Abstract
Interdigital tissue regression is one of the most well-known examples of embryonic programmed cell death, providing the mechanism behind separation of developing digits. Caspases have been shown to play a key part in this process, with activated caspase-3 localized between the developing digits. In caspase-3 knock-out adult mice, however, the digits are completely separated with no webbing. In other mutants with defects in the apoptotic machinery, such as Apaf1 deficient mice, interdigital tissue regression is initially inhibited but the webbing eventually disappears as alternative/additional cell death mechanisms step in. In order to investigate whether a similar temporal effect occurs after loss of caspase-3, we have used an in vitro approach to inhibit caspase-3 at specific times during digit separation. Previous limb explant culture approaches have encountered problems with proper limb development in culture, and thus a modified technique was used. The new approach enables detailed observation of the effects of caspase-3 inhibition on interdigital regression. Using these methods, we show that caspase-3 inhibition caused a delay in the loss of interdigital tissue compared with control explants, similar to that observed in Apaf1 mutant mice. Along with immunohistochemistry, active caspase-3 positive cells of the interdigital vs. digital regions were measured by flow cytometry. Notably, activated caspase-3 in vivo was found not only in the interdigital mesenchyme but also in the TUNEL negative digit region, supporting a role for caspase-3 in nonapoptotic events.
Keywords:
Introduction
Digitalization of vertebrate limbs represents a classic example of the role of apoptosis in morphogenesis and development (Doseff Citation2004). Individualization of the digits is achieved by removal of the interdigital tissue freeing the digits from each other. Removal of the interdigital tissue involves cell death, as indicated by high levels of TdT-mediated dUTP-biotin nick end labeling (TUNEL) and Acridine orange positive cells (Montero and Hurle Citation2010). The function of cell death in digit separation has recently been reviewed (Hernandez-Martinez and Covarrubias Citation2011), however, despite our increasing molecular knowledge, the exact roles of individual caspases, and their essentiality has not yet been elucidated. Caspases involved in the mitochondrial apoptotic pathway, caspase-9 and caspase-3 are both activated during interdigital cell death in the mouse (Nakanishi et al. Citation2001), but the digits become individualized in the absence of either of these proteases (Kuida et al. Citation1996; Lakhani et al. Citation2006). Inhibition of all caspases, however, led to a loss of TUNEL positive cells with defects in digit separation (Chautan et al. Citation1999; De Valck and Luyten Citation2001). In Apaf1 mutant mice, where the intrinsic mitochondrial pathway is disrupted upstream of caspase-3, the digits are also normally separated at birth (Chautan et al. Citation1999). Importantly, however, earlier on during embryonic development a delay in interdigital regression is evident in Apaf1 mutants, followed by increased necrosis, indicating that other forms of cell death may compensate for loss of the apoptotic cell death pathway (Cecconi et al. Citation1998; Yoshida et al. Citation1998; Chautan et al. Citation1999). Similar transient changes in organ morphology were reported in the developing teeth of caspase-3 knock-out mice (Matalova et al. Citation2006), where the mitochondrial pathway was reported in signaling centers (Setkova et al. Citation2007). Therefore, caspase-3 deficient mice might also display a transient defect in removal of interdigital cells. To assess these findings in more detail, we turned to an in vitro culture system to study the effect of specific inhibition of caspase-3 on interdigital cell removal.
Materials and methods
Culture technique
Limbs were cultured either separately or in rosettes on filters suspended on grids over the culture medium (A-C), allowing for easier touch-free manipulation and finger morphology documentation. Forelimbs from CD1 mouse embryos were excised and transferred onto membrane discs (Millipore, VCWP02500) or transparent filters (Becton Dickinson Labware, 353090) (A,B). The disc was subsequently placed on a metal grid in culture dishes filled with Dulbecco's Modified Eagle's Medium (Sigma–Aldrich, UK) supplemented with 10% fetal bovine serum, 1% L-glutamine and 1% Penicillin Streptomycin. The culture dishes were placed into a humidified incubator with 5% CO2 atmosphere at a temperature of 37°C.
Figure 1. Cultivation of mouse front limb explant cultures. Setting of individual limbs on a transparent filter (A) and on Millipore filters (B) supported by a metal grid in the Falcon dish. (C) Closer look at rosette cultivation of limb explants. (D–F) Macroscopical limb morphology after culture. (D) 12 hour of cultivation. (E) 24 hour of cultivation with progressing digit separation similar to that observed at E13.5 in vivo. (F) 48 hour of cultivation with advanced digit separation similar to that observed at E14.5 in vivo. (G–I) Histological sections showing proliferation as indicated by PCNA in limbs cultured for 12 hour (G), 24 hour (H), and 48 hour (I). PCNA positive cells are brown, blue background stained by haematoxylin. Alcian blue stains cartilage blue in cultures.
