ABSTRACT
The LINC complex is a multifunctional protein complex that is involved in various processes at the nuclear envelope, such as nuclear migration, mechanotransduction and chromatin tethering in the meiotic phase. However, it remains unknown how these functions are regulated in different cell contexts. An inner nuclear membrane component of the LINC complex, SUN1, is ubiquitously expressed. The human SUN1 gene produces over 10 variants by alternative splicing. Although functions of SUN1 are relatively well characterized, functional differences among SUN1 splice variants are poorly characterized. LINC complex components are associated with a wide range of human diseases; therefore, it is important to understand the functional diversity among SUN1 splice variants. Here, we identified a novel human SUN1 splice variant, SUN1_888. overexpression of the SUN1 splice variants, SUN1_888 or SUN1_785, but not the predominant isoform, SUN1_916, activated directional cell migration. Knockdown of SUN1_888 suppressed cell migration; in contrast depletion of SUN1_916 activated cell migration. In addition, all of investigated SUN1 splicing variants rescued cell migration in SUN1 knock out cell. These results indicate that redundant and non-redundant functions of SUN1 splice variant in directional cell migration and suggest that variable LINC complexes with distinct task may exit. Furthermore, in contrast to previous studies, we showed association between SUN1 and B-type lamins. Interestingly, B-type lamin preferentially interacts with SUN1 but not SUN2. These results suggest that tissue-specific SUN1 variants variably interact with nucleoplasmic partners and allow variable assembly of LINC complexes that can be assigned to distinct tasks.
Introduction
The Sad1-UNC-84 (SUN) homology domain proteins of the inner nuclear membrane (INM) are widely conserved in all eukaryotes and share a common C-terminal motif of ∼200 amino acids, termed the SUN domain. SUN proteins and the outer nuclear membrane (ONM)-spanning nesprin proteins interact with each other in the perinuclear space via their luminal domains, SUN and KASH (Klarsicht/Anc1/Syne1 homology), respectively, to form a multifunctional nuclear membrane protein assembly, the LINC complex (linker of nucleoskeleton and cytoskeleton).Citation1 SUN proteins interact with the nucleoskeleton, including chromatin and the nuclear lamina and nesprins interact with the cytoskeleton; therefore, the LINC complex physically connects the nuclear interior to the cytoskeleton.Citation1-4 Mammalian SUN proteins are encoded by at least 5 genes, SUN1∼5. SUN1 and SUN2 are widely expressed in mammalian somatic cells.Citation1 SUN3, SUN4 (sperm associated antigen4, SPAG4), and SUN5 (SPAG4 Like, SPAGL) are largely, but not entirely, restricted to the germ cells.Citation5-9
LINC complexes and nuclear lamins form a solid scaffold for diverse functions including nuclear migration,Citation10 nuclear shaping and positioning,.Citation11,12 maintaining the centrosome-nucleus connection,Citation13,14 mechanotransduction,Citation15,16 DNA repair,Citation17,18 nuclear membrane spacing,Citation19 cell migration,Citation14,20-23 and moving chromosomes within the nucleus during meiosis.Citation24 Because these functions are critical for cell viability, the LINC complex and its interacting lamins are associated with a wide range of diseases, including muscular dystrophy, lipodystrophy, progeria, cancer, and recessive cerebellar ataxia.Citation25,26,27 However, for several reasons, it is not known how cells regulate these multiple LINC complex functions. First, mammalian somatic cells express at least 2 SUN domain proteins, SUN1 and SUN2, which have partially overlapping functions, and up to 4 KASH domain partners (i.e., nesprins 1, 2, 3 and 4). Each LINC complex is composed of 3 SUN and 3 nesprin molecules.Citation28,29 Therefore, there is a diverse range of LINC complexes that connect nuclear structures to different cytoskeletal elements. Second, various post-translational modifications can regulate diverse functions. For example, in human and C. elegans, SUN1 is phosphorylated in cell cycle progression, and the yeast SUN1 homolog, Mps3, is acetylated in the region upstream of the transmembrane domain during chromosome recruitment to the nuclear membrane.Citation30-32 Third, in addition to variations of oligomer formation and post-translational modification, alternative splicing generates a huge number of SUN and nesprin variants.Citation4,33,34 The human SUN1 gene is located at chromosome position 7p22.3 and has 22 exons that encode up to 916 amino acids residues. Thus splice variants of SUN and nesprin may be responsible for diverse functions via different interacting components. In contrast to the well characterized functional diversity of nesprin variants, SUN1 splice variants have been collectively studied, assuming they function as singly entity and thus variant specific functions remain unknown. This study aims to determine the splice variant-specific functions of SUN1. As a variant-specific function, we focused on cell migration because LINC complex-mediated nucleus-cytoskeleton connections have an essential function in cell migration.
Results
SUN1 splice variants in human cells
The human SUN1 gene generates more than 10 splice variants that are distinguished by variable deletions just upstream from the transmembrane domain, between exons 6 and 9 (). The largest human SUN1 splice variant is composed of 22 exons and encodes 916 amino acids residues (aa) (EAW87177).Citation35 This variant, which is referred to as SUN1_916 in this report, corresponds to the largest mouse SUN1 variant, composed of 913 aa, that predominates in most mouse tissues.Citation34 In addition to well-known variants, we identified a novel SUN1 splice variant; SUN1_888, which encodes 888 aa (AB648918, and , and details in the Materials and Methods). As previously reported,Citation1 SUN1 expression was ubiquitous when a universal SUN1 primer set was used (Fig. S1 and Table S1). To examine the expression levels of individual SUN1 splice variants, a primer set was designed at the junctions of exon 3/4 and exon 10/11, which amplifies the variable region as different amplicon sizes ( and Table S1). Reverse transcription-polymerase chain reaction (RT-PCR) analysis of mRNA from various human tissues showed at least 7 discrete SUN1 transcripts (, 6 variants in the upper panel and a testis specific variant in the lower panel). Amplicon sizes of SUN1_916, SUN1_888, and SUN1_785 were confirmed using plasmids as positive controls (data not shown). The largest splice form, SUN1_916, was ubiquitously expressed as the predominant product; however, other variants were expressed in a tissue-dependent manner suggesting diverse and distinct functions. In addition to the tissue-specific expression pattern of SUN1 variants, the expression of SUN1 in cultured cells, including both normal and tumor cells, was also variable (Fig. S1B). It is noteworthy that western blot analysis of total cell lysate of various cultured human cells using an anti-SUN1 polyclonal antibody (pAb), which recognizes the domain common to all variants, mainly showed a single band around 100 kDa which corresponds to the exogenously expressed SUN_916 (Fig. S1C). Transfection with siRNA specifically targeting SUN1_916 reduced the intensity of this band (see below, Fig. S1E). This band disappeared with hundred-fold dilution of the cell lysates (Fig. S1D) suggesting that expression levels of other splice variants in cultured cells are not detectable by western blotting. To detect other SUN1 variants, cells were lysed in RIPA buffer and then immunoprecipitation was performed with anti-SUN1 or anti-SUN2 pAbs. The anti-SUN1 pAb detected both SUN1_916 and faint smaller bands in input cell lysate (). In addition, the endogenous smaller SUN1 variants were detected in both anti-SUN2 and anti-SUN1 immune-pellets (). These results demonstrate that although SUN1_916 is predominant, the smaller SUN1 variant proteins are actually expressed. The data also suggest that SUN2 may preferentially bind to the smaller SUN1 variants.
