Abstract
This study was performed to produce a strong antibody against repeated peptides for a specific region of an antigen. Nine amino acids at C-terminal from human brain β-tubulin III were selected as a target sequence since it is well known for a high conservancy of sequence and resistance to antibody production. The synthetic oligonucleotides had six bases (5′-CGAC—GT-3′, 5′-TC—CAGC-3′) appended at each end and were repeatedly linked and directly joined by ligase in the presence of restriction enzymes (AccI, AvaI) to allow for the construction of head-to-tail repeats. Ligated oligonucleotides containing more than 10-unit repeats were eluted from agarose gels and cloned into the AccI site of a modified pGEX expression vector. The cloned repeated target sequences were subsequently expressed in a bacterial system. A large amount of glutathione-S-transferase-fusion protein containing the unit repeats was purified by affinity chromatography on glutathione-agarose beads and injected into Balb/c mice to produce polyclonal and monoclonal antibodies (Abs). Total protein from several mammalian brains was extracted and utilised to test the quality of the generated Abs in immunoblot assays. The generated Abs specifically recognised the 55-kDa human brain β-tubulin protein but not any other mammalian brain β-tubulins. The potential uses of this system could be applied to an effective epitope generation for an antibody and to the development of a new type of subunit vaccine.
Introduction
When the amino acid or nucleotide sequence of a gene is known, synthetic peptides can be made and used as immunogens to produce antibodies (Abs; Sutcliffe et al. Citation1980; Lerner Citation1984; Walter Citation1986). Later, an ideal alternative to the use of biochemically produced synthetic peptides has emerged as an antigen expression by a cloned gene in bacterial systems (Carrol & Laughon Citation1987). However, the rate of Ab production using such systems was lower than that from the natural antigen. This lower rate of Ab production resulted from either a structural property of the peptide itself or from the non-efficient functioning of the smaller peptides in vivo. To compensate for this defect, strategies linking small peptides to carrier proteins, such as bovine serum albumin (BSA) and keyhole impet hemocyanin (KLH), were introduced and have been popular since their introduction (Harlow Citation1988). This still did not completely exclude the substantial possibility that the carrier protein itself may result in a stronger immune reaction than the intended antigen, thereby resulting in little or no production of Ab against the specific peptide. Subsequently, an alternative idea was presented in which a macro-molecule was assembled by repetitive linkage of small specific peptides to generate a strong and efficient Ab response (Astori & Kraehenbuhl Citation1996; Yu et al. Citation2000; Schroeder et al. Citation2009).
Repetitive synthetic peptide technology can be applied to vaccine development. Since vaccines were introduced against smallpox in the late eighteenth century, rapid progress has been made using dead or inactivated antigens. As the problems of safety, stability, efficacy and cost of vaccines have become key issues and as advances in molecular biology and biochemistry have allowed for analyses at the gene level in infectious material, vaccine research has proceeded further with diversity. One of the outcomes of this diversification has been the introduction and the development of subunit vaccines that utilise a specific part of the infectious organism (Foged Citation2011; Patarroyo et al. Citation2011). Such recombinant vaccines may utilise chemically synthesised protein/peptides or genetic manipulation that allows for expression of antigens in other species. Subunit vaccines generated from peptides containing only a single epitope are typically not fully functional and thus may not be efficient to induce a safe immune response in vivo. However, peptides containing numerous epitopes generated by linkage of multiple repetitive small peptides can cause a strong immune response and function efficiently to prevent secondary infection (Billetta et al. Citation1991; Yankai et al. Citation2006). Repetitive synthetic peptides can be also used in the development of diagnostic reagents for various diseases. It is known that the percentage of Abs recognising only one specific epitope is high in blood and that most proteins have as few as two–four epitopes (Welling et al. Citation1985; Kolaskar & Tongaonkar Citation1990). Therefore, if a repetitive peptide is generated using the amino acids of a specific epitope, it can be used as a sensitive diagnostic tool to detect a strong immune response.
