ABSTRACT
DNA double-strand break (DSB) repair is essential for genome stability maintenance, but the joining of distant DNA double strand ends (DSEs) inevitably leads to genome rearrangements. Therefore, DSB repair should be tightly controlled to secure genome stability while allowing genetic variability.
Tethering of the proximal ends of a 2-ended DSB limits their mobility, protecting thus against their joining with a distant DSE. However, replication stress generates DSBs with only one DSE, on which tethering is impossible. Consistently, we demonstrated that the joining of 2 DSBs only 3.2 kb apart is repressed in the S, but not the G1, phase, revealing an additional mechanism limiting DNA ends mobility in S phase. The cohesin complex, by maintaining the 2 sister chromatids linked, limits DSEs mobility and thus represses the joining of distant DSEs, while allowing that of adjacent DSEs. At the genome scale, the cohesin complex protects against deletions, inversions, translocations and chromosome fusion.
Genomes are routinely challenged by genotoxic stresses from exogenous sources, such as radiations or chemicals, and endogenous origins, such as reactive oxygen species (ROS) resulting from cell metabolism or spontaneous DNA replication stress. DNA double-strand break (DSB) is a harmful lesion that can lead to cell death or genome rearrangements, routing to senescence or carcinogenesis. DSB repair is essential to maintain genome stability but can also generate genetic rearrangements. However, DSBs can also be used to generate beneficial genetic diversity in essential physiological processes, such as meiosis and establishment of the immune repertoire. In these cases, DSB production is controlled by cellular enzymes, and the same pathways for repair of accidental DSB are used. Therefore, DSB repair should be tightly controlled to maintain genome stability while allowing genome diversity in very specific pathways.
Two general processes repair DSBs: 1) the first DSB repair processes is based on the invasion and copy of an intact homologous DNA and is referred to as homologous recombination (HR), although it corresponds to several sub-pathways,Citation1 and 2) the second DSB repair process joins the double-strand DNA ends (DSE) in a sequence homology-independent manner. Several mechanisms can refer to this process, such as the KU80-KU70/ligase 4-dependent conservative canonical non-homologous end-joining (C-NHEJ) pathway or the mutagenic alternative end-joining (A-EJ) pathway, which is KU80-KU70/ligase 4-independent.Citation2 In mammalian cells, the end-joining of 2 DSEs is a prominent DSB repair pathway; it is essential in class switch recombination (CSR) and in V(D)J recombination, as well as for repair of DSBs generated by ionizing radiation (IR).Citation3 Remarkably, end-joining is active throughout the cell cycle but competes with HR in the S and G2 phases.Citation4,5
End-joining can generate genetic diversity/instability at 2 levels: 1) at the seal junction through mutagenic end-joining or 2) through the joining of distant DSE, resulting in a rearrangement (). Importantly, these 2 processes can be associated, as for instance in V(D)J recombination which associates error-prone joining to the rearrangements, increasing thus genetic diversity. Therefore, the joining of distant DSEs, although essential for CSR and V(D)J recombination, which ensures the diversity of the immune repertoire, can jeopardize genome stability, resulting in potential deleterious consequences, such as carcinogenesis.
Figure 1. Genetic instability generated by DSB repair. (A) Two types of genetic instability generated by DSB repair: error-prone end-joining resulting in mutations at the junctions (left panel) and rearrangements resulting from the joining of distant DSB (right panels). Mutagenic joining and rearrangements can be associated (right panel). (B) Intra-chromosomal substrate monitoring end-joining. Reporter genes (GFP or CD4 membrane antigen) are not expressed because of an intervening sequence (in blue) between the reporter gene and promoter. Two cleavage sites for the meganuclease I-SceI (red arrows) are inserted in the intervening sequence. Expression of I-SceI excises the intervening sequence and end-joining places the reporter gene under the control of the promoter. The expression of the reporter genes enables monitoring of end-joining events, and sequencing of the repair junctions enables monitoring of the repair accuracy. (C) Two-ended DSB (left and middle panels) vs. one-ended DSB induced by replication fork arrest (right panel). The tethering (gray spheres) maintains genome organization. With two distant DSBs (middle panels), bypass of the tethering leads to genetic rearrangements. Intermolecular end-joining (upper middle panel) result in translocation. Intramolecular end-joining (lower middle panel) result in either deletion or inversion of the intervening sequence. The joining of one-ended DSBs (right panel) inevitably leads to genetic rearrangements.
