ABSTRACT
Functional gastrointestinal disorders (FGIDs), chronic disorders characterized by either abdominal pain, altered intestinal motility, or their combination, have a worldwide prevalence of more than 40% and impose a high socioeconomic burden with a significant decline in quality of life. Recently, FGIDs have been reclassified as disorders of gut–brain interaction (DGBI), reflecting the key role of the gut-brain bidirectional communication in these disorders and their impact on psychological comorbidities. Although, during the past decades, the field of DGBIs has advanced significantly, the molecular mechanisms underlying DGBIs pathogenesis and pathophysiology, and the role of the gut microbiome in these processes are not fully understood. This review aims to discuss the latest body of literature on the complex microbiota-gut-brain interactions and their implications in the pathogenesis of DGBIs. A better understanding of the existing communication pathways between the gut microbiome and the brain holds promise in developing effective therapeutic interventions for DGBIs.
Introduction
Functional gastrointestinal disorders (FGIDs) are recurrent and chronic gastrointestinal disorders characterized by abdominal pain, intestinal motility alterations, diarrhea, constipation, nausea, bloating, vomiting, or a combination of these symptoms.Citation1–3 FGIDs are very frequent with a prevalence of more than 40% worldwide, more common in women than men.Citation1 The most common FGIDs are irritable bowel syndrome (IBS) and functional dyspepsia (FD), with both disorders significantly affecting quality of life and global health-care costs.Citation4–6 Despite the high prevalence, individual and societal impact of these conditions, their etiology, and pathophysiology remain mostly unclear. Indeed, the absence of organic and structural pathologic changes leads to the struggle in diagnosis and treatment approaches.Citation7 The most recent Rome IV criteria re-classified FGIDs as disorders of gut–brain interaction (DGBI), acknowledging the role of the psychological aspects in FGIDs physiopathology, as more than two-third of patients suffer from psychological comorbidities.Citation2,Citation3,Citation8 The adoption of this novel definition has played a pivotal role in accelerating the progress of the field of neurogastroenterology, with anxiety, depression, and neuroticism emerging as the most frequently reported psychological comorbidities in these patients.Citation9 The gut microbiome, the collection of microorganisms present in the gastrointestinal tract, is a major player in gut–brain interactionsCitation10,Citation11 and alterations of gut microbiome composition are commonly associated with gastrointestinal disorders.Citation12–14 Therefore, this review aims first to cover the latest evidence on the role of the gut-brain axis and the microbiome in DGBI, with specific attention to IBS and FD. Next, our focus will shift toward elucidating the most recent progress in comprehending the mechanisms that contribute to this disturbed microbiome-gut-brain interaction in these disorders. Finally, we will recapitulate the existing treatments and promising novel therapies for DGBI, targeting not only gut microbiome and gut function, but also the brain and the psychological aspects of these disorders.
The gut microbiome
The gut microbiome, a key regulator of gut–brain interaction, is the collection of bacteria, viruses, archaea, and eukaryotes that reside in the human intestinal environment. After rapid colonization at birth, the human intestinal microbiome changes throughout the first 2 years of life, diversifying and then stabilizing into adulthood.Citation15,Citation16 Each adult ultimately develops a distinctive microbiome configuration, which appears to be primarily influenced by stochastic environmental exposures in the past,Citation17 and additional factors like diet, antibiotic therapy, and host genetics.Citation18 Though the origin of microbiome research is often debated, 19th century European scientists Theodor Escherich, Henry Tissier, Ilya Metchnikov, and Alfred Nissle are believed to have pioneered the field of gut microbiome research in human health and disease.Citation19–25 Studies on germ-free, microbiome-depleted, or gnotobiotic animals, have highlighted the central role of the microbiome in normal gut physiology, motility, metabolism, and immune functioning.Citation26–34 Indeed, it is now well known that the gut microbiome is beneficial to multiple aspects of host function, from host-metabolism, biochemical regulation of processes such as gluconeogenesis, lipogenesis, and cholesterol synthesis,Citation35 to healthy aging.Citation36,Citation37 This also applies to the gut function, including motility, permeability, and visceral sensitivity. We have recently shown that the gut microbiota drives the physiological development of small intestinal transit via Toll-like receptor (TLR) signaling and small intestinal vasoactive intestinal polypeptide (VIP) regulation of cholinergic nerves.Citation38 The microbiota is also key to the development of a normal perception of inflammatory, mechanical, and visceral pain.Citation39–45 We have found that the absence of gut microbiota increases visceral sensitivity to colorectal distention through elevated production of calcitonin gene-related peptide (CGRP) by dorsal root ganglia (DRG) neurons in a sex-dependent manner.Citation46 Besides the use of gnotobiotic mouse models, the incorporation of modern techniques such as whole genome and targeted metagenomic sequencing,Citation47,Citation48 as well as the use of ingestible sampling devices,Citation49,Citation50 have aided our understanding of gut microbial influence on health and disease.
Diet is one major factor affecting microbial community structure and function. However, different diets are found to be associated with different beneficial health effects depending on the person.Citation51 It has been shown that the same food can elicit divergent responses in different individuals, and the use of personalized nutrition versus a “one size fits all” approach has been widely advocated.Citation52,Citation53
Environmental lifestyle is another factor that heavily influences intestinal microbiota composition in multiple ways. Factors including living conditions, antibiotic and medication use, geographical region, lifestyle choices, number of household members, and even pet ownership can contribute to the composition of human microbiota.Citation54,Citation56 Alcohol and tobacco consumption as well have been shown to affect microbiota composition and diversity, disrupting the gut microbiome.Citation57,Citation58 Very recently, it has been proposed that social microbial transmission, occurring through social interactions and relationships, also plays an important role shaping host health.Citation59 Indeed, the authors speculate that socially transmissible commensals and mutualists may modify disease risk for both communicable and non-communicable diseases.Citation59 Thus, while it is evident that the gut microbiome is crucial to gut health and homeostasis, we should also remember that it does not live in isolation within the GI tract. Instead, it is in constant “contact” with its host, influencing its symbiont not only locally, but distally as well.
Microbiota gut-brain axis
The idea of a gut-brain axis dates far back in time within ancient Greece.Citation60,Citation61 Studies of gastric acid secretion in human subjects with gastric fistulas conducted in the early 1800s by Beaumont first, and by Wolf and Wolff later, as well as the studies conducted on dogs by Pavlov, scientifically consolidated the concept that human emotions affect host physiology (for a historical overview, see Wolf (1981)).Citation60 The gut-brain axis, defined as the bidirectional communication between the central nervous system and the digestive tract, has been linked to the emotional and cognitive centers of the brain with peripheral intestinal functions.Citation62 This bidirectional communication system also includes the autonomic nervous system and the hypothalamic- pituitary-adrenal (HPA) axis.Citation62 Over the past three decades, scientific knowledge on the gut-brain axis has grown exponentially, and the influence of the gut microbiota on the gut-brain axis has become better understood through the increasing availability of gnotobiotic models. We know today that our microbiome is important for brain structure, development, neurophysiology, and behavior.Citation10 This bidirectional communication between the gut, its microbiome, and the brain has also been shown to influence sugar preference,Citation63,Citation64 mental health,Citation65 mood,Citation66 and even decision-making.Citation67 Even though the idea that the gut microbiome possibly manipulates host’s behavior to its benefit might be intriguing, the ideas of evolved dependence or local manipulation are likely more realistic,Citation68 with the gut microbiome “involuntarily” influencing host’s behavior and brain biochemistry. Clinical and experimental evidence suggests that the gut microbiome, gut, and brain might communicate through multiple direct and indirect pathways, including neural, immunological, endocrine, and metabolic mechanisms.Citation62
The vagus nerve, extending from the brain stem to the intestine, is responsible for regulating digestion, vasomotor activity, and the contraction of smooth muscle and glandular secretion.Citation69 Additionally, it is considered one of the major communication pathways between gut bacteria and the brain. Vagal nerve afferent fibers reach the brain in the dorsal motor nucleus of the vagus, the area postrema (AP), and the nucleus of the solitary tract (NTS).Citation70 Vagal mediation of microbial signals may occur both centrally and peripherally. While more attention has been paid to the NTS as a docking site for vagal afferents, not many studies have looked at the AP, despite its privileged location within the central nervous system (CNS) but outside the blood-brain barrier (BBB), where neurons can be directly influenced by circulating signals.Citation70 In the periphery, vagal neurons themselves synapse with intestinal enteroendocrine cells called neuropod cells,Citation71 and convey the presence of sugar, regulating sugar preference.Citation63,Citation72 Centrally, however, this preference relies on reward neurons, those that mediate motivation and dopamine activity that reside in the right vagus.Citation73
Another way of communication between the gut and brain is through modulation of the immune system, which can also be multifaceted. During the last decade, we have learnt that bacteria and bacterial products (peptidoglycan (PGN), lipopolysaccharide (LPS), polysaccharide A (PSA), and short-chain fatty acids (SCFAs) among others) influence the brain and behavior directly or indirectly.Citation74–78 Recently, a plethora of studies have shown that bacteria and their by-products can influence brain gene expression, cytokines release, and behavior.Citation79–82 These changes can occur through local stimulation of pattern-associated molecular patternsCitation83,Citation84 or through the education of immune cells, which then migrate to CNS-associated lymphoid tissue (cervical lymph nodes).Citation75 We have recently shown that the establishment of normal behavior in mice depends critically on bacteria-induced activation and migration of intestinal dendritic cells into the brain through the innate immune system and TLR signaling.Citation85 Throughout the course of infection, bacteria, endotoxins, and subsequent cytokine-release not only trigger sickness behaviorCitation86,Citation87 and activate specific neuronal populations,Citation88–91 but can also exploit the meningeal neuroimmune barrier, particularly in the case of bacterial meningitis, to facilitate their penetration into the CNS.Citation92
While there was some controversy during the past 5 years on whether bacteria themselves can directly access the brain and thus modulate brain biochemistry and behavior,Citation93–98 it is essential to learn from the cautionary tale of the extended dispute surrounding the fetal microbiome.Citation99 Until robust evidence is presented confirming that bacteria can be found in the brain in non-extreme conditions, a cautious approach is essential.
Finally, bacteria themselves may directly modulate gut-brain communication, as they secrete hormones, catecholamines, neurotransmitters, and lipopeptides,Citation100–104 metabolize host hormones,Citation105 and modify the structure and the efficacy of commonly used medications.Citation106–111 Altogether, these studies highlight how microbial influence on gut-brain communication may occur through multiple routes; thus, the discovery of specific pathways of action will lead to better tailored treatments for microbiome-gut-brain axis disorders.
Gut brain interactive disorders
The growing recognition of bidirectional interactions between the gut and the brain has greatly contributed to our understanding and management of FGIDs. Indeed, until 2016, FGIDs were defined as a heterogeneous group of chronic or recurrent gastrointestinal conditions that could not be explained by structural or biochemical abnormalities.Citation7 Consequently, physicians struggled to identify and understand FGIDs, as they were characterized by the absence of demonstrable anatomic, inflammatory, immunologic, neoplastic, or metabolic response biomarkers, making it difficult to explain patient’s symptoms.Citation7 Since 2006, FGIDs were diagnosed and classified using the Rome III criteria which classified adult FGIDs into six categories: esophageal, gastroduodenal, bowel, functional abdominal pain syndrome, functional gallbladder, sphincter of Oddi, and anorectal.Citation7 This classification system was updated by the Rome IV criteria system in 2016 resulting in the following categories: esophageal, gastroduodenal, bowel, centrally mediated disorders of gastrointestinal pain, functional gallbladder disorders, sphincter of Oddi disorders, and anorectal disorders.Citation2,Citation3 This latest classification included biological in addition to social and psychological aspects of the pathogenesis, with a new definition of FGIDs as DGBI (). Several evidence showed that DGBI can result from the combination of genetic, environmental factors such as antibiotic exposure, infections, and physical or sexual abuse, resulting in synergetic gut and psychological health dysfunctions.Citation7,Citation112–115 Importantly, the updated definition acknowledged the crucial role of the gut-brain axis and the bidirectional communication between the enteric nervous system (ENS) and central nervous system (CNS) in DGBI pathophysiology.Citation116 While the validity of the Rome criteria has been criticized and debated in the past,Citation117,Citation118 recent global population-based studies have demonstrated that the Rome IV criteria is a valid tool to diagnose DGBI.Citation1,Citation119
Figure 1. The role of microbiome in disorders of gut brain interaction (DGBI).
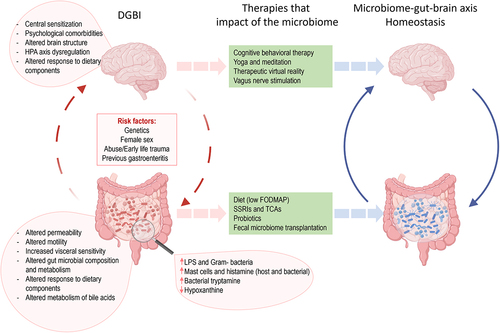
One of the most common and studied DGBI is IBS, with a worldwide prevalence estimated at 4.1–6.1% (Rome IV), varying significantly depending on cohort country and sex, with a higher prevalence in women than men.Citation1,Citation120 IBS is a disorder characterized by abdominal pain and altered gut motor function, where patients often present with enhanced visceral perception, altered gut microbial composition and function,Citation3,Citation12–14,Citation121–123 as well as genetic and psychosocial components.Citation7,Citation112,Citation124–127 Using the Bristol Stool scale, IBS is classified into four different categories according to stool form and bowel habits: IBS with constipation (IBS-C), IBS with diarrhea (IBS-D), Mixed IBS, and Unsubtyped IBS (IBS-U).Citation128 While this classification has been created to guide treatment, it has been deemed insufficient to capture the complexity of IBS, a complex multifactorial DGBI. Indeed, seven distinct IBS subgroups have been identified, depending on the severity of gastrointestinal symptoms, extraintestinal symptoms, and psychological comorbidity,Citation5,Citation129,Citation130 all factors that weigh not only on patients’ quality of life but also on health-care utilization and costs.
Another common chronic DGBI with an important impact on patient’s quality of life is FD. Large cohort-based studies reported a prevalence of FD varying from 10% to 30% worldwide.Citation131,Citation132 FD putative causes include impaired gastric accommodation to a meal, delayed gastric emptying, duodenal sensitivity to acids and visceral hypersensitivity, often associated with genetic and psychosocial factors.Citation3,Citation131 Of note, IBS and FD often manifest with similar symptoms; therefore, these disorders may frequently co-exist. It has been shown that patients with an overlap of IBS and FD reported significantly more gastrointestinal symptoms with a greater impact on quality of life and were more likely to report abnormal anxiety and depression scores.Citation133,Citation134
As mentioned earlier, the psychosocial and psychological components are among the key factors involved in the pathophysiology of DGBI. The increasing literature about psychiatric comorbidities in patients with DGBI supports the idea of an altered gut–brain interaction.Citation9,Citation135–140 The most common psychiatric comorbidities associated with DGBI include but are not limited to: anxiety occurring in 30–50% of DGBI subjects, depressive disorders, neuroticism,Citation136,Citation138,Citation141–145 and sleep disorders.Citation146 This is mainly evident in patients with IBS, where many studies revealed increased levels of depression and anxiety in comparison to healthy subjects.Citation147–149 Psychological comorbidities have been positively associated with higher IBS symptom severity.Citation150 A meta-analysis review of 73 cohort studies found that patients with IBS present with three times more risk of developing depression or anxiety when compared to healthy controls.Citation147 Similarly, in 2023, an internet survey of the Rome Foundation Global Epidemiology Study on patients from 26 countries worldwide found that psychological comorbidities were reported in 37.5% of the participants and that those participants had 4.45 times higher odds to meet criteria for at least one DGBI.Citation138 In line with these findings, a community telephone survey reported a fivefold higher risk of generalized anxiety disorder in responders with diagnosed IBS in comparison to non-IBS individuals.Citation151 Indeed, the prevalence of anxiety and depression comorbidities increases with the number of coexistent DGBI, as well as the severity and frequency of gastrointestinal symptoms.Citation12 Interestingly, anxiety, but not depression, has been associated with FD,Citation152,Citation153 while both depression and anxiety disorders have been associated with functional constipation (FC), another common DGBI with a prevalence rate of 9.5% worldwide in adults and children.Citation154–158 Of interest, IBS was shown to share extensive genetic traits with anxiety, depression, and neuroticism,Citation126,Citation159 pointing toward the bidirectional link between IBS and psychological conditions.
Hence, it is now evident and widely acknowledged that there is a substantial association between DGBI and psychological health. This recognition has helped to understand the existence of a possible causal link between the two components. However, the direction of the causality remains a “chicken and egg” question, with uncertainty on whether psychological conditions are the cause or the consequence of gut dysfunction and symptoms. Few studies have approached this question and found that psychological comorbidities often precede the development of IBS symptoms.Citation160,Citation161 A population-based study conducted in Sweden on FD patients reported that anxiety level at baseline predicted FD 10 years later using the Rome III criteria.Citation161 Another study involving 4966 patients with IBS, FD, or FC in the United Kingdom indicated that two-thirds of the patients were diagnosed with anxiety or mood disorders prior to DGBI onset. In 2012, a 12-year prospective population-based study suggested a bidirectional gut-brain effect in people with IBS and FD, revealing that gut dysfunction influences the development of anxiety and depression, and vice versa.Citation162 In this study, the authors found higher levels of depression and anxiety at baseline as predictors of DGBI symptoms at follow-up, but also demonstrated that patients meeting DGBI criteria at baseline showed significantly increased levels of depression and anxiety at follow-up visits.Citation162 These findings have been further supported by the same authors in a one-year prospective population-based study.Citation163 However, it is important to note that this shorter study revealed a higher subset of people with IBS and FD symptoms before the development of psychological conditions, suggesting a role of gut dysfunction as a primary driver of psychological impairment. While more studies have attempted to answer the question on the DGBI originating in the gut or brain,Citation164 we should always remember the importance of the gut microbiome, which has been shown to play a central role in gut-brain axis communication, DGBI pathophysiology, and pathogenesis.