Figure 1. Structure and expression of SUN1 splice variants. (A) A comparison of SUN1 splice variants, including a novel variant, SUN1_888. The SUN1 gene contains 22 exons, which are indicated by black boxes. Lines are introns. Exons 6−9 are alternatively spliced to generate numerous SUN1 variants. SUN1 variant, SUN1–001, composed of 822 aa (ensemble SUN1–001), UniprotKB-O94901 variant composed of 812 aa (KIAA0810, Nagase et al., 1998), SUN1 variant1 composed of 785 aa (NM_001130965), and testis specific SUN1 variant2 composed of 702 aa (NM_025154), are here referred to as SUN1_822, SUN1_812, SUN1_785, and SUN1_702, respectively. (B) Schematic diagram of SUN1_888, SUN1_785, and SUN1_916 proteins and amino acid sequence alignment of the variable region of SUN1 proteins. Human SUN1 proteins are single pass type II transmembrane proteins of the INM with an approximately 450 amino acid C-terminal region extending into the perinuclear space (PNS), which includes the SUN domain, and an approximately 200−400 amino acid N-terminal region extending into the nucleoplasm.Citation1,33 Identical residues in the amino- and carboxy-regions are shown as solid lines. Functional domains are indicated; the transmembrane domain (TM) and the lamin A/C binding site are retained in all of these variants. SUN domains are boxed.Citation1,5 (C) Expression of various SUN1 splice variants in human tissues was analyzed by reverse transcription PCR (RT-PCR). The amplicons were analyzed in 6% TBE polyacrylamide gels. Similar to mouse SUN1,Citation34 the shortest SUN1 variant, SUN1_702, could be detected exclusively in testis but not in any somatic tissues tested. * corresponds to a non-specific amplicon, as judged by direct sequencing and SUN1 knockdown (data not shown). Exon 7-, 8-, or 6-deleted SUN1 variants were not found in any database but we detected corresponding bands (indicated as ***) in various tissues, including the brain, heart and skeletal muscle. (D and E) HeLa cells were lysed with RIPA buffer and immunoprecipitated with indicated antibodies. Then input lysate (D) or precipitated proteins (E) were analyzed by western blotting using anti-SUN1 pAb.
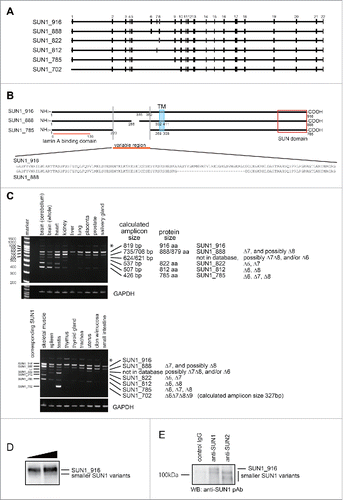
Depletion of SUN1 suppresses cell migration
Two major roles of the LINC complex are nuclear anchoring and nuclear migration,Citation10,11,12 which is fundamental step for directional cell migration. Expression of a dominant-negative KASH in mouse MEF cells decreased directional cell migration,Citation36 suggesting that interaction(s) between SUN and nesprin is important for cell migration. Moreover, it has been reported that nesprin2 and SUN2 play roles in cell migration in transmembrane actin-associated nuclear (TAN) lines.Citation14,20 However it is not clear whether SUN1 is involved in cell migration and if so which SUN1 variants are important. Specific siRNA pools targeting SUN1 (siSUN1) or SUN2 (siSUN2, Table S2) were transfected into MDA-MB-231 cells, which are aggressive breast cancer cells and show high motility. After incubation for 48 hours, knockdown efficiency was examined by western blotting () and cell migration activity was quantified using a Boyden chamber. As expected from TAN line function, cell migration was reduced in SUN2-depleted cells. However, the level of reduction was not large; migration was approximately 70% of that of non-treated or negative control siRNA-transfected cells (). In contrast, cell migration of SUN1-depleted cells was more obviously reduced. The level of cell migration in SUN1-depleted cells was approximately 10% of that of control cells () indicating a critical function for SUN1 in cell migration. The cell migration activity in double SUN1/SUN2-depleted cells was similar to that in SUN1-depleted cells suggesting a critical or upstream function of SUN1 in cell migration. Cell invasion requires cell migration; therefore, we also examined cell invasion activity using trans-wells. As expected, SUN1 depletion remarkably suppressed cell invasion compared with negative control siRNA-transfected or SUN2-depleted cells (Fig. S2A).
Figure 2. SUN1 and SUN2 are essential for cell migration. (A) MDA-MB-231 cells were transfected with siRNA pools that specifically target SUN1 or SUN2, termed siSUN1 or siSUN2, respectively, or with non-targeting siRNA as a negative control. Forty-eight hours later, total cell lysates were analyzed by western blotting using anti-SUN1 or anti-SUN2 pAbs. (B) Forty-eight hours after SUN1 and/or SUN2 knockdown, cell migration activities were analyzed. Each bar represents the mean number of cells per field ± SD. *; p < 0.05, **; p < 0.01.
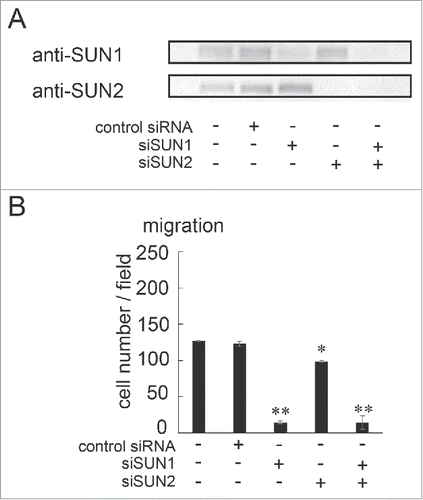
Expression of SUN1_785 and SUN1_888 promotes cell migration
To confirm the involvement of SUN1 in cell migration, SUN1 proteins were transiently overexpressed in MDA-MB-231 cells. This study focused on the SUN1 splice variants, SUN1_785, SUN1_888 and SUN1_916, because the mRNA expression levels of these variants are higher than those of other SUN1 variants in human tissues (). Each GFP-tagged splice variant or GFP alone, as a control, was transfected into cells. Twenty-four hours after transfection, expression of SUN1 variants was analyzed by western blotting using an anti-GFP pAb and migration activity was examined using a Boyden chamber. All transfected SUN1 variants were expressed at the expected protein size (). Surprisingly, SUN1_785 or SUN1_888 overexpression promoted statistically significant cell migration while there was no detectable change in cell migration between SUN1_916-transfected and non-transfected cells (). This result was also observed in aggressive human sarcoma HT1080 cells; overexpression of SUN1_785 and SUN1_888 but not SUN1_916 significantly activated cell migration (Fig. S2B and C). Moreover, SUN1_785 and SUN1_888 but not SUN1_916 overexpression also promoted cell invasion activity both in MDA-MB-231 and HT1080 cells (Fig. S2D and E).
