ABSTRACT
Enterococcus faecium HDRsEf1 strain used as a probiotic to inhibit intestine inflammation and improve animal growth performance has been proved by our research team; however, it remains unclear how HDRsEf1 was recognized by intestine cells and how it activates the downstream pathway which benefit intestine health. In this study, HDRsEf1 was used to stimulate HT-29 cell line to partially uncover the intestine benefit mechanism of HDRsEf1. The results of cell viability assays showed that HDRsEf1 had no toxicity on HT-29 at concentrations up to 1 × 108 CFU/mL, HDRsEf1 could upregulate the TLR1, TLR2, and TLR6 mRNA level, especially TLR2, and significantly downregulate the mRNA level of TLR4, TLR5, TLR7, TLR8, but did not significantly affect the mRNA or protein level of MyD88, which suggests that HDRsEf1 activates the TLR2 pathway in an MyD88-independent pattern. HDRsEf1 could significantly downregulate the mRNA level of pro-inflammatory factors IL-1β, IL-6, IL-8, IL-12p35, IL-17, and TNF-α and did not affect the anti-inflammatory factors IL-10, PPAR-γ, and TSLP; besides HDRsEf1 did not upregulate the degradation of IκB in HT-29 cells. By contrast, enterohemorrhagic E. coli (EHEC) O157:H7 strongly up-regulated the mRNA level of pro-inflammatory factors IL-1β, IL-6, IL-8, IL-23, and TNF-α, downregulated obviously anti-inflammatory factor PPAR-ɣ, and obviously upregulated the degradation of IκB, which suggested that HDRsEf1 may act as an antagonist to regulate intestine inflammation response to intestine pathogen. These findings shed a light on the intestine benefit mechanism of HDRsEf1.
1. Introduction
Probiotics is a kind of living microorganism which confers a great health benefit on the consumer when administered in adequate amounts (Parvez et al. Citation2006). The earliest research on probiotics dates back to 1907, when Metchnikoff claimed that the people administrated lactic acid-producing bacteria in yoghurt resulting in balance of intestinal micro-flora and longevity (Metchnikoff Citation1987; Bron et al. Citation2012). It has been reported that administrated infant bifidobacteria could become the dominant of the intestinal micro-flora, reduce the putrefactive bacteria, and improve the immunity of the host (Tojo et al. Citation2014). Probiotics has a great effect on the absorption of nutrition, the innate and acquired immunity, and many other activities (Akira Citation2006). Animal experiments also proved that probiotics can protect animals from experimental colitis (Mazmanian et al. Citation2008). Therefore, the application of probiotics or their component against animal gastrointestinal diseases has a broad prospective.
E. faecium has already been used successfully in human for the treatment of acute diarrheal diseases and prevention of antibiotic-associated diarrhea (Wunderlich et al. Citation1989; Buydens & Debeuckelaere Citation1996). The probiotic E. faecium HDRsEf1 strain which was separated by our research group has been licensed a China nation patent (Shi et al. Citation2013) and already used as a feed additive for chicken and piglets; the feeding results demonstrate that HDRsEf1 can reduce diarrhea incidence and severity in weaning piglets and regulate the intestinal flora in chicken (Xiao et al. Citation2014; Gong et al. Citation2015), but the mechanism of intestine benefit remains unknown.
The interaction between intestinal epithelial cells and microorganisms is a complex process (Sherman et al. Citation2009; Di Mauro et al. Citation2013). A toll-like receptor (TLR) family on intestinal epithelial cells plays an important role in recognizing the intestine foreigners, which subsequently initiates the inflammation response (Akira et al. Citation2006; Kawai & Akira Citation2009; O’Neill et al. Citation2009; Lavelle et al. Citation2010). MyD88 is the main adaptor molecule of TLRs; most of signal transduction of TLRs needs MyD88 to activate the downstream molecules, and finally leads nuclear factors such as NF-κB enter into nuclear and regulate inflammatory cytokines expression. Some research results proved that some probiotics could regulate IECs to avoid an acute inflammatory response to intestinal pathogen by expressing a variety of anti-inflammatory cytokines (Artis Citation2008). In this study, the HDRsEf1 intestine benefit mechanism was explored in vitro by using enterocyte-like HT-29 to mimic IEC. This study focuses on whether HDRsEf1 can regulate TLRs, NF-κB, pro-inflammatory cytokines, and anti-inflammatory cytokines, and EHEC O157:H7 was used as an intestine pathogen representative based on the fact that it is a common human and animal pathogenic bacterium and it can cause severe hemolytic-uremic syndrome (Kanamaru et al. Citation2000).
