muscleCitation23 whereas the translocation of Porphyromonas gingivalis bMVs to the liver reduced glycogen synthesis in response to insulin signalling.Citation25 Interestingly, oral administration of pasteurized Akkermansia municiphila and its bMVs decreased HFD induced measures of inflammation (Interleukin 6 (IL6), Tumor Necrosis Factor alpha (TNFα)) in AT and the liver in mice.Citation24 As master regulator of insulin and glucose homeostasis, AT removes FFAs and TAGs from systemic circulation, thereby preventing lipotoxicity induced insulin resistance in liver and skeletal muscle tissue.Citation6 Via endocrine signalling AT delivers cytokines and adipokines to skeletal muscle tissue and the liver, decreasing insulin sensitivity and fatty acid oxidation in the first, and increasing gluconeogenesis in the latter.Citation88 For the sake of simplicity, the effects of the interaction between the microbiome, its vesicle produce and the cells lining the intestinal barrier on metabolic health, as well as the role of tissue-infiltrating immune cells and crosstalk between skeletal muscle cells, liver and other organs are not illustrated here. TAG: triacylglycerol. FFA: free fatty acids.
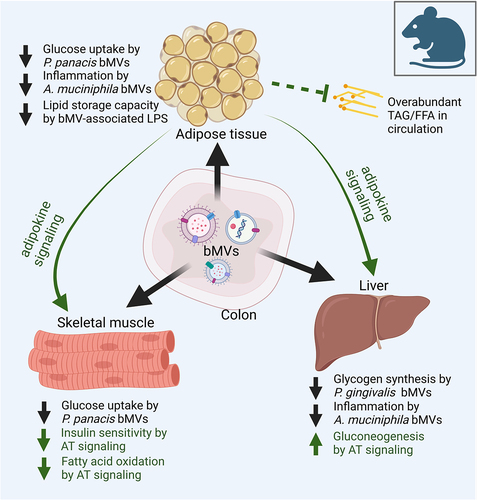
In this review, we will focus on the available knowledge regarding the nature and functionalities of these gut-derived bMVs. We provide an overview of bMV biogenesis, and on mechanisms through which gut bMVs could affect host substrate metabolism in AT, skeletal muscle and the liver. We furthermore highlight potential relations between microbial dysbiosis and metabolic disease, mediated by differences in bMV repertoire (content and functionality) that might arise in overweight/obesity and related metabolic diseases.
Biogenesis of bMVs in the intestinal lumen
Depending on isolation and counting methods used, 10^10 bMVs can easily be purified from their bacterial producer strains per gram of human fecal matter.Citation11,Citation26 The formation of bMVs was first observed to occur through blebbing of the outer membrane of Gram-negative bacteria, typically as a result of cell wall recycling and exposure to stress conditions.Citation27,Citation28 In addition to these specific bMVs termed outer membrane vesicles (OMVs), Gram-negative bacteria are known to produce outer–inner membrane vesicles and explosive outer-membrane vesicles whose formations are observed during bacterial cell lysis.Citation12 Vesicle biogenesis in Gram-positive bacteria, lacking an outer membrane, is less well understood, but some of the mechanisms describing the formation of bMVs herein involve stress-induced fluidity of the plasma membrane and subsequent membrane protrusion/constriction through the peptidoglycan layer.Citation29 This process as well as the lysis of Gram-positive bacteria gives rise to inner-membrane or cytoplasmic membrane vesicles.Citation12 Lastly, formation of bMVs is also described for Gram-neutral Mycobacteria.Citation30 This evolutionary conservation of bacterial vesiculation is justified by the various roles and functionalities attributed to bMVs, mitigating interactions between microbesCitation10,Citation16 and between microbe and host.Citation31 It is however important to note that different types of bacteria have various types of partially overlapping vesiculation processes in response to environmental stimuli, and that the classification of bacterial vesicle types is subject to change as new vesicle types and vesiculation strategies are discovered.Citation12 A deeper fundamental categorization of bMV nomenclature is described elsewhere.Citation10
Importantly, the factors that could influence bacterial vesicle production and characteristics in the human gut are overlapping those that might influence the bacterial producer strains themselves, and include tropism by bacteriophages,Citation32,Citation33 nutrient availability,Citation23,Citation34,Citation35 exercise,Citation36,Citation37 medication use,Citation38,Citation39 and oxidative and/or chemical stressCitation34 (). However, to date, the potential of altering the gut-derived bMV repertoire to improve human (metabolic) health has not been explored in human clinical interventions. Notably, specific targeting of colonic vesicle production could be attained through deprivation of certain nutrients, given that such measures rapidly induce elaborate stress responses resulting in a change in bacterial vesicle production and characteristics. For example, different bacterial growth stages have been found to produce different vesicle repertoires, as Gram-negative Bacteroides thetaiotaomicron produces lipoprotein loaded OMVs at early stages and (lytic) cytoplasmic vesicles at later growth stages.Citation40 Authors postulate that such differential vesicle production could be the result of B. thetaiotaomicron adapting to nutrient availabilities, and suggest that production of lytic cytoplasmic vesicles is not trivial but a tightly controlled process involved long term bacterial survival.Citation12,Citation41 Other examples include the deprivation of iron causing Vibrio cholerae to downregulate phospholipid turnover, resulting in increased formation of bMVs in the human host,Citation42 as well as cysteine starvation inducing oxidative stress in Neisseria meningitidis, yielding increased bMV production in vitro.Citation43 In addition to nutrient availability, the exposure to antibiotics has also been found to influence rates of vesicle formation. In Gram-positive bacteria such as Staphylococcus aureus,Citation38 the exposure to β-lactam antibiotics decreases the integrity of the bacterial peptidoglycan layer leading to increased vesiculation in vitro. For Gram-negative bacteria such as Pseudomonas aeruginosa, the exposure to the aminoglycoside antibiotic gentamicin leads to vesiculation by disruption of the cell membrane by interacting with phospholipid moieties.Citation39
Figure 2. Determinants of bacterial vesiculation and of vesicle characteristics in the large intestine.
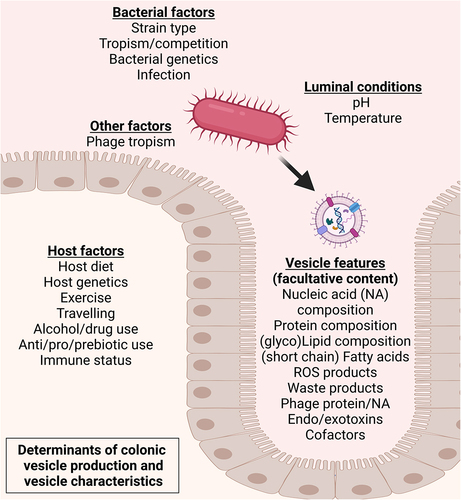
Given this dynamic nature of vesicle production by the intestinal microbiome, both diet and lifestyle can also impact the nature of bMV (production) by affecting the microbiota themselves. For example, an increased availability of dietary fibers favors the growth of genera such as Lactobacilli or Bifidobacteria.Citation44,Citation45 Simultaneously, this increased availability of dietary fibers has been found to decrease abundancies of colonic mucus-degrading bacteriaCitation46 and Clostridium species.Citation45 A decrease in abundance for a certain taxon could occur through competition and selective pressure, both sources of stress. Given that bMV production is described as part of normal bacterial biology as well as a stress response, this suggests that for a given species a decrease in abundance could occur simultaneously with an increase in vesicle production by that species. Such examples illustrate why it is warranted to investigate vesicle repertoires alongside their bacterial producer strains. Additionally, physical exercise has also been found to be a determinant of microbial composition, with most human intervention studies reporting an exercise-associated bloom of acetate and butyrate producing bacteria such as Bifidobacterium,Citation36 and an increase of benign species such as Akkermansia muciniphila.Citation37 Again, the effects of diet and lifestyle factors on colonic bacterial vesiculation, and consequential bMV mediated effects on human metabolic health need clarification.
Significant health benefits could be attained when bMV production and properties in the intestine are modulated for health improvement. By for example manipulating agitation rate and lowering pH in in vitro cultures of Lactobacillus casei and Lactobacillus plantarum, immunomodulatory properties of bMVs released by these bacteria changed as measured by their potential in inducing Interleukin 10 (IL-10) and Tumor Necrosis Factor alpha (TNFα) release by Tohoku Hospital Pediatrics-1 (THP-1) macrophages.Citation47 As such, nutritional intervention strategies might benefit from stimulating bacteria to produce bMVs with more benign immunomodulatory properties, for example through inducing a decrease in colonic pH as reported to occur through microbial fermentation.Citation48,Citation49 Herein, consumption of indigestible fermentable fibers could be considered as interventional strategy in modulating vesicle production, as well as stimulating SCFA production (extensively reviewed elsewhereCitation9). In porcine stool samples a bloom in bMVs as secreted by Clostridiales, Bacilli and Enterobacteriales was observed as a result of dietary administration with the complex fiber β-mannan.Citation35 For mice it has previously been found that feeding with a high-fat diet (HFD) results in increased numbers of bMVs in systemic circulation through a decrease in specialized macrophages that clear these vesicles.Citation50 Others report an intestinal bloom of Proteobacteria-produced bMVs and concomitant increase in bMV associated lipopolysaccharide (LPS) as a result of a HFD in mice.Citation23 Also in mice, a high-protein diet (HPD) was found to significantly increase microbiota vesicle production altogether, when compared to HFD or high-carbohydrate diets.Citation34 Such examples illustrate how the intestinal production of bMVs is subject to environmental cues that could be exploited in dietary or lifestyle interventions. Most of such current research however focuses on the effects that specific diets have on the microbiota themselves, with far less focus on bMV biology. A further understanding of molecular pathways governing vesicle formation is crucial, particularly regarding the determinants of their immunomodulatory capabilities and impact on host energy metabolism. Elucidating these determinants holds promise for shaping interventional approaches aimed at modulating bioactive vesicle production in the human host.