In this study, we aimed to develop a method for the efficient synthesis of repetitive peptides and production of polyclonal (pAb) and monoclonal (mAb) Abs against these repetitive synthetic peptides. The nucleotide sequence for nine amino acids at the C-terminus of human brain β-tubulin III was chosen as a target sequence and synthesised with a designated linker at the 5′- and 3′-ends. The synthesised oligonucleotide was repetitively linked through random ligation in the presence of specific restriction enzymes, and the repetitive oligonucleotides generated with a head-to-tail orientation were selected. The selected repetitive oligonucleotide was then cloned into a modified expression vector and expressed as a fusion protein in a bacterial system. The purified repetitive peptide was used as an immunogen to generate pAb and mAbs. The resulting Abs were evaluated to confirm their specificity for human brain proteins as compared to brain proteins from other mammals.
Materials and methods
Materials
Oligonucleotides were synthesised at the Research Facility in Seoul National University. Kinase, ligase and restriction enzymes including AccI were obtained from Boehringer Mannheim GmbH (Ingelheim, Germany). All reagents needed for production of Abs were purchased from Gibco BRL (Carlsbad, CA). Glutathione-agarose beads were purchased from Amersham Biosciences (Pittsburgh, PA), and other chemical reagents including mAbs to β-tubulin type III (T5076) and pAb to β-tubulin (SAB4500088) were obtained from Sigma-Aldrich (St. Louis, MO). Balb/c mice were purchased from the Experimental Animal Center of Hallym University. All experimental procedures involving animals and their care conformed to the Guide for the Care and Use of Laboratory Animals of the National Veterinary Research and Quarantine Service of Korea and were approved by the Hallym Medical Center Institutional Animal Care and Use Committee.
DNA
Construction of the pGEX-Acc vector
pGEX-KT is a modified version of the pGEX-1 vector into which five consecutive glycine linkers were inserted to allow for easy removal of the glutathione-S-transferase (GST) carrier protein by BamHI and thrombin cleavage (Hakes & Dixon Citation1992). pGEX-KT was double-digested with EcoRI/BamHI (5′-CGT G.….A ATT CAT-3′) and then ligated to a synthetic linker (5′-GA TCC GTC GAC-3′) that included an AccI restriction site. Successful cloning of the synthetic linker into pGEX-KT (pGEX-Acc) was confirmed by digestion with AccI (5′-CGT GGA TCC GT.….C GAC AAT TCA-3′).
Generation of a repetitive oligonucleotide corresponding to the C-terminus of human β-tubulin III
10 µl of a 1 M sodium chloride solution was added to 40 µl of a mixture of complementary oligonucleotides (50 µg, ). The mixture was incubated at 90°C for 10 min and then cooled to room temperature to allow for annealing. Oligonucleotides were precipitated by addition of 2 vol. of 100% ethanol (EtOH) and 0.1 vol. of sodium acetate, followed by centrifugation at 4°C for 10 min. The double-stranded oligonucleotides were phosphorylated in a kinase reaction (5 units of T4 DNA kinase, 10X kinase buffer and 10 mM adenosine triphosphate (ATP)) at 37°C for 2 hr. The phosphorylated oligonucleotides were incubated in a combined ligation (5 units of T4 DNA ligase, 10X ligase buffer and 10 mM ATP) and digestion reaction (2 units of AccI/AvaI) at 37°C for 4 hr. Oligonucleotides larger than 300-bp were isolated either by gel electrophoresis or using a spin column method. The repetitive oligonucleotides were then subjected to double digestion with AccI/AvaI and a kinase reaction.
Table 1. Nine amino acids of the human brain β-tubulin type III C-terminus, E. coli codon usage in the target peptide, and the linkers at the 5′- and 3′-ends for ligation of repetitive oligonucleotides.
