ABSTRACT
In eukaryotic cells, the nuclear envelope (NE) is a membrane partition between the nucleus and the cytoplasm to compartmentalize nuclear contents. It plays an important role in facilitating nuclear functions including transcription, DNA replication and repair. In mammalian cells, the NE breaks down and then reforms during cell division, and in interphase it is restored shortly after the NE rupture induced by mechanical force. In this way, the partitioning effect is regulated through dynamic processes throughout the cell cycle. A failure in rebuilding the NE structure triggers the mixing of nuclear and cytoplasmic contents, leading to catastrophic consequences for the nuclear functions. Whereas the precise details of molecular mechanisms for NE reformation during cell division and NE restoration in interphase are still being investigated, here, we mostly focus on mammalian cells to describe key aspects that have been identified and to discuss the crosstalk between them.
Structure and organization of the NE
In eukaryotic cell nuclei, the genome is surrounded with the nuclear envelope (NE) that consists of the double phospholipid bilayers of the inner and outer nuclear membranes (INM, ONM) and NE-associated proteins (). In mammalian cells, the nuclear lamina (NL) underlines the nuclear side of the INM to maintain the nuclear shape, size and stiffness [Citation1–4]. The major structural determinants of the NL are type-V intermediate filaments proteins, the nuclear lamins. They are subdivided into A-types (lamins A (LA) and C (LC)) and B-types (lamins B1 (LB1) and B2 (LB2)). Lamins assembles into a filament with a diameter of ~3.5 nm, and these lamin filaments are nonrandomly distributed to form a fiber of meshworks in the NL with a thickness of ~14 nm [Citation5,Citation6]. The INM is inserted with a group of proteins with a transmembrane domain (TM) that associate with the NL such as lamina-associated polypeptide (LAP) 1, LAP2/Emerin/MAN1 (LEM)-domain proteins including LAP2β, emerin, MAN1, LEM2 and Ankle2, lamin B receptor (LBR) and Sad1p/UNC-84 (SUN) domain proteins SUN1/2. Another LEM-domain protein LAP2α does not have the TM but interacts with A-type lamins in the nucleoplasm [Citation7]. Barrier-to-autointegration factor (BAF) forms dimers and interact with chromatin [Citation8,Citation9]. BAF dimers also bind to A-type lamins and LEM-domain proteins through the immunoglobulin-like fold (Ig-fold) domain and the LEM domain, respectively [Citation9–11]. Lamins and INM proteins associate with heterochromatin at the nuclear periphery [Citation12]. The ONM structurally continues with the endoplasmic reticulum (ER) [Citation13] on the cytoplasmic side and is inserted with another group of proteins with the TM including nesprins [Citation14,Citation15]. Nesprins interacts with SUN1/2 dimers through the klarsicht/ANC-1/SYNE homology (KASH) domain inside the lumen between the INM and the ONM called the perinuclear space (PNS), forming the linker of nucleoskeleton and cytoskeleton (LINC) complex to connect the INM and the ONM with cytoskeletal systems including F-actin, microtubules and intermediate filaments [Citation16]. Nuclear pore complexes (NPCs) are embedded in annular junctions between the INM and the ONM to facilitate nucleo-cytoplasmic transport [Citation17] whereas bound to lamin filaments in the NL [Citation18,Citation19]. The overall structure is a cylindrical shape with a diameter of ~120 nm and contains more than 30 proteins known as nucleoporins (Nups) [Citation20–25]. Nups interact with euchromatin on the nuclear side of NPCs for gene activation [Citation26,Citation27].
Figure 1. Schematic depiction of the nuclear periphery including the nuclear envelope (NE), chromatin and the cytoskeleton. Nuclear membranes, separating between the nucleoplasm and the cytoplasm consist of the inner and outer nuclear membranes (INM, ONM) and are gapped with the perinuclear space (PNS). The ONM continues with the endoplasmic reticulum (ER) on the cytoplasmic side whereas the nuclear side of the INM is underlined with the nuclear lamina (NL), the major structural components of which are A- and B-type lamins. The INM is inserted with INM proteins including LAP1, LAP2β, emerin, MAN1, LEM2, Ankle2, LBR and SUN1/2. LAP2α interacts with A-type lamins in the nucleoplasm. BAF dimers bind to A-type lamins and LEM-domains. Lamins and INM proteins associate with heterochromatin at the nuclear periphery. SUN1/2 dimers interact with nesprins in the PNS to form the LINC complex, which mediate nuclear membranes and cytoskeletal systems. The nuclear pore complex (NPC) penetrates INM and the ONM, and the structural components nucleoporins (nups) interact with euchromatin.
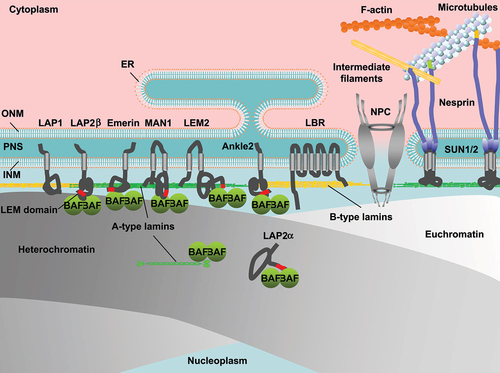
Mitotic NE disassembly
In mitosis, a mother cell divides into two daughter cells that usually have the same number of chromosomes as the original one. There are ‘closed’ and ‘open’ mitosis among different organisms [Citation28]. Closed mitosis occurs without NE breakdown (NEBD), and chromosomes remain inside the nucleus. In contrast, chromosomes become exposed to the cytoplasm in open mitosis (hereinafter called mitosis) as nuclear compartments including the NE disintegrates during NEBD. Then, these compartments are reformed on daughter chromosomes by the exit. In mammalian cells, as the cell enters prophase, the chromatin in the nucleus begins to condense into chromosomes. The NE starts disassembling at the onset of NEBD, subsequentially followed by the retraction of nuclear membranes into the ER [Citation29–31]. In prometaphase, NEBD is complete as the NL and NPCs disassemble, followed by the fragmentation of nuclear membranes. Then, microtubules attach to kinetochores and chromosomes congress to the metaphase plate. The initiation of NE disassembly is coupled to the phosphorylation of NE components including lamins [Citation32–35], BAF [Citation9,Citation36], INM and ONM proteins [Citation7,Citation37–40] and Nups [Citation41–44] by cyclin-dependent kinase 1 (CDK1), protein kinase C (PKC), vaccinia-related kinase 1 (VRK1), aurora kinases, polo-like kinase 1 (PLK1) and never in mitosis gene A (NIMA)-related kinases (NEK) [Citation45]. Before NEBD starts in a large scale, a CDK1-cyclin B1 complex is actively transported to the nucleus in a cyclin A2-dependent manner so that CDK1 can access to the phosphorylation sites of NE components inside the nucleus [Citation46–49]. Supporting this idea, the NE is found permeable to cytoplasmic macromolecules before NEBD as some of phosphorylated Nups are disassembled from NPCs to form holes in the NE [Citation50–52]. The NE might be also torn by the mechanical tension with the spindle microtubules and dynein motors as dynein/dynactin component p62 complex is translocated to the ONM just before NEBD [Citation53]. Elongating microtubules from spindle asters apparently push on the nuclear surface and eventually penetrate the NE [Citation54] and the holes of the NE expand until the NE becomes fragmented [Citation53,Citation55]. The disassociation of NE components from the NE differs in timing. For example, the hyperphosphorylation of Nup98 by CDK1 and NEK is the initial step of the NPC disassembly [Citation43]. Simultaneously or subsequently, CDK1 might also phosphorylate the Nup107–160 subcomplex, an important building block of the NPC, and embryonic large molecule derived from yolk sac (ELYS) for their disassociations from the NPC [Citation56,Citation57]. CDK1 and PKC concertedly phosphorylate the serines of lamins which are located just outside the N-terminal and C-terminal ends of the rod domain, resulting in the depolymerization of the head-tail polymers in the NL [Citation32,Citation33,Citation58–60]. Then, the depolymerized LA/C appears to start dissociating from the NL and diffuse into the nucleoplasm, followed by the fragmentation of the NL with the depolymerized LB1 and LB2 at the prophase/prometaphase transition [Citation54].