Notably, vesicle production rates can vary greatly between bacteria, with a recent study using standard liquid monocultures indicating a bMV/CFU (colony forming unit) count of over 10^5 for Helicobacter pylori, and approximately 50 for Pseudomonas aeruginosa over the course of 16 h.Citation51 A bMV repertoire as produced by the microbiota does therefore not represent the composition and species’ abundancies of the bacterial producer strains with high fidelity, as was recently demonstrated.Citation11,Citation52
Herein it is imperative to understand that bMVs are indispensable in microbe-to-microbe interactions, either by for example assisting other microbes in nutrient acquisition, or in the form of mitigating tropism/competition with other bacteria.Citation10,Citation53 Exemplifying, these vesicles could facilitate microbial growth through transport of vitamins, cofactors or proteins,Citation54 or through encapsulation of hydrolases that facilitate degradation of carbohydrates to be used as nutrients.Citation55 Alternatively, microbes have been shown to utilize bMVs for offensive purposes in microbe–microbe communication as well, as bMVs from Myxococcus species have for example been found to contain various factors with antimicrobial activityCitation56 or some Escherichia coli strains produce bMVs that enclose peptidoglycan-degrading enzymes.Citation19 Effects on host metabolism could then be a consequence of bMV mediated delivery of bioactive enzymes to host tissues, leading to localized degradation of various substrates. In addition, microbes affecting each other’s growth through secretion of bMVs could influence the abundance of various bacterial taxa. Effects on host metabolism could then be a consequence of a change in composition of the microbiome itself.Citation7,Citation8 Nevertheless, bMVs are also a pivotal player in directed interkingdom communication between microbes and the host, and have been found to be well tailored to their role as mediators.Citation10,Citation28,Citation31 Exemplifying is the observation that bMVs are equipped with specific adhesion molecules that facilitate the physical interaction with host cells,Citation19 and they exhibit various functionalities to escape and modulate the host’s immune system (). Effects on (human) host metabolic health as a result of delivery of bacterial factors through bMVs require further exploration however, to identify cues for modulation of ‘beneficial’ bMV production.
Table 1. Overview of in vivo and in vitro studies investigating involvement of gut-derived bMvs in translocation, (host) inflammation, and/or effects on intestinal barrier function, with potential implications on host metabolic health.
Summarizing, research focusing on the health consequences of microbial dysbiosis, in which the metagenomic composition of the microbiome (i.e. relative abundance of gut-residing taxa) is determined in relation to participant characteristics, fails to take into account an aspect of microbial activity and functionality. Given that gut-luminal bMV production reflects a degree of the microbiome’s activity and functionalities, we argue that the studying of these vesicles allows for an increased understanding of (eventual) disease mechanisms. The physiological relevance of studying the astronomical numbers of bMVs in the intestinal lumen is further warranted given that their cargo and membrane associated biomolecules are derived from the bacterial producer under specific conditions, conferring biological, physical, and a plethora of immunological properties.Citation10 Again, bMVs can contain bacterial DNA,Citation13 (functional) RNA,Citation14,Citation67 proteins,Citation15 and various cytosolic contents including enzymes, toxins, and bacterial waste products,Citation16 yet herein it is imperative to understand that not all bMVs contain (producer-strain specific) biologicals ().
Crossing the intestinal barrier
It is noted that various tissues including blood,Citation68–71 AT,Citation50,Citation70,Citation72 the liver,Citation73 and skeletal muscleCitation74 from otherwise healthy human individuals contain bacterial DNA. One group recently reported the occurrence of live bacteria in AT of subjects with T2D and/or obesity using fluorescent in-situ hybridization techniques.Citation70 Considering the isolation and detection of bacterial DNA from biological samples, commonly used nucleic acid isolation and purification methods employ chaotropic lysis reagents to release DNA and RNA from complex sample mixtures.Citation50,Citation68–72 Such procedures do not allow distinguishing between previously vesiculated DNA, DNA within intact bacteria and “loose” free-floating DNA. In such mentioned studies, the detection of extraintestinal bacterial DNA could potentially be attributed to vesicle translocation from the intestine either via transcellular migration or through paracellular leakage of colonic contents (). This in vivo translocation of bacterial vesicles from the intestinal lumen to distant host organs was clearly demonstrated in a seminal mouse study, employing mice with a fluorescent reporter background colonized with Cre-recombinase expressing E. coli.Citation66 The delivery of bacterially expressed recombinase enzyme to host tissues was herein mediated by bMVs. Delivery of the enzyme to host cells resulted in excision of a stop-cassette upstream of the reporter gene, yielding induced expression in at least the brain, liver, heart, kidney and spleen.Citation66 Importantly, considerable knowledge gaps exist in the understanding of gut-borne bMVs, spanning from their point of origin to their mediation of effects on host tissues. A concise overview of this interkingdom communication (encompassing bMV production, translocation, distribution, host-interactions, and elimination) and persisting knowledge gaps has been summarized previously.Citation31
Figure 3. Contextual setting in which the gut microbiota yield a diverse vesicle repertoire interacting with bacterial producer strains and the gut epithelium. Vesicles exiting the intestinal lumen is reported to occur through transcellular migration and through (vesicle induced) disruption of junction proteins regulating intestinal barrier function.Citation10 Vesicle-associated bacterial proteases such as B. fragilis fragolysinsCitation83,Citation84 or possibly P. gingivalis gingipainsCitation85 have been reported able to disrupt barrier function leading to increased leakage of colonic contents. In the intestinal submucosa the (increased) presence with bMVs could induce polarization of extraintestinal immune cellsCitation64 whereas translocation to vasculature facilitates transport of bMVs to distal metabolically relevant tissues and organs.
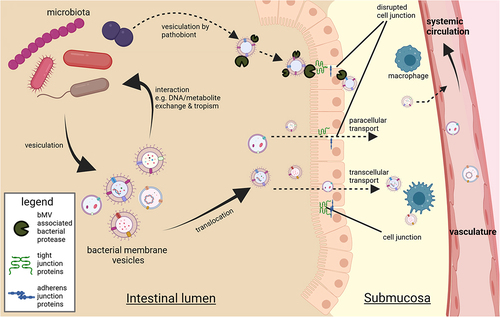
In human studies, much research on this topic focuses on extraintestinal bacterial DNA or ‘signatures’ rather than bMVs. Interventional studies on microbiota and metabolic health report for example the presence of bacterial DNA in muscle biopsies and blood of healthy male participants, prior to and following 6 weeks of endurance training.Citation74 The authors report a differential abundance of DNA from certain taxa in muscle tissue, with a bloom in for example Staphylococcus and Acetinobacter signatures post exercise, and suggest underlying changes in gut barrier permeability are involved. Arguably the detection of bacterial DNA in host tissues outside the gastrointestinal tract could involve bMVs, as circulating cell-free DNA (cfDNA) is subject to nuclease activity in blood plasma.Citation75 Herein, it is noted that for microbial cfDNA a biological half-life of 16 min to 2 h has previously been reported.Citation76 Encompassment within a bMV would shield this DNA from nuclease degradation in circulation. A plausible explanation for an increased extraintestinal presence of bacterial signatures such as cfDNA and bMVs is the compromised intestinal barrier function as observed in a number of conditions including obesity,Citation77 inflammatory bowel disease (IBD),Citation78 HIV,Citation79 and older age.Citation80 Microbial dysbiosis is typically concomitant with this intestinal barrier dysfunction, in which paracellular transport of luminal components to systemic circulation is strongly increased.Citation72 Indeed, increased amounts of bMV associated LPS were detected in plasma samples of IBD and HIV patients when compared to healthy controls, together with increased systemic concentrations of zonulin; a protein believed to be involved in tight-junction maintenance.Citation81 Additionally, alcohol consumption has been found to increase the translocation of intestinal and bacterial components such as LPS, from an otherwise healthy human gut into to systemic circulation.Citation82 Disease mechanisms and immunological responses in host tissues because of such bMV translocation, enabling transport of bacterial components and metabolites to systemic circulation and peripheral tissues, have been identified in a number of in vivo murine and in vitro studies discussed in this review (, ).
