Abstract
The antidiabetic properties in silk materials, such as silkworm and silk fibroin, have been described in traditional folk medicine. In a previous study, we reported that the silk fibroin hydrolysate (SFH) had a protective effect against glucotoxicity in HIT-15 cells and an antidiabetic effect in a noninsulin-dependent diabetes mellitus (type 2 diabetes, T2DM) mouse model. This study aimed to determine whether SFH ameliorates diabetic dyslipidemia and promotes pancreatic β-cell function in a T2DM animal model (C57BL/KsJdb/db). SFH significantly improved blood glucose levels after 4 weeks of treatment. T2DM animals had a normal serum blood glucose level after 6 weeks of SFH treatment. Hemoglobin A1c (HbA1c) levels were significantly lower in T2DM animals that were treated with SFH for 6 weeks. Glucose tolerance in T2DM animals also significantly improved after 6 weeks of SFH treatment. Furthermore, plasma total cholesterol, high-density lipoprotein cholesterol, nonesterified fatty acid, and triglyceride concentrations significantly decreased in SFH-treated T2DM animals compared to T2DM animals. This result showed that SFH ameliorated diabetic dyslipidemia in the T2DM model mice. Taken together, our results suggest that SFH could be used as a potential agent to treat dyslipidemia in patients who have type 2 diabetes mellitus.
Introduction
Type 2 diabetes mellitus (T2DM) is characterized by noninsulin-dependent diabetes mellitus, a deficiency in insulin secretion associated with an insulin resistance of peripheral tissues (Nesher et al. Citation1987). T2DM is a metabolic disorder that induces hyperglycemia and/or dyslipidemia. Diabetic dyslipidemia increases plasma lipid levels, and contributes to the pathogenesis of diabetes by impairing β-cell function (Newfield et al. Citation2008). Oral antidiabetic drugs play an important role in the treatment of T2DM and reduce the complications of T2DM. Many antidiabetic drugs directly decrease blood glucose levels and improve lipid metabolism, but long-term drug intake may cause undesirable side effects (Watkins & Whitcomb Citation1998; Forman et al. Citation2000; Cheng & Fantus Citation2005). Recently, studies have shown that natural food or agents that have been used in folk remedies could be also used as antidiabetic drugs (Obatomi et al. Citation1994; Konno et al. Citation2001). These are suggested to treat T2DM effectively with minimal side effects in clinical experience at relatively low costs.
Silk-related materials have hypoglycemic substances such as Moran A, Moran 20K, and moranoline (1-deoxynojirimycin) that are extracted from mulberry root bark (Hikino et al. Citation1985; Kim et al. Citation1999). Silk-related materials have been used in antidiabetic treatments in oriental medicine. In the previous study, silk fibroin hydrolysate (SFH) prevents pancreatic β-cell death by glucotoxicity and increases pancreatic β-cell function (Park et al. Citation2011, Do et al. Citation2012). In addition, SFH enhances insulin sensitivity and glucose metabolism in 3T3-L1 adipocytes (Hyun et al. Citation2004). However, most SFH functional studies were focused on hyperglycemia and the effect of SFH on diabetic dyslipidemia in type 2 diabetic model mice was not explored.
This study aimed to determine whether SFH ameliorates diabetic dyslipidemia and promotes pancreatic β-cell function in a T2DM animal model (C57BL/KsJdb/db, DB). SFH-treated groups displayed decreased serum lipids levels. Insulin levels were increased compared to nontreated DB mice. Immunohistological staining showed that SFH-treated DB mice had higher insulin immunoreactivity in their pancreatic islets. The present study strongly suggests that SFH has improved lipid metabolism and pancreatic β-cell function in a T2DM animal model.
Materials and methods
Animals
We used C57BL/KsJdb/db (DB) and normal nondiabetic mice (C57BL/KsJdb/+ or +/ +, ND) that were supplied from SLC, Inc. (Shizuoka, Japan) at 7 weeks of age. The animals were housed in a specific pathogenic-free room with controlled temperature (23 ± 2°C), humidity (55 ± 10%), and light (08:00 am–20:00 pm). The mice were given normal commercial laboratory chow for mice (Jeiljedang, South Korea) and water ad libitum, except for mice that were given 20% SFH solution instead of water. Animals were divided into four groups at 7 weeks of age as follows: C57BL/KsJdb/db mice (DB-NT group, n = 10) and normal nondiabetic (ND-NT group, n = 10) were used as control groups, a 20% drinking water admixture of SFH was prepared and DB mice (DB-SF group, n = 10) and normal nondiabetic mice (ND-SF group, n = 10) were treated with it for 6 weeks. The animal study was conducted in accordance with the Institutional Animal Care and Use Committees of Hallym University.