Figure 3. overexpression of SUN1_785 and SUN1_888 but not SUN1_916 promotes cell migration activities. (A) MDA-MB-231 cells were transfected with GFP-tagged SUN1 variants (GFP-SUN1_785, GFP-SUN1_888, GFP-SUN1_916), or GFP alone. After incubation for 24 hours, total cell lysates were separated by a 10% acrylamide gel and analyzed by western blotting. (B) Cell migration activities were analyzed 24 hours after transfection. Transfection efficiency was ∼70% (determined using a Tali image cytometer) and all cells were counted including GFP-positive and -negative cells. Each bar represents the mean number of cells per field ±SD. *; p < 0.05, **; p < 0.01.
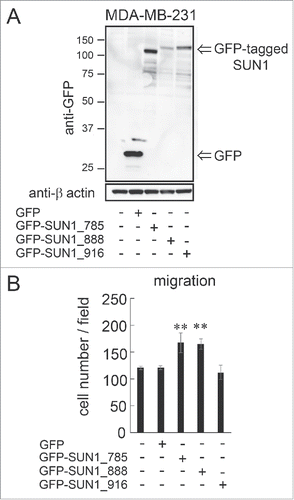
SUN1_888 but not SUN1_916 is essential for cell migration
To further explore the functions of SUN1_888 and SUN1_916 in cell migration, each SUN1 splice variant was depleted in MDA-MB-231 cells using siRNA. Two SUN1_916 variant-specific siRNAs were designed and termed siSUN1_916(#1) and siSUN1_916(#2). Targeting positions are shown in . A SUN1_888 variant-specific siRNA was designed to junction of exon 6 and exon 8 and termed siSUN1_888 (). It was not possible to design an siRNA specifically targeting SUN1_785 because the SUN1_785 sequence mostly overlaps those of SUN1_916 and SUN1_888. Cells were transfected with indicated siRNAs and incubated for 48 hours. Then, migration activity was analyzed. Although the treatment with siSUN1_916(#1) or siSUN1_916(#2) reduced SUN1_916 mRNA expression, the cell migration activity was not decreased, but was instead activated (). By contrast, depletion of SUN1_888 or all SUN1 splice variants strongly suppressed cell migration (). The knockdown efficiency was confirmed by RT-PCR with the splice variant detecting primer sets used in (). Again there was no significant difference in cell invasion between SUN1_916-depleted and control siRNA-transfected cells, while depletion of SUN1_888 or all SUN1 variants clearly suppressed invasion activity (Fig. S3). These results indicate that SUN1_888 is essential for directional cell migration, instead the dominant isoform, SUN1_916 has inhibitory effects.
Figure 4. SUN1_888 but not SUN1_916 is required for cell migration. (A) Design of siRNA against SUN1 splice variants. (B) and (C). MDA-MB-231 cells were transfected with siRNA against SUN1_916, SUN1_888, total SUN1, or non-targeting siRNA and after 48 hours, total RNAs were analyzed by PCR (B) and migration activities were analyzed (C). Each bar represents the mean number of cells per field ±SD. *; p < 0.05, **; p < 0.01.
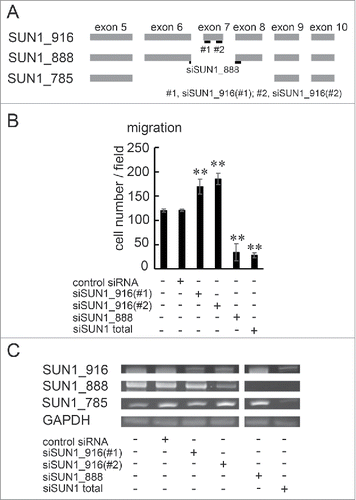
SUN1 interacts with B-type lamin
The variable region of SUN1 is located in the nucleoplasmic domain, which interacts with nuclear components such as lamins.Citation1,5 To achieve the multiple functions mentioned above, SUN proteins associate with various binding partners. To investigate this, we performed a yeast-2 hybrid screen using the nucleoplasmic domain of SUN1_888 as bait (see Materials and Methods) and identified lamin B2 as the sole prey with high confidence. We then examined the interaction between SUN1 and lamin B2 by immunoprecipitation. It was previously reported that SUN1 and SUN2 interact with lamin A while SUN1 binding to B-type lamins is very weak.Citation1,5 To explore SUN1 interacting proteins, GFP-tagged SUN1 splice variants, SUN1_916, SUN1_888, and SUN1_785, were transfected into HeLa cells. Then GFP-tagged proteins were precipitated with an anti-GFP pAb. As expected, all precipitates showed the interaction between lamin A and the 3 SUN1 splice variants. Our results also showed that both lamin B1 and lamin B2 associate with all the investigated SUN1 splice variants (), while association between SUN2 and B-type lamins was not detected (). In addition, having observed the association between SUN1 and NPC, we investigated whether SUN1 interacts with nucleoporins. Actually, mAb414 detected several Nups in the anti-SUN1 immune-pellet ( and data not shown).
Figure 5. SUN1 interacts with B-type lamins. (A) Carboxy-terminus GFP-tagged SUN1 variants or GFP were transfected into HeLa cells and precipitated with anti-GFP pAbs. Then co-precipitated proteins were subjected to western blotting using indicated antibodies. (B) Carboxy-terminus GFP-tagged SUN1 variants or GFP-tagged SUN2 was transfected into HeLa cells and precipitated with anti-GFP pAbs. Then co-precipitated proteins were subjected to western blotting using anti-GFP, -lamin B1, and -lamin B2 antibodies. (C) The location of primer sets are indicated. Detailed sequence information is shown in Table S1. (D and E) Genomic DNA was obtained from parental HeLa, clone #1, or clone #2 cells. Then PCR was performed using indicated primer sets. Clone #1 showed single allele disruption, while clone #2 showed biallelic disruption. (F) SUN1 mRNA expression in parental HeLa, clone #1, and clone #2 cells was measured by real-time PCR. Results are presented as means ±SD. The values were normalized against GAPDH mRNA. (G) The expression level of SUN1 was analyzed by western blotting using anti-SUN1 pAbs and anti-actin mAb. (H) GFP tagged SUN1 variants or GFP were transfected into SUN1 knockout HeLa clone #2 cells and immunoprecipitation was performed with anti-GFP pAbs. Then precipitated proteins were subjected to western blotting using indicated antibodies.