bMVs themselves have also been found able to directly influence intestinal barrier function; the interaction between a Caco-2 cell model for the intestinal epithelium and E. coli bMVs indicated vesicle uptake by Caco-2 cells resulting in a change in inflammatory signaling in these epithelial cells.Citation61 The bMV-associated metalloproteinase fragolysin (a.k.a. Bacteroides fragilis toxin or BFT), a prominent virulence factor produced by B. fragilis, can disrupt the murine intestinal barrier in vivo through cleavage of E-cadherins that regulate adherence between enterocytesCitation83,Citation84 (). Interestingly, fragolysin causes epithelial cell contact disruption exclusively when associated to a vesicle membrane.Citation21 Similar to this, the presence of intestinal Porphyromonas gingivalis in mice has been found to exacerbate gastrointestinal inflammation by disruption of the intestinal barrier function, which is believed to be the result of gingipain (a bacterial cysteine protease) mediated degradation of tight-junction proteins.Citation85 Although this work did not focus on the contributing role of secreted bMVs in this pathogenesis, considerable bMV production by P. gingivalisCitation86 and vesicle association of gingipain proteinCitation87 are reported in literature, delineating a potentially clinically relevant mechanism through which bMVs could affect the gut barrier function and host health. Where earlier paradigms considered the presence of bMVs in extraintestinal tissues solely as the consequence of a compromised intestinal barrier, more recent findings indicate that bMVs can also pass through an uncompromised intestinal barrier to readily reach peripheral organs such as the liver, lungs, and heart of healthy C57BL/6 mice.Citation17 Consolidation of epithelial cell junctions by bMVs has also been reported, with vesicles produced by E. coli strains Nissle 1917 (EcN) and commensal ECOR63 reported to upregulate expression of tight-junction proteins in vitro.Citation62 The same group described how these ‘benign’ bMVs can counteract intestinal barrier dysfunction caused by enteropathogenic E. coli (EPEC).Citation63 Similar benign effects are described for A. muciniphila bMVs, able to counteract increases in Caco-2 monolayer permeability as a result of exposure to LPS, whilst in this study no effects where observed for E. coli bMVs.Citation57 In mice, the commensal B. fragilis has been reported to produce bMVs associated with polysaccharide A, and these vesicles stimulated anti-inflammatory cytokine production in the intestinal lumen.Citation58 Additionally, immunomodulatory molecules secreted through B. fragilis bMVs protected against experimentally induced colitis in a similar mouse model.Citation59 The favorable properties of these vesicles were mitigated through ATG16L1 and NOD2 signaling in autophagy by host’s immune cells, and authors illustrate how knockout of ATG16L1 abolishes the OMV’s protective effects against colitis. This exemplifies how the interaction between bMVs and specific host genes can contribute to intestinal barrier regulation. Others report B. fragilis bMVs to be involved in maintaining gut homeostasis by encapsulating enzymes facilitating fiber breakdown, promoting digestion and microbial energy harvest from complex carbohydrates.Citation55 In contrast, the aforementioned fragolysin-associated B. fragilis bMVs are reported as harmful.Citation21 This illustrates that vesicles produced by a given species could have benign or pathogenic effects on the host, depending on context and associated PAMP repertoire (). In studying bioactivity of bMVs on host cells, it is noted that the enclosed or associated factors of these bMVs are not always reported or investigated in great detail (). Arguably, a more holistic approach to assessing bMVs involves consideration of their entire antigenic repertoire. For instance, researchers focusing on bMV associated LPS in E. coli report immunomodulatory effects for one strain and pro-inflammatory effects for another.Citation61 Detection or quantification of bMV-associated LPS alone herein does not capture the essence of the bioactive effects of bMVs. A more comprehensive understanding of vesicle immunogenicity therefore requires an understanding of all relevant associated molecules, either as presented on the vesicle surface such as receptors or LPS, or as enclosed factors that might be delivered into host cells. In addition to such intrinsic bMV factors, the host microenvironment in which these vesicles interact with host cells also plays a pivotal role, as (circulating) immune cells exhibit heightened sensitivity to pathogen-associated molecular pattern (PAMP) cues compared to other somatic cell types.Citation50,Citation88
Regarding biodistribution studies of bMVs, in which gut-derived bMVs are detected in extraintestinal niches, most researchers still rely on animal models. Notably, the bMVs’ distribution and ability to cross barriers is for example a focal point in research investigating the gut-brain axis. Oral administration of bMVs from Paenalcaligenes hominis to murine models for Alzheimer’s disease (AD) resulted in a significant increase in detected bacterial 16S ribosomal DNA in brain tissues, concomitant with local inflammation, and cognitive decline.Citation89 In the context of metabolic disease, Choi et al. reported orally administered vesicles from Pseudomonas panacis to induce insulin resistance and impaired glucose metabolism in mice.Citation23
In humans, postmortem analysis of brain tissue of AD patients and controls readily indicated the presence of DNA originating from Actinobacteria, Firmicutes, Proteobacteria and Bacteroides.Citation90 However once more the use of chaotropic lysis reagents used in this work, to release nucleic acids from tissue samples by dissolving DNA-enveloping structures, disenables the detection of bMVs. In such research it is noted that bacterial DNA could have been contained within whole bacteria, phagocytosing immune cells, protein (aggregates), or bMVs. Generally, authors however do not elaborate on the exact nature of the biological envelope of this DNA, shielding it from nuclease degradation, but postulate that an aging-related decrease in the integrity of endothelial barriers could contribute to translocation of bacteria and their products.Citation90,Citation91 A process involving translocation of gut-derived bMVs through the blood–brain barrier might herein also help explain the detected presence of bacterial LPS and E. coli pili proteins in the older healthy human brain.Citation92 Interestingly, a diverse gut-derived bMV repertoire was recently characterized in human breast milk,Citation93 suggesting that bMVs could potentially translocate from the gut to the mammary glands. Ongoing advances in the development of sensitive bMV separation and detection methods using complex matrices (e.g. blood, milk, or liquor) are herein essential to understand the impact of circulating gut-borne bMVs.Citation31 To date, however, clinical human evidence linking translocation of gut-derived bMVs (as opposed to bacterial DNA or ‘signatures’) to the etiology of (metabolic) disease, is lacking.
bMVs and insulin sensitive tissues
bMVs in AT
The consequences of gut-derived bMVs homing to metabolic tissues, particularly AT, have been studied in obese mouse models.Citation50,Citation94 In these models, the translocation of gut-derived bMVs is associated with AT inflammation and tissue-specific insulin resistance.Citation50 This finding was substantiated by incubation of human microbiome derived bMVs with human and mice AT and liver cells in vitro.Citation50 The effects of bMV-induced inflammation were exacerbated in CRIg+ (specialized macrophages clearing bMVs from circulation) knockout mice and diminished upon depletion of bMV DNA,Citation50 suggesting that the delivery of bacterial DNA is at least in part responsible for bMV-induced inflammatory effects on the hostCitation95 (). In obese mouse models, the CRIg+ macrophage population has been found significantly reduced, resulting in a strong increase of bMV translocation to AT.Citation50 The subsequent enrichment of gut-derived bMVs in AT, as detected by a marked increase in bacterial DNA, is accompanied by local inflammation and aggravated insulin resistance.Citation50 In human subjects, however, a clinically significant influence of bMV capture by CRIg+ macrophages, on parameters such as AT inflammation has not been demonstrated yet. Another important notion is that the mesenteric adipose tissue (mAT) surrounding the large and small intestine is increasingly recognized in acting as a second intestinal barrier, through sequestration of bacterial signatures originating from the intestinal lumen.Citation96,Citation97 In line with this idea is that in mice the removal of mAT resulted in hepatic inflammation and reduced overall glucose tolerance concomitant with an increase in bacterial load (DNA) in the liver,Citation98 suggesting a causal link between translocation of intestinal contents and metabolic disease.
Figure 4. Interactions between bMVs and metabolic tissues. Reported consequences implicated in metabolic disease mostly involve proinflammatory signalling in AT, the liver and skeletal muscle. Mechanisms through which bMVs mediate their biological effects are via activation of pathogen recognition receptors (PPR) such as Toll-like receptors TLR4Citation100 & TLR2Citation101 and cyclic GMP-AMP Synthase (cGAS).Citation95 In addition, bMV associated proteases can dysregulate glucose homeostasis through inhibition of insulin signalling in the liver,Citation25 via mechanisms not fully understood. Upregulated expression of pro-inflammatory genes following PRR activation occurs through translocation of nuclear factor kappa-light-chain-enhancer of activated B cells (NF-kB) to the cell nucleus.Citation107 Proinflammatory signalling in AT is concomitant to a decreased AT lipid storage capacity. A subsequent lipid overflow to non-AT tissues acts in concert with proinflammatory endocrine AT signalling to the liver and skeletal muscle.Citation6
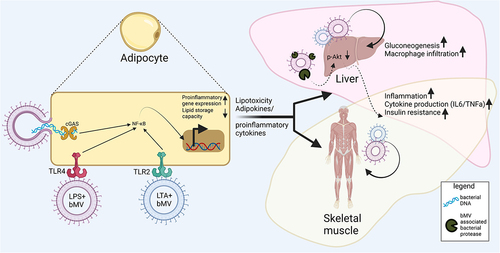
In humans, more recent work on visceral AT depots sampled during bariatric surgery of 40 patients with severe obesity (average BMI 50.5 kg/m2), a considerable load of bacterial DNA (average >10.000 copies of bacterial 16S rRNA/mg of tissue) was detected in omental, mesenteric, and subcutaneous AT whilst not in plasma (average <100 copies of bacterial 16S rRNA/mL).Citation99 The authors herein also investigated these extraintestinal bacterial signatures in T2D and found striking differences in bacterial signatures in mAT dictated by glucose sensitivity, independent of BMI. Interestingly, when comparing all sampledATs the highest average number of operational taxonomical units was detected in mAT, further illustrating its role in sequestration of bacterial signatures surpassing the ‘first’ intestinal barrier.Citation99 Such work provides evidence for selective and tissue specific microbial signatures in obesity, potentially identifying new therapeutic targets and/or biomarkers in metabolic disease. The presence of bMVs (as envelope of this bacterial DNA) was however not investigated in this work of Anhê et al.,Citation99 and given the low concentrations of bacterial DNA in bMVsCitation11 it is important to take along stringent and elaborate controls to recognize detection of contaminating bacterial DNA from the environment, resultant from laborious sample collection and processing.Citation72
Thus far, adipocyte dysfunction as a result of gut-derived bMV exposure has been largely explained through vesicle mediated inflammation by transport of TLR4Citation100 and TLR2Citation101 ligands and bacterial DNACitation50 (). Subcutaneous administration of peptidoglycan, a prominent TLR2 ligand as found on Gram-positive bacteria as well as their vesicles,Citation10 increased serum triglyceride concentrations in miceCitation102 which was concomitant with macrophage infiltration and inflammation of AT.Citation103 Similarly, for the Gram-positive endotoxin lipotechoic acid (LTA), also present on bMVs,Citation12 a dose-dependent increase in serum triglycerides and carbohydrate oxidation was observed following intraperitoneal administration in rats.Citation104 AT infiltration by macrophages could herein also be the result of bMV-induced immune signaling through cGAS-STING in these macrophages, where the exposure of these macrophages to vesicle-enclosed bacterial DNA is responsible for pro-inflammatory responses.Citation95 In human plasma, the presence of bMV-associated LPS was found upregulated in IBD patients, HIV patients, and people undergoing radiation- or chemotherapy, and this upregulation was found to be concomitant with intestinal barrier dysfunction.Citation81 Human peripheral blood mononuclear cell activation by such bMV-associated LPS was also reported, although the authors did however not focus on eventual immune infiltration of AT in this setting.Citation81 In the context of host energy and substrate metabolism, there is currently still a need for studies investigating what the consequences of association of a microbiome-derived bMV with specific PAMPs are.
Nevertheless, for both Gram-positive and Gram-negative vesicles, many described pathophysiological effects on host cells are likely mediated through recognition of bMV-associated PAMPs. Binding of PAMPs to a host receptor herein results in translocation of pro-inflammatory transcription factors to the host cell nucleus to yield a pro-inflammatory response (). Properties of bMVs can therefore be characterized by means of analyzing their associated and enclosed PAMPs. Although not all aforementioned studies that investigate PAMP signaling focus on bMV association, it is emphasized that co-administration of antigens with bMVs could boost stability and immunogenicity of an antigen.Citation22 Investigations into specific homing of gut-derived bMVs to certain target tissues in vivo, dictated by the PAMP repertoire of these vesicles, are currently lacking but could facilitate improved understanding of these microbiome-host interactions.
