ABSTRACT
Faithful duplication of the entire genome during each cell cycle is key for genome maintenance. Each stage of DNA replication, including initiation, progression, and termination, is tightly regulated. Some of these regulations enable replisomes to overcome tens of thousands of template obstacles that block DNA synthesis. Previous studies have identified a large number of proteins that are dedicated to this mission, including protein modification enzymes and scaffold proteins. Protein modification enzymes can bestow fast and reversible changes on many substrates, and thus are ideal for coordinating multiple events needed to promptly overcome replication impediments. Scaffold proteins can support specific protein-protein interactions that enable protein complex formation, protein recruitment, and partner enzyme functions. Taken together with previous studies, our recent work elucidates that a group of modification and scaffold proteins form several complexes to aid replication progression and are particularly important for synthesizing large replicons. Additionally, our work reveals that the intrinsic plasticity of the replication initiation program can be used to compensate for deficient replication progression.Citation1
Our understanding of genome replication has greatly benefited from the use of simple model organisms such as budding yeast. Studies of this system have led to the discovery of numerous conserved pathways and factors that aid replication under DNA damage conditions such as those caused by methyl methane sulfonate (MMS). One of these factors is Rtt107 (or Esc4), a scaffold protein containing 6 BRCT domains ().Citation2-5 The BRCT domain, initially found in the tumor suppressor BRCA1 protein,Citation6 mediates protein-protein interactions.Citation7-10 Rtt107 contains the greatest number of BRCT domains among yeast proteins, with 4 at its N-terminal region and 2 at its C-terminal region (). The C-terminal BRCT domains of Rtt107 bind to γH2A, a phosphorylated form of H2A,Citation11 and its N-terminal ones are associated with 3 factors: another scaffold protein Slx4, the cullin ubiquitin ligase complex composed of Rtt101 cullin and adapter and linker subunits Mms22 and Mms1 (referred to as the Rtt101 complex), as well as the octomeric Smc5/6 SUMO ligase complex ().Citation2-5,12-17 Similar to Rtt107, these interactors, collectively referred to as the Rtt107 interactome, affect genome stability. For example, Rtt107 and Rtt101 (Rtt: regulation of Ty1 transposition) were initially identified by their mutants' increased rates of retro-transposition, an indicator of genomic instability.Citation18 Moreover, Mms22 and Mms1 were identified through a screen for mutants with MMS sensitivity, another indicator of genome instability.Citation19
Figure 1. The Rtt107 interactome. The six BRCT domains of Rtt107 are depicted as blue ovals. The proteins associated with the N- and C-terminal regions of Rtt107 are indicated. The Smc5/6 complex is drawn based on Duan et al., 2009. The Slx4 scaffold protein associates with Dpb11, while Mms22 associates with Mms1 and Rtt101 as part of a SCF type of ubiquitin E3 complex.
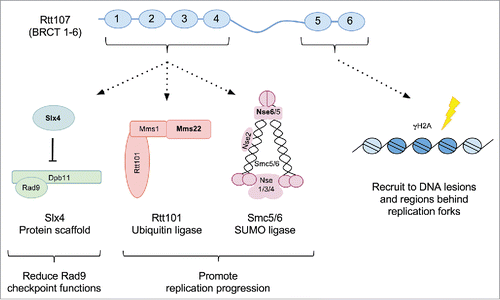
More recent studies from several laboratories have further defined the functions of Rtt107 interactors. In particular, Slx4, in complex with Rtt107, was shown to reduce Rad53 checkpoint kinase mediated phosphorylation, a process termed checkpoint dampening.Citation20-23 Mechanistically, Rtt107-Slx4 disfavors the interaction between checkpoint adaptor Rad9 and checkpoint activator Dpb11, leading to decreased activation of Rad53.Citation20-23 While Rad53 activation allows cells to cope with DNA damage through multiple means, including blocking cell cycle progression, Rad53 dampening enables cells to finish replication after MMS treatment and permits cells to proceed with cell division.Citation20,24
The Rtt101 complex regulates ubiquitination, and a recent study has revealed histone H3 as an important substrate. During replication, newly synthesized histone H3, in association with H4 and chaperone Asf1, is acetylated by the acetyl transferase Rtt109 at lysine 56.Citation25,26 This modification triggers Rtt101 complex-mediated H3 ubiquitination, allowing the H3-H4 dimer to be transferred from Asf1 to downstream chaperones for nucleosome assembly.Citation27 The acetylation event is reversed later in the cell cycle by the deacetylation enzymes Hst3 and Hst4.Citation28,29 Genetic studies suggest that H3K56 acetylation levels are tightly regulated in cells to ensure optimal replication and growth. For example, temperature sensitivity and MMS sensitivity caused by loss of both Hst3 and Hst4 are suppressed by the removal of Rtt109 and the Rtt101 complex, or by mutating H3K56.Citation28-30 Rtt107 loss also suppresses the temperature sensitivity of hst3Δ hst4Δ cells, but does not affect Rtt101-mediated H3 ubiquitination, suggesting that it acts downstream of Rtt101.Citation27,31 For simplicity, the Rtt101 complex and proteins involved in H3 K56 acetylation and deacetylation are hereafter referred to as the H3 modification pathway.