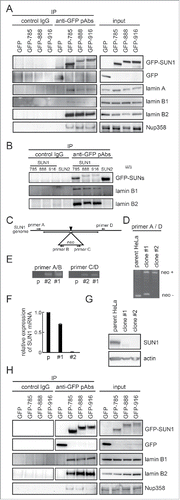
A LINC complex contains 3 SUN1 molecules; therefore, transiently transfected GFP-tagged SUN1 may form a LINC complex with other endogenous SUN1 splice variants or SUN2 and it is difficult to conclude which SUN1 variant interacts with lamin B2 and lamin B1. To clarify this point, we attempted to obtain SUN1 knockout cells. HeLa cells were transfected with pX330-SUN1 along with the donor plasmid pNT1.1-SUN1. The SUN1 gene disruption of the resultant G418-resistant colonies was analyzed by PCR. One clone, termed clone #2, showed biallelic gene disruption (–). Clone #2 cells did not express SUN1 mRNA or protein ( and ). GFP-tagged SUN1_785, SUN1_888, and SUN1_916 were transfected into clone #2, and immunoprecipitation using the anti-GFP pAbs was again performed. The result showed that both lamin B1 and lamin B2 clearly interact with all the SUN1 splice variants ().
Finally, using the SUN1 knockout HeLa cell line, the contribution of the SUN1 variants to cell migration was examined. The SUN1 knockout cell line exhibited decreased migration activity than parental HeLa cells. The decreased migration activity was rescued by overexpression of GFP-tagged SUN1_785 or SUN1_888. In addition, unexpectedly, expression of SUN1_916 in SUN1 knockout cells also rescued migration (discussed below).
Discussion
The human SUN1 gene undergoes alternative splicing to generate more than 10 splicing variants (Ensemble, www.ensembl.org/). The present study demonstrated functional diversity among the SUN1 splice variants in cell migration. In addition, we revealed that SUN1 variants interact with B-type lamin, which differs from the previously demonstrated weak binding between B-type lamins and SUN1.
Redundant and non-redundant functions of SUN1 splice variants in directional cell migration
A dominant-negative KASH protein mutant suppressed directional cell migration,Citation36 suggesting that an interaction(s) between SUN(s) and nesprin(s) is essential for cell migration. SUN2 is required for fibroblast cell migration as a TAN line member.Citation20 However, SUN2 depletion in cultured cancer cells promotes cell migration and its expression is decreased in breast and lung cancer tissues.Citation37,38 Until now, the SUN1 requirement for cancer cell migration has not been examined. Here, we revealed that knockdown of the SUN1 protein, but not SUN2 protein, robustly suppressed cell migration. Depletion of both SUN1 and SUN2 did not show further suppression. These results indicate a critical or upstream function of SUN1 rather than SUN2 in cancer cell migration.
In addition, SUN1 variant-specific knockdown and overexpression experiments in wild type cells expressing different SUN1 variants () showed that SUN1 variants, SUN1_888 and SUN1_785 are crucial for directional cell migration, whereas the predominant and largest SUN1 splice variant, SUN1_916 has an inhibitory effect on cell migration. Therefore, SUN1 splice variants have a non-redundant function in cell migration. Surprisingly, in contrast, in SUN1 knockout HeLa cells, all the investigated SUN1 variants, i.e., SUN1_785, SUN1_888, and SUN1_916, rescued cell migration (). This indicates that the SUN1 variants play redundant roles in cell migration.
Figure 6. Rescued the cell migration of SUN1 knockout cells. (A) SUN1 knock out HeLa cells (clone #2) were transfected with GFP tagged SUN1 variants. Twenty-four hours after transfection, cell migration activities of parental HeLa cells, untransfected clone #2 and the SUN1 variants transfected cells were analyzed. Cells were stained with hematoxylin. Representative images are shown. Small holes in the images are pore of the membrane. (B) The number of migrated cells was counted. Each bar represents the mean number of cells per field ±SD.
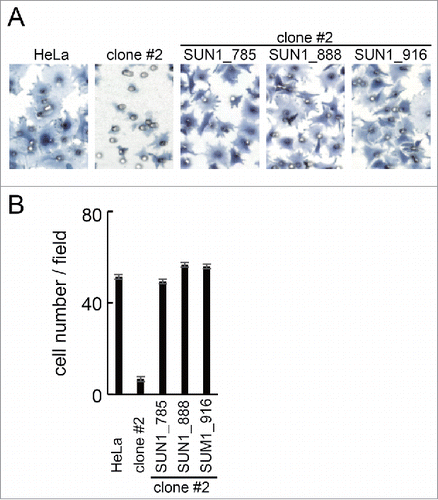
SUN1_916 has an inhibitory effect on cell migration in several SUN1 variant-expressing cells (), while SUN1_916 showed a positive effect on cell migration in the SUN1 knockout cell line (). How can this complicated situation be explained? Because a LINC complex includes 3 SUN molecules (either SUN1 or SUN2 in somatic cells) and different combinations of SUN1 variants and SUN2 are possible, we hypothesize that variable LINC complexes with distinct cellular tasks may exist. In this scenario, we assume the involvement of different SUN molecules confers distinct functions; i.e., suppression, promotion or no effect on cell migration. The first LINC complex (red arrow in Fig. S4, left) containing only SUN1_916 suppresses cell migration. This is supported by the observations that SUN1_916 is the predominant form, that the smaller SUN1 variants have higher affinity to SUN2 than to SUN1_916 and that decreased levels of SUN1_916 activated cell migration (). The second complex containing only SUN2 (black arrow in Fig. S4, middle) does not affect cell migration, which is supported by our observations that SUN1 knockdown reduced cell migration ( and ), whereas SUN2 knockdown did not change cell migration. The third complex consists of different combinations of SUN1, SUN2 and SUN1 variants (blue arrow in Fig. S4, right) activates cell migration. Although these possibilities need to be experimentally examined, the idea of these variable LINC complexes can clearly explain all of the observed results. It is noteworthy in this context that some tissues express higher levels of SUN1_888 or SUN1_785 compared with SUN1_916, and aggressive human cancer cells, such as HT1080, express less SUN1_916 compared with non-cancerous cells.
Regulation of protein levels of SUN1 splice variants
The RT-PCR results showed that several SUN1 transcripts were expressed and that the expression levels of SUN1_916 and SUN1_888 were similar. However, western blot analysis showed that the level of SUN1_916 was much higher than that of other variants, although smaller variants can also be detected by immunoprecipitation (). These results indicate that the protein level of SUN1 might be regulated through post-transcriptional mechanisms, such as miRNA or protein degradation, as suggested by Chen et al.Citation40
Pathological functions of the LINC complex
Mutations in genes encoding components of the LINC complex and its associating proteins, such as lamins or emerin, are related to a wide range of tissue-restricted inherited disorders. These are termed laminopathies and can affect striated muscle, bone, fat or neurons and in some cases can cause premature aging syndromes.Citation25,26,27 There are several proposed mechanisms to explain the tissue specificity of laminopathies. SUN1 expression is ubiquitous, but the expression level of splice variants is tissue-specific (). For example, in muscle cells, SUN1_888 is dominantly expressed (). In addition, mutations just up or downstream of the variable region were found in muscular dystrophies (A201V, G338S, W377C).Citation39 Therefore, non-redundant function of SUN1 variants might contribute to the pathogenic mechanism of laminopathies.
