Abstract
Treatment options for peripheral neuropathy are very limited. In order to develop a therapeutic strategy using stem cell therapy for the peripheral nervous system, we explored the feasibility of a new delivery method. Using ultrasonography-guided transplantation, human mesenchymal stem cells (hMSCs) were delivered into the perineural region of small rodent models. An optical imaging system revealed that hMSCs stained with CFSE or PKH26 reside for a week post-transplantation in both the mouse and rat. Immunofluorescence analysis of tissues revealed the presence of transplanted hMSCs in the perineural region of mice. At the end of the experiments, no behavioral or phenotypic abnormalities were observed. In addition, H&E and toluidine blue staining showed the integrity of the sciatic nerve after transplantation. This is the first study, to the best of our knowledge, to deliver cells into the perineural region under ultrasonography guidance. Since hMSCs can reside for a considerable time at a transplanted site, an anti-inflammatory efficacy of the transplanted hMSCs can be expected in the perineural region. In conclusion, we established a new administration route for inflammatory peripheral neuropathy without complications, and this may be used for delivery of chemical drugs or stem cells.
Introduction
Inflammatory neuropathy occurs as a result of various conditions such as autoimmunity and infection (Newsom-Davis Citation1988). Along with disease progression, the immune system attacks the nerves, causing sensory symptoms such as burning, tingling or numbness, or motor symptoms such as muscle weakness. Although the treatment options include corticosteroids or intravenous immunoglobulins (IVIg) for acute inflammatory demyelinating polyradiculoneuropathy (AIDP) and chronic inflammatory demyelinating polyneuropathy (CIDP), further significant evidence of efficacy is required (Hughes et al. Citation2007; Bright et al. Citation2014) due to the heterogeneity of the disease pathogenesis.
Treatment of disease with stem cells is currently widely used. In particular, mesenchymal stem cells (MSCs) have been extensively researched for the treatment of various disorders, including immunological, neurological, and cardiovascular diseases (http://clinicaltrials.gov). Their potencies are well known for paracrine effects such as anti-inflammation and anti-apoptotic effects (Meirelles et al. Citation2009; Li et al. Citation2010; Murphy et al. Citation2013). The delivery route for MSCs is usually a vein, which is the easiest way to transplant. However, the majority of the cells are entrapped within the lung (Gao et al. Citation2001; Fisher et al. Citation2009). Conversely, target organ-specific delivery has been attempted for several diseases, especially neurological diseases with better cellular retention (Hayek et al. Citation2011; Larsen et al. Citation2014).
Since peripheral neuropathy is caused by defects in neurons or Schwann cells, targeted approaches for treatment are required. In this context, application of recently advanced stem cell technology using a target-specific route may be an alternative therapeutic option. In this report, we develop and evaluate a new delivery route using ultrasonography-guided stem cell transplantation.
Materials and methods
Preparation of human MSCs
Human adipose MSCs (hMSC; Lonza, Walkersville, MD) were cultured in Dulbecco's modified Eagle medium containing 10% fetal bovine serum and penicillin/streptomycin (Life Technologies, Grand Island, NY). Before transplantation, hMSCs were labeled with CFSE (Invitrogen, Carlsbad, CA) or PKH26 (Sigma, St. Louis, MO) for 30 min, according to the manufacturer's protocol. Briefly, cells were treated with 2.5 μM CFSE or 2 μM PKH26 for 5 min, then washed with PBS by centrifugation. The cells were subsequently resuspended in 1 mg/ml Matrigel (Sigma) for transplantation.
Ultrasonography-guided perineural transplantation
Ultrasonography-guided perineural transplantation was performed in 8-week-old female BL/6J mice and Sprague–Dawley rats. The mice and rats were anesthetized with CO2 gas and maintained with 1.5% isoflurane using a nose cone for the duration of the procedure. The fur over the hind limb was clipped with an animal clipper, and the skin was rinsed with 70% ethanol. A Vevo 2100 (Visual Sonics, Toronto, Canada) micro-ultrasound machine with a 55 MHz probe was used to image the transverse plane of the sciatic nerve in the proximal hind limb. A 31G needle was inserted into the thigh and the tip was positioned near the sciatic nerve. A total 3 × 105 labeled hMSCs in 0.1 ml PBS/Matrigel were transplanted at a single point. All experiments were conducted according to protocols approved by the Institutional Animal Care and Use Committee of the Samsung Medical Center.