The role of gut microbiota in DGBI
The gut microbiome evolves in a symbiotic relationship with its host and is well known to play a major role in many vital physiological functions including intestinal barrier integrity, immune system maturity, digestion, metabolic activities, and more recently CNS development. Thus, it is not surprising to see the ever-growing interest in the role of the microbiome in the pathophysiology of DGBI.Citation11,Citation165,Citation166
While it has been shown that both the mycobiomeCitation167,Citation168 and the viromeCitation169–171 are altered in IBS patients, and may play an important, yet under-investigated role, it is still very difficult to disentangle the fluctuations of these populations from those of the bacterial microbiome.Citation168 Interestingly, a recent multi-omics study found the virome to be temporally stable and not affected by symptomatic flares in IBS patients but to vary among subsets of IBS patients.Citation171 In addition, phages can either directly or indirectly (through affecting the bacterial communities) influence host gene expression.Citation171,Citation172 As for the mycobiome, it has been shown that Candida albicans species abundance is increased in IBS, and that specific C. albicans strains may aid in distinguishing subsets of IBS patients.Citation167 However, larger studies that investigate further the role of both gut virome and mycobiome in relation to host and gut bacterial communities are needed.
Post-infectious (PI) IBS is a great example of the involvement of gut microbiome in the pathogenesis of DGBI, as it is characterized by the development of IBS symptoms following infectious gastroenteritis.Citation173,Citation174 While for PI-IBS the trigger of symptoms has been quite clear for several decades,Citation175–177 the underlying mechanisms are still poorly understood. As much as about 9% of IBS cases in the community have been imputed to previous gastrointestinal enteritis.Citation178 It has been suggested that a synergistic interaction occurs between intestinal inflammation and alterations of gut microbiota, stemming from the infection.Citation178 In addition, the microbiome might also confer different susceptibility to infection.Citation178 Indeed, mechanistic studies have provided evidence to show how infectious gastroenteritis can alter the function and structure of the gut via activation of mast cells, lymphocytes, enterochromaffin cells, enteric nerves, as well as change intestinal permeability, ultimately leading to DGBI symptoms.Citation178–181 While in the case of PI-IBS one would think that the root of the disorder clearly lies in the gut and gut microbiome, increasing evidence also suggests an additional role for psychological factors and stress as major risk factors increasing the severity of infection and susceptibility to develop IBS after gastroenteritis.Citation182–184 Female gender and prior exposure to antibiotics as well add to the risk of developing PI-IBS following an infectious gastroenteritis.Citation178 Genetic predisposition might also be playing a role in the susceptibility to develop PI-IBS,Citation185,Citation186 however more studies are needed to confirm these results. With more than 600 million people recovering from the recent COVID-19 pandemic, caused by SARS-CoV-2Citation187 and with evidence that SARS-CoV-2 can infect the gastrointestinal tract,Citation188 it is plausible to wonder whether the incidence of DGBI, and particularly PI-IBS might increase significantly in the near future. Indeed, a recent study found that 12 months after COVID-19 infection the prevalence of IBS was higher compared with controls.Citation189 IBS risk was increased among patients with a history of allergies, chronic intake of proton pump inhibitors and dyspnea at hospitalization.Citation189
Antibiotic exposure, commonly seen after infectious enteritis, is known to induce microbiota alterations and appears to be a risk factor for DGBI.Citation190,Citation191 Indeed, various studies demonstrated that the development of new-onset IBS symptoms was associated with previous antibiotic use in adults and infants.Citation192–195
Many studies have reported alterations of microbiota composition and diversity in DGBI patients when compared to healthy controlsCitation12–14,Citation196–202 supporting the role of the gut microbiome in the pathophysiology of DGBI. While the research into the gut microbiome in DGBI has grown exponentially during the past decade, and although some bacterial taxa have been reported as altered in a few studies in IBS patients compared to healthy subjects, a particular DGBI microbiome profile does not seem to exist. IBS patients have been shown to have an increased abundance of Firmicutes and a decreased abundance of Bacteroidetes, associated with an increased Firmicutes/Bacteroidetes ratio.Citation12,Citation197,Citation199,Citation203 Similarly, various studies reported increased Clostridia and Clostridiales and decreased Bacteroidia and Bacteroidales as well as a reduction in butyrate-producing and methane-producing bacteriaCitation12,Citation197,Citation199,Citation204 in IBS microbiota. In 2019, a systematic review explored the evidence associating IBS with specific microbiome compositionsCitation201 and found that IBS patients had microbiomes enriched in facultative anaerobes such as Enterobacteriaceae, and depleted in Uncultured Clostridiales I, Faecalibacterium spp., including Faecalibacterium prausnitzii and Bifidobacterium spp.Citation201 However, one of the most striking findings of this analysis was the lack of consistency between the individual studies.Citation201 A previous metaanalysis had similarly shown a down-regulation of Faecalibacterium prausnitzii and Bifidobacterium spp but also Lactobacillus spp in IBS patients.Citation205 A common finding among many of these studies is the overlap between a subgroup of IBS patients and healthy controls,Citation198,Citation199,Citation206 highlighting the heterogeneity of the IBS patient population. While all these studies used stool microbiota, it may well be that the attention should be pointed to other sections of the gastrointestinal tract. While most studies focus on the fecal microbiome as it has a higher bacterial density and is readily accessible, recent research has proposed the small intestine and its microbiome to play a role in IBS and FD.Citation121,Citation207,Citation208 Indeed, the small intestine microbiota has been reported to be altered and to contribute to symptoms in a subset of IBS patients.Citation121 Symptomatic IBS patients present with significant differences in relative abundance of Porphyromonas, Fusobacterium, and Prevotella associated with a lower alpha diversity, richness, and evenness in the small intestine, when compared with healthy volunteers.Citation121 Advanced age, antibiotic use, history of GI surgery, and PPI use were found to significantly contribute to these differences in SI microbiome.Citation121 Similarly, alterations of small intestine microbiota composition have been found in the duodenum of FD patients with differences in the relative abundance of Actinomyces, Prevotella, Veillonella, and Streptococcus, along with correlations between bacterial load and FD symptoms.Citation207
Small intestinal bacterial overgrowth (SIBO), defined as a quantitative increase of the small intestinal bacteria, has been suggested to drive gastrointestinal symptoms such as diarrhea, abdominal pain, and bloating in a subset of IBS patients.Citation121,Citation209 However, several systematic reviews and meta-analyses that investigated a link between IBS and SIBO found an overall low quality of evidence, mainly due to the clinical heterogeneity of subjects included and the limited sensitivity and specificity of the available diagnostic tests. Citation210–212 This controversy is also reflected in recent studies. Although patients with IBS and more than 103 colony forming unit per mL of duodenal aspirates, enrichment in specific E. coli and Klebsiella strains, have been shown to have abdominal pain, gas and diarrhea,Citation209 another study found no correlation between SIBO and gastrointestinal symptoms, and attributed SIBO to environmental influences such as dietary preferences.Citation121
Factors hindering our capacity to find a clear IBS or DGBI bacterial signature include: the lack of consistent methodologies used to assess microbiome composition, the lack of rigorous statistical testing, the cross-sectional nature of most of the data, the geographical variability of data, the omission of dietary information in most studies, the focus on fecal and colonic microbiome alone, and the inherent variability of patients.Citation201,Citation213 In addition, the mucosal microbiota may also play an important role in DGBI pathophysiology. However, the same confounding factors discussed above (location of tested sample, methodology used to assess the microbiome, etc …) apply also to those few studies that ventured into looking at the mucosal microbiota of patients with DGBI, particularly IBS.Citation214–218 Of note, despite all these confounding factors, few studies have found a common decrease in microbiome richness and diversity in IBS patients and an increase in Proteobacteria, particularly Pseudomonas spp.Citation215,Citation217,Citation218 In addition, low-grade inflammation has been recently shown to accompany alterations in the mucosal-associated microbiome.Citation217 Whether an altered mucosal microbiome induces low-grade inflammation or low-grade inflammation impacts the microbiome remains to be determined. Recent studies have revealed enhanced translocation of live bacteria and mucosal immune activation in colonic biopsies of patients with IBS,Citation219 supporting the idea of a compromised intestinal barrier in IBS.Citation220 Likewise, our preclinical study using IBS humanized mice reveal a thinning of the mucus layer and bacterial translocation into the lamina propria.Citation221 This underscores the significance of mucus layer integrity in averting further immune activation, with the microbiome likely playing a significant role in this process.
Furthermore, the microbial metabolic activity, and not necessarily its structure, may be important in DGBI pathogenesis. The metabolome of IBS patients is unique and characterized by altered bile acids, amino acids, fatty acids, organic acids, histamine, tyramine, decreased degradation of lactose and galactose, and increased fermentation of carbohydrates when compared to healthy individuals.Citation14,Citation199,Citation200,Citation202,Citation222,Citation223 In a longitudinal multi-omics study, Mars et al. nicely described not only microbiota composition changes in IBS patients, with higher abundance of multiple Streptococcus spp. and lower abundance of the phylum Synergistetes compared to healthy controls, but also identified the host-microbial pathway of purine metabolism as an important player in the pathophysiology of IBS.Citation14 They identified hypoxanthine that was decreased in IBS likely due to an increased xanthine dehydrogenase/oxidase activity from both the microbiome and the host, suggesting a possible therapeutical target for this subset of IBS patients. In addition, the fecal metabolome of IBS patients has been shown to be able to discriminate between subtypes of IBS.Citation14,Citation199,Citation202,Citation224,Citation225
Abnormal bile acid metabolism with increased fecal primary bile acids or idiopathic bile acid malabsorption (BAM) has been reported in about 30% of patients with IBS-D.Citation226–228 These patients have a significantly faster colonic transit and an altered microbiome with decreased dihydroxylation capacity and sulfatases that distinguish them from other IBS patients.Citation199,Citation200,Citation228 They also present with an upregulation of barrier, immune, and inflammatory markers in the colon, as well as a loss of mucin, pointing toward the detergent and proinflammatory effects of bile acids.Citation228
Besides bile acids, subtypes of IBS may also be differentiated by the abundance of fecal Clostridiales and SCFAs.Citation229 Further, a recent study reported that while IBS-D and IBS-U patient’s microbiome presents with a decreased capacity to degrade lactose and galactose as well as an increased capacity to produce hydrogen sulfide, IBS-C patient’s microbiome has an elevated capacity to degrade phenylethylamine and synthesize palmitoleate.Citation223
Altogether, these data suggest the potential role of the microbial metabolome as a biomarker tool to discriminate DGBI. However, the exploration of microbiome function via the analysis of the fecal metabolome, though initially considered groundbreaking, has clearly produced highly variable results.Citation230 This underscores the importance of adopting a standardized longitudinal multi-omics integrative approach.Citation14,Citation213 The ultimate objective of this approach is to advance the development of mechanism-based targeted therapeutics and to pinpoint the specific patient cohorts that would benefit from these therapies. To this end, translational animal studies provide the mechanistic insight that clinical trials are not able to offer and have significantly advanced the field throughout the past few years. Furthermore, translational studies can contribute to resolving the question regarding whether modifications in the gut microbiome, encompassing shifts in relative abundance, composition, diversity, and function, are cause or consequences of disturbed gastrointestinal motility, and gastrointestinal dysfunction.
We have shown that the gut microbiome plays a causal and functional role in the pathophysiology of IBS, as transplantation of fecal microbiota from IBS patients into germ-free mice resulted in alterations of gastrointestinal transit, gut barrier function, innate immune responses, and behavior.Citation231 In this study, we demonstrated that the metabolism of the microbiome in individuals with IBS is crucial to the model. While the composition of the microbiome was quite similar in mice receiving samples from both healthy individuals and patients with IBS, the serum metabolome was significantly different. Using this model to explore additional microbiota-driven pathogenic mechanisms, we have found that histamine produced by the microbiome can engage the host immune system, in particular mast cells, via activation of histamine 4 receptor (H4R) and lead to the development of visceral hypersensitivity in a subset of IBS patients.Citation221,Citation232 In addition, we show that targeting either H4R or bacterial histamine production through dietary modifications may constitute a novel therapeutic approach for this subset of IBS patients.Citation221,Citation232 However, multiple mechanisms likely play a role in IBS pathophysiology and hypersensitivity, as we also found that in a subset of IBS patients that respond to a low FODMAP (Fermentable Oligosaccharides, Disaccharides, Monosaccharides, and Polyols) diet, histamine together with protease signaling, both likely of bacterial origin, modulate nociceptive nerve activity.Citation233 Indeed, the role of mast cells and histamine, of host and bacterial sources, is central in pain sensation in DGBI.Citation123,Citation221,Citation232–235 Several studies have suggested a mechanistic role of histamine-mediated transient receptor potential channel sensitization in IBS pathophysiology via histamine 1 receptor (H1R) activation, resulting in increased visceral pain perception,Citation181,Citation237–239 where treatment with an H1R antagonist is of moderate efficacy in a subgroup of patients.Citation237–239 These translational studies suggest that multiple mechanisms may be at play in the same subset of patients, thus explaining the modest efficacy of certain medications and advocating for the design of combination therapies that target both the microbiome and the host.
Another recent translational study implicated bacterial proteases in the pathophysiology of PI-IBS, revealing high gut proteolytic activity driven by host serine proteases,Citation240 confirming previous findings.Citation241 This study suggests that a reduction in microbial β-glucuronidase activity may contribute to IBS pathogenesis, as β-glucuronidases released by commensal microbes suppressed host proteolytic activity, thereby protecting the intestinal epithelium.Citation240 Aberrant amino acid metabolism has been reported in IBS patients, and a recent mechanistic study demonstrated how tryptamine, a tryptophan-derived monoamine, regulates ion flux across the intestinal epithelium via 5-Hydroxytryptamine4 (5-HT4) receptor activation, affecting intestinal motility.Citation242
All these studies have not only advanced our understanding of the pathophysiology and pathogenesis of IBS, but also revealed several possible targets for the design of novel therapeutic approaches. While the functional role of the gut microbiota in DGBI is well demonstrated, one must remember to consider its interaction with the central nervous system through the microbiota-gut-brain axis as a potential mechanism for both pathogenesis and treatment. In the next section, we will describe the current most used microbiome-directed therapies for the treatment of symptoms in DGBI.
Current treatments
Within the field of DGBI research, the therapeutic interventions being developed are focusing on symptom relief (). Currently, there are no long-term cures available for DGBI, as our understanding of the underlying mechanisms is incomplete. However, therapies directed to the management of bowel symptoms and the psychological aspect of these disorders have progressed. The latest guidelines for treatment of DGBI from the American Gastroenterological Association (AGA) have recommended to involve nonpharmacologic therapies early in the treatment plan, while moving away from opioid prescription for pain relief.Citation243 While the emphasis is on non-pharmacological therapies, in 2022 the AGA made the recommendation for some pharmacological agents to be used as treatments for IBS-D, such as eluxadoline, rifaximin, and alosetron,Citation244 leaving the use of selective serotonin reuptake inhibitors (SSRIs) and tricyclic antidepressants to the physician’s discretion, given the efficacy for a subset of patients.Citation244 A recent randomized, double-blind, placebo-controlled trial found a beneficial effect of low-dose amitriptyline in IBS, suggesting this inexpensive and safe treatment for general practitioners.Citation245
As the focus of this review is on the microbiome in DGBI, and thus microbiome-directed therapies for DGBI, we should remember that many of the commonly used medications have an overlooked effect on the microbiome.Citation106,Citation108
Bile acid sequestrants, such as cholestyramine, colestipol, and colesevelam, have been used to treat IBS patients with BAD, and while their efficacy needs to be tested with larger clinical trials, it appears that bile acid sequestrants are effective in improving abdominal symptoms and both stool frequency and consistency, without targeting the underlying pathophysiology.Citation226,Citation246 Interestingly, clinical response to bile acid sequestrants appears to be associated with compositional and functional alterations in the gut microbiome, highlighting a possible interplay between bile acids and the gut microbiome.Citation247,Citation248
While antibiotic exposure has been described as a risk factor for development of DGBI, some antibiotics appear to be helpful in treating existing DGBI.Citation249 One of the first antibiotics used in a randomized controlled trial with IBS patients was neomycin which resulted in a greater relief of symptoms in 35% of patients compared to only 11% in placebo subjects.Citation250 However, due to its potential side-effect, in particular ototoxicity, the use of neomycin has been limited. Other antibiotics have been shown to successfully improve both IBS gastrointestinal symptoms and SIBO. Among them, we can find norfloxacinCitation251 and the more commonly used rifaximin, a broad-spectrum, non-absorbable antibiotic.Citation252 Although a recent systematic review and network meta-analysis evaluating pharmacological treatments for IBS-D patients found rifaximin to have limited efficacy in alleviating overall IBS symptoms and abdominal pain, its approval by the Food and Drug Administration (FDA) for treating IBS-D stems from its favorable safety profile and minimal adverse effects.Citation253 While the exact mechanisms of action remain unclear, it has been demonstrated that rifaximin treatment is associated with acceleration of ascending colon transitCitation253 and changes in gut microbiota composition with specific increase in Faecalibacterium abundance correlated to clinical improvement.Citation254 Rifaximin has also been suggested to have direct anti-inflammatory activity, effects on microbial mucosal adherence or bacterial virulence,Citation255 and may act on the CNS given the neuroactive potential of antibiotics.Citation256 Furthermore, rifaximin seems to prevent the development of increased intestinal permeability and visceral hyperalgesia in a model of chronic psychological stress.Citation257
Neuromodulators, such as antidepressants, also possess antimicrobial properties.Citation258 The SSRI fluoxetine, for example, has been shown to act indirectly as a bacteriostatic agent on the bacterium Turicibacter sanguinis that can import serotonin and use it to thrive.Citation259 Thus, the efficacy of some anti-depressants in symptoms of DGBI may be uncoupled from their central effects, and instead be solely peripheral or microbiome mediated. Furthermore, besides the microbiome, genetics may also play a role in influencing neuromodulator metabolism and efficacy.Citation260 As the microbiome is central to both gut physiology and psychological wellbeing, microbiome-directed therapies have been increasingly advocated for DGBI. Diet, as a major modulator of gut microbial composition and function, is also considered a first-line treatment for patients with DGBI,Citation261–263 due to a great proportion of patients often complaining about food triggering or worsening symptoms. While some studies reported a beneficial effect of a gluten-free diet in subsets of patients with IBS,Citation264,Citation265 the evidence is against recommending gluten-free diet for patients with IBS.Citation266,Citation267 Certainly, it remains unclear whether the reported positive effects were a result of gluten protein withdrawal, the reduction of fermentable fructans, the microbiome’s capability to process gluten antigens and alter their immunogenicity, or potentially the presence of amylase trypsin inhibitors (ATIs) found in wheat.Citation264,Citation265,Citation267–272 In addition, our recent study highlights the importance of central mechanisms in a subset of IBS patients with self-perceived gluten sensitivity.Citation273 This may also be the case of FODMAPs, which were shown to modulate the activity of several brain areas related to pain, such as the cerebellum, supramarginal gyrus, anterior and midcingulate cortex, insula, and thalamus in IBS patients.Citation274 The low FODMAP diet is currently one of the most adopted diet interventions for IBS and has been found to be more effective at reducing IBS symptoms than other dietary approaches.Citation275 While insoluble fiber significantly worsens IBS symptoms, soluble fiber has been positively associated with IBS symptom improvements.Citation261 Gunn et al. reported that psyllium reduces inulin-related gas production in IBS patients, suggesting the use of soluble and poorly fermented fibers like psyllium as a prebiotic pairing with FODMAP-rich foods, to achieve the reduction of colonic gas and breath hydrogen response.Citation276 In healthy individuals, however, a low-fiber diet leads to development of gastrointestinal symptoms as well as changes in intestinal permeability and microbial diversity,Citation121 underscoring the importance of individual microbiomes underlying specific dietary responses.
