ABSTRACT
The gut microbial ecosystem communicates bidirectionally with the brain in what is known as the gut-microbiome-brain axis. Bidirectional signaling occurs through several pathways including signaling via the vagus nerve, circulation of microbial metabolites, and immune activation. Alterations in the gut microbiota are implicated in Alzheimer’s disease (AD), a progressive neurodegenerative disease. Perturbations in gut microbial communities may affect pathways within the gut-microbiome-brain axis through altered production of microbial metabolites including ɣ-aminobutyric acid (GABA), the primary inhibitory mammalian neurotransmitter. GABA has been shown to act on gut integrity through modulation of gut mucins and tight junction proteins and may be involved in vagus nerve signal inhibition. The GABAergic signaling pathway has been shown to be dysregulated in AD, and may be responsive to interventions. Gut microbial production of GABA is of recent interest in neurological disorders, including AD. Bacteroides and Lactic Acid Bacteria (LAB), including Lactobacillus, are predominant producers of GABA. This review highlights how temporal alterations in gut microbial communities associated with AD may affect the GABAergic signaling pathway, intestinal barrier integrity, and AD-associated inflammation.
Introduction
The gut microbiota, which is the aggregate of all microbial life in the gastrointestinal tract (GI), contributes to host physiologic functions through production of metabolites,Citation1,Citation2 neurotransmitters,Citation1,Citation3–5 and digestion of food.Citation6,Citation7 Communication between the brain and gut microbiota is known as the gut-microbiome-brain axis and functions bidirectionally via pathways such as the vagus nerveCitation8–10 immune activationCitation11–13 and circulation of microbial metabolites.Citation9,Citation14 Alterations of the gut microbiota and associated metabolites are implicated in a myriad of diseases including those related directly to gut health, such as celiacCitation12 and Crohn’s disease,Citation15,Citation16 and neurodegenerative diseases such as Parkinson’s diseaseCitation17,Citation18 and Alzheimer’s disease (AD).Citation19–22
AD is a debilitating neurodegenerative disease and the most common cause of dementia, affecting approximately 1:9 Americans over the age of 6523. Hallmark pathologies of AD include amyloid-β plaques, neurofibrillary tangles, and neuroinflammation.Citation23 Factors contributing to AD pathogenesis are complex. Age is the greatest risk factor for AD and others include genetics, lifestyle, education, social engagement, obesity, diabetes, and traumatic brain injury.Citation24 Recently, scientists have begun to understand that the gut microbiome, which is affected by genetics and lifestyle factors, is also a risk factor for AD. Several recent studies have demonstrated altered gut microbial composition in humans and in animal models of AD pathologies.Citation19–22,Citation25
Studies of the gut microbiota in AD in both human and animal models indicate a shift in the gut microbiota composition, preceding and concomitantly with onset of pathologies.Citation19,Citation20,Citation22,Citation26 Several studies find Bacteroides differentially abundant in the fecal microbiota of both humans and animal models of AD pathologies.Citation19,Citation21,Citation27,Citation28 Borsom et al. demonstrated that Bacteroides acidifaciens was enriched after pathological onset in triple transgenic mice modeling AD pathologies (3×Tg-AD) whereas Lactobacillus salivarius in 3×Tg-AD mice was depleted compared to WT controls, prior to emergence of characteristic pathologies.Citation22 Changes in the gut microbial communities may be a direct result of changing neurochemical balances within the host,Citation29 leading to greater demand on microbes themselves to create and regulate neurotransmitters as energy sources. Key gut microbial taxa, including those altered in AD, such as Bacteroides and Lactobacillus, are known to produce the mammalian inhibitory neurotransmitter, ɣ-aminobutyric acid (GABA).Citation4,Citation9,Citation30 The role of GABA in the gut in AD may include stimulating the vagus nerve to send afferent signals to the brain or altering gut mucosal membrane barrier integrity through changes in mucin production.Citation9,Citation31,Citation32 The mucus layer in the GI tract forms a physiologic barrier between microbes and gut epithelia and facilitates the movement of solutes within and out of the gut.Citation9,Citation33 Reduced mucus layers allow closer proximity of microbes and their metabolites to the gut epithelial cells, compromising tight junctions and allowing passage of microbial products into the lamina propria.Citation34–36 To this end, endogenous GABA produced into the gut may escape through the intestinal epithelia,Citation37 into the lamina propria, and travel through the bloodstream to the brain.
Tight junctions are a complex network of proteins which regulate gut epithelial and blood–brain barrier (BBB) integrity and modulate the movement of solutes across cell barriers.Citation38–40 Some studies suggest that gut barrier integrity may be compromised in AD,Citation36,Citation41 which may allow microbial metabolites and byproducts into the lamina propria and access to the bloodstream.Citation42–44 Regulation of tight junction proteins occurs through host and microbial metabolites and host inflammatory processes.Citation38,Citation40,Citation45 Dysregulated tight junction proteins may allow the translocation of host- and microbe-derived metabolites to escape into the bloodstream and reach the BBB, which is also damaged in AD.Citation46–49 Increased intestinal permeability, coupled with neuroinflammation affecting the integrity of the BBB, may be an important pathway in the gut-microbiome-brain axis. The goal of this review is to highlight how alterations in the gut microbiota in AD influence intestinal barrier integrity, with a focus on the emerging role of microbiome-derived neurotransmitters in signaling through the GABAergic pathway.
Alzheimer’s disease
AD is a neurodegenerative disease characterized by amyloid-β plaques and neurofibrillary tangles and is the most common cause of dementia among elderly individuals (~60–80% of dementia cases) in the United States.Citation50 While the incidence of AD appears to be declining,Citation51 the population of people living with AD continues to rise with the planet’s growing number of aging individuals.Citation50 Symptoms of this disease include memory loss, trouble with fine motor movement, behavioral changes, and eventually, death.Citation50,Citation52 While each of these symptoms are common manifestations of aging, even in healthy individuals, patients living with AD experience these symptoms at greater severities than what would be expected for their age.Citation52 Behavioral changes often accompanying AD include depression, anxiety, fear, and paranoia.Citation52 These symptoms may be observed by family, friends, and caregivers prior to a formal diagnosis; AD pathogenesis is thought to begin decades before the development of behavioral changes.Citation52 Accordingly, recent research suggests that pathological changes in brain and cerebral spinal fluid chemistry may occur up to 25 years prior to cognitive decline.Citation53,Citation54
Key characteristics of AD are aggregation of amyloid-β plaques, neurofibrillary tangles, neuroinflammation, and BBB breakdown.Citation46 Amyloid-β is naturally produced in the brain, first as amyloid precursor protein (APP).Citation55 In a healthy brain, presenilin proteins (PSEN), presenilin-1, and presenilin-2, cleave APP most often into amyloid-β40 (~90%).Citation55,Citation56 Commonly in familial AD, mutations in APP or in PSEN cause cleavage of APP into amyloid-β42. The amyloid-β42 peptide is more hydrophobic and the most common isoform of amyloid-β plaques.Citation55–58 These insoluble peptides aggregate in the brain, forming amyloid-β plaques, and are associated with neurotoxicity.Citation55,Citation57,Citation59 Neurofibrillary tangles caused by breakdown of the stabilizing protein Tau follow amyloid-β plaque onset.Citation29,Citation60 In AD, Tau is hyperphosphorylated, leading these proteins to dissociate from the neuron and tangle on other tau proteins.Citation29,Citation54,Citation60 This causes neurons to collapse and prevents communication between synapses. Despite being well characterized in AD, therapeutics targeting amyloid or tau pathologies have had limited success in human clinical trialsCitation61–63 so understanding how peripheral factors, such as the gut microbiota, contribute to pathology development is critical.
Breakdown in the BBB is implicated in AD and is thought to begin in the hippocampus, which modulates long-term memory, learning, and emotional processing, preceding cognitive decline.Citation49 Compromised integrity of the BBB can allow translocation of toxic materials such as circulating iron into the brain.Citation49,Citation64 BBB integrity is maintained by a network of tight junction proteins including occludin and several claudins.Citation65,Citation66 Damage to the BBB may precede hallmark amyloid-β plaques and tau tangles,Citation47 or may be a consequence of pathological onset.Citation46,Citation49 As few studies have investigated the effect of AD pathologies on tight junction protein and mRNA expression, future research should aim to identify which and how proteins are being compromised due to AD. The AD-associated gut microbiota can drive disease pathologies by increasing inflammation, signaling through the vagus or enteric nervous system, or by compromising gut barrier integrity and allowing microbial products to reach the compromised BBB.Citation28,Citation32,Citation36,Citation67,Citation68
Dysregulation of GABAergic signaling in AD
GABA is a downstream product of the excitatory amino acid, glutamate. Glutamate can be produced in the brain via astrocytes and is also a component of human diets.Citation69–71 The gut and associated microbes have a high capacity for dietary glutamate; it is converted into several important molecules in the gut including proline, arginine, glutamine, and GABA.Citation71,Citation72 The enzyme, glutamate decarboxylase (GAD), catalyzes the transformation of glutamate into GABA.Citation30,Citation73 The GAD gene is found in the genomes of several key AD-associated bacteria including Bacteroides, Bifidobacterium, Alistipes, Blautia, and Lactobacillus.Citation74 In AD patients, low plasma levels of glutamate are associated with lowered ability to name objects, follow directions, and behavioral symptoms.Citation75 Furthermore, human patients and animal models of AD exhibit decreased levels of glutamate in the brain.Citation76,Citation77 By contrast, glutamine, the precursor of glutamate, is found elevated in the plasma of AD patients.Citation78 The glutamate concentration in the gut, as it pertains to AD, has not yet been investigated. Future research in AD should seek to close the knowledge gap existing about glutamate/GABA levels in the gut of AD patients and mouse models.
Correlated with AD development are obesity and diabetes; diseases which can also be affected by microbe-derived GABA or bacterial GAD genes. Supplementation with GABA in mice fed high fat diets promotes fat browning, which is involved in thermoregulation as opposed to energy storage, and regulates lipid metabolism.Citation79,Citation80 Transplantation of fecal material from lean donors into metabolic syndrome recipients has been shown to improve insulin sensitivity, altered microbiome composition, and changes in plasma metabolites including GABA.Citation81 Thus, GABA’s role in AD may be peripheral or may affect both AD and associated comorbidities. In type-1 diabetes, in silico analysis suggests that the gut microbiome may become depleted of bacteria expressing the GAD gene.Citation82 As GAD-expressing bacteria die, they can release bacterial GAD, which mimics human GAD, and is recognized as an antigen in the gut by type-1 diabetes autoantibodies.Citation82 Bacterial GAD then activates submucosal T cells which subsequently destroy pancreatic beta cells expressing the human GAD enzyme.Citation82 As type-1 diabetes is positively correlated with the development of AD,Citation83,Citation84 it is plausible that the dysregulation of the GABAergic system in AD is not due to GABA itself but the genes involved in its production.
Recent studies indicate that GABAergic signaling is dysregulated in AD.Citation85–87 In one study, GABA concentration in AD and age-matched cognitively normal participants demonstrated decreased GABA/creatine ratios in the parietal, but not frontal, region in patients experiencing AD as compared to cognitively normal controls.Citation88 No significant differences were observed, however, between GABA concentration and the results of the Mini Mental Status Examination, suggesting the GABAergic pathway may not be implicated in cognitive manifestations of the disease.Citation88 These results contrast a study on cerebrospinal fluid levels of GABA which found that GABA was more abundant in study participants experiencing mild AD as compared to three other groups ranging from subjective cognitive impairment, used as control, to participants experiencing cognitive impairment which would progress to AD within 2 y.Citation86 These differences may, in part, be attributable to a difference in GABA transport mechanisms as opposed to levels of GABA itself. In postmortem brain tissue analysis of probable and definite AD diagnoses, researchers found region-specific inhibition in GABA reuptake transporter protein expression in the hippocampus, subiculum, entorhinal cortex, and superior temporal gyrus in AD.Citation89 Inhibition of GABAergic signaling has also been studied in several strains of mice modeling AD pathologies.Citation90–92 In hippocampus samples collected from a mouse model of AD, researchers determined that reactive astrocytes, which gather around amyloid-β plaques, produce high levels of GABA, resulting in impaired synaptic plasticity, learning, and memory.Citation93 GABA is further implicated in AD as administration of GABAA receptor antagonists, bicuculline, and/or picrotoxin can remediate cognitive deficits in mouse models of AD pathologies.Citation94 Interestingly, this study demonstrated age-related alterations in the GABAergic pathway regardless of strain, which indicates that changes are an intrinsic part of aging.Citation94 Little is known about the implications of gut-derived GABA on AD disease pathogenesis and its role in the bidirectional communication between the brain and gut in AD.
