Abstract
It has been suggested that chromatin is organized into the stable structures that provide fundamental units of chromosome architecture in interphase mammalian cells. The stable structures of chromatin can be visualized as replication foci when replicating DNA is labeled with thymidine analogs. Previously, we showed that the chromosome territory expanded after BAF53 knockdown. In this study, we found that BAF53 is required for the formation of replication foci. DNA replication was not impaired in BAF53 knockdown cells, suggesting that the decrease in the number of replication foci is due to disintegration of replication foci, but not suppression of DNA replication. The attractive forces that maintain structural integrity of replication foci could be disrupted by BAF53 knockdown, and it may be responsible, at least in part, for the expansion of chromosome territories after BAF53 knockdown.
Introduction
In eukaryotic cells, replicating DNA is observed as replication foci when pulse-labeled with thymidine analogs such as BrdU and CldU (Nakamura et al. Citation1986; Jackson and Pombo Citation1998; Ma et al. Citation1998). Each replication focus represents a cluster of replicons which are synchronously activated during S phase. The replication of DNA in a replication focus is completed within 1 h. Replication foci range between 300 nm and 500 nm in size and contain 3–5 replicons within ~1 Mbp of DNA.
A study in which Chinese hamster ovary cells were incubated in [3H]thymidine for 30 min before fiber autoradiography demonstrated that adjacently located replicons fire DNA replication at approximately the same time (Huberman and Tsai Citation1973). Grouping of replicons located adjacently in a chromosome and quasi-synchronous firing of the replicons in each group have been further demonstrated on extended DNA fibers pulse-labeled with thymidine analogs (Jackson and Pombo Citation1998). These results suggest that replicons adjacent to each other on the same chromosome build a stable structure and synchronous initiation of replicons within a given structure results in the formation of replication foci. When replication foci are labeled with thymidine analogs and chased thereafter, replication foci persist for many generations with similar brightness and size. In addition, the size of replication foci remains constant independently of pulse-labeling time (Ma et al. Citation1998; Zink et al. Citation1998). Accordingly, replication foci are considered as persistent higher-order structural units.
Two predominant features of mammalian interphase chromosomes have been consistently observed. First, individual chromosomes occupy their own discrete territories in the interphase nucleus called chromosome territories (Cremer and Cremer Citation2001). Second, chromosomes are made up of chromosomal subdomains that have been visualized as bead images with diameters of 300 to 800 nm in fluorescence in situ hybridization (FISH) studies (Muller et al. Citation2004). Each chromosomal subdomain contains ~1 Mbp of DNA on average, which is similar to the size of replication foci. It is generally accepted that chromosomal subdomains correspond to replication foci. Therefore, chromosomal subdomains and replication foci represent the same stable and fundamental unit of chromosome structure.
Chromatin folding within chromosomal subdomains still remains elusive. DNA loopings, chromonema fiber folding and self-assembling as ‘fractal globules’ have been proposed to explain the formation of chromosomal subdomains (Belmont and Bruce Citation1994; Münkel et al. Citation1999; Lieberman-Aiden et al. Citation2009). It is noteworthy that most of the models postulate attractive interactions within the chromatin fiber as a key element to drive the formation of chromosomal subdomains. Since attractive interactions limit free diffusion of chromatin fiber, it is highly likely that the attractive interaction which drives the formation of chromosomal subdomains plays a role, at least in part, in keeping each chromosome within its territory. As a matter of fact, chromatin folding into rosette-like structures with fixed loop attachment points is sufficient for chromatin fibers to repel each other due to entropic forces (Cook and Marenduzzo Citation2009).