2. Materials and methods
2.1. Bacteria strains and culture conditions
HDRsEf1 was isolated and identified by the Department of Veterinary Microorganism & Immunity, Huazhong Agricultural University (Xiong Citation2010). HDRsEf1 was cultivated in MRS (Qingdao Hope Bio-Technology Co., Ltd., China). enterohemorrhagic E. coli (EHEC) O157:H7 was kindly provided by Shaowen Li (Huazhong Agricultural University, China) , O157:H7 was cultivated in tryptic soy broth (TSB; Becton, Dickinson and Company, San Jose, CA). It was incubated overnight at 37°C, and subcultures of bacteria were grown for 3–4 h until mid-log phase and then centrifuged. Cell pellets were washed thrice in phosphate-buffered saline. The bacteria were re-suspended in antibiotic-free DMEM medium at 1 × 108 CFU/mL.
2.2. Cell culture
Human colonic epithelial cells HT-29 were purchased from cell bank of type culture collection of Chinese Academy of Sciences (Shanghai Institute of Cell Biology of Chinese Academy of Sciences). HT-29 cells were cultured in Dulbecco’s modified Eagle’s medium (DMEM; HyClone, Laboratories Inc., Logan, UT, USA) supplemented with 10% heat-inactivated fetal bovine serum (gibco, Australia), 100 U mL–1 penicillin, and 100 μg mL–1 streptomycin at 37°C in a humidified atmosphere of 5% CO2 for 48 h.
2.3. Assessment of cellular toxicity of HDRsEf1
MTT[3-(4,5-Dimethylthiazol-2-yl)-2,5-diphenyl tetrazolium bromide] (Sigma, Switzerland) was used to assess cellular toxicity of HDRsEf1. Different concentrations of HDRsEf1 ranging from 1 × 104 CFU/mL to 1 × 109 CFU/mL were added to HT-29 cell monolayers in a 96-well plate (Nest Biotech co., Ltd) for 3 h. At the end of the incubation period, the MTT viability assay was carried out as described previously (Meshitsuka et al. Citation1987; Chai et al. Citation2013). The cell survival rate was determined as bacteria average OD value/control average OD value, the non-cytotoxic maximal concentration of HDRsEf1 was selected for continuous assays.
2.4. Stimulation of cells
HT-29 cells (1 × 106 cells well–1) were allowed to attach and grow in 6-well culture plates (Corning, USA) for 48 h, then HT-29 cells were washed with sterile PBS (pH 6.7) thrice. The cells were incubated with O157:H7 (1 × 108 CFU/mL) and HDRsEf1 (1 × 108 CFU/mL) for 3 h, respectively.
2.5. Extraction of total RNA and synthesis of cDNA
After 3 h incubation with O157:H7 (1 × 108 CFU/mL) and HDRsEf1 (1 × 108 CFU/mL) respectively, HT-29 cells were harvested and washed with ice-cold PBS (pH 6.7) for three times. Total RNA from HT-29 cells was extracted with an RNATM.iso PLUS Kit (Takara Biotechnology, Dalian, China). Reverse transcription (RT) was performed using a RevertAidTM First Strand cDNA Synthesis Kit (Fermentas, Thermo Fischer Scientific, USA) according to the manufacturer’s instruction.
2.6. Quantitative real-time PCR
The mRNA level of cytokines (IL-1β, IL-6, IL-8, IL-10, IL-12p35, IL-17, IL-23, TNF-a, IFN-γ, PPAR-γ, TSLP), toll-like receptors (TLR-1, -2, -3, -4, -6, -8, -9), and MyD88 were analyzed by quantitative real-time PCR (qRT-PCR),which was performed using SYBR Premix EXTaqTM (Takara Biotechnology, Dalian, China). The primer sequences are given in . The data represent mean values obtained in three independent experiments.
Table 1. Detailed primers used for qRT-PCR.
2.7. Western blot
The expression of IκB and MyD88 was analyzed by Western blot according to the previous method (Wang et al. Citation2009).
3. Results
3.1. Cytotoxicity of HDRsEf1 for HT-29 cells
First, the non-toxic concentration on HDRsEf1 for HT-29 was defined by the MTT assay. The results () showed that HDRsEf1 was non-toxic at concentrations up to 1 × 108 CFU/mL, the viability rate of HT-29 cells was 100%, and no morphological differences were observed at this concentration. Therefore, 1 × 108 CFU/mL of HDRsEf1 was chosen for the following experiments.