The gut microbiota is altered AD
The gut microbiome assists the body in digestion, production of neurotransmitters, and microbial metabolites and is a contributor to healthy aging or age-related decline.Citation95 The gut microbiome undergoes dynamic temporal changes throughout the lifespan in response to host and environmental factors.Citation96 An analysis of the gut microbiome of 9,000 individuals between the ages of 18 and 101 demonstrated some interesting patterns associated with health and longevity. One pattern that emerged in healthy aging was depletion of Bacteroides later in life. High abundance of Bacteroides in older adults (over 80) was predictive of decreased survival.Citation96 Aging is also associated with increased uniqueness of the gut microbiome, or high interpersonal variability.Citation95,Citation96 However, it is difficult to generalize patterns across studies because of confounding effects such as variability in study designs, altered diets between elderly and young adults, and living environments. Generally, as humans age into elderly adults, the gut microbiome decreases in diversity and demonstrates taxonomic changes with microbial taxa that are often associated with altered metabolic profiles and disease phenotypes.Citation97–99 In AD, recent literature has consistently demonstrated that altered microbial communities are correlated with disease state (preclinical, mild cognitive impairment, or cognitively impaired AD). Generally, the gut microbiota in patients living with AD is less diverse and taxonomically distinct when compared to age- and sex-matched controls.Citation19,Citation20,Citation100,Citation101 In this review, we focus on the role of Bacteroides and Lactic Acid Bacteria (LAB)Citation102–104 because these taxa have previously been identified as differentially abundant in human and mouse studies of AD and AD pathologies,Citation19,Citation21,Citation22,Citation28 and are the predominant producers of GABA in the human gut microbiome.
Gut microbiome alterations may precede cognitive decline in AD. A recent study identified bacterial taxa associated with preclinical AD, when patients are cognitively normal but biomarkers of AD are present.Citation105 In this study, members of the genus Dorea, Oscillibacter, and Faecalibacterium were enriched in patients with preclinical AD, while members of the genus Bacteroides were more prevalent in healthy participants.Citation105 In another study of individuals with mild AD compared to age- and sex-matched cognitively normal participants, Bacteroides were enriched in AD, and LAB (in this study, Lactococcus) were enriched in healthy participants, independent of dietary factors.Citation19 Mouse studies demonstrate alterations in similar taxa. In a longitudinal study of 3×Tg-AD mice modeling AD pathologies, we found enrichment of Bacteroides species after the onset of amyloid-β plaque formation, and Lactobacillus species were enriched in wild-type control mice at early timepoints,Citation22,Citation28 but this trend is not consistent and is likely dependent on species and strain. A longitudinal study using metagenomic sequencing found that several species of Lactobacillus were enriched in 5×FAD mice, but L. johnsonii were less abundant.Citation28 These findings indicate a potential role for GABA-producing microbiota in AD.
Current strategies targeting the gut microbiota to yield mechanistic insights into AD typically colonize the gut microbiota with species or microbial communities of interest via fecal microbiota transplantation (FMT). Several FMT studies in animal models of AD indicate that the gut microbiome can reverse cognitive decline and reduce disease pathologies, either through direct methods to transfer fecal microbiota or cohousing mice modeling AD with WT controls.Citation106–108 When WT mice are cohoused with 5×FAD mice for the first seven months of life, the gut microbiota was transferred via coprophagy and resembled an intermediate phenotype with some features of a WT and some features of a 5×FAD microbiome.Citation109 This led to a brain transcriptome resembling 5×FAD mice, increased infiltrating Th1 cells into the brain, and activated microglia in co-housed WT mice compared to mice WT caged with their genotype.Citation109 Likewise, a study in which FMT was directly administered from transgenic mice modeling AD pathologies into wild-type mice demonstrated microglia activation and colonic inflammation when compared to the wild-type mice receiving vehicle control or FMT from other wild-type mice.Citation110 In humans, FMT treatment has shown promising results in other neurological disorders, most prominently in studies of children with autismCitation2 and one case study of an AD patient demonstrated rapid improvement in cognitive function following FMT for Clostrium difficile infection.Citation111 Currently, there is no cure or preventative therapeutics for AD, but promising research into the gut microbiome offers the potential for customized therapeutics targeting distinct niches of the gut microbiome.
Bacteroides in AD
Bacteroides are Gram negative, anaerobic bacteria commonly found colonizing the colon. Gram negative bacterial cells express lipopolysaccharide (LPS) on the cell surface, a compound which is known to induce inflammation and can act on tight-junction proteins in the gut through regulation of inflammatory cytokines.Citation2,Citation7,Citation112 In a study of germ-free 3×Tg-AD mice humanized with fecal microbiota from AD and healthy participants, Bacteroides was enriched in mice receiving AD-associated fecal microbiota, and correlated with increased microglial activation.Citation113 Another study, using Thy1-C/EBPβ transgenic mice that overexpress EBPβ, a critical transcription factor for APOE, humanized mice with fecal samples from AD and age-matched donors. They demonstrated increased abundance of Bacteroides fragilis when treated with AD fecal microbiota. Levels of B. fragilis in the gut correlated with elevated serum and brain levels of 12-hydroxy-heptadecatrienoic acid (12-HHTrE) and Prostaglandin E2, metabolites known to stimulate microglia activation. Furthermore, mice treated with AD donor stool demonstrated an increase in brain levels of polyunsaturated fatty acid enzymes, cyclooxygenases, COX-1, and COX-2, which promote prostaglandin E2 synthesis.Citation114 In the brain, COX-1 is expressed predominantly by microglia whereas COX-2 is expressed by hippocampal neurons.Citation114 As Bacteroides are often, but not always, enriched in humans and animal models of AD pathologies, understanding its effects on the brain in AD, especially microglia activation, can help us better understand the bidirectional pathways that lead to its enrichment or depletion in the gut microbiome.
The molecular mechanism underlying Bacteroides effects in AD are not yet known but are likely multifactorial, and may include inflammation due to the release of LPS, alteration of microbiome composition through metabolic cross feeding, and production of GABA. Bacteroides fragilis LPSs are structurally unique and are potent activators of proinflammatory pathways in primary human brain cells in vitro.Citation115,Citation116 LPS administered to CD-1 mice, which are outbred multipurpose mice, via intraperitoneal injection exhibit altered amyloid-β transport at the BBB. This resulted in increased burden of amyloid-β in the brain and increased neuronal expression of LRP-1, a protein which mediates amyloid-β uptake into neurons, astrocytes, and microglia.Citation48 The effects of LPS were likely a consequence of increased inflammation rather than a direct effect on BBB cells; serum levels of IL-6, IL-10, IL-13, and major cationic protein (MCP)-1 were found upregulated and corresponded to amyloid-β influx into the brain.Citation48 Increased LPS production by gut microbes may stimulate local inflammation, altering gut epithelial tight junctions similar to BBB tight junctions, and escape into the bloodstream or interstitium and reach the brain.Citation68,Citation117 Finally, Bacteroides are known producers of the inhibitory neurotransmitter, GABA (discussed in detail below).Citation30 Very little research has been done on microbe-derived neurotransmitter effects on neurodegeneration although there are strong indications of alterations in the GABAergic pathway in AD.Citation88–120 The effects of Bacteroides in the gut may also be indirect, as introduction of one bacteria may modulate the microbial community structure as a whole,Citation121,Citation122 wherein Bacteroides acts as a keystone species in the gut microbial ecosystem.
Lactobacillus in AD
Lactobacillus is a Gram positive, facultative anaerobe, and is commonly studied for its potential for neuroprotection. Lactobacillus is a primary producer of lactic acid. Two enantiomers of lactic acid exist. L-lactic acid is a common host metabolite, but D- and L-lactic acid can be produced by strains of Lactobacillus in the host microbiome.Citation123,Citation124 L-lactic acid is associated with health, but D-lactic acid has been demonstrated to have some toxicity to the host. In the brain, accumulation of D-lactic acid is associated with memory impairment and neuronal inhibition due to competitive blocking of L-lactic acid uptake.Citation125 In addition, similar to Bacteroides, Lactobacillus are emerging as key GABA producersCitation4,Citation9,Citation104 Given that species of Lactobacillus produce both GABA and L-/D-lactic acid, the findings discussed below may be driven by either or both metabolites. Since this review is focused on GABA, we narrowed our discussion to neurotransmitter activity.
Several studies indicate protective effects of taxa within the genus Lactobacillus against neurodegeneration or cognitive deficits.Citation102,Citation122,Citation126,Citation127 The effects of Lactobacillus may be direct or indirect. Supplementation of exogenous Lactobacillus into the gut microbiome can alter the overall microbiome composition, modulate intestinal permeability, and gut epithelial tight junction integrity.Citation102,Citation128 Intervention with exogenous Lactobacillus plantarum PS128 is protective in a 3×Tg-AD mouse model.Citation102 Mice in this study were treated either with L. plantarum PS128 or vehicle control for one month before receiving an intracerebroventricular injection of either streptozotocin (which exacerbates AD pathologies in this model) or vehicle control. Mice receiving L. plantarum PS128 were protected against streptozotocin-mediated damage, characterized by reduced amyloid deposition, tau hyperphosphorylation, gliosis, and astrocytosis compared to the untreated group.Citation102 The authors suggest that gliosis in this model is mediated by fecal propionic acid and GSK3β levels, and that L. plantarum P128 regulates fecal propionic acid.Citation102 In a mouse model of anxiety and depression, administration of Lactobacillus reduced anxiety and depression symptoms which correlated with altered GABA receptor expression in several brain regions including the hippocampus.Citation9 These effects were lost when the vagus nerve, which innervates the gastrointestinal tract, was cut, suggesting afferent vagal signaling as a mode of bidirectional communication between gut microbes and the brain.Citation9 While more research, particularly in human participants, is necessary, exogenous Lactobacillus may confer a health benefit in neurological disease.
Endogenous Lactobacilli can also have a role in health status. Borsom et al. demonstrated depletion of Lactobacillus salivarius in mice modeling AD pathologies prior to pathology development, possibly indicating that gut microbiota at a preclinical stage may play an important role in neuroprotection.Citation22 Another study demonstrated depletion of L. johnsonii, but enrichment of Lactobacillus P38 in 5×FAD mice compared to controls.Citation28 In AD, it is conceivable that increased production of GABA and/or lactic acid L- and D-enantiomers by Lactobacillus during preclinical stages of disease inhibits vagus nerve stimulation or otherwise affects gut epithelial tight junctions and that this may have downstream effects on disease pathogenesis.
Tight junction protein regulation by the microbiome
The integrity of the intestinal epithelial layer is carefully maintained through host- and microbe-derived metabolites and cytokines. In a healthy gut ecosystem, the gut epithelial cells do not come into direct contact with the microbes due to a highly glycosylated mucus layer secreted by goblet cells. A compromised epithelial barrier can allow translocation of metabolites that otherwise would not cross this highly regulated barrier.
Several gut taxa within the genera Akkermansia, Bacteroides, Prevotella, Clostridium, and Streptomyces are known degraders of mucins, meaning they can use mucin as a carbon source and this thins the gut mucus layer.Citation129–131 In a healthy gut ecosystem, gut bacteria mediate a dynamic equilibrium of the gut mucus layer which prevents direct contact with gut epithelial cells but allows movement of microbe-derived products to the host.Citation132 While each of the aforementioned taxa can use mucins as their carbon/nitrogen sources, complete degradation of mucin relies on a diverse community of microbiota and associated mucin-degrading enzymes, collectively known as “mucinases”.Citation129 In a disease-associated gut microbiome, enrichment of mucin-degrading bacteria could plausibly degrade the mucus layer faster than it is replenished and damage the gut epithelial cells or associated tight junction proteins.