Construction of pGEX-hβ-tubulin-C9 expression vector
pGEX-Acc was digested with AccI, followed by dephosphorylation with calf intestine alkaline phosphatase (CIP) at 37°C for 30 min, and then 0.1 vol. of 200 mM EGTA was added, and incubation was continued at 65°C for 10 min to inactivate enzyme activity. Dephosphorylated, AccI-digested pGEX-Acc (30 ng) was ligated with the phosphorylated, 300-bp or greater hβ-tubulin III C-terminal repetitive oligonucleotides at 4°C for 10 hr.
Sequence determination
In order to make sure that the repetitive oligonucleotides cloned into pGEX-Acc had the correct head-to-tail orientation, the pGEX-hβ-tubulin-C9 expression vector was sequenced with Sequenase 2.0 (US Biochemicals, Cleveland, OH) and ATP labelled with S35 according to the manufacturers' manual. A modified sequencing gel system of Lang & Burger (Citation1990) was used for running the gel. After gel electrophoresis, the gel was transferred to 3 MM paper, dried for 1 hr using a gel dryer, placed in a cassette with X-ray film (Kodak BioMax MR Film), exposed at 4°C for 10 hr and then developed.
Proteins
Purification of recombinant peptides
A GST expression construct encoding the repetitive nine C-terminal amino acids of human β-tubulin III was expressed following isopropylthio-beta–galactoside (IPTG) induction in BL21 (DE3) Escherichia coli and purified using glutathione-agarose beads (50% slurry) in the presence of the reduced form of glutathione. Proteins were eluted with 5 mM reduced glutathione, dialysed against a solution containing 20 mM Tris-HCl, pH 8.0, 100 mM NaCl, 10% glycerol, and then clarified by centrifugation (Smith & Johnson Citation1988). In order to purify the repetitive nine C-terminal amino acids of human β-tubulin, 1ml of glutathione-agarose bead-bound GST-fusion protein was washed twice in Phosphate Buffered Saline Tween-20 (PBST) (PBS + 1% Triton X-100), once in GST wash buffer (50 mM Tris, 150 mM NaCl and pH 7.5) and treated with thrombin protease in thrombin cleavage buffer (50 mM Tris, 150 mM NaCl, 2.5 mM CaCl2 and pH 7.5) for 4 hr. Protein was retrieved from the supernatant after centrifugation, quickly frozen in liquid nitrogen and stored at −70°C until use.
SDS-PAGE and immunoblotting
Proteins were denatured by heating for 5 min at 95°C in 2X SDS sample buffer and separated on SDS-polyacrylamide gels (Laemmli Citation1970). Protein concentration was determined using the Bradford assay method (Citation1976). Gels were either stained for total protein with Coomassie Blue or electrotransferred to nitrocellulose (0.45 µm pore size; Towbin et al. Citation1979) for immunoblot analyses. Nitrocellulose blots were blocked with 5% non-fat powdered milk and probed with primary Abs for 2 hr at room temperature or overnight at 4°C. Bound Abs were visualised using anti-mouse IgG Abs conjugated to horseradish peroxidase and an enhanced chemiluminescence (ECL) substrate kit (Pierce, Rockford, IL). Reactive polypeptides were detected by exposure to Biomax-MS X-ray film (Eastman Kodak, Rochester, NY).
Preparation of Abs
The immunisations with human β-tubulin III repetitive C-terminal peptide, determination of antibody titer, cell fusion, screening of hybridomas by ELISA and production of ascitic fluids were conducted according to a standard protocol (Goding Citation1986). In brief, 6- to 8-wk-old Balb/c mice were immunised with 50 µg of immunogen premixed with an equal volume of complete Freund's adjuvant. This first injection was followed by three or four booster injections with the same amount of immunogen mixed with incomplete Freund's adjuvant every other week. Two fusion experiments were conducted using the spleens of immunised mice and myeloma cells to select many hybridomas responding to different epitopes of the immunogen. Ascitic fluid was generated for large-scale production of mAbs, cleared by centrifugation and applied onto a Protein G column to purify mAbs. The mAbs were eluted with 0.1 M glycine-HCl (pH 2.5), neutralised with 1 M Tris (pH 8.0), dialysed against PBS and then stored at −70°C until use.