The attractive interactions could be provided by DNA-binding condensing/linking proteins like cohesin (Nasmyth and Haering Citation2009), CTCF (Phillips and Corces Citation2009), or structural RNAs (Ng et al. Citation2007). However, none of the proteins or structural RNAs has been proven to be essential for chromosomal subdomains and chromosome territories. BAF53 is an actin-related protein (Arp) found in mammalian cell nuclei (Zhao et al. Citation1998; Sung et al. Citation2001). Previously we showed that BAF53 is required for the maintenance of chromosome territories (Lee et al. Citation2007). When the expression of BAF53 was suppressed by siRNA interference, chromosome territories were expanded by 2 to 2.4 fold. One likely cause of the expansion of chromosome territories is a loss of the attractive interactions within the chromatin fiber. Since these interactions that keep the chromosome territories are likely to drive the formation of chromosomal subdomains as well, these findings raise a possibility that suppression of BAF53 leads to disintegration of chromosomal subdomains. In order to address this question, we here examined whether replicating DNA labeled with thymidine analogs is able to aggregate into replication foci after the expression of BAF53 is suppressed by siRNA interference.
Materials and methods
Synchronization and siRNA transfection
NIH3T3 cells were cultured in Dulbecco's Modified Eagles Medium (DMEM) containing 10% calf serum with 100 U/ml penicillin and 100 µg/ml streptomysin at 37°C in a CO2 incubator. To synchronize the cell cycle at the G1/S border, NIH3T3 cells were incubated in complete medium with 5 mM thymidine for 12 h, washed and incubated in complete medium for 9 h, and then incubated in 5 mM thymidine again for 14 h. The cells were transfected with siRNA duplexes using siPORTamine as described (Lee et al. Citation2007).
Flow cytometry analysis
NIH3T3 cells were harvested by trypsinization and then stained with propidium iodide (PI) solution (50 µg/ml PI, 0.1% sodium citrate, 0.3% NP-40, 50 µg/ml RNase A) at 37°C for 15 min. The cell cycle profile was analyzed on FACSCalibur (BD).
Immunoblotting analysis
Cells were lysed in 1×SDS sample buffer (62.5 mM Tris-Cl, pH 6.8, 10% glycerol, 2% SDS, 1% β-mercaptoethanol, 0.01% bromphenol blue). The protein concentrations were determined by a BCA kit (PIERCE) and the lysates were subjected to 10% SDS polyacrylamide gel electrophoresis. Immunoblotting analysis was performed as described (Lee et al. Citation2011).
Immunostaining
Cells were labeled with 20 µM BrdU, IdU, or CldU for 20 min and then fixed with 3.7% paraformaldehyde in 1×PBS at 4°C for 15 min. The cells were incubated in 1.5 N HCl for 30 min in order to denature DNA. After being washed with distilled water, the cells were neutralized with 0.1 M sodium borate for 5 min at room temperature. The cells were permeabilized with 0.5% PBS-T for 5 min, washed with 0.1% PBS-T briefly, and then incubated with blocking solution (10% heat-inactivated calf serum and 0.5% gelatin in TBS) for 30 min. After a quick washing with 0.1% PBS-T, the cells were incubated for 1 h at room temperature with mouse BrdU antibody (BD, #555627), rat BrdU antibody (Oxford Biotechnology, OBT0030G), or anti-PCNA antibody (Santa Cruz, #sc-7907). They were then detected with FITC-anti-mouse or FITC-anti-rat IgG.
Results
Formation of early S-phase replication foci is defective in cells with BAF53 knockdown
The formation of replication foci follows its specific spatiotemporal order. Time course analysis of incorporated BrdU revealed that formation of replication foci in NIH3T3 cells follows faithfully the specific spatiotemporal order which was previously demonstrated in CHO cells (A) (O'Keefe et al. Citation1992). During early S phase (up to 2 h into S phase), replication foci were distributed at sites throughout the nucleoplasm, excluding the nucleolus and the periphery of the nucleus. As S phase progressed (mid S phase, 4–6 h), replication foci at sites near perinucleolar and peripheral regions became predominant and replication at the nucleoplasm decreased. In late S phase (7 h), replication occurred at a few large regions which were presumably interior regions of heterochromatin.