The tight junction is composed of several transmembrane proteins, including claudins, occludins, and zonula occludens, which interact to maintain epithelial barrier integrity.Citation133 Mice with knockout tight junction proteins such as claudins and occludins show severe immune infiltration and chronic inflammation along with hyperplasia of the gastric epithelium in the occludin knockout mice,Citation134 and claudin-7 knockout mice died in the perinatal period.Citation135 These results underscore the critical role that these proteins play in gut barrier functioning and the consequences of their dysregulation. Tight junction protein dysregulation is implicated in myriad gut and neurological disorders including metabolic disorders, inflammatory bowel disease, Crohn’s disease, and autism spectrum disorder (ASD).Citation136–138 The role of intestinal barrier function in AD is less well understood. Loss of intestinal barrier integrity has been observed in 5×FAD and APP/PS1 models of AD pathologies,Citation36,Citation45 and in some small human studies of preclinical AD and dementia.Citation139 Gut microbiota play a critical role in maintaining barrier integrity. In a mouse model of ASD, researchers challenged mice with B. fragilis every other day for 6 d immediately after weaning.Citation2 Results from this study indicate that B. fragilis corrects intestinal barrier integrity and restores tight junction proteins. B. fragilis also corrected behavioral deficits in mice modeling features of ASD.Citation2 Taken together, these data suggest that B. fragilis may modulate the gut-microbiome-brain axis through regulation of tight-junctions proteins and related cytokines and/or through the regulation of other enteric microbiota which then influence expression of tight junctions and inflammatory cytokines.
The BBB, which maintains the delicate influx of necessary nutrients into the brain while blocking neurotoxic proteins, metals, immune cells, and microbes, is also compromised in AD.Citation40,Citation46,Citation49,Citation66 The BBB, similar to the gut, is maintained by tight junction proteins, claudins and occludins.Citation39,Citation65 BBB degeneration is found even in healthy brains as people age, but degeneration is accelerated in AD and other progressive, neurodegenerative diseases.Citation49 This may be due to infiltration of blood-born macrophages which appear to be recruited by neuronal cells experiencing amyloid-β aggregation.Citation40 Here, we explore whether compromised intestinal and BBB integrity may constitute a mechanism by which the gut microbiome affects AD.
Emerging evidence suggests that the gut microbiome and intestinal barrier integrity are altered in AD.Citation36,Citation45,Citation139 Studies have demonstrated decreased gut tight junction protein integrity measured by decreased levels of occludin, ZO-1, and claudin1 mRNA and protein expression in humans and mouse models of AD.Citation36,Citation140 These observations led us to explore this as a potential mechanism contributing to disease progression. A compromised tight-junction layer in the gut epithelium could allow translocation of gut- and host-derived metabolites which, in turn, act on the brain. A compromised BBB integrity may then expose the brain and associated inflammatory cells to an exogenous, gut derived metabolic milieu. Thus, damage to tight junctions in the gut epithelial layer may be an unseen pathology of neurodegenerative diseases.
Effect of Bacteroides on barrier integrity
Several studies investigating the role of the gut microbiota in neurological diseases point to the genus, Bacteroides, as a potential neuroactive member of the gut microbiome.Citation2,Citation141 Bacteroides can act through several mechanisms, including production and translocation of LPS from the lumen to the bloodstream, in the context of a damaged epithelial barrier.Citation8,Citation142 LPS in the bloodstream, a condition known as metabolic endotoxemia,Citation143 is known to stimulate inflammation of both the gut and peripheral organs, including the brain.Citation7,Citation8,Citation112,Citation117 The role of Gram negative, LPS-producing bacteria remains controversial as other research, examining the role of Bacteroides fragilis in mice modeling Autism Spectrum Disorder (ASD), indicates Bacteroides remediates damage to gut epithelial tight-junctions through regulation of claudins (CLDNs 8 and 15).Citation2 Bacteroides can use gut mucins as a carbon source.Citation144 In the context of a healthy microbiome, this can help maintain a healthy dynamic equilibrium between mucin production and degradation, but in the context of disease could lead to direct interaction with the epithelial layer and compromise barrier integrity.Citation35,Citation130 The specific effect of Bacteroides in AD is poorly understood and is likely strain-specific. Implications of Bacteroides regulation of intestinal barrier integrity in AD may be beneficial, increasing expression of tight junction proteins, or detrimental through degradation of the mucosal layer. Additional research is needed to characterize the strain-specific effects of Bacteroides on gut barrier integrity in AD.
Effect of Lactobacillus on barrier integrity
Lactobacillus are known to confer benefits to human hosts through resistance to pathogenic invasion,Citation145 gastrointestinal motility and mucin production,Citation146 tight junction regulation,Citation128,Citation147,Citation148 and neurotransmitter activity.Citation4,Citation102,Citation104,Citation146 Several studies indicate that administration of Lactobacillus improves gut barrier integrity through an increase in gene and protein expression of occludin, a tight junction protein important in gut barrier stability and function.Citation128,Citation147,Citation149,Citation150 Administration of Lactobacillus plantarum MB452 to cultured human colon cancer cells altered 19 genes involved in the tight junction regulation pathway.Citation149 In particular, gene expression of occludin and occludin-associated plaque proteins, ZO-1, ZO-2, and cingulin were increased and this was associated with increased barrier integrity.Citation149 Interestingly, of the 19 genes that were altered in the tight junction pathway, 2 of those genes, ITCH, and SNAI1, were involved in tight junction disassembly, suggesting that L. plantarum MB452 modulates the tight junction pathway as opposed to a single gene or protein.Citation149
Lactobacillus is further implicated in gut health through modulation of gut mucin production, especially MUC-2,Citation146,Citation151 the dominant mucin in the gut. In mice modeling increased amyloid-β aggregation and cerebral amyloid pathology, mice at 6 months of age demonstrated decreased mucus maturation in comparison to an age-matched WT cohort but this same difference was not observed in mice with advanced pathologies (15 months)41, suggesting that shifts in gut mucus integrity may precede and potentially dictate future pathological onset. Furthermore, symptomatic mice in this study had an increased abundance of Lactobacillus in comparison to age-matched WT controls and this was not observed at the baseline stage.Citation41 The potentially protective effects of Lactobacillus or other LAB are not well understood in AD. Further research needs to be done to evaluate their potential in modulating the gut epithelial barrier in preclinical stages of AD.
GABA production by the gut microbiome; effects on mucosal barrier integrity, vagus, and enteric nerve stimulation, and neuroinflammation
Neurotransmitters produced by the gut microbiota are important mediators of the gut-microbiome-brain axis, and this is emerging as a field of “microbial endocrinology”.Citation3,Citation152 Gut microbiome-derived neurotransmitter signaling pathways that are hypothesized to act along the gut-microbiome-brain axis include tryptophan metabolism, which affects both the serotonin and kynurenine pathways,Citation1,Citation3,Citation153 and the GABAergic pathway,Citation3,Citation9,Citation14,Citation30,Citation154 which inhibits excitation in the central nervous system. We focus here on microbial influence on GABAergic signaling; microbial modulation of other neurotransmitters and signaling pathways have been reviewed in.Citation155,Citation156 Several bacterial taxa are known producers of molecules implicated in the GABAergic pathway, including glutamate and GABA itself ().Citation3 GABA and glutamate producing taxa encode Glutamate decarboxylase, which converts glutamate into GABA, and putrescine aminotransferase, which catalyzes the reaction that produces L-glutamate, the main excitatory neurotransmitter and precursor for GABA (see for references). The local effects of GABA in the gut of people living with AD and animals modeling AD pathologies is poorly understood, although in vitro research in healthy gut cell lines indicates that GABA can significantly increase the expression of mucins.Citation31 Other studies in live mice and cultured human colon cancer cells suggest that increased GABA may damage the mucus layer.Citation33,Citation157 Studies investigating the role of GABA on healthy animals or tissue indicate beneficial effects of GABA such as immune system regulation and increased intestinal integrity.Citation31,Citation158 Other studies in disease models such as colitis indicate that GABA may negatively affect host health through damage to the mucus layer.Citation33,Citation157 Damage to the mucus layer allows closer proximity of microbes and their metabolic byproducts to the gut epithelial layer, compromising tight junction proteins,Citation33,Citation34 and stimulating epithelial and systemic inflammation (). Identifying the disease-specific role of GABA in AD will further our understanding of the bidirectional cross talk of the gut-microbiome-brain axis.
Figure 1. The gut mucus layer in AD is damaged by increased GABA-producing microbes in the context of a disease-associated microbiome. Damage to the mucus allows closer proximity of microbes and their metabolites to gut epithelial cells, damaging tight junction proteins and stimulating inflammation.
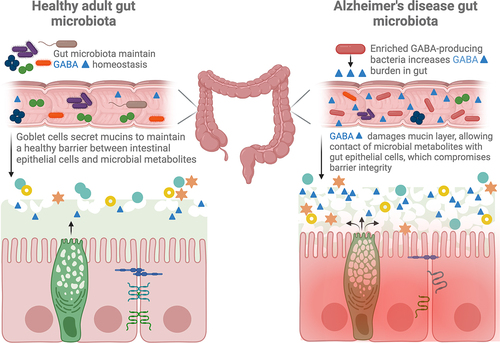
Table 1. GABA-producing microbiota with relevance to AD.
So far, few studies have investigated the interactions between GABA and tight junction protein expression as it pertains to AD. Gene and protein expression of claudin-5, one of the dominant tight junction proteins in the brain, is decreased in AD, and associated with cognitive decline.Citation32 Cognitive decline is associated with decreased long-term potentiation of synaptic efficacy in the hippocampus, which is the means by which the brain is thought to strengthen synaptic connection and store memories.Citation159 Knockout heterozygous claudin-5± mice demonstrate impaired long-term potentiation due to enhanced GABAergic transmission.Citation32 Administration of a GABAA receptor antagonist in claudin-5± mice remediates changes in long-term potentiation which further supports a causal role of claudin-5 mediated GABAergic transmission.Citation32
GABA in the colon may act entirely differently than in the brain. In humans and mouse models of dextran sulfate sodium (DSS)-induced colitis, several studies indicate that GABA administration damages the mucus barrier of the colon, reduces tight junction protein expression, increases gut epithelial permeability, and induces colonic inflammation.Citation33,Citation157 Furthermore, GABA inhibited colonic epithelial cell proliferation when bound to GABAA receptors,Citation33,Citation157 and previous research suggests that increased GABA receptor expression correlates with increased epithelial inflammation.Citation157 While more research is needed to understand GABA’s effects on tight junction gene and protein expression in AD, these results underscore the interconnected nature of GABA and intestinal integrity.
Exogenously produced GABA by gut microbes may also act on the intestinal microenvironment and signal via afferent vagus nerve or enteric nerve pathways. Vagus nerve stimulation (VNS), which is currently used in the treatment of epilepsy, may be beneficial in AD. Vargas-Caballero et al. propose VNS for treatment of AD as its anti-inflammatory effects may modulate neuroinflammation.Citation160 Evidence from animal and clinical studies show that VNS stimulation transiently increases GABA, serotonin, dopamine, and norepinephrine in the CSF and various brain regions including the hippocampus.Citation67,Citation161,Citation162 In some studies, increased GABA or GABA receptor density following VNS is associated with improved neuroplasticity or behavior,Citation161,Citation162 but the number of studies assessing the exact mechanism are limited. Further, no studies have measured whether VNS changes the quantity of GABA in the gut. One study, however, demonstrated that vagotomy can increase GABA production in the gut.Citation163 Zou and colleagues investigated the effect of vagotomy on susceptibility to intestinal infection and anxiety-like behaviors in a mouse model. In their study, vagotomy decreased the susceptibility to infection with Salmonella, reduced anxiety-like behaviors, and increased GABA production and GABA-producing microbes in the gut.Citation163 Enrichment of GABA-producing bacteria in the gut of AD patients and mice modeling AD pathologies may inhibit VNS thus upregulating neuroinflammation (). If gut microbes enriched in AD such as Bacteroides and Lactobacillus are effective at antagonizing VNS, gut microbial therapeutics may aim to reduce their abundance in the gut or recolonize the gut with more stimulatory microbes. The specific effects of the role of the vagus nerve in relation to microbe-derived GABA are discussed below, with a specific focus on Bacteroides and Lactobacillus.