Figure 1. Effect of HDRsEf1 on the viability of HT-29 cells. HDRsEf1 was added to 80% confluent cells in a 96-well plate, which were then incubated at 37°C for 3 h. Cell viability was tested by the MTT assay. The cell survival rate was determined by comparing the optical density values from HDRsEf1-treated cells with those from non-treated cells. The cell survival rates at different concentrations of HDRsEf1 are given. Results represent means ± standard deviations from three independent experiments. The presence of various asterisks (*, **, and ***) indicates statistical differences with significant levels of p < .05, p < .01, and p < .001, respectively.
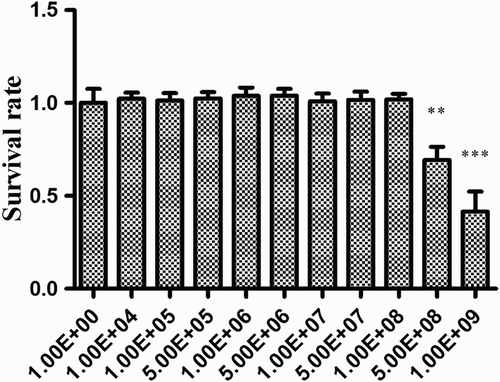
3.2. Cytokine mRNA level in HT-29 stimulated by HDRsEf1 and O157:H7
Inflammatory response of HT-29 cells to HDRsEf1 and O157:H7 was evaluated by the measurement of cytokines by qRT-PCR. When HT-29 cells were stimulated by HDRsEf1 (1 × 108 CFU/mL) and O157:H7 (1 × 108 CFU/mL) for 3 h respectively, HDRsEf1 obviously downregulated the mRNA level of IL-1β, IL-6, IL-8, IL-12p35, IL-17, and TNF-ɑ, and had no effect on IL-10, IL-23,TSLP, PPAR-γ, and IFN-γ after while O157:H7 could powerfully upregulated pro-inflammatory cytokines IL-1β, IL-6, IL-8, IL-23, and TNF-ɑ. The mRNA level of IL-10, IL-12p35, IL-17, TSLP, and IFN-γ was similar to HDRsEf1, moreover, O157:H7 could downregulate cytokines PPAR-γ ().
Figure 2. mRNA of cytokines in HT-29 cells stimulated by HDRsEf1(1 × 108CFU/mL) and O157:H7(1 × 108CFU/mL), respectively, for 3 h. 80% confluence HT-29 cells were incubated with these stimuli for 3 h, then mRNA of cytokines was determined by qRT-PCR. (a) The mRNA level of pro-inflammatory factors. (b) The mRNA level of anti-inflammatory factors. Results represent means ± standard deviations from three independent experiments. The presence of various asterisks (*, **, and ***) indicates statistical differences with significant levels of p <.05, p < .01, and p < .001, respectively.
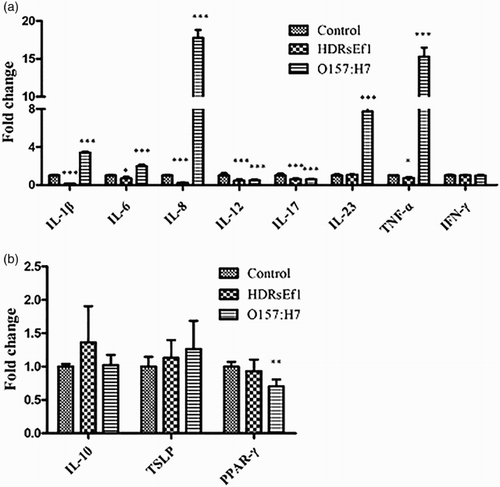
3.3. The degradation of IκB in HT-29 stimulated by HDRsEf1 and O157:H7
HDRsEf1 (1 × 108 CFU/mL) and O157:H7 (1 × 108 CFU/mL) were used to stimulate HT-29 cells for 3 h, respectively. The results showed that HDRsEf1 could not cause the degradation of IκB, while O157:H7 could cause the obvious degradation of IκB (), which suggested that HDRsEf1 and O157:H7 may play different roles in the intestine inflammatory response.
Figure 3. IκB in HT-29 cells stimulated by HDRsEf1(1 × 108CFU/mL) and O157:H7 (1 × 108CFU/mL), respectively, for 3 h. HT-29 cells were incubated with these stimuli respectively, 3 h later the protein level of IκB was determined by Western blot. Intensities of proteins bands were calculated from the peak area of densitograms by using an image software. Results represent means ± standard deviations from three independent experiments. The presence of various asterisks (**) indicates statistical differences with significant levels of .001 < p < .01.