Optical imaging
To determine the transplantation of hMSCs, optical imaging was performed using a Xenogen IVIS Spectrum system (Caliper Life Science, Hopkinton, MA) with a Hamamatsu charge-coupled device (CCD) camera. Fluorescence was detected using 500/560 nm (excitation/emission) and 500/580 nm filters for CFSE and PKH26, respectively. The background signals were calibrated using the 430 nm filter. Identical illumination settings were used for each fluorescence image, and fluorescence emission was normalized to photons per second per centimeter squared per steradian (p/s/cm2/sr). Images were acquired and analyzed using the Living Image 3.2 software (Caliper Life Science).
Histology
To detect hMSCs stained with CFSE and PKH26 fluorescence, sample tissues taken from the mouse hind limb were fixed in 4% paraformaldehyde in PBS after removal of the femoral bone and incubation with 30% sucrose. Subsequently, the frozen sample was cut into 6 μm sections on a sliding microtome. The fluorescent cells were detected using a fluorescence microscope (Olympus BX-51-FL, Olympus, Tokyo, Japan). Toluidine blue and H&E staining were performed for assessment of damage and inflammatory response in the nerve and perineural region.
Results
Ultrasonography-guided perineural transplantation of hMSCs
To detect the sciatic nerve in the mouse and rat, ultrasonography was performed. After anesthesia, the hind limb of the mouse and rat were scanned from proximal to distal ((a) and 1(b)), and the sciatic nerve could be identified as a circular structure detected in the biceps femoris muscle at the mid-thigh level of the mouse ((c)) and the rat ((e)). Subsequently, ultrasonography-guided transplantation of stem cells was performed. Human adipose MSCs (hMSCs, 3 × 105), labeled with either CFSE or PKH26, were transplanted into the perineural region of the mouse ((d)) and the rat ((d)). The transplanted hMSCs could be detected in the perineural region by ultrasonography images.
Figure 1. Ultrasonography-guided transplantation of hMSCs. (a and b) Apparatus and image of ultrasonography-guided transplantation of hMSCs. (c–f) Detection of the sciatic nerve (arrow) in the mouse (c) and the rat, (e) and hMSCs transplanted into perineural region (dotted line) in the mouse (d) and the rat (f).
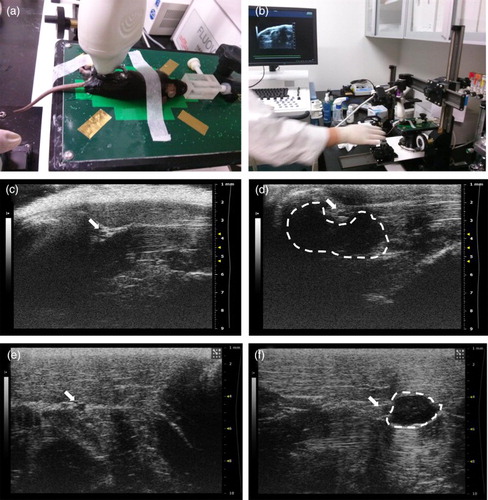
Fluorescence optical imaging visualized the transplanted hMSCs
The transplanted hMSCs were immediately visualized by optical imaging. Using fluorescence detection, CFSE- ((a)) or PKH26- ((d)) labeled cells in the mouse and rat sciatic nerve (data not shown) could be detected in the thigh region. Background signal was also detected in the mouth and tail region. To determine the long-term survival of the transplanted hMSCs in vivo, the mice were housed, and the detection of transplanted hMSCs was followed. Three and 7 days post-transplantation, hMSCs labeled with either CFSE ((b) and 2(c)) or PKH26 ((e) and 2(f)) could be detected, and their remaining fluorescence was 47% and 82%, respectively, of the initial transplantation at 7 days post-transplantation.