Mitotic NE reassembly
Whereas kinetochore microtubules get shortened to pull the sister chromatids of each mitotic chromosome apart during the metaphase-anaphase transition and telophase until the chromosomes reach the poles of the cell, the NE reforms around daughter chromosomes. For mitotic NE reassembly, phosphorylated forms of NE components are directed to the surface of the chromosomes through various pathways [Citation61,Citation62]. Processes of the NE reassembly are linked to the dephosphorylation of these NE components [Citation63]. At the entry of mitosis, protein phosphatase (PP) 1 and PP2A are inactivated through CDK1-mediated phosphorylation [Citation64–66]. As the CDK1 expression level declines in the late stages, reactivating PP1 and PP2A [Citation67,Citation68], PP1 could remove phosphates from phosphorylated NE components at sites of NE reformation [Citation69]. PP1 directly binds to an ER/NE membrane integrated protein A-kinase anchoring protein 149 (AKAP149) to remain anchored at the NE during G1 phase until it is released from AKAP149 upon S phase entry through the phosphorylation [Citation69–71]. PP1 is also recruited to the chromosome surface in anaphase by its binding to Repo-man [Citation72]. Repo-man also binds to Importin β to promote the recruitment, and the phosphorylation of Repo-man by the CDK1-cyclin B1 complex inhibit the binding [Citation73]. In this way, PP1 localization at the NE allows the temporal and spatial regulation of mitotic NE reassembly.
BAF accumulates at the central region of chromosomes close to kinetochores and telomeres during late anaphase and telophase, designated as the ‘core’ region (~5 min) immediately followed by LAP2α accumulation [Citation62,Citation74] (). Then, it sequentially recruits LA/C and LEM-domain containing INM proteins including LAP2β, Emerin, MAN1, LEM2 and Ankle2 to the core region [Citation62,Citation74,Citation75]. The BAF accumulation requires the DNA-binding ability [Citation8,Citation10] as BAF can bind to double-stranded (ds) DNA in a sequence-independent manner [Citation8,Citation76–78] and form a dimer or even an oligomer with dsDNA using two pairs of helix-hairpin-helix motifs located on opposite surfaces of the dimer without changing its conformation [Citation79]. The dephosphorylation of BAF might be also required for its accumulation (). To support this idea, VRK1 efficiently phosphorylates the N-terminal residues Ser-4 and/or Thr-2/Thr-3 of BAF inside the nucleus [Citation9,Citation36,Citation80] (). After the phosphorylated form is released from chromatin during NEBD [Citation81], it could bind to A-type lamins and LEM-domain proteins in the cytoplasm for the recruitment to the core region through the Ig-fold domain and the LEM domain, respectively () [Citation8,Citation10,Citation62,Citation74,Citation78,Citation83–89], and later it becomes non-phosphorylated at the NE [Citation75,Citation80,Citation90] (). In addition, Ankle2 targets PP2A to BAF and associates with VRK1 to inhibit its kinase activity, which enhances BAF dephosphorylation [Citation75]. Therefore, the kinase and phosphatase could be involved in regulating BAF accumulation at the core region. BAF and LA/C are mutually dependent on each other for the accumulation to the core region [Citation74,Citation91,Citation92], which is likely to be mediated by BAF binding to the Ig-fold domain and facilitated by the regulation of BAF phosphorylation with LA/C [Citation82,Citation92].
Figure 2. Representative single confocal section images and schematic depictions of the core region and the NE plaque. HeLa cells expressing sfGFP-DARPin-LA6 [Citation82] were fixed with 4% PFA, followed by permeabilization with 0.1% Triton X-100 and DNA staining with Hoechst 33342 at telophase (top) and after the induction of NE rupture by 405-nm laser-microirradiation (bottom). Arrow: core region. Arrowhead: NE plaque. Bar: 5 μm.
![Figure 2. Representative single confocal section images and schematic depictions of the core region and the NE plaque. HeLa cells expressing sfGFP-DARPin-LA6 [Citation82] were fixed with 4% PFA, followed by permeabilization with 0.1% Triton X-100 and DNA staining with Hoechst 33342 at telophase (top) and after the induction of NE rupture by 405-nm laser-microirradiation (bottom). Arrow: core region. Arrowhead: NE plaque. Bar: 5 μm.](/cms/asset/144e90d2-d1d2-40fb-843b-358c4b802cea/kncl_a_2352203_f0002_oc.jpg)
Figure 3. Sequential order of NE components in accumulation at or localization to the core region and the non-core region, and the NE plaque. The order and time (min) of the indicated proteins in mitotic NE reassembly (top) and repair of NE rupture (bottom).
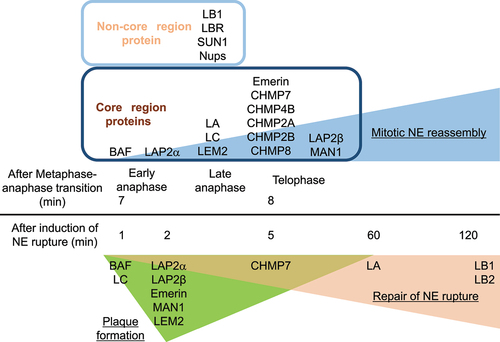
Figure 4. Schematic diagram of NE-component accumulation at or localization to the core region and the NE plaque. (Top) during mitosis, LA/C and LEM-domain proteins are recruited to the core region with BAF, followed by the accumulation of CHMPs to form a macromolecular O-ring. (Bottom) Phosphorylated BAF (p-BAF) recruits LC from the nucleoplasm to form the NE plaque on the surface of chromatin exposed to the cytoplasm at the opening area of a rupture site.
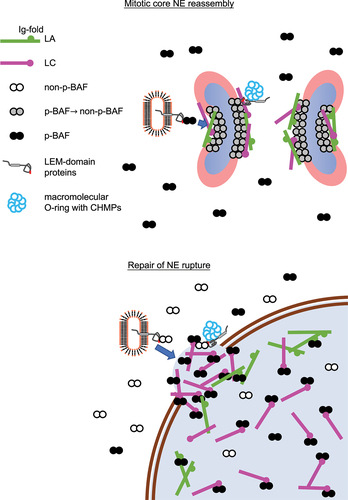
The endosomal sorting complex required for transport (ESCRT)-III/vacuolar protein sorting-associated protein 4 (VPS4) is another machinery for resealing nuclear membranes during mitosis [Citation93–96]. ESCRT-III forms a membrane-interacting oligomeric filament [Citation97] and the AAA+ ATPase VPS4 drives the dynamic exchanges of ESCRT-III complex subunits for assembling and disassembling ESCRT-III filaments [Citation98]. The LEM2 winged helix domain directly binds to an ESCRT-III subunit charged multivesicular body protein (CHMP) 7 [Citation99], inducing the formation of a LEM2-CHMP7 copolymer [Citation99]. At the sites where the membrane is intersected by microtubule bundles, LEM2 accumulates and undergoes liquid-phase separation, thereby triggering CHMP7 activation and ESCRT-III assembly. LEM2 recruits CHMP7 to the core region at telophase, followed by the sequential accumulation of other ESCRT-III complex subunits CHMP4B, CHMP2A and CHMP3 [Citation93–96]. Coiled-coil- and C2 domain-containing protein 1B (CC2D1B) also accumulates at the core region in a CHMP7-dependent manner but it slows the accumulation of CHMP7, CHMP4B and CHMP2A [Citation100]. CDK1 phosphorylates CHMP7 upon the entry of mitosis, which could prevent CHMP7 from interacting with LEM2 [Citation96], suggesting that local CHMP7 dephosphorylation at the core region might license the LEM2-CHMP7 interaction that triggers ESCRT-III recruitment.
The tips of ER tubules could attach to mitotic chromosomes [Citation101,Citation102], supplying nuclear membranes containing B-type lamins, LBR and SUN1/2 to the peripheral chromosomal regions excluded from the core region at early telophase (the ‘non-core’ region) [Citation62,Citation74,Citation93,Citation94,Citation103]. Nups are also localized to the non-core region, and this timely association of Nups could be regulated by a mechanism involving Importin β and RanGTP [Citation104–106]. One of the first stages in NPC reassembly is the recruitment to the chromosome surface of ELYS [Citation57,Citation107,Citation108]. ELYS interacts with the Nup107–160 complex and is essential for the recruitment of the Nup107–160 complex to chromatin [Citation56,Citation108,Citation109].