Figure 2. GABA-producing bacteria enriched in the disease-associated gut microbiome increase the local concentration of GABA. GABA inhibits the vagus nerve in the gut and afferent signaling to the brain downregulates the release of norepinephrine. Norepinephrine is responsible for inhibiting microglia activation and subsequent microgliosis.
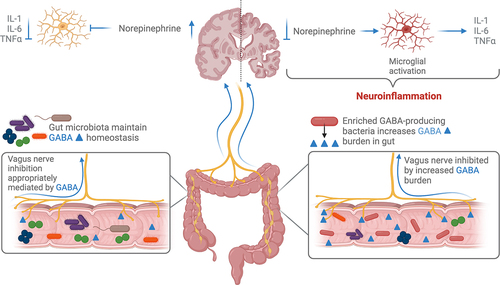
The enteric nervous system (ENS) is composed of enteric neurons and enteric glial cells, and regulates GI motility, secretions, and the enteric immune system.Citation164,Citation165 Within the ENS, approximately 5–8% of the myenteric neurons, which regulate GI motility and mucosal function, contain GABA.Citation166,Citation167 In these enteric neuronal cells, GABA is likely involved in secretory and motor GI function.Citation165 Enteric glial cells, which have similar function to the glial cells in the central nervous system, contribute to regulation of inflammation in the intestines. However, the role of intestinal GABA on regulating enteric glial cell function has not yet been extensively studied. A recent study found that enteric glial cells do express GABA signaling receptors, and that administration of GABA inhibits the pro-inflammatory NF-kB pathway in enteric glial cells in a model of intestinal inflammation.Citation168 Microbe-derived GABA may well influence the function of the ENS and regulation of inflammation in the intestines, but future research is needed in this exciting but nascent field.
Bacteroides production of GABA
Bacteroides are the predominant producers of GABA in the human gut. In a screen of 961 Bacteroides genomes, 95% of the human gut isolates of Bacteroides were found to contain the GAD genes, enabling Bacteroides to transform glutamate into GABA ().Citation30 The same study evaluated in vitro production of GABA and found 16 distinct species of Bacteroides produced GABA at concentrations ranging from 0.09 to 60.84 mM.Citation30 Functionally, GABA may contribute to acid stress tolerance for Bacteroides and has been implicated in metabolic cross-feeding in the gut microbiota with GABA consuming microbes.Citation30,Citation169 As discussed above, the composition of the gut microbiome is altered in AD, and Bacteroides is frequently identified as differentially abundant in disease. In the context of a disease-associated microbiome, it’s possible that GABA production by Bacteroides is also altered. If GABA is increased in a diseased state, the epithelial layer may be more susceptible to damage through GABA-mediated disruption of the mucus layer. Thus, GABA production by Bacteroides may be a double-edged sword in AD, as increased GABA signaling to the brain positively affects cognitive symptoms and molecular pathologies,Citation67 but also disrupt the gut mucus layer and compromise the gut epithelial integrity.Citation33,Citation157
Lactobacillus production of GABA
LAB are also predominant producers of GABA, including the species in the genus Lactobacillus .Citation170 Many species in the genus Lactobacillus have been studied for their beneficial effects on host health in a variety of diseases and conditions.Citation4,Citation104,Citation145,Citation150,Citation171 In the gut-microbiome-brain axis, the majority of research has focused on exogenous Lactobacillus mediated GABAergic signaling in Major Depressive Disorder (MDD).Citation4,Citation9,Citation104,Citation141,Citation142 In these studies, Lactobacillus administration in animal models of MDD characteristics show behavioral changes including reduced anxiety, depression,Citation4,Citation9,Citation104 reduced inflammation,Citation170 and improved metabolism.Citation104 In one study, GABA producing L. brevis reduced depressive-like behavior and corticosterone production, which correlated with increased intestinal GABA concentration, in a mouse model of metabolic syndrome.Citation104 In another study, this time in healthy mice, treatment with GABA producing L. rhamnosus induced region-specific changes in GABAA and GABAB receptors in the hippocampus, amygdala, and locus coeruleus in the brain, reduced corticosterone production, and reduced depressive-like behavior.Citation9 Subdiaphragmatic vagotomy reversed the protective effects of L. rhamnosus on depression and anxiety-like behaviors and expression of GABAA and GABAB receptors, highlighting the role of the vagus nerve in the gut-microbiome-brain axis.Citation9 Studies using exogenous GABA producing Lactobacillus administration are beginning to emerge in AD literature. One such study suggested that administration of Lactobacillus prior to development of pathologies, when other studies in mice have shown Lactobacillus to be depleted in mice modeling AD pathologies,Citation22,Citation28 can delay or reduce pathology burden.Citation126 Species in the genus Lactobacillus are emerging as key players in the gut-microbiome-brain axis. In the future, Lactobacilli may be leveraged to target the gut microbiome via GABAergic signaling pathways early in AD.
Conclusion
In this review, we explored potential effects of microbe-derived GABA epithelial barrier integrity as a potential mechanism underlying the gut-microbiome-brain axis in AD. We highlight the contribution of gut microbial communities for their role in gut barrier integrity and neurotransmitter synthesis. Emerging evidence suggests that AD, a complex neurodegenerative disease, is associated with preclinical changes in the gut microbiome, potentially allowing damage to gut epithelial cells and an altered neurotransmitter profile. Increased GABAergic microbes in the gut after AD onset may be the body’s attempt to increase ambient GABA. This however, may be a double-edged sword, as increased GABA may also increase gut permeability, allowing translocation of harmful microbial products across the gut and BBB. This temporal interaction between tight junctions in the gut and brain may be, in part, mediated by microbial metabolites such as GABA. To date, little research has been conducted on the effects of GABA on the mucin layer and tight junction integrity in AD. Future research should seek to understand the disease-specific mechanisms by which microbial derived GABA interacts with gut barrier integrity and AD.
Author contributions
KAC conceived and wrote the manuscript, EMB and EKC wrote and edited the manuscript.
Disclosure statement
No potential conflict of interest was reported by the author(s).
Additional information
Funding
References
- O’Mahony SM, Clarke G, Borre YE, Dinan TG, Cryan JF. Serotonin, tryptophan metabolism and the brain-gut-microbiome axis. Behav Brain Res. 2015;277:32–20. doi:10.1016/j.bbr.2014.07.027.
- Hsiao EY, McBride S, Hsien S, Sharon G, Hyde E, McCue T, Codelli J, Chow J, Reisman S, Petrosino J, et al. Microbiota modulate behavioral and physiological abnormalities associated with neurodevelopmental disorders. Cell. 2013;155(7):1451–1463. doi:10.1016/j.cell.2013.11.024.
- Mazzoli R, Pessione E. The neuro-endocrinological role of microbial glutamate and GABA signaling. Front Microbiol. 2016;7:1934. doi:10.3389/fmicb.2016.01934.
- Tette F-M, Kwofie SK, Wilson MD. Therapeutic anti-depressant potential of Microbial GABA Produced by Lactobacillus rhamnosus Strains for GABAergic Signaling Restoration and Inhibition of Addiction-Induced HPA Axis Hyperactivity. Curr Issues Mol Biol. 2022;44(4):1434–1451. doi:10.3390/cimb44040096.
- Sun B, Halabisky B, Zhou Y, Palop JJ, Yu G, Mucke L, Gan L. Imbalance between GABAergic and Glutamatergic Transmission Impairs Adult Neurogenesis in an Animal Model of Alzheimer’s Disease. Cell STEM Cell. 2009;5:624–633. doi:10.1016/jotem.2009.10.003.
- Mokhtari P, Holzhausen EA, Chalifour BN, Schmidt KA, Babaei M, Machle CJ, Adise S, Alderete TL, Goran MI. Associations between dietary sugar and fiber with infant gut microbiome colonization at 6 mo of age. The J Nutr. 2024;154(1):152–162. doi:10.1016/j.tjnut.2023.09.009.
- Hu R, He Z, Liu M, Tan J, Zhang H, Hou D-X, He J, Wu S. Dietary protocatechuic acid ameliorates inflammation and up-regulates intestinal tight junction proteins by modulating gut microbiota in LPS-challenged piglets. J Anim Sci Biotechnol. 2020;11(1):92. doi:10.1186/s40104-020-00492-9.
- Zhang J, Ma L, Chang L, Pu Y, Qu Y, Hashimoto K. A key role of the subdiaphragmatic vagus nerve in the depression-like phenotype and abnormal composition of gut microbiota in mice after lipopolysaccharide administration. Transl Psychiatry. 2020;10(1):186. doi:10.1038/s41398-020-00878-3.
- Bravo JA, Forsythe P, Chew MV, Escaravage E, Savignac HM, Dinan TG, Bienenstock J, Cryan JF. Ingestion of Lactobacillus strain regulates emotional behavior and central GABA receptor expression in a mouse via the vagus nerve. Proc Natl Acad Sci USA. 2011;108(38):16050–16055. doi:10.1073/pnas.1102999108.
- Kaczmarczyk R, Tejera D, Simon BJ, Heneka MT. Microglia modulation through external vagus nerve stimulation in a murine model of Alzheimer’s disease. J Neurochem. 2017; doi:10.1111/jnc.14284.
- Chung H, Pamp S, Hill J, Surana N, Edelman S, Troy E, Reading N, Villablanca E, Wang S, Mora J, et al. Gut immune maturation depends on colonization with a host-specific microbiota. Cell. 2012;149(7):1578–1593. doi:10.1016/j.cell.2012.04.037.
- De Palma G, Nadal I, Medina M, Donat E, Ribes-Koninckx C, Calabuig M, Sanz Y. Intestinal dysbiosis and reduced immunoglobulin-coated bacteria associated with coeliac disease in children. BMC Microbiol. 2010;10(1):63. doi:10.1186/1471-2180-10-63.
- Ganesh BP, Klopfleisch R, Loh G, Blaut M, Ryffel B. Commensal Akkermansia muciniphila exacerbates gut inflammation in Salmonella Typhimurium-infected gnotobiotic mice. PLOS ONE. 2013;8(9):e74963. doi:10.1371/journal.pone.0074963.
- Ahmed H, Leyrolle Q, Koistinen V, Kärkkäinen O, Layé S, Delzenne N, Hanhineva K. Microbiota-derived metabolites as drivers of gut–brain communication. Gut Microbes. 2022;14(1):2102878. doi:10.1080/19490976.2022.2102878.
- Øyri SF, Műzes G, Sipos F. Dysbiotic gut microbiome: A key element of Crohn’s disease. Comp Immunol Microbiol Infect Dis. 2015;43:36–49. doi:10.1016/j.cimid.2015.10.005.
- Frank DN, St. Amand AL, Feldman RA, Boedeker EC, Harpaz N, Pace NR. Molecular-phylogenetic characterization of microbial community imbalances in human inflammatory bowel diseases. Proc Natl Acad Sci USA. 2007;104(34):13780–13785. doi:10.1073/pnas.0706625104.
- Petrov VA, Saltykova IV, Zhukova IA, Alifirova VM, Zhukova NG, Dorofeeva YB, Tyakht AV, Kovarsky BA, Alekseev DG, Kostryukova ES, et al. Analysis of Gut Microbiota in Patients with Parkinson’s Disease. Bull Exp Biol Med. 2017;162(6):734–737. doi:10.1007/s10517-017-3700-7.