Transplanted hMSCs were detected in perineural tissue
To further determine whether the transplanted cells reside in the perineural region, immunofluorescence detection was performed. Seven days post-transplantation, the mice were euthanized and thigh samples collected for histology. Immunofluorescence microscopy could detect the CFSE-labeled hMSCs around the sciatic nerve ((a)). Since the hMSCs were mixed with Matrigel, the hMSCs appeared in an aggregated form. To investigate the side effects of hMSC transplantation, we observed the behavioral and biochemical abnormalities. However, there was no detectable aberrant behavior after transplantation. In addition, there was no deterioration of the integrity of the sciatic nerve, which was determined by toluidine blue (data not shown) or H&E staining, although there were some migrated inflammatory cells ((b)).
Figure 3. Detection of transplanted hMSCs in the tissue. (a) Immunofluorescence analysis using CFSE and DAPI was performed to detect the residual hMSCs in the perineural region of the mouse thigh after 7 days. Dotted line indicates the sciatic nerve. (b) H&E staining was performed to detect damage in the transplanted tissues (100×).
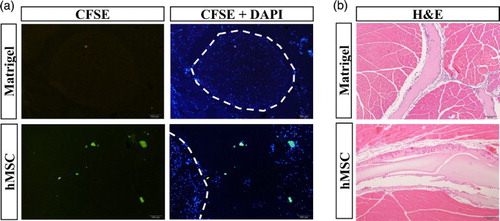
Discussion
Utilization of stem cells has been attempted in inflammatory neuropathy such as CIDP and autoimmune diseases such as myasthenia gravis (MG). Clinically, administration of autologous peripheral blood stem cell have been tried; however, the efficacy could not be sufficiently assessed due to the limited number of patients and the lack of a control (Press et al. Citation2014; Mahdi-Rogers et al. Citation2009). Conversely, human bone-marrow-derived MSCs have been intravenously injected into mouse models of experimental autoimmune myasthenia gravis, and exhibited potential therapeutic efficacy (Yu et al. Citation2010). These data indirectly indicate that the effectiveness of MSCs is better than that of blood stem cells. A direct comparison of immune-modulating efficacy between MSCs and hematopoietic stem cells (HSCs) in an animal model of liver injury demonstrated that MSCs are more competent than HSCs in alleviating the immunoreaction-related fibrosis in the liver (Pulavendran et al. Citation2010). Thus, transplantation of MSCs should be considered as a major treatment option for inflammatory neuropathy.
Currently, ultrasonography has been widely used in small rodent models. However, application to the peripheral nerve is limited, although ultrasonography-guided nerve stimulation is frequently used for regional anesthesia (Wadhwa et al. Citation2011). Recently, ultrasonography-guided injection has facilitated the safe delivery into the perineural region (Belavy et al. Citation2013), demonstrating the safety of ultrasonography gels and needle injection. There was a minimal inflammatory response and no damage to the nerve. Therefore, administration of therapeutic agents is achievable using ultrasonography in the peripheral nerve.
This is the first study, to the best of our knowledge, to deliver cells into the perineural region under ultrasonography guidance. The detection of the sciatic nerve in both the mouse and rat could be achieved within 10 min, and there was no impediment in transplantation of hMSCs using a 31G needle. Since hMSCs could reside for a considerable time at the transplanted site, the delivered stem cells may exert therapeutic efficacy for the perineural region in inflammation. In addition, there was minimal complications in the transplantation of hMSCs. In conclusion, stem cell therapy for inflammatory peripheral neuropathy may be feasible using ultrasonography-guided transplantation.
Disclosure
No potential conflict of interest was reported by the authors.