Considering MetS, in which related features of diabetes and obesity co-occur, AT plays a central role in the interplay between metabolic organs. Considering the often observed enrichment of gut-derived bMVs and other bacterial signatures in dysfunctional and inflamed AT,Citation50,Citation70,Citation99 we postulate that these bMVs merit attention in studying organs with dysfunctional energy/substrate metabolism in metabolic disease (). For example, white adipocytes are largely responsible for the removal of triacylglycerol from systemic circulation, sequestering them for energy storage, and allowing release of this energy through the process of lipolysis in which free fatty acids are released.Citation105 When (white) adipocytes’ lipid storage capacity is exceeded or compromised as a result of disease, lifestyle, genetics,r inflammation (e.g. by gut-derived bMVs and/or subsequent macrophage infiltration), excess lipids will enter systemic circulation and accumulate in non-ATs.Citation88 This ectopic fat accumulation in other insulin sensitive tissues such as skeletal muscle and the liver, can induce lipotoxicity, a condition in which excess lipids and accumulated bioactive lipid metabolites in non-AT impair endogenous cellular functions, resulting in impaired insulin signaling in skeletal muscle and the liver.Citation88 The development of insulin resistance is a main precursor for T2D, and commonly observed in obesity.Citation5 Yet, the nuances affecting the interplay between various tissues in the pathogenesis of insulin resistance, are still under investigation. Mounting evidence suggests that he (gut-)microbe-host interactions play a critical role.Citation4 We postulate that bMVs are a potentially critical player in the etiology of metabolic disease, given their production by the microbiome and ability to induce localized inflammation and changes in substrate metabolism in the most relevant metabolic tissues. This is further exemplified by the observation that intestinal barrier (dys)function itself influences the crosstalk between AT, skeletal muscle and liver cells in obesity and T2D.Citation106 In metabolic endotoxemia, defined as a condition in which two to threefold increased levels of LPS enter the systemic circulation due to compromised intestinal barrier integrity, increased levels of pro-inflammatory cytokines (e.g. IL6 and TNFα) are detected in visceral and subcutaneous AT, muscle, and liver tissues.Citation106 Again, a compromised intestinal barrier has also been linked to the presence of bMV-associated LPS in systemic circulation.Citation81 This illustrates the urgent need for human investigations looking into bMV infiltration of these tissues in metabolic disease. Nevertheless, in the previously discussed workCitation106 no emphasis was placed on the potential role that gut-derived bMVs could have played in the intestinal barrier dysfunction, or the subsequent local production of pro-inflammatory cytokines by metabolic tissues.
To summarize, translocation of bMVs from intestinal lumen to AT would be concomitant to translocation of non-bMV bacterial products such as LPS, and involved in the onset and progression of metabolic disease as host tissues can exhibit inflammatory responses in the presence of these biologicals. This is evidenced by the observation of in vivo sequestration of bacterial signatures in various AT depots and systemic circulation. The specific consequences of this sequestration might be tissue specific and most likely determined by the concentration and nature of these biologicals (bMV type, bMV with or without PAMPs, PAMP type, etc.), and secondarily through resultant interorgan crosstalk complicating the study of direct mechanistic effects. However, in vivo studies with a primary focus on the effects of gut-derived bMVs on human AT function are necessary to fully understand the role of these vesicles in the pathophysiology of metabolic disease.
bMVs in the liver and skeletal muscle tissue
There is a lack of human studies examining the consequences of translocation of various bMVs and vesicle-associated PAMPs from the intestinal lumen, on metabolic tissues like the liver and skeletal muscle. It is noted that the nature and amount of such vesicle-associated PAMPs are strong determinants of the effects these bMVs would have on host tissue function. For example, bacterial peptidoglycan and LTA by themselves are potent activators of innate immune receptorsCitation107 and subcutaneous administration of peptidoglycan has been found to induce liver fibrosis and inflammation in mice.Citation102 Upon intraperitoneal LTA administration in rats, elevated levels of serum triglycerides have been detected as a result of increased hepatic secretion.Citation104 Although peptidoglycan, LTA and LPS can all be detected on gut-derived bMVs,Citation10 such work is often performed with non-bMV associated PAMPs. Hence, given that bMV-association could boost immunomodulatory potential of such antigens,Citation22 we advocate that investigations looking into the role of vesicles in these host-microbe interactions are urgently needed.
Exemplifying PAMP-bMV functionality; the aforementioned P. gingivalis associated proteases such as gingipains, can exploit the vesicle’s ability to readily translocate into systemic circulation, and their translocation to the liver has been found to induce local insulin resistance in mice, as demonstrated by a decrease in protein kinase B (Akt) phosphorylationCitation25 (). This example illustrates how a single bacterial protease has tissue-specific pathophysiological consequences as a result of being vesicle-associated. Nevertheless, not many such examples are known given the heterogeneity of gut bacteria and their metabolites. With respect to a species-specific bMV responsible for a disease phenotype, an elegant study conducted by Choi et al. identified P. panacis bMVs from a heterogeneous stool bMV population as able to inhibit insulin signaling in murine skeletal muscle cells as well as in AT.Citation23 The authors further report the presence of P. panacis bMVs, but not bacteria, in skeletal muscle tissue 12-h post oral administration. Strikingly, this infiltration was concomitant to typical diabetic phenotypes as signified by glucose intolerance after glucose administration or insulin injection, suggesting the homing of a gut-derived bMV to host organs might hold much clinical relevance in the context of metabolic disease.Citation23
It is complicated, however, to discern direct effects of specific bMVs on specific-host tissues from the effects mediated through pro-inflammatory signaling through immune cells, cytokines or adipokines. In the study of bMVs in vivo, often involving oralCitation23–25,Citation58,Citation60,Citation66 administration of bMVs, the main metabolic tissues such as the liver, skeletal muscle, and AT are affected simultaneously. Shi et al. for example studied the effects of orally administered E. coli Nissle 1917 bMVs on microbiome composition and liver metabolism in mice.Citation60 It was found that two weeks of treatment with these bMVs modulated microbiome composition and reduced body weight in obese and T2D mice, and authors suggest this might be related to increased SCFA concentrations measured in the livers of bMV treated mice.Citation60 The underlying molecular mechanisms however remain unknown, and no focus was put on biodistribution of these E. coli bMVs to the liver or other metabolically relevant tissues. Studying tissue homing of bMVs as performed by Bittel et al.Citation66 would herein further the understanding of effects that these vesicles might have on host tissues, either through or in concert with SCFAs. Nevertheless, studying direct effects of bMVs on the liver, skeletal muscle, and other tissues might benefit from an in vitro setup that does not take into account confounding signaling between AT, liver and skeletal muscle.
In humans, previous work on a cohort of liver cirrhosis patients highlighted that disease severity, defined in terms of intrahepatic endothelial dysfunction, exhibited a positive correlation with the presence of gut-derived bacterial DNA in plasma.Citation108 Again, however, here no distinction was made between vesiculated and ‘free’ DNA, suggesting that perhaps the translocation of bacterial DNA here is in the form of bMV translocation concomitant with various PAMPs. As such, effects of bMVs on skeletal muscle and liver could readily occur through inflammation mediated by adipokine signaling () or by macrophage polarization,Citation11 resulting in tissue infiltration and insulin resistance.Citation50
Evidence for direct mechanistic effects of bMVs on host substrate metabolism other than through inflammation is scarce, but recent work on A. muciniphila and its derived bMVs indicated that these vesicles might directly increase transcription of peroxisome proliferator-activated receptor (PPAR) genes in HepG-2 (hepatocyte-derived) and Caco-2 cells.Citation65 PPAR genes are members of a family of nuclear receptors best known for their involvement in host glucose and lipid metabolism.Citation109 The reported dose dependent effects in increasing expression of PPAR genes at the transcriptional level in Caco-2 and Hep-G2 cells, suggests that A. muciniphila bMVs could aid in prevention of obesity and metabolic disease.Citation65 The authors furthermore note that A. muciniphila readily produces SCFAs which are also known to activate expression of PPARα (promoting fatty acid oxidation), and indicate that the bacteria itself, either heat-inactivated and in active form, as well as cell-free bacterial supernatant, can induce increased expression of these genes.Citation65 However, as no focus was placed on the effect of treatment on expression of (anti-)inflammatory genes, it is hard to discern if observed results on PPAR genes are due to direct signaling through bMV enveloped PPAR ligands or (in part) by a change in the cell’s inflammatory state by bMV PAMPs. In addition it would be interesting to investigate whether the SCFA uptake by host cells is promoted by bMVs, for example through SCFA encapsulation and subsequent delivery through membrane fusion. The use of lab-grown bacterial (mono)cultures in the study could herein however have yielded bMVs and metabolites different in nature or concentration than those found in vivo. Nonetheless, increased hepatic PPAR expression might protect against liver disease in obesity and T2D,Citation110 but current findings on A. muciniphila bMVs remain to be confirmed in vivo and on expression level.
Summarizing, there currently is no good understanding of how gut-derived bMV signaling affects substrate metabolism in skeletal muscle and the liver in humans, and what the implications of such signaling on whole body energy metabolism would be in the context of obesity and T2D. However, the association of gut-derived bMVs with PAMPs or vesicle encapsulation of bacterial metabolites could mitigate significant effects on host metabolism following translocation to these tissues.