Mechanistic studies have highlighted the role of bacterial LPS, bacterial histamine, and proteases as possible modulators of host responses to diets high in fermentable carbohydrates.Citation233,Citation277–279 Indeed, the microbiome has been postulated as a driver for the individual response to a low FODMAP diet, given that the colonic microbiota ferments undigested carbohydrates reaching the large bowel.Citation263 Furthermore, host genetics have been implicated in host responses to diet modifications in IBS patients.Citation125 For instance, the efficacy of a sucrose and starch-restricted diet or low-FODMAP diet has been recently linked to the dysfunction of the sucrase-isomaltase gene in IBS-D patients.Citation125 Thus, all these data highlight the need to contemplate both host microbiome and genotype when considering dietary restrictions in DGBI, particularly when considering a diet as restrictive as the low FODMAP diet.
The use of probiotics for DGBI treatment has gained traction over the last decade. Despite reports of some efficacy of probiotics in IBS,Citation280 the latest guidelines for IBS and FD have not endorsed their use for global relief of symptoms or specifically bloating and distension, due to the relatively low quality of evidence.Citation281,Citation282 Confounding factors for the assessment of probiotics’ efficacy lie not only in the heterogeneity of the patients’ populations (diagnostic criteria, geographic location, etc …) included in each study, but also in the different probiotic formulations tested (i.e., different preparation and storage, different strains or combination of strains, contained in each formulation, and so on). A recent meta-analysis identifying 82 clinical trials investigating the use of probiotics in IBS determined only 24 to have a relatively low risk of bias using the Cochrane risk of bias tool.Citation283 Of note, certain probiotic strains have been recommended to treat global IBS symptoms in children in Italy.Citation284 Probiotics might affect central symptoms in DGBI patients rather than directly improve GI symptoms, as evidenced by few studies in both patients and animal models.Citation285–287 However, to comprehensively grasp the potential benefits, both at the central and peripheral, of probiotic administration for patients with DGBIs, future large-scale and well-designed randomized controlled clinical trials (RCTs) are necessary.
Another microbiome-directed approach that has engaged a considerable amount of recent research is the fecal microbiota transplantation (FMT), a process that involves the transfer of stool from a healthy donor to a recipient via colonoscopy, nasogastric tube, colonic enema, or capsules; a well-known and highly effective treatment for recurrent Clostridioides difficile gastrointestinal infections.Citation288 Given the evidence highlighting a central role for the gut microbiota in DGBI pathophysiology, FMT has been proposed as a treatment strategy for IBS. A recently published randomized placebo-controlled trial on patients with treatment-refractory IBS reported an improvement of symptoms when receiving FMT compared to placebo. In this study, patients were followed for several months and the observed beneficial effects of FMT decreased over 1 year, while a second FMT led to a restoration of the effects.Citation289 In contrast, long-term efficacy has been reported at 2 and 3 years after a single FMT, with better outcomes, including fewer IBS symptoms and fatigue, and without long-term adverse effects.Citation290 However, a recent meta-analysis on the efficacy of FMT in IBS reported no overall advantage of FMT over placebo, although delivery to the small intestine (vs. colon or capsules) appeared to be effective.Citation291 Indeed, the authors stressed the need for well-designed RCTs and long-term follow-up registries to ensure the efficacy and safety of FMT. The same conclusions were also reached in another meta-analysis published in 2023 highlighting the overall low quality of evidence for FMT in IBS.Citation292 Repeated delivery of FMT to the small intestine appears to yield prolonged relief from symptoms and an enhancement in quality of life, as highlighted by a recent study.Citation293 While there appears to be a future for FMT in treating IBS, the specific mechanisms responsible for its effectiveness remain unclear. In addition to considering the microbiomes of both the patient and the donor, other variables such as the patient’s clinical characteristics, diverse administration routes, and the quantity of fecal material administered play a role in FMT clinical outcomes. Recently, the idea that strain engraftment may be correlated with clinical improvement post-FMT has been introduced as an additional factor influencing the success of FMT in IBS and other disorders.Citation294
Besides microbiome-directed therapies, the field has also seen a rise in therapies aimed at addressing the central dysfunction and psychological comorbidities of DGBI. Assessing the efficacy of over 40 eligible randomized controlled trials undergoing a form of psychological intervention for IBS, Black et al. identified evidence supporting the efficacy of cognitive behavioral therapy (CBT) and gut-directed hypnotherapy.Citation295 Indeed, CBT improves IBS symptomsCitation296 and its response has been associated with baseline intestinal microbiota and serotonin levels.Citation297 These data provide further evidence that not only microbial signals can modulate central processes involved in symptom generation and sensation in IBS, but also that, in a subset of patients, the brain exerts a large influence on the gut microbiome. Therapies focusing on mind–body interactions and stress reduction may also be integrated into the management of DGBI.Citation298 For example, in-person and virtual yoga practices have been reported to be effective at reducing symptoms as well as decreasing anxiety, depression, and stress in IBS patients.Citation299–307 While psychiatric influence on the gut microbiome has been moderately investigated, there is limited evidence on the effect of yoga and meditation practices on gut microbial composition and function in DGBI.
Over the last few years, therapeutic virtual reality (VR) technology has become a topic of discussion for effective DGBI symptomatic relief, so much so that it has recently become an FDA-recognized area of medicine known as “medical extended reality”.Citation308 Using an electronic headset over the eyes, patients immerse their mind into a virtual world that acts as a distraction from noxious peripheral stimuli, creating a therapeutic illusion.Citation308 Interestingly, VR has been shown to be effective in treating psychological disorders such as anxiety and depression, which are frequently comorbid with DGBIs and exacerbate symptoms such as abdominal pain.Citation308 While VR seemed to be effective for FD,Citation309 it has yet to be tested as a treatment for IBS.Citation310 Further studies investigating not only the therapeutic benefits of VR on DGBI as well as the impact of this form of therapy on the microbiome are needed.
Finally, given the importance of the vagus nerve in microbiome-gut-brain communication, the use of vagus nerve stimulation (VNS) as a potential therapeutic intervention for DGBI, particularly for dysmotility, inflammation, and pain, has prompted interest.Citation311,Citation312 VNS has been approved by the US FDA for epilepsy and pharmaco-resistant depression, but due to its invasive nature, has not been approved for any gastrointestinal disease. However, noninvasive VNS techniques have been recently used to treat abdominal pain and constipation. A recent RCT testing the efficacy of transcutaneous auricular VNS in patients with IBS-C found a significant reduction in abdominal pain, constipation, pro-inflammatory cytokines, and serotonin production, as well as a significant improvement in quality of life.Citation312 The results of this study prompted the hypothesis that auricular VNS decreases inflammation by activating the cholinergic anti-inflammatory pathway and, in turn, alleviating visceral pain.Citation312,Citation313 This hypothesis is supported by a recent animal study reporting that VNS improves visceral sensitivity, depression, and inflammatory mediators via the a7nAChR-mediated inflammatory pathway in a mouse model of IBS.Citation314 Likewise, auricular percutaneous electrical nerve field stimulation (aPENFS), believed to target the vagus nerve, has demonstrated encouraging outcomes in mitigating visceral sensitivity and abdominal pain in three clinical trials involving adolescent IBS patients.Citation315–317 Additionally, although one of these trials did not observe a strong microbiome change in pre- versus post-therapy, or between responders and non-responders, the authors showed a higher abundance of Blautia in responders compared to non-responders.Citation316
These data substantiate the involvement of gut-brain communication through autonomic function and vagus nerve activity in DGBI, underlining the importance of initiating new clinical trials in adult populations with DGBI that comprehensively evaluate all aspects of these disorders, encompassing gut symptoms, psychological comorbidities, and gut microbiome composition and function. Taken together, these discoveries reiterate the significant heterogeneity among DGBI patients concerning both clinical manifestations and responses to treatment.
Conclusions
The growing body of literature on the microbiota-gut-brain axis and DGBI is aiding our understanding of the mechanisms underlying DGBI pathophysiology. While the pathways involved in the communication between the intestinal microbiota or specific bacterial strains, the gut, and the brain in the context of DGBI are still not fully understood, recent evidence has demonstrated different microbes and their metabolites to play a crucial role in a variety of patient subsets, driving specific symptoms such as abdominal pain or dysmotility. Large-scale longitudinal multi-omics trials, combining the study of both host and microbiome, with a focus on microbiome function, coupled with translational studies, appear to be the recommended approach to generate findings that will help understand specific mechanisms driving symptoms in subsets of patients. These studies will aid the development of new microbiome-targeted diagnostic techniques and therapies, aimed at streamlining patients’ management and treatment, and reduce the overall socioeconomic impact of these challenging disorders.
Disclosure statement
No potential conflict of interest was reported by the author(s).
Additional information
Funding
References
- Sperber AD, Bangdiwala SI, Drossman DA, Ghoshal UC, Simren M, Tack J, Whitehead WE, Dumitrascu DL, Fang X, Fukudo S. et al. Worldwide prevalence and burden of functional gastrointestinal disorders, results of Rome Foundation global study. Gastroenterology. 2021;160(1):99–114.e3. doi:10.1053/j.gastro.2020.04.014.
- Drossman DA, Hasler WL. Rome IV—functional GI disorders: disorders of gut-brain interaction. Gastroenterology. 2016;150(6):1257–28. doi:10.1053/j.gastro.2016.03.035.
- Drossman DA. Functional gastrointestinal disorders: history, pathophysiology, clinical features, and Rome IV. Gastroenterology. 2016;150(6):1262–1279.e2. doi:10.1053/j.gastro.2016.02.032.
- Goodoory VC, Guthrie EA, Ng CE, Black CJ, Ford AC. Factors associated with lower disease-specific and generic health-related quality of life in Rome IV irritable bowel syndrome. Aliment Pharmacol Ther. 2023;57(3):323–334. doi:10.1111/apt.17356.
- Black CJ, Ng CE, Goodoory VC, Ford AC. Novel symptom subgroups in individuals with irritable bowel syndrome predict disease impact and burden. Clin Gastroenterol Hepatol. 2023;22(2):386–396. doi:10.1016/j.cgh.2023.02.016.
- Knowles SR, Skvarc D, Ford AC, Palsson OS, Bangdiwala SI, Sperber AD, Mikocka-Walus A. Negative impact of disorders of gut-brain interaction on health-related quality of life: results from the Rome foundation global epidemiology survey. Gastroenterology. 2023;164(4):655–668. doi:10.1053/j.gastro.2022.12.009.
- Drossman DA. The functional gastrointestinal disorders and the Rome III process. Gastroenterology. 2006;130(5):1377–1390. doi:10.1053/j.gastro.2006.03.008.
- Yan L, Zhang X, Li Y, Liu C, Yang H, Yang C. The role of psychological factors in functional gastrointestinal disorders: a systematic review and meta-analysis. Int J Colorectal Dis. 2023;38(1):38. doi:10.1007/s00384-023-04333-9.
- Mayer EA, Ryu HJ, Bhatt RR. The neurobiology of irritable bowel syndrome. Mol Psychiatry. 2023;28(4):1451–1465. doi:10.1038/s41380-023-01972-w.
- Collins SM, Surette M, Bercik P. The interplay between the intestinal microbiota and the brain. Nat Rev Microbiol. 2012;10(11):735–742. doi:10.1038/nrmicro2876.
- Mayer EA, Tillisch K, Gupta A. Gut/brain axis and the microbiota. J Clin Invest. 2015;125(3):926–938. doi:10.1172/JCI76304.
- Rajilić-Stojanović M, Biagi E, Heilig HGHJ, Kajander K, Kekkonen RA, Tims S, De Vos WM. Global and deep molecular analysis of microbiota signatures in fecal samples from patients with irritable bowel syndrome. Gastroenterology. 2011;141(5):1792–1801. doi:10.1053/j.gastro.2011.07.043.
- Simreń M, Barbara G, Flint HJ, Spiegel BMR, Spiller RC, Vanner S, Verdu EF, Whorwell PJ, Zoetendal EG. Intestinal microbiota in functional bowel disorders: a Rome foundation report. Gut. 2013;62(1):159–176. doi:10.1136/gutjnl-2012-302167.
- Mars RAT, Yang Y, Ward T, Houtti M, Priya S, Lekatz HR, Tang X, Sun Z, Kalari KR, Korem T. et al. Longitudinal multi-omics reveals subset-specific mechanisms underlying irritable bowel syndrome. Cell. 2020;182(6):1460–1473. doi:10.1016/j.cell.2020.08.007.
- Wernroth ML, Peura S, Hedman AM, Hetty S, Vicenzi S, Kennedy B, Fall K, Svennblad B, Andolf E, Pershagen G. et al. Development of gut microbiota during the first 2 years of life. Sci Rep. 2022;12(1):1–13. doi:10.1038/s41598-022-13009-3.
- Yatsunenko T, Rey FE, Manary MJ, Trehan I, Dominguez-Bello MG, Contreras M, Magris M, Hidalgo G, Baldassano RN, Anokhin AP. et al. Human gut microbiome viewed across age and geography. Nature. 2012;486(7402):222–227. doi:10.1038/nature11053.
- Walker AW, Hoyles L. Human microbiome myths and misconceptions. Nat Microbiol. 2023;8(8):1392–1396. doi:10.1038/s41564-023-01426-7.
- Goodrich JK, Waters JL, Poole AC, Sutter JL, Koren O, Blekhman R, Beaumont M, Van Treuren W, Knight R, Bell JT. et al. Human genetics shape the gut microbiome. Cell. 2014;159(4):789–799. doi:10.1016/j.cell.2014.09.053.
- Farré-Maduell E, Casals-Pascual C. The origins of gut microbiome research in Europe: from Escherich to Nissle. Hum Microb J. 2019;14:14. doi:10.1016/j.humic.2019.100065.
- Escherich T. Die darmbakterien des säuglings und ihre beziehungen zur physiologie der Verdauung. Stuttgart: F. Enke; 1886.
- Escherich T. Die Darmbakterien des Neugeborenen und Sauglings. Fortschr Med. 1885;3(16 und 17):515–554.
- Metchnikoff E. La Vieillesse. Rev Sci. 1904;2:65–70, 100–5.
- Tissier H, Gasching P. Recherches sur la fermentation du lait. Ann l’Institut Pasteur. 1903;8:540–563.
- Tissier H. Étude d’une varieté d’infection intestinale chez le nourrisson. Ann l’Institut Pasteur. 1905;1:273–316.
- Nissle A, Nissle A. Über die Grundlagen einer neuen ursächlichen Bekämpfung der pathologischen Darmflora. Deutsche Medizinische Wochenschrift. 1916;42:1181–1184.
- Anitha M, Vijay-Kumar M, Sitaraman SV, Gewirtz AT, Srinivasan S. Gut microbial products regulate murine gastrointestinal motility via toll-like receptor 4 signaling. Gastroenterology. 2012;143(4):1006–1016. doi:10.1053/j.gastro.2012.06.034.
- De Vadder F, Grasset E, Mannerås Holm L, Karsenty G, Macpherson AJ, Olofsson LE, Bäckhed F. Gut microbiota regulates maturation of the adult enteric nervous system via enteric serotonin networks. Proc Natl Acad Sci U S A. 2018;115(25):6458–6463. doi:10.1073/pnas.1720017115.
- Yarandi SS, Kulkarni S, Saha M, Sylvia KE, Sears CL, Pasricha PJ. Intestinal bacteria maintain adult enteric nervous system and nitrergic neurons via toll-like receptor 2-induced neurogenesis in mice. Gastroenterology. 2020;159(1):200–213. doi:10.1053/j.gastro.2020.03.050.