- Lai F, Jiang R, Xie W, Liu X, Tang Y, Xiao H, Gao J, Jia Y, Bai Q. Intestinal Pathology and Gut Microbiota Alterations in a Methyl-4-phenyl-1,2,3,6-tetrahydropyridine (MPTP) Mouse Model of Parkinson’s Disease. Neurochem Res. 2018;43(10):1986–1999. doi:10.1007/s11064-018-2620-x.
- Vogt NM, Kerby RL, Dill-McFarland KA, Harding SJ, Merluzzi AP, Johnson SC, Carlsson CM, Asthana S, Zetterberg H, Blennow K, et al. Gut microbiome alterations in Alzheimer’s disease. Sci Rep. 2017;7(1):13537. doi:10.1038/s41598-017-13601-y.
- Ling Z, Zhu M, Yan X, Cheng Y, Shao L, Liu X, Jiang R, Wu S. Structural and functional dysbiosis of fecal microbiota in chinese patients with alzheimer’s disease. Front Cell Dev Biol. 2021;8:634069. doi:10.3389/fcell.2020.634069.
- Haran JP, Bhattarai SK, Foley SE, Dutta P, Ward DV, Bucci V, McCormick BA, et al. Alzheimer’s disease microbiome is associated with dysregulation of the anti-inflammatory p-glycoprotein pathway. MBio. 2019;10(3). doi:10.1128/mBio.00632-19.
- Borsom Emily M, Conn K, Keefe CR, Herman C, Orsini GM, Hirsch AH, Palma Avila M, Testo G, Jaramillo SA, Bolyen E, et al. Predicting neurodegenerative disease using prepathology gut microbiota composition: a longitudinal study in mice modeling alzheimer’s disease pathologies. Microbiol Spectr. 2023;11(2):e03458–22. doi:10.1128/spectrum.03458-22.
- Alzheimer’s Disease Facts and Figures. Alzheimer’s Disease And Dementia. https://www.alz.org/alzheimers-dementia/facts-figures.
- 2023 Alzheimer’s disease facts and figures. Alzheimers Dement. 2023;19(4):1598–1695. doi:10.1002/alz.13016.
- Sampson TR, Debelius JW, Thron T, Janssen S, Shastri GG, Ilhan ZE, Challis C, Schretter CE, Rocha S, Gradinaru V, et al. Gut Microbiota Regulate Motor Deficits and Neuroinflammation in a Model of Parkinson’s Disease. Cell. 2016;167(6):1469–1480.e12. doi:10.1016/j.cell.2016.11.018.
- Chen Y, Fang L, Chen S, Zhou H, Fan Y, Lin L, Li J, Xu J, Chen Y, Ma Y, et al. Gut Microbiome Alterations Precede Cerebral Amyloidosis and Microglial Pathology in a Mouse Model of Alzheimer’s Disease. Biomed Res Int. 2020;2020:1–15. doi:10.1155/2020/8456596.
- Borsom EM, Conn KA, Keefe CR, Hirsch AH, Orsini GM, Jaramillo SA, Testo G, Avila MP, Bolyen EK, Dillon MR, et al. Predicting neurodegenerative disease using the unique gut microbiota composition of transgenic mice modeling amyloid‐β plaques and neurofibrillary tangles. Alzheimers Dement. 2022;18(S5). doi:10.1002/alz.066299.
- Dunham SJB, McNair KA, Adams ED, Avelar-Barragan J, Forner S, Mapstone M, Whiteson KL. Longitudinal Analysis of the Microbiome and Metabolome in the 5xfAD Mouse Model of Alzheimer’s Disease. MBio. 2022;13(6):e0179422. doi:10.1128/mbio.01794-22.
- Levenga J, Krishnamurthy P, Rajamohamedsait H, Wong H, Franke TF, Cain P, Sigurdsson EM, Hoeffer CA. Tau pathology induces loss of GABAergic interneurons leading to altered synaptic plasticity and behavioral impairments. Acta Neuropathol Commun. 2013;1(1):34. doi:10.1186/2051-5960-1-34.
- Otaru N, Ye K, Mujezinovic D, Berchtold L, Constancias F, Cornejo FA, Krzystek A, de Wouters T, Braegger C, Lacroix C, et al. GABA Production by Human Intestinal Bacteroides spp.: Prevalence, Regulation, and Role in Acid Stress Tolerance. Front Microbiol. 2021;12:656895. doi:10.3389/fmicb.2021.656895.
- Braun H-S, Sponder G, Pieper R, Aschenbach JR, Deiner C. GABA selectively increases mucin-1 expression in isolated pig jejunum. Genes Nutr. 2015;10(6):47. doi:10.1007/s12263-015-0497-8.
- Zhu N, Wei M, Yuan L, He X, Chen C, Ji A, Zhang G. Claudin-5 relieves cognitive decline in Alzheimer’s disease mice through suppression of inhibitory GABAergic neurotransmission. Aging. 2022;14(8):3554–3568. doi:10.18632/aging.204029.
- Ma X, Sun Q, Sun X, Chen D, Wei C, Yu X, Liu C, Li Y, Li J. Activation of GABAA Receptors in Colon Epithelium Exacerbates Acute Colitis. Front Immunol. 2018;9:987. doi:10.3389/fimmu.2018.00987.
- Qu S, Zheng Y, Huang Y, Feng Y, Xu K, Zhang W, Wang Y, Nie K, Qin M. Excessive consumption of mucin by over-colonized Akkermansia muciniphila promotes intestinal barrier damage during malignant intestinal environment. Front Microbiol. 2023;14:1111911. doi:10.3389/fmicb.2023.1111911.
- Glover JS, Ticer TD, Engevik MA. Characterizing the mucin-degrading capacity of the human gut microbiota. Sci Rep. 2022;12(1):8456. doi:10.1038/s41598-022-11819-z.
- He J, Liu Y, Li J, Zhao Y, Jiang H, Luo S, He G. Intestinal changes in permeability, tight junction and mucin synthesis in a mouse model of Alzheimer’s disease. Int J Mol Med. 2023;52(6). doi:10.3892/ijmm.2023.5316.
- Thwaites DT, Basterfield L, McCleave PM, Carter SM, Simmons NL. Gamma-Aminobutyric acid (GABA) transport across human intestinal epithelial (Caco-2) cell monolayers. Br J Pharmacol. 2000;129(3):457–464. doi:10.1038/sj.bjp.0703069.
- Anderson JM, Van Itallie CM. Physiology and function of the tight junction. Cold Spring Harb Perspect Biol. 2009;1(2):a002584. doi:10.1101/cshperspect.a002584.
- Sugiyama S, Sasaki T, Tanaka H, Yan H, Ikegami T, Kanki H, Nishiyama K, Beck G, Gon Y, Okazaki S, et al. The tight junction protein occludin modulates blood–brain barrier integrity and neurological function after ischemic stroke in mice. Sci Rep. 2023;13(1):2892. doi:10.1038/s41598-023-29894-1.
- Fiala M, Liu QN, Sayre J, Pop V, Brahmandam V, Graves MC, Vinters HV. Cyclooxygenase-2-positive macrophages infiltrate the Alzheimer’s disease brain and damage the blood–brain barrier. Eur J Clin Invest. 2002;32(5):360–371. doi:10.1046/j.1365-2362.2002.00994.x.
- Honarpisheh P, Reynolds CR, Blasco Conesa MP, Moruno Manchon JF, Putluri N, Bhattacharjee MB, Urayama A, McCullough LD, Ganesh BP. Dysregulated Gut Homeostasis Observed Prior to the Accumulation of the Brain Amyloid-β in Tg2576 Mice. IJMS. 2020;21(5):1711. doi:10.3390/ijms21051711.
- Dheer R, Davies JM, Quintero MA, Damas OM, Deshpande AR, Kerman DH, Sawyer WP, Pignac-Kobinger J, Ban Y, Fernandez I, et al. Microbial Signatures and Innate Immune Gene Expression in Lamina Propria Phagocytes of Inflammatory Bowel Disease Patients. Cell Mol Gastroenterol Hepatol. 2020;9(3):387–402. doi:10.1016/j.jcmgh.2019.10.013.
- Wikoff WR, Anfora AT, Liu J, Schultz PG, Lesley SA, Peters EC, Siuzdak G. Metabolomics analysis reveals large effects of gut microflora on mammalian blood metabolites. Proc Natl Acad Sci USA. 2009;106(10):3698–3703. doi:10.1073/pnas.0812874106.
- Fuke N, Yamashita T, Shimizu S, Matsumoto M, Sawada K, Jung S, Tokuda I, Misawa M, Suzuki S, Ushida Y, et al. Association of Plasma Lipopolysaccharide-Binding Protein Concentration with Dietary Factors, Gut Microbiota, and Health Status in the Japanese General Adult Population: A Cross-Sectional Study. Metabolites. 2023;13(2):250. doi:10.3390/metabo13020250.
- Shukla PK, Delotterie DF, Xiao J, Pierre JF, Rao R, McDonald MP, Khan MM. Alterations in the Gut-Microbial-Inflammasome-Brain Axis in a Mouse Model of Alzheimer’s Disease. Cells. 2021;10(4):779. doi:10.3390/cells10040779.
- Blair LJ, Frauen HD, Zhang B, Nordhues BA, Bijan S, Lin Y-C, Zamudio F, Hernandez LD, Sabbagh JJ, Selenica MLB, et al. Tau depletion prevents progressive blood-brain barrier damage in a mouse model of tauopathy. Acta Neuropathol Commun. 2015;3(1). doi:10.1186/s40478-015-0186-2.
- Ujiie M, Dickstein DL, Carlow DA, Jefferies WA. Blood—Brain Barrier Permeability Precedes Senile Plaque Formation in an Alzheimer Disease Model. Microcirculation. 2003;10(6):463–470. doi:10.1038/sj.mn.7800212.
- Jaeger LB, Dohgu S, Sultana R, Lynch JL, Owen JB, Erickson MA, Shah GN, Price TO, Fleegal-Demotta MA, Butterfiled DA, et al. Lipopolysaccharide alters the blood–brain barrier transport of amyloid β protein: A mechanism for inflammation in the progression of Alzheimer’s disease. Brain Behav Immun. 2009;23(4):507–517. doi:10.1016/j.bbi.2009.01.017.
- Montagne A, Barnes S, Sweeney M, Halliday M, Sagare A, Zhao Z, Toga A, Jacobs R, Liu C, Amezcua L, et al. Blood-brain barrier breakdown in the aging human hippocampus. Neuron. 2015;85(2):296–302. doi:10.1016/j.neuron.2014.12.032.
- alzheimers-facts-and-figures.pdf.
- Wolters FJ, Chibnik LB, Waziry R, Anderson R, Berr C, Beiser A, Bis JC, Blacker D, Bos D, Brayne C, et al. Twenty-seven-year time trends in dementia incidence in Europe and the United States: The Alzheimer Cohorts Consortium. Neurology. 2020;95(5):e519–e531. doi:10.1212/WNL.0000000000010022.
- Alzheimer’s Association. 2019 Alzheimer’s disease facts and figures. Alzheimers & Dementia. 2019;15(3):321–387. doi:10.1016/j.jalz.2019.01.010.
- Beason-Held LL, Goh JO, An Y, Kraut MA, O’Brien RJ, Ferrucci L, Resnick SM. Changes in brain function occur years before the onset of cognitive impairment. J Neurosci. 2013;33(46):18008–18014. doi:10.1523/JNEUROSCI.1402-13.2013.
- Bateman RJ, Xiong C, Benzinger TLS, Fagan AM, Goate A, Fox NC, Marcus DS, Cairns NJ, Xie X, Blazey TM, et al. Clinical and biomarker changes in dominantly inherited Alzheimer’s disease. N Engl J Med. 2012;367(9):795–804. doi:10.1056/NEJMoa1202753.
- Murphy MP, LeVine H 3rd, Lovell MA. Alzheimer’s Disease and the Amyloid-β Peptide. J Alzheimers Dis. 2010;19(1):311–323. doi:10.3233/JAD-2010-1221.
- Gu L, Guo Z. Alzheimer’s aβ42 and Aβ40 peptides form interlaced amyloid fibrils. J Neurochem. 2013;126(3):305–311. doi:10.1111/jnc.12202.