The core region becomes gradually indistinguishable from the non-core region by the end of mitosis (~10 min) as the chromosomes decondense, establishing two distinct nuclei [Citation10,Citation74]. Concomitantly, lamins are dephosphorylated to form polymers in the NL [Citation32,Citation33,Citation58,Citation59]. A fraction of phosphorylated LA/C is transported into daughter nuclei at late telophase and diffusible throughout the nucleoplasm once the NE surrounds daughter chromosomes [Citation61,Citation74]. With the progression of the nuclear transport, the non-phosphorylated forms could become incorporated into the NL [Citation110]. During the NE reformation, cytokinesis occurs through the construction of a contractile ring composed of actin and myosin filaments to separate into two daughter cells containing their own nuclei. When LA/C is expressed at high levels, the core region appears to remain in the area which are enriched in LA/C and devoid of NPCs during the following G1 phase of HeLa cells, designated as the ‘pore-free’ island [Citation111].
NE rupture
Mechanical stress can arise from external forces acting on the cell or from internal forces generated inside the cell itself. When these mechanical forces are excessive, the NE can locally burst with the immediate leakage of nuclear contents to the cytoplasm [Citation112,Citation113]. NE rupture occurs in pathological conditions including cancer cell migration [Citation114,Citation115]. Cancer cells often experience increased mechanical stress during migration, especially when passing through narrow spaces, such as blood vessels or tissue barriers. These mechanical forces can exert pressure on the nucleus and induce NE rupture [Citation116]. Cancer cells secrete various proteolytic enzymes including matrix metalloproteinases (MMPs), which can degrade components of the extracellular matrix (ECM) to promote tumor growth in the early stages of tumorigenesis [Citation117]. These enzymes can also facilitate cell migration leading to metastasis in the late stages [Citation118], which could protect from NE rupture [Citation114,Citation119,Citation120]. Internal forces generated by the cytoskeleton can exert mechanical stress on the NE through the LINC complex [Citation121]. Disruptions in connections or alterations in the cytoskeletal dynamics can transmit mechanical forces directly to the NE, potentially resulting in NE rupture [Citation112,Citation122,Citation123]. Numerous mutations that have been found throughout the A-type lamin gene cause a spectrum of human genetic disorders, correctively termed as the primary laminopathies [Citation124]. It includes cardiac disorders characterized by dilated cardiomyopathy (DCM), hypertrophic cardiomyopathy (HCM), muscular dystrophy (MD), familial partial lipodystrophy (FPLD) and progeroid syndromes including Hutchinson–Gilford progeria syndrome (HGPS) [Citation124]. Laminopathy mutations associated with DCM and MD frequently cause NE rupture in nuclear migration during skeletal muscle maturation [Citation125]. When NE integrity is weakened by some of laminopathy mutations and lamin depletion, the NE can spontaneously rupture without an external force applied to the cell [Citation126–132]. These phenotypes could be due to high curvature of the NE with locally diluted lamins in misshapen nuclei [Citation133–135]. NE rupture can expose nuclear DNA to the cytoplasmic environment, which can potentially lead to DNA damage mediated by the ER-associated exonuclease 1 (TREX1) and activate DNA repair mechanisms [Citation116]. DNA damage response proteins, such as ataxia telangiectasia mutated (ATM) and ataxia telangiectasia and Rad3-related (ATR), accumulates at the rupture sites to assess and repair any DNA damage that may have occurred [Citation115,Citation116,Citation136]. The active form of a DNA sensor, cyclic GMP-AMP synthase (cGAS) in the cytoplasm binds to nuclear DNA at the opening area of the rupture sites [Citation82,Citation114,Citation115,Citation137] whereas the inactive form in the nucleus probably does not because of the preferential binding to histone H2A/H2B of nucleosomes [Citation82,Citation138–140]. The cytoplasmic cGAS binding to nuclear DNA can trigger immune responses and potentially lead to genomic instability and cell death through the signaling pathway with the downstream effector, stimulator of interferon genes (STING) [Citation114,Citation115].
Repair of NE rupture
Upon the induction of NE rupture induced by mechanical stress, some of NE components are recruited to the rupture sites to help in sealing the holes or gaps and restoring its continuity [Citation82,Citation114,Citation115,Citation137,Citation141]. BAF recruits A-type lamins and LEM-domain proteins to the rupture sites through the interactions with the Ig-fold domain and the LEM-domain, respectively [Citation11,Citation82,Citation137,Citation141–143], and the sequential order of the recruitment is similar to that of the accumulation at the core region. First, BAF rapidly accumulates at the rupture sites, which could be mediated by its ability to bind to nuclear DNA exposed to the cytoplasm [Citation137]. It binds to exogenous dsDNA immediately after its appearance in the cytoplasm at endosome breakdown to form NE-like membranes to avoid autophagy [Citation144] and outcompetes with cytoplasmic cGAS for binding nuclear DNA at the rupture sites to suppress an immune response through the cGAS-STING pathway [Citation145]. However, BAF accumulation at the opening area of the rupture sites but not the DNA area might restrict the influx of cytoplasmic cGAS into the nucleus [Citation82]. In addition, BAF is involved in DNA repair processes that may be triggered by NE rupture. Its binding to poly (ADP-ribose) polymerase 1 (PARP1) negatively regulates the DNA damage response to oxidative stress [Citation146,Citation147]. BAF also bind to DNA-dependent protein kinase catalytic subunit (DNA-PKcs) and directly inhibit the activity to increase non-homologous end joining [Citation148]. BAF recruits LC from the nucleoplasm to the rupture sites and highly concentrate to form a relatively thick layer designated as the NE ‘plaque’ (within 1 min after the induction of NE rupture; ) [Citation82,Citation137]. Unlike the accumulation at the core region, LA is significantly slower (about 60 min) and weaker in localization to the NE plaque than LC because it is less abundant in the nucleoplasm than LC [Citation82]. As with the accumulation at the core region, LA/C could target BAF to the NE plaque in reverse through BAF binding to the Ig-fold domain and BAF phosphorylation [Citation74,Citation82,Citation82,Citation92,Citation141,Citation142]. Then, LEM-domain proteins including LAP2α and β, Emerin, MAN1, LEM2 and Ankle2 accumulate at the NE plaque within 5 min () [Citation137]. Because BAF phosphorylation suppresses its interaction with DNA but neither A-type lamins nor LEM-domain proteins [Citation9], the phosphorylated form could recruit A-type lamins and LAP2α from the nucleoplasm to the NE plaque whereas LEM-domain containing INM proteins are brought from the cytoplasm with the non-phosphorylated form [Citation8,Citation10,Citation137] (). LB1 and LB2 are localized in later stages compared to LA and LC () [Citation82], and NPCs are absent from the rupture sites. Because LB1 and several Nups in the central part of the NPC exhibit extremely low molecular turnovers and form immobile networks at the NE [Citation149,Citation150], the newly synthesized ones could slowly assemble at the rupture sites in interphase and/or their losses might not be completely recovered until they are recycled for NE reformation during mitosis.
As is the case with the recruitment to the core region, ESCRT-III and VPS4 are transiently recruited to the rupture site [Citation114,Citation115]. LEM2 recruits CHMP7 to the NE plaque [Citation137], which probably directs CHMP4B, CHMP2A and CHMP3 to the edge of the NE plaque in a sequential order [Citation114,Citation115]. This LEM2-CHMP7 interaction can mediate the sealing of small holes (<100 nm) as LEM2-CHMP7 copolymers are formed around the diameter of ~100 nm in vitro [Citation151], and ESCRTs are found in ~ 30–50 nm holes at the reforming NE [Citation94]. On the other hand, BAF is involved in repairing much larger gaps at the rupture sites [Citation82,Citation114,Citation115,Citation137]. Just like the disassembly and reassembly of the NE in mitosis, the phosphorylation and dephosphorylation of NE components by specific kinases and phosphatases might be involved in repair of NE rupture, but the mechanisms remain poorly understood. The phosphorylation of LA could increase its nucleoplasmic pool to facilitate its rapid accumulation to the NE plaque [Citation82,Citation142,Citation152,Citation153]. The structure of the NE plaque remains for a while, depending on the size, and then gradually smooths out to become less prominent [Citation82].