Therapeutic modulation of bMV production in vivo
Vesicle production by the intestinal microbiome is subject to external cues, and as such this yields possibilities for therapeutic modulation to steer the microbiota vesicle production toward producing bMVs with benign properties. Various factors could influence bacterial vesicle production and characteristics in the human gut (), and of particular relevance in the context of obesity and T2D are modulation through lifestyle interventions. In humans, nutritional interventionCitation4 as well as exerciseCitation36 have been found to affect bacterial composition, but studies focusing on vesicle production and therapeutic modulation hereof are currently lacking. In vitro characterization of factors and conditions that affect vesiculation might provide essential insights in ways to modulate microbiome bMV production in vivo. Exemplifying; dietary fiber supplementation could promote microbial fermentation to induce a decrease in colonic pH, and changes in overall microbial compositions.Citation48,Citation49 In vitro, such a decrease in ambient pH is reported to induce LPS modifications in Salmonella enterica, followed by the release of these modified LPS through vesiculation.Citation111 Studying examples of such modulation of bMV production by specific gut microbes is complex however, given the heterogeneity and dynamic nature of the microbiome. The effects of physical exercise interventions on gut-derived bMV repertoire are currently unexplored, but exercise-associated blooms of specific taxa such as Bifidobacterium have previously been reported in humans.Citation36,Citation37
Animal studies have provided some insights in effects of nutritional supplementation on colonic bMV production; complex fiber has been found to increase bMV production by certain taxa (Clostridiales, Bacilli, and Enterobacteriales) in pigs.Citation35 In mice, HFD increased overall bMV production by ProteobacteriaCitation23 whilst simultaneously decreasing systemic bMV clearance by macrophages.Citation50 Also in mice, a HPD increased microbiota vesicle production altogether.Citation34 It is hereby noted that in the characterization of vesicles by means of identifying their bacterial producer strains (e.g. by sequencing bacterial DNA/RNA), differences at the lowest taxonomical level could still be relevant. Exemplifying; within species even strain differences can be determinants of vesicle properties, as different Staphylococcus aureus strains cultured under identical conditions produce vesicles that vary in the amount to peptidoglycan they carry.Citation107 In line with this is that in vitro characterized bMVs derived from E. coli and B. fragilis have been reported as benign by some and pathogenic by others ().
Investigating possibilities for lifestyle-modulation of gut-derived bMVs repertoire would be of much interest to researchers studying microbiome–host interactions, yet clinical human evidence for amelioration of host metabolism mediated through gut-derived bMVs is currently lacking.
Concluding remarks
Addressing the global obesity pandemic with its associated conditions such as T2D requires a holistic understanding of the disease pathophysiology. It is now understood that the gut microbiome plays a critical role in maintaining (metabolic) health, given that various diseases can be exacerbated by – or be the result of microbial dysbiosis. In addition to microbiome derived SCFA’s, bMVs have gained attention because of their rigid nature in combination with the ability to transport bacterial molecules to host organs. Previous work on functional bMV in-vivo mouse studies employed administration of purified vesicles originating from a donorCitation23,Citation89 or from monoculture,Citation17,Citation24,Citation25,Citation66 indicating context-specific effects on host inflammatory status and/or glucose sensitivity. In vitro work readily allows studying of tissue-specific molecular mechanisms of bMV signaling (), but lacks a degree of complexity that is the result of signaling between the prime insulin sensitive organs AT, liver and skeletal muscle. Additionally, both bMV administration in vivoCitation17,Citation23,Citation24,Citation25,Citation66,Citation89 as well as incubation in vitroCitation11,Citation21,Citation57,Citation58,Citation61,Citation62,Citation63,Citation64,Citation65 might employ localized doses of bMVs that would probably not occur under normal physiological conditions. Investigating extraintestinal gut-derived bMV repertoires under normal physiological conditions is difficult however, notably by experimental complications in purifying and characterizing bMVs from these tissues. For example, sensitive techniques employing PCR-based amplification of bacterial nucleic acids can specifically detect small amounts of vesicle-enclosed DNA, but it is possible that bMVs carrying antigens do not contain genetic material. As such it is paramount to take along stringent controls when sampling host tissues,Citation72 since contaminating environmental bacteria contain much more DNA and PAMPs than vesicles alone.
Particularly in human studies, the effects of extraintestinal gut-derived bMVs on host metabolism need much further elucidation, but an understanding of this intricate aspect of microbiota-host interaction could pave the way for therapeutic modulation of the microbiota and their vesicle production to ameliorate metabolic health. We argue that gut-bacterial signatures detected outside of the gastrointestinal tract in healthy human subjects could well be the result of bMV translocation, whilst numerous examples already illustrate how microbiota-derived vesicles could exert benign and pathogenic effects on the host. The fact that gut-derived bMV repertoires can be subject to external cues dictating aspects such as physiochemical properties and cargo, explains how they can constitute significant additional complexity in microbiome-host interaction,Citation26,Citation52 adding to the complexity of the bacterial communities that produced them. A better perspective on metabolic health thus requires an understanding of the distinction between the effects of intestinal microbes, microbiota-derived metabolites, and bMVs on host metabolism. Except for a few defined molecular mechanisms however, ways which in which gut residing bacterial species, genera, or co-abundance groups can contribute to metabolic disease via bMVs in vivo are still largely unknown.
In conclusion, we hypothesize that the role of bMVs in microbiota–host communication is much more prominent than previously thought. Even though there is currently only limited clinical human evidence for gut-derived bMVs as drivers of metabolic disease, exploring their impact on host tissues (notably through pro-inflammatory signaling) holds great promise for expanding our comprehension of the microbiome-host interplay. As such, the characterization of these vesicles, their biogenesis, and their properties could pave the way for novel therapeutic interventions in the context of obesity and related co-morbidities such as T2D.
Author contributions
JV wrote the manuscript; JWEJ, EEB, PS and FRS, reviewed and edited the manuscript. All authors approved the final version of the manuscript.
Key messages
What is already known about this subject?
The interkingdom communication between gut bacteria and their human host is mediated by bacterial products (including e.g. gut-derived hormones and metabolites), able to exit the intestinal lumen to reach systemic circulation. The production of such bacteria derived molecules can be modulated by nutrition and lifestyle.
bMVs are nanosized membrane-delimited particles produced in large amounts by most bacteria, containing bacterial cell constituents, metabolites, and toxins alike.
What are new findings in this field and how could they impact future research and clinical practice?
Rigid, thermostable, and bioactive bMVs can be isolated from fecal matter to shed light on what the bacteria are producing. Future research on the nature and functionalities of these vesicles could help explain metabolic disease phenotypes.
The bacterial composition of the gut-microbiome is generally different than the bacteria-derived vesicle composition, suggesting characterization of baseline microbial composition does only partly explain metabolic disease phenotype.
A holistic understanding of microbiome-host interactions taking into account all players paves the way for more personalized interventions to treat and/or prevent metabolic disease.
Acknowledgments
BioRender.com is acknowledged as tool for creating figures used in this article.
Disclosure statement
No potential conflict of interest was reported by the author(s).
Additional information
Funding
References
- Engin A. The definition and prevalence of obesity and metabolic syndrome. Obesity Lipotoxicity. Adv Exp Med Biol. 2017;960:1–22. doi:10.1007/978-3-319-48382-5_1.
- Caballero B. Humans against obesity: who will win? Advances in nutrition. Adv Nutr. 2019;10(suppl_1):S4–S9. doi:10.1093/advances/nmy055.
- Solomon CG, Manson JE. Obesity and mortality: a review of the epidemiologic data. Am J Clin Nutr. 1997;66(4):1044S–1050S. doi:10.1093/ajcn/66.4.1044S.
- Cotillard A, Kennedy SP, Kong LC, Prifti E, Pons N, Le Chatelier E, Almeida M, Quinquis B, Levenez F, Galleron N. et al. Dietary intervention impact on gut microbial gene richness. Nature. 2013;500(7464):585–588. doi:10.1038/nature12480.
- Boucher J, Kleinridders A, Kahn CR. Insulin receptor signaling in normal and insulin-resistant states. Cold Spring Harb Perspect Biol. 2014;6(1):a009191. doi:10.1101/cshperspect.a009191.
- Boden G. Obesity and free fatty acids. Endocrinol Metab Clin North Am. 2008;37(3):635–646. doi:10.1016/j.ecl.2008.06.007.
- Cuevas-Sierra A, Ramos-Lopez O, Riezu-Boj JI, Milagro FI, Martinez JA. Diet, gut microbiota, and obesity: links with host genetics and epigenetics and potential applications. Adv Nutr. 2019;10(suppl_1):S17–S30. doi:10.1093/advances/nmy078.
- Sen T, Cawthon CR, Ihde BT, Hajnal A, DiLorenzo PM, Claire B, Czaja K. Diet-driven microbiota dysbiosis is associated with vagal remodeling and obesity. Physiology & Behavior. 2017;173:305–317. doi:10.1016/j.physbeh.2017.02.027.
- Canfora EE, Jocken JW, Blaak EE. Short-chain fatty acids in control of body weight and insulin sensitivity. Nat Rev Endocrinol. 2015;11(10):577–591. doi:10.1038/nrendo.2015.128.
- Díaz‐Garrido N, Badia J, Baldomà L. Microbiota‐derived extracellular vesicles in interkingdom communication in the gut. J Extracell Vesicles. 2021;10(13):e12161. doi:10.1002/jev2.12161.
- Kameli N, Borman R, López-Iglesias C, Savelkoul P, Stassen FR. Characterization of feces-derived bacterial membrane vesicles and the impact of their origin on the inflammatory response. Front Cell Infect Microbiol. 2021;11:667987. doi:10.3389/fcimb.2021.667987.
- Toyofuku M, Nomura N, Eberl L. Types and origins of bacterial membrane vesicles. Nat Rev Microbiol. 2019;17(1):13–24. doi:10.1038/s41579-018-0112-2.
- Bitto NJ, Chapman R, Pidot S, Costin A, Lo C, Choi J, D’Cruze T, Reynolds EC, Dashper SG, Turnbull L. et al. Bacterial membrane vesicles transport their DNA cargo into host cells. Sci Rep. 2017;7(1):1–11. doi:10.1038/s41598-017-07288-4.
- Dauros-Singorenko P, Blenkiron C, Phillips A, Swift S. The functional RNA cargo of bacterial membrane vesicles. FEMS Microbiol Lett. 2018;365(5):fny023. doi:10.1093/femsle/fny023.
- Haurat MF, Aduse-Opoku J, Rangarajan M, Dorobantu L, Gray MR, Curtis MA, Feldman MF. Selective sorting of cargo proteins into bacterial membrane vesicles. Journal Of Biological Chemistry. 2011;286(2):1269–1276. doi:10.1074/jbc.M110.185744.
- Toyofuku M, Schild S, Kaparakis-Liaskos M, Eberl L. Composition and functions of bacterial membrane vesicles. Nat Rev Microbiol. 2023;21(7):1–16. doi:10.1038/s41579-023-00875-5.