Another Rtt107 interactor, the Smc5/6 complex, has multiple roles in DNA replication and repair. Some of its functions are likely carried out by its SUMO ligase activity, though in many cases, how modification of specific substrates affects a particular process remained to be defined.Citation16,32 Smc5/6 also interacts with and inhibits the DNA helicase Mph1 to prevent excessive levels of recombination intermediates that are harmful to cells.Citation33,34 However, the biological effects of the Smc5/6-Rtt107 complex during replication have been unclear.
While the studies described above elucidate many features of Rtt107 and its interactors, significant gaps in our knowledge regarding this group of proteins remain, particularly the relationship among Rtt107 interactors. Since other multi-BRCT scaffold proteins, such as Dpb11, bind to multiple interactors simultaneously to form one complex,Citation35 Rtt107 and its interactors might follow the same rule to form a mega-complex with the ability to regulate checkpoint phosphorylation, ubiquitination, and sumoylation. Alternatively, Rtt107 may interact with each partner separately to form 3 complexes, each modulating a different type of protein modification. Other outstanding issues include how the Rtt107 interactome contributes to each replication stage, whether Rtt107 interactors have overlapping functions, and what the roles of Smc5/6 are when collaborating with Rtt107?
Stemming from our long-standing interest in the Smc5/6 complex, we identified Rtt107 as an Smc5 interacting protein in an affinity purification-mass spectrometry experiment several years ago. Building upon our work and others, we investigated whether Rtt107 interacted with Smc5/6 and other proteins at the same time or separately. Using complementary approaches, we found that Rtt107 formed independent complexes with each binding partner of its N-terminal BRCT domains, regardless of whether cells were exposed to MMS.Citation1 We observed that Slx4, the Rtt101 complex, and the Smc5/6 complex could all bind to Rtt107, but not each other, and none of these interactions required the other partners. Moreover, while rtt107Δ mutants exhibited defects in checkpoint dampening, the H3 modification pathway, and sumoylation, mutants of each Rtt107 partner only exhibited defects in its relevant pathway and not the others. Specifically, slx4Δ cells, but not rtt101Δ and smc5/6 mutants, were defective in checkpoint dampening, and the rtt101 pathway mutants, but not slx4Δ and smc5/6 mutants, suppressed hst3Δ hst4Δ defects. We also uncovered a unique effect of Rtt107 and Smc5/6 complex in sumoylation, as their mutants, but not slx4Δ or rtt101Δ mutants, were defective in Smc5 sumoylation. This effect is specific for Rtt107, since Mph1, the other known Smc5 binding partner that does not interact with Rtt107, did not affect sumoylation. Collectively, these findings suggest that Rtt107 forms at least 3 complexes whose functions are specified by its binding partner (). In addition, each partner, as exemplified by Smc5/6, has Rtt107-independent roles.