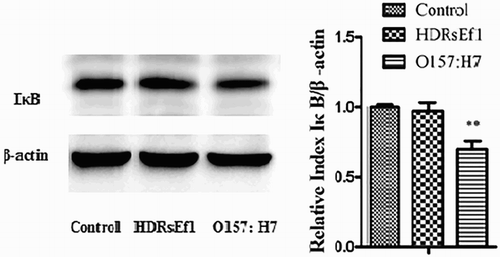
3.4. The mRNA level of TLRs in HT-29 stimulated by HDRsEf1
To determine whether HDRsEf1 could influence TLR expression of HT-29 cells, HT-29 cells were treated with 1 × 108 CFU/mL HDRsEf1 in DMEM or DMEM-alone as control. The mRNA level of TLR1, TLR2, and TLR6 was increased, especially TLR2, while that of TLR4, TLR5, TLR7, and TLR8 was decreased obviously by HDRsEf1 stimulation, the mRNA level of TLR3 and TLR9 has no obvious response to the stimulation ().
Figure 4. mRNA of TLRs in HT-29 stimulated by HDRsEf1(1 × 108CFU/mL) for 3 h. 80% confluence HT-29 cells were incubated with HDRsEf1 for 3 h, then mRNA of TLRs was determined by qRT-PCR. Results represent means ± standard deviations from three independent experiments. The presence of various asterisks (*, **, and ***) indicates statistical differences with significant levels of p < .05, p < .01, and p < .001, respectively.
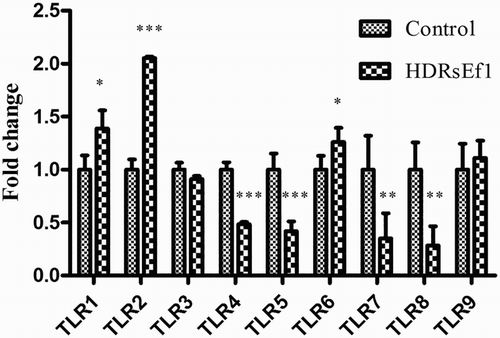
3.5. The mRNA and protein levels of MyD88 in HT-29 stimulated by HDRsEf1
The activation of TLRs may lead to NF-kB activation in either MyD88-dependent or MyD88-independent manner (Fischer et al. Citation2008). In order to determine whether HDRsEf1 regulates the inflammatory response through MyD88, 1 × 108 CFU/mL of HDRsEf1 in DMEM was used to stimulate HT-29 cells, mRNA and protein levels of MyD88 from HT-29 cells were tested by qRT-PCR and Western blot, respectively. The results showed that MyD88 was not upregulated in the transcriptional level or translational level by HDRsEf1 ().
Figure 5. mRNA and protein levels of MyD88 in HT-29 cells stimulated by HDRsEf1 (1 × 108 CFU/mL) for 3 h. 80% confluence HT-29 cells were incubated with HDRsEf1, (a) the mRNA level of MyD88 was determined by qRT-PCR, (b) the protein level of MyD88 was determined by Western blot. Intensities of proteins bands were calculated from the peak area of densitograms by using an image software. Results represent means ± standard deviations from three independent experiments.
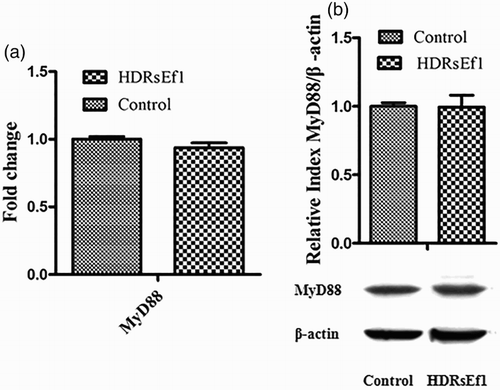
4. Discussion
The aim of this study was to elucidate the inflammation regulatory mechanism of HDRsEf1 on intestinal epithelium, and to compare the cytokine profile after stimulation by HDRsEf1 and O157:H7. By comparing the cytokine profile, clarify whether HDRsEf1 could be an antagonist to regulate intestine inflammation response to intestine pathogen.
In addition to providing physical barrier, intestinal epithelial cells are able to express mediators of inflammation, such as cytokines, chemokines, and other signal molecules, such as TLRs (Arce et al. Citation2010; Kajikawa et al. Citation2011). TLRs are a component of the innate immune system, which can recognize the invading microbes and initiate non-specific immune response (Roger et al. Citation2010). Cammarota et al. (Citation2009) found that Lactobacillus casei upregulated the expression of TLR1, 2, 3, 4, and 6 in Caco-2 cells, while Lactobacillus plantarum downregulated all these TLRs except TLR2, but fairly insignificant either way. Our study showed that when HT-29 cells were stimulated by HDRsEf1 for 3 h, the mRNA level of TLR1, TLR2, and TLR6 increased, especially TLR2, the mRNA level of TLR4, TLR5, TLR7, and TLR8 decreased obviously in HT-29 cells.