Figure 1. Defect in the formation of early S-phase replication foci induced by BAF53 knockdown. (A) Temporal pattern of replication foci in NIH3T3 cells. The cell cycle of NIH3T3 cells was arrested at the G1/S border by double thymidine treatment. After release from the G1/S border, BrdU was added for 20 min at the indicated time after the release. Incorporated BrdU was visualized by immnustaining with an anti-BrdU antibody. (B) Suppression of BAF53 by siRNA interference. NIH3T3 cells were transfected with siRNA-BAF53. BAF53 and β-tubulin were detected by immunoblotting analysis. (C) Confocal midsections of a nucleus of NIH3T3 cell immunostained with anti-BrdU antibody or anti-PCNA antibody. After transfection with siRNA-BAF53, NIH3T3 cells were arrested at the G1/S border by double thymidine treatment. At 1 h after release from G1/S arrest, the cells were pulse-labeled with BrdU for 20 mins and then fixed. Note that an aberrant pattern of replication foci in addition to the typical early S-phase replication foci is observed in BAF53-knockdown cells. The aberrant pattern is characterized by diffused signals from nucleoplasm with discrete foci at nuclear periphery and perinucleolar regions. (D, E) Distributions of the temporal patterns of replication foci in the control and the BAF53-knockdown cells immunostained with anti-BrdU antibody (D) or anti-PCNA antibody (E).
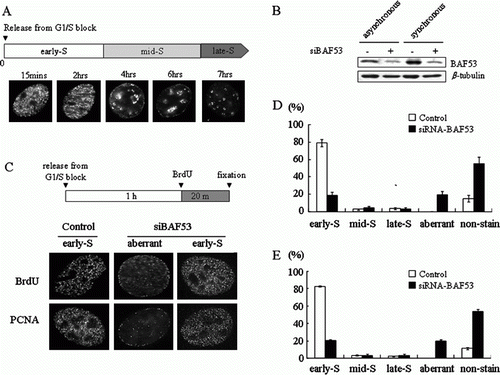
In order to examine whether BAF53 is required for the formation of replication foci, we first suppressed the expression of BAF53 by siRNA transfection (B), and then arrested cell cycle progression at the G1/S border by double thymidine treatment and released it into S phase in the presence of BrdU. At 1 h into S phase, most of the control cells showed the typical early S-phase pattern demonstrated by immunostaining with anti-BrdU antibody (C). On the other hand, an aberrant pattern, which was not observed in the control cells throughout the S phase, appeared in the BAF53-knockdown cells. In this aberrant pattern, replication foci near the nuclear periphery and a few regions inside the nucleoplasm were evident with a diffused staining throughout the nucleoplasm. The aberrant pattern is somewhat similar to but clearly distinct from the mid S-phase pattern in that it lacks clear perinucleolar staining and it has a diffused staining throughout the nucleoplasm. The aberrant pattern (17.7%) was found as frequently as the early S-phase pattern (17.4%) in BAF53-knockdown cells (D). We also observed an increase of non-stained cells from 14% to 57.9% by BAF53 knockdown, which is consistent with the previous observation that BAF53 knockdown caused cell cycle arrest at G1 in NIH3T3 cells (Lee et al. Citation2007). We got virtually identical results by immunostaining with anti-PCNA antibody (C,E).
Since the aberrant pattern is somewhat similar to the mid S-phase pattern and cell cycle synchronization cannot be perfect, the aberrant pattern may result simply from an increase of cell population arrested at mid S phase but not at the G1/S border. In fact, a small number of cells (2.7%) exhibited the mid S-phase pattern even in the control cells (D,E). We next examined whether the aberrant pattern found in the BAF53-knockdown cells is exhibited by cells arrested at mid S phase. We took advantage of the fact that replication normally take place before the second thymidine treatment or just after the release from thymidine block in cells arrested at mid S phase. Cells were pulse-labeled with IdU for 1 h just before the second thymidine treatment and then pulse-labeled with CldU at 1 h into S phase (A). In the control cells, the cells stained with CldU by the mid S-phase pattern were labeled with IdU. On the other hand, the cells stained with CldU as the aberrant pattern found in the BAF53-knockdown cells were not stained with IdU. When cells were incubated with IdU for 30 min just after the release from the second thymidine block and then pulse-labeled with CldU at 1 h into S phase, most of the control cells stained with CldU, regardless of whether they exhibited the early S-phase or the mid S-phase pattern, were labeled with IdU (B). In contrast, the cells stained with CldU as the aberrant pattern in the BAF53-knockdown cells were not stained with IdU.