We further investigated Rtt107 and Smc5/6 in sumoylation and found that both were required for optimal sumoylation of selective members of the replication machinery, including subunits of DNA polymerase epsilon and MCM replicative helicase.Citation1 This result is consistent with our finding that Rtt107 interacted with these proteinsCitation1 as well as previous studies showing that Rtt107 and Smc5/6 localize at stalled replication forks.Citation31,36 One interpretation is that Rtt107 and Smc5/6 association aids sumoylation, though other possibilities may exist. The Rtt101 complex also interacts with replisomes through an association between Mms22 and the replisome member Ctf4.Citation14,37 In contrast, Slx4 was recently shown to be targeted by Rtt107 at regions behind replication forks via its interaction with γH2A ().Citation38 It thus appears that the Slx4-Rtt107 complex exhibits a chromatin localization pattern distinct from the SUMO and ubiquitin ligase-Rtt107 complexes.
For a better understanding of how Rtt107-containing complexes collectively promote replication in MMS conditions, we used 2-dimensional gel electrophoresis and genome wide assays to assess replication defects in the absence of Rtt107. We found that Rtt107 loss did not affect the firing of ∼100 early origins or replication progression at regions within 10–20 kb from early origins.Citation1 As the average replicon size in yeast is about 20 kb, Rtt107 loss therefore did not appear to affect the replication of most replicons. However, yeast cells contain more than a dozen large replicons, and we found that their replication was impaired. Specifically, rtt107Δ mutants exhibited reduced levels of replication structures at regions greater than 40 kb from fired origins.Citation1 This defect contrasts with that of replication checkpoint and DNA repair mutants, which exhibit impaired replication progression within 5–10 kb region from origins.Citation39 Consistent with this notion, rtt107Δ and mutants of 4 major DNA repair pathways that act on MMS induced lesions showed synergy in genotoxic sensitivity, suggesting that the lack of DNA repair is unlikely the main cause of our observed defect.Citation1
Based on analysis of replication defects in cells lacking Rtt107, we hypothesized that Rtt107 contributes to the synthesis of DNA regions far from origins.Citation1 To test this model, we examined whether reducing the number of large replicons genome-wide might improve chromosome synthesis in rtt107Δ cells. To this end, we used a null allele of the replisome component Mrc1, mrc1Δ, and a separation of function allele of the Mec1 checkpoint kinase, mec1–100. In an earlier study, mrc1Δ and mec1–100 were placed in a group of mutants that exhibited the highest level of de-repressed origins when cells were treated with hydroxyurea.Citation40 Though Mec1 has other functions, mec1–100 is quite specific in de-repressing origins.Citation41-43 As such, mrc1Δ and mec1–100 allowed us to probe the consequence of increasing origin firing and the concomitant elimination of large replicons. After confirming that mrc1Δ and mec1–100 lead to the expected effects on origin firing in MMS-treated rtt107Δ cells, we found that they rescued replication defects and the MMS sensitivity of rtt107Δ.Citation1 Moreover, the degree of suppression by mrc1Δ and mec1–100 correlated with their ability to increase origin firing.Citation1 These findings support the notion that Rtt107 loss particularly impairs large replicon synthesis, and that the plasticity of the origin-firing program might compensate for this function.
As one cannot rule out whether mrc1Δ and mec1–100 share yet-to-be identified effects that might be relevant to the examined process, we used an alternative strategy to examine the effect of different replicon sizes in rtt107Δ cells. This strategy takes advantage of 2 previously generated chromosome III variants bearing either 12 or 7 origins, with the former containing smaller replicons and the latter larger replicons.Citation44,45 Using pulsed field gel electrophoresis, which distinguishes fully replicated chromosomes from those undergoing replication, we found that both chromosome variants replicated at similar efficiency in wild-type cells; however, the chromosome variant with 12 origins displayed higher levels of synthesis than that with 7 origins in rtt107Δ cells.Citation1 This finding concurs with the results from our experiment in which replicon sizes were altered on a genome-wide scale as described above, and indicates that Rtt107 is required for replicating large replicons and regions far from origins.