TLR2, TLR1, and TLR6 can form heterodimer TLR1/TLR2 or TLR6/TLR2, and identify bacteria and bacterial metabolites (Takeuchi et al. Citation2002; Kawai & Akira Citation2011). In this study, TLR2 together with TLR1and TLR6 was upregulated by HDRsEf1, which suggests that HDRsEf1 probably be recognized by heterodimer TLR1/TLR2 or TLR6/TLR2, to further activate these pathways.
Probiotic could regulate TLRs and the negative regulators of TLRs (Villena & Kitazawa Citation2013), so we think that the decreased mRNA level of TLR4, TLR5, TLR7, and TLR8 after stimulated with HDRsEf1 might relate to the increasing TLR’s negative regulators. In our later study, we found that HDRsEf1 could regulate several negative regulators of TLR, and TLR2 plays an important role (data not shown).
MyD88 is a ubiquitous linking protein. It can bond to the C-terminal area of TLR and induce a series of phosphorylation reactions which can be resulted in nuclear transfer of NF-κB and pro-inflammatory factor expression. Almost all TLRs can deliver signal to MyD88. But recent research has found that TLR2, TLR3, and TLR4 can be independent of MyD88 to transfer signal (Fischer et al. Citation2008; Gao et al. Citation2012). In this investigation, HDRsEf1 was not able to increase the MyD88 in mRNA and protein levels, which suggests that HDRsEf1 activates inflammatory response independently of MyD88, which should be confirmed by further study.
The activation of NF-κB is triggered by microorganisms, which results in degradation of IκB and NF-κB translocation into the nucleus to activate the transcription of inflammatory genes (Medvedev et al. Citation2000; O’Neill & Bowie Citation2007; Zeytun et al. Citation2010; Haddad & Abdel-Karim Citation2011). Our data showed that the degradation of the IκB in HT-29 cells induced by HDRsEf1 was not obvious, but it was obvious by O157:H7. HDRsEf1 was similar to Lactobacillus amylovorus which can block the phosphorylation of IKK complex, IκBɑ, and p65 (Finamore et al. Citation2014).
Plenty of bacteria in the gut of a normal host could stimulate the innate immune system and then trigger physiological inflammation through the membrane-bound or soluble factors, including cytokines (IL-10, TGF-β1,TNF-α, IL-1β, IL-6, IL-12 and IFN-γ) and chemokines (IL-8 and MCP-1) (Clavel & Haller Citation2007). Lactic acid bacteria (LAB) could augment the production of pro-inflammatory cytokines, such as IL-1β, IL-6, IL-12, TNF-α and IFN-γ, thereby triggering physiological inflammation. Several LAB strains could induce the secretion of TNF-α by dendritic cells and peripheral blood mononuclear cells and increase the number of TNF-α-producing cells in the gut lamina propria (de Moreno et al. Citation2008; Zoumpopoulou et al. Citation2008). But in our study, HDRsEf1 could decrease the expression of IL-1β, IL-6, IL-12p35, and TNF-ɑ and could not affect the expression of IFN-γ in HT-29 cells, which indicates that HDRsEf1 enables animal intestinal immunity in low levels of inflammation. Commensal probiotics inhibits the IL-23/IL-17 axis in an in-vitro model of the intestinal mucosal immune system (Ghadimi et al. Citation2012). This is in agreement with our study, although HDRsEf1 only reduces the expression of IL-17 and had no effect on IL-23. IL-8 governs the progress of most local bowel inflammations. It attracts and directs neutrophils to the site of inflammation, an instant response that is triggered to eliminate the pathogen, which may result in severe inflammatory response. Enterococcus faecalis from healthy infants decrease the secretion of IL-8, thus relieve intestinal epithelial cells of excessive inflammatory response caused by the inflammatory mediator (Wang et al. Citation2014). Our study shows that HDRsEf1 could remarkably decrease the expression of IL-8 in HT-29 cells; this may be one of important beneficial properties of HDRsEf1 strain. The Enterococcus faecalis separated from Children feces could regulate the activity of endogenous PPAR-γ and the expression of IL-10 that regulate the balance of intestinal tract (Are et al. Citation2008). TSLP is a cytokines that released constitutively by ECs, which can help maintain the homeostasis of the gut by generating a non-inflammatory environment (Rimoldi et al. Citation2005). However, in our study HDRsEf1 could not change the expression of IL-10, PPAR-γ, and TSLP. By contrast, O157:H7 could distinctly increase the mRNA expression of IL-1β, IL-6, IL-8, IL-23, and TNF-α, which agrees with the other study which found that E. coli could induce pro-inflammatory gene expression in intestinal epithelial cells (Ghadimi et al. Citation2012; Taranu et al. Citation2015); however the expression of IL-10, IL-12p35, IL-17, TSLP, and IFN-γ is similar to HDRsEf1 except the expression of PPAR-γ. This demonstrates that IL-10, PPAR-γ, and TSLP are very much stable against foreigners.