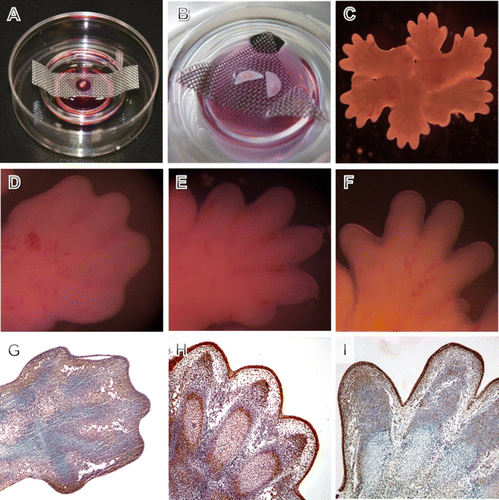
Experimental design
Three groups of mouse front limbs collected at embryonic day (E) 12.5 were cultured over 72 hours, the first group as the cultivation control (medium only), the second as the vehicle control (1% dimethyl sulfoxide [DMSO] corresponding to inhibitor dilution), and the third with caspase-3 inhibitor (R&D System, FMK 004). Fresh medium was added to the cultures everyday. The experiment was repeated independently 3 times with 10 samples per each group. Penetration of the inhibitor in the culture was confirmed using a biotinylated pan caspase inhibitor (R&D System, FMK 011) (data not shown).
Sample collection and processing
Samples were harvested every 12 hours to follow macroscopic morphology and to perform further microscopic analysis. For this purpose, the limbs were fixed overnight in 4% buffered paraformaldehyde, dehydrated in the ethanol series (30%, 50%, 70%, 80%, 90%, 96%, and 100%), treated with xylene and embedded in paraffin.
Flow cytometry
Cells from the collected samples were dissociated using Dispase II (Invitrogen). A FITC-DEVD-FMK inhibitor and corresponding Caspase-3 Detection Kit (QIA91, Calbiochem) were engaged for detection and quantification of caspase-3 positive cells.
In situ labeling
Immunohistochemistry of the proliferating cell nuclear antigen (PCNA, Santa Cruz sc-7907 primary antibody was applied overnight at a concentration of 20 µg/ml) was used to detect proliferating cells in the cultures. Immunohistochemistry of active caspase-3 (Cleaved caspase-3, Cell Signaling, 9664, Asp175, 10 min citrate pretreatment, 20 min blocking serum, 50×diluted primary antibody was applied overnight at 4°C) was employed to localize caspase-3 positive cells. Vectastain PK4001 visualization kit, peroxidase-diaminobenzidine substrate reaction (Dako S3022) and haematoxylin counterstain were used for final visualization of positive cells in brown. TUNEL (Chemicon S7100, peroxidase-diaminobenzidine substrate reaction) was utilized to detect apoptosis, along with evaluation of apoptotic bodies in hematoxylin stained sections.
Results and discussion
Explant cultures represent a modern approach in accordance to the 3R principle of animal science. Particularly in paired organs, such as the limbs, tissues from the same individual can be used as control and experimental samples. Moreover, explant systems allow access to organs, facilitating manipulation, such as bead implantation (Storm and Kingsley Citation1999) or chemical modulation of the medium (Chautan et al. Citation1999). The ability to manipulate a genetic pathway at a specific time point is particularly important in the case of caspases, where the knock-out approach can be developmentally lethal (Meier et al. Citation2000) or requires the generation of conditional or double/triple knock-outs, which is demanding and expensive (Ranger et al. Citation2001). Explants cultures, therefore, provide a welcome technique that can also be applied for RNAi-mediated knockdown.
Previous limb culture experiments have used sponge mounted or freely rotating culture techniques to follow limb development which faced difficulties to achieve proper limb development (De Valck and Luyten Citation2001). Therefore, we have modified an approach formerly applied in other explanted tissue (e.g. Matalova et al. Citation2005; Amin et al. Citation2007; Diep et al. Citation2009).