Figure 2. The aberrant pattern of replication foci occurs at early S phase. (A) NIH3T3 cells were pulse-labeled with IdU for 1 h just before the second thymidine block, and then the cells were pulse-labeled with CldU for 20 min at 1 h after release from G1/S arrest. (B) NIH3T3 cells were pulse-labeled with IdU for 30 min just after termination of the second thymidine block and subjected to a washing. The cells were incubated for 30 min and then pulse-labeled with CldU for 20 min. (C) NIH3T3 cells were pulse-labeled with IdU for 20 min at 1 h after release from G1/S arrest and subjected to a washing. The cells were further incubated for 3 h and then pulse-labeled with CldU for 20 min. Summary of numbers of the cells showing each pattern of S-phase progress.
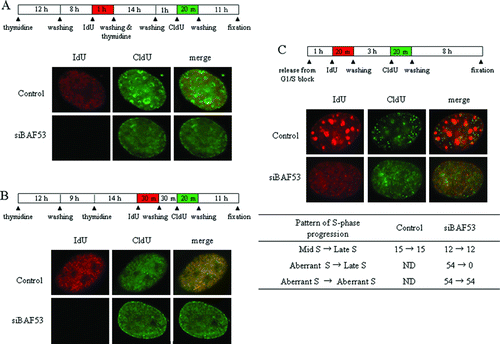
Cells arrested at mid S phase should progress into late S phase within 3 h after the release. We next examined whether the BAF53-knockdown cells stained as the aberrant pattern progress into late S-phase pattern. At 1 h into S phase, cells were pulse-labeled with IdU for 20 min, the IdU was washed away, and then after 3 h the cells were labeled with CldU for 20 min (C). In both control and BAF53-knockdown cells, cells stained with IdU as the mid S-phase pattern were labeled with CldU as the late S-phase pattern. In contrast, all of the cells stained with IdU as the aberrant pattern were labeled by the aberrant pattern, but not by the late S-phase pattern with CldU. Taken together we conclude that the aberrant pattern found in the BAF53-knockdown cells is a bona fide new pattern, taking place at early S phase, and the disintegration of early S-phase replication foci could account for this aberrant pattern.
BAF53 knockdown cells labeled with BrdU complete mitosis and then become arrested at G1 of the next cell cycle
It has been previously observed that BAF53 knockdown leads to cell cycle arrest at G1/S in NIH3T3 cells. FACS analysis showed that BrdU-labeled knockdown cells were able to complete mitosis and then cell cycle progression was halted with 2n DNA contents (Lee et al. Citation2007). When BAF53-knockdown cells were released into S phase in the presence of nocodazole, we did not observe any increase of S-phase cells compared to control cells (A). These results suggest that the cells with the aberrant replication complete DNA replication. We next addressed whether the cells showing the aberrant pattern of replication foci are able to undergo the next round of replication. At 1 h into S phase, cells were pulse-labeled with IdU for 20 min and washed out. After 24 h, cells were incubated with CldU for 5 h. All of the IdU-labeled cells were stained with CldU in the control group (B). In the BAF53-knockdown group, on the other hand, none of cells labeled with IdU as the aberrant pattern was stained with CldU, while all of cells labeled with IdU as the early S-phase pattern were stained with CldU.
Figure 3. Cell cycle arrest of BAF53-knockdown cells after the aberrant replication. (A) Progress of BAF53-knockdown cells into G2/M phase. NIH3T3 cells were treated with 50 ng/ml nocodazole for 36 h at 2 h after release from G1/S arrest. The cells were incubated with propidium iodide and subjected to FACS analysis. Note that the cell cycle of BAF53-knockdown cells was arrested either at G1 or G2/M phase, not at S phase. Control cells (green) and the BAF53-knockdown cells (red). (B) BAF53-knockdown cells that underwent the aberrant pattern of replication did not replicate at the next cell cycle. NIH3T3 cells were pulse-labeled with IdU for 20 min at 1 h after release from G1/S arrest. The cells were further incubated for 24 h and then pulse-labeled with CldU for 5 h. Summary of numbers of the cells showing each labeling pattern. (C) Induction of p21 in BAF53-knockdown cells that underwent the aberrant pattern of replication. NIH3T3 cells were pulse-labeled with BrdU for 20 min at 1 h after release from G1/S arrest. The cells were further incubated for 24 h and then fixed and immunostained with anti-p21 antibody. Histograms show distributions of the temporal patterns of replication foci determined by BrdU labeling with or without induction of p21.