Results and discussion
Target sequence and preparation of pGEX-Acc vector
Human brain-specific β-tubulin has eight isotypes, and it is very difficult to produce type specific Abs due to the high degree of sequence conservation among isotypes (Sullivan & Cleveland Citation1986; Lopata & Cleveland Citation1987; Banerjee et al. Citation1990). In vertebrates, comparison of the C-terminal portion of β-tubulin isotypes revealed a couple of amino acids differences. Since 5 to 10 amino acids, in general, comprise an epitope, we hypothesised that a nine amino acid unit, ‘EESESQGPK’, from the C-terminus of a human brain β-tubulin minor isotype could be utilised as a repetitive epitope to generate a type-specific Ab through immunisation. Two oligonucleotide sequences encoding these amino acids were synthesised using the codon usage pattern of E. coli ().
We designed one oligonucleotide for the nine amino acids of the C-terminus of human brain β-tubulin to have extra CGAG and GT nucleotides at the 5′ and 3′ ends, respectively. The complementary oligonucleotide contained CGAC and CT at the 5′ and 3′ ends, respectively. Introduction of these six extra bases at the sequence ends made it possible to clone the generated repetitive oligonucleotide into the AccI restriction site of a modified expression vector (pGEX-KT) and to determine the direction of cloning by digestion with specific two restriction enzymes (AccI, AvaI). In order to express the specific polypeptides, two prerequisites had to be met with respect to cloning the oligonucleotides: oligonucleotides had to be cloned in the correct direction and had to be in-frame for translation. Oligonucleotides having six extra bases have cohesive ends that can be linked in three possible orientations to assemble repetitive oligonucleotides when ligated: head-to-head, tail-to-tail, and head-to-tail (). In our strategy, when repetitive oligonucleotides assembled in a head-to-tail orientation, the oligonucleotide sequence for any gene would be placed in-frame into the expression vector and thus enable successful expression of the repetitive polypeptide.
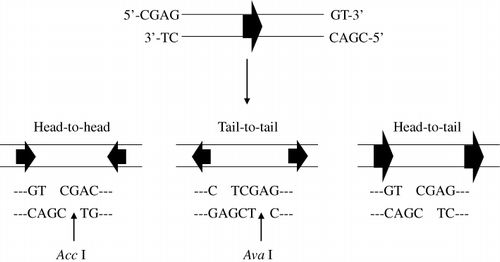
We modified the pGEX-KT expression vector to allow for introduction of the repetitively ligated oligonucleotide in the desired orientation by double digestion with BamHI/EcoRI and subsequent insertion of a synthetic linker that included an AccI restriction site (). Successful construction of the pGEX-Acc vector (pGEX-KT + synthetic linker) was confirmed by AccI digestion and gel electrophoresis of the digested vector. As shown in , pGEX-KT was not digested (Lane 1), while treatment of pGEX-Acc with AccI resulted in a 4.9 kb linear DNA fragment (Lanes 2 and 3), indicating that the pGEX-Acc vector was constructed properly as it was able to provide the AccI restriction site.