In order to exclude the influence of in vitro culture on limb development, control cultured limbs were compared with those developing in vivo at corresponding time points (Boehm et al. Citation2011). Importantly, there was no significant difference in the overall morphology of cultured control mouse front limbs compared with the corresponding developmental stage in vivo (D–F and G–I). The explanted limbs were sectioned and the distribution of PCNA-positive proliferating cells was assessed to confirm viability of the cultures (G–I). Cartilage differentiation, establishment of future joint interzones, and digit separation were also comparable to the in vivo situation. In general, localization of active caspase-3 in vivo (A, D, G, and J) positively correlated with TUNEL (B, E, H, and K) and negatively with PCNA positive cells (C, F, I, and L). Active caspase-3 initially appeared at the marginal part of the interdigital segments at E12.5 (A) and gradually expanded deeper into the interdigital mesenchyme (D and G), thus corresponding with the TUNEL pattern (B, E, and H). Notably, at E15.5 caspase-3 was also found in the mesenchyme around the digit cartilage (J), where no apoptosis was evident (K) and this tendency was even more apparent at later stages (data not shown).
Figure 2. Caspase-3 correlation with apoptosis and proliferation in the mouse front limbs in vivo (A–L) and quantification of active caspase-3 positive cells (M–O). Collocalization of caspase-3 immunohistochemistry (IHC) (A, D, G, and J), TUNEL assay (B, E, H, and K), and PCNA immunohistochemistry (C, F, I, and L) in serial sections at E12.5 (A–C), at E13.5 (D–F), at 14.5 (G–I), and at 15.5 (J–L). Positive cells are brown (black arrows), negative blue (white arrows), magnification 200×. Flow cytometry of interdigital (M) and digital (N) cells at 13.5 and percentage comparisons after multiple measurements (O).
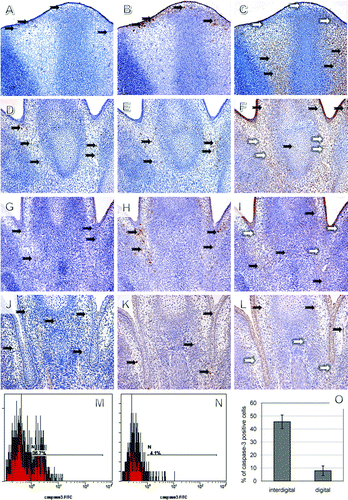
To quantify the number of cells positive for active caspase-3 in the interdigit and digit regions, we turned to a flow cytometry measurement (Matalova et al. Citation2010). This method clearly confirmed the localization of active caspase-3 positive cells in the interdigits at E13.5 (M-O). Our results indicate that this combined technique is an appropriate tool for analysis of the caspase network, allowing for exact separation of individual cells from cultured tissue.
Having confirmed that our culture method gave comparable results to in vivo, we investigated limbs from cultures treated with a specific caspase-3 inhibitor contra control DMSO-treated cultures. E12.5 was selected as the appropriate stage for initiation of our cultures as at this stage was before strong expression of active caspase-3 observed in the interdigit region (G). Both groups developed normally with similar distribution of PCNA positive cells (C, F). In the control group, there were localized TUNEL (B) and caspase-3 (A) positive cells in the interdigital spaces, in the joint interzones, and scattered in the digit region. In the caspase-3 inhibited samples, however, there was no sign of apoptotic bodies or TUNEL positive cells after 24 hours in culture (E), similar to reports published using the general caspase inhibitor (Chautan et al. Citation1999). After 48 and 72 hours (J,K) in culture, TUNEL positive cells reappeared in the interdigital tissue of the caspase-3-inhibited samples. However, webbing was still apparent between the digits after 48 hour, when compared with controls (H and I). Nevertheless, after 72 hours in culture, digit separation in the caspase-3 groups was comparable to the control samples, indicating that the inhibited cultures had managed to catch up with controls (L). In the course of inhibition, no active caspase-3 was detected in the inhibited samples, compared with the controls (D and J). Specific inhibition of caspase-3, therefore, leads to a delay but not a complete inhibition of cell death in the interdigit region, similar to loss of Apaf1. As the medium in the cultures was changed every day, and immunohistochemistry showed no active caspase-3 at any point, the rescue of digit separation was not caused by degradation of the inhibitor in the culture medium.
Figure 3. Pharmacological inhibition of caspase-3 in mouse limb explant cultures. (A–C) control group, (D–F) inhibited group, magnification 200×. (A and D) Caspase-3 activation in serial sections after 24 hours of cultivation based on IHC shows no active caspase-3 in the inhibited samples. (B and E) TUNEL assay in serial sections after 24 hours of cultivation shows dramatic reduction of apoptosis in the inhibited samples. (C and F) Comparable growth of the explants cultures checked using PCNA immunohistochemistry (IHC). (G) Macroscopic view on the cultivation starting point stage limb. (H) Control limb after 48 hours of cultivation with loss of the interdigit tissue. (I) Caspase-3-inhibited explant showing interdigital webbing. (J) Caspase-3 was negative after 72 h of cultivation but (K) TUNEL positive cells reappeared and (L) digit separation continued.