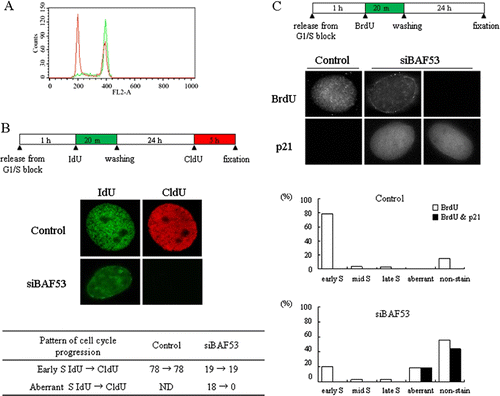
Since it was shown that cell cycle arrest of BAF53 knockdown cells is accompanied by induction of p21 (Lee et al. Citation2007), we next examined whether the BAF53 knockdown cells that replicated aberrantly were subject to p21 induction at the next G1 stage by immunostaining (C). At 1 h into S phase, cells were labeled with BrdU for 20 min and then after 24 h cells were fixed and stained with anti-p21 antibody. None of cells in the control group expressed p21. In the BAF53-knockdown group, however, all of the cells labeled with BrdU as the aberrant pattern expressed p21. The cells labeled with BrdU as the normal early, mid, and late S-phase pattern in BAF53-knockdown group did not express p21. We found p21 staining in the BAF53-knockdown cells that were not labeled with BrdU. These cells seem to be the cells that were already arrested at G1 at the time when they were labeled with BrdU.
Discussion
In this study, we demonstrated that BAF53 is essential for the de novo formation of replication foci. If DNA replication is suppressed by BAF53 knockdown, the number of replication foci pulse-labeled with BrdU will decrease. Even though DNA replication proceeds undisturbed, on the other hand, if replicating DNA is not able to fold to bring them together, replicating DNA labeled with BrdU will be not observed as foci. Previously, we showed that the cell cycle of BAF53-knockdown cells labeled with BrdU at S phase was arrested at the G1/S border of the next cell cycle (Lee et al. Citation2007). In this study, we showed that the cell cycle of BAF53-knockdown cells was arrested at either G1 or G2/M phase, not at S phase, after the treatment of nocodozole. In addition, G1-phase cyclin-dependent kinase inhibitor p21 was induced in the aberrantly labeled BAF53-knockdown cells. These results indicate that BAF53 knockdown does not block S-phase progression and the decrease in the number of replication foci in BAF53-knockdown cells could be due to a loss of the ability of replicating DNA to aggregate into foci.
Since replication foci and chromosomal subdomains represent the same fundamental unit of chromosome structure in interphase nuclei (Zink et al. Citation1998; Albiez et al. Citation2006), these results suggest that BAF53 is essential for the formation of chromosomal subdomains. We previously reported that CTs expanded after BAF53 knockdown (Lee et al. Citation2007). Loss of the attractive interactions such as interactions between adjacent chromatin fibers or the anchorage of the chromatin fiber onto a protein-rich scaffold could account for the expansion of CTs. The data in this study indicate that the attractive interactions that provide a primary force to drive the formation of chromosomal subdomains could be disrupted by BAF53 knockdown. The same disruption of the attractive interactions may be responsible, at least in part, for the expansion of CTs after BAF53 knockdown.