Structure and functions of the core region and the NE plaque
In mitotic disassembly and interphase rupture of the NE, the partition between the nucleus and the cytoplasm is temporarily lost but these phenomena are very distinct from each other. For example, the NE completely disappears after NEBD whereas holes or gaps are locally made at the rupture sites. NE disassembly is not accompanied by DNA damage during normal segregation of mitotic chromosomes, but NE rupture clearly does. The disassembly is programed as a part of the cell-division processes. In contrast, the rupture can be randomly triggered by mechanical stress at any given time. Despite these differences, molecular mechanisms for mitotic NE reassembly and repair of NE rupture have some key features in common.
The core region and the NE plaque transiently emerge as precursor structures that are formed on the surface of chromatin and chromosomes with BAF and its downstream targets including A-type lamins, LEM-domain proteins and CHMPs, respectively (). These structures appear to be stable because BAF is significantly immobile in the core region compared to that in the NE in interphase, forming a stable complex with LA and emerin [Citation74,Citation88], and LA is immobile in the NE plaque [Citation142]. LEM2, as recruited to the core region with BAF, condenses into a liquid-like phase and forms a macromolecular O-ring with CHMP7 to seal nuclear membranes [Citation99]. The O-ring structure could be also formed on the surface of the NE plaque to prevent the leakage of nuclear contents. Consequently, these dense structures might exclude B-type lamins and Nups from the core region and the NE plaque [Citation74,Citation82].
The thick layers of the core region and the NE plaque could temporally act as partition walls to prevent the unwanted interference of cytoplasmic components with chromatin (or mitotic chromosomes) until the resealing of nuclear membranes is complete [Citation82,Citation137,Citation141]. The core region with a thickness of 24–64 nm that overlaps with the localization of BAF is clearly observed on the surface of the chromosome mass [Citation74]. Even though the thickness of the NE plaque considerably depends on the size of holes or gaps, it could be in the range between ~76 nm and ~160 nm according to the recruitment of ESCRT-III complex subunits to the NE plaque [Citation114]. This stopgap could be very effective for maintaining the functions of mitotic chromosomes and chromatin in interphase without interruption. The accumulation of BAF and its targets hardens the chromosome surface at the core region to block the penetration of spindle fibers into the chromosomes and cross-bridges the chromosomes to limit access of nuclear membranes to the surface [Citation74,Citation154,Citation155]. These BAF-driven processes are critical for condensation and segregation of mitotic chromosomes and NE reassembly [Citation8,Citation76,Citation156]. Formation of the NE plaque with BAF and its downstream targets could minimize the mixing of contents between the nucleus and the cytoplasm at the rupture sites to restore nuclear functions quickly [Citation82,Citation137,Citation141].
Concluding remarks
Evidence is accumulating to indicate that mitotic NE reassembly and repair of NE rupture are active areas of research, and our current understanding of the process is still evolving. Further studies are needed to uncover additional details and identify any variations in the restoration mechanisms under physiological and pathological conditions. Chromatin decondensation in G1 phase and DNA replication during interphase are coupled to a significant increase in nuclear size. The growing NE during these phases could be supplemented with the recycled and newly synthesized components by the mechanisms which could be similar to those that facilitate the mitotic reassembly and the repair. Dysregulation of these functions could be linked to some of laminopathy mutations and the BAF A12T mutation causing Néstor–Guillermo progeria syndrome (NGPS) [Citation62,Citation82,Citation125,Citation126,Citation131,Citation142,Citation143,Citation147,Citation157].
Acknowledgments
We thank Dr. Watanya Trakarnphornsombat and Dr. Hiroshi Kimura at Tokyo Institute of Technology for supporting by microscopy.
Disclosure statement
No potential conflict of interest was reported by the author(s).
Data availability statement
The image data included in this article is available from the corresponding author, TS, upon reasonable request.
Additional information
Funding
References
- Lammerding J, Schulze PC, Takahashi T, et al. Lamin A/C deficiency causes defective nuclear mechanics and mechanotransduction. J Clin Invest. 2004;113(3):370–15. doi: 10.1172/JCI200419670
- Shimi T, Pfleghaar K, Kojima S, et al. The A- and B-type nuclear lamin networks: microdomains involved in chromatin organization and transcription. Genes Dev. 2008;22(24):3409–3421. doi: 10.1101/gad.1735208
- Levy DL, Heald R. Nuclear size Is regulated by importin α and Ntf2 in xenopus. Cell. 2010;143(2):288–298. doi: 10.1016/j.cell.2010.09.012
- Swift J, Ivanovska IL, Buxboim A, et al. Nuclear lamin-A scales with tissue stiffness and enhances matrix-directed differentiation. Science. 2013;341(6149):1240104. doi: 10.1126/science.1240104
- Shimi T, Kittisopikul M, Tran J, et al. Structural organization of nuclear lamins A, C, B1, and B2 revealed by superresolution microscopy. Mol Biol Cell. 2015;26(22):4075–4086. doi: 10.1091/mbc.E15-07-0461
- Turgay Y, Eibauer M, Goldman AE, et al. The molecular architecture of lamins in somatic cells. Nature. 2017;543(7644):261–264. doi: 10.1038/nature21382
- Foisner R, Gerace L. Integral membrane-proteins of the nuclear-envelope interact with lamins and chromosomes, and binding is modulated by mitotic phosphorylation. Cell. 1993;73(7):1267–1279. doi: 10.1016/0092-8674(93)90355-T
- Segura-Totten M, Kowalski AK, Craigie R, et al. Barrier-to-autointegration factor: major roles in chromatin decondensation and nuclear assembly. J Cell Bio. 2002;158(3):475–485. doi: 10.1083/jcb.200202019
- Marcelot A, Petitalot A, Ropars V, et al. Di-phosphorylated BAF shows altered structural dynamics and binding to DNA, but interacts with its nuclear envelope partners. Nucleic Acids Res. 2021;49(7):3841–3855. doi: 10.1093/nar/gkab184
- Haraguchi T, Koujin T, Segura-Totten M, et al. BAF is required for emerin assembly into the reforming nuclear envelope. J Cell Sci. 2001;114(24):4575–4585. doi: 10.1242/jcs.114.24.4575
- Samson C, Petitalot A, Celli F, et al. Structural analysis of the ternary complex between lamin A/C, BAF and emerin identifies an interface disrupted in autosomal recessive progeroid diseases. Nucleic Acids Res. 2018;46(19):10460–10473. doi: 10.1093/nar/gky736
- Zullo JM, Demarco IA, Piqué-Regi R, et al. DNA sequence-dependent compartmentalization and silencing of chromatin at the nuclear lamina. Cell. 2012;149(7):1474–1487. doi: 10.1016/j.cell.2012.04.035
- Voeltz GK, Rolls MM, Rapoport TA. Structural organization of the endoplasmic reticulum. EMBO Rep. 2002;3(10):944–950. doi: 10.1093/embo-reports/kvf202