Preparation of low-molecular weight SFH
SFH was prepared from the cocoons of bombyx mori (Chinese silk worm). Briefly, serin and other impurities of the raw cocoons (1 kg) were removed with 5% Na2CO3. Pure silk fibroin was treated with 2N HCl at 100°C for 48 hours and neutralized using a 2N NaOH solution. The solution was used to carry out proteolytic reactions using Chymotrypsin (Sigma, USA). Low-molecular weight silk fibroin was acquired through a desalting process using ion exchange chromatography (Nam & Oh Citation1995; Park et al. Citation2011). The amino acid composition of SFH was determined by an amino acid analyzer (Pico-Tag System, Waters 486/600/626, USA) (Batra et al. Citation1989; Do et al. Citation2012).
Measurement of body weight, glucose level, lipid profiles, and insulin
Body weight was measured with an animal balance every week (Mettler, USA). Blood samples were collected from the infraorbital plexus under anesthesia. Glucose levels were detected and measured by ONETOUCH glucose meter (LifeScan, USA). Cholesterol, high-density lipoprotein cholesterol (HDL-C), and triglycerides (TGs) were measured by an enzymatic method with dry chemistry (Reflotron, Roche Diagnostics, Switzerland). Nonesterified free fatty acids (NEFA) were also measured by an enzymatic method with a micro flow-cell photometer (AE-100F, Erma, Japan) using reagent kits (Eiken Chemical, Japan). A radioimmunoassay (RIA) method was used for measuring plasma insulin with a commercial RIA kit (Linco Research, USA) and validated by ELISA kit (Shibayagi, Japan).
Intraperitoneal glucose tolerance test (IPGTT)
IPGTTs were performed for 3 days before the mice were sacrificed. The mice were fasted overnight and then 2 g/kg D-glucose (Sigma, USA) solution was administered. Blood samples were obtained at 0, 30, 60, and 120 minutes after glucose challenge. Plasma glucose and insulin were measured as described below.
Pancreatic islet isolation
The peritoneum was surged and the pancreas was removed after the injection of collagenase P (Beohringer Mannheim, German) into the common pancreatic duct. The removed pancreas was incubated for 10 min at 37°C and effused tissues from the digested pancreas were harvested. The harvested tissues were washed twice with HBSS (Gibco BRL, USA) by centrifugation, resuspended evenly in Ficoll (Sigma, USA) with a density of 1.086 g/ml, and overlaid with Ficoll with a density of 1.076 and 1.053 g/ml serially. Thereafter, the tube was centrifuged for 10 min at 800×g in a refrigerated centrifuge, and islets with densities of between 1.076 and 1.053 were taken carefully and washed twice with Hank's Balanced Salt Solution (HBSS).
Immunohistochemistry
After 6 weeks of experiment, whole pancreatic tissue was resected from each mouse and weighed. Pancreatic tissue was fixed in 10% neutral buffered formalin, blocked in paraffin, and sectioned at 4 µm on an automatic rotary microtome (Zeiss Super Cut 2050 microtom, Germany). The sections of pancreatic portions were stained with rabbit antibodies against insulin (diluted 1:50 with blocking solution, sc-9168, Santa Cruz Inc., USA) and glucagon (diluted 1:50 with blocking solution, sc-13091, Santa Cruz Inc., USA). After overnight incubation of the primary antibody at 4°C, goat antirabbit IgG second antibodies (1:1000 with blocking solution, Invitrogen, Carlsbad, USA) were treated. Samples were observed under a Zeiss Axio imager microscope (Zeiss, Germany).
Statistical analysis
For comparison between two groups, the unpaired Student's t-test was used, for multiple comparisons, the one-way analysis of variance was used. All data were analyzed by the GraphPad Prism 4 program (GraphPad Software, USA). Statistically significant difference was defined as a p-value of less than 0.05.