We showed that early S-phase replication foci were preferentially affected by BAF53 depletion. It has been proposed that early S-phase replication foci are mostly constituted of euchromatin abundant with active genes while late S-phase replication foci are constituted of heterochromatin (Hatton et al. Citation1988). Our observations, therefore, suggest that BAF53 is essential for the formation of chromosomal subdomains of euchromatin but not of heterochromatin. Since BAF53 knockdown left a residual amount of BAF53 in the cells, however, we cannot rule out the possibility that chromosomal subdomains of heterochromatin still require BAF53, but are resistant to the disintegration in the presence of a low concentration of BAF53.
The detailed structure of chromosomal subdomains remains still elusive. Nevertheless, DNA loops anchored to a protein scaffold have been a predominant model of the higher-order chromatin architecture of interphase chromatin. The multi-loop subcompartment model states that a series of ~100 kb chromatin loops are clustered to form ~1 Mb rosette-like multi-loop subcompartments (Münkel et al. Citation1999). Assuming chromatin is compacted into a 30-nm fiber, the subcompartment turns out to be a ~500 nm diameter compact structure in computer modeling, which is well consistent with the size of chromosomal subdomains and replication foci. On the other hand, EM images of thin sections of CHO and HeLa cells have demonstrated several features characteristic of a fiber-like structure at a scale much smaller than the average diameter of ~500 nm (Belmont and Bruce Citation1994). Based on these observations, the chromonema fiber model has been proposed in which chromatin constructs a series of progressive folds via intrafiber interactions generating a series of thicker fibers: 60–80 nm and 100–130 nm in thickness. Recently, Hi-C data obtained for human cells have turned out to be consistent with the fractal globule model on the scale of up to 10 Mb (Lieberman-Aiden et al. Citation2009). A fractal globule is characterized by a hierarchy of crumples which is formed spontaneously by polymer collapse. Polymer collapse in interphase chromatin fiber could be provided by chromatin folding into several Mb loops or a rosette-like structure (Mirny Citation2011).
All of these models propose a certain kind of attractive interactions to drive the formation of higher-order structural units. The data in this study suggest that BAF53 is essential for these attractive interactions. BAF53 is an actin-related protein, and is shown to be able to mediate the formation of branching networks of actin filaments in vitro (Rando et al. Citation2002). An actin branching network is the most versatile and dynamic scaffold present in the cells. In addition, BAF53 is also required for the focus formation of γ-H2AX in response to DNA damage (Park et al. Citation2009). Accordingly, a BAF53/actin branching network is an ideal candidate for a nuclear scaffold providing the attractive interactions which are essential for the formation of the fundamental units of chromatin which have been observed as chromosomal subdomains, replication foci and repair foci.
Acknowledgements
This work was supported by the National Research Foundation of Korea (NRF) grant funded by the Korea government (MEST) (No. 2009-0074596).
References
- Albiez , H , Cremer , M Tiberi , C . 2006 . Chromatin domains and the interchromatin compartment form structurally defined and functionally interacting nuclear networks . Chromosome Res. , 14 : 707 – 733 .
- Belmont , AS and Bruce , K. 1994 . Visualization of G1 chromosomes: a folded, twisted, supercoiled chromonema model of interphase chromatid structure . J Cell Biol. , 127 : 287 – 302 .
- Cook , PR and Marenduzzo , D. 2009 . Entropic organization of interphase chromosomes . J Cell Biol. , 186 : 825 – 834 .
- Cremer , T and Cremer , C. 2001 . Chromosome territories, nuclear architecture and gene regulation in mammalian cells . Nat Rev Genet. , 2 : 292 – 301 .
- Hatton , KS , Dhar , V , Brown , EH , Iqbal , MA , Stuart , S , Didamo , VT and Schildkraut , CL. 1988 . Replication program of active and inactive multigene families in mammalian cells . Mol Cell Biol. , 8 : 2149 – 2158 .
- Huberman , JA and Tsai , A. 1973 . Direction of DNA replication in mammalian cells . J Mol Biol. , 75 : 5 – 12 .
- Jackson , DA and Pombo , A. 1998 . Replicon clusters are stable units of chromosome structure: evidence that nuclear organization contributes to the efficient activation and propagation of S phase in human cells . J Cell Biol. , 140 : 1285 – 1295 .