- Zhen YY, Libotte T, Munck M, et al. NUANCE, a giant protein connecting the nucleus and actin cytoskeleton. J Cell Sci. 2002;115(15):3207–3222. doi: 10.1242/jcs.115.15.3207
- Libotte T, Zaim H, Abraham S, et al. Lamin A/C–dependent localization of nesprin-2, a giant scaffolder at the Nuclear Envelope. Mol Biol Cell. 2005;16(7):3411–3424. doi: 10.1091/mbc.e04-11-1009
- Crisp M, Liu Q, Roux K, et al. Coupling of the nucleus and cytoplasm: role of the LINC complex. J Cell Bio. 2006;172(1):41–53. doi: 10.1083/jcb.200509124
- Paine PL, Moore LC, Horowitz SB. Nuclear envelope permeability. Nature. 1975;254(5496):109–114. doi: 10.1038/254109a0
- Xie W, Chojnowski A, Boudier T, et al. A-type lamins form distinct filamentous networks with differential nuclear pore complex associations. Curr Biol. 2016;26(19):2651–2658. doi: 10.1016/j.cub.2016.07.049
- Kittisopikul M, Shimi T, Tatli M, et al. Computational analyses reveal spatial relationships between nuclear pore complexes and specific lamins. J Cell Bio. 2021;220(4):220. doi: 10.1083/jcb.202007082
- Cronshaw JM, Krutchinsky AN, Zhang W, et al. Proteomic analysis of the mammalian nuclear pore complex. J Cell Bio. 2002;158(5):915–927. doi: 10.1083/jcb.200206106
- Maimon T, Elad N, Dahan I, et al. The human nuclear pore complex as revealed by cryo-electron tomography. Structure. 2012;20(6):998–1006. doi: 10.1016/j.str.2012.03.025
- Bui KH, von Appen A, DiGuilio AL, et al. Integrated structural analysis of the human nuclear pore complex scaffold. Cell. 2013;155(6):1233–1243. doi: 10.1016/j.cell.2013.10.055
- von Appen A, Kosinski J, Sparks L, et al. In situ structural analysis of the human nuclear pore complex. Nature. 2015;526(7571):140–143. doi: 10.1038/nature15381
- Mahamid J, Pfeffer S, Schaffer M, et al. Visualizing the molecular sociology at the HeLa cell nuclear periphery. Science. 2016;351(6276):969–972. doi: 10.1126/science.aad8857
- Schuller AP, Wojtynek M, Mankus D, et al. The cellular environment shapes the nuclear pore complex architecture. Nature. 2021;598(7882):667–671. doi: 10.1038/s41586-021-03985-3
- Jacinto FV, Benner C, Hetzer MW. The nucleoporin Nup153 regulates embryonic stem cell pluripotency through gene silencing. Genes Dev. 2015;29(12):1224–1238. doi: 10.1101/gad.260919.115
- Ibarra A, Benner C, Tyagi S, et al. Nucleoporin-mediated regulation of cell identity genes. Genes Dev. 2016;30(20):2253–2258. doi: 10.1101/gad.287417.116
- Dey G, Baum B. Nuclear envelope remodelling during mitosis. Curr Opin Cell Biol. 2021;70:67–74. doi: 10.1016/j.ceb.2020.12.004
- Yang L, Guan T, Gerace L. Integral membrane proteins of the nuclear envelope are dispersed throughout the endoplasmic reticulum during mitosis. J Cell Bio. 1997;137(6):1199–1210. doi: 10.1083/jcb.137.6.1199
- Ellenberg J, Siggia ED, Moreira JE, et al. Nuclear membrane dynamics and reassembly in living cells: targeting of an inner nuclear membrane protein in interphase and mitosis. J Cell Bio. 1997;138(6):1193–1206. doi: 10.1083/jcb.138.6.1193
- Puhka M, Vihinen H, Joensuu M, et al. Endoplasmic reticulum remains continuous and undergoes sheet-to-tubule transformation during cell division in mammalian cells. J Cell Bio. 2007;179(5):895–909. doi: 10.1083/jcb.200705112
- Heald R, McKeon F. Mutations of phosphorylation sites in lamin a that prevent nuclear lamina disassembly in mitosis. Cell. 1990;61(4):579–589. doi: 10.1016/0092-8674(90)90470-Y
- Peter M, Nakagawa J, Dorée M, et al. In vitro disassembly of the nuclear lamina and M phase-specific phosphorylation of lamins by cdc2 kinase. Cell. 1990;61(4):591–602. doi: 10.1016/0092-8674(90)90471-P
- Hocevar BA, Burns DJ, Fields AP. Identification of protein kinase C (PKC) phosphorylation sites on human lamin B. Potential role of PKC in nuclear lamina structural dynamics. J Biol Chem. 1993;268(10):7545–7552. doi: 10.1016/S0021-9258(18)53210-5
- Goss VL, Hocevar BA, Thompson LJ, et al. Identification of nuclear beta II protein kinase C as a mitotic lamin kinase. J Biol Chem. 1994;269(29):19074–19080. doi: 10.1016/S0021-9258(17)32276-7
- Nichols RJ, Wiebe MS, Traktman P. The Vaccinia-related kinases phosphorylate the N' terminus of BAF, regulating its interaction with DNA and its retention in the nucleus. Mol Biol Cell. 2006;17(5):2451–2464. doi: 10.1091/mbc.e05-12-1179
- Pfaller R, Smythe C, Newport JW. Assembly/Disassembly of the nuclear envelope membrane: cell cycle-dependent binding of nuclear membrane vesicles to chromatin in vitro. Cell. 1991;65(2):209–217. doi: 10.1016/0092-8674(91)90155-R
- Courvalin JC, Segil N, Blobel G, et al. The lamin B receptor of the inner nuclear membrane undergoes mitosis-specific phosphorylation and is a substrate for p34cdc2-type protein kinase. J Biol Chem. 1992;267(27):19035–19038. doi: 10.1016/S0021-9258(18)41734-6
- Olsen JV, Blagoev B, Gnad F, et al. Global, in vivo, and site-specific phosphorylation dynamics in signaling networks. Cell. 2006;127(3):635–648. doi: 10.1016/j.cell.2006.09.026
- Dephoure N, Zhou C, Villén J, et al. A quantitative atlas of mitotic phosphorylation. Proc Natl Acad Sci USA. 2008;105(31):10762–10767. doi: 10.1073/pnas.0805139105
- Macaulay C, Meier E, Forbes DJ. Differential mitotic phosphorylation of proteins of the nuclear pore complex. J Biol Chem. 1995;270(1):254–262. doi: 10.1074/jbc.270.1.254
- Favreau C, Worman HJ, Wozniak RW, et al. Cell cycle-dependent phosphorylation of nucleoporins and nuclear pore membrane protein Gp210. Biochemistry. 1996;35(24):8035–8044. doi: 10.1021/bi9600660
- Laurell E, Beck K, Krupina K, et al. Phosphorylation of Nup98 by multiple kinases is crucial for NPC disassembly during mitotic entry. Cell. 2011;144(4):539–550. doi: 10.1016/j.cell.2011.01.012
- Linder MI, Köhler M, Boersema P, et al. Mitotic disassembly of nuclear pore complexes involves CDK1- and PLK1-mediated phosphorylation of key interconnecting nucleoporins. Dev Cell. 2017;43(2):141–56 e7. doi: 10.1016/j.devcel.2017.08.020
- Güttinger S, Laurell E, Kutay U. Orchestrating nuclear envelope disassembly and reassembly during mitosis. Nat Rev Mol Cell Biol. 2009;10(3):178–191. doi: 10.1038/nrm2641
- Nurse P. Universal control mechanism regulating onset of M-phase. Nature. 1990;344(6266):503–508. doi: 10.1038/344503a0
- Hagting A, Jackman M, Simpson K, et al. Translocation of cyclin B1 to the nucleus at prophase requires a phosphorylation-dependent nuclear import signal. Curr Biol. 1999;9(13):680–689. doi: 10.1016/S0960-9822(99)80308-X
- Toyoshima-Morimoto F, Taniguchi E, Shinya N, et al. Polo-like kinase 1 phosphorylates cyclin B1 and targets it to the nucleus during prophase. Nature. 2001;410(6825):215–220. doi: 10.1038/35065617
- Gong D, Pomerening JR, Myers JW, et al. Cyclin A2 regulates nuclear-envelope breakdown and the nuclear accumulation of cyclin B1. Curr Biol. 2007;17(1):85–91. doi: 10.1016/j.cub.2006.11.066
- Terasaki M, Campagnola P, Rolls MM, et al. A new model for nuclear envelope breakdown. Mol Biol Cell. 2001;12(2):503–510. doi: 10.1091/mbc.12.2.503