In summary, our study showed that HDRsEf1 could regulate the inflammatory response of human enterocyte-like HT-29 cells. HDRsEf1 could active TLR2 pathway in an MyD88-independent pattern; in addition, HDRsEf1 could significantly downregulate the mRNA level of pro-inflammatory factors IL-1β, IL-6, IL-8, IL-12p35, IL-17, and TNF-α, and did not upregulate the degradation of IκB in HT-29 cells. By contrast, O157:H7 could powerfully degrade IκB and upregulate pro-inflammatory cytokines IL-1β, IL-6, IL-8, IL-23, and TNF-ɑ in HT-29 cells. According to our knowledge, this study is the first to report the immunomodulatory effects of E. faecium on human enterocyte-like HT-29 cells.
Disclosure statement
No potential conflict of interest was reported by the authors.
Additional information
Funding
References
- Akira S. 2006. TLR signaling. Curr Top Microbiol Immunol. 311:1–16.
- Akira S, Uematsu S, Takeuchi O. 2006. Pathogen recognition and innate immunity. Cell. 124:783–801. doi: 10.1016/j.cell.2006.02.015
- Arce C, Ramirez-Boo M, Lucena C, Garrido JJ. 2010. Innate immune activation of swine intestinal epithelial cell lines (IPEC-J2 and IPI-2I) in response to LPS from Salmonella typhimurium. Compa Immunol Infect Dis. 33:161–174. doi: 10.1016/j.cimid.2008.08.003
- Are A, Aronsson L, Wang S, Greicius G, Lee YK, Gustafsson J, Pettersson S, Arulampalam V. 2008. Enterococcus faecalis from newborn babies regulate endogenous PPARγ activity and IL-10 levels in colonic epithelial cells. Proc Natl Acad Sci U S A. 105:1943–1948. doi: 10.1073/pnas.0711734105
- Artis D. 2008. Epithelial-cell recognition of commensal bacteria and maintenance of immune homeostasis in the gut. Nat Rev Immunol. 8:411–420. doi: 10.1038/nri2316
- Bron PA, van Baarlen P, Kleerebezem M. 2012. Emerging molecular insights into the interaction between probiotics and the host intestinal mucosa. Nat Rev Microbiol. 10:66–78.
- Buydens P, Debeuckelaere S. 1996. Efficacy of SF 68 in the treatment of acute diarrhea. A placebo-controlled trial. Scand J Gastroenterol. 31:887–891. doi: 10.3109/00365529609051997
- Cammarota M, De Rosa M, Stellavato A, Lamberti M, Marzaioli I, Giuliano M. 2009. In vitro evaluation of Lactobacillus plantarum DSMZ 12028 as a probiotic: emphasis on innate immunity. Int J Microbiol. 135:90–98. doi: 10.1016/j.ijfoodmicro.2009.08.022
- Chai W, Burwinkel M, Wang Z, Palissa C, Esch B, Twardziok S, Rieger J, Wrede P, Schmidt MF. 2013. Antiviral effects of a probiotic Enterococcus faecium strain against transmissible gastroenteritis coronavirus. Arch Virol. 158:799–807. doi: 10.1007/s00705-012-1543-0
- Clavel T, Haller D. 2007. Molecular interactions between bacteria, the epithelium, and the mucosal immune system in the intestinal tract: implications for chronic inflammation. Curr Issues Intest Microbiol. 8:25–43.