- Obata Y, Castaño Á, Boeing S, Bon-Frauches AC, Fung C, Fallesen T, de Agüero MG, Yilmaz B, Lopes R, Huseynova A. et al. Neuronal programming by microbiota regulates intestinal physiology. Nature. 2020;578(7794):284–289. doi:10.1038/s41586-020-1975-8.
- Hapfelmeier S, A E LM, Slack E, Kirundi JK, Stoel M, Heikenwalder M, Cahenzli J, Velykoredko Y, Balmer ML, Endt K. et al. Reversible microbial colonization of germ-free mice reveals the dynamics of IgA immune responses. Science. 2010;328(5986):1705–1709. doi:10.1126/science.1188454.
- Olszak T, An D, Zeissig S, Vera MP, Richter J, Franke A, Glickman JN, Siebert R, Baron RM, Kasper DL. et al. Microbial exposure during early life has persistent effects on natural killer T cell function. Science (1979). 2012;336(6080):489–493. doi:10.1126/science.1219328.
- Slack E, Hapfelmeier S, Stecher B, Velykoredko Y, Stoel M, Lawson MAE, Geuking MB, Beutler B, Tedder TF, Hardt WD. et al. Innate and adaptive immunity cooperate flexibly to maintain host-microbiota mutualism. Science (1979). 2009;325(5940):617–620. doi:10.1126/science.1172747.
- Geuking MB, Cahenzli J, Lawson MAE, Dck N, Slack E, Hapfelmeier S, Kd M, Macpherson AJ. Intestinal bacterial colonization induces mutualistic regulatory T cell responses. Immunity. 2011;34(5):794–806. doi:10.1016/j.immuni.2011.03.021.
- Collins J, Borojevic R, Verdu EF, Huizinga JD, Ratcliffe EM. Intestinal microbiota influence the early postnatal development of the enteric nervous system. Neurogastroent Motil. 2014;26(1):98–107. doi:10.1111/nmo.12236.
- Li C, Stražar M, Mohamed AMT, Pacheco JA, Walker RL, Lebar T, et al. Gut microbiome and metabolome profiling in Framingham heart study reveals cholesterol-metabolizing bacteria. Cell 2024; 187:1834-52.e19. doi:10.1016/j.cell.2024.03.014.
- Pellanda P, Ghosh TS, O’Toole PW. Understanding the impact of age-related changes in the gut microbiome on chronic diseases and the prospect of elderly-specific dietary interventions. Curr Opin Biotechnol. 2021;70:48–55. doi:10.1016/j.copbio.2020.11.001.
- Cӑtoi AF, Corina A, Katsiki N, Vodnar DC, Andreicuț AD, Stoian AP, Rizzo M, Pérez-Martínez P. Gut microbiota and aging – a focus on centenarians. Biochim Biophys Acta Mol Basis Dis. 2020;1866(7):165765. doi:10.1016/j.bbadis.2020.165765.
- Bai X, De Palma G, Boschetti E, Nishihara Y, Lu J. Vasoactive intestinal polypeptide plays a key role in the microbial-neuroimmune control of intestinal motility. Cell Mol Gastroenterol Hepatol. 2023;17(3):383–398. doi:10.1016/j.jcmgh.2023.11.012.
- Luczynski P, Tramullas M, Viola M, Shanahan F, Clarke G, Mahony SO, Dinan TG, Cryan JF. Microbiota regulates visceral pain in the mouse. Elife. 2017;6:1–21. doi:10.7554/eLife.25887.
- Esquerre N, Basso L, Defaye M, Vicentini FA, Cluny N, Bihan D, Hirota SA, Schick A, Jijon HB, Lewis IA. et al. Colitis-induced microbial perturbation promotes postinflammatory visceral hypersensitivity. Cmgh. 2020;10(2):225–244. doi:10.1016/j.jcmgh.2020.04.003.
- Gao J, Xiong T, Grabauskas G, Owyang C. Mucosal serotonin reuptake transporter expression in irritable bowel syndrome is modulated by gut microbiota via mast cell–prostaglandin E2. Gastroenterology. 2022;162(7):1962–1974. doi:10.1053/j.gastro.2022.02.016.
- Lucarini E, Di Pilato V, Parisio C, Micheli L, Toti A, Pacini A, Bartolucci G, Baldi S, Niccolai E, Amedei A. et al. Visceral sensitivity modulation by faecal microbiota transplantation: the active role of gut bacteria in pain persistence. Pain. 2022;163(5):861–877. doi:10.1097/j.pain.0000000000002438.
- Amaral FA, Sachs D, Costa VV, Fagundes CT, Cisalpino D, Cunha TM, Ferreira SH, Cunha FQ, Silva TA, Nicoli JR. et al. Commensal microbiota is fundamental for the development of inflammatory pain. Proc Natl Acad Sci U S A. 2008;105(6):2193–2197. doi:10.1073/pnas.0711891105.
- Shen S, Lim G, You Z, DIng W, Huang P, Ran C, Doheny J, Caravan P, Tate S, Hu K. et al. Gut microbiota is critical for the induction of chemotherapy-induced pain. Nat Neurosci. 2017;20(9):1213–1216. doi:10.1038/nn.4606.
- Chiu IM, Heesters BA, Ghasemlou N, Von Hehn CA, Zhao F, Tran J, Wainger B, Strominger A, Muralidharan S, Horswill AR. et al. Bacteria activate sensory neurons that modulate pain and inflammation. Nature. 2013;501(7465):52–57. doi:10.1038/nature12479.
- Pujo J, De Palma G, Lu J, Galipeau HJ, Surette MG, Collins SM, Bercik P. Gut microbiota modulates visceral sensitivity through calcitonin gene-related peptide (CGRP) production. Gut Microbes. 2023;15(1):2188874. doi:10.1080/19490976.2023.2188874.
- Lin X, Hu T, Chen J, Liang H, Zhou J, Wu Z, Ye C, Jin X, Xu X, Zhang W. et al. The genomic landscape of reference genomes of cultivated human gut bacteria. Nat Commun. 2023;14(1):1–11. doi:10.1038/s41467-023-37396-x.
- Siegwald L, Audebert C, Even G, Viscogliosi E, Caboche S, Chabé M. Targeted metagenomic sequencing data of human gut microbiota associated with Blastocystis colonization. Sci Data. 2017;4(1):1–7. doi:10.1038/sdata.2017.81.
- Rehan M, Al-Bahadly I, Thomas DG, Young W, Cheng LK, Avci E. Smart capsules for sensing and sampling the gut: status, challenges and prospects. Gut. 2023;73(1):186–202. doi:10.1136/gutjnl-2023-329614.
- Shalon D, Culver RN, Grembi JA, Folz J, Treit PV, Shi H, Rosenberger FA, Dethlefsen L, Meng X, Yaffe E. et al. Profiling the human intestinal environment under physiological conditions. Nature. 2023;617(7961):581–591. doi:10.1038/s41586-023-05989-7.
- Asnicar F, Berry SE, Valdes AM, Nguyen LH, Piccinno G, Drew DA, Leeming E, Gibson R, Le Roy C, Khatib HA. et al. Microbiome connections with host metabolism and habitual diet from 1,098 deeply phenotyped individuals. Nat Med. 2021;27(2):321–332. doi:10.1038/s41591-020-01183-8.
- Kolodziejczyk AA, Zheng D, Elinav E. Diet–microbiota interactions and personalized nutrition. Nat Rev Microbiol. 2019;17(12):742–753. doi:10.1038/s41579-019-0256-8.
- Zmora N, Suez J, Elinav E. You are what you eat: diet, health and the gut microbiota. Nat Rev Gastroenterol Hepatol. 2019;16(1):35–56. doi:10.1038/s41575-018-0061-2.
- Caugant DA, Levin BR, Selander RK. Distribution of multilocus genotypes of Escherichia coli within and between host families. Epidemiol Infect. 1984;92(3):377–384. doi:10.1017/S0022172400064597.
- Kates AE, Jarrett O, Skarlupka JH, Sethi A, Duster M, Watson L, Suen G, Poulsen K, Safdar N. Household pet ownership and the microbial diversity of the human gut microbiota. Front Cell Infect Microbiol. 2020;10:73. doi:10.3389/fcimb.2020.00073.
- Tun HM, Konya T, Takaro TK, Brook JR, Chari R, Field CJ, Guttman DS, Becker AB, Mandhane PJ, Turvey SE. et al. Exposure to household furry pets influences the gut microbiota of infants at 3–4 months following various birth scenarios. Microbiome. 2017;5(1):1–14. doi:10.1186/s40168-017-0254-x.
- Bajaj JS. Alcohol, liver disease and the gut microbiota. Nat Rev Gastroenterol Hepatol. 2019;16(4):235–246. doi:10.1038/s41575-018-0099-1.
- Fluhr L, Mor U, Kolodziejczyk AA, Dori-Bachash M, Leshem A, Itav S, Cohen Y, Suez J, Zmora N, Moresi C. et al. Gut microbiota modulates weight gain in mice after discontinued smoke exposure. Nature. 2021;600(7890):713–719. doi:10.1038/s41586-021-04194-8.
- Sarkar A, McInroy CJA, Harty S, Raulo A, Ibata NGO, Valles-Colomer M, Johnson K-A, Brito IL, Henrich J, Archie EA. et al. Microbial transmission in the social microbiome and host health and disease. Cell. 2024;187(1):17–43. doi:10.1016/j.cell.2023.12.014.
- Wolf S. The psyche and the stomach. A historical vignette. Gastroenterology. 1981;80(3):605–614. doi:10.1016/0016-5085(81)90027-5.
- Kleisiaris CF, Sfakianakis C, Papathanasiou IV. Health care practices in ancient Greece: the hippocratic ideal. J Med Ethics Hist Med. 2014;7:3–7.
- Bercik P, Collins SM, Verdu EF. Microbes and the gut-brain axis. Neurogastroent Motil. 2012;24(5):405–413. doi:10.1111/j.1365-2982.2012.01906.x.
- Buchanan KL, Rupprecht LE, Kaelberer MM, Sahasrabudhe A, Klein ME, Villalobos JA, Liu WW, Yang A, Gelman J, Park S. et al. The preference for sugar over sweetener depends on a gut sensor cell. Nat Neurosci. 2022;25(2):191–200. doi:10.1038/s41593-021-00982-7.
- Han H, Yi B, Zhong R, Wang M, Zhang S, Ma J, Yin Y, Yin J, Chen L, Zhang H. From gut microbiota to host appetite: gut microbiota-derived metabolites as key regulators. Microbiome. 2021;9(1):1–16. doi:10.1186/s40168-021-01093-y.
- Rogers GB, Keating DJ, Young RL, Wong M, Licinio J, Wesselingh S. From gut dysbiosis to altered brain function and mental illness: mechanisms and pathways. Mol Psychiatry. 2016;21(6):738–748. doi:10.1038/mp.2016.50.
- Steenbergen L, Sellaro R, Van HS, Bosch JA, Colzato LS. A randomized controlled trial to test the effect of multispecies probiotics on cognitive reactivity to sad mood q. Brain Behav Immun. 2015;48:258–264. doi:10.1016/j.bbi.2015.04.003.
- Bagga D, Reichert JL, Koschutnig K, Aigner CS, Holzer P, Koskinen K, Moissl-Eichinger C, Schöpf V. Probiotics drive gut microbiome triggering emotional brain signatures. Gut Microbes. 2018;0:1–11. doi:10.1080/19490976.2018.1460015.
- Johnson KVA, Foster KR. Why does the microbiome affect behaviour? Nat Rev Microbiol. 2018;16(10):647–655. doi:10.1038/s41579-018-0014-3.
- Breit S, Kupferberg A, Rogler G, Hasler G. Vagus nerve as modulator of the brain-gut axis in psychiatric and inflammatory disorders. Front Psychiatry. 2018;9. doi:10.3389/fpsyt.2018.00044.
- Price CJ, Hoyda TD, Ferguson AV. The area postrema: a brain monitor and integrator of systemic autonomic state. Neuroscientist. 2008;14(2):182–194. doi:10.1177/1073858407311100.
- Liu WW, Bohórquez DV. The neural basis of sugar preference. Nat Rev Neurosci. 2022;23(10):584–595. doi:10.1038/s41583-022-00613-5.
- Kaelberer MM, Buchanan KL, Klein ME, Barth BB, Montoya MM, Shen X, Bohórquez DV. A gut-brain neural circuit for nutrient sensory transduction. Science (1979). 2018;361(6408):361. doi:10.1126/science.aat5236.
- Han W, Tellez LA, Perkins MH, Perez IO, Qu T, Ferreira J, Ferreira TL, Quinn D, Liu ZW, Gao XB. et al. A Neural Circuit for Gut-Induced Reward. Cell. 2018;175(3):665–678.
- Arentsen T, Qian Y, Gkotzis S, Femenia T, Wang T, Udekwu K, Forssberg H, Heijtz RD. The bacterial peptidoglycan-sensing molecule Pglyrp2 modulates brain development and behavior. Mol Psychiatry. 2017;22(2):257–266. doi:10.1038/mp.2016.182.
- Ochoa-Repáraz J, Mielcarz DW, Wang Y, Begum-Haque S, Dasgupta S, Kasper DL, Kasper LH. A polysaccharide from the human commensal Bacteroides fragilis protects against CNS demyelinating disease. Mucosal Immunol. 2010;3(5):487–495. doi:10.1038/mi.2010.29.
- Quan N, Stern EL, Whiteside MB, Herkenham M. Induction of pro-inflammatory cytokine mRnas in the brain after peripheral injection of subseptic doses of lipopolysaccharide in the rat. J Neuroimmunol. 1999;93(1–2):72–80. doi:10.1016/S0165-5728(98)00193-3.
- Breder CD, Hazuka C, Ghayurt T, Klug C, Huginint M, Yasuda K, Tengii M, Saper CB. Regional induction of tumor necrosis factor alpha expression in the mouse brain after systemic lipopolysaccharide administration. Proc Natl Acad Sci USA. 1994;91(24):11393–11397. doi:10.1073/pnas.91.24.11393.
- Elmquist JK, Scammell TE, Jacobson CD, Saper CB. Distribution of Fos-like immunoreactivity in the rat brain following intravenous lipopolysaccharide administration. J Comp Neurol. 1996;371(1):85–103. doi:10.1002/(SICI)1096-9861(19960715)371:1<85:AID-CNE5>3.0.CO;2-H.
- Needham BD, Funabashi M, Adame MD, Wang Z, Boktor JC, Haney J, Wu WL, Rabut C, Ladinsky MS, Hwang SJ. et al. A gut-derived metabolite alters brain activity and anxiety behaviour in mice. Nature. 2022;602(7898):647–653. doi:10.1038/s41586-022-04396-8.
- Stewart Campbell A, Needham BD, Meyer CR, Tan J, Conrad M, Preston GM, Bolognani F, Rao SG, Heussler H, Griffith R. et al. Safety and target engagement of an oral small-molecule sequestrant in adolescents with autism spectrum disorder: an open-label phase 1b/2a trial. Nat Med. 2022;28(3):528–534. doi:10.1038/s41591-022-01683-9.
- Xia Y, Xiao Y, Wang ZH, Liu X, Alam AM, Haran JP, McCormick BA, Shu X, Wang X, Ye K. Bacteroides Fragilis in the gut microbiomes of Alzheimer’s disease activates microglia and triggers pathogenesis in neuronal C/EBPβ transgenic mice. Nat Commun. 2023;14(1):1–20. doi:10.1038/s41467-023-41283-w.
- Hsiao EY, McBride SW, Hsien S, Sharon G, Hyde ER, McCue T, Codelli JA, Chow J, Reisman SE, Petrosino JF. et al. Microbiota modulate behavioral and physiological abnormalities associated with neurodevelopmental disorders. Cell. 2013;155(7):1451–1463. doi:10.1016/j.cell.2013.11.024.
- Wuchert F, Ott D, Murgott J, Rafalzik S, Hitzel N, Roth J, Gerstberger R. Rat area postrema microglial cells act as sensors for the toll-like receptor-4 agonist lipopolysaccharide. J Neuroimmunol. 2008;204(1–2):66–74. doi:10.1016/j.jneuroim.2008.07.017.
- Laflamme N, Rivest S. Toll‐like receptor 4: the missing link of the cerebral innate immune response triggered by circulating gram‐negative bacterial cell wall components. Faseb J. 2001;15(1):155–163. doi:10.1096/fj.00-0339com.
- Kraimi N, Vivek P, Zhang H, Lu J, De Palma G, Shimbori C, Verdu EF, McCoy KD, Hapfelmeier S, Macpherson AJ. et al. Microbial activation of intestinal dendritic cells is critical for the establishment of normal behavior. J Canadian Assoc Gastroenterol. 2023;6(Suppl Supplement_1):4–5. doi:10.1093/jcag/gwac036.008.
- Dantzer R, Bluthé RM, Gheusi G, Cremona S, Layé S, Parnet P, Kelley KW. Molecular basis of sickness behavior a. Ann N Y Acad Sci. 1998;856(1):132–138. doi:10.1111/j.1749-6632.1998.tb08321.x.
- Elmquist JK, Scammell TE, Saper CB. Mechanisms of CNS response to systemic immune challenge: the febrile response. Trends Neurosci. 1997;20(12):565–570. doi:10.1016/S0166-2236(97)01138-7.
- Gaykema RPA, Goehler LE, Lyte M. Brain response to cecal infection with Campylobacter jejuni: analysis with Fos immunohistochemistry. Brain Behav Immun. 2004;18(3):238–245. doi:10.1016/j.bbi.2003.08.002.
- Goehler LE, Gaykema RPA, Opitz N, Reddaway R, Badr N, Lyte M. Activation in vagal afferents and central autonomic pathways: early responses to intestinal infection with Campylobacter jejuni. Brain Behav Immun. 2005;19(4):334–344. doi:10.1016/j.bbi.2004.09.002.