Previously, we showed decreased SUN1 expression in human breast cancer tissue by immunohistochemistry using an anti-SUN1 pAb that recognizes all splice variants; however, it is an open question as to which SUN1 variant is reduced.
Human SUN1 splice variants interact with B-type lamins
It was reported that overexpressed SUN1 or deletion mutants of SUN1 are mis-localized to the Golgi apparatus and simultaneously induce Golgi localization of lamin B1,Citation33,40 suggesting an association between SUN1 and lamin B1. However, weak interaction between mammalian SUN1 and B-type lamin has been reported.Citation1,5 In this study we demonstrated that both lamin B1 and lamin B2 interact with all of the investigated SUN1 variants (). One reason for the difference between previous reports and our results may be either the experimental system or the species from which the SUN1 derived; here we used human SUN1 transfected into cultured cells and endogenously expressing lamins, whereas previous reports used in vitro translated mouse SUN1 and lamin, suggesting the interaction between B-type lamins and SUN1 might require post-translational modification(s) or that there might be a difference between mouse and human SUN1.
Is the interaction between SUN1 variants and B-type lamins relevant to neuronal cell migration?
Coffiner et al., showed that both lamin B1 and lamin B2 are essential for brain development.Citation41,42 Lamin B1 deficiency resulted in striking abnormalities in the shape of nuclei in cortical neurons.Citation42 Lamin B2-deficient mice show a failure of cortical neuron migration and organization into proper layers within the cerebral cortex during development. This phenotype is reminiscent of lissencephaly and indicates that lamin B2 is crucial for cell migration during neural development.Citation42,43 Most strikingly, SUN1/SUN2-deficient mice also show similar defects in neuronal cell migration during development.Citation13 In addition, it was recently shown that SUN1 deficiency leads to cerebellar ataxia.Citation44 Here we show an interaction between lamin B2 and SUN1, which may be important in neuronal migration and may indicate how B-type lamins contribute to brain development and function. It is noteworthy that Lamin B1 and lamin B2 are each expressed at high levels in different neurogenic regions of the brainCitation45 and that the expression of SUN1_785 mRNA is dominant in the cerebellum (). Therefore we assume that interaction between SUN1 and B-type lamins is important in neuronal cell migration.
Materials and methods
Cell culture and transfection
HeLa cells were maintained as described in previously.Citation38 MDA-MB-231 cells were described by Yokoyama et al., 2013.Citation46 Transfections were performed using Lipofectamine 2000 (Invitrogen) or Gene Juice (Merck Millipore). Unless stated otherwise 18–20 hours after transfection, cells were used.
Antibodies and reagents
Rabbit anti-GFP pAbs (catalog number 598) were from Medical & Biological Laboratories, MBL. Rabbit anti-SUN1 pAbs (catalog number HPA008346), whose epitope is located in the luminal domain, were from Sigma-Aldrich. Rabbit anti-SUN2 pAbs (catalog number 06–1038) were obtained from Millipore. Mouse anti-β-tubulin mAb was purchased from Sigma-Aldrich (T4026). Goat anti-lamin B pAbs (M-20, sc-6217), which mainly react with lamin B1, and Goat anti-lamin A/C pAbs (N-18, sc-6215) were from Santa Cruz Biotechnology. Mouse anti-lamin A mAb was from Acris (BM4500, clone 133A2). Mouse anti-lamin B2 mAb and mAb414 were from Millipore (MAB3536) and BAbCO (MMS-120P), respectively. Anti-β-actin mAb (A1978) was from Sigma Aldrich.
Western blotting
Cell lysate or immunoprecipitated samples were separated in 10% or gradient precast gel (SuperSep Ace, Wako) and transferred to nitrocellulose using iblot 2 (Invitrogen). The membrane was blocked with 3% skim milk, and then incubated with the first antibody followed by HRP-conjugated second antibodies.
Cloning of SUN1 variants and plasmid construction
For the cloning of SUN1, the coding region of human SUN1 was amplified by PCR using HeLa cell cDNA as a template and primer sets, 5′-AAATTAGATCTGCCACCATGGATTTTTCTCGGCTTCACATGTACAGT-3′ and 5′-AAATTGTCGACCTTGACAGGTTCGCCATGAACTCTGAACCG-3′, which correspond to the N-terminal and C-terminal regions of SUN1_916 (EAW87177.1), respectively. A PCR fragment was cloned into the BglII/SalI sites of pEGFP-N3 (Clontech) and sequenced. The PCR fragment contained 2667 bp and encodes a 888 aa protein. The nucleotide sequence completely overlapped the SUN1 locus (7p22) in the genome (); however, part of the nucleotide sequence did not overlap known SUN1 variants. In addition, we could not find another genome sequence for SUN1_888 by Blast searches (data not shown). Alignments of these SUN1 variants with the human genome database in Spidey (http://www.ncbi.nlm.nih.gov/spidey/) showed this fragment to be a splice variant of SUN1. Therefore we termed this fragment SUN1_888 and registered it as AB648918.
SUN1_916 (EAW87177), was amplified by overlapping PCR using HeLa cDNA as a template and primer sets 5′-AAATTAGATCTGCCACCATGGATTTTTCTCGGCTTCACATGTACAGT-3′ and 5′-ATTCTCCCATAGTAACTGGCATGAG-3′ for the first half and 5′-CTCATGCCAGTTACTATGGGAGAAT-3′ and 5′-AAATTGTCGACCTTGACAGGTTCGCCATGAACTCTGAACCG-3′ for the second half. The second PCR was performed with 5′-AAATTAGATCTGCCACCATGGATTTTTCTCGGCTTCACATGTACAGT-3′ and 5′-AAATTGTCGACCTTGACAGGTTCGCCATGAACTCTGAACCG-3′ which correspond to N-terminal and C-terminal regions of SUN1_916, respectively. The PCR fragments were inserted into pEGFP-N3. The sequence showed that 4 nucleotides were deleted from the resultant fragment. Thus, the deleted 4 nucleotides were inserted using the primer set, 5′-TTCCTTTTACTAGCAGGTCTCTCCTTACGG-3′ and 5′-GAGTGGGATGAGCAAGACTAAAAACTTGCA-3′ by inverse PCR. For the cloning of SUN1_916, one Shot TOP10 Chemically Competent E. coli (Life Technology) were used.
Human SUN1 variant 1 (SUN1_785; NM001130965) was amplified by overlapping PCR using SUN1_888 as a template and primer sets 5′-AAATTAGATCTGCCACCATGGATTTTTCTCGGCTTCACATGTACAGT-3′ and 5′-TGCAGCAAGAAGTAACATTTTTGATTCCTGTC-3′ for the first half and 5′-CAGGAATCAAAAATGTTACTTCTTGCTGCAGA-3′ and 5′-AAATTGTCGACCTTGACAGGTTCGCCATGAACTCTGAACCG-3′ for the second half. The second PCR was performed with 5′-AAATTAGATCTGCCACCATGGATTTTTCTCGGCTTCACATGTACAGT-3′ and 5′-AAATTGTCGACCTTGACAGGTTCGCCATGAACTCTGAACCG-3′. Then PCR products were inserted into pEGFP-N3.