- Lénárt P, Rabut G, Daigle N, et al. Nuclear envelope breakdown in starfish oocytes proceeds by partial NPC disassembly followed by a rapidly spreading fenestration of nuclear membranes. J Cell Bio. 2003;160(7):1055–1068. doi: 10.1083/jcb.200211076
- Terasaki M, Okumura E, Hinkle B, et al. Localization and dynamics of Cdc2-cyclin B during meiotic reinitiation in starfish oocytes. Mol Biol Cell. 2003;14(11):4685–4694. doi: 10.1091/mbc.e03-04-0249
- Salina D, Bodoor K, Eckley DM, et al. Cytoplasmic dynein as a facilitator of nuclear envelope breakdown. Cell. 2002;108(1):97–107. doi: 10.1016/S0092-8674(01)00628-6
- Georgatos SD, Pyrpasopoulou A, Theodoropoulos PA. Nuclear envelope breakdown in mammalian cells involves stepwise lamina disassembly and microtubule-drive deformation of the nuclear membrane. J Cell Sci. 1997;110(Pt 17):2129–2140. doi: 10.1242/jcs.110.17.2129
- Beaudouin J, Gerlich D, Daigle N, et al. Nuclear envelope breakdown proceeds by microtubule-induced tearing of the lamina. Cell. 2002;108(1):83–96. doi: 10.1016/S0092-8674(01)00627-4
- Glavy JS, Krutchinsky AN, Cristea IM, et al. Cell-cycle-dependent phosphorylation of the nuclear pore Nup107–160 subcomplex. Proc Natl Acad Sci USA. 2007;104(10):3811–3816. doi: 10.1073/pnas.0700058104
- James C, Möller U, Spillner C, et al. Phosphorylation of ELYS promotes its interaction with VAPB at decondensing chromosomes during mitosis. EMBO Rep. 2024. doi: 10.1038/s44319-024-00125-6
- Gerace L, Blobel G. The nuclear envelope lamina is reversibly depolymerized during mitosis. Cell. 1980;19(1):277–287. doi: 10.1016/0092-8674(80)90409-2
- Peter M, Heitlinger E, Häner M, et al. Disassembly of in vitro formed lamin head-to-tail polymers by CDC2 kinase. Embo J. 1991;10(6):1535–1544. doi: 10.1002/j.1460-2075.1991.tb07673.x
- Mall M, Walter T, Gorjánácz M, et al. Mitotic lamin disassembly is triggered by lipid-mediated signaling. J Cell Bio. 2012;198(6):981–990. doi: 10.1083/jcb.201205103
- Moir RD, Yoon M, Khuon S, et al. Nuclear lamins a and B1: different pathways of assembly during nuclear envelope formation in living cells. J Cell Bio. 2000;151(6):1155–1168. doi: 10.1083/jcb.151.6.1155
- Dechat T, Gajewski A, Korbei B, et al. LAP2α and BAF transiently localize to telomeres and specific regions on chromatin during nuclear assembly. J Cell Sci. 2004;117(25):6117–6128. doi: 10.1242/jcs.01529
- Kutay U, Jühlen R, Antonin W. Mitotic disassembly and reassembly of nuclear pore complexes. Trends Cell Biol. 2021;31(12):1019–1033. doi: 10.1016/j.tcb.2021.06.011
- Dohadwala M, da Cruz e Silva EF, Hall FL, et al. Phosphorylation and inactivation of protein phosphatase 1 by cyclin-dependent kinases. Proc Natl Acad Sci USA. 1994;91(14):6408–6412. doi: 10.1073/pnas.91.14.6408
- Kwon YG, Lee SY, Choi Y, et al. Cell cycle-dependent phosphorylation of mammalian protein phosphatase 1 by cdc2 kinase. Proc Natl Acad Sci USA. 1997;94(6):2168–2173. doi: 10.1073/pnas.94.6.2168
- Grallert A, Boke E, Hagting A, et al. A PP1–PP2A phosphatase relay controls mitotic progression. Nature. 2015;517(7532):94–98. doi: 10.1038/nature14019
- Murray AW, Solomon MJ, Kirschner MW. The role of cyclin synthesis and degradation in the control of maturation promoting factor activity. Nature. 1989;339(6222):280–286. doi: 10.1038/339280a0
- Skoufias DA, Indorato RL, Lacroix F, et al. Mitosis persists in the absence of Cdk1 activity when proteolysis or protein phosphatase activity is suppressed. J Cell Bio. 2007;179(4):671–685. doi: 10.1083/jcb.200704117
- Thompson LJ, Bollen M, Fields AP. Identification of protein phosphatase 1 as a mitotic lamin phosphatase. J Biol Chem. 1997;272(47):29693–29697. doi: 10.1074/jbc.272.47.29693
- Steen RL, Martins SB, Taskén K, et al. Recruitment of protein phosphatase 1 to the nuclear envelope by A-kinase anchoring protein AKAP149 is a prerequisite for nuclear lamina assembly. J Cell Bio. 2000;150(6):1251–1262. doi: 10.1083/jcb.150.6.1251
- Steen RL, Beullens M, Landsverk HB, et al. AKAP149 is a novel PP1 specifier required to maintain nuclear envelope integrity in G1 phase. J Cell Sci. 2003;116(11):2237–2246. doi: 10.1242/jcs.00432
- Trinkle-Mulcahy L, Andersen J, Lam YW, et al. Repo-Man recruits PP1γ to chromatin and is essential for cell viability. J Cell Bio. 2006;172(5):679–692. doi: 10.1083/jcb.200508154
- Vagnarelli P, Ribeiro S, Sennels L, et al. Repo-Man coordinates chromosomal reorganization with nuclear envelope reassembly during mitotic exit. Dev Cell. 2011;21(2):328–342. doi: 10.1016/j.devcel.2011.06.020
- Haraguchi T, Kojidani T, Koujin T, et al. Live cell imaging and electron microscopy reveal dynamic processes of BAF-directed nuclear envelope assembly. J Cell Sci. 2008;121(15):2540–2554. doi: 10.1242/jcs.033597
- Asencio C, Davidson IF, Santarella-Mellwig R, et al. Coordination of kinase and phosphatase activities by Lem4 enables nuclear envelope reassembly during mitosis. Cell. 2012;150(1):122–135. doi: 10.1016/j.cell.2012.04.043
- Zheng R, Ghirlando R, Lee MS, et al. Barrier-to-autointegration factor (BAF) bridges DNA in a discrete, higher-order nucleoprotein complex. Proc Natl Acad Sci USA. 2000;97(16):8997–9002. doi: 10.1073/pnas.150240197
- Harris D, Engelman A. Both the structure and DNA binding function of the barrier-to-autointegration factor contribute to reconstitution of HIV type 1 integration in vitro. J Biol Chem. 2000;275(50):39671–39677. doi: 10.1074/jbc.M002626200
- Lee KK, Haraguchi T, Lee RS, et al. Distinct functional domains in emerin bind lamin a and DNA-bridging protein BAF. J Cell Sci. 2001;114(24):4567–4573. doi: 10.1242/jcs.114.24.4567
- Bradley CM, Ronning DR, Ghirlando R, et al. Structural basis for DNA bridging by barrier-to-autointegration factor. Nat Struct Mol Biol. 2005;12(10):935–936. doi: 10.1038/nsmb989
- Zhuang X, Semenova E, Maric D, et al. Dephosphorylation of barrier-to-autointegration factor by protein phosphatase 4 and its role in cell mitosis. J Biol Chem. 2014;289(2):1119–1127. doi: 10.1074/jbc.M113.492777
- Gorjánácz M, Klerkx EP, Galy V, et al. Caenorhabditis elegans BAF-1 and its kinase VRK-1 participate directly in post-mitotic nuclear envelope assembly. Embo J. 2007;26(1):132–143. doi: 10.1038/sj.emboj.7601470
- Kono Y, Adam SA, Sato Y, et al. Nucleoplasmic lamin C rapidly accumulates at sites of nuclear envelope rupture with BAF and cGAS. J Cell Bio. 2022;221(12):221. doi: 10.1083/jcb.202201024
- Furukawa K. LAP2 binding protein 1 (L2BP1/BAF) is a candidate mediator of LAP2-chromatin interaction. J Cell Sci. 1999;112(Pt 15):2485–2492. doi: 10.1242/jcs.112.15.2485
- Lin F, Blake DL, Callebaut I, et al. MAN1, an inner nuclear membrane protein that shares the LEM domain with lamina-associated polypeptide 2 and emerin. J Biol Chem. 2000;275(7):4840–4847. doi: 10.1074/jbc.275.7.4840
- Dechat T, Korbei B, Vaughan OA, et al. Lamina-associated polypeptide 2alpha binds intranuclear A-type lamins. J Cell Sci. 2000;113(Pt 19):3473–3484. doi: 10.1242/jcs.113.19.3473