- Jones EJ, Booth C, Fonseca S, Parker A, Cross K, Miquel-Clopés A, Hautefort I, Mayer U, Wileman T, Stentz R. et al. The uptake, trafficking, and biodistribution of Bacteroides thetaiotaomicron generated outer membrane vesicles. Front Microbiol. 2020;11:57. doi:10.3389/fmicb.2020.00057.
- Park J-Y, Choi J, Lee Y, Lee J-E, Lee E-H, Kwon H-J, Yang J, Jeong B-R, Kim Y-K, Han P-L. et al. Metagenome analysis of bodily microbiota in a mouse model of Alzheimer disease using bacteria-derived membrane vesicles in blood. Exp Neurobiol. 2017;26(6):369. doi:10.5607/en.2017.26.6.369.
- Lee EY, Bang JY, Park GW, Choi DS, Kang JS, Kim HJ, Park K-S, Lee J-O, Kim Y-K, Kwon K-H. et al. Global proteomic profiling of native outer membrane vesicles derived from Escherichia coli. Proteomics. 2007;7(17):3143–3153. doi:10.1002/pmic.200700196.
- O’donoghue EJ, Krachler AM. Mechanisms of outer membrane vesicle entry into host cells. Cell Microbiol. 2016;18(11):1508–1517. doi:10.1111/cmi.12655.
- Zakharzhevskaya NB, Tsvetkov VB, Vanyushkina AA, Varizhuk AM, Rakitina DV, Podgorsky VV, Vishnyakov IE, Kharlampieva DD, Manuvera VA, Lisitsyn FV. et al. Interaction of Bacteroides fragilis toxin with outer membrane vesicles reveals new mechanism of its secretion and delivery. Front Cell Infect Microbiol. 2017;7:2. doi:10.3389/fcimb.2017.00002.
- Bitto NJ, Kaparakis-Liaskos M. The therapeutic benefit of bacterial membrane vesicles. Int J Mol Sci. 2017;18(6):1287. doi:10.3390/ijms18061287.
- Choi Y, Kwon Y, Kim D-K, Jeon J, Jang SC, Wang T, Ban M, Kim M-H, Jeon SG, Kim M-S. et al. Gut microbe-derived extracellular vesicles induce insulin resistance, thereby impairing glucose metabolism in skeletal muscle. Sci Rep. 2015;5(1):1–11. doi:10.1038/srep15878.
- Ashrafian F, Keshavarz Azizi Raftar S, Lari A, Shahryari A, Abdollahiyan S, Moradi HR, Masoumi M, Davari M, Khatami S, Omrani MD. et al. Extracellular vesicles and pasteurized cells derived from Akkermansia muciniphila protect against high-fat induced obesity in mice. Microb Cell Fact. 2021;20(1):1–17. doi:10.1186/s12934-021-01709-w.
- Seyama M, Yoshida K, Yoshida K, Fujiwara N, Ono K, Eguchi T, Kawai H, Guo J, Weng Y, Haoze Y. et al. Outer membrane vesicles of Porphyromonas gingivalis attenuate insulin sensitivity by delivering gingipains to the liver. Biochim Biophys Acta - Mol Basis Dis. 2020;1866(6):165731. doi:10.1016/j.bbadis.2020.165731.
- Kameli N, Becker HE, Welbers T, Jonkers DM, Penders J, Savelkoul P, Stassen FR. Metagenomic profiling of fecal-derived bacterial membrane vesicles in crohn’s disease patients. Cells. 2021;10(10):2795. doi:10.3390/cells10102795.
- Deatherage BL, Lara JC, Bergsbaken T, Barrett SLR, Lara S, Cookson BT. Biogenesis of bacterial membrane vesicles. Mol Microbiol. 2009;72(6):1395–1407. doi:10.1111/j.1365-2958.2009.06731.x.
- Volgers C, Savelkoul PH, Stassen FR. Gram-negative bacterial membrane vesicle release in response to the host-environment: different threats, same trick? Crit Rev Microbiol. 2018;44(3):258–273. doi:10.1080/1040841X.2017.1353949.
- Schlatterer K, Beck C, Hanzelmann D, Lebtig M, Fehrenbacher B, Schaller M, Ebner P, Nega M, Otto M, Kretschmer D. et al. The mechanism behind bacterial lipoprotein release: phenol-soluble modulins mediate Toll-like receptor 2 activation via extracellular vesicle release from Staphylococcus aureus. MBio. 2018;9(6):e01851–18. doi:10.1128/mBio.01851-18.
- Palacios A, Gupta S, Rodriguez GM, Prados-Rosales R. Extracellular vesicles in the context of Mycobacterium tuberculosis infection. Mol Immunol. 2021;133:175–181. doi:10.1016/j.molimm.2021.02.010.
- Hendrix A, De Wever O. Systemically circulating bacterial extracellular vesicles: Origin, fate, and function. Trends Microbiol. 2022;30(3):213–216. doi:10.1016/j.tim.2021.12.012.
- Yasuda M, Yamamoto T, Nagakubo T, Morinaga K, Obana N, Nomura N, Toyofuku M. Phage genes induce quorum sensing signal release through membrane vesicle formation. Microb Environ. 2022;37(1):ME21067. doi:10.1264/jsme2.ME21067.
- Mandal PK, Ballerin G, Nolan LM, Petty NK, Whitchurch CB. Bacteriophage infection of Escherichia coli leads to the formation of membrane vesicles via both explosive cell lysis and membrane blebbing. Microbiology. 2021;167(4). doi:10.1099/mic.0.001021.
- Tan J, Ni D, Taitz J, Pinget GV, Read M, Senior A, Wali JA, Elnour R, Shanahan E, Wu H. et al. Dietary protein increases T-cell-independent sIgA production through changes in gut microbiota-derived extracellular vesicles. Nat Commun. 2022;13(1):4336. doi:10.1038/s41467-022-31761-y.
- Lagos L, Leanti La Rosa SØ, Arntzen M, Ånestad R, Terrapon N, Gaby JC, Westereng B. Isolation and characterization of extracellular vesicles secreted in vitro by porcine microbiota. Microorganisms. 2020;8(7):983. doi:10.3390/microorganisms8070983.
- Erlandson KM, Liu J, Johnson R, Dillon S, Jankowski CM, Kroehl M, Robertson CE, Frank DN, Tuncil Y, Higgins J. et al. An exercise intervention alters stool microbiota and metabolites among older, sedentary adults. Ther Adv Infect Dis. 2021;8:20499361211027067. doi:10.1177/20499361211027067.
- Zhong X, Powell C, Phillips CM, Millar S, Carson BP, Dowd KP, Perry IJ, Kearney PM, Harrington JM, O’Toole PW. et al. The influence of different physical activity behaviours on the gut microbiota of older Irish adults. J Nutr Health Aging. 2021;25(7):854–861. doi:10.1007/s12603-021-1630-6.
- Andreoni F, Toyofuku M, Menzi C, Kalawong R, Mairpady Shambat S, François P, Zinkernagel AS, Eberl L. Antibiotics stimulate formation of vesicles in staphylococcus aureus in both phage-dependent and -independent fashions and via different routes. Antimicrob Agents Chemother. 2019;63(2):e01439–18. doi:10.1128/AAC.01439-18.
- Kadurugamuwa J, Beveridge T. Natural release of virulence factors in membrane vesicles by Pseudomonas aeruginosa and the effect of aminoglycoside antibiotics on their release. J Antimicrob Chemother. 1997;40(5):615–621. doi:10.1093/jac/40.5.615.
- Juodeikis R, Martins C, Saalbach G, Richardson J, Koev T, Baker DJ, Defernez M, Warren M, Carding SR. Differential temporal release and lipoprotein loading in B. thetaiotaomicron bacterial extracellular vesicles. J Of Extracellular Vesicle. 2024;13(1):12406. doi:10.1002/jev2.12406.
- Turnbull L, Toyofuku M, Hynen AL, Kurosawa M, Pessi G, Petty NK, Osvath SR, Cárcamo-Oyarce G, Gloag ES, Shimoni R. et al. Explosive cell lysis as a mechanism for the biogenesis of bacterial membrane vesicles and biofilms. Nat Commun. 2016;7(1):11220. doi:10.1038/ncomms11220.
- Zingl FG, Kohl P, Cakar F, Leitner DR, Mitterer F, Bonnington KE, Rechberger GN, Kuehn MJ, Guan Z, Reidl J. et al. Outer membrane vesiculation facilitates surface exchange and in vivo adaptation of Vibrio cholerae. Cell Host Microbe. 2020;27(2):225–37. e8. doi:10.1016/j.chom.2019.12.002.
- van de Waterbeemd B, Zomer G, van den IJssel J, van Keulen L, Eppink MH, van der Ley P, van der Pol LA. Cysteine depletion causes oxidative stress and triggers outer membrane vesicle release by Neisseria meningitidis; implications for vaccine development. PLOS ONE. 2013;8(1):e54314. doi:10.1371/journal.pone.0054314.
- Marotti I, Bregola V, Aloisio I, Di Gioia D, Bosi S, Di Silvestro R, Quinn R, Dinelli G. Prebiotic effect of soluble fibres from modern and old durum‐type wheat varieties on Lactobacillus and Bifidobacterium strains. J Sci Food Agric. 2012;92(10):2133–2140. doi:10.1002/jsfa.5597.
- Tomova A, Bukovsky I, Rembert E, Yonas W, Alwarith J, Barnard ND, Kahleova H. The effects of vegetarian and vegan diets on gut microbiota. Front Nutr. 2019;6:47. doi:10.3389/fnut.2019.00047.
- Thomson C, Garcia AL, Edwards CA. Interactions between dietary fibre and the gut microbiota. Proc Nutr Soc. 2021;80(4):398–408. doi:10.1017/S0029665121002834.
- Müller L, Kuhn T, Koch M, Fuhrmann G. Stimulation of probiotic bacteria induces release of membrane vesicles with augmented anti-inflammatory activity. ACS Appl Bio Mater. 2021;4(5):3739–3748. doi:10.1021/acsabm.0c01136.
- Farmer AD, Mohammed SD, Dukes GE, Scott SM, Hobson AR. Caecal pH is a biomarker of excessive colonic fermentation. World J Gastroenterol: WJG. 2014;20(17):5000. doi:10.3748/wjg.v20.i17.5000.