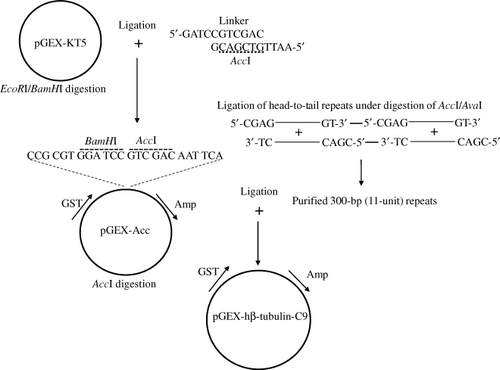
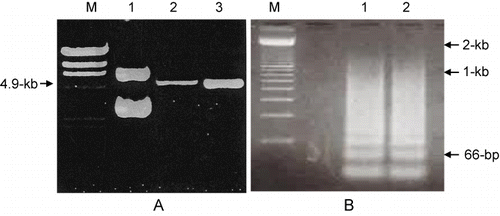
Construction and cloning of repetitive oligonucleotides
A mixture of equal amounts of two single-stranded complementary oligonucleotides was completely denatured by heating at 90°C for 10 min and converted to a double-stranded form by cooling to RT for 1 hr to allow for annealing to occur. Annealed oligonucleotides were subsequently 5′-end phosphorylated in a kinase reaction. The double-strand target oligonucleotide was ligated in the presence of two restriction enzymes, AccI and AvaI, to prevent linkage of head-to-head or tail-to-tail orientated oligonucleotides, as shown in . The ligation reaction was not performed at 4°C where T4 DNA ligase is highly active, but rather at 37°C in order to maintain the activity of restriction enzymes. The various ligation products were analysed, and the most abundant product was found to be a 2-unit repeat (66 bases) that was derived from two single 33-base units ligated to each other. However, products as large as 2 kb consisting of a 60-unit repeat of oligonucleotides were also detected ().
Oligonucleotides containing repeats of greater than 10 units, corresponding to greater than 330-bp, were isolated, cloned into AccI-digested pGEX-Acc, and the resulting ligation products were transformed in DH5α cells. Bacteria from individual colonies were induced to express the recombinant GST-fusion protein, and total protein was evaluated in Western blot assays using an anti-GST mAb to determine whether the fusion protein was expressed. Since the molecular weight of GST is 26-kDa, it was reasonable to assume that the bands identified by Western blotting that migrate at higher molecular weights were fusion proteins expressing the repetitive hβ-tubulin-C9 peptide.
A variety of fusion protein sizes were detected, as shown in . A single unit of 33-bp encodes 11 amino acids, and the molecular weight of one amino acid is 115-Da (Sambrook et al. Citation1989). Using this standard, we calculated the size of and number of ligated single units in the detected peptides. After multiple experiments were performed to evaluate the expression of the fusion protein in numerous colonies, we finally obtained seven different sizes of fusion proteins, ranging from 34-kDa to 63-kDa. From among these, we chose an abundant 40-kDa protein containing 11-unit repeats as the target peptide for use as an immunogen.
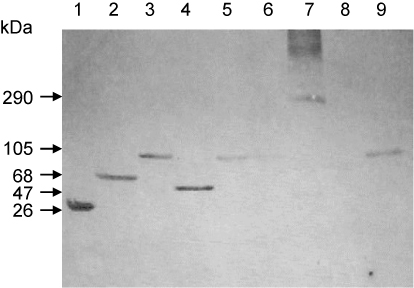
In order to confirm that the cloning of the repetitive oligonucleotide into the pGEX-Acc vector had occurred in the correct orientation and that the double-stranded target oligonucleotide was ligated in a head-to-tail orientation, DNA sequencing was performed using a pGEX primer. As expected, the single-unit target sequence of hβ-tubulin-C9, 5′-CGAG GAA GAA TCT GAA TCT CAG GGT CCG AAA GT-3′, was found repetitively (). Furthermore, the sequence just prior to the first repetitive oligonucleotide was 5′-TGCCTAG GTGCACCTT-3′, indicating that the insertion site for the repetitive oligonucleotide in pGEX-Acc was properly generated during pGEX-KT modification (data not shown).