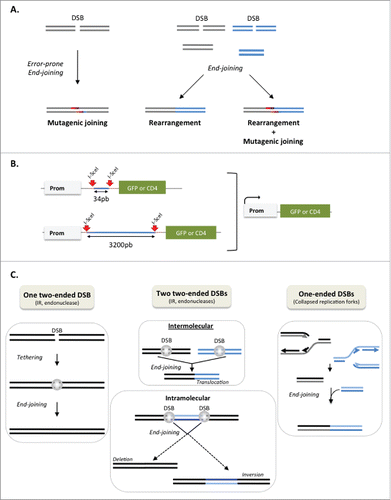
Genome rearrangements induced by joining distant DSBs
Joining two distant DSEs first requires bringing them together in a “synaptic” step. According to nucleus size, such events occur at different scales, which should impact their efficiency: at a small scale with 2 DSBs at a distance of some kb or at a large scale (at the chromosome level) intra- or inter-chromosome exchange. Note that the chromosome territories may limit inter-chromosome rearrangements. Using intra-chromosomal substrates, in which 2 DSBs are generated by the meganuclease I-SceI, we compared end-joining of DSBs 34 bp versus 3200 bp apart (); 34 bp is sufficiently small to be equivalent to a 2-ended DSB, whereas a 3200 bp distance requires the synapsis of the 2 DSEs prior to ligation. We demonstrated that although this distance is small at the whole nucleus scale, only a few kb (3200 bp) between the 2 DSBs is sufficient to significantly decrease both the efficiency and accuracy of DSB repair in asynchronous cell populations (Guirouilh-Barbat et al., submitted).
DSBs can be classified into 2 categories: 1) DSBs with 2 adjacent DSEs (2-ended DSB), which are generated by IR or endonuclease (, and 2) DSBs with a single DSE (one-ended DSB), which are generated by replication stress (). Therefore, whereas 2-ended DSBs can occur in all cell cycle phases, one-ended DSBs are primarily restricted to the S/G2 phases. Importantly, joining 2 one-ended DSBs inevitably results in chromosome fusion and rearrangement (). Therefore, protection against the joining of distant DSBs should be particularly efficient during the S/G2 phases to secure genome stability. We addressed the question of the existence of a process specifically repressing the joining of distant DSEs, which should be more efficient in the S/G2 phase, and should allow essential processes such as V(D)J recombination and CSR to occur. We hypothesized that the cohesin complex may play this role. First, cohesins are recruited to DSBs.Citation6 In addition, the cohesin complex is also involved in the protection against breaks in telomeres and fragile sites.Citation7–9 We showed that the cohesion complex represses the joining of distant DSEs specifically in the S phase, while it does not impact the joining of close DSEs.Citation10
Impact of cell cycle phase on joining distant DSEs
In contrast with the S/G2 phases, 3200 bp between 2 DSBs is not sufficient to significantly affect end-joining in the G1 phase, although this leads to local genetic alteration (excision/deletion). However, it is possible that different processes protect against long-range genome rearrangements. Nevertheless, the tethering of 2 close DSEs should actively act on genome stability maintenance in the G1 phase. Indeed, DSBs generated by nucleases or IR mostly correspond to 2-ended DSBs (see ) and can occur in all phase of cell cycle, including the G1 phase. Tethering of the 2 close DSEs protects against the mobility of the DSE and favors joining with the proximal DSEs, thus limiting genome rearrangements. Ablation of the KU70-KU80 heterodimer, which is involved in the tethering of the 2 DSEs of one DSB, consistently leads to increased mobility of the DSEs and genome rearrangements.Citation11 One-ended DSBs arise mainly through replication forks collapse, thus in S/G2 phase. The tethering of 2 DSEs cannot act on one-ended DSBs because they are too distant from each other. Therefore, the joining of distant DSE should be efficiently restricted during the S/G2 phases by additional mechanisms. We demonstrated that a few kb of distance (3200 bp) are sufficient to strongly affect the efficiency of end-joining in the S/G2 phases but not the G1 phase.Citation10 This shows that mechanisms additional to the tethering of close ends (which acts both in the G1 and S/G2 phases) should operate in the S/G2 phase. Since it is not a tethering process, such process(es) might operate on nuclease (or IR)-induced DSBs (2-ended DSB) as well as on replication stress-induced DSBs (one-ended DSBs). Indeed, the synapsis step is required in both cases. We showed that the cohesion complex plays this additional role.Citation10