Do any Rtt107 partners share this property? As cells lacking a functional Smc5/6 or Rtt101 complex exhibit impaired replication at regions far from origins, and their MMS sensitivity was suppressed by mrc1Δ and mec1–100, these 2 E3s likely cooperate with Rtt107 in promoting replication progression.Citation1 mrc1Δ and mec1–100 suppression did not extend to slx4Δ, highlighting a genetic distinction among the Rtt107-containing complexes.Citation1 We noted that this result does not exclude a role for Slx4-Rtt107 in replication progression, as additional functions of involved factors may interfere with a suppressive effect. We also addressed 2 other plausible explanations for the observed suppression by mrc1Δ or mec1–100, namely Rad53 checkpoint reduction or slower replication. However, our data suggest that these do not account for the suppression, since Rad53 activation in rtt107Δ mrc1Δ cells was not reduced and replisome mutants known to slow replication did not suppress rtt107Δ defects.Citation1
Taken together, parts of our findings suggest the existence of 3 constitutive Rtt107 complexes, each containing a partner specifying a distinct protein modification-based pathway. The mode of Rtt107 interaction with partners appears to differ from that of other multi-BRCT scaffolds, which simultaneously engage multiple partners. Our data have also provided insight into the roles of Rtt107-Smc5/6 in promoting the sumoylation of specific proteins. Future biochemical tests will be needed to elucidate the underlying mechanisms. Our genome-wide and locus-specific replication analysis also revealed that Rtt107 loss did not affect the replication initiation program nor synthesis within 10–20 kb from the origins, but rather reduces DNA synthesis at regions far from origins. That Smc5/6 and Rtt101 complex mutants share this defect and the mrc1Δ and mec1–100 suppression supports the idea that Rtt107 collaborates with these E3 complexes to promote duplication of large replicons. This conclusion implies that replication progression is not a uniform process and the later part of it requires the action of specific factors. This requirement could be due to alterations in replisome properties or cellular states during late S phase. Considering that Rtt107-Smc5/6 affects the sumoylation of MCM helicase and polymerase subunits and that Rtt101 complex affects histone modifications, we favor a model in which Rtt107 and its SUMO and ubiquitin E3s partner help remodel the replisome and surrounding nucleosomes upon fork stalling to promote DNA synthesis across long distances.
In conclusion, it is becoming clear that Rtt107 acts in several replication stress response pathways – each involving a distinct type of post-translational modification (). While we have primarily focused on the relationships between Rtt107 and 3 of its binding partners and how they affect replication in MMS conditions, many questions remain. For example, do the same functions uncovered here also affect the normal replication program? In addition, are there various modes of recruiting Rtt107-based complexes to stalled replication forks? Moreover, the detailed mechanisms by which Rtt107 and its associated E3s facilitate the synthesis of large replicons remains to be defined. Finally, as each member of the Rtt107 interactome is conserved, are the complexes formed by these proteins also present in higher eukaryotes? Future studies of these questions will shed light on how cells convert these Rtt107-based assemblies into an organized response to replication fork stalling, and how they might be co-opted in disease states.
Disclosure of potential conflicts of interest