- Murphy MP, Hickman LJ, Eckman CB, Uljon SN, Wang R, Golde TE. γ-Secretase, Evidence for Multiple Proteolytic Activities and Influence of Membrane Positioning of Substrate on Generation of Amyloid β Peptides of Varying Length. J Biol Chem. 1999;274(17):11914–11923. doi:10.1074/jbc.274.17.11914.
- Scheuner D, Eckman C, Jensen M, Song X, Citron M, Suzuki N, Bird TD, Hardy J, Hutton M, Kukull W, et al. Secreted amyloid β–protein similar to that in the senile plaques of Alzheimer’s disease is increased in vivo by the presenilin 1 and 2 and APP mutations linked to familial Alzheimer’s disease. Nat Med. 1996;2(8):864–870. doi:10.1038/nm0896-864.
- Zlokovic BV. Neurovascular mechanisms of Alzheimer’s neurodegeneration. Trends Neurosci. 2005;28:202–208. doi:10.1016/j.tins.2005.02.001.
- Yoshiyama Y, Higuchi M, Zhang B, Huang S-M, Iwata N, Saido T, Maeda J, Suhara T, Trojanowski JQ, Lee VMY, et al. Synapse loss and microglial activation precede tangles in a P301S tauopathy mouse model. Neuron. 2007;53(3):337–351. doi:10.1016/j.neuron.2007.01.010.
- Schneider LS, Sano M. Current Alzheimer’s disease clinical trials: methods and placebo outcomes. Alzheimers Dement. 2009;5:388–397. doi:10.1016/j.jalz.2009.07.038.
- Self WK, Holtzman DM. Emerging diagnostics and therapeutics for Alzheimer disease. Nat Med. 2023;29(9):2187–2199. doi:10.1038/s41591-023-02505-2.
- Lian Y, Jia Y-J, Wong J, Zhou X-F, Song W, Guo J, Masters CL, Wang Y-J. Clarity on the blazing trail: clearing the way for amyloid-removing therapies for Alzheimer’s disease. Mol Psychiatry. 2023;29(2):297–305. doi:10.1038/s41380-023-02324-4.
- Cullen KM, Kócsi Z, Stone J. Pericapillary haem-rich deposits: evidence for microhaemorrhages in aging human cerebral cortex. J Cereb Blood Flow Metab. 2005;25(12):1656–1667. doi:10.1038/sj.jcbfm.9600155.
- Berndt P, Winkler L, Cording J, Breitkreuz-Korff O, Rex A, Dithmer S, Rausch V, Blasig R, Richter M, Sporbert A, et al. Tight junction proteins at the blood–brain barrier: far more than claudin-5. Cell Mol Life Sci. 2019;76(10):1987–2002. doi:10.1007/s00018-019-03030-7.
- Donahue JE, Johanson CE. Apolipoprotein E, Amyloid-β, and Blood-Brain Barrier Permeability in Alzheimer Disease. J Neuropathol Exp Neurol. 2008;67(4):261–270. doi:10.1097/NEN.0b013e31816a0dc8.
- Shen H, Fuchino Y, Miyamoto D, Nomura H, Matsuki N. Vagus nerve stimulation enhances perforant path-CA3 synaptic transmission via the activation of β-adrenergic receptors and the locus coeruleus. Int J Neuropsychopharmacol. 2012;15(4):523–530. doi:10.1017/S1461145711000708.
- Ye X, Zhu M, Che X, Wang H, Liang X-J, Wu C, Xue X, Yang J. Lipopolysaccharide induces neuroinflammation in microglia by activating the MTOR pathway and downregulating Vps34 to inhibit autophagosome formation. J Neuroinflammation. 2020;17(1):18. doi:10.1186/s12974-019-1644-8.
- Salcedo C, Pozo Garcia V, García‐Adán B, Ameen AO, Gegelashvili G, Waagepetersen HS, Freude KK, Aldana BI. Increased glucose metabolism and impaired glutamate transport in human astrocytes are potential early triggers of abnormal extracellular glutamate accumulation in hiPSC -derived models of Alzheimer’s disease. J Neurochem. 2023;168(5):822–840. doi:10.1111/jnc.16014.
- Kulijewicz-Nawrot M, Syková E, Chvátal A, Verkhratsky A, Rodríguez JJ. Astrocytes and glutamate homoeostasis in Alzheimer’s disease: a decrease in glutamine synthetase, but not in glutamate transporter-1, in the prefrontal cortex. ASN Neuro. 2013;5:273–282. doi:10.1042/AN20130017.
- Tomé D. The Roles of Dietary Glutamate in the Intestine. Ann Nutr Metab. 2018;73 Suppl(5):15–20. doi:10.1159/000494777.
- Reeds PJ, Burrin DG, Stoll B, Jahoor F. Intestinal glutamate metabolism. J Nutr. 2000;130(4):978S–982S. doi:10.1093/jn/130.4.978S.
- Yunes RA, Poluektova EU, Dyachkova MS, Klimina KM, Kovtun AS, Averina OV, Orlova VS, Danilenko VN. GABA production and structure of gadB/gadC genes in Lactobacillus and Bifidobacterium strains from human microbiota. Anaerobe. 2016;42:197–204. doi:10.1016/j.anaerobe.2016.10.011.
- Yates AD, Allen J, Amode RM, Azov AG, Barba M, Becerra A, Bhai J, Campbell L, Carbajo Martinez M, Chakiachvili M, et al. Ensembl Genomes 2022: an expanding genome resource for non-vertebrates. Nucleic Acids Res. 2022;50(D1):D996–D1003. doi:10.1093/nar/gkab1007.
- Lin C-H, Yang H-T, Lane H-Y. D-glutamate, D-serine, and D-alanine differ in their roles in cognitive decline in patients with Alzheimer’s disease or mild cognitive impairment. Pharmacol Biochem Behav. 2019;185:172760. doi:10.1016/j.pbb.2019.172760.
- Fayed N, Modrego PJ, Rojas-Salinas G, Aguilar K. Brain glutamate levels are decreased in Alzheimer’s disease: a magnetic resonance spectroscopy study. Am J Alzheimers Dis Other Demen. 2011;26(6):450–456. doi:10.1177/1533317511421780.
- Haris M, Nath K, Cai K, Singh A, Crescenzi R, Kogan F, Verma G, Reddy S, Hariharan H, Melhem, ER, et al. Imaging of glutamate neurotransmitter alterations in Alzheimer’s disease. NMR Biomed. 2013;26:386–391. doi:10.1002/nbm.2875.
- Niedzwiecki MM, Walker DI, Howell JC, Watts KD, Jones DP, Miller GW, Hu WT. High-resolution metabolomic profiling of Alzheimer’s disease in plasma. Ann Clin Transl Neurol. 2020;7(1):36–45. doi:10.1002/acn3.50956.
- Jin H, Han H, Song G, Oh H-J, Lee B-Y. Anti-Obesity Effects of GABA in C57BL/6J Mice with High-Fat Diet-Induced Obesity and 3T3-L1 Adipocytes. Int J Mol Sci. 2024;25(2):995. doi:10.3390/ijms25020995.
- Ma X, Yan H, Hong S, Yu S, Gong Y, Wu D, Li Y, Xiao H. Gamma-Aminobutyric Acid Promotes Beige Adipocyte Reconstruction by Modulating the Gut Microbiota in Obese Mice. Nutrients. 2023;15(2):456. doi:10.3390/nu15020456.
- Kootte RS, Levin E, Salojärvi J, Smits LP, Hartstra AV, Udayappan SD, Hermes G, Bouter KE, Koopen AM, Holst JJ, et al. Improvement of Insulin Sensitivity after Lean Donor Feces in Metabolic Syndrome Is Driven by Baseline Intestinal Microbiota Composition. Cell Metab. 2017;26(4):611–619.e6. doi:10.1016/j.cmet.2017.09.008.
- Bedi S, Richardson TM, Jia B, Saab H, Brinkman FSL, Westley M. Similarities between bacterial GAD and human GAD65: Implications in gut mediated autoimmune type 1 diabetes. PLOS ONE. 2022;17(2):e0261103. doi:10.1371/journal.pone.0261103.
- Jolivalt CG, Hurford R, Lee, CA, Dumaop W, Rockenstein E, Masliah E. Type 1 diabetes exaggerates features of Alzheimer’s disease in APP transgenic mice. Exp Neurol. 2010;223:422–431. doi:10.1016/j.expneurol.2009.11.005.
- Ouwens DM, van Duinkerken E, Schoonenboom SNM, Herzfeld de Wiza D, Klein M, van Golen L, Pouwels PJW, Barkhof F, Moll AC, Snoek FJ, et al. Cerebrospinal fluid levels of Alzheimer’s disease biomarkers in middle-aged patients with type 1 diabetes. Diabetologia. 2014;57(10):2208–2214. doi:10.1007/s00125-014-3333-6.
- Rothstein JD, Martin LJ, Kuncl RW. Decreased glutamate transport by the brain and spinal cord in amyotrophic lateral sclerosis. N Engl J Med. 1992;326(22):1464–1468. doi:10.1056/NEJM199205283262204.
- Samakashvili S, Ibáñez C, Simó C, Gil‐Bea FJ, Winblad B, Cedazo‐Mínguez A, Cifuentes A. Analysis of chiral amino acids in cerebrospinal fluid samples linked to different stages of Alzheimer disease. Electrophoresis. 2011;32(19):2757–2764. doi:10.1002/elps.201100139.
- Carello-Collar G, Bellaver B, Ferreira PCL, Ferrari-Souza JP, Ramos VG, Therriault J, Tissot C, De Bastiani MA, Soares C, Pascoal TA, et al. The GABAergic system in Alzheimer’s disease: a systematic review with meta-analysis. Mol Psychiatry. 2023;28(12):5025–5036. doi:10.1038/s41380-023-02140-w.
- Bai X, Edden, RA, Gao F, Wang G, Wu L, Zhao B, Wang M, Chan Q, Chen W, Barker, PB. Decreased γ-aminobutyric acid levels in the parietal region of patients with Alzheimer’s disease. J Magn Reson Imag. 2015;41:1326–1331. doi:10.1002/jmri.24665.
- Fuhrer TE, Palpagama TH, Waldvogel HJ, Synek BJL, Turner C, Faull RL, Kwakowsky A. Impaired expression of GABA transporters in the human Alzheimer’s disease hippocampus, subiculum, entorhinal cortex and superior temporal gyrus. Neuroscience. 2017;351:108–118. doi:10.1016/j.neuroscience.2017.03.041.
- Verret L, Mann E, Hang G, Barth AI, Cobos I, Ho K, Devidze N, Masliah E, Kreitzer A, Mody I, et al. Inhibitory interneuron deficit links altered network activity and cognitive dysfunction in Alzheimer model. Cell. 2012;149(3):708–721. doi:10.1016/j.cell.2012.02.046.
- Flanigan TJ, Xue Y, Kishan Rao S, Dhanushkodi A, McDonald MP. Abnormal vibrissa-related behavior and loss of barrel field inhibitory neurons in 5xFAD transgenics. Genes Brain And Behav. 2014;13(5):488–500. doi:10.1111/gbb.12133.
- Palop JJ, Mucke L. Network abnormalities and interneuron dysfunction in Alzheimer disease. Nat Rev Neurosci. 2016;17(12):777–792. doi:10.1038/nrn.2016.141.
- Jo S, Yarishkin O, Hwang YJ, Chun YE, Park M, Woo DH, Bae JY, Kim T, Lee J, Chun H, et al. GABA from reactive astrocytes impairs memory in mouse models of Alzheimer’s disease. Nat Med. 2014;20(8):886–896. doi:10.1038/nm.3639.
- Yoshiike Y, Kimura T, Yamashita S, Furudate H, Mizoroki T, Murayama M, Takashima A. GABA(A) receptor-mediated acceleration of aging-associated memory decline in APP/PS1 mice and its pharmacological treatment by picrotoxin. PLOS ONE. 2008;3:e3029. doi:10.1371/journal.pone.0003029.