- Shumaker DK, Lee KK, Tanhehco YC, et al. LAP2 binds to BAF·DNA complexes: requirement for the LEM domain and modulation by variable regions. Embo J. 2001;20(7):1754–1764. doi: 10.1093/emboj/20.7.1754
- Cai M, Huang Y, Ghirlando R, et al. Solution structure of the constant region of nuclear envelope protein LAP2 reveals two LEM-domain structures: one binds BAF and the other binds DNA. Embo J. 2001;20(16):4399–4407. doi: 10.1093/emboj/20.16.4399
- Shimi T, Koujin T, Segura-Totten M, et al. Dynamic interaction between BAF and emerin revealed by FRAP, FLIP, and FRET analyses in living HeLa cells. J Struct Biol. 2004;147(1):31–41. doi: 10.1016/j.jsb.2003.11.013
- Torras-Llort M, Medina-Giró S, Escudero-Ferruz P, et al. A fraction of barrier-to-autointegration factor (BAF) associates with centromeres and controls mitosis progression. Commun Biol. 2020;3(1):454. doi: 10.1038/s42003-020-01182-y
- Mehsen H, Boudreau V, Garrido D, et al. PP2A-B55 promotes nuclear envelope reformation after mitosis in Drosophila. J Cell Bio. 2018;217(12):4106–4123. doi: 10.1083/jcb.201804018
- Haraguchi T, Koujin T, Osakada H, et al. Nuclear localization of barrier-to-autointegration factor is correlated with progression of S phase in human cells. J Cell Sci. 2007;120(12):1967–1977. doi: 10.1242/jcs.03461
- Lin Q, Yu B, Wang X, et al. K6-linked SUMOylation of BAF regulates nuclear integrity and DNA replication in mammalian cells. Proc Natl Acad Sci USA. 2020;117(19):10378–10387. doi: 10.1073/pnas.1912984117
- Vietri M, Schink KO, Campsteijn C, et al. Spastin and ESCRT-III coordinate mitotic spindle disassembly and nuclear envelope sealing. Nature. 2015;522(7555):231–235. doi: 10.1038/nature14408
- Olmos Y, Hodgson L, Mantell J, et al. ESCRT-III controls nuclear envelope reformation. Nature. 2015;522(7555):236–239. doi: 10.1038/nature14503
- Gu M, LaJoie D, Chen OS, et al. LEM2 recruits CHMP7 for ESCRT-mediated nuclear envelope closure in fission yeast and human cells. Proc Natl Acad Sci USA. 2017;114(11):E2166–E75. doi: 10.1073/pnas.1613916114
- Gatta AT, Olmos Y, Stoten CL, et al. CDK1 controls CHMP7-dependent nuclear envelope reformation. Elife. 2021;10:10. doi: 10.7554/eLife.59999
- Schöneberg J, Pavlin MR, Yan S, et al. ATP-dependent force generation and membrane scission by ESCRT-III and Vps4. Science. 2018;362(6421):1423–1428. doi: 10.1126/science.aat1839
- Mierzwa BE, Chiaruttini N, Redondo-Morata L, et al. Dynamic subunit turnover in ESCRT-III assemblies is regulated by Vps4 to mediate membrane remodelling during cytokinesis. Nat Cell Biol. 2017;19(7):787–798. doi: 10.1038/ncb3559
- von Appen A, LaJoie D, Johnson IE, et al. LEM2 phase separation promotes ESCRT-mediated nuclear envelope reformation. Nature. 2020;582(7810):115–118. doi: 10.1038/s41586-020-2232-x
- Ventimiglia LN, Cuesta-Geijo MA, Martinelli N, et al. CC2D1B coordinates ESCRT-III activity during the mitotic reformation of the nuclear envelope. Dev Cell. 2018;47(5):547–563 e6. doi: 10.1016/j.devcel.2018.11.012
- Anderson DJ, Hetzer MW. Nuclear envelope formation by chromatin-mediated reorganization of the endoplasmic reticulum. Nat Cell Biol. 2007;9(10):1160–1166. doi: 10.1038/ncb1636
- Anderson DJ, Hetzer MW. Shaping the endoplasmic reticulum into the nuclear envelope. J Cell Sci. 2008;121(2):137–142. doi: 10.1242/jcs.005777
- Chi YH, Haller K, Peloponese JM Jr., et al. Histone acetyltransferase hALP and nuclear membrane protein hsSUN1 function in de-condensation of mitotic chromosomes. J Biol Chem. 2007;282(37):27447–27458. doi: 10.1074/jbc.M703098200
- Kutay U, Bischoff FR, Kostka S, et al. Export of importin α from the nucleus Is mediated by a specific nuclear transport factor. Cell. 1997;90(6):1061–1071. doi: 10.1016/S0092-8674(00)80372-4
- Walther TC, Askjaer P, Gentzel M, et al. RanGTP mediates nuclear pore complex assembly. Nature. 2003;424(6949):689–694. doi: 10.1038/nature01898
- Harel A, Chan RC, Lachish-Zalait A, et al. Importin β negatively regulates nuclear membrane fusion and nuclear pore complex assembly. Mol Biol Cell. 2003;14(11):4387–4396. doi: 10.1091/mbc.e03-05-0275
- Franz C, Walczak R, Yavuz S, et al. MEL-28/ELYS is required for the recruitment of nucleoporins to chromatin and postmitotic nuclear pore complex assembly. EMBO Rep. 2007;8(2):165–172. doi: 10.1038/sj.embor.7400889
- Rasala BA, Ramos C, Harel A, et al. Capture of AT-rich chromatin by ELYS recruits POM121 and NDC1 to initiate nuclear pore assembly. Mol Biol Cell. 2008;19(9):3982–3996. doi: 10.1091/mbc.e08-01-0012
- Rasala BA, Orjalo AV, Shen Z, et al. ELYS is a dual nucleoporin/kinetochore protein required for nuclear pore assembly and proper cell division. Proc Natl Acad Sci USA. 2006;103(47):17801–17806. doi: 10.1073/pnas.0608484103
- Yang L, Guan T, Gerace L. Lamin-binding fragment of LAP2 inhibits increase in nuclear volume during the cell cycle and progression into S phase. J Cell Bio. 1997;139(5):1077–1087. doi: 10.1083/jcb.139.5.1077
- Maeshima K, Yahata K, Sasaki Y, et al. Cell-cycle-dependent dynamics of nuclear pores: pore-free islands and lamins. J Cell Sci. 2006;119(21):4442–4451. doi: 10.1242/jcs.03207
- Hatch EM, Hetzer MW. Nuclear envelope rupture is induced by actin-based nucleus confinement. J Cell Bio. 2016;215(1):27–36. doi: 10.1083/jcb.201603053
- Zhang Q, Tamashunas AC, Agrawal A, et al. Local, transient tensile stress on the nuclear membrane causes membrane rupture. Mol Biol Cell. 2019;30(7):899–906. doi: 10.1091/mbc.E18-09-0604
- Denais CM, Gilbert RM, Isermann P, et al. Nuclear envelope rupture and repair during cancer cell migration. Science. 2016;352(6283):353–358. doi: 10.1126/science.aad7297
- Raab M, Gentili M, de Belly H, et al. ESCRT III repairs nuclear envelope ruptures during cell migration to limit DNA damage and cell death. Science. 2016;352(6283):359–362. doi: 10.1126/science.aad7611
- Nader GPF, Agüera-Gonzalez S, Routet F, et al. Compromised nuclear envelope integrity drives TREX1-dependent DNA damage and tumor cell invasion. Cell. 2021;184(20):5230–46 e22. doi: 10.1016/j.cell.2021.08.035
- Liotta LA, Tryggvason K, Garbisa S, et al. Metastatic potential correlates with enzymatic degradation of basement membrane collagen. Nature. 1980;284(5751):67–68. doi: 10.1038/284067a0
- Wolf K, Wu YI, Liu Y, et al. Multi-step pericellular proteolysis controls the transition from individual to collective cancer cell invasion. Nat Cell Biol. 2007;9(8):893–904. doi: 10.1038/ncb1616
- Wolf K, Te Lindert M, Krause M, et al. Physical limits of cell migration: control by ECM space and nuclear deformation and tuning by proteolysis and traction force. J Cell Bio. 2013;201(7):1069–1084. doi: 10.1083/jcb.201210152
- Infante E, Castagnino A, Ferrari R, et al. LINC complex-Lis1 interplay controls MT1-MMP matrix digest-on-demand response for confined tumor cell migration. Nat Commun. 2018;9(1):2443. doi: 10.1038/s41467-018-04865-7
- Kalukula Y, Stephens AD, Lammerding J, et al. Mechanics and functional consequences of nuclear deformations. Nat Rev Mol Cell Biol. 2022;23(9):583–602. doi: 10.1038/s41580-022-00480-z