No potential conflicts of interest were disclosed.
Funding
Work in the Zhao lab is supported by the US National Institutes of Health grant GM080670.
References
- Hang LE, Peng J, Tan W, Szakal B, Menolfi D, Sheng Z, Lobachev K, Branzei D, Feng W, Zhao X. Rtt107 is a multi-functional scaffold supporting replication progression with partner SUMO and ubiquitin ligases. Mol Cell 2015; 60:268-79; PMID:26439300; http://dx.doi.org/10.1016/j.molcel.2015.08.023
- Rouse J. Esc4p, a new target of Mec1p (ATR), promotes resumption of DNA synthesis after DNA damage. EMBO J 2004; 23:1188-97; PMID:14988729; http://dx.doi.org/10.1038/sj.emboj.7600129
- Chin J, Bashkirov V, Heyer W, Romesberg F. Esc4/Rtt107 and the control of recombination during replication. DNA Repair 2006; 5:618-28; PMID:16569515; http://dx.doi.org/10.1016/j.dnarep.2006.02.005
- Roberts T, Kobor M, Bastin-Shanower S, Ii M, Horte SA, Gin JW, Emili A, Rine J, Brill SJ, Brown GW. Slx4 regulates DNA damage checkpoint-dependent phosphorylation of the BRCT domain protein Rtt107/Esc4. Mol Biol Cell 2006; 17:539-48; PMID:16267268; http://dx.doi.org/10.1091/mbc.E05-08-0785
- Zappulla DC, Maharaj ASR, Connelly JJ, Jockusch RA, Sternglanz R. Rtt107/Esc4 binds silent chromatin and DNA repair proteins using different BRCT motifs. BMC Mol Biol 2006; 7:40; PMID:17094803; http://dx.doi.org/10.1186/1471-2199-7-40
- Koonin EV, Altschul SF, Bork P. BRCA1 protein products. Functional motifs. Nat Genet 1996; 13:266-8; PMID:8673121; http://dx.doi.org/10.1038/ng0796-266
- Bork P, Hofmann K, Bucher P, Neuwald AF, Altschul SF, Koonin EV. A superfamily of conserved domains in DNA damage-responsive cell cycle checkpoint proteins. FASEB J 1997; 11:68-76; PMID:9034168
- Gerloff DL, Woods NT, Farago AA, Monteiro ANA. BRCT domains: A little more than kin, and less than kind. FEBS Lett 2012; 586:2711-6; PMID:22584059; http://dx.doi.org/10.1016/j.febslet.2012.05.005
- Woods NT, Mesquita RD, Sweet M, Carvalho MA, Li X, Liu Y, Nguyen H, Thomas CE, Iversen ES, Jr, Marsillac S, et al. Charting the landscape of tandem BRCT domain-mediated protein interactions. Sci Sign 2012; 5:rs6
- Leung CCY, Glover JNM. BRCT domains: easy as one, two, three. Cell Cycle 2011; 10:2461-70; PMID:21734457; http://dx.doi.org/10.4161/cc.10.15.16312
- Li X, Liu K, Li F, Wang J, Huang H, Wu J, Shi Y. Structure of the C-terminal tandem BRCT repeats of Rtt107 reveals a critical role in the interaction with H2A during DNA damage repair. J Biol Chem 2012; 287:9137-46; PMID:22262834; http://dx.doi.org/10.1074/jbc.M111.311860
- Ohouo PY, Bastos de Oliveira FM, Almeida BS, Smolka MB. DNA damage signaling recruits the Rtt107-Slx4 scaffolds via Dpb11 to mediate replication stress response. Mol Cell 2010; 39:300-6; PMID:20670896; http://dx.doi.org/10.1016/j.molcel.2010.06.019
- Leung GP, Lee L, Schmidt TI, Shirahige K, Kobor MS. Rtt107 is required for recruitment of the Smc5/6 complex to DNA double strand breaks. J Biol Chem 2011; 286:26250-7; PMID:21642432; http://dx.doi.org/10.1074/jbc.M111.235200
- Mimura S, Yamaguchi T, Ishii S, Noro E, Katsura T, Obuse C, Kamura T. Cul8/Rtt101 forms a variety of protein complexes that regulate DNA damage response and transcriptional silencing. J Biol Chem 2010; 285:9858-67; PMID:20139071; http://dx.doi.org/10.1074/jbc.M109.082107
- Zaidi IW, Rabut G, Poveda A, Scheel H, Malmstrom J, Ulrich H, Hofmann K, Pasero P, Peter M, Luke B. Rtt101 and Mms1 in budding yeast form a CUL4(DDB1)-like ubiquitin ligase that promotes replication through damaged DNA. EMBO Reports 2008; 9:1034-40; PMID:18704118; http://dx.doi.org/10.1038/embor.2008.155