- Lee , K , Kang , MJ , Kwon , SJ , Kwon , YK , Kim , KW , Lim , JH and Kwon , H. 2007 . Expansion of chromosome territories with chromatin decompaction in BAF53-depleted interphase cells . Mol Biol Cell. , 18 : 4013 – 4023 .
- Lee , K , Lee , AY , Kwon , YK and Kwon , H. 2011 . Suppression of HPV E6 and E7 expression by BAF53 depletion in cervical cancer cells . Biochem Biophys Res Commun. , 412 : 328 – 333 .
- Lieberman-Aiden , E , van Berkum , NL Williams , L . 2009 . Comprehensive mapping of long-range interactions reveals folding principles of the human genome . Science. , 326 : 289 – 293 .
- Ma , H , Samarabandu , J , Devdhar , RS , Acharya , R , Cheng , P-C , Meng , C and Berezney , R. 1998 . Spatial and temporal dynamics of DNA replication sites in mammalian cells . J Cell Biol. , 143 : 1415 – 1425 .
- Mirny , LA. 2011 . The fractal globule as a model of chromatin architecture in the cell . Chromosome Res. , 19 : 37 – 51 .
- Müller , WG , Rieder , D , Kreth , G , Cremer , C , Trajanoski , Z and McNally , JG. 2004 . Generic features of tertiary chromatin structure as detected in natural chromosomes . Mol Cell Biol. , 24 : 9359 – 9370 .
- Münkel , C , Eils , R , Dietzel , S , Zink , D , Mehring , C , Wedemann , G , Cremer , T and Langowski , J. 1999 . Compartmentalization of interphase chromosomes observed in simulation and experiment . J Mol Biol. , 285 : 1053 – 1065 .
- Nakamura , H , Morita , T and Sato , C. 1986 . Structural organizations of replicon domains during DNA synthetic phase in the mammalian nucleus . Exp Cell Res. , 165 : 291 – 297 .
- Nasmyth , K and Haering , CH. 2009 . Cohesion: its roles and mechanisms . Annu Rev Genet. , 43 : 525 – 558 .
- Ng , K , Pullirsch , D , Leeb , M and Wutz , A. 2007 . Xist and the order of silencing . EMBO Rep. , 8 : 34 – 39 .
- O'Keefe , RT , Henderson , SC and Spector , DL. 1992 . Dynamic organization of DNA replication in mammalian cell nuclei: spatially and temporally defined replication of chromosome-specific alpha-satellite DNA sequences . J Cell Biol. , 116 : 1095 – 1110 .
- Park , PK , Kang , DH and Kwon , H. 2009 . BAF53 is critical for focus formation of γ-H2AX in response to DNA damage . Anim Cells Syst. , 13 : 405 – 409 .
- Phillips , JE and Corces , VG. 2009 . CTCF: master weaver of the genome . Cell. , 137 : 1194 – 1211 .
- Rando , OJ , Zhao , K , Janmey , P and Crabtree , GR. 2002 . Phosphatidylinositol-dependent actin filament binding by the SWI/SNF-like BAF chromatin remodeling complex . Proc Natl Acad Sci U S A. , 99 : 2824 – 2829 .
- Sung , YH , Choi , EY and Kwon , H. 2001 . Identification of a nuclear protein ArpN as a component of human SWI/SNF complex and its selective association with a subset of active genes . Mol Cells. , 11 : 75 – 81 .
- Zhao , K , Wang , W , Rando , OJ , Xue , Y , Swiderek , K , Kuo , A and Crabtree , GR. 1998 . Rapid and phosphoinositol-dependent binding of the SWI/SNF-like BAF complex to chromatin after T lymphocyte receptor signaling . Cell. , 95 : 625 – 636 .
- Zink , D , Cremer , T , Saffrich , R , Fischer , R , Trendelenburg , MF , Ansorge , W and Stelzer , EHK. 1998 . Structure and dynamics of human interphase chromosome territories in vivo . Hum Genet. , 102 : 241 – 251 .