- Duncan SH, Louis P, Thomson JM, Flint HJ. The role of pH in determining the species composition of the human colonic microbiota. Environ Microbiol. 2009;11(8):2112–2122. doi:10.1111/j.1462-2920.2009.01931.x.
- Luo Z, Ji Y, Gao H, Dos Reis FCG, Bandyopadhyay G, Jin Z, Ly C, Chang Y-J, Zhang D, Kumar D. et al. CRIg+ macrophages prevent gut microbial DNA–containing extracellular vesicle–induced tissue inflammation and insulin resistance. Gastroenterology. 2021;160(3):863–874. doi:10.1053/j.gastro.2020.10.042.
- Bitto NJ, Zavan L, Johnston EL, Stinear TP, Hill AF, Kaparakis-Liaskos M, Edelmann MJ. Considerations for the analysis of bacterial membrane vesicles: methods of vesicle production and quantification can influence biological and experimental outcomes. Microbiol Spectr. 2021;9(3):e01273–21. doi:10.1128/Spectrum.01273-21.
- Li C-C, Hsu W-F, Chiang P-C, Kuo M-C, Wo AM, Tseng YJ. Characterization of markers, functional properties, and microbiome composition in human gut-derived bacterial extracellular vesicles. Gut Microbes. 2023;15(2):2288200. doi:10.1080/19490976.2023.2288200.
- Xiu L, Wu Y, Lin G, Zhang Y, Huang L. Bacterial membrane vesicles: orchestrators of interkingdom interactions in microbial communities for environmental adaptation and pathogenic dynamics. Front Immunol. 2024;15:1371317. doi:10.3389/fimmu.2024.1371317.
- Faddetta T, Renzone G, Vassallo A, Rimini E, Nasillo G, Buscarino G, Agnello S, Licciardi M, Botta L, Scaloni A. et al. Streptomyces coelicolor vesicles: many molecules to be delivered. Appl Environ Microb. 2022;88(1):e01881–21. doi:10.1128/AEM.01881-21.
- Elhenawy W, Debelyy MO, Feldman MF, Whiteley M, Greenberg EP. Preferential packing of acidic glycosidases and proteases into Bacteroides outer membrane vesicles. MBio. 2014;5(2):e00909–14. doi:10.1128/mBio.00909-14.
- Evans AG, Davey HM, Cookson A, Currinn H, Cooke-Fox G, Stanczyk PJ, Whitworth DE. Predatory activity of Myxococcus xanthus outer-membrane vesicles and properties of their hydrolase cargo. Microbiology. 2012;158(11):2742–2752. doi:10.1099/mic.0.060343-0.
- Chelakkot C, Choi Y, Kim D-K, Park HT, Ghim J, Kwon Y, Jeon J, Kim M-S, Jee Y-K, Gho YS. et al. Akkermansia muciniphila-derived extracellular vesicles influence gut permeability through the regulation of tight junctions. Experimental & Molecular Medicine. 2018;50(2):e450–e. doi:10.1038/emm.2017.282.
- Shen Y, Torchia MLG, Lawson GW, Karp CL, Ashwell JD, Mazmanian SK. Outer membrane vesicles of a human commensal mediate immune regulation and disease protection. Cell Host Microbe. 2012;12(4):509–520. doi:10.1016/j.chom.2012.08.004.
- Chu H, Khosravi A, Kusumawardhani IP, Kwon AH, Vasconcelos AC, Cunha LD, Mayer AE, Shen Y, Wu W-L, Kambal A. et al. Gene-microbiota interactions contribute to the pathogenesis of inflammatory bowel disease. Science. 2016;352(6289):1116–1120. doi:10.1126/science.aad9948.
- Shi J, Ma D, Gao S, Long F, Wang X, Pu X, Cannon RD, Han T-L. Probiotic Escherichia coli Nissle 1917-derived outer membrane vesicles modulate the intestinal microbiome and host gut-liver metabolome in obese and diabetic mice. Front Microbiol. 2023;14:14. doi:10.3389/fmicb.2023.1219763.
- Fábrega MJ, Aguilera L, Gimenez R, Varela E, Alexandra CañCañAs M, Antolín M, Badía J, Baldomà L. Activation of immune and defense responses in the intestinal mucosa by outer membrane vesicles of commensal and probiotic Escherichia coli strains. Front Microbiol. 2016;7:705. doi:10.3389/fmicb.2016.00705.
- Alvarez C-S, Badia J, Bosch M, Giménez R, Baldomà L. Outer membrane vesicles and soluble factors released by probiotic Escherichia coli Nissle 1917 and commensal ECOR63 enhance barrier function by regulating expression of tight junction proteins in intestinal epithelial cells. Front Microbiol. 2016;7:1981. doi:10.3389/fmicb.2016.01981.
- Alvarez C-S, Giménez R, CañCañAs M-A, Vera R, Díaz-Garrido N, Badia J, Baldomà L. Extracellular vesicles and soluble factors secreted by Escherichia coli Nissle 1917 and ECOR63 protect against enteropathogenic E. coli-induced intestinal epithelial barrier dysfunction. BMC Microbiol. 2019;19(1):1–12. doi:10.1186/s12866-019-1534-3.
- Fleetwood AJ, Lee MK, Singleton W, Achuthan A, Lee M-C, O’Brien-Simpson NM, Cook AD, Murphy AJ, Dashper SG, Reynolds EC. et al. Metabolic remodeling, inflammasome activation, and pyroptosis in macrophages stimulated by Porphyromonas gingivalis and its outer membrane vesicles. Front Cell Infect Microbiol. 2017;7:351. doi:10.3389/fcimb.2017.00351.
- Ghaderi F, Sotoodehnejadnematalahi F, Hajebrahimi Z, Fateh A, Siadat SD. Effects of active, inactive, and derivatives of Akkermansia muciniphila on the expression of the endocannabinoid system and PPARs genes. Sci Rep. 2022;12(1):10031. doi:10.1038/s41598-022-13840-8.
- Bittel M, Reichert P, Sarfati I, Dressel A, Leikam S, Uderhardt S, Stolzer I, Phu TA, Ng M, Vu NK. et al. Visualizing transfer of microbial biomolecules by outer membrane vesicles in microbe‐host‐communication in vivo. J Extracell Vesicles. 2021;10(12):e12159. doi:10.1002/jev2.12159.
- Turunen J, Tejesvi MV, Suokas M, Virtanen N, Paalanne N, Kaisanlahti A, Reunanen J, Tapiainen T. Bacterial extracellular vesicles in the microbiome of first-pass meconium in newborn infants. Pediatr Res. 2023;93(4):887–896. doi:10.1038/s41390-022-02242-1.
- Nikkari S, McLaughlin IJ, Bi W, Dodge DE, Relman DA. Does blood of healthy subjects contain bacterial ribosomal DNA? J Clin Microbiol. 2001;39(5):1956–1959.
- Païssé S, Valle C, Servant F, Courtney M, Burcelin R, Amar J, Lelouvier B. Comprehensive description of blood microbiome from healthy donors assessed by 16 S targeted metagenomic sequencing. Transfusion. 2016;56(5):1138–1147. doi:10.1111/trf.13477.
- Massier L, Chakaroun R, Tabei S, Crane A, Didt KD, Fallmann J, von Bergen M, Haange S-B, Heyne H, Stumvoll M. et al. Adipose tissue derived bacteria are associated with inflammation in obesity and type 2 diabetes. Gut. 2020;69(10):1796–1806. doi:10.1136/gutjnl-2019-320118.
- Gosiewski T, Ludwig-Galezowska A, Huminska K, Sroka-Oleksiak A, Radkowski P, Salamon D, Wojciechowicz J, Kus-Slowinska M, Bulanda M, Wolkow PP. et al. Comprehensive detection and identification of bacterial DNA in the blood of patients with sepsis and healthy volunteers using next-generation sequencing method-the observation of DNAemia. Eur J Clin Microbiol Infect Dis. 2017;36(2):329–336. doi:10.1007/s10096-016-2805-7.
- Chakaroun RM, Massier L, Kovacs P. Gut microbiome, intestinal permeability, and tissue bacteria in metabolic disease: perpetrators or bystanders? Nutrients. 2020;12(4):1082. doi:10.3390/nu12041082.
- Balmer ML, Slack E, De Gottardi A, Lawson MA, Hapfelmeier S, Miele L, Grieco A, Van Vlierberghe H, Fahrner R, Patuto N. et al. The liver may act as a firewall mediating mutualism between the host and its gut commensal microbiota. Sci Transl Med. 2014;6(237):ra23766–ra66. doi:10.1126/scitranslmed.3008618.
- Villarroel J, Donkin I, Champion C, Burcelin R, Barrès R. Endurance training in humans modulates the bacterial DNA signature of skeletal muscle. Biomedicines. 2021;10(1):64. doi:10.3390/biomedicines10010064.
- Pietrzak B, Kawacka I, Olejnik-Schmidt A, Schmidt M. Circulating microbial cell-free DNA in health and disease. Int J Mol Sci. 2023;24(3):3051. doi:10.3390/ijms24033051.
- Han DS, Lo YD. The nexus of cfDNA and nuclease biology. Trends Genet. 2021;37(8):758–770. doi:10.1016/j.tig.2021.04.005.
- Thaiss CA, Levy M, Grosheva I, Zheng D, Soffer E, Blacher E, Braverman S, Tengeler AC, Barak O, Elazar M. et al. Hyperglycemia drives intestinal barrier dysfunction and risk for enteric infection. Science. 2018;359(6382):1376–1383. doi:10.1126/science.aar3318.
- Chang J, Leong RW, Wasinger VC, Ip M, Yang M, Phan TG. Impaired intestinal permeability contributes to ongoing bowel symptoms in patients with inflammatory bowel disease and mucosal healing. Gastroenterology. 2017;153(3):723–31. e1. doi:10.1053/j.gastro.2017.05.056.
- Epple H-J, Schneider T, Troeger H, Kunkel D, Allers K, Moos V, Amasheh M, Loddenkemper C, Fromm M, Zeitz M. et al. Impairment of the intestinal barrier is evident in untreated but absent in suppressively treated HIV-infected patients. Gut. 2009;58(2):220–227. doi:10.1136/gut.2008.150425.