The cohesin complex protects against the joining of distant DSEs in the S phase
The cohesion complex is a ring-shape protein complex that mediates chromosomal cohesin by embracing the 2 sister chromatids resulting from replication. We proposed that this action should restrict the mobility required from the end-joining of distant DSEs. Upon replication stress, the cohesin complex favors HR with the sister chromatid, leading to sister chromatid exchange (SCE).Citation12–14 Because the 2 sister chromatids are identical, SCE enables replication to resume without genetic impact, thus maintaining genome stability. Our hypothesis suggested that maintaining the cohesin between the 2 sister chromatids might also restrict the trafficking of DNA extremities. This hypothesis is consistent with DNA mobility analyses performed in yeastCitation15 but is contradicted by findings in mammalian cells, wherein ablating the cohesin complex protein SMC1 did not affect the mobility of the DNA ends of one DSB.Citation11 However, this latter study analyzed a 2-ended DSB with 2 close DSEs that can be tethered in an asynchronous cell population, whereas our hypothesis speculated a specific S/G2 phase role. Finally, note that the cohesin complex can also act in the G1 phase, generating chromatid remodeling loops that bring together promoters and enhancers for gene expression and insulation,Citation16 and bringing together replication origins through the interaction with proteins of the pre-replication complexes.Citation17
Using the intra-chromosomal substrates described above (see ), we found that ablation of the cohesin complex proteins RAD21 or NIPBL (the cohesin loader) abolished repression of the end-joining of distant DSEs. Importantly, the end-joining of close DSEs was unaffected.Citation10 Both C-NHEJ and A-EJ were affected, indicating that the cohesin complex does not repress a specific repair mechanism but likely a common step, i.e., the synapsis of the distant ends. Because repression of the end-joining of distal 3200 bp distant occurs in the S/G2 phases, the above data suggest that cohesins act during these phases. We confirmed this hypothesis by ablating Sororin, which stabilizes the cohesin complex on sister chromatids and is therefore essential for the post-replication role of the cohesin complex.Citation12,14,18 Ablation of Sororin abolished repression of the end-joining of distant DSEs without affecting that of close DSEs in an epistatic manner with RAD21. We conclude that cohesin complex represses the end-joining (both C-NHEJ and A-EJ) of distant ends (even of only few kb in distance) in the S/G2 phases. At the genome scale, we performed a “Differential Exome Sequencing” analysis: we analyzed structural variations in the exome of cells silenced for RAD21 compared to the same cell line (isogenic) treated with a control siRNA. We thus determined rearrangements occurring during the 48 h (2 cell generations) of RAD21 silencing. Although this method analyses only one part of the genome (the exome), it is tractable and sufficient to reveal a general state of genetic instability. The “Differential Exome Sequencing” method revealed that the cohesin complex protects against long-range rearrangements (translocations, duplications, inversions, and deletions). Finally, a cytogenetic analysis revealed that the association of a treatment with aphidicolin (an inhibitor of replicative polymerase, which generates a replication stress) and ablation of the cohesin complex synergistically generates chromosome fusions.Citation10 Because chromosome fusions result from the end-joining between DSEs of different chromosomes (i.e., distant DSEs), the synergistic effect underlines the role of the cohesin complex in protection against the joining of distant DSEs, including at the chromosome scale.