- Osterhout JA, Kapoor V, Eichhorn SW, Vaughn E, Moore JD, Liu D, Lee D, DeNardo LA, Luo L, Zhuang X. et al. A preoptic neuronal population controls fever and appetite during sickness. Nature. 2022;606(7916):937–944. doi:10.1038/s41586-022-04793-z.
- Ilanges A, Shiao R, Shaked J, Luo JD, Yu X, Friedman JM. Brainstem ADCYAP1+ neurons control multiple aspects of sickness behaviour. Nature. 2022;609(7928):761–771. doi:10.1038/s41586-022-05161-7.
- Pinho-Ribeiro FA, Deng L, Neel DV, Erdogan O, Basu H, Yang D, Choi S, Walker AJ, Carneiro-Nascimento S, He K. et al. Bacteria hijack a meningeal neuroimmune axis to facilitate brain invasion. Nature. 2023;615(7952):472–481. doi:10.1038/s41586-023-05753-x.
- Servick K. Do gut bacteria make a second home in our brains. Science (1979). 2018;10:92. doi:10.1126/science.aaw0147.
- Chaudhuri D, Chowdhury AR, Biswas B, Chakravortty D. Salmonella Typhimurium infection leads to colonization of the mouse brain and is not completely cured with antibiotics. Front Microbiol. 2018;9:1–12. doi:10.3389/fmicb.2018.01632.
- Arabi TZ, Alabdulqader AA, Sabbah BN, Ouban A. Brain-inhabiting bacteria and neurodegenerative diseases: the “brain microbiome” theory. Front Aging Neurosci. 2023;15:1–9. doi:10.3389/fnagi.2023.1240945.
- Peh A, Dinakis E, Nakai M, Muralitharan RR, Rupasinghe S, Wilson JL, Wong CHY, Jama H, Barker CMO, Modarresi M. et al. Gut bacteria translocation to the brain after ischaemic stroke occurs via the sympathetic nervous system. bioRxiv. 2023. doi:10.1101/2023.04.03.535309.
- Thapa M, Kumari A, Chin C-Y, Choby JE, Jin F, Bogati B, Chopyk DM, Koduri N, Pahnke A, Elrod EJ. et al. Translocation of gut commensal bacteria to the brain. bioRxiv. 2023. doi:10.1101/2023.08.30.555630.
- Hu X, Mckenzie C-A, Smith C, Haas JG, Lathe R. The remarkable complexity of the brain microbiome in health and disease. bioRxiv. 2023;2023–02. doi:10.1101/2023.02.06.527297.
- Kennedy KM, de Goffau MC, Perez-Muñoz ME, Arrieta MC, Bäckhed F, Bork P, Braun T, Bushman FD, Dore J, de Vos WM. et al. Questioning the fetal microbiome illustrates pitfalls of low-biomass microbial studies. Nature. 2023;613(7945):639–649. doi:10.1038/s41586-022-05546-8.
- Pérez-Berezo T, Pujo J, Martin P, Le Faouder P, Galano JM, Guy A, Knauf C, Tabet JC, Tronnet S, Barreau F. et al. Identification of an analgesic lipopeptide produced by the probiotic Escherichia coli strain Nissle 1917. Nat Commun. 2017;8(1):1–12. doi:10.1038/s41467-017-01403-9.
- Lenard J. Mammalian hormones in microbial cells. Trends Biochem Sci. 1992;17(4):147–150. doi:10.1016/0968-0004(92)90323-2.
- Minuk GY. Gamma-aminobutyric acid (GABA) production by eight common bacterial pathogens. Scand J Infect Dis. 1986;18(5):465–467. doi:10.3109/00365548609032366.
- O’Donnell MP, Fox BW, Chao PH, Schroeder FC, Sengupta P. A neurotransmitter produced by gut bacteria modulates host sensory behaviour. Nature. 2020;583(7816):415–420. doi:10.1038/s41586-020-2395-5.
- Asano Y, Hiramoto T, Nishino R, Aiba Y, Kimura T, Yoshihara K, Koga Y, Sudo N. Critical role of gut microbiota in the production of biologically active, free catecholamines in the gut lumen of mice. Am J Physiol Gastrointest Liver Physiol. 2012;303(11):G1288–G1295. doi:10.1152/ajpgi.00341.2012.
- Li D, Liu R, Wang M, Peng R, Fu S, Fu A, Le J, Yao Q, Yuan T, Chi H. et al. 3β-Hydroxysteroid dehydrogenase expressed by gut microbes degrades testosterone and is linked to depression in males. Cell Host Microbe. 2022;30(3):329–339. doi:10.1016/j.chom.2022.01.001.
- Zimmermann M, Zimmermann-Kogadeeva M, Wegmann R, Goodman AL. Mapping human microbiome drug metabolism by gut bacteria and their genes. Nature. 2019;570(7762):462–467. doi:10.1038/s41586-019-1291-3.
- Zimmermann M, Zimmermann-Kogadeeva M, Wegmann R, Goodman AL. Separating host and microbiome contributions to drug pharmacokinetics and toxicity. Science (1979). 2019;363(6427). doi:10.1126/science.aat9931.
- Klünemann M, Andrejev S, Blasche S, Mateus A, Phapale P, Devendran S, Vappiani J, Simon B, Scott TA, Kafkia E. et al. Bioaccumulation of therapeutic drugs by human gut bacteria. Nature. 2021;597(7877):533–538. doi:10.1038/s41586-021-03891-8.
- van Kessel SP, Frye AK, El-Gendy AO, Castejon M, Keshavarzian A, van Dijk G, El Aidy S, van Kessel SP. Gut bacterial tyrosine decarboxylases restrict levels of levodopa in the treatment of Parkinson’s disease. Nat Commun. 2019;10(1):1–11. doi:10.1038/s41467-019-08294-y.
- Rekdal VM, Bess EN, Bisanz JE, Turnbaugh PJ, Balskus EP. Discovery and inhibition of an interspecies gut bacterial pathway for Levodopa metabolism. Science (1979). 2019;364(6445). doi:10.1126/science.aau6323.
- Mager LF, Burkhard R, Pett N, Cooke NCA, Brown K, Ramay H, Paik S, Stagg J, Groves RA, Gallo M. et al. Microbiome-derived inosine modulates response to checkpoint inhibitor immunotherapy. Science (1979). 2020;369(6510):1481–1489. doi:10.1126/science.abc3421.
- Drossman DA. Abuse, trauma, and GI illness: is there a link. Am J Gastroenterol. 2011;106(1):14–25. doi:10.1038/ajg.2010.453.
- D’Amato M. Genes and functional GI disorders: from casual to causal relationship. Neurogastroent Motil. 2013;25(8):638–649. doi:10.1111/nmo.12173.
- Geeraerts B, Van Oudenhove L, Fischler B, Vandenberghe J, Caenepeel P, Janssens J, Tack J. Influence of abuse history on gastric sensorimotor function in functional dyspepsia. Neurogastroent Motil. 2009;21(1):33–41. doi:10.1111/j.1365-2982.2008.01178.x.
- Villarreal AA, Aberger FJ, Benrud R, Gundrum JD. Use of broad-spectrum antibiotics and the development of irritable bowel syndrome. Wis Med J. 2012;111:17–20.
- Schmulson MJ, Drossman DA. What’s new in Rome IV? Neurogastroent Motil. 2017;29(2):151–163. doi:10.5056/jnm16214.
- Siah KTH, Gong X, Yang XJ, Whitehead WE, Chen M, Hou X, Pratap N, Ghoshal UC, Syam AF, Abdullah M. et al. Rome foundation-asian working team report: Asian functional gastrointestinal disorder symptom clusters. Gut. 2018;67(6):1071–1077. doi:10.1136/gutjnl-2016-312852.
- Holtmann GJ, Talley NJ. Inconsistent symptom clusters for functional gastrointestinal disorders in Asia: is Rome burning? Gut. 2018;67(11):1911–1915. doi:10.1136/gutjnl-2017-314775.
- Hreinsson JP, Törnblom H, Tack J, Drossman DA, Whitehead WE, Bangdiwala SI, Sperber AD, Palsson OS, Simrén M. Factor analysis of the Rome IV criteria for major Disorders of Gut-Brain Interaction (DGBI) globally and across geographical, sex, and age groups. Gastroenterology. 2023;164(7):1211–1222. doi:10.1053/j.gastro.2023.02.033.
- Almario CV, Sharabi E, Chey WD, Lauzon M, Higgins CS, Spiegel BMR. Prevalence and burden of illness of Rome IV irritable bowel syndrome in the United States: results from a nationwide cross-sectional study. Gastroenterology. 2023;165(6):1475–1487. doi:10.1053/j.gastro.2023.08.010.
- Saffouri GB, Shields-Cutler RR, Chen J, Yang Y, Lekatz HR, Hale VL, Cho JM, Battaglioli EJ, Bhattarai Y, Thompson KJ. et al. Small intestinal microbial dysbiosis underlies symptoms associated with functional gastrointestinal disorders. Nat Commun. 2019;10(1):1–11. doi:10.1038/s41467-019-09964-7.
- Brandt LJ, Chey WD, Foxx-Orenstein AE, Quigley EMM, Schiller LR, Schoenfeld PS, Spiegel BM, Talley NJ, Moayyedi P. An evidence-based position statement on the management of irritable bowel syndrome. Am J Gastroenterol. 2008;104:7–10. doi:10.14309/00000434-200901001-00002.
- Simrén M. Targeting the gut microenvironment in IBS to improve symptoms. Nat Rev Gastroenterol Hepatol. 2023;20(2):69–70. doi:10.1038/s41575-022-00718-3.
- Camilleri M, Zhernakova A, Bozzarelli I, D’Amato M. Genetics of irritable bowel syndrome: shifting gear via biobank-scale studies. Nat Rev Gastroenterol Hepatol. 2022;19(11):689–702. doi:10.1038/s41575-022-00662-2.
- Zamfir-Taranu A, Löscher BS, Husein DM, Hoter A, Garcia-Etxebarria K, Etxeberria U, Gayoso L, Mayr G, Nilholm C, Gustafsson RJ. et al. Sucrase-isomaltase genotype and response to a starch-reduced and sucrose-reduced diet in IBS-D patients. Gut. 2023;0:1–2. doi:10.1136/gutjnl-2023-329695.
- Alemany S, Soler-Artigas M, Cabana-Domínguez J, Fakhreddine D, Llonga N, Vilar-Ribó L, Rodríguez-Urrutia A, Palacio J, González-Castro AM, Lobo B. et al. Genome-wide multi-trait analysis of irritable bowel syndrome and related mental conditions identifies 38 new independent variants. J Transl Med. 2023;21(1):21. doi:10.1186/s12967-023-04107-5.
- Tanaka Y, Kanazawa M, Fukudo S, Drossman DA. Biopsychosocial model of irritable bowel syndrome. J Neurogastroenterol Motil. 2011;17(2):131–139. doi:10.5056/jnm.2011.17.2.131.
- Longstreth GF, Thompson WG, Chey WD, Houghton LA, Mearin F, Spiller RC. Functional bowel disorders. Gastroenterology. 2006;130(5):1480–1491. doi:10.1053/j.gastro.2005.11.061.
- Byale A, Lennon RJ, Byale S, Breen-Lyles M, Edwinson AL, Gupta R, Lacy BE, Olson JE, Houghton LA, Grover M. High-dimensional clustering of 4000 irritable bowel syndrome patients reveals seven distinct disease subsets. Clinical Gastroenterology and Hepatology. 2024;22(1):173–184. doi:10.1016/j.cgh.2022.09.019.
- Black CJ, Yiannakou Y, Guthrie EA, West R, Houghton LA, Ford AC. A novel method to classify and subgroup patients with IBS based on gastrointestinal symptoms and psychological profiles. Am J Gastroenterol. 2021;116(2):372–381. doi:10.14309/ajg.0000000000000975.
- Ford AC, Mahadeva S, Carbone MF, Lacy BE, Talley NJ. Functional dyspepsia. Lancet. 2020;396(10263):1689–1702. doi:10.1016/S0140-6736(20)30469-4.
- Mahadeva S, Goh KL. Epidemiology of functional dyspepsia: a global perspective. World J Gastroenterol. 2006;12(17):2661–2666. doi:10.3748/wjg.v12.i17.2661.
- Barberio B, Yiannakou Y, Houghton LA, Black CJ, Savarino EV, Ford AC. Overlap of Rome IV irritable bowel syndrome and functional dyspepsia and effect on natural history: a longitudinal follow-up study. Clin Gastroenterol Hepatol. 2022;20(2):e89–101. doi:10.1016/j.cgh.2021.04.011.
- Sperber AD, Freud T, Aziz I, Palsson OS, Drossman DA, Dumitrascu DL, Fang X, Fukudo S, Ghoshal UC, Kellow J. et al. Greater overlap of Rome IV disorders of gut-brain interactions leads to increased disease severity and poorer quality of life. Clin Gastroenterol Hepatol. 2022;20(5):e945–56. doi:10.1016/j.cgh.2021.05.042.
- Fadgyas-Stanculete M, Buga A-M, Popa-Wagner A, Dumitrascu DL. The relationship between irritable bowel syndrome and psychiatric disorders: from molecular changes to clinical manifestations. J Mol Psychiatry. 2014;2(1):1–7. doi:10.1186/2049-9256-2-4.
- De Palma G, Collins SM, Bercik P. The microbiota-gut-brain axis in functional gastrointestinal disorders. Gut Microbes. 2014;5(3):419–429. doi:10.4161/gmic.29417.
- Jones MP, Dilley JB, Drossman D, Crowell MD. Brain–gut connections in functional GI disorders: anatomic and physiologic relationships. Neurogastroent Motil. 2006;18(2):91–103. doi:10.1111/j.1365-2982.2005.00730.x.
- Trindade IA, Hreinsson JP, Melchior C, Algera JP, Colomier E, Törnblom H, Drossman D, Tack J, Palsson OS, Bangdiwala SI. et al. Global prevalence of psychological distress and comorbidity with disorders of gut-brain interactions. Am J Gastroenterol. 2023;119(1):165–175. doi:10.14309/ajg.0000000000002500.
- Black CJ, Drossman DA, Talley NJ, Ruddy J, Ford AC. Functional gastrointestinal disorders: advances in understanding and management. Lancet. 2020;396(10263):1664–1674. doi:10.1016/S0140-6736(20)32115-2.
- Drossman DA. Psychosocial factors and the disorders of GI function: what is the link? Am J Gastroenterol. 2004;99(2):358–360. doi:10.1111/j.1572-0241.2004.04076.x.
- Van Oudenhove L, Levy RL, Crowell MD, Drossman DA, Halpert AD, Keefer L, Lackner JM, Murphy TB, Naliboff BD. Biopsychosocial aspects of functional gastrointestinal disorders: how central and environmental processes contribute to the development and expression of functional gastrointestinal disorders. Gastroenterology. 2016;150(6):1355–1367. doi:10.1053/j.gastro.2016.02.027.
- Pinto-Sanchez MI, Ford AC, Avila CA, Verdu EF, Collins SM, Morgan D, Moayyedi P, Bercik P. Anxiety and depression increase in a stepwise manner in parallel with multiple FGIDs and symptom severity and frequency. Am J Gastroenterol. 2015;110(7):1038–1048. doi:10.1038/ajg.2015.128.
- Van Oudenhove L, Törnblom H, Störsrud S, Tack J, Simrén M. Depression and somatization are associated with increased postprandial symptoms in patients with irritable bowel syndrome. Gastroenterology. 2016;150(4):866–874. doi:10.1053/j.gastro.2015.11.010.
- Pohl D, Van Oudenhove L, Törnblom H, Le Nevé B, Tack J, Simrén M. Functional dyspepsia and severity of psychologic symptoms associate with postprandial symptoms in patients with irritable bowel syndrome. Clinical Gastroenterology and Hepatology. 2018;16(11):1745–1753. doi:10.1016/j.cgh.2018.04.034.
- Midenfjord I, Polster A, Sjövall H, Törnblom H, Simrén M. Anxiety and depression in irritable bowel syndrome: exploring the interaction with other symptoms and pathophysiology using multivariate analyses. Neurogastroent Motil. 2019;31(8):1–14. doi:10.1111/nmo.13619.
- Kim SY, Choung RS, Lee SK, Choe JW, Jung SW, Hyun JJ, Koo JS, Lee SW, Shin C. Self-reported sleep impairment in functional dyspepsia and irritable bowel syndrome. J Neurogastroenterol Motil. 2018;24(2):280–288. doi:10.5056/jnm17098.
- Zamani M, Alizadeh-Tabari S, Zamani V. Systematic review with meta-analysis: the prevalence of anxiety and depression in patients with irritable bowel syndrome. Aliment Pharmacol Ther. 2019;50(2):132–143. doi:10.1111/apt.15325.
- Fond G, Loundou A, Hamdani N, Boukouaci W, Dargel A, Oliveira J, Roger M, Tamouza R, Leboyer M, Boyer L. Anxiety and depression comorbidities in irritable bowel syndrome (IBS): a systematic review and meta-analysis. Eur Arch Psychiatry Clin Neurosci. 2014;264(8):651–660. doi:10.1007/s00406-014-0502-z.
- Lee C, Doo E, Choi JM, Ho JS, Ryu HS, Lee JY, Oh JH, Park JH, Kim YS. The increased level of depression and anxiety in irritable bowel syndrome patients compared with healthy controls: systematic review and meta-analysis. J Neurogastroenterol Motil. 2017;23(3):349–362. doi:10.5056/jnm16220.
- Goodoory VC, Mikocka-Walus A, Yiannakou Y, Houghton LA, Black CJ, Ford AC. Impact of psychological comorbidity on the prognosis of irritable bowel syndrome. Am J Gastroenterol. 2021;116(7):1485–1494. doi:10.14309/ajg.0000000000001247.