- Tran L, Greenwood-Van Meerveld B. Age-associated remodeling of the intestinal epithelial barrier. J Gerontol A Biol Sci Med Sci. 2013;68(9):1045–1056. doi:10.1093/gerona/glt106.
- Tulkens J, Vergauwen G, Van Deun J, Geeurickx E, Dhondt B, Lippens L, De Scheerder M-A, Miinalainen I, Rappu P, De Geest BG. et al. Increased levels of systemic LPS-positive bacterial extracellular vesicles in patients with intestinal barrier dysfunction. Gut. 2020;69(1):191–193. doi:10.1136/gutjnl-2018-317726.
- Parlesak A, Schäfer C, Schütz T, Bode JC, Bode C. Increased intestinal permeability to macromolecules and endotoxemia in patients with chronic alcohol abuse in different stages of alcohol-induced liver disease. J Hepatol. 2000;32(5):742–747. doi:10.1016/S0168-8278(00)80242-1.
- Cheng WT, Kantilal HK, Davamani F. The mechanism of Bacteroides fragilis toxin contributes to colon cancer formation. Malaysian J Med Sci: MJMS. 2020;27(4):9. doi:10.21315/mjms2020.27.4.2.
- Wick EC, Rabizadeh S, Albesiano E, Wu X, Wu S, Chan J, Rhee K-J, Ortega G, Huso DL, Pardoll D. et al. Stat3 activation in murine colitis induced by enterotoxigenic Bacteroides fragilis. Inflamm Bowel Dis. 2014;20(5):821–834. doi:10.1097/MIB.0000000000000019.
- Tsuzuno T, Takahashi N, Yamada‐Hara M, Yokoji‐Takeuchi M, Sulijaya B, Aoki‐Nonaka Y, Matsugishi A, Katakura K, Tabeta K, Yamazaki K. et al. Ingestion of Porphyromonas gingivalis exacerbates colitis via intestinal epithelial barrier disruption in mice. J Periodontal Res. 2021;56(2):275–288. doi:10.1111/jre.12816.
- Furuta N, Tsuda K, Omori H, Yoshimori T, Yoshimura F, Amano A. Porphyromonas gingivalis outer membrane vesicles enter human epithelial cells via an endocytic pathway and are sorted to lysosomal compartments. Infect Immun. 2009;77(10):4187–4196. doi:10.1128/IAI.00009-09.
- Furuta N, Takeuchi H, Amano A. Entry of Porphyromonas gingivalis outer membrane vesicles into epithelial cells causes cellular functional impairment. Infect Immun. 2009;77(11):4761–4770. doi:10.1128/IAI.00841-09.
- Apovian CM, Bigornia S, Mott M, Meyers MR, Ulloor J, Gagua M, McDonnell M, Hess D, Joseph L, Gokce N. et al. Adipose macrophage infiltration is associated with insulin resistance and vascular endothelial dysfunction in obese subjects. Arterioscler Thromb Vasc Biol. 2008;28(9):1654–1659. doi:10.1161/ATVBAHA.108.170316.
- Lee K-E, Kim J-K, Han S-K, Lee DY, Lee H-J, Yim S-V, Kim D-H. The extracellular vesicle of gut microbial Paenalcaligenes hominis is a risk factor for vagus nerve-mediated cognitive impairment. Microbiome. 2020;8(1):1–18. doi:10.1186/s40168-020-00881-2.
- Emery DC, Shoemark DK, Batstone TE, Waterfall CM, Coghill JA, Cerajewska TL, Davies M, West NX, Allen SJ. 16S rRNA next generation sequencing analysis shows bacteria in Alzheimer’s post-mortem brain. Front Aging Neurosci. 2017;9:195. doi:10.3389/fnagi.2017.00195.
- Bell RD, Winkler EA, Singh I, Sagare AP, Deane R, Wu Z, Holtzman DM, Betsholtz C, Armulik A, Sallstrom J. et al. Apolipoprotein E controls cerebrovascular integrity via cyclophilin A. Nature. 2012;485(7399):512–516. doi:10.1038/nature11087.
- Zhan X, Stamova B, Jin L-W, DeCarli C, Phinney B, Sharp FR. Gram-negative bacterial molecules associate with Alzheimer disease pathology. Neurology. 2016;87(22):2324–2332. doi:10.1212/WNL.0000000000003391.
- Kim SY, Yi DY. Analysis of the human breast milk microbiome and bacterial extracellular vesicles in healthy mothers. Experimental & Molecular Medicine. 2020;52(8):1288–1297. doi:10.1038/s12276-020-0470-5.
- Gao H, Luo Z, Ji Y, Tang K, Jin Z, Ly C, Sears DD, Mahata S, Ying W. Accumulation of microbial DNAs promotes to islet inflammation and β cell abnormalities in obesity in mice. Nat Commun. 2022;13(1):565. doi:10.1038/s41467-022-28239-2.
- Erttmann SF, Swacha P, Aung KM, Brindefalk B, Jiang H, Härtlova A, Uhlin BE, Wai SN, Gekara NO. The gut microbiota prime systemic antiviral immunity via the cGAS-STING-IFN-I axis. Immunity. 2022;55(5):847–61. e10. doi:10.1016/j.immuni.2022.04.006.
- Kredel LI, Batra A, Stroh T, Kühl AA, Zeitz M, Erben U. et al. Adipokines from local fat cells shape the macrophage compartment of the creeping fat in Crohn’s disease. Gut. 2013;62(6):852–862. doi:10.1136/gutjnl-2011-301424.
- Bilski J, Mazur-Bialy A, Wojcik D, Surmiak M, Magierowski M, Sliwowski Z, Pajdo R, Kwiecien S, Danielak A, Ptak-Belowska A. et al. Role of obesity, mesenteric adipose tissue, and adipokines in inflammatory bowel diseases. Biomolecules. 2019;9(12):780. doi:10.3390/biom9120780.
- Wu Z, Tan J, Chi Y, Zhang F, Xu J, Song Y, Cong X, Wu N, Liu Y. Mesenteric adipose tissue contributes to intestinal barrier integrity and protects against nonalcoholic fatty liver disease in mice. Am J Physiol-Gastr L. 2018;315(5):G659–G670. doi:10.1152/ajpgi.00079.2018.
- Anhê FF, Jensen BAH, Varin TV, Servant F, Van Blerk S, Richard D, Marceau S, Surette M, Biertho L, Lelouvier B. et al. Type 2 diabetes influences bacterial tissue compartmentalisation in human obesity. Nat Metab. 2020;2(3):233–242. doi:10.1038/s42255-020-0178-9.
- Ali S, Malloci M, Safiedeen Z, Soleti R, Vergori L, Vidal-Gómez X, Besnard C, Dubois S, Le Lay S, Boursier J. et al. LPS-enriched small extracellular vesicles from metabolic syndrome patients trigger endothelial dysfunction by activation of TLR4. Metabolism. 2021;118:154727. doi:10.1016/j.metabol.2021.154727.
- Prados-Rosales R, Baena A, Martinez LR, Luque-Garcia J, Kalscheuer R, Veeraraghavan U, Camara C, Nosanchuk JD, Besra GS, Chen B. et al. Mycobacteria release active membrane vesicles that modulate immune responses in a TLR2-dependent manner in mice. J Clin Invest. 2011;121(4):1471–1483. doi:10.1172/JCI44261.
- Jin M, Lai Y, Zhao P, Shen Q, Su W, Yin Y, Zhang W. Effects of peptidoglycan on the development of steatohepatitis. Biochimica et Biophysica Acta (BBA) - Mol Cell Biol Lipids. 2020;1865(4):158595. doi:10.1016/j.bbalip.2019.158595.
- Chen H, Sun L, Feng L, Mulholland M, Zhang W, Yin Y. Peptidoglycan inhibits beigeing of adipose tissue. Acta Pharm Sin B. 2022;12(2):990. doi:10.1016/j.apsb.2021.11.015.
- Nonogaki K, Moser A, Pan X-M, Staprans I, Grunfeld C, Feingold K. Lipoteichoic acid stimulates lipolysis and hepatic triglyceride secretion in rats in vivo. J Lipid Res. 1995;36(9):1987–1995. doi:10.1016/S0022-2275(20)41116-2.
- Galton DJ. The human adipose cell: a model for errors in metabolic regulation. London (UK): Butterworth & Co. Ltd.; 1971.
- Cani PD, Amar J, Iglesias MA, Poggi M, Knauf C, Bastelica D, Neyrinck AM, Fava F, Tuohy KM, Chabo C. et al. Metabolic endotoxemia initiates obesity and insulin resistance. Diabetes. 2007;56(7):1761–1772. doi:10.2337/db06-1491.
- Bitto NJ, Cheng L, Johnston EL, Pathirana R, Phan TK, Poon IK, O’Brien‐Simpson NM, Hill AF, Stinear TP, Kaparakis‐Liaskos M. et al. Staphylococcus aureus membrane vesicles contain immunostimulatory DNA, RNA and peptidoglycan that activate innate immune receptors and induce autophagy. J Extracell Vesicles. 2021;10(6):e12080. doi:10.1002/jev2.12080.
- Bellot P, García‐Pagán JC, Francés R, Abraldes JG, Navasa M, Pérez‐Mateo M, Such J, Bosch J. Bacterial DNA translocation is associated with systemic circulatory abnormalities and intrahepatic endothelial dysfunction in patients with cirrhosis. Hepatology. 2010;52(6):2044–2052. doi:10.1002/hep.23918.
- Goto T, Lee J-Y, Teraminami A, Kim Y-I, Hirai S, Uemura T, Inoue H, Takahashi N, Kawada T. Activation of peroxisome proliferator-activated receptor-alpha stimulates both differentiation and fatty acid oxidation in adipocytes. Journal Of Lipid Research. 2011;52(5):873–884. S. doi:10.1194/jlr.M011320.
- Yang X, Gonzalez FJ, Huang M, Bi H. Nuclear receptors and non-alcoholic fatty liver disease: An update. Liver Res. 2020;4(2):88–93. doi:10.1016/j.livres.2020.03.001.
- Bonnington KE, Kuehn MJ, Miller SI, Greenberg EP. Outer membrane vesicle production facilitates LPS remodeling and outer membrane maintenance in Salmonella during environmental transitions. MBio. 2016;7(5): doi:10.1128/mBio.01532-16.