Preparation of immunogen and generation of Abs
The fusion protein containing the 11-unit repeated peptide was purified using glutathione-agarose beads, and its purity was evaluated by SDS-PAGE. The bead-bound fusion protein was eluted five times with 5 mM reduced glutathione. Additionally, the bacterial supernatant remaining after the first treatment with beads was reincubated with glutathione-agarose beads. In total, 5 mg of fusion protein was obtained from a 15-ml bacterial culture. As shown in , we were able to confirm the purification of a highly pure fusion protein of 40-kDa by SDS-PAGE and Western blotting. Even though repetitive oligonucleotides with greater than 10-unit repeats were gel-isolated and cloned, the sizes of the expressed peptides did not always correspond well to the expected or predicted sizes. For example, 5-unit and 7-unit repeated peptides were detected in bacterial cultures transformed with an 11-unit repeated target sequence (data not shown). We postulate that an expressed peptide may be a target for digestion or that the repeated oligonucleotide may become modified in bacteria (Sørensen & Mortensen Citation2005). In order to retrieve the repeated peptide from the fusion protein, the fusion protein bound to glutathione-agarose beads was incubated with the thrombin protease for various periods of time. Ninety per cent of the 14-kDa repeated peptide was cleaved and released from the GST carrier after 4-hr incubation with thrombin ().
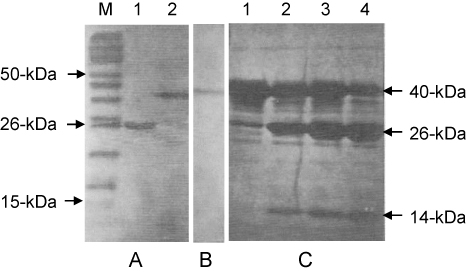
Hybridomas were produced through fusion of a myeloma cell line and mouse spleen cells immunised with either the GST-fusion protein or with the purified 11-unit repeated peptide. When the GST-fusion protein was used as an immunogen, the resulting hybridoma cell medium was evaluated by dot blotting, using simultaneous detection for the GST carrier and the fusion protein. Hybridomas showing a positive colour reaction to the fusion protein but not to the GST carrier were selected as positive cells.
We utilised a specific method throughout this study to produce pAbs in mice using the fusion protein. Pristane, 0.5 ml, was injected at the time of injection of the third boost of immunogen. Immunogen was injected without adjuvant 5 days later. Finally, myeloma cells were introduced into the intraperitoneum after an additional 5 days. As expected, mouse abdomens were enlarged and began to fill with ascetic fluid 15–20 days post-pristane injection. We were able to retrieved 5 ml of ascetic fluid from the mouse intraperitoneum at a time. The generated pAbs recognised a major 55-kDa of β-tubulin and some minor bands (). Although pAbs are generally produced in rabbits, we were able, using our method, to produce pAbs that were highly concentrated, cost-effective and required less effort. Once the ascetic fluid was harvested, we used spleen cells from the mice for fusion reactions with myeloma cells to produce mAbs.
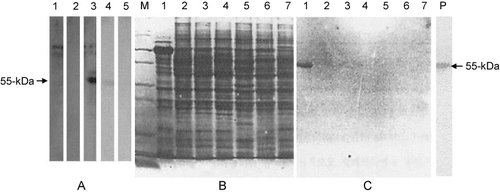
In order to test the pAbs and the mAbs generated in this study, Western blot analysis was performed with total protein extracted from human brain. Interestingly, a commercially purchased human β-tubulin pAb failed to recognise any proteins from human brain (, Lane 2). The commercially available mAb to human β-tubulin III bound most strongly to a ∼100-kDa protein, presumably corresponding to β-tubulin, than to the 55-kDa protein, as shown in Lane 1 of . When the generated pAb was used to probe total human brain protein, it strongly recognised the 55-kDa form of the β-tubulin protein, along with other minor proteins of around 100-kDa. Use of the generated mAbs resulted in detection of only a single protein band at 55-kDa as shown in Lanes 5 and 6 of . Together, these results suggest that pAb and mAbs against the nine amino acids repeated peptide derived from the human β-tubulin III C-terminus were successfully produced. Unfortunately, we were not able to confirm a previous report that the production rate of Ab is proportional to the length of the synthesised repetitive peptide (Welling & Fries Citation1985). In order to check the epitope specificity of the generated mAbs, we extracted total protein from various animal brains, including human, separated them by SDS-PAGE (), and performed Western blotting () with the generated mAb. As expected, the mAb reacted strongly with only the 55-kDa protein from human brain but not with proteins from other mammals. Even β-tubulin from the chicken brain, which contains only a single amino acid difference from that of human brain (Field et al. Citation1984), was not recognised by the mAb.