- Jeppsson K, Kanno T, Shirahige K, Sjögren C. The maintenance of chromosome structure: positioning and functioning of SMC complexes. Nat Rev Mol Cell Biol 2014; 15:601-14; PMID:25145851; http://dx.doi.org/10.1038/nrm3857
- Rouse J. Control of genome stability by SLX protein complexes. Biochem Soc Trans 2009; 37:495-510; PMID:19442243; http://dx.doi.org/10.1042/BST0370495
- Scholes DT, Banerjee M, Bowen B, Curcio MJ. Multiple regulators of Ty1 transposition in Saccharomyces cerevisiae have conserved roles in genome maintenance. Genetics 2001; 159:1449-65; PMID:11779788
- Prakash L, Prakash S. Isolation and characterization of MMS-sensitive mutants of Saccharomyces cerevisiae. Genetics 1977; 86:33-55; PMID:195865
- Ohouo PY, de Oliveira FMB, Liu Y, Ma CJ, Smolka MB. DNA-repair scaffolds dampen checkpoint signalling by counteracting the adaptor Rad9. Nature 2012; 493:120-4; PMID:23160493; http://dx.doi.org/10.1038/nature11658
- Cussiol JR, Jablonowski CM, Yimit A, Brown GW, Smolka MB. Dampening DNA damage checkpoint signalling via coordinated BRCT domain interactions. EMBO J 2015; 34(12):1704-17; PMID:25896509; http://dx.doi.org/10.15252/embj.201490834
- Dibitetto D, Ferrari M, Rawal CC, Balint A, Kim T, Zhang Z, Smolka MB, Brown GW, Marini F, Pellicioli A. Slx4 and Rtt107 control checkpoint signalling and DNA resection at double-strand breaks. Nucleic Acids Res 2015; 44(2):669-82: gkv1080; PMID:26490958; http://dx.doi.org/10.1093/nar/gkv1080
- Jablonowski CM, Cussiol JR, Oberly S, Yimit A, Balint A, Kim T, Zhang Z, Brown GW, Smolka MB. Termination of replication stress signaling via concerted action of the Slx4 scaffold and the PP4 phosphatase. Genetics 2015; 201:937-49; PMID:26362319; http://dx.doi.org/10.1534/genetics.115.181479
- Gritenaite D, Princz LN, Szakal B, Bantele SCS, Wendeler L, Schilbach S, Habermann BH, Matos J, Lisby M, Branzei D, et al. A cell cycle-regulated Slx4-Dpb11 complex promotes the resolution of DNA repair intermediates linked to stalled replication. Genes Dev 2014; 28:1604-19; PMID:25030699; http://dx.doi.org/10.1101/gad.240515.114
- Driscoll R, Hudson A, Jackson SP. Yeast Rtt109 promotes genome stability by acetylating histone H3 on lysine 56. Science 2007; 315:649-52; PMID:17272722; http://dx.doi.org/10.1126/science.1135862
- Han J, Zhou H, Horazdovsky B, Zhang K, Xu RM, Zhang Z. Rtt109 acetylates histone H3 lysine 56 and functions in DNA replication. Science 2007; 315:653-5; PMID:17272723; http://dx.doi.org/10.1126/science.1133234
- Han J, Zhang H, Zhang H, Wang Z, Zhou H, Zhang Z. A Cul4 E3 ubiquitin ligase regulates histone hand-off during nucleosome assembly. Cell 2013; 155:817-29; PMID:24209620; http://dx.doi.org/10.1016/j.cell.2013.10.014
- Celic I, Masumoto H, Griffith WP, Meluh P, Cotter RJ, Boeke JD, Verreault A. The sirtuins Hst3 and Hst4p preserve genome integrity by controlling histone H3 lysine 56 deacetylation. Curr Biol 2006; 16:1280-9; PMID:16815704; http://dx.doi.org/10.1016/j.cub.2006.06.023
- Maas NL, Miller KM, DeFazio LG, Toczyski DP. Cell cycle and checkpoint regulation of histone H3 K56 acetylation by Hst3 and Hst4. Mol cell 2006; 23:109-19; PMID:16818235; http://dx.doi.org/10.1016/j.molcel.2006.06.006
- Collins SR, Miller KM, Maas NL, Roguev A, Fillingham J, Chu CS, Schuldiner M, Gebbia M, Recht J, Shales M, et al. Functional dissection of protein complexes involved in yeast chromosome biology using a genetic interaction map. Nature 2007; 446:806-10; PMID:17314980; http://dx.doi.org/10.1038/nature05649
- Roberts T, Zaidi I, Vaisica J, Peter M, Brown G. Regulation of Rtt107 recruitment to stalled DNA replication forks by the cullin Rtt101 and the Rtt109 acetyltransferase. Mol Biol Cell 2008; 19:171; PMID:17978089; http://dx.doi.org/10.1091/mbc.E07-09-0961