- Ghosh TS, Shanahan F, O’Toole PW. The gut microbiome as a modulator of healthy ageing. Nat Rev Gastroenterol Hepatol. 2022;19(9):565–584. doi:10.1038/s41575-022-00605-x.
- Wilmanski T, Diener C, Rappaport N, Patwardhan S, Wiedrick J, Lapidus J, Earls JC, Zimmer A, Glusman G, Robinson M, et al. Gut microbiome pattern reflects healthy ageing and predicts survival in humans. Nat Metab. 2021;3(2):274–286. doi:10.1038/s42255-021-00348-0.
- Salazar N, Arboleya S, Valdés L, Stanton C, Ross P, Ruiz L, Gueimonde M, de Los Reyes-Gavilán CG. The human intestinal microbiome at extreme ages of life. Dietary intervention as a way to counteract alterations. Front Genet. 2014;5:406. doi:10.3389/fgene.2014.00406.
- Renson A, Mullan Harris K, Dowd JB, Gaydosh L, McQueen MB, Krauter KS, Shannahan M, Aiello AE. Early Signs of Gut Microbiome Aging: Biomarkers of Inflammation, Metabolism, and Macromolecular Damage in Young Adulthood. J Gerontol A Biol Sci Med Sci. 2020;75(7):1258–1266. doi:10.1093/gerona/glaa122.
- Salazar N, López P, Valdés L, Margolles A, Suárez A, Patterson ÁM, Cuervo A, Reyes-Gavilán CGDL, Ruas-Madiedo P, Gonzalez S, et al. Microbial targets for the development of functional foods accordingly with nutritional and immune parameters altered in the elderly. J Am Coll Nutr. 2013;32(6):399–406. doi:10.1080/07315724.2013.827047.
- Zhu Z, Ma X, Wu J, Xiao Z, Wu W, Ding S, Zheng L, Liang X, Luo J, Ding D, et al. Altered Gut Microbiota and Its Clinical Relevance in Mild Cognitive Impairment and Alzheimer’s Disease: Shanghai Aging Study and Shanghai Memory Study. Nutrients. 2022;14(19):3959. doi:10.3390/nu14193959.
- Hou M, Xu G, Ran M, Luo W, Wang H. APOE-ε4 Carrier Status and Gut Microbiota Dysbiosis in Patients with Alzheimer Disease. Front Neurosci. 2021;15:619051. doi:10.3389/fnins.2021.619051.
- Huang H-J, Chen J-L, Liao J-F, Chen Y-H, Chieu M-W, Ke Y-Y, Hsu C-C, Tsai Y-C, Hsieh-Li HM. Lactobacillus plantarum PS128 prevents cognitive dysfunction in Alzheimer’s disease mice by modulating propionic acid levels, glycogen synthase kinase 3 beta activity, and gliosis. BMC Complement Med Ther. 2021;21(1):259. doi:10.1186/s12906-021-03426-8.
- Rezaeiasl Z, Salami M, Sepehri G. The Effects of Probiotic Lactobacillus and Bifidobacterium Strains on Memory and Learning Behavior, Long-Term Potentiation (LTP), and Some Biochemical Parameters in β-Amyloid-Induced Rat’s Model of Alzheimer’s Disease. Prev Nutr Food Sci. 2019;24(3):265–273. doi:10.3746/pnf.2019.24.3.265.
- Patterson E, Ryan PM, Wiley N, Carafa I, Sherwin E, Moloney G, Franciosi E, Mandal R, Wishart DS, Tuohy K, et al. Gamma-aminobutyric acid-producing lactobacilli positively affect metabolism and depressive-like behaviour in a mouse model of metabolic syndrome. Sci Rep. 2019;9(1):16323. doi:10.1038/s41598-019-51781-x.
- Ferreiro AL, Choi J, Ryou J, Newcomer EP, Thompson R, Bollinger RM, Hall-Moore C, Ndao IM, Sax L, Benzinger TL, et al. Gut microbiome composition may be an indicator of preclinical Alzheimer’s disease. Sci Transl Med. 2023;15(700):eabo2984. doi:10.1126/scitranslmed.abo2984.
- Elangovan S, Borody TJ, Holsinger RMD. Fecal Microbiota Transplantation Reduces Pathology and Improves Cognition in a Mouse Model of Alzheimer’s Disease. Cells. 2022;12(1):119. doi:10.3390/cells12010119.
- Sun J, Xu J, Ling Y, Wang F, Gong T, Yang C, Ye S, Ye K, Wei D, Song Z, et al. Fecal microbiota transplantation alleviated Alzheimer’s disease-like pathogenesis in APP/PS1 transgenic mice. Transl Psychiatry. 2019;9(1):189. doi:10.1038/s41398-019-0525-3.
- Kim M-S, Kim Y, Choi H, Kim W, Park S, Lee D, Kim DK, Kim HJ, Choi H, Hyun D-W, et al. Transfer of a healthy microbiota reduces amyloid and tau pathology in an Alzheimer’s disease animal model. Gut. 2020;69(2):283–294. doi:10.1136/gutjnl-2018-317431.
- Wang X, Sun G, Feng T, Zhang J, Huang X, Wang T, Xie Z, Chu X, Yang J, Wang H, et al. Sodium oligomannate therapeutically remodels gut microbiota and suppresses gut bacterial amino acids-shaped neuroinflammation to inhibit Alzheimer’s disease progression. Cell Res. 2019;29(10):787–803. doi:10.1038/s41422-019-0216-x.
- Kim N, Jeon SH, Ju IG, Gee MS, Do J, Oh MS, Lee JK. Transplantation of gut microbiota derived from Alzheimer’s disease mouse model impairs memory function and neurogenesis in C57BL/6 mice. Brain Behav Immun. 2021;98:357–365. doi:10.1016/j.bbi.2021.09.002.
- Hazan S. Rapid improvement in Alzheimer’s disease symptoms following fecal microbiota transplantation: a case report. J Int Med Res. 2020;48(6):300060520925930. doi:10.1177/0300060520925930.
- Reisinger N, Emsenhuber C, Doupovec B, Mayer E, Schatzmayr G, Nagl V, Grenier B. Endotoxin Translocation and Gut Inflammation Are Increased in Broiler Chickens Receiving an Oral Lipopolysaccharide (LPS) Bolus during Heat Stress. Toxins. 2020;12(10):622. doi:10.3390/toxins12100622.
- Chen C, Liao J, Xia Y, Liu X, Jones R, Haran J, McCormick B, Sampson TR, Alam A, Ye K, et al. Gut microbiota regulate Alzheimer’s disease pathologies and cognitive disorders via PUFA-associated neuroinflammation. Gut. 2022;71(11):2233–2252. doi:10.1136/gutjnl-2021-326269.
- Xia Y, Xiao Y, Wang Z-H, Liu X, Alam AM, Haran JP, McCormick BA, Shu X, Wang X, Ye K, et al. Bacteroides Fragilis in the gut microbiomes of Alzheimer’s disease activates microglia and triggers pathogenesis in neuronal C/EBPβ transgenic mice. Nat Commun. 2023;14(1):5471. doi:10.1038/s41467-023-41283-w.
- Lukiw WJ. Bacteroides fragilis Lipopolysaccharide and Inflammatory Signaling in Alzheimer’s Disease. Front Microbiol. 2016;7:213385. Preprint at. doi:10.3389/fmicb.2016.01544.
- Mancuso G, Midiri A, Biondo C, Beninati C, Gambuzza M, Macri D, Bellantoni A, Weintraub A, Espevik T, Teti G, et al. Bacteroides fragilis-derived lipopolysaccharide produces cell activation and lethal toxicity via toll-like receptor 4. Infect Immun. 2005;73(9):5620–5627. doi:10.1128/IAI.73.9.5620-5627.2005.
- Lykhmus O, Mishra N, Koval L, Kalashnyk O, Gergalova G, Uspenska K, Komisarenko S, Soreq H, Skok M. Molecular Mechanisms Regulating LPS-Induced Inflammation in the Brain. Front Mol Neurosci. 2016;9(19). doi:10.3389/fnmol.2016.00019.
- Govindpani K, Turner C, Waldvogel HJ, Faull RLM, Kwakowsky A. Impaired Expression of GABA Signaling Components in the Alzheimer’s Disease Middle Temporal Gyrus. IJMS. 2020;21(22):8704. doi:10.3390/ijms21228704.
- Salazar AM, Leisgang AM, Ortiz AA, Murtishaw AS, Kinney JW. Alterations of GABA B receptors in the APP/PS1 mouse model of Alzheimer’s disease. Neurobiol Aging. 2021;97:129–143. doi:10.1016/j.neurobiolaging.2020.10.013.
- Chen C, Tang X, Lan Z, Chen W, Su H, Li W, Li Y, Zhou X, Gao H, Feng X, et al. GABAergic signaling abnormalities in a novel CLU mutation Alzheimer’s disease mouse model. Transl Res. 2023;260:32–45. doi:10.1016/j.trsl.2023.05.003.
- Liu L, Xu M, Lan R, Hu D, Li X, Qiao L, Zhang S, Lin X, Yang J, Ren Z, et al. Bacteroides vulgatus attenuates experimental mice colitis through modulating gut microbiota and immune responses. Front Immunol. 2022;13:1036196. doi:10.3389/fimmu.2022.1036196.
- Tan FHP, Liu G, Lau SYA, Jaafar MH, Park Y-H, Azzam G, Li Y, Liong M-T. Lactobacillus probiotics improved the gut microbiota profile of a Drosophila melanogaster Alzheimer’s disease model and alleviated neurodegeneration in the eye. Benef Microbes. 2020;11(1):79–90. doi:10.3920/BM2019.0086.
- Pohanka M. D-Lactic Acid as a Metabolite: Toxicology, Diagnosis, and Detection. Biomed Res Int. 2020;2020:1–9. doi:10.1155/2020/3419034.
- France M, Alizadeh M, Brown S, Ma B, Ravel J. Towards a deeper understanding of the vaginal microbiota. Nat Microbiol. 2022;7(3):367–378. doi:10.1038/s41564-022-01083-2.
- Scavuzzo CJ, Rakotovao I, Dickson CT. Differential effects of L- and D-lactate on memory encoding and consolidation: Potential role of HCAR1 signaling. Neurobiol Learn Mem. 2020;168:107151. doi:10.1016/j.nlm.2019.107151.
- Song X, Zhao Z, Zhao Y, Wang Z, Wang C, Yang G, Li S. Lactobacillus plantarum DP189 prevents cognitive dysfunction in D-galactose/AlCl3 induced mouse model of Alzheimer’s disease via modulating gut microbiota and PI3K/Akt/GSK-3β signaling pathway. Nutri Neurosci. 2022;25(12):2588–2600. doi:10.1080/1028415X.2021.1991556.
- Zhong H-J, Wang S-Q, Zhang R-X, Zhuang Y-P, Li L, Yi S-Z, Li Y, Wu L, Ding Y, Zhang J, et al. Supplementation with high-GABA-producing Lactobacillus plantarum L5 ameliorates essential tremor triggered by decreased gut bacteria-derived GABA. Transl Neurodegener. 2023;12(1):58. doi:10.1186/s40035-023-00391-9.
- Wang J, Ji H, Wang S, Liu H, Zhang W, Zhang D, Wang Y. Probiotic Lactobacillus plantarum Promotes Intestinal Barrier Function by Strengthening the Epithelium and Modulating Gut Microbiota. Front Microbiol. 2018;9:1953. doi:10.3389/fmicb.2018.01953.
- Derrien M, van Passel MWJ, van de Bovenkamp JHB, Schipper R, de Vos W, Dekker J. Mucin-bacterial interactions in the human oral cavity and digestive tract. Gut Microbes. 2010;1(4):254–268. doi:10.4161/gmic.1.4.12778.
- Huang JY, Lee SM, Mazmanian SK. The human commensal Bacteroides fragilis binds intestinal mucin. Anaerobe. 2011;17(4):137–141. doi:10.1016/j.anaerobe.2011.05.017.