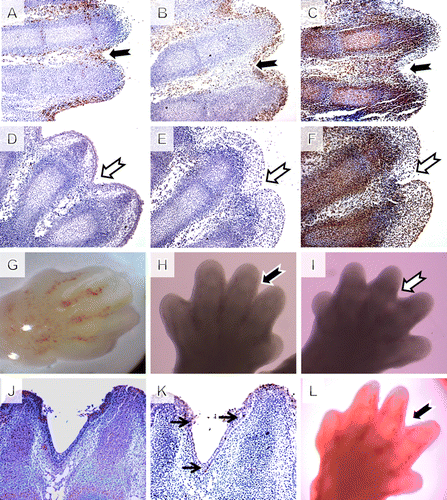
A number of studies have established that regression of interdigital webbing occurs through a caspase-dependent apoptotic process (van der Hoeven et al. Citation1994; Hurle et al. Citation1996) and have demonstrated the presence of active caspase-3 (Mirkes et al. Citation2001). Our findings show that caspase-3 inhibition alone can cause a temporal block in interdigital apoptosis and consequently in limb digitalization. However, in culture, as well as in vivo, cell death and final interdigital tissue regression still occur after loss of caspase-3, indicating activation of some compensatory mechanism. One possibility is that other caspases can compensate in our cultures, and in vivo, for the lack of caspase-3. This is supported by the fact that the inhibition of webbing appears to last longer when a general caspase inhibitor is applied to limb cultures (De Valck and Luyten Citation2001), and the fact that TUNEL positive cells were observed in our inhibited cultures after 48 hours but not 24 hours. It has been proposed that the effector caspases-3, -6, and -7 act redundantly as they share several target proteins (Zuzarte-Luis et al. Citation2006). It is also possible that a caspase-independent and/or necrotic mechanism starts to act later in the cultures, similar to that observed in Apaf1 mutants (Chautan et al. Citation1999).
Finally, the finding that at later stages activated caspase-3 was detected in regions of the limb where no apoptosis was evident, supports an increasing body of evidence that caspases have nonapoptotic roles during development. For example, caspase-3 was reported to be required for osteoclast differentiation (Szymczyk et al. Citation2006), and accordingly caspase-3 knock-out mice display abnormal bone remodeling (Miura et al. Citation2004).
Acknowledgements
The research was supported by the Grant Agency of the Czech Academy of Sciences (embryonal apoptosis – IAA600450904) and the Grant Agency of the Czech Republic (diagnostics of apoptotic cells – 203/08/1680). International cooperation was supported by the Royal Society (JP080875) and the student work (J. Kudelova, I. Chlastakova) by the IGA UVPS (IG101201). The Brno labs are supported by the European Regional Development Fund and the State Budget of the Czech Republic (RECAMO, CZ.1.05/2.1.00/03.0101) and IRP IPAG No. AVOZ 50450515. Thanks for technical help to M. Pospichal, D. Autrata, and A. Norek.
References
- Amin , SA , Matalova , E , Simpson , C , Yoshida , H and Tucker , AS. 2007 . Incudomalleal joint formation: the roles of apoptosis, migration and downregulation . BMC Dev Biol , 7 : 134 – 141 .
- Boehm , B , Rautschka , M , Quintana , L , Raspopovic , J , Jan , Z and Sharpe , J. 2011 . A landmark-free morphometric staging system for the mouse limb bud . Development , 138 : 1227 – 1234 .
- Cecconi , F , Alvares-Bolago , G , Meyer , BI , Roth , KA and Gruss , P. 1998 . Apaf1 (CED-4 homolog) regulates programmed cell death in mammalian development . Cell , 94 : 727 – 737 .
- Chautan , M , Chazal , G , Cecconi , F , Gruss , P and Golstein , P. 1999 . Interdigital cell death can occur through a necrotic and caspase-independent pathway . Curr Biol , 9 : 967 – 970 .
- De Valck , D and Luyten , FP. 2001 . Caspase inhibition supports proper gene expression in ex vivo mouse limb cultures . Cell Death Differ , 8 : 985 – 994 .
- Diep , L , Matalova , E , Mitsiadis , T and Tucker , AS. 2009 . Contribution of the tooth bud mesenchyme to alveolar bone . J Exp Zoolog B Mol Dev Evol , 312B : 510 – 517 .