Results
Effect of SFH on blood glucose
In order to explore whether SFH had an antidiabetic effect, we used 7-week-old diabetic (db/db, DB) and nondiabetic mice (db/ +, ND). As shown in , we measured serum glucose levels. Almost all DB mice had hyperglycemia before SFH treatment (DB-SF) and/or nontreatment (DB-NT). DB-SF and ND-SF mice were given drinking water containing dissolved SFH for 6 weeks. In the DB-SF group, serum glucose levels started to decrease significantly at the third week of SF treatment. At 6 weeks, DB-SF mice had normal serum glucose levels (). Hemoglobin A1c (HbA1c) levels were significantly lower in DB-SF mice than DB-NT mice after 6 weeks of treatment with SFH ().
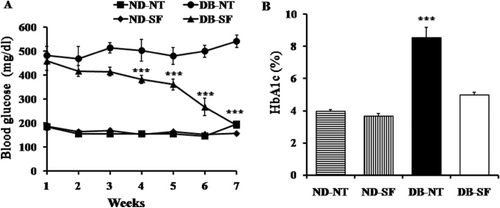
Throughout the experimental period, SFH treatment did not affect body weight (). Food consumption of the DB-SF was not significantly different compared to that of the DB-NT mice (), but the water consumption of the DB-NT was significantly higher than that of the ND-NT, ND-SF, and DB-SF mice indicating that DB-NT mice have polydipsia ().
Effect of SFH on the glucose tolerance test
IPGTTs were performed after 6 weeks of treatment of DB-NT and DB-SF mice. DB-NT mice had hyperglycemia before glucose injection. These mice showed hyperglycemia at 120 min after glucose injection, indicating that the DB-NT mice had glucose tolerance. After 6 weeks of SFH treatment of DB mice, glucose tolerance was significantly ameliorated (). DB-SF mice had normal serum glucose levels at 0 min, and returned to the normal range at 120 min after glucose injection, indicating that SFH had ameliorated glucose tolerance. Furthermore, the plasma insulin levels of DB-SF mice were higher at 0–120 min after glucose injection than those of the DB-NT mice ().
Effect of SFH on lipid profiles
To investigate the antilipidemic effect of SFH, we measured plasma lipids levels at weeks 0 and 6. Plasma total cholesterol, HDL-C, NEFA, and TG concentration significantly decreased in DB-SF mice compared to the DB-NT mice after 6 weeks (–). Previous studies found that DB mice develop insulinopenic diabetes at around 13 weeks and express decreased serum insulin levels. As shown in , DB-NT mice develop insulinopenic diabetes after 6 weeks. Plasma insulin levels were not significantly decreased in DB-SF mice after SFH treatment ().
Effect of SFH on pancreatic β-cells
To further explore the effect of SFH on pancreatic β-cell survival and function, we performed a pancreatic islet histological study. DB-NT mice pancreatic islets had weak insulin immunoreactivity and an elevated glucagon positive cell number. However, the pancreatic islets of DB-SF mice have well-granulated β-cells with normal insulin immunoreactivity and the number of glucagon positive cell was not elevated (). We examined whether SFH induced pancreatic β-cell insulin synthesis. To determine this, we also checked the insulin amount of isolated pancreatic islets, and it was found that DB-SF mice have significantly increased insulin levels in isolated pancreatic islets compared to those of DB-NT mice ().
Discussion
The present study was undertaken to investigate the antidiabetic and antilipidemic effects of SFH in a type 2 diabetic C57BL/KsJ-db/db mouse model. The DB mouse has become a popular model for type 2 diabetes mellitus (T2DM), because it is genetically well defined and similar to human T2DM (Chen et al. Citation1996; Kobayashi et al. Citation2000). SFH treatment to DB mice for 6 weeks improved plasma glucose levels. The body weight of SFH treatment and SFH nontreatment mice did not significantly differ, indicating that SFH did not have a nutritional effect. Hemoglobin A1c was significantly reduced in DB-SF mice compared to DB-NT mice. More importantly, the IPGTT results clearly showed that SFH ameliorated glucose tolerance. These results were in accordance with those of our previous study and suggest that SFH had an antidiabetic effect in a type 2 diabetic mouse model.