- Lee S, Wu J, Ma YL, Tsang A, Guo WJ, Sung J. Irritable bowel syndrome is strongly associated with generalized anxiety disorder: a community study. Aliment Pharmacol Ther. 2009;30(6):643–651. doi:10.1111/j.1365-2036.2009.04074.x.
- Beh KH, Chuah KH, Mahamad Rappek NA, Mahadeva S, Lin C-Y. The association of body mass index with functional dyspepsia is independent of psychological morbidity: a cross-sectional study. PLOS ONE. 2021;16(1):1–13. doi:10.1371/journal.pone.0245511.
- Mahadeva S, Goh KL. Anxiety, depression and quality of life differences between functional and organic dyspepsia. J Gastroenterol Hepatol (Australia). 2011;26(s3):49–52. doi:10.1111/j.1440-1746.2011.06656.x.
- Waters AM, Schilpzand E, Bell C, Walker LS, Baber K. Functional gastrointestinal symptoms in children with anxiety disorders. J Abnorm Child Psychol. 2013;41(1):151–163. doi:10.1007/s10802-012-9657-0.
- Hosseinzadeh ST, Poorsaadati S, Radkani B, Forootan M. Psychological disorders in patients with chronic constipation. Gastroenterol Hepatol Bed Bench. 2011;4(3):159–163. doi:10.1177/1756283X11401773.
- Liang J, Zhao Y, Xi Y, Xiang C, Yong C, Huo J, Zou H, Hou Y, Pan Y, Wu M. et al. Association between depression, anxiety symptoms and gut microbiota in Chinese elderly with functional constipation. Nutrients. 2022;14(23):14. doi:10.3390/nu14235013.
- Sangari RS, Hashemi M, Salehi B, Yousefichaijan P, Rafiei M, Rezagholizamenjany M. Anxiety disorders in children with functional constipation: a case-control study. J Compr Pediatr. 2022;13(2). doi:10.5812/compreped.119997.
- Bharucha AE, Mechanisms LB. Evaluation, and management of chronic constipation. Gastroenterology. 2020;158(5):1232–1249. doi:10.1053/j.gastro.2019.12.034.
- Eijsbouts C, Zheng T, Kennedy NA, Bonfiglio F, Anderson CA, Moutsianas L, Holliday J, Shi J, Shringarpure S, Agee M. et al. Genome-wide analysis of 53,400 people with irritable bowel syndrome highlights shared genetic pathways with mood and anxiety disorders. Nat Genet. 2021;53(11):1543–1552. doi:10.1038/s41588-021-00950-8.
- Sykes MA, Blanchard EB, Lackner J, Keefer L, Krasner S. Psychopathology in irritable bowel syndrome: support for a psychophysiological model. J Behav Med. 2003;26(4):361–372. doi:10.1023/A:1024209111909.
- Aro P, Talley NJ, Ronkainen J, Storskrubb T, Vieth M, Johansson SE, Bolling-Sternevald E, Agréus L. Anxiety is associated with uninvestigated and functional dyspepsia (Rome III Criteria) in a Swedish population-based study. Gastroenterology. 2009;137(1):94–100. doi:10.1053/j.gastro.2009.03.039.
- Koloski NA, Jones M, Kalantar J, Weltman M, Zaguirre J, Talley NJ. The brain–gut pathway in functional gastrointestinal disorders is bidirectional: a 12-year prospective population-based study. Gut. 2012;61(9):1284–1290. doi:10.1136/gutjnl-2011-300474.
- Koloski NA, Jones M, Talley NJ. Evidence that independent gut-to-brain and brain-to-gut pathways operate in the irritable bowel syndrome and functional dyspepsia: a 1-year population-based prospective study. Aliment Pharmacol Ther. 2016;44(6):592–600. doi:10.1111/apt.13738.
- Sjölund J, Kull I, Bergström A, Ljótsson B, Törnblom H, Olén O, Simrén M. Quality of life and bidirectional gut-brain interactions in irritable bowel syndrome from adolescence to adulthood. Clin Gastroenterol Hepatol. 2023;22(4):858–866. doi:10.1016/j.cgh.2023.09.022.
- Collins SM, Bercik P. The relationship between intestinal microbiota and the central nervous system in normal gastrointestinal function and disease. Gastroenterology. 2009;136(6):2003–2014. doi:10.1053/j.gastro.2009.01.075.
- Collins SM. A role for the gut microbiota in IBS. Nat Rev Gastroenterol Hepatol. 2014;11(8):497–505. doi:10.1038/nrgastro.2014.40.
- van Thiel I, de Jonge W, van den Wijngaard R. Fungal feelings in the irritable bowel syndrome: the intestinal mycobiome and abdominal pain. Gut Microbes. 2023;15(1):15. doi:10.1080/19490976.2023.2168992.
- Das A, O’Herlihy E, Shanahan F, Pw O, Jeffery IB. The fecal mycobiome in patients with irritable bowel syndrome. Sci Rep. 2021;11(1):1–9. doi:10.1038/s41598-020-79478-6.
- Ansari MH, Ebrahimi M, Fattahi MR, Gardner MG, Safarpour AR, Faghihi MA, Lankarani KB. Viral metagenomic analysis of fecal samples reveals an enteric virome signature in irritable bowel syndrome. BMC Microbiol. 2020;20(1):1–12. doi:10.1186/s12866-020-01817-4.
- Coughlan S, Das A, O’Herlihy E, Shanahan F, O’Toole PW, Jeffery IB. The gut virome in irritable bowel syndrome differs from that of controls. Gut Microbes. 2021;13(1):1–15. doi:10.1080/19490976.2021.1887719.
- Mihindukulasuriya KA, Mars RAT, Johnson AJ, Ward T, Priya S, Lekatz HR, Kalari KR, Droit L, Zheng T, Blekhman R. et al. Multi-omics analyses show disease, diet, and transcriptome interactions with the Virome. Gastroenterology. 2021;161(4):1194–1207. doi:10.1053/j.gastro.2021.06.077.
- Strathdee SA, Hatfull GF, Mutalik VK, Schooley RT. Phage therapy: from biological mechanisms to future directions. Cell. 2023;186(1):17–31. doi:10.1016/j.cell.2022.11.017.
- Mearin F. Postinfectious functional gastrointestinal disorders. J Clin Gastroenterol. 2011;45:102–105. doi:10.1097/MCG.0b013e31821fbf58.
- Jalanka J, Salonen A, Fuentes S, de Vos WM. Microbial signatures in post-infectious irritable bowel syndrome – toward patient stratification for improved diagnostics and treatment. Gut Microbes. 2015;6(6):364–369. doi:10.1080/19490976.2015.1096486.
- Chaudhary NA, Truelove SC. The irritable colon syndrome. A study of the clinical features, predisposing causes, and prognosis in 130 cases. Q J Med. 1962;31:307–322.
- Barbara G, Vallance BA, Collins SM. Persistent intestinal neuromuscular dysfunction after acute nematode infection in mice. Gastroenterology. 1997;113(4):1224–1232. doi:10.1053/gast.1997.v113.pm9322517.
- Neal KR, Hebden J, Spiller R. Prevalence of gastrointestinal symptoms six months after bacterial gastroenteritis and risk factors for development of the irritable bowel syndrome: postal survey of patients. Br Med J. 1997;314(7083):779–779. doi:10.1136/bmj.314.7083.779.
- Barbara G, Grover M, Bercik P, Corsetti M, Ghoshal UC, Ohman L, Rajilić-Stojanović M. Rome foundation working team report on post-infection irritable bowel syndrome. Gastroenterology. 2019;156(1):46–58. doi:10.1053/j.gastro.2018.07.011.
- Balemans D, Mondelaers SU, Cibert-Goton V, Stakenborg N, Aguilera-Lizarraga J, Dooley J, Liston A, Bulmer DC, Vanden Berghe P, Boeckxstaens GE. et al. Evidence for long-term sensitization of the bowel in patients with post-infectious-IBS. Sci Rep. 2017;7(1):1–11. doi:10.1038/s41598-017-12618-7.
- Thabane M, Marshall JK. Post-infectious irritable bowel syndrome. World J Gastroenterol. 2009;15(29):3591–3596. doi:10.3748/wjg.15.3591.
- Aguilera-Lizarraga J, Florens MV, Viola MF, Jain P, Decraecker L, Appeltans I, Cuende-Estevez M, Fabre N, Van Beek K, Perna E. et al. Local immune response to food antigens drives meal-induced abdominal pain. Nature. 2021;590(7844):151–156. doi:10.1038/s41586-020-03118-2.
- Wouters MM, Van Wanrooy S, Nguyen A, Dooley J, Aguilera-Lizarraga J, Van Brabant W, Garcia-Perez JE, Van Oudenhove L, Van Ranst M, Verhaegen J. et al. Psychological comorbidity increases the risk for postinfectious IBS partly by enhanced susceptibility to develop infectious gastroenteritis. Gut. 2016;65(8):1279–1288. doi:10.1136/gutjnl-2015-309460.
- Marshall JK, Thabane M, Garg AX, Clark WF, Moayyedi P, Collins SM. Eight year prognosis of postinfectious irritable bowel syndrome following waterborne bacterial dysentery. Gut. 2010;59(5):605–611. doi:10.1136/gut.2009.202234.
- Dunlop SP, Jenkins D, Neal KR, Spiller RC. Relative importance of enterochromaffin cell hyperplasia, anxiety, and depression in postinfectious IBS. Gastroenterology. 2003;125(6):1651–1659. doi:10.1053/j.gastro.2003.09.028.
- Villani AC, Lemire M, Thabane M, Belisle A, Franchimont D, Marshall JK. Genetic risk factors for post-infectious irritable bowel syndrome following a waterborne outbreak of gastroenteritis. Gastroenterology. 2010;138(4):1502–1513. doi:10.1053/j.gastro.2009.12.049.
- Swan C, Duroudier NP, Campbell E, Zaitoun A, Hastings M, Dukes GE, Cox J, Kelly FM, Wilde J, Lennon MG. et al. Identifying and testing candidate genetic polymorphisms in the irritable bowel syndrome (IBS): association with TNFSF15 and TNFα. Gut. 2013;62(7):985–994. doi:10.1136/gutjnl-2011-301213.
- World Health Organization. Number of COVID-19 cases reported to WHO. https://data.who.int/dashboards/covid19/cases?n=c.
- Lamers MM, Beumer J, Van Der VJ, Knoops K, Puschhof J, Breugem TI, Ravelli RBG, Van SJ, Mykytyn AZ, Duimel HQ. et al. SARS-CoV-2 productively infects human gut enterocytes. Science (1979). 2020;369(6499):50–54. doi:10.1126/science.abc1669.
- Marasco G, Cremon C, Barbaro MR, Cacciari G, Falangone F, Kagramanova A, Bordin D, Drug V, Miftode E, Fusaroli P. et al. Post COVID-19 irritable bowel syndrome. Gut. 2023;72(3):484–492. doi:10.1136/gutjnl-2022-328483.
- Pimentel M, Lembo A. Microbiome and its role in irritable bowel syndrome. Dig Dis Sci [Internet]. 2020;65(3):829–839. doi:10.1007/s10620-020-06109-5.
- Klem F, Wadhwa A, Prokop LJ, Sundt WJ, Farrugia G, Camilleri M, Singh S, Prevalence GM. Risk factors, and outcomes of irritable bowel syndrome after infectious enteritis: a systematic review and meta-analysis. Gastroenterology. 2017;152(5):1042–1054. doi:10.1053/j.gastro.2016.12.039.
- Vajravelu RK, Shapiro JM, Ni J, Thanawala SU, Lewis JD, El-Serag HB. Risk for post-colonoscopy irritable bowel syndrome in patients with and without antibiotic exposure: a retrospective cohort study. Clin Gastroenterol Hepatol. 2022;20(6):e1305–22. doi:10.1016/j.cgh.2021.08.049.
- Maxwell PR, Rink E, Kumar D, Mendall MA. Antibiotics increase functional abdominal symptoms. Am J Gastroenterol. 2002;97(1):104–108. doi:10.1111/j.1572-0241.2002.05428.x.
- Paula H, Grover M, Halder SL, Locke GR, Schleck CD, Zinsmeister AR, Talley NJ. Non-enteric infections, antibiotic use, and risk of development of functional gastrointestinal disorders. Neurogastroent Motil. 2015;27(11):1580–1586. doi:10.1111/nmo.12655.
- Baldassarre ME, Di Mauro A, Salvatore S, Tafuri S, Bianchi FP, Dattoli E, Morando L, Pensabene L, Meneghin F, Dilillo D. et al. Birth weight and the development of functional gastrointestinal disorders in infants. Pediatr Gastroenterol Hepatol Nutr. 2020;23(4):366–376. doi:10.5223/pghn.2020.23.4.366.
- Marasco G, Cremon C, Barbaro MR, Stanghellini V, Barbara G. Gut microbiota signatures and modulation in irritable bowel syndrome. Microbiome Res Rep. 2022;1:1–12. doi:10.20517/mrr.2021.12.
- Duan R, Zhu S, Wang B, Duan L. Alterations of gut microbiota in patients with irritable bowel syndrome based on 16s rRNA-targeted sequencing: a systematic review. Clin Transl Gastroenterol. 2019;10(2):e00012. doi:10.14309/ctg.0000000000000012.
- Jeffery IB, Toole PWO, Lena O, Claesson MJ, Deane J, Quigley EMM, Simre M. An irritable bowel syndrome subtype defined by species-specific alterations in faecal microbiota. Gut. 2012;61(7):997–1006. doi:10.1136/gutjnl-2011-301501.
- Jeffery IB, Das A, O’Herlihy E, Coughlan S, Cisek K, Moore M, Bradley F, Carty T, Pradhan M, Dwibedi C. et al. Differences in fecal microbiomes and metabolomes of people with vs without irritable bowel syndrome and bile acid malabsorption. Gastroenterology. 2020;158(4):1016–1028. doi:10.1053/j.gastro.2019.11.301.
- Jacobs JP, Lagishetty V, Hauer MC, Labus JS, Dong TS, Toma R, Vuyisich M, Naliboff BD, Lackner JM, Gupta A. et al. Multi-omics profiles of the intestinal microbiome in irritable bowel syndrome and its bowel habit subtypes. Microbiome. 2023;11(1):1–18. doi:10.1186/s40168-022-01450-5.
- Pittayanon R, Lau JT, Yuan Y, Leontiadis GI, Tse F, Surette M, Moayyedi P. Gut microbiota in patients with irritable bowel syndrome—a systematic review. Gastroenterology. 2019;157(1):97–108. doi:10.1053/j.gastro.2019.03.049.
- Vich Vila A, Imhann F, Collij V, Jankipersadsing SA, Gurry T, Mujagic Z, Kurilshikov A, Bonder MJ, Jiang X, Tigchelaar EF. et al. Gut microbiota composition and functional changes in inflammatory bowel disease and irritable bowel syndrome. Sci Transl Med. 2018;10(472):1–12. doi:10.1126/scitranslmed.aap8914.
- Tap J, Derrien M, Törnblom H, Brazeilles R, Cools-Portier S, Doré J, Störsrud S, Le Nevé B, Öhman L, Simrén M. Identification of an intestinal microbiota signature associated with severity of irritable bowel syndrome. Gastroenterology. 2017;152(1):111–123. doi:10.1053/j.gastro.2016.09.049.
- Pozuelo M, Panda S, Santiago A, Mendez S, Accarino A, Santos J, Guarner F, Azpiroz F, Manichanh C. Reduction of butyrate- and methane-producing microorganisms in patients with irritable bowel syndrome. Sci Rep. 2015;5(1):1–12. doi:10.1038/srep12693.
- Liu HN, Wu H, Chen YZ, Chen YJ, Shen XZ, Liu TT. Altered molecular signature of intestinal microbiota in irritable bowel syndrome patients compared with healthy controls: a systematic review and meta-analysis. Dig Liver Dis. 2017;49(4):331–337. doi:10.1016/j.dld.2017.01.142.
- Labus JS, Hollister EB, Jacobs J, Kirbach K, Oezguen N, Gupta A, Acosta J, Luna RA, Aagaard K, Versalovic J. et al. Differences in gut microbial composition correlate with regional brain volumes in irritable bowel syndrome. Microbiome. 2017;5(1):1–17. doi:10.1186/s40168-017-0260-z.
- Zhong L, Shanahan ER, Raj A, Koloski NA, Fletcher L, Morrison M, Walker MM, Talley NJ, Holtmann G. Dyspepsia and the microbiome: time to focus on the small intestine. Gut. 2017;66(6):1168–1169. doi:10.1136/gutjnl-2016-312574.
- Brown G, Hoedt EC, Keely S, Shah A, Walker MM, Holtmann G, Talley NJ. Role of the duodenal microbiota in functional dyspepsia. Neurogastroent Motil. 2022;34(11):1–16. doi:10.1111/nmo.14372.
- Leite G, Rezaie A, Mathur R, Barlow GM, Rashid M, Hosseini A, Wang J, Parodi G, Villanueva-Millan MJ, Sanchez M. et al. Defining small intestinal bacterial overgrowth by culture and high throughput sequencing. Clin Gastroenterol Hepatol. 2024;22(2):259–270. doi:10.1016/j.cgh.2023.06.001.
- Shah A, Talley NJ, Jones M, Kendall BJ, Koloski N, Walker MM, Morrison M, Holtmann GJ. Small intestinal bacterial overgrowth in irritable bowel syndrome: a systematic review and meta-analysis of case-control studies. Am J Gastroenterol. 2020;115(2):190–201. doi:10.14309/ajg.0000000000000504.
- Gandhi A, Shah A, Jones MP, Koloski N, Talley NJ, Morrison M, Holtmann G. Methane positive small intestinal bacterial overgrowth in inflammatory bowel disease and irritable bowel syndrome: a systematic review and meta-analysis. Gut Microbes. 2021;13(1):1–16. doi:10.1080/19490976.2021.1933313.