- Doseff , AI. 2004 . Apoptosis: the sculptor of development . Stem Cells Dev , 13 : 473 – 483 .
- Hernandez-Martinez , R and Covarrubias , L. 2011 . Interdigital cell death function and regulation . Dev Growth Differ , 53 : 245 – 258 .
- Hurle , JM , Ros , MA , Climent , V and Garcia-Martinez , V. 1996 . Morphology and significance of programmed cell death in the developing limb bud of the vertebrate embryo . Microsc Res Techniq , 34 : 236 – 246 .
- Kuida , K , Zheng , TS , Na , SQ , Kuan , CY , Yang , D , Karasuyama , H , Rakic , P and Flavell , RA. 1996 . Decreased apoptosis in the brain and premature lethality in CPP32-deficient mice . Nature , 384 : 368 – 372 .
- Lakhani , SS , Masud , A , Kuida , K , Porter , GA , Booth , CJ , Mehal , WZ , Inayat , I and Flavell , RA. 2006 . Caspase-3 and 7: key mediators of mitochondrial events in apoptosis . Science , 311 : 847 – 851 .
- Matalova , E , Antonarakis , GS , Sharpe , PT and Tucker , AS. 2005 . Cell lineage of primary and secondary enamel knots . Dev Dyn , 233 : 754 – 759 .
- Matalova , E , Sharpe , PT , Lakhani , SA , Roth , KA , Flavell , RA , Setkova , J , Misek , I and Tucker , AS. 2006 . Molar tooth development in caspase-3 deficient mice . Int J Dev Biol , 50 : 491 – 497 .
- Matalova , E , Dubska , L , Fleischmannova , J , Chlastakova , I , Janeckova , E and Tucker , AS. 2010 . Primary enamel knot apoptosis evaluated by flow cytometry of laser capture microdissected samples . Arch Oral Biol , 55 : 570 – 575 .
- Meier , P , Finch , A and Evan , G. 2000 . Apoptosis in development . Nature , 407 : 796 – 801 .
- Mirkes , PE , Little , SA and Umpierre , CC. 2001 . Co-localization of active caspase-3 and DNA fragmentation (TUNEL) in normal and hyperthermia-induced abnormal mouse development . Teratology , 63 : 134 – 143 .
- Miura , M , Chen , XD , Allen , MR , Bi , YM , Gronthos , S , Seo , BM , Lakhani , S , Flavell , RA , Feng , XH Robey , PG . 2004 . A crucial role of caspase-3 in osteogenic differentiation of bone marrow stromal stem cells . J Clin Invest , 114 : 1704 – 1713 .
- Montero , JA and Hurle , JM. 2010 . Sculpturing digit shape by cell death . Apoptosis , 15 : 365 – 375 .
- Nakanishi , K , Maruyama , M , Shibata , T and Morishama , N. 2001 . Identification of a caspase-9 substrate and detection of its cleavage in programmed cell death during mouse development . J Biol Chem , 276 : 41237 – 41244 .
- Ranger , AM , Malynn , BA and Kosmeyer , SJ. 2001 . Mouse models of cell death . Nat Gen , 28 : 113 – 118 .
- Setkova , J , Matalova , E , Sharpe , PT , Misek , I and Tucker , AS. 2007 . Primary enamel knot cell death in Apaf-1 and caspase-9 deficient mice . Arch Oral Biol , 52 : 15 – 19 .
- Storm , EE and Kingsley , DM. 1999 . GDF5 coordinates bone and joint formation during digit development . Dev Biol , 209 : 11 – 27 .
- Szymczyk , KH , Freeman , CS , Adams , V , Srinivas , V and Steinbeck , MJ. 2006 . Active caspase-3 is required for osteoclast differentiation . J Cell Physiol , 209 : 836 – 844 .
- van der Hoeven , F , Schimmang , T , Volkmann , A , Mattei , MG , Kyewski , B and Ruther , U. 1994 . Programmed cell death is affected in the novel mouse mutant Fused toes (Ft) . Development , 120 : 2601 – 2607 .
- Yoshida , H , Kong , YY , Yoshida , R , Elia , AJ , Hakem , A , Hakem , R , Penninger , JM and Mak , TW. 1998 . Apaf1 is required for mitochondrial pathways of apoptosis and brain development . Cell , 94 : 739 – 750 .
- Zuzarte-Luis , V , Berciano , MT , Lafarga , M and Hurle , JM. 2006 . Caspase redundancy and release of mitochondrial apoptotic factors characterize interdigital apoptosis . Apoptosis , 11 : 701 – 715 .