Additional information
Funding
References
- Belavy D, Sunn N, Lau Q, Robertson T. 2013. Absence of neurotoxicity with perineural injection of ultrasound gels: assessment using an animal model. BMC Anesthesiol. 13:18. doi:10.1186/1471-2253-13-18
- Bright RJ, Wilkinson J, Coventry BJ. 2014. Therapeutic options for chronic inflammatory demyelinating polyradiculoneuropathy: a systematic review. BMC Neurol. 14:26. doi:10.1186/1471-2377-14-26
- Fischer UM, Harting MT, Jimenez F, Monzon-Posadas WO, Xue H, Savitz SI, Laine GA, Cox CS Jr. 2009. Pulmonary passage is a major obstacle for intravenous stem cell delivery: the pulmonary first-pass effect. Stem Cells Dev. 18:683–692. doi: 10.1089/scd.2008.0253
- Gao, J, Dennis JE, Muzic RF, Lundberg M, Caplan AI. 2001. The dynamic in vivo distribution of bone marrow-derived mesenchymal stem cells after infusion. Cells Tissues Organs. 169:12–20. doi: 10.1159/000047856
- Hayek SM, Deer TR, Pope JE, Panchal SJ, Patel VB. 2011. Intrathecal therapy for cancer and non-cancer pain. Pain Physician. 14:219–248.
- Hughes RA, Swan AV, Raphaël JC, Annane D, van Koningsveld R, van Doorn PA. 2007. Immunotherapy for Guillain–Barré syndrome: a systematic review. Brain. 130:2245–2257. doi: 10.1093/brain/awm004
- Larsen JM, Martin DR, Byrne ME. 2014. Recent advances in delivery through the blood–brain barrier. Curr Top Med Chem. 14:1148–1160. doi: 10.2174/1568026614666140329230311
- Li N, Sarojini H, An J, Wang E. 2010. Prosaposin in the secretome of marrow stroma-derived neural progenitor cells protects neural cells from apoptotic death. J Neurochem. 112:1527–1538. doi: 10.1111/j.1471-4159.2009.06565.x
- Mahdi-Rogers M, Kazmi M, Ferner R, Hughes RA, Renaud S, Steck AJ, Fuhr P, Halter J, Gratwohl A, Tyndall A. 2009. Autologous peripheral blood stem cell transplantation for chronic acquired demyelinating neuropathy. J Peripher Nerv Syst. 14:118–124. doi: 10.1111/j.1529-8027.2009.00221.x
- Meirelles Lda S, Fontes AM, Covas DT, Caplan AI. 2009. Mechanisms involved in the therapeutic properties of mesenchymal stem cells. Cytokine Growth Factor Rev. 20:419–427. doi: 10.1016/j.cytogfr.2009.10.002
- Murphy MB, Moncivais K, Caplan AI. 2013. Mesenchymal stem cells: environmentally responsive therapeutics for regenerative medicine. Exp Mol Med. 45:e54. doi:10.1038/emm.2013.94
- Newsom-Davis J. 1988. Autoimmunity in neuromuscular disease. Ann N Y Acad Sci. 540:25–38. doi: 10.1111/j.1749-6632.1988.tb27048.x
- Press R, Askmark H, Svenningsson A, Andersen O, Axelson HW, Strömberg U, Wahlin A, Isaksson C, Johansson JE, Hägglund H. 2014. Autologous haematopoietic stem cell transplantation: a viable treatment option for CIDP. J Neurol Neurosurg Psychiatry. 85:618–624. doi: 10.1136/jnnp-2013-306014
- Pulavendran S, Vignesh J, Rose C. 2010. Differential anti-inflammatory and anti-fibrotic activity of transplanted mesenchymal vs. hematopoietic stem cells in carbon tetrachloride-induced liver injury in mice. Int Immunopharmacol. 10:513–519. doi: 10.1016/j.intimp.2010.01.014
- Wadhwa A, Kandadai SK, Tongpresert S, Obal D, Gebhard RE. 2011. Ultrasound guidance for deep peripheral nerve blocks: a brief review. Anesthesiol Res Pract. 2011:262070. doi:10.1155/2011/262070
- Yu J, Zheng C, Ren X, Li J, Liu M, Zhang L, Liang L, Du W, Han ZC. 2010. Intravenous administration of bone marrow mesenchymal stem cells benefits experimental autoimmune myasthenia gravis mice through an immunomodulatory action. Scand J Immunol. 72:242–249. doi: 10.1111/j.1365-3083.2010.02445.x