Reverse transcription-polymerase chain reaction (RT-PCR)
Total RNA was isolated using a PureLink RNA mini kit (Life technologies). A human total RNA panel was purchased from Takara Bio. RNA were reverse-transcribed using Takara Prime Script RT reagent. PCR amplification was performed using Emerald Amp PCR (Takara Bio) and primers indicated in Table S1. PCR products were separated on 6% TBE polyacrylamide (Novex®, Invitrogen) or agarose gels. All PCR products span the intron region of the gene.
siRNA Knockdown
Cells were transfected with targeting siRNA (Table S2) or a non-targeting siRNA pool (Thermo Scientific) using RNAi Max (Invitrogen). siRNAs against SUN1_888 and SUN1–916 specific regions (Table S2) were designed by and obtained from Nippon Gene. Predesigned siRNA pools (4 siRNAs) against SUN1 and SUN2 were obtained from Thermo Scientific. Each siRNA pool contained 4 siRNAs and used at 10 nM unless otherwise indicated. Cells were used 48 hours later unless otherwise indicated. To verify knockdown efficiency, the expression of the corresponding mRNA was detected by RT-PCR using appropriate primer sets (Table S1).
Establishment of a SUN1 knockout cell line
The guide sequence was designed using Optimized CRISPR design (http://crispr.mit.edu/). An annealed synthetic oligo DNA pair 5′-CACCGAAACTACGGCGGGACATCCG-3′ and 5′-AAACCGGATGTCCCGCCGTAGTTTC-3′ was inserted into the px330 vector (Addgene), which had been linearized with BbsI. The resulting plasmid was termed px330-SUN1. Genomic DNA fragments of the human SUN1 gene, which contained ∼500 bp upstream and downstream of the CRISPR/Cas9 targeting site, were amplified by PCR using appropriate primer sets. The DNA fragments were inserted to the pNT1.1 vector.Citation47 The resulting donor plasmid, termed pNT1.1-SUN1, harbors the neomycin resistant gene cassette in between the genomic DNA fragments. HeLa cells co-transfected with px330-SUN1 and pNT1.1-SUN1 were selected for G418-resistance. Two weeks later, 43 colonies were screened for biallelic gene disruption by PCR. SUN1 expression in positive clones was examined by RT-PCR, immunofluorescence and western blotting. Finally, one clone (clone #2), which was devoid of detecTable SUN1 expression, was used for further analysis.
Cell migration assay
A cell migration assay was performed as previously described.Citation48 Briefly, cells were assessed using a 48-well microchemotaxis chamber (Boyden chamber, Neuro Probe) and a polycarbonate filter with 8-µm pores (Neuro Probe). The lower side of the filter was precoated with 10 µg/ml collagen type I-C (Nitta Gelatin). Medium containing 10% FBS was placed into the bottom chamber. Cells were trypsinized, washed twice with and suspended in serum-free medium supplemented with 0.1% BSA. Fifty microliters of cell suspension containing 1.1 × 105 MDA-MB-231 cells was added to the upper well. Cells were incubated for 3 hours, fixed with 4% paraformaldehyde, and stained with hematoxylin. The filter was placed on a glass slide after the remaining cells on the upper side were scraped off with a cotton swab. The number of cells that had migrated to the lower side through the pores was counted at x 20 magnification in 3 independent fields. At least 3 experiments were performed. Results of cell migration show averages of the data.
Immunoprecipitation
HeLa cells were lysed in RIPA buffer (Thermo Scientific, #89900, and ) or cells were transfected with GFP-tagged SUN1_916, SUN1_888, SUN1_785, or GFP alone. Cells were lysed in ice-cold lysis buffer A (100 mM Tris pH 8.3, 500 mM NaCl, 0.5% Triton X100) for Nup358 precipitation or lysis buffer B containing PBS (−), 50 mM Tris-HCl pH 7.5, 150 mM NaCl, and 1% Triton X-100 for lamin A/C, lamin B or lamin B2 precipitation (Isokane et al., 2008). Cell lysates were rotated for 90 minutes at 4°C and centrifuged at 20,000 ×g for 1 hour. Then total cell lysates were incubated with Protein G Dynabeads (Invitrogen) and rabbit anti-GFP pAbs or rabbit anti-Flag pAbs (Sigma Aldrich, F7425) as control IgG for 4 hours at 4°C. Then, protein G Dynabeads were washed 4 times with lysis buffer A or lysis buffer B. The bound proteins were analyzed by western blotting.
Yeast two hybrid system
A yeast 2 hybrid screen using an N-terminal region of SUN1_888 corresponding to 1–391 aa was performed as a contract analysis by Life technologies Japan.
Abbreviations
aa | = | amino acid residues |
INM | = | inner nuclear membrane |
LINC complex | = | linker of nucleoskeleton and cytoskeleton complex |
mAb | = | monoclonal antibody |
NE | = | nuclear envelope |
ONM | = | outer nuclear membrane |
pAb | = | polyclonal antibody |
RT-PCR | = | reverse transcription polymerase chain reaction |
Disclosure of potential conflicts of interest
No potential conflicts of interest were disclosed.
KNCL_A_1260802_Supplementary_Figures.zip
Download Zip (408.6 KB)KNCL_A_1260802_Supplementary_Materials.zip
Download Zip (82.7 KB)Funding
This study was performed as part of a joint research program with Wako Pure Chemical Industries Ltd. This was supported by the Education and Research Grant Program of Ehime Prefectural University of Health Science (2016) to MH.