- Ghoshal UC, Nehra A, Mathur A, Rai S. A meta-analysis on small intestinal bacterial overgrowth in patients with different subtypes of irritable bowel syndrome. J Gastroenterol Hepatol (Australia). 2020;35(6):922–931. doi:10.1111/jgh.14938.
- Mars RAT, Frith M, Kashyap PC. Functional gastrointestinal disorders and the microbiome—what is the best strategy for moving microbiome-based therapies for functional gastrointestinal disorders into the clinic? Gastroenterology. 2021;160(2):538–555. doi:10.1053/j.gastro.2020.10.058.
- Maharshak N, Ringel Y, Katibian D, Lundqvist A, Sartor RB, Carroll IM, Ringel-Kulka T. Fecal and Mucosa-associated intestinal microbiota in patients with diarrhea-predominant irritable bowel syndrome. Dig Dis Sci. 2018;63(7):1890–1899. doi:10.1007/s10620-018-5086-4.
- Matsumoto H, Shiotani A, Katsumata R, Fukushima S, Handa Y, Osawa M, Murao T, Handa O, Umegaki E, Inoue R. et al. Mucosa-associated microbiota in patients with irritable bowel syndrome: a comparison of subtypes. Digestion. 2021;102(1):49–56. doi:10.1159/000512167.
- Rangel I, Sundin J, Fuentes S, Repsilber D, De Vos WM, Brummer RJ. The relationship between faecal-associated and mucosal-associated microbiota in irritable bowel syndrome patients and healthy subjects. Aliment Pharmacol Ther. 2015;42(10):1211–1221. doi:10.1111/apt.13399.
- Wu BY, Xu P, Cheng L, Wang QQ, Qiu HY, Yan XJ, Chen SL. Mucosa-associated microbiota dysbiosis in the terminal ileum correlates with bowel symptoms in diarrhea-predominant irritable bowel syndrome. Clin Transl Gastroenterol. 2023;13:1–11. Publish Ah.
- Kerckhoffs APM, Ben-Amor K, Samsom M, Van Der Rest ME, De Vogel J, Knol J, Akkermans LMA. Molecular analysis of faecal and duodenal samples reveals significantly higher prevalence and numbers of Pseudomonas aeruginosa in irritable bowel syndrome. J Med Microbiol. 2011;60(2):236–245. doi:10.1099/jmm.0.022848-0.
- Bednarska O, Walter SA, Casado-Bedmar M, Ström M, Salvo-Romero E, Vicario M, Mayer EA, Keita ÅV. VIP and mast cells regulate increased passage of colonic bacteria in patients with irritable bowel syndrome. Gastroenterology. 2017;153(4):948–960.e3. doi:10.1053/j.gastro.2017.06.051.
- Hanning N, Edwinson AL, Ceuleers H, Peters SA, De Man JG, Hassett LC, De Winter BY, Grover M. Intestinal barrier dysfunction in irritable bowel syndrome: a systematic review. Therap Adv Gastroenterol. 2021;14:1–31. doi:10.1177/1756284821993586.
- Shimbori C, De Palma G, Baerg L, Lu J, Verdu EF, Reed DE, Vanner S, Collins SM, Bercik P. Gut bacteria interact directly with colonic mast cells in a humanized mouse model of IBS. Gut Microbes. 2022;14(1):1–9. doi:10.1080/19490976.2022.2105095.
- Keshteli AH, Madsen KL, Mandal R, Boeckxstaens GE, Bercik P, De Palma G, Reed DE, Wishart D, Vanner S, Dieleman LA. Comparison of the metabolomic profiles of irritable bowel syndrome patients with ulcerative colitis patients and healthy controls: new insights into pathophysiology and potential biomarkers. Aliment Pharmacol Ther. 2019;49(6):723–732. doi:10.1111/apt.15141.
- Su Q, Tun HM, Liu Q, Yeoh YK, Mak JWY, Chan FKL, Ng SC. Gut microbiome signatures reflect different subtypes of irritable bowel syndrome. Gut Microbes. 2023;15(1):2157697. doi:10.1080/19490976.2022.2157697.
- Mujagic Z, Kasapi M, Jonkers DMAE, Garcia-Perez I, Vork L, Weerts ZZRM, Serrano-Contreras JI, Zhernakova A, Kurilshikov A, Scotcher J. et al. Integrated fecal microbiome–metabolome signatures reflect stress and serotonin metabolism in irritable bowel syndrome. Gut Microbes. 2022;14(1):2063016. doi:10.1080/19490976.2022.2063016.
- Han L, Zhao L, Zhou Y, Yang C, Xiong T, Lu L, Deng Y, Luo W, Chen Y, Qiu Q. et al. Altered metabolome and microbiome features provide clues in understanding irritable bowel syndrome and depression comorbidity. Isme J. 2022;16(4):983–996. doi:10.1038/s41396-021-01123-5.
- Camilleri M, Vijayvargiya P. The role of bile acids in chronic diarrhea. Am J Gastroenterol. 2020;115(10):1596–1603. doi:10.14309/ajg.0000000000000696.
- Marasco G, Cremon C, Barbaro MR, Falangone F, Montanari D, Capuani F, Mastel G, Stanghellini V, Barbara G. Pathophysiology and clinical management of bile acid diarrhea. J Clin Med. 2022;11(11):3102. doi:10.3390/jcm11113102.
- Camilleri M, Carlson P, Bousaba J, McKinzie S, Vijayvargiya P, Magnus Y, Sannaa W, Wang XJ, Chedid V, Zheng T. et al. Comparison of biochemical, microbial and mucosal mRNA expression in bile acid diarrhoea and irritable bowel syndrome with diarrhoea. Gut. 2023;72(1):54–65. doi:10.1136/gutjnl-2022-327471.
- Gargari G, Taverniti V, Gardana C, Cremon C, Canducci F, Pagano I, Barbaro MR, Bellacosa L, Castellazzi AM, Valsecchi C. et al. Fecal Clostridiales distribution and short-chain fatty acids reflect bowel habits in irritable bowel syndrome. Environ Microbiol. 2018;20(9):3201–3213. doi:10.1111/1462-2920.14271.
- Bennet SM, Keshteli AH, Bercik P, Madsen KL, Reed D, Vanner SJ. Application of metabolomics to the study of irritable bowel syndrome. Neurogastroent Motil. 2020;32(6):e13884. doi:10.1111/nmo.13884.
- De Palma G, Lynch MDJ, Lu J, Dang VT, Deng Y, Jury J, Umeh G, Miranda PM, Pastor MP, Sidani S. et al. Transplantation of fecal microbiota from patients with irritable bowel syndrome alters gut function and behavior in recipient mice. Sci Transl Med. 2017;9(379):1–15. doi:10.1126/scitranslmed.aaf6397.
- De Palma G, Shimbori C, Reed DE, Yu Y, Rabbia V, Lu J, Jimenez-Vargas N, Sessenwein J, Lopez-Lopez C, Pigrau M. et al. Histamine production by the gut microbiota induces visceral hyperalgesia through histamine 4 receptor signaling in mice. Sci Transl Med. 2022;14(655):1–13. doi:10.1126/scitranslmed.abj1895.
- Tuck CJ, Abu Omar A, De Palma G, Osman S, Jiménez-Vargas NN, Yu Y, Bennet SMP, Lopez-Lopez C, Jaramillo-Polanco JO, Baker CC. et al. Changes in signalling from faecal neuroactive metabolites following dietary modulation of IBS pain. Gut. 2023;72(9):1678–1691. doi:10.1136/gutjnl-2022-327260.
- Barbara G, Stanghellini V, De Giorgio R, Cremon C, Cottrell GS, Santini D, Pasquinelli G, Morselli-Labate AM, Grady EF, Bunnett NW. et al. Activated mast cells in proximity to colonic nerves correlate with abdominal pain in irritable bowel syndrome. Gastroenterology. 2004;126(3):693–702. doi:10.1053/j.gastro.2003.11.055.
- Barbara G, Wang B, Stanghellini V, de Giorgio R, Cremon C, Di Nardo G, Trevisani M, Campi B, Geppetti P, Tonini M. et al. Mast cell-dependent excitation of visceral-nociceptive sensory neurons in irritable bowel syndrome. Gastroenterology. 2007;132(1):26–37. doi:10.1053/j.gastro.2006.11.039.
- Balemans D, Aguilera-Lizarraga J, Florens MV, Jain P, Denadai-Souza A, Viola MF, Alpizar YA, Van Der Merwe S, Vanden Berghe P, Talavera K. et al. Histamine-mediated potentiation of transient receptor potential (TRP) ankyrin 1 and TRP vanilloid 4 signaling in submucosal neurons in patients with irritable bowel syndrome. Am J Physiol Gastrointest Liver Physiol. 2019;316(3):G338–49. doi:10.1152/ajpgi.00116.2018.
- Wouters MM, Balemans D, Van Wanrooy S, Dooley J, Cibert-Goton V, Alpizar YA, Valdez-Morales EE, Nasser Y, Van Veldhoven PP, Vanbrabant W. et al. Histamine receptor H1–mediated sensitization of TRPV1 mediates visceral hypersensitivity and symptoms in patients with irritable bowel syndrome. Gastroenterology. 2016;150(4):875–887. doi:10.1053/j.gastro.2015.12.034.
- Decraecker L, De LD, Hirsch DP, De SH, Arts J, Caenepeel P, Bredenoord AJ, Kolkman J, Bellens K, Van BK. et al. Treatment of non-constipated irritable bowel syndrome with the histamine 1 receptor antagonist ebastine: a randomised, double-blind, placebo-controlled trial. Gut. 2024;73:459–469. doi:10.1136/gutjnl-2023-331634.
- Khasawneh M, Black CJ, Ford AC. Efficacy of drugs acting on histamine 1 receptors in irritable bowel syndrome: systematic review and meta-analysis. Gastroenterology. 2024 Mar. 16:S0016–5085(24)00297–X. doi:10.1053/j.gastro.2024.03.014.
- Edwinson AL, Yang L, Peters S, Hanning N, Jeraldo P, Jagtap P, Simpson JB, Yang TY, Kumar P, Mehta S. et al. Gut microbial β-glucuronidases regulate host luminal proteases and are depleted in irritable bowel syndrome. Nat Microbiol. 2022;7(5):680–694. doi:10.1038/s41564-022-01103-1.
- Caminero A, Guzman M, Libertucci J, Lomax AE. The emerging roles of bacterial proteases in intestinal diseases. Gut Microbes. 2023;15(1):2181922. doi:10.1080/19490976.2023.2181922.
- Bhattarai Y, Williams BB, Battaglioli EJ, Whitaker WR, Till L, Grover M, Linden DR, Akiba Y, Kandimalla KK, Zachos NC. et al. Gut microbiota-produced tryptamine activates an epithelial G-protein-coupled receptor to increase colonic secretion. Cell Host Microbe. 2018;23(6):775–785. doi:10.1016/j.chom.2018.05.004.
- Keefer L, Ko CW, Ford AC. AGA clinical practice update on management of chronic gastrointestinal pain in disorders of gut–brain interaction: expert review. Clin Gastroenterol Hepatol. 2021;19(12):2481–2488. doi:10.1016/j.cgh.2021.07.006.
- Lembo A, Sultan S, Chang L, Heidelbaugh JJ, Smalley W, Verne GN. AGA clinical practice guideline on the pharmacological management of irritable bowel syndrome with diarrhea. Gastroenterology. 2022;163(1):137–151. doi:10.1053/j.gastro.2022.04.017.
- Ford AC, Wright-Hughes A, Alderson SL, Ow PL, Ridd MJ, Foy R, Bianco G, Bishop FL, Chaddock M, Cook H. et al. Amitriptyline at low-dose and titrated for irritable bowel syndrome as second-line treatment in primary care (ATLANTIS): a randomised, double-blind, placebo-controlled, phase 3 trial. Lancet. 2023;402(10414):1773–1785. doi:10.1016/S0140-6736(23)01523-4.
- Borup C, Vinter-Jensen L, Jørgensen SPG, Wildt S, Graff J, Gregersen T, Zaremba A, Borup Andersen T, Nøjgaard C, Timm HB. Efficacy and safety of colesevelam for the treatment of bile acid diarrhoea: a double-blind, randomised, placebo-controlled, phase 4 clinical trial. Lancet Gastroenterol Hepatol. 2023;8(4):321–331. doi:10.1016/S2468-1253(22)00401-0.
- Li B, Zhang J, Chen Y, Wang Q, Yan L, Wang R, Wei Y, You Z, Li Y, Miao Q. et al. Alterations in microbiota and their metabolites are associated with beneficial effects of bile acid sequestrant on icteric primary biliary Cholangitis. Gut Microbes. 2021;13(1):1946366. doi:10.1080/19490976.2021.1946366.
- Kumar A, Quraishi MN, Al-Hassi HO, El-Asrag ME, Segal JP, Jain M, Steed H, Butterworth J, Farmer A, Mclaughlin J. et al. The analysis of gut microbiota in patients with bile acid diarrhoea treated with colesevelam. Front Microbiol. 2023;14:1–11. doi:10.3389/fmicb.2023.1134105.
- Black CJ, Ford AC. How effective are antibiotics for the treatment of irritable bowel syndrome? Expert Opin Pharmacother. 2020;21(18):2195–2197. doi:10.1080/14656566.2020.1808623.
- Pimentel M, Chow EJ, Lin HC. Normalization of lactulose breath testing correlates with symptom improvement in irritable bowel syndrome: a double-blind, randomized, placebo-controlled study. Am J Gastroenterol. 2003;98(2):412–419. doi:10.1111/j.1572-0241.2003.07234.x.
- Ghoshal UC, Srivastava D, Misra A, Ghoshal U. A proof-of-concept study showing antibiotics to be more effective in irritable bowel syndrome with than without small-intestinal bacterial overgrowth: a randomized, double-blind, placebo-controlled trial. Eur J Gastroenterol Hepatol. 2016;28(3):281–289. doi:10.1097/MEG.0000000000000557.
- Acosta A, Camilleri M, Shin A, Nord SL, Neill JO, Gray AV, Lueke AJ, Donato LJ, Burton DD, Szarka LA. et al. Effects of rifaximin on transit, permeability, fecal microbiome, and organic acid excretion in irritable bowel syndrome. Clin Transl Gastroenterol. 2016;7(5):e173. doi:10.1038/ctg.2016.32.
- Black CJ, Burr NE, Camilleri M, Earnest DL, Quigley EMM, Moayyedi P, Houghton LA, Ford AC. Efficacy of pharmacological therapies in patients with IBS with diarrhoea or mixed stool pattern: systematic review and network meta-analysis. Gut 2020;69(1):74–82. doi:10.1136/gutjnl-2018-318160.
- Ponziani FR, Scaldaferri F, De Siena M, Mangiola F, Matteo MV, Pecere S, Petito V, Paroni Sterbini F, Lopetuso LR, Masucci L. et al. Increased Faecalibacterium abundance is associated with clinical improvement in patients receiving rifaximin treatment. Benef Microbes. 2020;11(6):519–525. doi:10.3920/BM2019.0171.
- Pimentel M. Review article: Potential mechanisms of action of rifaximin in the management of irritable bowel syndrome with diarrhoea. Aliment Pharmacol Ther. 2016;43(S1):37–49. doi:10.1111/apt.13437.
- Champagne-Jorgensen K, Kunze WA, Forsythe P, Bienenstock J, McVey Neufeld KA. Antibiotics and the nervous system: more than just the microbes? Brain Behav Immun. 2019;77:7–15. doi:10.1016/j.bbi.2018.12.014.
- Xu D, Gao J, Gillilland M, Wu X, Song I, Kao JY, Owyang C. Rifaximin alters intestinal bacteria and prevents stress-induced gut inflammation and visceral hyperalgesia in rats. Gastroenterology. 2014;146(2):484–496.e4. doi:10.1053/j.gastro.2013.10.026.
- Macedo D, Filho AJMC, de Sousa C, Quevedo J, Barichello T, Júnior HVN, Freitas de Lucena D. Antidepressants, antimicrobials or both? Gut microbiota dysbiosis in depression and possible implications of the antimicrobial effects of antidepressant drugs for antidepressant effectiveness. Journal Of Affective Disorders. 2017;208:22–32. doi:10.1016/j.jad.2016.09.012.
- Fung TC, Vuong HE, Luna CDG, Pronovost GN, Aleksandrova AA, Riley NG, Vavilina A, McGinn J, Rendon T, Forrest LR. et al. Intestinal serotonin and fluoxetine exposure modulate bacterial colonization in the gut. Nat Microbiol. 2019;4(12):2064–2073. doi:10.1038/s41564-019-0540-4.
- Varma R, Staab JP, Matey ET, Wright JA, Deb B, Lazaridis KN, Szarka LA, Bailey KR, Bharucha AE. Most patients with disorders of gut-brain interaction receive pharmacotherapy with major or moderate drug-gene interactions. Neurogastroent Motil. 2023;36(2):1–13. doi:10.1111/nmo.14722.
- Chey WD, Hashash JG, Manning L, Chang L. AGA clinical practice update on the role of diet in irritable bowel syndrome: expert review. Gastroenterology. 2022;162(6):1737–1745.e5. doi:10.1053/j.gastro.2021.12.248.
- De Palma G, Reed DE, Bercik P. Diet–microbial cross–talk underlying increased visceral perception. Gut Microbes. 2023;15(1):2166780. doi:10.1080/19490976.2023.2166780.
- De Palma G, Bercik P. Long-term personalized low FODMAP diet in IBS. Neurogastroent Motil. 2022;34(4):1–6. doi:10.1111/nmo.14356.