In conclusion, we produced an epitope-specific Ab using a repetitive peptide derived from nine C-terminal amino acids of the human brain β-tubulin III protein; a region of the protein known to be highly conserved between species. The duplexed synthetic oligonucleotides, which had six bases (5′-CGAC—GT-3′, 5′-TC—CAGC-3′) appended at the ends, were directly ligated in the presence of restriction enzymes (AccI, AvaI) in order to construct head-to-tail repeats. The gel-purified repetitive oligonucleotide was cloned into the AccI site of a modified pGEX vector, and this assembled pGEX-hβ-tubulin-C9 DNA was expressed as a GST-fusion protein in a bacterial system. This fusion protein or the 11-unit repetitive target peptide was purified by affinity chromatography and injected into Balb/c mice to obtain pAb and mAbs. The generated Abs specifically recognised the 55-kDa form of human brain β-tubulin, but not several other mammalian brain β-tubulins. The potential uses of this system could be applied to an effective epitope generation for antibody and to the development of a new type of subunit vaccine.
References
- Astori M, Kraehenbuhl JP. 1996. Recombinant fusion peptides containing single or multiple repeats of a ubiquitous T-helper epitope are highly immunogenic. Mol Immunol. 33:1017–1024.
- Banerjee A, Roach MC, Trcka P, Ludueña RF. 1990. Increased microtubule assembly in bovine brain tubulin lacking the type III isotype of beta-tubulin. J Biol Chem. 265:1794–1799.
- Billetta R, Hollingdale MR, Zanetti M. 1991. Immunogenicity of an engineered internal image antibody. Proc Natl Acad Sci USA. 88:4713–4717.10.1073/pnas.88.11.4713
- Bradford MM. 1976. A rapid and sensitive method for the quantitation of microgram quantities of protein utilizing the principle of protein-dye binding. Anal Biochem. 72:248–254.
- Carrol SB, Layghon A. 1987. Production and purification of polyclonal antibodies to the foreign segment of β-galactosidase fusion proteins. In: Glover DM, editor. DNA cloning: a practical approach. Oxford; Washington, DC: IRL Press; vol. 3: p. 89–111.
- Field DJ, Collins RA, Lee JC. 1984. Heterogeneity of vertebrate brain tubulins. Proc Natl Acad Sci USA. 8113:4041–4045.10.1073/pnas.81.13.4041
- Foged C. 2011. Subunit vaccines of the future: The need for safe, customized and optimized particulate delivery systems. Ther Deliv. 2:1057–1077.10.4155/tde.11.68
- Goding JW. 1986. Monoclonal antibodies: principles and practice. Orlando (FL): Academic Press; p. 59–93.
- Hakes DJ, Dixon JE. 1992. New vectors for high level expression of recombinant proteins in bacteria. Anal Biochem. 202:293–298.
- Harlow DL. 1988. Antibodies: a laboratory manual. New York: Cold Spring Harbor Laboratory; p. 72–87.
- Kolaskar AS, Tongaonkar PC. 1990. A semi-empirical method for prediction of antigenic determinants on protein antigens. FEBS Lett. 276:172–174.