References
- Liu Q, Roux K, Rattner JB, Shanahan C, Burke B, Stahl PD, Hodzic D. Coupling of the nucleus and cytoplasm: role of the LINC complex. J Cell Biol 2006; 172:41-53; PMID:16380439; http://dx.doi.org/10.1083/jcb.200606020
- Chi YH, Haller K, Peloponese JM Jr, Jeang KT. Histone acetyltransferase hALP and nuclear membrane protein hsSUN1 function in de-condensation of mitotic chromosomes. J Biol Chem 2007; 282:27447-58; PMID:17631499; http://dx.doi.org/10.1074/jbc.M703098200
- Hodzic DM, Yeater DB, Bengtsson L, Otto H, Stahl PD. Sun2 is a novel mammalian inner nuclear membrane protein.J Biol Chem 2004; 279:25805-12; PMID:15082709; http://dx.doi.org/10.1074/jbc.M313157200
- Rajgor D, Shanahan CM. Nesprins: from the nuclear envelope and beyond. Expert Rev Mol Med 2013; 15:e5; PMID:23830188; http://dx.doi.org/10.1017/erm.2013.6
- Haque F, Lloyd DJ, Smallwood DT, Dent CL, Shanahan CM, Fry AM, Trembath RC, Shackleton S. SUN1 interacts with nuclear lamin A and cytoplasmic nesprins to provide a physical connection between the nuclear lamina and the cytoskeleton.Mol Cell Biol 2006; 26:3738-51; PMID:16648470; http://dx.doi.org/10.1128/MCB.26.10.3738-3751.2006
- Göb E, Schmitt J, Benavente R, Alsheimer M. Mammalian sperm head formation involves different polarization of two novel LINC complexes. PLoS One 2010; 5:e12072; PMID:20711465; http://dx.doi.org/10.1371/journal.pone.0012072
- Shao X, Tarnasky HA, Lee JP, Oko R, van der Hoorn FA. Spag4, a novel sperm protein, binds outer dense-fiber protein Odf1 and localizes to microtubules of manchette and axoneme. Dev Biol 1999; 211:109-23; PMID:10373309; http://dx.doi.org/10.1006/dbio.1999.9297
- Frohnert C, Schweizer S, Hoyer-Fender S. SPAG4L/SPAG4L-2 are testis-specific SUN domain proteins restricted to the apical nuclear envelope of round spermatids facing the acrosome.Mol Hum Reprod 2011; 17:207-18; PMID:21159740; http://dx.doi.org/10.1093/molehr/gaq099
- Jiang XZ, Yang MG, Huang LH, Li CQ, Xing XW SPAG4L, a novel nuclear envelope protein involved in the meiotic stage of spermatogenesis. DNA Cell Biol 2011; 30:875-82; PMID:21711156; http://dx.doi.org/10.1089/dna.2010.1161
- Bone CR, Starr DA. Nuclear migration events throughout development. J Cell Sci 2016; 129:1951-61; PMID:27182060; http://dx.doi.org/10.1242/jcs.179788
- Gundersen GG, Worman HJ. Nuclear positioning. Cell 2013; 152:1376-89; PMID:23498944; http://dx.doi.org/10.1016/j.cell.2013.02.031
- Wilhelmsen K, Ketema M, Truong H, Sonnenberg A. KASH-domain proteins in nuclear migration, anchorage and other processes. J Cell Sci 2006; 119(Pt 24):5021-9; PMID:17158909; http://dx.doi.org/10.1242/jcs.03295
- Zhang X, Lei K, Yuan X, Wu X, Zhuang Y, Xu T, Xu R, Han M. SUN1/2 and Syne/Nesprin-1/2 complexes connect centrosome to the nucleus during neurogenesis and neuronal migration in mice. Neuron 2009; 64:173-87; PMID:19874786; http://dx.doi.org/10.1016/j.neuron.2009.08.018
- Chang W, Antoku S, Östlund C, Worman HJ, Gundersen GG. Linker of nucleoskeleton and cytoskeleton (LINC) complex-mediated actin-dependent nuclear positioning orients centrosomes in migrating myoblasts. Nucleus 2015; 6:77-88; PMID:25587885; http://dx.doi.org/10.1080/19491034.2015.1004947
- Chambliss AB, Khatau SB, Erdenberger N, Robinson DK, Hodzic D, Longmore GD, Wirtz D. The LINC-anchored actin cap connects the extracellular milieu to the nucleus for ultrafast mechanotransduction. Sci Rep 2013; 3:1087; PMID:23336069; http://dx.doi.org/10.1038/srep01087
- Wang N, Tytell JD, Ingber DE. Mechanotransduction at a distance: mechanically coupling the extracellular matrix with the nucleus. Nat Rev Mol Cell Biol 2009; 10:75-82; PMID:19197334; http://dx.doi.org/10.1038/nrm2594
- Lei K, Zhu X, Xu R, Shao C, Xu T, Zhuang Y, Han M. Inner nuclear envelope proteins SUN1 and SUN2 play a prominent role in theDNA damage response.Curr Biol 2012; 22:1609-15; PMID:22863315; http://dx.doi.org/10.1016/j.cub.2012.06.043
- Lottersberger F, Karssemeijer RA, Dimitrova N, de Lange. T53BP1 and the LINC Complex Promote Microtubule-Dependent DSB Mobility and DNA Repair. Cell 2015; 163:880-93; PMID:26544937; http://dx.doi.org/10.1016/j.cell.2015.09.057
- Cain NE, Starr DA. SUN proteins and nuclear envelope spacing. Nucleus 2015; 6:2-7; PMID:25425085; http://dx.doi.org/10.4161/19491034.2014.990857
- Luxton GW, Gomes ER, Folker ES, Vintinner E, Gundersen GG. Linear arrays of nuclear envelope proteins harness retrograde actin flow for nuclear movement. Science 2010; 329:956-9; PMID:20724637; http://dx.doi.org/10.1126/science.1189072
- Chancellor TJ, Lee J, Thodeti CK, Lele T. Actomyosin tension exerted on the nucleus through nesprin-1 connections influences endothelial cell adhesion, migration, and cyclic strain-induced reorientation. Biophys J 2010; 99:115-23; PMID:20655839; http://dx.doi.org/10.1016/j.bpj.2010.04.011
- Maninová M, Klímová Z, Parsons JT, Weber MJ, Iwanicki MP, Vomastek T. The reorientation of cell nucleus promotes the establishment of front-rear polarity in migrating fibroblasts. J Mol Biol 2013; 425:2039-55; PMID:23524135; http://dx.doi.org/10.1016/j.jmb.2013.02.034
- King SJ, Nowak K, Suryavanshi N, Holt I, Shanahan CM, Ridley AJ. Nesprin-1 and nesprin-2 regulate endothelial cell shape and migration. Cytoskeleton 2014; 71:423-34; PMID:24931616; http://dx.doi.org/10.1002/cm.21182
- Hiraoka Y, Dernburg AF The SUN rises on meiotic chromosome dynamics. Dev Cell 2009; 17:598-605; PMID:19922865; http://dx.doi.org/10.1016/j.devcel.2009.10.014
- Burke B, Stewart CL. Functional architecture of the cell's nucleus in development, aging, and disease. Curr Top Dev Biol 2014; 109:1-52; PMID:24947235; http://dx.doi.org/10.1016/B978-0-12-397920-9.00006-8
- Meinke P, Nguyen TD, Wehnert MS. The LINC complex and human disease. Biochem Soc Trans 2011; 39:1693-7; http://dx.doi.org/10.1042/BST20110658
- Méndez-López I, Worman HJ. Inner nuclear membrane proteins: impact on human disease. Chromosoma 2012; 121:153-67; http://dx.doi.org/10.1007/s00412-012-0360-2
- Wang W, Shi Z, Jiao S, Chen C, Wang H, Liu G, Wang Q, Zhao Y, Greene MI, Zhou Z. Structural insights into SUN-KASH complexes across the nuclear envelope. Cell Res 2012; 22:1440-52; PMID:22945352; http://dx.doi.org/10.1038/cr.2012.126
- Sosa BA, Rothballer A, Kutay U, Schwartz TU. LINC Complexes Form by Binding of Three KASH Peptides to the Interfaces of Trimeric SUN proteins Cell 2012; 149:1035-1047.