- Di Mauro A, Neu J, Riezzo G, Raimondi F, Martinelli D, Francavilla R, Indrio F. 2013. Gastrointestinal function development and microbiota. Ital J Pediatr. 39:15. doi: 10.1186/1824-7288-39-15
- Finamore A, Roselli M, Imbinto A, Seeboth J, Oswald IP, Mengheri E. 2014. Lactobacillus amylovorus inhibits the TLR4 inflammatory signaling triggered by enterotoxigenic Escherichia coli via modulation of the negative regulators and involvement of TLR2 in intestinal Caco-2 cells and pig explants. PLoS One. 9:e94891. doi: 10.1371/journal.pone.0094891
- Fischer J, Suire C, Hale-Donze H. 2008. Toll-like receptor 2 recognition of the microsporidia Encephalitozoon spp. induces nuclear translocation of NF-kappaB and subsequent inflammatory responses. Infect Immun. 76:4737–4744. doi: 10.1128/IAI.00733-08
- Gao Q, Qi L, Wu T, Wang J. 2012. Clostridium butyricum activates TLR2-mediated MyD88-independent signaling pathway in HT-29 cells. Mol Cell Biochem. 361:31–37. doi: 10.1007/s11010-011-1084-y
- Ghadimi D, Helwig U, Schrezenmeir J, Heller KJ, de Vrese M. 2012. Epigenetic imprinting by commensal probiotics inhibits the IL-23/IL-17 axis in an in vitro model of the intestinal mucosal immune system. J Leukoc Biol. 92:895–911. doi: 10.1189/jlb.0611286
- Gong Q, Zeng JJ, Shi DS, Wang XL, Wang T, Xiao HD, Xiao YC. 2015. Effects of Enterococcus faecium HDRsEf1 on development of cecal bacterial flora in broilers. J Huazhong Agri Univ. 01: 78–82.
- Haddad JJ, Abdel-Karim NE. 2011. NF-kappaB cellular and molecular regulatory mechanisms and pathways: therapeutic pattern or pseudoregulation? Cell Immunol. 271:5–14. doi: 10.1016/j.cellimm.2011.06.021
- Kajikawa A, Nordone SK, Zhang L, Stoeker LL, LaVoy AS, Klaenhammer TR, Dean GA. 2011. Dissimilar properties of two recombinant Lactobacillus acidophilus strains displaying Salmonella FliC with different anchoring motifs. Appl Environ Microbiol. 77:6587–6596. doi: 10.1128/AEM.05153-11
- Kanamaru K, Kanamaru K, Tatsuno I, Tobe T, Sasakawa C. 2000. SdiA, an Escherichia coli homologue of quorum-sensing regulators, controls the expression of virulence factors in enterohaemorrhagic Escherichia coli O157:H7. Mol Microbiol. 38:805–816. doi: 10.1046/j.1365-2958.2000.02171.x
- Kawai T, Akira S. 2009. The roles of TLRs, RLRs and NLRs in pathogen recognition. Int Immunol. 21:317–337. doi: 10.1093/intimm/dxp017
- Kawai T, Akira S. 2011. Toll-like receptors and their crosstalk with other innate receptors in infection and immunity. Immunity. 34:637–650. doi: 10.1016/j.immuni.2011.05.006
- Lavelle EC, Murphy C, O’Neill LA, Creagh EM. 2010. The role of TLRs, NLRs, and RLRs in mucosal innate immunity and homeostasis. Mucosal Immunol. 3:17–28. doi: 10.1038/mi.2009.124
- Mazmanian SK, Round JL, Kasper DL. 2008. A microbial symbiosis factor prevents intestinal inflammatory disease. Nature. 453:620–625. doi: 10.1038/nature07008
- Medvedev AE, Kopydlowski KM, Vogel SN. 2000. Inhibition of lipopolysaccharide-induced signal transduction in endotoxin-tolerized mouse macrophages: dysregulation of cytokine, chemokine, and toll-like receptor 2 and 4 gene expression. J Immunol. 164:5564–5574. doi: 10.4049/jimmunol.164.11.5564
- Meshitsuka S, Ishizawa M, Nose T. 1987. Uptake and toxic effects of heavy metal ions: interactions among cadmium, copper and zinc in cultured cells. Experientia. 43:151–156. doi: 10.1007/BF01942833
- Metchnikoff II. 1987. The prolongation of life: optimistic studies. London: William Heinemann.
- de Moreno DLA, Chaves S, Carmuega E, Weill R, Antoine J, Perdigon G. 2008. Effect of long-term continuous consumption of fermented milk containing probiotic bacteria on mucosal immunity and the activity of peritoneal macrophages. Immunobiology. 213:97–108. doi: 10.1016/j.imbio.2007.07.002
- O’Neill LA, Bowie AG. 2007. The family of five: TIR-domain-containing adaptors in Toll-like receptor signalling. Nat Rev Immunol. 7:353–364. doi: 10.1038/nri2079
- O’Neill LA, Bryant CE, Doyle SL. 2009. Therapeutic targeting of Toll-like receptors for infectious and inflammatory diseases and cancer. Pha Revi. 61:177–197. doi: 10.1124/pr.109.001073
- Parvez S, Malik KA, Ah KS, Kim HY. 2006. Probiotics and their fermented food products are beneficial for health. J Appl Microbiol. 100:1171–1185. doi: 10.1111/j.1365-2672.2006.02963.x
- Rimoldi M, Chieppa M, Salucci V, Avogadri F, Sonzogni A, Sampietro GM, Nespoli A, Viale G, Allavena P, Rescigno M. 2005. Intestinal immune homeostasis is regulated by the crosstalk between epithelial cells and dendritic cells. Nat Immunol. 6:507–514. doi: 10.1038/ni1192
- Roger T, Casson N, Croxatto A, Entenza JM, Pusztaszeri M, Akira S, Reymond MK, Le Roy D, Calandra T, Greub G. 2010. Role of MyD88 and Toll-like receptors 2 and 4 in the sensing of Parachlamydia acanthamoebae. Infect Immun. 78:5195–5201. doi: 10.1128/IAI.00786-10
- Sherman PM, Ossa JC, Johnson-Henry K. 2009. Unraveling mechanisms of action of probiotics. N Cli Pr. 24:10–14. doi: 10.1177/0884533608329231
- Shi DS, Xiao YC, Bi DR, Xiong YY, Wang XL, Gao XC, Xu GS, Li ZL, Zhou ZT, Liu M et al. 2013. Date of authorization proclamation: 2013-11-13. A beneficial enterococcus strains screening and application. China National invention patent: ZL201110452087.2.