The characteristic features of diabetic dyslipidemia are an increased plasma TG level and free fatty acids (Newfield et al. Citation2008). The DB mice have high blood TG and cholesterol, and they are well known for being a hyperlipidemia model (Nishina et al. Citation1994). Our lipid data showed a decreased level of total cholesterol, HDL-C, NEFA, and TG which are known as an antiatherosclerotic index. These reduced levels of lipid markers are important clinical symptoms because hyperlipidemia is generally associated with pancreatic β-cell malfunction (Zhou & Grill Citation1994; McGarry & Dobbins Citation1999; Poitout & Robertson Citation2002) and induced diabetic cardiovascular disease (Mooradian Citation2009). A decreased level of lipid indicators might contribute to a reduction in lipotoxicity on the pancreatic β-cells of DB-SF mice.
The DB mice markedly increased plasma HDL-C levels compared with that of normal mice (Silver et al. Citation1999). Elevated total cholesterol levels were mainly due to an increase of HDL-C in DB mice (Nishina et al. Citation1994). In our experiment, SFH decreased HDL-C and total cholesterol levels in DB mice. Decreased HDL-C levels were mainly due to a decrease of total cholesterol in SFH-treated DB mice.
At 15 weeks of age, DB mice are known to develop pancreas islet atrophy and insulinopenia (Kobayashi et al. Citation2000; Garris & Garris Citation2004). After 6 weeks of SFH treatment of DB mice, insulin density in pancreatic islets increased in DB-SF mice compared to DB-NT mice. These immunohistochemical observations indicated that SFH treatment of DB mice increased pancreatic β-cell survival and function.
In summary, our results indicate that SFH treatment significantly ameliorated diabetic symptoms and improved pancreatic β-cell function in a T2DM animal model. Furthermore, SFH treatment ameliorated diabetic dyslipidemia in a T2DM animal model. Taken together, our results suggest that SFH could be used as a potential therapeutic agent for diabetic dyslipidemia in T2DM patients.
Acknowledgments
This research was supported by Basic Science Research Program through the National Research Foundation of Korea (NRF) funded by the Ministry of Education, Science and Technology (2011-0024062), by Priority Research Centers Program through the National Research Foundation of Korea (NRF) funded by the Ministry of Education, Science and Technology (NRF-2009-0094071), and by Hallym University Specialization Fund (HRF-S-41).
References
- Batra SP, Lark RH, Colman RF. 1989. Identification of histidyl peptide labeled by 2-(4-bromo-2,3-dioxobutylthio)adenosine 5′-monophosphate in an ADP regulatory site of glutamate dehydrogenase. Arch Biochem Biophys. 270:277–285. doi:10.1016/0003-9861(89)90029-5
- Chen H, Charlat O, Tartaglia LA, Woolf EA, Weng X, Ellis SJ, Lakey ND, Culpepper J, Moore KJ, Breitbart RE, et al. 1996. Evidence that the diabetes gene encodes the leptin receptor: identification of a mutation in the leptin receptor gene in db/db mice. Cell. 84:491–495. doi:10.1016/S0092-8674(00)81294-5
- Cheng AY, Fantus IG. 2005. Oral antihyperglycemic therapy for type 2 diabetes mellitus. CMAJ. 172:213–226. 10.1503/cmaj.1031414
- Do SG, Park JH, Nam H, Kim JB, Lee JY, Oh YS, Suh JG. 2012. Anti-diabetic effect of silk fibroin hydrolysate via increasing pancreatic β cell mass in C57BL/KsJ-db/db mice. J Vet Sci. 13:339–344. 10.4142/jvs.2012.13.4.339
- Forman LM, Simmons DA, Diamond RH. 2000. Hepatic failure in a patient taking rosiglitazone. Ann Intern Med. 132:118–121. 10.7326/0003-4819-132-2-200001180-00005
- Garris DR, Garris BL. 2004. Cytochemical analysis of pancreatic islet hypercytolipidemia following diabetes (db/db) and obese (ob/ob) mutation expression: influence of genomic background. Pathobiology. 71:231–240. 10.1159/000080056
- Hikino H, Mizuno T, Oshima Y, Konno C. 1985. Isolation and hypoglycemic activity of moran A, a glycoprotein of Morus alba root barks. Planta Med. 51:159–160. 10.1055/s-2007-969435
- Hyun CK, Kim IY, Frost SC. 2004. Soluble fibroin enhances insulin sensitivity and glucose metabolism in 3T3-L1 adipocytes. J Nutr. 134:3257–3263.