- Patteson AE, Vahabikashi A, Pogoda K, et al. Vimentin protects cells against nuclear rupture and DNA damage during migration. J Cell Bio. 2019;218(12):4079–4092. doi: 10.1083/jcb.201902046
- Thiam HR, Wong SL, Qiu R, et al. Netosis proceeds by cytoskeleton and endomembrane disassembly and PAD4-mediated chromatin decondensation and nuclear envelope rupture. Proc Natl Acad Sci USA. 2020;117(13):7326–7337. doi: 10.1073/pnas.1909546117
- Worman HJ, Bonne G. “Laminopathies”: a wide spectrum of human diseases. Exp Cell Res. 2007;313(10):2121–2133. doi: 10.1016/j.yexcr.2007.03.028
- Earle AJ, Kirby TJ, Fedorchak GR, et al. Mutant lamins cause nuclear envelope rupture and DNA damage in skeletal muscle cells. Nat Mater. 2020;19(4):464–473. doi: 10.1038/s41563-019-0563-5
- De Vos WH, Houben F, Kamps M, et al. Repetitive disruptions of the nuclear envelope invoke temporary loss of cellular compartmentalization in laminopathies. Hum Mol Genet. 2011;20(21):4175–4186. doi: 10.1093/hmg/ddr344
- Vargas JD, Hatch EM, Anderson DJ, et al. Transient nuclear envelope rupturing during interphase in human cancer cells. Nucleus. 2012;3(1):88–100. doi: 10.4161/nucl.18954
- Robijns J, Molenberghs F, Sieprath T, et al. In silico synchronization reveals regulators of nuclear ruptures in lamin A/C deficient model cells. Sci Rep. 2016;6(1):30325. doi: 10.1038/srep30325
- Chen NY, Kim P, Weston TA, et al. Fibroblasts lacking nuclear lamins do not have nuclear blebs or protrusions but nevertheless have frequent nuclear membrane ruptures. Proc Natl Acad Sci USA. 2018;115(40):10100–10105. doi: 10.1073/pnas.1812622115
- Chen NY, Yang Y, Weston TA, et al. An absence of lamin B1 in migrating neurons causes nuclear membrane ruptures and cell death. Proc Natl Acad Sci USA. 2019;116(51):25870–25879. doi: 10.1073/pnas.1917225116
- Kim PH, Chen NY, Heizer PJ, et al. Nuclear membrane ruptures underlie the vascular pathology in a mouse model of Hutchinson-Gilford progeria syndrome. JCI Insight. 2021;6(16):6. doi: 10.1172/jci.insight.151515
- Vahabikashi A, Sivagurunathan S, Nicdao FAS, et al. Nuclear lamin isoforms differentially contribute to LINC complex-dependent nucleocytoskeletal coupling and whole-cell mechanics. Proc Natl Acad Sci USA. 2022;119(17):e2121816119. doi: 10.1073/pnas.2121816119
- Xia Y, Ivanovska IL, Zhu K, et al. Nuclear rupture at sites of high curvature compromises retention of DNA repair factors. J Cell Bio. 2018;217(11):3796–3808. doi: 10.1083/jcb.201711161
- Xia Y, Pfeifer CR, Zhu K, et al. Rescue of DNA damage after constricted migration reveals a mechano-regulated threshold for cell cycle. J Cell Bio. 2019;218(8):2545–2563. doi: 10.1083/jcb.201811100
- Ivanovska IL, Tobin MP, Bai T, et al. Small lipid droplets are rigid enough to indent a nucleus, dilute the lamina, and cause rupture. J Cell Bio. 2023;222(8):222. doi: 10.1083/jcb.202208123
- Kidiyoor GR, Li Q, Bastianello G, et al. ATR is essential for preservation of cell mechanics and nuclear integrity during interstitial migration. Nat Commun. 2020;11(1):4828. doi: 10.1038/s41467-020-18580-9
- Halfmann CT, Sears RM, Katiyar A, et al. Repair of nuclear ruptures requires barrier-to-autointegration factor. J Cell Bio. 2019;218(7):2136–2149. doi: 10.1083/jcb.201901116
- Pathare GR, Decout A, Glück S, et al. Structural mechanism of cGAS inhibition by the nucleosome. Nature. 2020;587(7835):668–672. doi: 10.1038/s41586-020-2750-6
- Zhao B, Xu P, Rowlett CM, et al. The molecular basis of tight nuclear tethering and inactivation of cGAS. Nature. 2020;587(7835):673–677. doi: 10.1038/s41586-020-2749-z
- Kujirai T, Zierhut C, Takizawa Y, et al. Structural basis for the inhibition of cGAS by nucleosomes. Science. 2020;370(6515):455–458. doi: 10.1126/science.abd0237
- Young AM, Gunn AL, Hatch EM, et al. BAF facilitates interphase nuclear membrane repair through recruitment of nuclear transmembrane proteins. Mol Biol Cell. 2020;31(15):1551–1560. doi: 10.1091/mbc.E20-01-0009
- Sears RM, Roux KJ. Mechanisms of A-type lamin targeting to nuclear ruptures are disrupted in LMNA- and BANF1-associated progerias. Cells. 2022:11(5): 865. doi: 10.3390/cells11050865
- Janssen A, Marcelot A, Breusegem S, et al. The BAF A12T mutation disrupts lamin A/C interaction, impairing robust repair of nuclear envelope ruptures in nestor–Guillermo progeria syndrome cells. Nucleic Acids Res. 2022;50(16):9260–9278. doi: 10.1093/nar/gkac726
- Kobayashi S, Koujin T, Kojidani T, et al. BAF is a cytosolic DNA sensor that leads to exogenous DNA avoiding autophagy. Proc Natl Acad Sci USA. 2015;112(22):7027–7032. doi: 10.1073/pnas.1501235112
- Guey B, Wischnewski M, Decout A, et al. BAF restricts cGAS on nuclear DNA to prevent innate immune activation. Science. 2020;369(6505):823–828. doi: 10.1126/science.aaw6421
- Montes de Oca R, Shoemaker CJ, Gucek M, et al. Barrier-to-autointegration factor proteome reveals chromatin-regulatory partners. PLoS One. 2009;4(9):e7050. doi: 10.1371/journal.pone.0007050
- Bolderson E, Burgess JT, Li J, et al. Barrier-to-autointegration factor 1 (Banf1) regulates poly [ADP-ribose] polymerase 1 (PARP1) activity following oxidative DNA damage. Nat Commun. 2019;10(1):5501. doi: 10.1038/s41467-019-13167-5
- Burgess JT, Cheong CM, Suraweera A, et al. Barrier-to-autointegration-factor (Banf1) modulates DNA double-strand break repair pathway choice via regulation of DNA-dependent kinase (DNA-PK) activity. Nucleic Acids Res. 2021;49(6):3294–3307. doi: 10.1093/nar/gkab110
- Daigle N, Beaudouin J, Hartnell L, et al. Nuclear pore complexes form immobile networks and have a very low turnover in live mammalian cells. J Cell Bio. 2001;154(1):71–84. doi: 10.1083/jcb.200101089
- Rabut G, Doye V, Ellenberg J. Mapping the dynamic organization of the nuclear pore complex inside single living cells. Nat Cell Biol. 2004;6(11):1114–1121. doi: 10.1038/ncb1184
- Vietri M, Schultz SW, Bellanger A, et al. Unrestrained ESCRT-III drives micronuclear catastrophe and chromosome fragmentation. Nat Cell Biol. 2020;22(7):856–867. doi: 10.1038/s41556-020-0537-5
- Kochin V, Shimi T, Torvaldson E, et al. Interphase phosphorylation of lamin A. J Cell Sci. 2014;127:2683–2696. doi: 10.1242/jcs.141820
- Cho S, Abbas A, Irianto J, et al. Progerin phosphorylation in interphase is lower and less mechanosensitive than lamin-A,C in iPS-derived mesenchymal stem cells. Nucleus. 2018;9(1):230–245. doi: 10.1080/19491034.2018.1460185
- Samwer M, Schneider MWG, Hoefler R, et al. DNA cross-bridging shapes a single nucleus from a set of mitotic chromosomes. Cell. 2017;170(5):956–972 e23. doi: 10.1016/j.cell.2017.07.038
- Schneider MWG, Gibson BA, Otsuka S, et al. A mitotic chromatin phase transition prevents perforation by microtubules. Nature. 2022;609(7925):183–190. doi: 10.1038/s41586-022-05027-y
- Margalit A, Vlcek S, Gruenbaum Y, et al. Breaking and making of the nuclear envelope. J Cell Biochem. 2005;95(3):454–465. doi: 10.1002/jcb.20433
- Dechat T, Shimi T, Adam SA, et al. Alterations in mitosis and cell cycle progression caused by a mutant lamin a known to accelerate human aging. Proc Natl Acad Sci USA. 2007;104(12):4955–4960. doi: 10.1073/pnas.0700854104