- Takeuchi O, Sato S, Horiuchi T, Hoshino K, Takeda K, Dong Z, Modlin RL, Akira S. 2002. Cutting edge: role of Toll-like receptor 1 in mediating immune response to microbial lipoproteins. J Immunol. 169:10–14. doi: 10.4049/jimmunol.169.1.10
- Taranu I, Marin DE, Pistol GC, Motiu M, Pelinescu D. 2015. Induction of pro-inflammatory gene expression by Escherichia coli and mycotoxin zearalenone contamination and protection by a Lactobacillus mixture in porcine IPEC-1 cells. Toxicon. 97:53–63. doi: 10.1016/j.toxicon.2015.01.016
- Tojo R, Suarez A, Clemente MG, de Los RC, Margolles A, Gueimonde M, Ruas-Madiedo P. 2014. Intestinal microbiota in health and disease: role of bifidobacteria in gut homeostasis. World J Gastroenterol. 20:15163–15176. doi: 10.3748/wjg.v20.i41.15163
- Villena J, Kitazawa H. 2013. Modulation of intestinal TLR4-inflammatory signaling pathways by probiotic microorganisms: lessons learned from Lactobacillus jensenii TL2937. Front Immunol. 4, 1–12.
- Wang JB, Qi LL, Zheng SD, Wang HZ, Wu TX. 2009. Curcumin suppresses PPARdelta expression and related genes in HT-29 cells. World J Gastroenterol. 15:1346–1352. doi: 10.3748/wjg.15.1346
- Wang S, Hibberd ML, Pettersson S, Lee YK. 2014. Enterococcus faecalis from healthy infants modulates inflammation through MAPK signaling pathways. PLoS One. 9:e97523. doi: 10.1371/journal.pone.0097523
- Wermuth PJ, Jimenez SA. 2012. Gadolinium compounds signaling through TLR4 and TLR7 in normal human macrophages: establishment of a proinflammatory phenotype and implications for the pathogenesis of nephrogenic systemic fibrosis. J Immunol. 189:318–327. doi: 10.4049/jimmunol.1103099
- Wunderlich PF, Braun L, Fumagalli I, D’Apuzzo V, Heim F, Karly M, Lodi R, Politta G, Vonbank F, Zeltner L. 1989. Double-blind report on the efficacy of lactic acid-producing Enterococcus SF68 in the prevention of antibiotic-associated diarrhoea and in the treatment of acute diarrhoea. J Int Med Res. 17:333–338.
- Xiao HD, Xiao YC, He XZ, Xu W, Wang XL, Bi DR, Shi DS. 2014. Study on Probiotic Agents Substituting for Antibiotics in Weaned Piglet Diets. Progress Veterinary Med. 35:53–58.
- Xiong YY. 2010. Identification and Screening of Enterococcus Probiotics from Swine Feces [master]: Huazhong Agri Univ.
- Zeytun A, Chaudhary A, Pardington P, Cary R, Gupta G. 2010. Induction of cytokines and chemokines by Toll-like receptor signaling: strategies for control of inflammation. Crit Rev Immunol. 30:53–67. doi: 10.1615/CritRevImmunol.v30.i1.40
- Zoumpopoulou G, Foligne B, Christodoulou K, Grangette C, Pot B, Tsakalidou E. 2008. Lactobacillus fermentum ACA-DC 179 displays probiotic potential in vitro and protects against trinitrobenzene sulfonic acid (TNBS)-induced colitis and Salmonella infection in murine models. Int J Microbiol. 121:18–26. doi: 10.1016/j.ijfoodmicro.2007.10.013