- Algera JP, Magnusson MK, Öhman L, Störsrud S, Simrén M, Törnblom H. Randomised controlled trial: effects of gluten-free diet on symptoms and the gut microenvironment in irritable bowel syndrome. Aliment Pharmacol Ther. 2022;56(9):1318–1327. doi:10.1111/apt.17239.
- Pinto-Sanchez MI, Nardelli A, Borojevic R, De Palma G, Calo NC, McCarville J, Caminero A, Basra D, Mordhorst A, Ignatova E. et al. Gluten-free diet reduces symptoms, particularly diarrhea, in patients with irritable bowel syndrome and antigliadin IgG. Clin Gastroenterol Hepatol. 2021;19(11):2343–2352. doi:10.1016/j.cgh.2020.08.040.
- Savarino E, Zingone F, Barberio B, Marasco G, Akyuz F, Akpinar H, Barboi O, Bodini G, Bor S, Chiarioni G. et al. Functional bowel disorders with diarrhoea: clinical guidelines of the United European gastroenterology and European society for neurogastroenterology and motility. United European Gastroenterol J. 2022;10(6):556–584. doi:10.1002/ueg2.12259.
- Dionne J, Ford AC, Yuan Y, Chey WD, Lacy BE, Saito YA, Quigley EMM, Moayyedi P. A systematic review and meta-analysis evaluating the efficacy of a gluten-free diet and a low FODMAPs diet in treating symptoms of irritable bowel syndrome. Am J Gastroenterol. 2018;113(9):1290–1300. doi:10.1038/s41395-018-0195-4.
- Mohseni F, Agah S, Ebrahimi-Daryani N, Taher M, Nattagh-Eshtivani E, Karimi S, Rastgoo S, Bourbour F, Hekmatdoost A. The effect of low FODMAP diet with and without gluten on irritable bowel syndrome: a double blind, placebo controlled randomized clinical trial. Clin Nutr ESPEN. 2022;47:45–50. doi:10.1016/j.clnesp.2021.12.019.
- Bojarski C, Tangermann P, Barmeyer C, Buchkremer J, Kiesslich R, Ellrichmann M, Schreiber S, Schmidt C, Stallmach A, Roehle R. et al. Prospective, double-blind diagnostic multicentre study of confocal laser endomicroscopy for wheat sensitivity in patients with irritable bowel syndrome. Gut. 2022;71(8):1567–1576. doi:10.1136/gutjnl-2021-325181.
- Gibson PR, Halmos EP, So D, Yao CK, Varney JE, Muir JG. Diet as a therapeutic tool in chronic gastrointestinal disorders: Lessons from the FODMAP journey. J Gastroenterol Hepatol (Australia). 2022;37(4):644–652. doi:10.1111/jgh.15772.
- Caminero A, McCarville JL, Zevallos VF, Pigrau M, Yu XB, Jury J, Galipeau HJ, Clarizio AV, Casqueiro J, Murray JA. et al. Lactobacilli degrade wheat amylase trypsin inhibitors to reduce intestinal dysfunction induced by immunogenic wheat proteins. Gastroenterology. 2019;156(8):2266–2280. doi:10.1053/j.gastro.2019.02.028.
- Caminero A, Galipeau HJ, McCarville JL, Johnston CW, Bernier SP, Russell AK, Jury J, Herran AR, Casqueiro J, Tye-Din JA. et al. Duodenal bacteria from patients with celiac disease and healthy subjects distinctly affect gluten breakdown and immunogenicity. Gastroenterology. 2016;151(4):670–683. doi:10.1053/j.gastro.2016.06.041.
- Seiler C, Rueda G, Miranda P, Nardelli A, Borojevic R, Schuppan D, Moayyedi P, Verdu EF, Collins SM, Pinto-Sanchez MI. et al. 683 investigating mechanisms that drive symptoms in patients with irritable bowel syndrome and perceived gluten sensitivity. Gastroenterology. 2023;164(6):S-136–S–137. doi:10.1016/S0016-5085(23)01290-8.
- Wu J, Masuy I, Biesiekierski JR, Fitzke HE, Parikh C, Schofield L, Shaikh H, Bhagwanani A, Aziz Q, Taylor SA. et al. Gut-brain axis dysfunction underlies FODMAP-induced symptom generation in irritable bowel syndrome. Aliment Pharmacol Ther. 2022;55(6):670–682. doi:10.1111/apt.16812.
- Black CJ, Staudacher HM, Ford AC. Efficacy of a low FODMAP diet in irritable bowel syndrome: systematic review and network meta-analysis. Gut. 2022;71(6):1117–1126. doi:10.1136/gutjnl-2021-325214.
- Gunn D, Abbas Z, Harris HC, Major G, Hoad C, Gowland P, Marciani L, Gill SK, Warren FJ, Rossi M. et al. Psyllium reduces inulin-induced colonic gas production in IBS: MRI and in vitro fermentation studies. Gut. 2022;71(5):919–927. doi:10.1136/gutjnl-2021-324784.
- McIntosh K, Reed DE, Schneider T, Dang F, Keshteli AH, De Palma G, Madsen K, Bercik P, Vanner S. FODMAPs alter symptoms and the metabolome of patients with IBS: a randomised controlled trial. Gut. 2017;66(7):1241–1251. doi:10.1136/gutjnl-2015-311339.
- Zhou SY, Gillilland M, Wu X, Leelasinjaroen P, Zhang G, Zhou H, Ye B, Lu Y, Owyang C. FODMAP diet modulates visceral nociception by lipopolysaccharide-mediated intestinal inflammation and barrier dysfunction. J Clin Invest. 2018;128(1):267–280. doi:10.1172/JCI92390.
- Singh P, Grabauskas G, Zhou SY, Gao J, Zhang Y, Owyang C. High FODMAP diet causes barrier loss via lipopolysaccharide-mediated mast cell activation. JCI Insight. 2021;6(22):6. doi:10.1172/jci.insight.146529.
- Yang R, Jiang J, Ouyang J, Zhao Y, Xi B. Efficacy and safety of probiotics in irritable bowel syndrome: a systematic review and meta-analysis. Clin Nutr ESPEN. 2024;60:362–372. doi:10.1016/j.clnesp.2024.02.025.
- Lacy BE, Pimentel M, Brenner DM, Chey WD, Keefer LA, Long MD, Moshiree B. ACG Clinical Guideline: management of Irritable Bowel Syndrome. Am J Gastroenterol. 2021;116(1):17–44. doi:10.14309/ajg.0000000000001036.
- Moshiree B, Drossman D, Shaukat A. AGA clinical practice update on evaluation and management of belching, abdominal bloating, and distention: expert review. Gastroenterology [Internet]. 2023;165(3):791–800. doi:10.1053/j.gastro.2023.04.039.
- Goodoory VC, Khasawneh M, Black CJ, Quigley EMM, Moayyedi P, Ford AC. Efficacy of probiotics in irritable bowel syndrome: systematic review and meta-analysis. Gastroenterology [Internet]. 2023;165(5):1206–1218. doi:10.1053/j.gastro.2023.07.018.
- Di Nardo G, Barbara G, Borrelli O, Cremon C, Giorgio V, Greco L, La Pietra M, Marasco G, Pensabene L, Piccirillo M. et al. Italian guidelines for the management of irritable bowel syndrome in children and adolescents. Ital J Pediatr. 2024;50(1):1–23. doi:10.1186/s13052-024-01607-y.
- Pinto-Sanchez MI, Hall GB, Ghajar K, Nardelli A, Bolino C, Lau JT, Martin FP, Cominetti O, Welsh C, Rieder A. et al. Probiotic Bifidobacterium longum NCC3001 reduces depression scores and alters brain activity: a pilot study in patients with irritable bowel syndrome. Gastroenterology. 2017;153(2):448–459. doi:10.1053/j.gastro.2017.05.003.
- Meynier M, Daugey V, Mallaret G, Gervason S, Meleine M, Barbier J, Aissouni Y, Lolignier S, Bonnet M, Ardid D. et al. Pasteurized akkermansia muciniphila improves irritable bowel syndrome-like symptoms and related behavioral disorders in mice. Gut Microbes. 2024;16(1):2298026. doi:10.1080/19490976.2023.2298026.
- Constante M, De Palma G, Lu J, Jury J, Rondeau L, Caminero A, Collins SM, Verdu EF, Bercik P. Saccharomyces boulardii CNCM I-745 modulates the microbiota–gut–brain axis in a humanized mouse model of irritable bowel syndrome. Neurogastroent Motil. 2021;33(3):1–9. doi:10.1111/nmo.13985.
- van Nood E, Vrieze A, Nieuwdorp M, Fuentes S, Zoetendal EG, de Vos WM, Visser CE, Kuijper EJ, Bartelsman JFWM, Tijssen JGP. et al. Duodenal infusion of donor feces for recurrent Clostridium difficile. N Engl J Med. 2013;368(5):407–415. doi:10.1056/NEJMoa1205037.
- Holvoet T, Joossens M, Vázquez-Castellanos JF, Christiaens E, Heyerick L, Boelens J, Verhasselt B, van Vlierberghe H, De Vos M, Raes J. et al. Fecal microbiota transplantation reduces symptoms in some patients with irritable bowel syndrome with predominant abdominal bloating: short- and long-term results from a placebo-controlled randomized trial. Gastroenterology. 2021;160(1):145–157. doi:10.1053/j.gastro.2020.07.013.
- El-Salhy M, Winkel R, Casen C, Hausken T, Gilja OH, Hatlebakk JG. Efficacy of fecal microbiota transplantation for patients with irritable bowel syndrome at 3 years after transplantation. Gastroenterology. 2022;163(4):982–994. doi:10.1053/j.gastro.2022.06.020.
- Rokkas T, Hold GL. A systematic review, pairwise meta-analysis and network meta-analysis of randomized controlled trials exploring the role of fecal microbiota transplantation in irritable bowel syndrome. Eur J Gastroenterol Hepatol. 2023;35(4):471–479. doi:10.1097/MEG.0000000000002519.
- Halkjær SI, Lo B, Cold F, Christensen AH, Holster S, König J, Brummer RJ, Aroniadis OC, Lahtinen P, Holvoet T. et al. Fecal microbiota transplantation for the treatment of irritable bowel syndrome: a systematic review and meta-analysis. World J Gastroenterol. 2023;29(20):3185–3202. doi:10.3748/wjg.v29.i20.3185.
- El-Salhy M, Gilja OH, Hatlebakk JG. Factors affecting the outcome of fecal microbiota transplantation for patients with irritable bowel syndrome. Neurogastroent Motil. 2024;36(1):e14641. doi:10.1111/nmo.14641.
- Ianiro G, Punčochář M, Karcher N, Porcari S, Armanini F, Asnicar F, Beghini F, Blanco-Míguez A, Cumbo F, Manghi P. et al. Variability of strain engraftment and predictability of microbiome composition after fecal microbiota transplantation across different diseases. Nat Med. 2022;28(9):1913–1923. doi:10.1038/s41591-022-01964-3.
- Black CJ, Thakur ER, Houghton LA, Quigley EMM, Moayyedi P, Ford AC. Efficacy of psychological therapies for irritable bowel syndrome: systematic review and network meta-analysis. Gut. 2020;69(8):1441–1451. doi:10.1136/gutjnl-2020-321191.
- Lackner JM, Jaccard J, Keefer L, Brenner DM, Firth RS, Gudleski GD, Hamilton FA, Katz LA, Krasner SS, Ma CX. et al. Improvement in gastrointestinal symptoms after cognitive behavior therapy for refractory irritable bowel syndrome. Gastroenterology. 2018;155(1):47–57. doi:10.1053/j.gastro.2018.03.063.
- Jacobs JP, Gupta A, Bhatt RR, Brawer J, Gao K, Tillisch K, Lagishetty V, Firth R, Gudleski GD, Elligson BM. et al. Cognitive behavioral therapy for irritable bowel syndrome induces bidirectional alterations in the brain-gut-microbiome axis associated with gastrointestinal symptom improvement. Microbiome. 2021;9(1):1–14. doi:10.1186/s40168-021-01188-6.
- Islam Z, D’Silva A, Raman M, Nasser Y. The role of mind body interventions in the treatment of irritable bowel syndrome and fibromyalgia. Front Psychiatry. 2022;13:1076763. doi:10.3389/fpsyt.2022.1076763.
- D’Silva A, Marshall DA, Vallance JK, Nasser Y, Rajagopalan V, Szostakiwskyj JH, Raman M. Meditation and yoga for irritable bowel syndrome: a randomized clinical trial. Am J Gastroenterol. 2023;118(2):329–337. doi:10.14309/ajg.0000000000002052.
- Kuttner L, Chambers CT, Hardial J, Israel DM, Jacobson K, Evans K. A randomized trial of yoga for adolescents with irritable bowel syndrome. Pain Res Manag. 2006;11(4):217–224. doi:10.1155/2006/731628.
- Ljótsson B, Andersson G, Andersson E, Hedman E, Lindfors P, Andréewitch S, Rück C, Lindefors N. Acceptability, effectiveness, and cost-effectiveness of internet-based exposure treatment for irritable bowel syndrome in a clinical sample: a randomized controlled trial. BMC Gastroenterol. 2011;11(1):1–13. doi:10.1186/1471-230X-11-110.
- Zernicke KA, Campbell TS, Blustein PK, Fung TS, Johnson JA, Bacon SL, Carlson LE. Mindfulness-based stress reduction for the treatment of irritable bowel syndrome symptoms: a randomized wait-list controlled trial. Int J Behav Med. 2013;20(3):385–396. doi:10.1007/s12529-012-9241-6.
- Evans S, Seidman LC, Lung K, Sternlieb B, Zeltzer LK. Yoga for teens with irritable bowel syndrome: results from a mixed-methods pilot study. Holist Nurs Pract. 2018;32(5):253–260. doi:10.1097/HNP.0000000000000288.
- Schumann D, Langhorst J, Dobos G, Cramer H. Randomised clinical trial: yoga vs a low-FODMAP diet in patients with irritable bowel syndrome. Aliment Pharmacol Ther. 2018;47(2):203–211. doi:10.1111/apt.14400.
- Taneja I, Deepak KK, Poojary G, Acharya IN, Pandey RM, Sharma MP. Yogic versus conventional treatment in diarrhea-predominant irritable bowel syndrome: a randomized control study. Appl Psychophysiol Biofeedback. 2004;29(1):19–33. doi:10.1023/B:APBI.0000017861.60439.95.
- Kavuri V, Selvan P, Malamud A, Raghuram N, Selvan SR. Remedial yoga module remarkably improves symptoms in irritable bowel syndrome patients: a 12-week randomized controlled trial. Eur J Integr Med. 2015;7(6):595–608. doi:10.1016/j.eujim.2015.11.001.
- Shahabi L, Naliboff BD, Shapiro D. Self-regulation evaluation of therapeutic yoga and walking for patients with irritable bowel syndrome: a pilot study. Psychol Health Med. 2016;21(2):176–188. doi:10.1080/13548506.2015.1051557.
- Lacy BE, Cangemi DJ, Spiegel BR. Virtual reality: a new treatment paradigm for disorders of gut-brain interaction? Gastroenterol Hepatol (N Y). 2023;19(2):86–94.
- Cangemi DJ, Montenegro M, Spiegel BR, Lacy BE. Virtual reality improves symptoms of functional dyspepsia: results of a randomized, double-blind, sham-controlled, pilot study. Am J Gastroenterol. 2023;119(1):210–213. doi:10.14309/ajg.0000000000002492.
- Spiegel BMR, Liran O, Gale R, Khalil C, Makaroff K, Chernoff R, Raber T, Chang B, Pichetshote N, Rezaie A. Qualitative validation of a novel VR program for irritable bowel syndrome: a VR1 study. Am J Gastroenterol. 2022;117(3):495–500. doi:10.14309/ajg.0000000000001641.
- Bonaz B, Bazin T, Pellissier S. The vagus nerve at the interface of the microbiota-gut-brain axis. Front Neurosci. 2018;12:1–9. doi:10.3389/fnins.2018.00049.
- Shi X, Hu Y, Zhang B, Li W, Chen JDZ, Liu F. Ameliorating effects and mechanisms of transcutaneous auricular vagal nerve stimulation on abdominal pain and constipation. JCI Insight. 2021;6(14):0–18. doi:10.1172/jci.insight.150052.
- Alam J, Chen JDZ. Non invasive neuromodulation: an emerging intervention for visceral pain in gastrointestinal disorders. Bioelectron Med. 2023;9(1):27. doi:10.1186/s42234-023-00130-5.
- Yan Q, Chen J, Ren X, Song Y, Xu J, Xuan S, Jiang X, Kuang Z, Tang Z. Vagus nerve stimulation relieves irritable bowel syndrome and the associated depression via α7nAChR-mediated anti-inflammatory pathway. Neuroscience. 2023;530:26–37. doi:10.1016/j.neuroscience.2023.08.026.
- Krasaelap A, Sood MR, Li BUK, Unteutsch R, Yan K, Nugent M, Simpson P, Kovacic K. Efficacy of auricular neurostimulation in adolescents with irritable bowel syndrome in a randomized, double-blind trial. Clinical Gastroenterology and Hepatology. 2020;18(9):1987–1994. doi:10.1016/j.cgh.2019.10.012.
- Bora G, Atkinson SN, Pan A, Sood M, Salzman N, Karrento K. Impact of auricular percutaneous electrical nerve field stimulation on gut microbiome in adolescents with irritable bowel syndrome: a pilot study. J Dig Dis. 2023;24(5):348–358. doi:10.1111/1751-2980.13203.
- Kovacic K, Hainsworth K, Sood M, Chelimsky G, Unteutsch R, Nugent M, Simpson P, Miranda A. Neurostimulation for abdominal pain-related functional gastrointestinal disorders in adolescents: a randomised, double-blind, sham-controlled trial. Lancet Gastroenterol Hepatol. 2017;2(10):727–737. doi:10.1016/S2468-1253(17)30253-4.