These data are summarized in the model presented in . Two points are essential: i) the cohesins complexe represses the end-joining (both C-NHEJ and A-EJ) of distant DSEs specifically in the S/G2 phases, and ii) cohesins do not repress the end-joining of close ends, even in the S/G2 phases. The latter result suggests that the cohesin complex enables repair of the DSBs generated by endonucleases or IR or by converging replication forks (see below). The former point suggests a role during replication. Indeed, the cohesin complex stabilizes the arrested replication forks,Citation13 allowing the converging replication forks to reach the blocked fork (, left panel), which leads to 2 close DSEs. Because the joining of 2 close DSEs is not inhibited in the S phase, this allows the DNA molecule to seal, maintaining genome stability. Importantly, NHEJ has been shown to act upon replication stress.Citation19–21 If the converging replication fork does not reach the arrested fork, then sister chromatid exchange (SCE), which is favored by the cohesin complex, allows replication to resume (, middle panel). In addition, the cohesin complex prevents the joining of distant DSEs and thus genome rearrangements. This role of the cohesin complex should act through the tight binding of the 2 sister chromatid and defects in the cohesin complex affect the cohesion of sister chromatid, increasing DSE motility. Therefore, the arrested replication forks are not stabilized, and SCEs are not favored, whereas genome rearrangements and chromosome fusions are fostered (, right panel). This model suggests that the cohesion complex restricts DNA end motility via cohesin of the sister chromatid. Therefore, this mechanism should be specific to the S phase and should not operate in the G1 phase. In this context, it worth noting that V(D)J recombination and CSR, which both involve end-joining of distant DNA sequences, both act in the G1 phaseCitation22–24 (). Moreover, the cohesin complex participates in CSR.Citation25,26. Restricting these essential processes in the G1 phase allows to avoid any potential negative effect of the cohesin complex on DNA mobility. Indeed, this mobility repression by the cohesin complex is restricted to the S/G2 phase likely through the tight binding of the sister chromatids.Citation10 In contrast, the cohesin complex might promote intrachomosomal loops, as also shown at DSBs,Citation6 favoring thus CSR. In the same manner role of cohesins in putting closer enhancers replication origins in replication factories is restricted to the G1 phase of the cell cycle.
Figure 2. Multiple roles for the cohesion complex. In the G1 phase (upper panels). In the S/G2 phase (lower panel): impact of sister chromatid cohesion in genome stability maintenance following replication stress. Arrested replication fork are stabilized by the cohesin complex (left panel). A converging migrating replication fork reaches the blocked fork, leading to the formation of 2 close ends. The cohesin complex does not prevent the joining of such DSEs. Moreover, the end-joining of close ends is more conservative (Guirouilh-Barbat et al., submitted). Alternatively, sister chromatid exchange (SCE) enables the arrested replication fork to resume (middle panel); because the sister chromatids are identical, this process favors genetic stability. In the absence of the cohesin complex (right panel), one one-ended DSB can interact with another distant DSE. The joining of such DSEs generates chromosome fusions and genome rearrangements. The cohesin complex not only favors the 2 former situations, which both maintain genome stability but also restrains the joining of distant ends, protecting against the associated genetic instability.
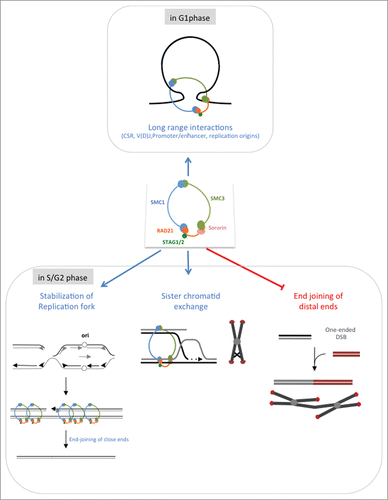
Concluding remarks
Tight regulation of DNA motility is essential for controlling genome plasticity. Indeed, DNA mobility of DNA is essential to processes creating genetic diversity, such as during the establishment of the immune repertoire. However, DNA mobility can generate genome rearrangements with deleterious consequences, such as carcinogenesis. The S phase is particularly sensitive because replication fork collapse generates one-ended DSBs, and joining such DSBs inevitably results in chromosome fusions and genome rearrangements. Inhibition of the end-joining process itself in the S phase is not an appropriate solution because it could be necessary for the repair of IR-induced damage or sealing converging replication forks. Therefore, acting on DNA mobility should avoid the risk of joining distant DSEs without affecting the joining of close DNA ends, provided that some processes, such as V(D)J recombination and CSR, escape to this repression. Restricting DNA mobility prevention to the S phase is thus a particularly well-adapted strategy because one-ended DSB are generated during this phase, enabling V(D)J recombination and CSR to occur in the G1 phase. The cohesin complex assists in maintaining genome stability by simultaneous acting on several processes (SCE, stabilization of arrested replication forks, and repressing the joining of distant DSEs), all based on the cohesin of sister chromatids. This strategy does not negatively affect the end-joining of close DSEs and limits this role to the S/G2 phase, when End Joining of distant ends would endanger genome integrity, and permitting end-joining of distant DNA in the G1 phase.