- Kim ES, Park SJ, Lee EJ, Kim BK, Huh H, Lee BJ. 1999. Purification and characterization of Moran 20K from Morus alba. Arch Pharm Res. 22:9–12. 10.1007/BF02976428
- Kobayashi K, Forte TM, Taniguchi S, Ishida BY, Oka K, Chan L. 2000. The db/db mouse, a model for diabetic dyslipidemia: molecular characterization and effects of Western diet feeding. Metabolism. 49:22–31. 10.1016/S0026-0495(00)90588-2
- Konno S, Tortorelis DG, Fullerton SA, Samadi AA, Hettiarachchi J, Tazaki H. 2001. A possible hypoglycemic effect of maitake mushroom on type 2 diabetic patients. Diabet Med. 18:1010. 10.1046/j.1464-5491.2001.00532-5.x
- McGarry JD, Dobbins RL. 1999. Fatty acids, lipotoxicity and insulin secretion. Diabetologia. 42:128–138. 10.1007/s001250051130
- Mooradian AD. 2009. Dyslipidemia in type 2 diabetes mellitus. Nat Clin Pract Endocrinol Metab. 5:150–159. 10.1038/ncpendmet1066
- Nam JH, Oh YS. 1995. A study of pharmacological effect of silk fibroin. Agric Sci. 37:145–157.
- Nesher R, Della Casa L, Litvin Y, Sinai J, Del Rio G, Pevsner B, Wax Y, Cerasi E. 1987. Insulin deficiency and insulin resistance in type 2 (noninsulin-dependent) diabetes: quantitative contributions of pancreatic and peripheral responses to glucose homeostasis. Eur J Clin Invest. 17:266–274. 10.1111/j.1365-2362.1987.tb01247.x
- Newfield RS, Dewan AK, Jain S. 2008. Dyslipidemia in children with type 2 diabetes vs. obesity. Pediatr Diabetes. 9:115–121. 10.1111/j.1399-5448.2007.00345.x
- Nishina PM, Lowe S, Wang J, Paigen B. 1994. Characterization of plasma lipids in genetically obese mice: the mutants obese, diabetes, fat, tubby, and lethal yellow. Metabolism. 43:549–553. 10.1016/0026-0495(94)90194-5
- Obatomi DK, Bikomo EO, Temple VJ. 1994. Anti-diabetic properties of the African mistletoe in streptozotocin-induced diabetic rats. J Ethnopharmacol. 43:13–17. 10.1016/0378-8741(94)90111-2
- Park JH, Nam Y, Park SY, Kim JK, Choe NH, Lee JY, Oh YS, Suh JG. 2011. Silk fibroin has a protective effect against high glucose induced apoptosis in HIT-T15 cells. J Biochem Mol Toxicol. 25:238–243. 10.1002/jbt.20381
- Poitout V, Robertson RP. 2002. Minireview: secondary beta-cell failure in type 2 diabetes–a convergence of glucotoxicity and lipotoxicity. Endocrinology. 143:339–342. 10.1210/en.143.2.339.
- Silver DL, Jiang X, Tall AR. 1999. Increased high density lipoprotein (HDL), defective hepatic catabolism of ApoA-I and ApoA-II, and decreased ApoA-I mRNA in ob/ob mice. Possible role of leptin in stimulation of HDL turnover. J Biol Chem. 274:4140–4146. 10.1074/jbc.274.7.4140
- Watkins PB, Whitcomb RW. 1998. Hepatic dysfunction associated with troglitazone. N Engl J Med. 338:916–917. 10.1056/NEJM199803263381314
- Zhou YP, Grill VE. 1994. Long-term exposure of rat pancreatic islets to fatty acids inhibits glucose-induced insulin secretion and biosynthesis through a glucose fatty acid cycle. J Clin Invest. 93:870–876. 10.1172/JCI117042