- Patel JT, Bottrill A, Prosser SL, Jayaraman S, Straatman K, Fry AM, Shackleton S. Mitotic phosphorylation of SUN1 loosens its connection with the nuclear lamina while the LINC complex remains intact. Nucleus 2014; 5:462-73; PMID:25482198; http://dx.doi.org/10.4161/nucl.36232
- Woglar A, Daryabeigi A, Adamo A, Habacher C, Machacek T, La Volpe A, Jantsch V. Matefin/SUN-1 phosphorylation is part of a surveillance mechanism to coordinate chromosome synapsis and recombination with meiotic progression and chromosome movement. PLoS Genet 2013; 9:e1003335; PMID:23505384; http://dx.doi.org/10.1371/journal.pgen.1003335
- Ghosh S, Gardner JM, Smoyer CJ, Friederichs JM, Unruh JR, Slaughter BD, Alexander R, Chisholm RD, Lee KK, Workman JL, Jaspersen SL. Acetylation of the SUN protein Mps3 by Eco1 regulates its function in nuclear organization. Mol Biol Cell 2012; 23:2546-59; PMID:22593213; http://dx.doi.org/10.1091/mbc.E11-07-0600
- Liu Q, Pante N, Misteli T, Elsagga M, Crisp M, Hodzic D, Burke B, Roux KJFunctional association of Sun1 with nuclear pore complexes. J Cell Biol 2007; 178:785-98; PMID:17724119; http://dx.doi.org/10.1083/jcb.200704108
- Göb E, Meyer-Natus E, Benavente R, Alsheimer M. Expression of individual mammalian Sun1 isoforms depends on the cell type. Commun Integr Biol 2011; 4:440-2; PMID:21966565; http://dx.doi.org/10.4161/cib.15369
- Hasan S, Güttinger S, Mühlhäusser P, Anderegg F, Bürgler S, Kutay U. Nuclear envelope localization of human UNC84A does not require nuclear lamins. FEBS Lett 2006; 580:1263-8; PMID:16445915; http://dx.doi.org/10.1016/j.febslet.2006.01.039
- Lombardi ML, Jaalouk DE, Shanahan CM, Burke B, Roux KJ, Lammerding J. The interaction between nesprins and sun proteins at the nuclear envelope is critical for force transmission between the nucleus and cytoskeleton. J Biol Chem 2011; 286:26743-53; PMID:21652697; http://dx.doi.org/10.1074/jbc.M111.233700
- Lv XB, Liu L, Cheng C, Yu B, Xiong L, Hu K, Tang J, Zeng L, Sang Y. SUN2 exerts tumor suppressor functions by suppressing the Warburg effect in lung cancer. Sci Rep 2015; 5:17940; PMID:26658802; http://dx.doi.org/10.1038/srep17940
- Matsumoto A, Hieda M, Yokoyama Y, Nishioka Y, Yoshidome K, Tsujimoto M, Matsuura N. Global loss of a nuclear lamina component, lamin A/C, and LINC complex components SUN1, SUN2, and nesprin-2 in breast cancer. Cancer Med 2015; 4:1547-57; PMID:26175118; http://dx.doi.org/10.1002/cam4.495
- Meinke P, Mattioli E, Haque F, Antoku S, Columbaro M, Straatman KR, Worman HJ, Gundersen GG, Lattanzi G, Wehnert M, Shackleton S. Muscular dystrophy-associated SUN1 and SUN2 variants disrupt nuclear-cytoskeletal connections and myonuclear organization. PLoS Genet 2014; 10:e1004605; PMID:25210889; http://dx.doi.org/10.1371/journal.pgen.1004605
- Chen CY, Chi YH, Mutalif RA, Starost MF, Myers TG, Anderson SA, Stewart CL, Jeang KT. Accumulation of the inner nuclear envelope protein Sun1 is pathogenic in progeric and dystrophic laminopathies. Cell 2012; 149:565-77; PMID:22541428; http://dx.doi.org/10.1016/j.cell.2012.01.059
- Coffinier C, Chang SY, Nobumori C, Tu Y, Farber EA, Toth JI, Fong LG, Young SG. Abnormal development of the cerebral cortex and cerebellum in the setting of lamin B2 deficiency. Proc Natl Acad Sci USA 2010; 107:5076-81; PMID:20145110; http://dx.doi.org/10.1073/pnas.0908790107
- Coffinier C, Jung HJ, Nobumori C, Chang S, Tu Y, Barnes RH 2nd, Yoshinaga Y, de Jong PJ, Vergnes L, Reue K, Fong LG, Young SG. Deficiencies in lamin B1 and lamin B2 cause neurodevelopmental defects and distinct nuclear shape abnormalities in neurons. Mol Biol Cell 2011; 22:4683-93; PMID:21976703; http://dx.doi.org/10.1091/mbc.E11-06-0504
- Coffinier C, Fong LG, Young SG. LINCing lamin B2 to neuronal migration: growing evidence for cell-specific roles of B-type lamins. Nucleus 2010; 1:407-11; PMID:21278813; http://dx.doi.org/10.4161/nucl.1.5.12830
- Wang JY, Yu IS, Huang CC, Chen CY, Wang WP, Lin SW, Jeang KT, Chi YH. Sun1 deficiency leads to cerebellar ataxia in mice. Dis Model Mech 2015; 8:957-67; PMID:26035387; http://dx.doi.org/10.1242/dmm.019240
- Takamori Y, Tamura Y, Kataoka Y, Cui Y, Seo S, Kanazawa T, Kurokawa K, Yamada H. Differential expression of nuclear lamin, the major component of nuclear lamina, during neurogenesis in two germinal regions of adult rat brain. Eur J Neurosci 2007; 25:1653-62; PMID:17432957; http://dx.doi.org/10.1111/j.1460-9568.2007.05450.x
- Yokoyama Y, Hieda M, Nishioka Y, Matsumoto A, Higashi S, Kimura H, Yamamoto H, Mori M, Matsuura S, Matsuura N. Cancer associated up-regulation of H3K9 methylation promotes cell motility in vitro and drives tumor formation in vivo. Cancer Science 2013; 104:889-8; PMID:23557258; http://dx.doi.org/10.1111/cas.12166
- Tokuhiro K, Ikawa M, Benham AM, Okabe M. Protein disulfide isomerase homolog PDILT is required for quality control of sperm membrane protein ADAM3 and male fertility [corrected]. Proc Natl Acad Sci USA 2012; 109:3850-55; PMID:22357757; http://dx.doi.org/10.1073/pnas.1117963109
- Hieda M, Matsuura N, Kimura H. Histone modifications associated with cancer cell migration and invasion. Methods Mol Biol 2015; 1238:301-17; PMID:25421667; http://dx.doi.org/10.1007/978-1-4939-1804-1_16