Disclosure of potential conflicts of interest
No potential conflicts of interest were disclosed.
Funding
This work was supported by La Ligue Nationale Contre le Cancer, ANR (Agence Nationale de la Recherche, ANR-14-CE10-0010-02), AFM-Téléthon and INCa (Institut National du Cancer, 2011-1-RT-01, 2011-1-PLBIO-09, 2013-1-PLBIO-14).
References
- Guirouilh-Barbat J, Lambert S, Bertrand P, Lopez BS. Is homologous recombination really an error-free process? Front Genet 2014; 5:175; PMID:24966870; http://dx.doi.org/10.3389/fgene.2014.00175
- Bétermier M, Bertrand P, Lopez BS. Is Non-Homologous End-Joining Really an Inherently Error-Prone Process? PLoS Genet 2014; 10:e1004086; http://dx.doi.org/10.1371/journal.pgen.1004086
- Deriano L, Roth DB. Modernizing the nonhomologous end-joining repertoire: alternative and classical NHEJ share the stage. Annu Rev Genet 2013; 47:433-55; PMID:24050180; http://dx.doi.org/10.1146/annurev-genet-110711-155540
- Rothkamm K, Kruger I, Thompson LH, Lobrich M. Pathways of DNA double-strand break repair during the mammalian cell cycle. Mol Cell Biol 2003; 23:5706-15; PMID:12897142; http://dx.doi.org/10.1128/MCB.23.16.5706-5715.2003
- Guirouilh-Barbat J, Huck S, Lopez BS. S-phase progression stimulates both the mutagenic KU-independent pathway and mutagenic processing of KU-dependent intermediates, for nonhomologous end joining. Oncogene 2008; 27:1726-36; PMID:17891177; http://dx.doi.org/10.1038/sj.onc.1210807
- Caron P, Aymard F, Iacovoni JS, Briois S, Canitrot Y, Bugler B, Massip L, Losada A, Legube G. Cohesin protects genes against ??H2AX induced by DNA double-strand breaks. PLoS Genet 2012; 8:e1002460.
- Sofueva S, Yaffe E, Chan WC, Georgopoulou D, Vietri Rudan M, Mira-Bontenbal H, Pollard SM, Schroth GP, Tanay A, Hadjur S. Cohesin-mediated interactions organize chromosomal domain architecture. EMBO J 2013; 321:3119–29; 1-11; PMID:23211745
- Deng Z, Wang Z, Stong N, Plasschaert R, Moczan A, Chen HS, Hu S, Wikramasinghe P, Davuluri RV, Bartolomei MS, et al. A role for CTCF and cohesin in subtelomere chromatin organization, TERRA transcription, and telomere end protection. EMBO J 2012; 31:4165-78; PMID:23010778; http://dx.doi.org/10.1038/emboj.2012.266
- Musio A, Montagna C, Mariani T, Tilenni M, Focarelli ML, Brait L, Indino E, Benedetti PA, Chessa L, Albertini A, et al. SMC1 involvement in fragile site expression. Hum Mol Genet 2005; 14:525-33; PMID:15640246; http://dx.doi.org/10.1093/hmg/ddi049
- Gelot C, Guirouilh-Barbat J, Le Guen T, Dardillac E, Chailleux C, Canitrot Y, Lopez BS. The Cohesin Complex Prevents the End Joining of Distant DNA Double-Strand Ends. Mol Cell 2016; 61:15-26; PMID:26687679; http://dx.doi.org/10.1016/j.molcel.2015.11.002
- Soutoglou E, Dorn JF, Sengupta K, Jasin M, Nussenzweig A, Ried T, Danuser G, Misteli T. Positional stability of single double-strand breaks in mammalian cells. Nat Cell Biol 2007; 9:675-82; PMID:17486118; http://dx.doi.org/10.1038/ncb1591
- Klein F, Mahr P, Galova M, Buonomo SB, Michaelis C, Nairz K, Nasmyth K. A central role for cohesins in sister chromatid cohesion, formation of axial elements, and recombination during yeast meiosis. Cell 1999; 98:91-103; PMID:10412984; http://dx.doi.org/10.1016/S0092-8674(00)80609-1
- Tittel-Elmer M, Lengronne A, Davidson MB, Bacal J, François P, Hohl M, Petrini JH, Pasero P, Cobb JA. Cohesin association to replication sites depends on rad50 and promotes fork restart. Mol Cell 2012; 48:98-108; PMID:22885006; http://dx.doi.org/10.1016/j.molcel.2012.07.004