- Zhu L, Lu X, Liu L, Voglmeir J, Zhong X, Yu Q. Akkermansia muciniphila protects intestinal mucosa from damage caused by S. pullorum by initiating proliferation of intestinal epithelium. Vet Res. 2020;51(1):34. doi:10.1186/s13567-020-00755-3.
- Birchenough G, Schroeder BO, Bäckhed F, Hansson GC. Dietary destabilisation of the balance between the microbiota and the colonic mucus barrier. Gut Microbes. 2019;10(2):246–250. doi:10.1080/19490976.2018.1513765.
- Günzel D, Yu ASL. Claudins and the modulation of tight junction permeability. Physiol Rev. 2013;93(2):525–569. doi:10.1152/physrev.00019.2012.
- Saitou M, Furuse M, Sasaki H, Schulzke J-D, Fromm M, Takano H, Noda T, Tsukita S. Complex phenotype of mice lacking occludin, a component of tight junction strands. Mol Biol Cell. 2000;11(12):4131–4142. doi:10.1091/mbc.11.12.4131.
- Xu C, Wang K, Ding Y-H, Li W-J, Ding L. Claudin-7 gene knockout causes destruction of intestinal structure and animal death in mice. World J Gastroenterol. 2019;25(5):584–599. doi:10.3748/wjg.v25.i5.584.
- Damms-Machado A, Louis S, Schnitzer A, Volynets V, Rings A, Basrai M, Bischoff SC. Gut permeability is related to body weight, fatty liver disease, and insulin resistance in obese individuals undergoing weight reduction. Am J Clin Nutr. 2017;105(1):127–135. doi:10.3945/ajcn.116.131110.
- Takeuchi K, Maiden L, Bjarnason I. Genetic aspects of intestinal permeability in inflammatory bowel disease. Novartis Found Symp. 2004;263:151–158. discussion 159–63, 211–8.
- Li F, Ke H, Wang S, Mao W, Fu C, Chen X, Fu Q, Qin X, Huang Y, Li B, et al. Leaky Gut Plays a Critical Role in the Pathophysiology of Autism in Mice by Activating the Lipopolysaccharide-Mediated Toll-Like Receptor 4–Myeloid Differentiation Factor 88–Nuclear Factor Kappa B Signaling Pathway. Neurosci Bull. 2023;39(6):911–928. doi:10.1007/s12264-022-00993-9.
- Duan M, Liu F, Fu H, Lu S, Wang T. Preoperative Microbiomes and Intestinal Barrier Function Can Differentiate Prodromal Alzheimer’s Disease from Normal Neurocognition in Elderly Patients Scheduled to Undergo Orthopedic Surgery. Front Cell Infect Microbiol. 2021;11:592842. doi:10.3389/fcimb.2021.592842.
- Qaisar R, Karim A, Iqbal, MS, Ahmad F, Shaikh A, Kamli H, Khamja, NA. A leaky gut contributes to postural dysfunction in patients with Alzheimer’s disease. Heliyon. 2023;9:e19485. doi:10.1016/j.heliyon.2023.e19485.
- Zhou Y, Zhi F. Lower Level of Bacteroides in the Gut Microbiota Is Associated with Inflammatory Bowel Disease: A Meta-Analysis. Biomed Res Int. 2016;2016:1–9. doi:10.1155/2016/5828959.
- Brahe LK, Le Chatelier E, Prifti E, Pons N, Kennedy S, Hansen T, Pedersen O, Astrup A, Ehrlich SD, Larsen LH, et al. Specific gut microbiota features and metabolic markers in postmenopausal women with obesity. Nutr Diabetes. 2015;5(6):e159. doi:10.1038/nutd.2015.9.
- André P, Laugerette F, Féart C. Metabolic Endotoxemia: A Potential Underlying Mechanism of the Relationship between Dietary Fat Intake and Risk for Cognitive Impairments in Humans? Nutrients. 2019;11(8):1887. doi:10.3390/nu11081887.
- Macfarlane GT, Gibson GR. Formation of glycoprotein degrading enzymes by Bacteroides fragilis. FEMS Microbiol Lett. 1991;61(2–3):289–294. doi:10.1111/j.1574-6968.1991.tb04363.x.
- Slattery C, Cotter PD, O’Toole PW. Analysis of Health Benefits Conferred by Lactobacillus Species from Kefir. Nutrients. 2019;11(6):1252. doi:10.3390/nu11061252.
- Chen C-M, Wu C-C, Huang C-L, Chang M-Y, Cheng S-H, Lin C-T, Tsai Y-C. Lactobacillus plantarum PS128 Promotes Intestinal Motility, Mucin Production, and Serotonin Signaling in Mice. Probiotics Antimicrob Proteins. 2022;14(3):535–545. doi:10.1007/s12602-021-09814-3.
- Kaminsky L, Al-Sadi R, Ma T. Lactobacillus acidophilus causes enhancement of the intestinal tight junction barrier by a toll-like receptor-2-dependent increase in occludin. J Allergy Clin Immunol. 2022;149(2):AB99. doi:10.1016/j.jaci.2021.12.347.
- Blackwood BP, Yuan CY, Wood DR, Nicolas JD, Grothaus JS, Hunter CJ. Probiotic Lactobacillus Species Strengthen Intestinal Barrier Function and Tight Junction Integrity in Experimental Necrotizing Enterocolitis. J Prob Health. 2017;5(01). doi:10.4172/2329-8901.1000159.
- Anderson RC, Cookson AL, McNabb WC, Park Z, McCann MJ, Kelly WJ, Roy NC. Lactobacillus plantarum MB452 enhances the function of the intestinal barrier by increasing the expression levels of genes involved in tight junction formation. BMC Microbiol. 2010;10(1):316. doi:10.1186/1471-2180-10-316.
- Karczewski J, Troost FJ, Konings I, Dekker J, Kleerebezem M, Brummer RJM, Wells JM. Regulation of human epithelial tight junction proteins by Lactobacillus plantarum in vivo and protective effects on the epithelial barrier. Am J Physiol Gastrointest Liver Physiol. 2010;298(6):G851–9. doi:10.1152/ajpgi.00327.2009.
- Gu Y, Qin X, Zhou G, Wang C, Mu C, Liu X, Zhong W, Xu X, Wang B, Jiang K, et al. Lactobacillus rhamnosus GG supernatant promotes intestinal mucin production through regulating 5-HT4R and gut microbiota. Food Funct. 2022;13(23):12144–12155. doi:10.1039/D2FO01900K.
- Lyte M, Freestone PPE. Microbial Endocrinology: interkingdom Signaling in Infectious Disease and Health. Switzerland: Springer Science & Business Media; 2010.
- Chen KH, Reese EA, Kim H-W, Rapoport SI, Rao JS. Disturbed neurotransmitter transporter expression in Alzheimer’s disease brain. J Alzheimers Dis. 2011;26(4):755–766. doi:10.3233/JAD-2011-110002.
- Garcia-Alloza M, Tsang, SW, Gil-Bea, FJ, Francis, PT, La, MK, Marcos B, Chen, CP, Ramirez, MJ. Involvement of the GABAergic system in depressive symptoms of Alzheimer’s disease. Neurobiol Aging. 2006;27:1110–1117. doi:10.1016/j.neurobiolaging.2005.06.003.
- Miri S, Yeo J, Abubaker S, Hammami R. Neuromicrobiology, an emerging neurometabolic facet of the gut microbiome? Front Microbiol. 2023;14:1098412. doi:10.3389/fmicb.2023.1098412.
- Jameson KG, Olson CA, Kazmi SA, Hsiao EY. Toward Understanding Microbiome-Neuronal Signaling. Mol Cell. 2020;78(4):577–583. doi:10.1016/j.molcel.2020.03.006.
- Zhang H, Wang Y, Gao F, Liu R, Chen W, Zhao X, Sun Q, Sun X, Li J, Liu C, et al. GABA increases susceptibility to DSS-induced colitis in mice. J Funct Foods. 2022;99:105339. doi:10.1016/j.jff.2022.105339.
- Chen S, Tan B, Xia Y, Liao S, Wang M, Yin J, Wang J, Xiao H, Qi M, Bin P, et al. Effects of dietary gamma-aminobutyric acid supplementation on the intestinal functions in weaning piglets. Food Funct. 2019;10(1):366–378. doi:10.1039/C8FO02161A.
- Dong Z, Han H, Li H, Bai Y, Wang W, Tu M, Peng Y, Zhou L, He W, Wu X, et al. Long-term potentiation decay and memory loss are mediated by AMPAR endocytosis. J Clin Invest. 2015;125(1):234–247. doi:10.1172/JCI77888.
- Vargas-Caballero M, Warming H, Walker R, Holmes C, Cruickshank G, Patel B. Vagus Nerve Stimulation as a Potential Therapy in Early Alzheimer’s Disease: A Review. Front Hum Neurosci. 2022;16:866434. doi:10.3389/fnhum.2022.866434.
- Ben-Menachem E, Hamberger A, Hedner T, Hammond EJ, Uthman BM, Slater J, Treig T, Stefan H, Ramsay RE, Wernicke JF, et al. Effects of vagus nerve stimulation on amino acids and other metabolites in the CSF of patients with partial seizures. Epilepsy Res. 1995;20(3):221–227. doi:10.1016/0920-1211(94)00083-9.
- Marrosu F, Serra A, Maleci A, Puligheddu M, Biggio G, Piga M. Correlation between GABA(A) receptor density and vagus nerve stimulation in individuals with drug-resistant partial epilepsy. Epilepsy Res. 2003;55(1–2):59–70. doi:10.1016/S0920-1211(03)00107-4.
- Zou Q, Han S, Liang J, Yan G, Wang Q, Wang Y, Zhang Z, Hu J, Li J, Yuan T, et al. Alleviating effect of vagus nerve cutting in Salmonella-induced gut infections and anxiety-like behavior via enhancing microbiota-derived GABA. Brain Behav Immun. 2024;119:607–620. doi:10.1016/j.bbi.2024.04.034.
- Wood JD. Enteric Nervous System: the Brain-in-the-Gut. Williston, Vermont: Biota Publishing; 2011.
- Auteri M, Zizzo MG, Serio R. GABA and GABA receptors in the gastrointestinal tract: from motility to inflammation. Pharmacol Res. 2015;93:11–21. doi:10.1016/j.phrs.2014.12.001.
- Krantis A. GABA in the Mammalian Enteric Nervous System. News Physiol Sci. 2000;15(6):284–290. doi:10.1152/physiologyonline.2000.15.6.284.
- Koussoulas K, Swaminathan M, Fung C, Bornstein JC, Foong JPP. Neurally released GABA acts via GABAC receptors to modulate Ca2+ transients evoked by trains of synaptic inputs, but not responses evoked by single stimuli, in myenteric neurons of mouse ileum. Front Physiol. 2018;9:97. doi:10.3389/fphys.2018.00097.
- Deng Z, Li D, Yan X, Lan J, Han D, Fan K, Chang J, Ma Y. Activation of GABA receptor attenuates intestinal inflammation by modulating enteric glial cells function through inhibiting NF-κB pathway. Life Sci. 2023;329:121984. doi:10.1016/j.lfs.2023.121984.
- Strandwitz P, Kim KH, Terekhova D, Liu JK, Sharma A, Levering J, McDonald D, Dietrich D, Ramadhar TR, Lekbua A, et al. GABA-modulating bacteria of the human gut microbiota. Nat Microbiol. 2019;4(3):396–403. doi:10.1038/s41564-018-0307-3.
- Bhat R, Axtell R, Mitra A, Miranda M, Lock C, Tsien RW, Steinman L. Inhibitory role for GABA in autoimmune inflammation. Proc Natl Acad Sci USA. 2010;107(6):2580–2585. doi:10.1073/pnas.0915139107.
- Kang Y, Kang X, Yang H, Liu H, Yang X, Liu Q, Tian H, Xue Y, Ren P, Kuang X, et al. Lactobacillus acidophilus ameliorates obesity in mice through modulation of gut microbiota dysbiosis and intestinal permeability. Pharmacol Res. 2022;175:106020. doi:10.1016/j.phrs.2021.106020.