- Murray JM, Carr AM. Smc5/6: a link between DNA repair and unidirectional replication? Nat Rev Mol Cell Biol 2008; 9:177-82; PMID:18059412; http://dx.doi.org/10.1038/nrm2309
- Chen YH, Choi K, Szakal B, Arenz J, Duan X, Ye H, Branzei D, Zhao X. Interplay between the Smc5/6 complex and the Mph1 helicase in recombinational repair. Proc Natl Acad Sci USA 2009; 106:21252-7; PMID:19995966; http://dx.doi.org/10.1073/pnas.0908258106
- Xue X, Choi K, Bonner J, Chiba T, Kwon Y, Xu Y, Sanchez H, Wyman C, Niu H, Zhao X, et al. Restriction of replication fork regression activities by a conserved SMC complex. Mol Cell 2014; 56:436-45; PMID:25439736; http://dx.doi.org/10.1016/j.molcel.2014.09.013
- Wardlaw CP, Carr AM, Oliver AW. TopBP1: A BRCT-scaffold protein functioning in multiple cellular pathways. DNA Repair 2014; 22:165-74; PMID:25087188; http://dx.doi.org/10.1016/j.dnarep.2014.06.004
- Lindroos HB, Strom L, Itoh T, Katou Y, Shirahige K, Sjogren C. Chromosomal association of the Smc5/6 complex reveals that it functions in differently regulated pathways. Mol Cell 2006; 22:755-67; PMID:16793545; http://dx.doi.org/10.1016/j.molcel.2006.05.014
- Buser R, Kellner V, Melnik A, Wilson-Zbinden C, Schellhaas R, Kastner L, Piwko W, Dees M, Picotti P, Maric M, et al. The replisome-coupled E3 ubiquitin ligase Rtt101-Mms22 counteracts Mrc1 function to tolerate genotoxic stress. PLoS Genetics 2016; 12:e1005843; PMID:26849847; http://dx.doi.org/10.1371/journal.pgen.1005843
- Balint A, Kim T, Gallo D, Cussiol JR, Bastos de Oliveira FM, Yimit A, Ou J, Nakato R, Gurevich A, Shirahige K, et al. Assembly of Slx4 signaling complexes behind DNA replication forks. EMBO J 2015; 34(16):2182-97; PMID:26113155; http://dx.doi.org/10.15252/embj.201591190
- Vázquez MV, Rojas V, Tercero JA. Multiple pathways cooperate to facilitate DNA replication fork progression through alkylated DNA. DNA Repair 2008; 7:1693-704; http://dx.doi.org/10.1016/j.dnarep.2008.06.014
- Crabbe L, Thomas A, Pantesco V, De Vos J, Pasero P, Lengronne A. Analysis of replication profiles reveals key role of RFC-Ctf18 in yeast replication stress response. Nat Struct Mol Biol 2010; 17:1391-7; PMID:20972444; http://dx.doi.org/10.1038/nsmb.1932
- Paciotti V, Clerici M, Scotti M, Lucchini G, Longhese MP. Characterization of mec1 kinase-deficient mutants and of new hypomorphic mec1 alleles impairing subsets of the DNA damage response pathway. Mol Cell Biol 2001; 21:3913-25; PMID:11359899; http://dx.doi.org/10.1128/MCB.21.12.3913-3925.2001
- Tercero JA, Longhese MP, Diffley JF. A central role for DNA replication forks in checkpoint activation and response. Mol Cell 2003; 11:1323-36; PMID:12769855; http://dx.doi.org/10.1016/S1097-2765(03)00169-2
- Zhong Y, Nellimoottil T, Peace JM, Knott SRV, Villwock SK, Yee JM, Jancuska JM, Rege S, Tecklenburg M, Sclafani RA, et al. The level of origin firing inversely affects the rate of replication fork progression. J Cell Biol 2013; 201:373-83; PMID:23629964; http://dx.doi.org/10.1083/jcb.201208060
- Theis JF, Irene C, Dershowitz A, Brost RL, Tobin ML, di Sanzo FM, Wang JY, Boone C, Newlon CS. The DNA damage response pathway contributes to the stability of chromosome III derivatives lacking efficient replicators. PLoS Genetics 2010; 6:e1001227; PMID:21151954; http://dx.doi.org/10.1371/journal.pgen.1001227
- Theis JF, Dershowitz A, Irene C, Maciariello C, Tobin ML, Liberi G, Tabrizifard S, Korus M, Fabiani L, Newlon CS. Identification of mutations that decrease the stability of a fragment of Saccharomyces cerevisiae chromosome III lacking efficient replicators. Genetics 2007; 177:1445-58; PMID:17720931; http://dx.doi.org/10.1534/genetics.107.074690