- Potts PR, Porteus MH, Yu H. Human SMC5/6 complex promotes sister chromatid homologous recombination by recruiting the SMC1/3 cohesin complex to double-strand breaks. EMBO J 2006; 25:3377-88; PMID:16810316; http://dx.doi.org/10.1038/sj.emboj.7601218
- Dion V, Kalck V, Seeber A, Schleker T, Gasser SM. Cohesin and the nucleolus constrain the mobility of spontaneous repair foci. EMBO Rep 2013; 14:984-91; PMID:24018421; http://dx.doi.org/10.1038/embor.2013.142
- Jeppsson K, Kanno T, Shirahige K, Sjögren C. The maintenance of chromosome structure: positioning and functioning of SMC complexes. Nat Rev Mol Cell Biol 2014; 15:601-14; PMID:25145851; http://dx.doi.org/10.1038/nrm3857
- Guillou E, Ibarra A, Coulon V, Casado-Vela J, Rico D, Casal I, Schwob E, Losada A, Méndez J. Cohesin organizes chromatin loops at DNA replication factories. Genes Dev 2010; 24:2812-22; PMID:21159821; http://dx.doi.org/10.1101/gad.608210
- Guacci V, Koshland D, Strunnikov aV. A Direct Link between Sister Chromatid Cohesion and Chromosome Condensation Revealed through the Analysis of MCD1 in S. cerevisiae 1997; 91:47-57
- Saintigny Y. Characterization of homologous recombination induced by replication inhibition in mammalian cells. EMBO J 2001; 20:3861-3870; PMID:11447127; http://dx.doi.org/10.1093/emboj/20.14.3861
- Couedel C, Mills KD, Barchi M, Shen L, Olshen A, Johnson RD, Nussenzweig A, Essers J, Kanaar R, Li GC, et al. Collaboration of homologous recombination and nonhomologous end-joining factors for the survival and integrity of mice and cells. Genes Dev 2004; 18:1293-1304; PMID:15175261; http://dx.doi.org/10.1101/gad.1209204
- Mills KD, Ferguson DO, Essers J, Eckersdorff M, Kanaar R, Alt FW. Rad54 and DNA Ligase IV cooperate to maintain mammalian chromatid stability. Genes Dev 2004; 18:1283-92; PMID:15175260; http://dx.doi.org/10.1101/gad.1204304
- Petersen S, Casellas R, Reina-San-Martin B, Chen HT, Difilippantonio MJ, Wilson PC, Hanitsch L, Celeste A, Muramatsu M, Pilch DR, et al. AID is required to initiate Nbs1/gamma-H2AX focus formation and mutations at sites of class switching. Nature 2001; 414:660-5; PMID:11740565; http://dx.doi.org/10.1038/414660a
- Desiderio S. Temporal and spatial regulatory functions of the V(D)J recombinase. Semin Immunol 2010; 22:362-69; PMID:21036059; http://dx.doi.org/10.1016/j.smim.2010.09.001
- Schrader CE, Guikema JEJ, Linehan EK, Selsing E, Stavnezer J. Activation-induced cytidine deaminase-dependent DNA breaks in class switch recombination occur during G1 phase of the cell cycle and depend upon mismatch repair. J Immunol 2007; 179:6064-71; PMID:17947680; http://dx.doi.org/10.4049/jimmunol.179.9.6064
- Enervald E, Du L, Visnes T, Björkman A, Lindgren E, Wincent J, Borck G, Colleaux L, Cormier-Daire V, van Gent DC, et al. A regulatory role for the cohesin loader NIPBL in nonhomologous end joining during immunoglobulin class switch recombination. J Exp Med 2013; 210:2503-13; PMID:24145515; http://dx.doi.org/10.1084/jem.20130168
- Thomas-Claudepierre A-S, Schiavo E, Heyer V, Fournier M, Page A, Robert I, Reina-San-Martin B. The cohesin complex regulates immunoglobulin class switch recombination. J Exp Med 2013; 210:2495-502; PMID:24145512; http://dx.doi.org/10.1084/jem.20130166