- Laemmli UK. 1970. Cleavage of structural proteins during the assembly of the head of bacteriophage T4. Nature. 227:680–655.10.1038/227680a0
- Lang BF, Burger G. 1990. A rapid, high resolution DNA sequencing gel system. Anal Biochem. 188:176–180.
- Lerner RA. 1984. Antibodies of predetermined specificity in biology and medicine. Adv Immunol. 36:1–44.
- Lopata MA, Cleveland DW. 1987. In vivo microtubules are copolymers of available β-tubulin isotype: localization of each of six vertebrate β-tubulin isotypes using polyclonal antibodies elicited by synthetic peptide antigens. J Cell Biol. 105:1707–1720.10.1083/jcb.105.4.1707
- Patarroyo ME, Bermúdez A, Patarroyo MA. 2011. Structural and immunological principles leading to chemically synthesized, multiantigenic, multistage, minimal subunit-based vaccine development. Chem Rev. 111:3459–3507.
- Sambrook J, Fritsch EF, Maniatis T. 1989. Molecular cloning: a laboratory manual. New York: Cold Spring Harbor Laboratory.
- Schroeder U, Graff A, Buchmeier S, Rigler P, Silvan U, Tropel D, Jockusch BM, Aebi U, Burkhard P, Schoenenberger CA. 2009. Peptide nanoparticles serve as a powerful platform for the immunogenic display of poorly antigenic actin determinants. J Mol Biol. 386:1368–1381.10.1016/j.jmb.2008.11.023
- Smith DB, Johnson KS. 1988. Single-step purification of polypeptides in Escherichia coli as fusions with glutathione S-transferase. Gene. 67:31–40.10.1016/0378-1119(88)90005-4
- Sørensen HP, Mortensen KK. 2005. Soluble expression of recombinant proteins in the cytoplasm of Escherichia coli. Microb Cell Fact. 4:1.10.1186/1475-2859-4-1
- Sullivan KF, Cleveland DW. 1986. Identification of conserved isotype-defining variable region sequence for four vertebrate β-tubulin polypeptide classes. Proc Natl Acad Sci USA. 83:4327–4331.10.1073/pnas.83.12.4327
- Sutcliffe JG, Shinick N, Green FT, Liu HL, Lerner RA. 1980. Chemical synthesis of a polypeptide predicted from nucleotide sequence allows detection of a new retroviral gene product. Nature. 287:801–805.10.1038/287801a0
- Towbin H, Staehelin T, Gordon J. 1979. Electrophoretic transfer of proteins from polyacrylamide gels to nitrocellulose sheets: procedure and some applications. Proc Natl Acad Sci USA. 76:4350–4354.10.1073/pnas.76.9.4350
- Walter G. 1986. Production and use of antibodies against synthetic peptides. J Immunol Methods. 88:149–161.10.1016/0022-1759(86)90001-3
- Welling GW, Fries H. 1985. Choice of peptide and peptide length for the generation of antibodies reactive with the intact protein. FEBS Lett. 182:81–84.10.1016/0014-5793(85)81158-3
- Welling GW, Weije WJ, Zee R, Welling-Wester S. 1985. Prediction of sequential antigenic regions in proteins. FEBS Lett. 188:215–218.10.1016/0014-5793(85)80374-4
- Yankai Z, Rong Y, Yi H, Wentao L, Rongyue C, Ming Y, Taiming L, Jingjing L, Jie W. 2006. Ten tandem repeats of beta-hCG 109–118 enhance immunogenicity and anti-tumor effects of beta-hCG C-terminal peptide carried by mycobacterial heat-shock protein HSP65. Biochem Biophys Res Commun. 345:1365–1371.10.1016/j.bbrc.2006.05.022
- Yu T, Bai Y, Dierich MP, Chen YH. 2000. Induction of high levels of epitope-specific antibodies by epitope/peptide candidate vaccines against human immunodeficiency virus type-1 (HIV-1). Microbiol Immunol. 44:105–110.